- 1MOA Laboratory for Risk Assessment of Quality and Safety of Livestock and Poultry Products, Huazhong Agricultural University, Wuhan, China
- 2National Reference Laboratory of Veterinary Drug Residues (HZAU) and MAO Key Laboratory for Detection of Veterinary Drug Residues, Wuhan, China
- 3Department of Biosciences, COMSATS Institute of Information Technology, Sahiwal, Pakistan
- 4Hubei Collaborative Innovation Center for Animal Nutrition and Feed Safety, Wuhan, China
Genotoxicity and carcinogenicity testing of pharmaceuticals prior to commercialization is requested by regulatory agencies. The bacterial mutagenicity test was considered having the highest accuracy of carcinogenic prediction. However, some evidences suggest that it always results in false-positive responses when the bacterial mutagenicity test is used to predict carcinogenicity. Along with major changes made to the International Committee on Harmonization guidance on genotoxicity testing [S2 (R1)], the old data (especially the cytotgenetic data) may not meet current guidelines. This review provides a compendium of retrievable results of genotoxicity and animal carcinogenicity of 136 antiparasitics. Neither genotoxicity nor carcinogenicity data is available for 84 (61.8%), while 52 (38.2%) have been evaluated in at least one genotoxicity or carcinogenicity study, and only 20 (14.7%) in both genotoxicity and carcinogenicity studies. Among 33 antiparasitics with at least one old result in in vitro genotoxicity, 15 (45.5%) are in agreement with the current ICH S2 (R1) guidance for data acceptance. Compared with other genotoxicity assays, the DNA lesions can significantly increase the accuracy of prediction of carcinogenicity. Together, a combination of DNA lesion and bacterial tests is a more accurate way to predict carcinogenicity.
Introduction
Antiparasitics are used widely throughout the world in humans and animals to kill or eliminate parasites in vivo and in vitro, and in public health to control diseases and prevent the spread of parasitism from livestock to humans. According to the pharmacological effects and the target parasite species, antiparasitics can be divided into three main groups: anthelmintics, antiprotozoal agents, and insecticides. Chemically based treatment remains the most frequently chosen tool to control parasitism. Unfortunately, the use of antiparasitics does not always result in the expected therapeutic success. The toxic effects were found to be responsible for the therapeutic failure of drug treatment (1). In the 1970s of the last century, it was reported that the chemicals had the capacity to cause cancer in both animals and humans (2, 3). Genetic and carcinogenic damage was found to have important health implications for the induction of diseases, such as lung cancer (4), pancreatic cancer (5), bladder cancer (6), leukemia (7–9), and non-Hodgkin’s lymphoma (10). Therefore, the regulatory agencies of Europe, the USA and Japan suggested that genotoxicity and carcinogenicity studies should be conducted to learn the benefit/risk ratio before commercial approval of pharmaceuticals.
It was recommended by the regulatory agencies that genotoxicity testing, which was considered to be a fundamental part of the carcinogenic risk assessment, should be performed prior to commercialization. It was forbidden to use compounds with proven genotoxic properties on humans except in rare cases with adequate justifications (11). According to the present guidelines for genotoxicity testing of pharmaceuticals (12–15), a standard test battery contains: (a) a test for gene mutations in bacteria, (b) an in vitro test with cytogenetic evaluation of chromosomal damage using mammalian cells or an in vitro mouse lymphoma thymidine kinase± gene mutation assay, and (c) an in vivo test for chromosomal damage using mammalian hematopoietic cells. These assays were considered the best approach for genotoxic hazard identification and potential carcinogenic risk prediction. However, some limitations of this standard test battery in detecting genotoxicity were found. The current revised guidelines of the Veterinary International Conference on Harmonization and ICH S2 (R1) suggested that it can detect the genetic toxicity of most substances. However, for some special chemicals such as antimicrobial, it was required to supply the bacterial assay with a validated in vitro test for gene mutation in mammalian cells to detect the genetic toxicity (12, 15).
How can we identify and analyze positive genotoxicity results, especially in vitro cytogenetics? Two main factors including cytotoxicity and the highest testing concentration of the tested chemicals have very important effects on the result of genotoxicity. The Organization for Economic Cooperation and Development (OECD) had changed over the years to find the most suitable toxicity required at the highest concentration. In the 1999 revision, it was recommended that at least 50% toxicity should be induced. The ICH S2B suggested that in vitro genotoxicity tests should be conducted up to a top concentration of 10 mM in 1997 (16). In fact, when the dose level exceeds 100 µM, the physiological biological reactions will be disorder and then result in positive findings in in vitro genotoxicity tests. Moreover, a study sponsored by the European Center for the Validation of Alternative Methods indicated that the high testing dose should be reduced because the false-positive results in in vitro genotoxicity occurred at concentration levels from 1 to 10 mM. Recently, the ICH updated the genotoxicity guidelines (Table 1) (11, 17). It reduced the highest dose to 1 mM and supported the in vivo genotoxicity assays.
Antiparasitics were used in the market for many years, and for a large proportion of them, genotoxicity and carcinotoxicity assays were performed prior to 1980, when the bioassays were not concordant with the present guidelines. Thus, it is necessary to re-evaluate the old data (especially the cytogenetic data) under the current guidelines of ICH S2 (R1) (17).
For pharmaceuticals, whose clinical use is continuous for at least 6 months or intermittent in chronic recurrent conditions, the long-term carcinogenicity studies in rats and mice using lifetime treatment are required (19). This has remained the most frequently chosen testing strategy since proposed by regulatory authorities in 1970s. The objective of carcinogenicity studies is to discover whether a drug has the ability to cause carcinogenicity in animals and whether this tumorigenic potential poses a relevant risk to humans (19, 20). To make an evaluation of carcinogenic risks to humans, the International Agency for Research on Cancer (IARC) in the 1–101 volumes of IARC monographs was published in the years from 1972 to 2011 (21). It examined 940 drugs in various groups: the carcinogenicity studies were sufficient for 107 drugs (11.4%), limited for 59 drugs (6.3%), and inadequate for 266 drugs (28.3%); and the remaining 508 drugs (54.0%) were not classifiable in terms of their carcinogenicity to humans. However, it included only 10 antiparasitics: 2 antiparasitics (Metronidazole and Dichlorvos) were classified as possibly carcinogenic to humans (Group 2B), and 8 antiparasitics (Chloroquine, Chlordimeform, Danex, Deltamethrin, Fenvalerate, Malathion, Permethrin, and Pyrimethamine) were considered non-classifiable in terms of their carcinogenicity to humans (Group 3).
Based on the above mentioned, it is meaningful to verify the extent of antiparasitics having the available results of genotoxicity and carcinogenicity studies. It is also necessary to re-evaluate in vitro genotoxicity results according to the present revised guidance. Due to the bacterial mutagenicity test alone produced misleading positive in predicting the carcinogens, we compared the combinations of bacterial mutagenicity test and other genotoxicity assays (such as cytogeneticity in vivo and in vitro, DNA lesions and mouse bone marrow micronucleus), aiming to work out a novel strategy to predict carcinogenicity.
The 136 antiparasitics that are listed in both the human andveterinary pharmacopeia were authorized by China. Forty-three and 107 antiparasitics were obtained from the human pharmacopeia and veterinary pharmacopeia, respectively. Since some parasites, including helminths, schistosome, and tapeworm, can infect both humans and animals, simultaneously, 14 antiparasitics (Albendazole, Amoscanate, Artesunate, Bithionol, Diethylcarbamazine, Ivermectin, Levamisole, Piperazine, Pyramine, Praziquantel, Mebendazole, Metronidazole, Niclosamide, and Semduramicin Soditium) can be used on both humans and animals.
The methodology of the major carcinogenicity and genotoxicity tests were summarized in Table 2. The collected information of genotoxicity and/or carcinogenicity of antiparasitics was obtained primarily from peer-reviewed journals (e.g., Medline, Toxline, and the Registry of Toxic Effects of Chemical Substances) (22), the US National Toxicology Program, the edition of Physician’s Desk Reference (23–25), the Center for Drug Evaluation and Research of the Food and Drug Administration and some relevant websites, such as http://www.updata.usa.com, http://www.osha.gov, http://www.toxnet.nlm.nih.gov, http://www.ntp.server.niehs.nih.gov, http://www.potency.berkeley.edu, http://www.fda.gov/cder, http://www.scirus.com, and http://www.inchem.org. For some antiparasitics, the genotoxicity and carcinogenicity data are incomplete in terms of the absence of the dose, the indication of an exogenous metabolic system in the genotoxicity assays, and the sex in carcinogenicity assays. In such cases, we presented our data in tables as obtained in these experimental conditions except for special markings. Moreover, regarding the present guidelines, the equivocal results that we found in extensive research were marked as positive in this review.
Results
Genotoxicity and Carcinogenicity of Antiparatics
For the present analyses, an antiparasitic was regarded as genotoxic when it produced positive or equivocal results in at least one of the standard battery tests, and as a rodent carcinogen when it increased tumor incidence. Table 3 covers the information available on genotoxicity and carcinogenicity findings for each tested antiparasitic. The following genotoxicity assays were used: Ames (bacterial mutagenesis), sex-linked recessive lethal, in vitro cytogenetics (chromosome aberrations), in vivo cytogenetics [chromosome aberrations, micronucleus and sister chromatid exchange (SCE)], unscheduled DNA synthesis in vitro (UDS), MLA (mouselymphoma L5178Y TK± assay), and other types of genotoxicity studies, including DNA fragmentation, mammalian mutagenesis HGPRT, SCE in vitro, DNA strand break analysis in vitro, and the micronucleus assay in vitro. The long-term carcinogenicity test was carried out in mice, rats, and other species.
Table 4 summarizes the total number of antiparasitics and the following are included: the number of antiparasitics with at least one genotoxicity or carcinogenicity test result and with data required by the present guidelines; the number of antiparasitics only tested for genotoxicity or carcinogenicity. It also presents the antiparasitics with results in in vitro data required by present guidelines; the number of antiparasitics that have at least one result in long-term carcinogenesis assays in rats or mice; and the number of antiparasitics in genotoxicity assays (bacterial mutagenicity, in vitro tests for gene mutation and for chromosomal damage, in vivo cytogenetic tests, and other types of genotoxicity assays). Of 136 antiparasitics examined, 52 (38.2%) had at least one genotoxicity or carcinogenicity test result, and 32 (23.5%) were tested only for either genotoxicity or carcinogenicity. Among 20 antiparasitics with results available for both genotoxicity and carcinogenicity, 16 had all the results required by the present guidelines for testing of pharmaceuticals: 8 of them—Albendazole, Coumaphos, Cypermethrin, Deltamethrin, Diazinon, Fenvalerate, Malathion and Tiabendazole—tested positive in genotoxicity assays but gave at least one negative result in carcinogenesis assays; 8 antiparasitics (Chlordimeform, Dichlorvos, Fenthion, Fipronil, Lindane, Metronidazole, Pyrimethamine, and Imidacloprid) gave positive responses in both genotoxicity and carcinogenicity. The remaining four with both genotoxicity and carcinogenicity data were not in agreement with the current guidelines: Amitraz and Praziquantel gave positive responses in genotoxicity but were non-carcinogenic; Atovaquone tested negative in genotoxicity but positive in mouse carcinogenicity; and Mefloquine produced negative responses in both genotoxicity and carcinogenicity.
Additional 32 antiparasitics were only tested in either genotoxicity or carcinogenicity. Only one (Ivermectin) had retrievable results in carcinogenicity. As for the rest, 31 antiparasitics had the data of genotoxicity. Twenty-one antiparasitics (Acriflavine, Closantel, Chloroquine, Cyfluthrin, Danex, Diaveridine, Dimetridazole, Fenbendazole, Fenchlorphos, Furapyrimidone, Furapromide, Mebendazole, Nitroscanate, Nitroxinil, Niclosamide, Oxfendazole, RH-5849, Tetramethrin, Thiophanate, Tinidazole, and Triclabendazole) gave positive responses in at least one genotoxicity assay; 10 antiparasitics (Amodiaquine, Amoscanate, Amphotericin B, Bithionol, Bromofenofos, Flubendazole, Pentamidine, Permethrin, Piperazine, and Quinine) were found to be negative in all the considered genotoxicity assays. With regard to the different types of genotoxicity assays: there were 47 antiparasitics with at least one result in tests for bacterial mutagenicity; 18 antiparasitics with at least one result in tests for gene mutation in mammalian cells; 33 antiparasitics in in vitro tests for SCE, chromosomal aberrations, aneuploidy, or micronucleus in animal or human cells; 15 antiparasitics with results in in vitro data required by present guidelines; 31 antiparasitics in in vivo tests for SCE, chromosomal aberrations, or micronucleus in animal or human cells; 19 antiparasitics in DNA damage or DNA repair synthesis; 17 antiparasitics in other types of genotoxicity assays; and 20 antiparasitics examined for genotoxicity in human cells. With respect to carcinogenesis assays, 19 and 20 antiparasitics were tested for carcinogenicity in mice and rats, respectively. Among the antiparasitics with both the genotoxicity and carcinogenicity data, 19 antiparasitics tested for carcinogenicity in both mice and rats and only 1 in hamsters.
Table 5 provides the number of antiparasitics tested for each type of assay, including the genotoxicity and carcinogenicity studies. The results are indicated as positive, negative and discordant. When carcinogenicity testing is considered, 57.9% of antiparasitics were tested negative in mice, and 73.7% in rats. Five antiparasitics (nos. 7, 10, 26, 32, and 36) and three antiparasitics (nos. 10, 26, and 36) were carcinogenic in mice and rats, respectively. The percentage of concordant results in carcinogenicity assays between mice and rats is 85.7% (12 out of 14) and only 2 (nos. 7 and 32) antiparasitics have discordant results: no. 32 tested positive in mice and negative in rats, while no. 7 produced the opposite result. The occurrence of discordant results between mice and rats may be the differences in species (e.g., metabolic enzymes). Ten antiparasitics were in IARC of 2B and 3 ground classifications of carcinogens: Chloroquine, Danex, and Permethrin do not have available carcinogenicity data; Deltamethrin, Fenvalerate, and Malathion tested negative in rodents while positive results were given by Chlordimeform and Metronidazole. Dichlorvos (DDVP) and Pyrimethamine have discordant results of carcinogenicity in mice and rats. To interpret the tumor findings in a carcinogenicity study and provide a perspective on the relevance of rodents to human, the mechanism and some investigations in tumor profile (trans-species, trans-sex, and multisite versus single species, single sex, and single site) were suggested by the guidelines (15).
Re-Evaluation of In Vitro Genotoxicity Results
Table 6 presents the incidence of misleading positive effects in in vitro cytogenicity when using the reduction in a top dose of 1 mM. Of 33 antiparasitics with at least one result in in vitro tests for SCE, chromosomal aberrations, or micronucleus in animal or human cells, 25 (75.8%) antiparasitics had at least one retrievable dose in in vitro cytogenicity assays, while 8 (24.2%) antiparasitics had no available dose. Under the current in vitro genotoxicity testing guidelines for dose limits, 10 (nos. 10, 14, 15, 16, 20, 21, 22, 32, 36, and 47) antiparasitics were identified as genotoxins at dose levels more than 1 mM. The re-evaluation results indicated the misleading positive response in the previous reports. Fifteen (nos. 1, 2, 3, 13, 17, 18, 19, 24, 25, 26, 28, 30, 42, 50, and 52) antiparasitics had in vitro genotoxicity results consistent with ICH S2 (R1).
Correlation between the Genotoxicity Assays
Table 7 provides the correlation among the different types of genotoxicity assays of antiparasitics, the numbers and percentages of antiparasitics that tested concordant and discordant between each other. On the whole, the degree of coincident correlation was higher than the discordant results, which ranged from 84.6% between bacterial mutagenicity and gene mutation in mammalian cells to 55.6% between gene mutation in mammalian cells and in vivo cytogenetics. When bacterial mutagenicity was compared with the following assays: gene mutation in mammalian cells, in vitro cytogenetics, in vivo cytogenetics and DNA lesions, 13 (nos. 3, 14, 15, 17, 19, 22, 24, 25, 32, 33, 45, 47, and 49) antiparasitics gave negative results in bacterial mutagenicity. Among these antiparasitics, there were 2 (nos. 32 and 45), 8 (nos. 15, 22, 24, 25, 32, 33, 47, and 49), 7 (nos. 14, 15, 17, 19, 24, 25, and 32) and 5 (nos. 3, 14, 32, 45, and 47) antiparasitics that tested positive in gene mutation in mammalian cells, in vitro cytogenetics, in vivo cytogenetics and DNA lesions, respectively.
The highly consistent correlation between bacterial mutagenicity and gene mutation in mammalian cells indicated that the same genetic end point tests might have the high consistency. The discordance (nos. 32 and 45) may be due to the xenobiotic metabolism in the liver and other organs between the bacteria and animals. With the comparison between in vitro cytogenetics and in vivo cytogenetics, 2 (nos. 18 and 49) antiparasitics gave positive responses in in vitro cytogenetics while no. 13 gave negative. These results were inconsistent with that in in vivo cytogenetics. With regard to the discordant results between DNA lesions and in vitro cytogenetics of the three (nos. 3, 19 and 33) antiparasitics, two (nos. 19 and 33) antiparasitics tested negative and no. 3 yield positive in DNA lesions, respectively. These results were opposite to that in in vitro cytogenetics.
A Novel Strategy for Predicting Carcinogenicity Based on the Genotoxicity Assays
Antiparasitics with both genotoxicity and carcinogenicity data are reported in Table 8 to analyze the correlation between the results of the various types of genotoxicity and carcinogenicity. The results are marked positive or negative or inconclusive. It is obvious that the concordant and discordant results occurred in all the 15 pairs of assays considered. When carcinogenicity in mice or rats was considered, the percentage of discordant results ranged from 71.4% between in vivo cytogenetics and carcinogenicity in both mice and rats to 10.0% between bacterial mutagenicity and carcinogenicity in both mice and rats. The rank order of the consistency between genotoxicity and carcinogenicity was bacterial mutagenicity > DNA lesions > in vitro cytogenetics > gene mutation in mammalian cells > in vivo cytogenetics.
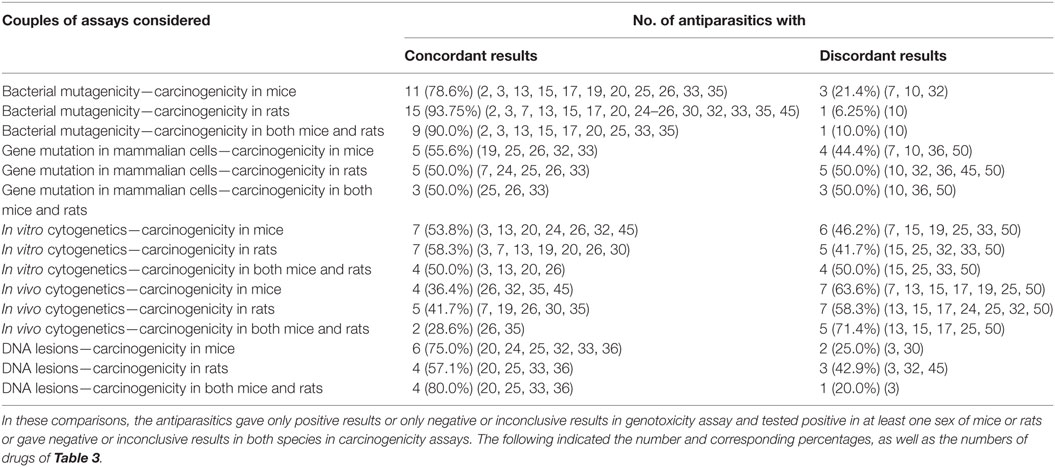
Table 8. Correlation between the multiple genotoxicity and carcinogenicity in mice and rats assays of antiparasitics.
Table 9 showed 2 types and 10 combinations of gene-tox assays based on bacterial mutagenicity to indicate the predictivity for rodent carcinogenicity. The sequence of the predictivity was (Ames–DNA lesions) = (Ames–DNA lesions–in vitro) = (Ames–DNA lesions–gene mutation in mammalian cells) = (Ames–In vivo–DNA) > (Ames–in vitro) = (Ames–in vivo) > (Ames–gene mutation in mammalian cells) > (Ames–in vivo–in vitro) > (Ames–gene mutation in mammalian cells–in vivo) = (Ames–gene mutation in mammalian cells–in vitro).
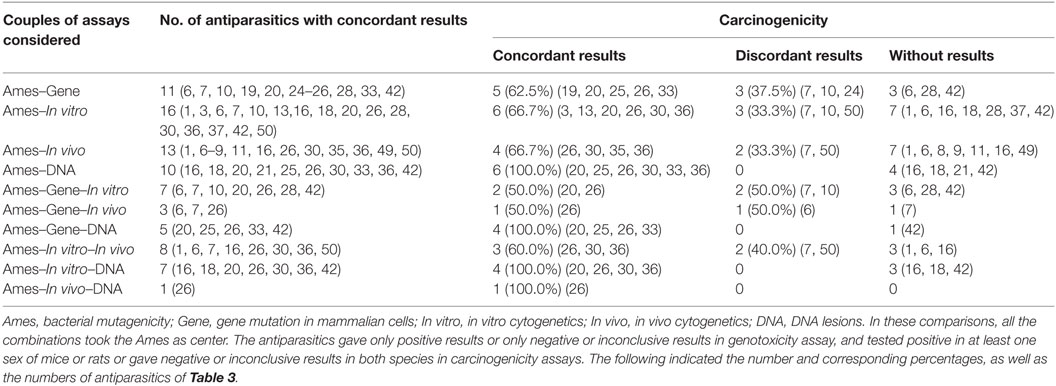
Table 9. Predictivity of multiple combinations with Ames for rodent carcinogenicity assays of antiparasitics.
Table 10 presents the number and the percentage of antiparasitics that were classified as non-genotoxic non-carcinogens, genotoxic non-carcinogens, non-genotoxic carcinogens, and genotoxic carcinogens according to the genotoxicity assays considered. An antiparasitic was regarded as genotoxic when a positive response was given in at least one genotoxicity assay, and carcinogenic when it was tested positive in at least one rodent sex. Of the 20 antiparasitics with retrievable results of both genotoxicity and carcinogenicity, Malathion, Diazinon, Deltamethrin, Fenvalerate, Coumaphos, Tiabendazole, Albendazole, Cypermethrin, Amitraz and Praziquantel might be classified as genotoxic non-carcinogens; Fenthion, Lindane, Chlordimeform, Fipronil, Dichlorvos, Metronidazole, Pyrimethamine, and Imidacloprid can be classified as genotoxic carcinogens; Mefloquine was considered a non-genotoxic non-carcinogen, while the non-genotoxic carcinogens only contained Atovaquone, which tested negative in bacterial mutagenicity, in vitro and in vivo cytogenetic assays, but was found to induce liver tumors in mice in a long-term carcinogenesis assay (75, 122, 123).
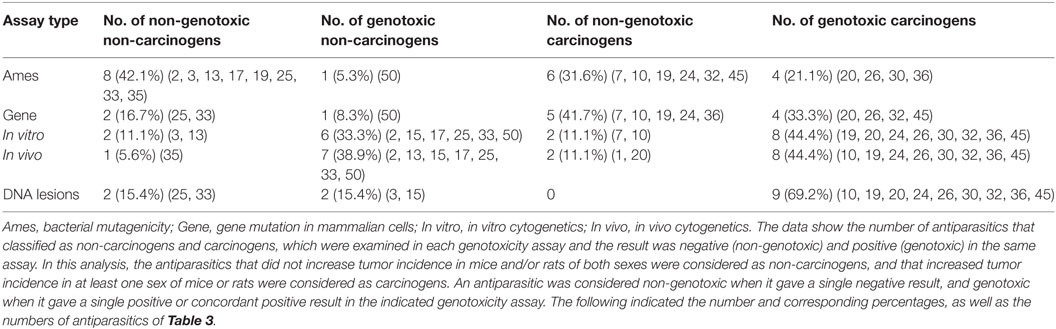
Table 10. Correlation between the results of genotoxicity and carcinogenicity assays of antiparasitics.
The bacterial mutagenicity has the highest specificity but the lowest sensitivity (Table 8), while DNA lesions (in vitro and/or in vivo) have the highest sensitivity and a lower specificity. A test with a low specificity induced a high proportion of misleading positive results. Therefore, the combination of bacterial mutagenicity and DNA lesions has high accuracy in relation to rodent cancer, which is consistent with the above analysis results. A proportion of 5.3% of antiparasitics gave positive in bacterial mutagenicity and was classified as non-carcinogens. There were 31.6% of antiparasitics that were regarded as carcinogenic while gave a negative result in bacterial mutagenicity.
Discussion
The economic importance of parasitic infections in livestock and humans has long been recognized. Meanwhile, the most important advances in antiparasitics have come from the animal health area. Although many antiparasitics have been developed and applied to control parasitism in humans and animals, genotoxicity and carcinogenicity studies have not been conducted on a large proportion of them. Since a relationship between exposure to genotoxic compounds and carcinogenesis has been established, genotoxicity tests have been proposed for all medicinal products for human use except for some compounds (e.g., anticancer) that can interact with DNA (11). Therefore, this review was to assess the extent of antiparasitics that have been tested for genotoxic and carcinogenic activity. In addition, the ability of various types of genotoxicity assays was summarized to discriminate rodent carcinogens, which benefit to analyze the relative predictivity of carcinogenicity in rodents and humans. Furthermore, it is necessary to re-evaluate in vitro genotoxicity according the present revised guidelines.
With regard to the genotoxicity assays, compared to the positive and discordant results, the incidence of negative responses is 61.7, 61.1, 21.2, 25.8, and 15.8% for bacterial mutagenicity, gene mutation in cultured mammalian cells, in vitro cytogenetics, in vivo cytogenetics, and DNA lesions (in vitro and in vivo), respectively. It was observed that the incidence of negative responses was higher than the positive and discordant results in bacterial mutagenicity and gene mutation in cultured mammalian cells. Kasper et al. (240) reviewed the advantages and limitations of the standard genotoxicity tests in predicting the ability and the mode of action for carcinogens, which demonstrated that a totally negative response in all the standard genotoxicity assays was sufficient to prove the non-genetic toxicity of the chemicals, while the presence of a positive response in some genotoxicity assays, particularly in Ames and in vitro genotoxicity studies, did not afford support for the genetic definition of the chemicals. There have been a number of experiences in the literature regarding the high correlation among the various types of genotoxicity assays with respect to carcinogens (241, 242), which suggested that a chemical that tested positive in Salmonella tended to yield positive responses in any other in vitro genotoxicity studies, for instance, chromosome aberrations (CA), SCEs, and mutations in mouse lymphoma cells (MLA) (243).
A high percentage of antiparasitics tested positive in the following assays: in vitro cytogenetics, in vivo cytogenetics, and DNA lesions (in vitro and in vivo). It is worth noting that the proportion of positive responses in in vitro cytogenetics is higher than in other types of assays. The in vitro cytogenetics seems to be more sensitive to genetic substance. However, the in vitro assays always lead to a number of false-positive results in genotoxicity and the carcinogenicity in rodents (244, 245). It was learned from the literature that the massive positive results only occurred at high levels of concentration. Recent surveys for in vitro cytogenetics were taken from compilations such as that of Müller et al. (246), Kirkland and Müller (247), Müller and Kasper (248), and Hilliard et al. (249). The conclusion was that the highest testing concentrations might lead to an increase in the emergence of misleading, toxicity-related positive results. In cytotoxicity and chromosome aberrations in vitro, Galloway (250) found that the positive response in genetic toxicology was caused by the cytotoxicity rather than the true drug or DNA interactions. Parry et al. (251) examined 24 carcinogens that gave positive results in in vitro genotoxicity at 1–10 mM, yet almost half of them were not mechanistically genotoxic carcinogens or had carcinogenic effects only in excessive doses. In the present review, we re-evaluate the in vitro genotoxicty according to current ICH S2 (R1) guidance. We find that the percentage of antiparasitics in agreement with the current ICH S2 (R1) guidance for in vitro genotoxicity data acceptance was 15 (45.5%). Thus, it is essential to re-evaluate in vitro genotoxicty that conducted prior to the update guideline of ICH S2 (R1) to provide a comprehensive assessment of the genotoxic effects.
Misleading positive results were found not only in in vitro but also in in vivo genotoxic assays. Increasing experience suggested that the occurrence of a positive response in rats and mice micronucleus tests was not the consequence of intrinsic genotoxicity but drug-related disturbances in the physiology (252), such as lysosomal damage, ATP depletion or impairment of mitochondrial function and the release of DNA endonucleases. However, at the time of writing, there has still been no amendment to the guidelines requirements of in vivo genotoxicity for dose limitations and toxicity to avoid irrelevant physiological responses. Furthermore, there is no consensus as to the highest testing concentration in in vitro genotoxicity assays. The method for the detection of toxicity has greatly changed in recent years, and the limitations of dose and toxicity in genotoxicity testing in OECD and ICH should be adjusted to adapt to the new changes. The standard genotoxicity system also needs to identify the cytotoxicity and genotoxicity clearly.
There are many explanations that could account for the existence of different results in the various types of genetic tests. The differences are the following: the detection of the genetic end point; the xenobiotic metabolism between bacterial mutagenicity and mammalian cells; the effective dose between in vitro and in vivo, especially the in vivo decomposition; the relative sensitivities of various genotoxicity assays to genetic damage; the metabolic activation pathway and metabolizing enzymes among species. In vivo activity, which is designed to study the mechanisms of mutagenicity in the potential target organs of rodents, is the best method to confirm the differences in cytogenetics between in vivo and in vitro. Except for the irrelevant biological reaction at high doses, it is also accepted that the metabolic activation process and metabolites could induce genetic toxicity. Some evidence suggested that the genetic toxicity of compounds may be prototypes or metabolites. For the drugs that are theoretically nitrosatable in the presence of amine, the interaction resulted in the formation of genotoxic–carcinogenic N-nitroso compounds (253). However, the current standard of genotoxicity assays cannot distinguish whether the positive results are derived from the drugs or their metabolites directly.
In Table 7, the percentage of concordant results between bacterial mutagenicity and carcinogenicity in both mice and rats is 90.0%, which is higher than any other correlation pairs. The same conclusion was drawn by Snyder and Green (19) in a review of the genotoxicity of marketed pharmaceuticals. Data from 467 marketed drugs were collected and no combination of gene-tox assays provided a higher predictivity of rodent carcinogenesis than the bacterial mutagenicity test itself (19). In two studies conducted by Zeiger, one identified 172 chemicals that gave negative or equivocal results in 2-year rodent assays, yet 38 (22.1%) chemicals produced positive results in Salmonella (243). Another found that among 158 drugs that tested negative in carcinogenicity assays, 33 (21%) were Salmonella mutagens (254). However, a chemical that tested negative in Salmonella testing cannot be regarded as a non-carcinogenicity because the percentage of rodent carcinogens that are not mutagenic is about 50% (254). It was also reported that the predictivity for rodent carcinogenicity of bacterial mutagenicity ranged from approximately 77 to 98% (254, 255). The remaining 2–23% was classified as non-carcinogen with positive result in bacterial mutagenicity, which demonstrated the flaw and insufficiency on the prediction carcinogenicity of bacterial mutagenicity.
Therefore, it requires efforts to overcome the deficiencies of bacterial mutagenicity and improve the predictivity for carcinogenicity. We try to find which genotoxicity assay(s) considered could enhance the prediction of bacterial mutagenicity to rodent carcinogenicity. Our approach has many differences and improvement compared to Snyder and Green (19), who examined only five combinations of gene-tox assays, such as Ames–in vitro cytogenetics, Ames–in vivo cytogenetics, In vitro cytogenetics–in vivo cytogenetics, MLA–in vivo cytogenetics, and MLA–in vitro cytogenetics (19). These combinations have no DNA lesions tests and no taking bacterial mutagenicity as center. A review suggested that DNA lesion alone could contribute to the prediction of carcinogenicity in mice (255). In the present article, as shown in Table 8, DNA lesion testing can significantly increase the predictivity of Ames from 90 to 100%, suggesting that the combination of DNA lesions and bacterial mutagenicity obtained higher prediction of carcinogenicity.
There are three types of DNA lesions: (a) the formation of DNA adducts; (b) DNA repair synthesis (UDS); and (c) the induction of DNA strand breaks and cross-links. An analysis of correlations between the induction of DNA lesions and carcinogenic activity was conducted in 2010 (256). It noted that the carcinogenic activity of some drugs can be correctly predicted by DNA lesion assays, yet neglected in the standard 3-test battery. Thus, DNA lesion assays were considered the best supplement for the standard 3-test battery. The occurrence of the highest predictivity in a combination of bacterial mutagenicity and DNA lesions in our review suggested a close relationship between genotoxicity and carcinogenic activity. The bacterial mutagenicity test was often used to measure the ability of a drug to cause mutations rather than a definitive test of the carcinogens. The in vivo DNA lesion tests can detect the chemicals that reach the appropriate target with an effective dose to convert into a permanent mutation by reacting with DNA. In a few cases, the mutation escaped monitoring to survive and subsequently, carcinogenicity was generated through a loss of restriction of cell division. The in vivo DNA lesions can identify this “survived mutation.” Thus, the combination of bacterial mutagenicity and DNA lesions showed a higher and more accurate predictivity of carcinogenicity.
The correlation between the results of genotoxicity and carcinogenicity assays of antiparasitics was indicated in Table 9. Among the antiparasitics that were classified as genotoxic carcinogens, 69.2% tested positive in in vitro and/or in vivo DNA lesions exhibiting a greater sensitivity to carcinogens than any other types of genotoxicity assays. Eight out of 19 (42.1%) antiparasitics gave negative results in bacterial mutagenicity and were identified as non-carcinogens. Sensitivity and specificity are commonly used to describe the capability of in vitro genotoxicity assays (257). Sensitivity is defined as the percentage of genotoxic carcinogens that produced positive results in the considered test, and specificity is regarded as the ratio of non-carcinogens that gave negative responses. The ability of a battery of three in vitro genotoxicity tests to discriminate between rodent carcinogens and non-carcinogens was made by Kirkland et al. to increase the specificity of a valid test (258). The conclusion was that the “profile” of the genotoxicity results, such as the concentration, the level of toxicity and magnitude of response, provided a body of evidence to predict the carcinogenic results (259).
The rodent bioassays were useful and relevant for predicting risks of human cancers (260). The epigenetic changes with a loss of restriction of cell division (261) and the DNA oxidative stress damage were likely to produce cancer. Trosko and Upham found that the changes in gene expression caused by cell communication systems play a key role in the imbalance of cell proliferation, differentiation, and apoptosis, eventually promoting the tumor process (262). A large number of rodent tumor findings were found not relevant for humans (262) recently. It is worth noting that traditional carcinogenicity studies are largely not predictive of human cancer risk, therefore the well-suited approaches were proposed, for instance, the genetically modified animal models (15), and in vitro carcinogenicity screening assays based on gene expression profiling (16, 263). From the perspective of prospects, a more useful and accurate method to predict the carcinogenicity in humans is very urgent.
Herein, 136 antiparasitics were collected from both human and veterinary pharmacopeia. Due to the design of toxicity and the highest concentration in in vitro genetic toxicity tests have changed enormously in current guidelines, the reliably of old data were evaluated and as low as 45.5%. For a larger proportion of antiparasitics, whose genotoxicity and/or carcinogenicity results were not retrievable, the retesting based on revised guidelines should be done to make a safety assessment of human health. The combination of DNA lesions and bacterial mutagenicity is more accurate for predicting carcinogenicity than bacterial mutagenicity alone or together with any other genotoxicity testing. Development of this method for predicting carcinogens should be applied to reduce the misleading hazard alerts of the new and effective drugs.
Author Contributions
ZY conceived the idea. XW analyzed and discussed data. QL analyzed and discussed data and wrote the article. ZL performed and revised the experiments. AI and FZ revised the article. All the authors discussed the results and contributed to the final manuscript.
Conflict of Interest Statement
The authors declare that the research was conducted in the absence of any commercial or financial relationships that could be construed as a potential conflict of interest.
Funding
This work was supported by the National Key Research and Development Program of China (2017YFD0501405 and 2017YFD0501401), Natural Science Foundation of China (grant nos. 31272614 and 31502115), grants from 2017 National Risk Assessment of Quality and Safety of Livestock and Poultry Products (GJFP2017008), and Research on the detection standard of veterinary drug residue (2662015PY021).
References
1. Pimentel D, Greiner A, Bashore T. Economic and Environmental Costs of Pesticide Use. Environmental Toxicology: Current Developments. UK: Gordon and Breach Science Publishers (1998). p. 121–87.
3. Hoover R, Fraumeni JF Jr. Drugs in clinical use which cause cancer. J Clin Pharmacol (1975) 15:16–23. doi: 10.1002/j.1552-4604.1975.tb01423.x
4. Barthel E. Increased risk of lung cancer in pesticide-exposed male agricultural workers. J Toxicol Environ Health (1981) 8:1027–40. doi:10.1080/15287398109530135
5. Ji BT, Silverman DT, Stewart PA, Blair A, Swanson GM, Baris D, et al. Occupational exposure to pesticides and pancreatic cancer. Am J Ind Med (2001) 39:92–9. doi:10.1002/1097-0274(200101)39:1<92::AID-AJIM9>3.0.CO;2-P
6. Viel JF, Challier B. Bladder cancer among French farmers: does exposure to pesticides in vineyards play a part? Occup Environ Med (1995) 52:587–92. doi:10.1136/oem.52.9.587
7. Brown LM, Blair A, Gibson R, Everett GD, Cantor KP, Schuman LM, et al. Pesticide exposures and other agricultural risk factors for leukemia among men in lowa and Minnesota. Cancer Res (1990) 50:6585–91.
8. Blair A, Zahm SH, Pearce NE, Heinemann EF, Fraumeni JF. Clues to cancer etiology from studies of farmers. Scand J Work Environ Health (1992) 18:209–15. doi:10.5271/sjweh.1578
9. Blair A, Zahm SH. Agricultural exposures and cancer. Environ Health Perspect (1995) 103:205–8. doi:10.2307/3432311
10. Meinert R, Schuz J, Kaletsch U, Kaatsch P, Michaelis J. Leukemia and non-Hodgkin’s lymphoma in childhood and exposure to pesticides: results of a register-based case-control study in Germany. Am J Epidemiol (2000) 151:639–46. doi:10.1093/oxfordjournals.aje.a010256
11. (ICH) S2B. Genotoxicity: A Standard Battery for Genotoxicity Testing of Pharmaceuticals. (2012).
12. VICH. Veterinary International Conference on Harmonization. Safety Studies for Veterinary Drug Residues in Human Food: Genotoxicity Studies. (2000).
13. FDA. Toxicological principles for the safety assessment of food ingredients. IV.C.1.a. Bacterial Reverse Mutation Test. (2000).
14. (ICH) S2 (R1). Guidance on Genotoxicity Testing and Data Interpretation for Pharmaceuticals Intended for Human Use. (2001).
15. (ICH) S2 (R1). Guidance for Industry Genotoxicity Testing and Data Interpretation for Pharmaceuticals Intended for Human use. (2012).
16. Vinken M, Doktorova T, Ellinger-Ziegelbauer H, Ahr HJ, Lock E, Carmichael P, et al. The carcinogenomics project: critical selection of model compounds for the development of omics-based in vitro carcinogenicity screening assays. Mutat Res (2008) 659:202–10. doi:10.1016/j.mrrev.2008.04.006
17. (ICH) S2 (R1). Revised Guidance on Genotoxicity Testing and Data Interpretation for Pharmaceuticals Intended for Human Use. (2010).
19. Snyder RD, Green JW. A review of the genotoxicity of marketed pharmaceuticals. Mutat Res (2001) 488:151–69. doi:10.1016/S1383-5742(01)00055-2
21. IARC. IARC Monographs on the Evaluation of Carcinogenic Risks to Humans. Lyon: IARC (1972). p. 1–90.
22. (ICH) S1B. Carcinogenicity: Testing for Carcinogenicity of Pharmaceuticals, CPMP/ICH/299/95. (1998).
23. HHS. Registry of Toxic Effects of Chemical Substances, 1985–1986. Washington: US Department of Health and Human Sciences (1988).
26. OECD. OECD Guidelines for the Testing of Chemicals. Test Guideline 471. Bacterial Reverse Mutation Test. (1997).
27. FDA. Toxicological principles for the safety assessment of food ingredients. IV.C.1.d. Mammalian Erythrocyte Micronucleus Test. (2000).
29. FDA. Toxicological principles for the safety assessment of food ingredients. IV.C.1.c. In Vitro Mouse Lymphoma TK ± Gene Mutation Assay. (2000).
30. OECD. OECD Guidelines for the Testing of Chemicals. Test No. 490: In Vitro Mammalian Cell Gene Mutation Tests Using the Thymidine Kinase Gene. OECD – Organisation for Economic Cooperation and Development (2015).
31. OECD. OECD Guidelines for the Testing of Chemicals. Test Guideline 473. In Vitro Mammalian Chromosomal Aberration Test. OECD – Organisation for Economic Cooperation and Development (2014).
32. FDA. Toxicological principles for the safety assessment of food ingredients. IV.C.1.b. In Vitro Mammalian Chromosomal Aberration Test. (2000).
33. OECD. OECD Guidelines for the Testing of Chemicals. Test Guideline 474. Mammalian Erythrocyte Micronucleus Test. OECD – Organisation for Economic Cooperation and Development (2014).
34. OECD. OECD Guidelines for the Testing of Chemicals. Test Guideline 476. In Vitro Mammalian Cell Gene Mutation Test. OECD – Organisation for Economic Cooperation and Development (1997).
36. OECD. Guideline for the Testing of Chemicals. Guideline 453:Combined. Paris: Chronic Toxicity\Carcinogenicity Studies (2009).
37. Brown JP, Brown RJ. Mutagenesis by 9,10-anthraquinone derivatives and related compounds in Salmonella typhimurium. Mutat Res (1976) 40:203–24. doi:10.1016/0165-1218(76)90046-X
38. Scott BR, Dorn GL, Kaefer E, Stafford R. Aspergillus nidulans: systems and results of tests for induction of mitotic segregation and mutation, 2. haploid assay systems and overall response of all systems: a report of the US EPA Gene–Tox Program. Mutat Res (1982) 98:49–94. doi:10.1016/0165-1110(82)90003-3
39. Oberly TJ, Rexroat MA, Bewsey BJ, Richardson KK, Michaelis KC. An evaluation of the CHO/hgprt mutation assay involving suspension cultures and soft agar cloning: results for 33 chemicals. Environ Mol Mutagen (1990) 16:260–71. doi:10.1002/em.2850160408
40. Preston RJ, Au W, Bender MA, Brewen JG, Carrano AV, Heddle JA. Mammalian in vivo and in vitro cytogenetic assays: a report of the U.S. EPA’s Gene–Tox Program. Mutat Res (1981) 87(2):143–88. doi:10.1016/0165-1110(81)90030-0
41. Propping P, Röhrborn G, Buselmaier W. Comparative investigations on the chemical induction of point mutations and dominant lethal mutations in mice. Mol Gen Genet (1972) 117:197–209.
42. Alba P, Ferrs MD, Xamena N, Creus A, Marcos R. Genotoxicity of acridine orange and acriflavine in Drosophila melanogaste. Mutat Res (1983) 121:199–203. doi:10.1016/0165-7992(83)90204-X
43. Heddle JA, Hite M, Kirkhart B, Mavournin K, Mac Gregor JT, Newell GW, et al. The induction of micronuclei as a measure of genotoxicity, a report of the U.S. Environmental Protection Agency Gene–Tox Program. Mutat Res (1983) 123:61–118. doi:10.1016/0165-1110(83)90047-7
44. Zimmermann FK, Von Borstel RC, Von Halle ES, Parry JM, Siebert D, Zetterberg G, et al. Testing of chemicals for genetic activity with Saccharomyces cerevisiae: a report of the U.S. Environmental Protection Agency Gene–Tox Program. Mutat Res (1984) 133:199–244. doi:10.1016/0165-1110(84)90017-4
45. Wyrobek AJ, Gordon LA, Burkhart JG. An evaluation of human sperm as indicators of chemically induced alterations of spermatogenic function: a report of the U.S. Environmental Protection Agency Gene–Tox Program. Mutat Res (1983) 115:73–148. doi:10.1016/0165-1110(83)90015-5
46. Wyrobek AJ, Gordon LA, Burkhart JG, Francis MW, Kapp RW Jr, Letz G, et al. An evaluation of the mouse sperm morphology test and other sperm tests in nonhuman mammals, a report of the US Environmental Protection Agency Gene–Tox Program. Mutat Res (1983) 115:1–72. doi:10.1016/0165-1110(83)90014-3
48. Oztas S, Salman AB, Tatar A, Yigiter M, Yazgi H, Ertek M, et al. Genotoxic effect of albendazole in pediatric patients with hepatic hydatid disease. Int J Infect Dis (2007) 11:446–9. doi:10.1016/j.ijid.2007.01.002
49. Ramírez T, Benítez-Bribiesca L, Ostrosky-Wegman P, Herrera LA. In vitro effects of albendazole and its metabolites on the cell proliferation kinetics and micronuclei frequency of stimulated human lymphocytes. Arch Med Res (2001) 32:119–22. doi:10.1016/S0188-4409(01)00259-4
50. Ermler S, Scholze M, Kortenkamp A. Seven benzimidazole pesticides combined at sub-threshold levels induce micronuclei in vitro. Mutagenesis (2013) 28:417–26. doi:10.1093/mutage/get019
51. Tudek B, Gajewska J, Szczypka M, Rahden-Staron I, Szymczyk T. Screening for genotoxic activity of amitraz with short-term bacterial assays. Mutat Res (1988) 204:585–91. doi:10.1016/0165-1218(88)90061-4
52. Osano O, Admiraal W, Klamer HJ, Pastor D, Bleeker EA. Comparative toxic and genotoxic effects of chloroacetanilides, formamidines and their degradation products on Vibrio fischeri and Chironomus riparius. Environ Pollut (2002) 119:195–202. doi:10.1016/S0269-7491(01)00334-7
53. Padula G, Ponzinibbio MV, Picco S, Seoane A. Assessment of the adverse effects of the acaricide amitraz: in vitro evaluation of genotoxicity. Toxicol Mech Methods (2012) 22:657–61. doi:10.3109/15376516.2012.666683
55. Chatterjee T, Mukopadhyay A, Khan KA, Giri K. Comparative mutagenic and genotoxic effects of three antimalarial drugs, chloroquine, primaquine and amodiaquine. Mutagenesis (1998) 13:619–24. doi:10.1093/mutage/13.6.619
56. Shubber EK, Jacobson–Kram D, Williams JR. Comparison of the Ames assay and the induction of sister chromatid exchanges: results with ten pharmaceuticals and five selected agents. Cell Biol Toxicol (1986) 2:379–99. doi:10.1007/BF00121853
57. Gupta RL, Kaur IP, Juneja TR. Mutagenicity of the antischistosomal agent amoscanate and its hydrolytic and related parasubstituted 4-nitrodiphenylamine products. Med Sci Res (1992) 20:365–8.
58. Egito LC, Medeiros SR, Medeiros MG, Price JC, Egito ES. Evaluation of the relationship of the molecular aggregation state of amphotericin B in medium to its genotoxic potential. J Pharm Sci (2004) 93:1557–65. doi:10.1002/jps.20038
59. Mourot D, Mourot A. Mutagenicity of bithionol sulfoxide and its metabolites in the salmonella/mammalian microsome test. Mutat Res (1987) 188:53–5. doi:10.1016/0165-1218(87)90114-5
60. Zeiger E, Anderson B, Haworth S, Lawlor T, Mortelmans K. Salmonella mutagenicity tests: IV. Results from the testing of 300 chemicals. Environ Mol Mutagen (1988) 11:1–157. doi:10.1002/em.2850110602
61. Yoshimura H, Nakamura M, Koeda T. Lack of mutagenicity of fasciolicides. Vet Res Commun (1985) 9:147–52. doi:10.1007/BF02215138
62. Simmon VF, Riccio ES, Robinson DE, Mitchell AD. In Vitro Microbiological Mutagenicity and Unscheduled DNA Synthesis Studies of Fifteen Pesticides. (1985). EPA/600/1–85/006, ntis pb. 85–193761.
63. IARC. IARC Monographs on the Evaluation of the Carcinogenic Risk of Chemicals to Humans (Chlordimeform). (Vol. 30). (1983). p. 61–72.
66. Mitchell AD, Casciano DA, Meltz ML, Robinson DE, San RC, Williams GM, et al. Unscheduled DNA synthesis tests: a report of the U.S. Environmental Protection Agency Gene–Tox Program. Mutat Res (1983) 123:363–410. doi:10.1016/0165-1110(83)90029-5
68. Lang R. The mammalian spot test and its use for testing of mutagenic and carcinogenic potential: experience with the pesticide chlordimeform, its principal metabolites and the drug lisuride hydrogen maleate. Mutat Res (1984) 135:219–24. doi:10.1016/0165-1218(84)90125-3
69. Li F. A research of chlorphenamidine on labour hygiene and the mutation effect on human. China Acad J Electron Publish House (1985).
70. Leslie C, Reidy GF, Murray M, Stacey NH. Induction of xenobiotic biotransformation by the insecticide chlordimeform, a metabolite 4-chloro-o-toluidine and a structurally related chemical o-toluidine. Biochem Pharmacol (1988) 37:2529–35. doi:10.1016/0006-2952(88)90242-0
71. Weisbuurger EK, Russfield AB, Homburger F, Weisburger JH, Boger Van E, Dongen CG, et al. Testing of twenty-one environmental aromatic amines or derivatives for long-term toxicity or carcinogenicity. Environ Pathol Toxicol (1978) 2:325–56.
72. Thomas SM, Silburn KA, MacPhee DG. Frameshift mutagenesis by chloroquine in Escherichia coli and Salmonella typhimurium. Mutat Res (1987) 192:233–7. doi:10.1016/0165-7992(87)90062-5
74. Espinosa-Aguirre JJ, Ramírez Santos J, Cortinas de Nava C. Influence of the UVR repair system on the mutagenicity of antiparasitic drugs. Mutat Res (1989) 222:161–6. doi:10.1016/0165-1218(89)90132-8
75. Available from: http://www.toxnet.nlm.nih.gov
76. Kadotani S, Arisawa M, Maruyama HB. Mutagenicity examination of several non-steroidal anti-inflammatory drugs in bacterial systems. Mutat Res (1984) 138:133–6. doi:10.1016/0165-1218(84)90035-1
77. O’Donovan MR. The comparative responses of Salmonella typhimurium TA1537 and TA97A to a range of reference mutagens and novel compounds. Mutagenesis (1990) 5:267–74. doi:10.1093/mutage/5.3.267
78. Sideropoulos AS, Specht SM. Evaluation of microbial testing methods for the mutagenicity of quinoline and its derivatives. Current Microbiol (1984) 11:59–65. doi:10.1007/BF01567705
79. Leifer Z, Kada T, Mandel M, Zeiger E, Stafford R, Rosenkranz HS. Evaluation of tests using DNA repair-deficient bacteria for predicting genotoxicity and carcinogenicity: a report of the U.S. EPA’s Gene–Tox Program. Mutat Res (1981) 87:211–97. doi:10.1016/0165-1110(81)90015-4
80. Donya SM, Souria M, Hassan EE. Clastogenetic effects of the fasciolicides closantel and nitroxynil on mice somatic and germ cells. Cytologia (2007) 72:29–36. doi:10.1508/cytologia.72.29
81. Dunkel VC, Zeiger E, Brusick D, McCoy E, McGregor D, Mortelmans K, et al. Reproducibility of microbial mutagenicity assays: II. Testing of carcinogens and noncarcinogens in Salmonella typhimurium and Escherichia coli. Environ Mol Mutagen (1985) 7:1–248. doi:10.1002/em.2860070902
83. Loveday KS, Lugo MH, Resnick MA, Anderson BE, Zeiger E. Chromosome aberration and sister chromatid exchange tests in Chinese hamster ovary cells in vitro: II. Results with 20 chemicals. Environ Mol Mutagen (1989) 13:60–94. doi:10.1002/em.2850130108
84. NCINTP. Carcinogenesis Technical Report Series. National Cancer Institute/National Toxicology Program; US Department of Health and Human Services. National Toxicology Program Technical Report Series 192. (1979).
85. NCINTP. Carcinogenesis Technical Report Series. National Cancer Institute/National Toxicology Program; US Department of Health and Human Services. National Toxicology Program Technical Report Series 96. (1979).
86. Ila HB, Topaktas M, Rencuzogullari E, Kayraldiz A, Donbak L, Daglioglu YK. Genotoxic potential of cyfluthrin. Mutat Res (2008) 656:49–54. doi:10.1016/j.mrgentox.2008.07.005
87. Tisch M, Faulde MK, Maier H. Genotoxic effects of pentachlorophenol, lindane, transfluthrin, cyfluthrin, and natural pyrethrum on human mucosal cells of the inferior and middle nasal conchae. Am J Rhinol (2005) 19:141–51.
88. Marinowic DR, Mergener M, Pollo TA, Maluf SW, Silva LB. In vivo genotoxicity of the pyrethroid pesticide beta-cyfluthrin using the comet assay in the fish Bryconamericus iheringii. Z Naturforsch C (2012) 67:308–11. doi:10.1515/znc-2012-5-610
89. Isidori M, Caterino E, Criscuolo E, Fatigati V, Liguori G, Parrella A. Antimutagenic and antigenotoxic effects of vegetable matrices on the activity of pesticides. Food Addit Contam Part A Chem Anal Control Expo Risk Assess (2009) 26:1049–62. doi:10.1080/02652030902896194
90. Sankar P, Telang AG, Manimaran A. Curcumin protects against cypermethrin-induced genotoxicity in rats. Environ Toxicol Pharmacol (2010) 30:289–91. doi:10.1016/j.etap.2010.07.005
91. Bhunya SP, Pati PC. Genotoxic effects of a synthetic pyrethroid insecticide, cypermethrin, in mice in vivo. Toxicol Lett (1988) 41:223–30. doi:10.1016/0378-4274(88)90058-6
92. Kocaman AY, Topaktaş M. Genotoxic effects of a particular mixture of acetamiprid and alpha-cypermethrin on chromosome aberration, sister chromatid exchange, and micronucleus formation in human peripheral blood lymphocytes. Environ Toxicol (2010) 25:157–68.
93. Cui Y, Guo J, Xu B, Chen Z. Genotoxicity of chlorpyrifos and cypermethrin to ICR mouse hepatocytes. Toxicol Mech Methods (2011) 21:70–4. doi:10.3109/15376516.2010.529192
94. Kocaman AY, Topakta M. The in vitro genotoxic effects of a commercial formulation of alpha-cypermethrin in human peripheral blood lymphocytes. Environ Mol Mutagen (2009) 50:27–36. doi:10.1002/em.20434
95. Ansari RA, Rahman S, Kaur M, Anjum S, Raisuddin S. In vivo cytogenetic and oxidative stress-inducing effects of cypermethrin in freshwater fish, Channa punctata Bloch. Ecotoxicol Environ Saf (2011) 74:150–6. doi:10.1016/j.ecoenv.2010.08.036
96. Patel S, Pandey AK, Bajpayee M, Parmar D, Dhawan A. Cypermethrin-induced DNA damage in organs and tissues of the mouse: evidence from the comet assay. Mutat Res (2006) 607:176–83. doi:10.1016/j.mrgentox.2006.04.010
97. Murkunde YV, Sathya TN, Subashini N, Murthy PB. Transplacental genotoxicity evaluation of cypermethrin using alkaline comet assay. Hum Exp Toxicol (2012) 31:185–92. doi:10.1177/0960327111412091
98. Kasimoglu C, Uysal H. Mutagenic biomonitoring of pirethroid insecticides in human lymphocyte cultures: use of micronuclei as biomarkers and recovery by Rosa canina extracts of mutagenic effects. Pharm Biol (2014) 21:1–5.
99. Surrallés J, Xamena N, Creus A, Marcos R. The suitability of the micronucleus assay in human lymphocytes as a new biomarker of excision repair. Mutat Res (1995) 342:43–59. doi:10.1016/0165-1218(95)90089-6
101. Brusick DJ, Simmon VF, Rosenkranz HS, Ray VA, Stafford RS. An evaluation of the Escherichia coli WP2 and WP2 UVRA reverse mutation assay. Mutat Res (1980) 76:169–90. doi:10.1016/0165-1110(80)90009-3
102. Barrueco C, Herrera A, de la Pena E. Mutagenic evaluation of trichlorfon using different assay methods with Salmonella typhimurium. Mutagenesis (1991) 6:71–6. doi:10.1093/mutage/6.1.71
103. Zeiger E, Anderson B, Haworth S, Lawlor T, Mortelmans K, Speck W. Salmonella mutagenicity tests: III. Results from the testing of 255 chemicals. Environ Mol Mutagen (1987) 9:1–110. doi:10.1002/em.2860090602
104. Aquilina G, Benigni R, Bignami M, Calcagnile A, Dogliotti E, Falcone E, et al. Genotoxic activity of dichlorvos, trichlorfon and dichloroacetaldehyde. Pestic Sci (1984) 15:439–42. doi:10.1002/ps.2780150504
105. Tian Y, Ishikawa H, Yamauchi T. Analysis of cytogenetic and developmental effects on preimplantation mouse embryos from dams treated with organophosphate insecticide trichlorfon. Huanjing yu zhiye yixue (2004) 21:391–3.
106. Igarashi M, Setoguchi M, Takada S, Itoh S, Furuhama K. Optimum conditions for detecting hepatic micronuclei caused by numerical chromosome aberration inducers in mice. Mutat Res (2007) 632:89–98. doi:10.1016/j.mrgentox.2007.04.012
107. Pluijmen M, Drevon C, Montesano R, Malaveille C, Hautefeuille A, Bartsch H. Lack of mutagenicity of synthetic pyrethroids in Salmonella typhimurium strains and in V-79 Chinese hamster cells. Mutat Res (1984) 137:7–15. doi:10.1016/0165-1218(84)90106-X
109. Grisolia CK. A comparison between mouse and fish micronucleus test using cyclophosphamide, mitomycin C and various pesticides. Mutat Res (2002) 518:145–50. doi:10.1016/S1383-5718(02)00086-4
110. Shukla Y, Arora A, Singh A. Tumorigenic studies on deltamethrin in swiss albino mice. Toxicology (2001) 163:1–9. doi:10.1016/S0300-483X(00)00416-9
111. Cabral JR, Galendo D, Laval M, Lyandrat N. Carcinogenicity studies with deltamethrin in mice and rats. Cancer Lett (1990) 49:147–52. doi:10.1016/0304-3835(90)90151-M
112. Ono T, Sekiya T, Takahashi Y, Sasaki YF, Izumiyama F, Nishidate E, et al. The genotoxicity of diaveridine and trimethoprim. Environ Toxicol Pharmacol (1997) 3:297–306. doi:10.1016/S1382-6689(97)00026-4
113. Yoshimura H. Mutagenicity of the coccidiostat diaveridine in the Salmonella mammalian microsome assay. Mutat Res (1991) 261:149–52. doi:10.1016/0165-1218(91)90061-P
114. Marshall TC, Dorough HW, Swim HE. Screening of pesticides for mutagenic potential using Salmonella typhimurium mutants. Jagric Food Chem (1976) 24:560–3. doi:10.1021/jf60205a013
115. Kier LD, Brusick DJ, Auletta AE, et al. The Salmonella typhimurium/mammalian microsome mutagenicity assay; an report of the US Environmental Protection Agency Gene–Tox Program. Mutat Res (1986) 168:67–238.
116. Kier LD, Brusick DJ, Auletta AE, Von Halle ES, Brown MM, Simmon VF, et al. The Salmonella typhimurium/mammalian microsomal assay, a report of the U.S. Environmental Protection Agency Gene–Tox Program. Mutat Res (1986) 168:69–240. doi:10.1016/0165-1110(86)90002-3
117. Wong PK, Wai CC, Liong E. Comparative study on mutagenicities of organophosphorus insecticides in salmonella. Chemosphere (1989) 18:2413–22.
118. Shadboorestan A, Shokrzadeh M, Ahangar N, Abdollahi M, Omidi M, Hosseini Payam SS. The chemoprotective effects of L-carnitine against genotoxicity induced by diazinon in rat blood lymphocyte. Toxicol Ind Health (2013).
119. Nishio A, Uyeki EM. Induction of sister chromatid exchanges in Chinese hamster ovary cells by organophosphate insecticides and their oxygen analogs. Toxicol Environ Health (1981) 8:939–46. doi:10.1080/15287398109530128
120. Sobti RC, Krishan A, Pfaffenberger CD. Cytokinetic and cytogenetic effects of some agricultural chemicals on human lymphoid cells in vitro: organophosphates. Mutat Res (1982) 102:89–102. doi:10.1016/0165-1218(82)90149-5
121. Shokrzadeh M, Ahmadi A, Ramezaninejhad S, Shadboorestan A. Hesperidin, a citrus bioflavonoid, ameliorates genotoxicity-induced by diazinon in human blood lymphocytes. Drug Res (2014) 65:57–60. doi:10.1055/s-0033-1363998
122. NCINTP. Carcinogenesis Technical Report Series. National Cancer Institute/National Toxicology Program; US Department of Health and Human Services. National Toxicology Program Technical Report Series. 24. (1978).
123. NCINTP. Carcinogenesis Technical Report Series. National Cancer Institute/National Toxicology Program; US Department of Health and Human Services. National Toxicology Program Technical Report Series 77. (1978).
124. Choi EJ, Kim YK, Roh IK. Genetic toxicity of pesticides used in Korea on salmonella typhimurium and saccharomyces cerevisiae. Environ Mutagens Carcinog (1985) 5:11–8.
125. NCINTP. Carcinogenesis Technical Report Series. National cancer institute/national toxicology program, US Department of Health and Human Services. National Toxicology Program Technical Report Series 342. (1989).
126. Breau AP, Mitchell WM, Swinson J, Field L. Mutagenic and cell transformation activities of representative phosphorothioate esters in vitro. J Toxicol Environ Health (1985) 16:403–13. doi:10.1080/15287398509530750
127. Moriya M, Ohta T, Watanabe K, Miyazawa T, Kato K, Shirasu Y. Further mutagenicity studies on pesticides in bacterial reversion assay systems. Mutat Res (1982) 116:185–216. doi:10.1016/0165-1218(83)90059-9
128. Buselmaier W, Roehrborn G, Propping P. Mutagenicity investigations with pesticides in the host-mediated assay and the dominant lethal test in mice. Biol Zentralbl (1972) 91:311–25.
129. Latt SA. Sister-chromatid exchanges: a report of the gene–tox program. Mutat Res (1981) 87:17–62. doi:10.1016/0165-1110(81)90003-8
131. Selden JR, Dolbeare F, Clair JH, Miller JE, McGettigan K, DiJohn JA, et al. Validation of a flow cytometric in vitro DNA repair (UDS) assay in rat hepatocytes. Mutat Res (1994) 315(2):147–67. doi:10.1016/0921-8777(94)90015-9
132. Shirasu Y, Moriya M, Kato K, Furuhashi A, Kada T. Mutagenicity screening of pesticides in the microbial system. Mutat Res (1976) 40:19–30. doi:10.1016/0165-1218(76)90018-5
133. Green MH, Muriel WJ, Bridges BA. Use of a simplified fluctuation test to detect low levels of mutagens. Mutat Res (1976) 38:33–42. doi:10.1016/0165-1161(76)90077-7
134. Paik SG, Lee SY. Genetic effects of pesticides in the mammalian cells: 1. Induction of micronucleus. Tongmul Hakhoe Chi (1977) 20:19–28.
135. Anderson BE, Zeiger E, Shelby MD, Resnick MA, Gulati DK, Ivett JL, et al. Chromosome aberration and sister chromatid exchange test results with 42 chemicals. Environ Mol Mutagen (1990) 16:55–137. doi:10.1002/em.2850160505
136. Grant WF. Chromosome aberration assays in allium, a report of the U.S. Environmental Protection Agency Gene–Tox Program. Mutat Res (1982) 99:273–91. doi:10.1016/0165-1110(82)90046-X
137. Dean BJ, Thorpe E. Studies with dichlorvos vapor in dominant lethal mutation tests on mice. Arch Toxikol (1970) 30:51–9. doi:10.1007/BF00605273
138. Oshiro Y, Piper CE, Balwierz PS, Soelter SG. Chinese hamster ovary cell assays for mutation and chromosome damage: data from non-carcinogens. J Appl Toxicol (1991) 11:167–77. doi:10.1002/jat.2550110304
139. Benford DJ, Price SC, Lawrence JN, Grasso P, Bremmer JN. Investigations of the genotoxicity and cell proliferative activity of dichlorvos in mouse forestomach. Toxicology (1994) 92:203–15. doi:10.1016/0300-483X(94)90178-3
140. Lee WR, Abrahamson S, Valencia R, Von Halle ES, Wuerglre FE, Zimmering S. The sex-linked recessive lethal test for mutagenesis in drosophila melanogaster: a report of the U.S. Environmental Protection Agency Gene–Tox Program. Mutat Res (1983) 123:183–279. doi:10.1016/0165-1110(83)90025-8
141. Nesnow S, Argus M, Bergman H, et al. Chemical carcinogens: a review and analysis of the literature of selected chemicals and the establishment of the gene–tox carcinogen data base: a report of the U.S. Environmental Protection Agency Gene–Tox Program. Mutat Res (1987) 185:1–195. doi:10.1016/0165-1110(87)90017-0
142. NCINTP. Carcinogenesis Technical Report Series. National Cancer Institute/National Toxicology Program; US Department of Health and Human Services. National Toxicology Program Technical Report Series 10. (1977).
143. Horn KH, Teichmann B, Schramm T, Nischan P. Investigation of dichlorvos (DDVP), part II. Testing of dichlorvos for carcinogenic activity in rats. Arch Geschwulstforsch (1988) 58:1–10.
144. Chan PC, Huff J, Haseman JK, Alison R, Prejean JD, et al. Carcinogenesis studies of dichlorvos in Fischer rats and B6C3F1 mice. J Cancer Res (1991) 82:157–64.
145. Rosenkranz HS Jr, Speck WT, Stambaugh JE. Mutagenicity of metronidazole: structure-activity relationships. Mutat Res (1976) 38:203–6. doi:10.1016/0165-1161(76)90191-6
146. McCann J, Choi E, Yamasaki E, Ames BN. Detection of carcinogens as mutagens in the salmonella/microsome test: assay of 300 chemicals. Proc Natl Acad Sci U S A (1975) 2:5135–9. doi:10.1073/pnas.72.12.5135
147. Vanelle P, Dmeo MP, Maldonado J, Nouguier R, Crozet MP, Laget M, et al. Genotoxicity in oxazolidine derivatives: influence of the nitro group. Eur J Med Chem (1990) 25:241–50. doi:10.1016/0223-5234(90)90207-J
148. Ré JL, De Méo MP, Laget M, Guiraud H, Castegnaro M, Vanelle P, et al. Evaluation of the genotoxic activity of metronidazole and dimetridazole in human lymphocytes by the comet assay. Mutat Res (1997) 375:147–55. doi:10.1016/S0027-5107(97)00010-9
149. Przybojewska B, Barański B, Spiechowicz E, Dziubałtowska E, Szymczak W. Potential carcinogenicity assessment ofalpha-aescin and phenbendasol. Acta Pol Pharmal (1994) 51:89–93.
150. Huang N, Cerepnalkoski L, Nwankwo JO, Dews M, Landolph JR. Induction of chromosomal aberrations, cytotoxicity, and morphological transformation in mammalian cells by the antiparasitic drug flubendazole and the antineoplastic drug harringtonine. Fundam Appl Toxicol (1994) 22:304–13. doi:10.1006/faat.1994.1034
151. Rani MV, Rao MS. In vitro effect of fenthion on human lymphocytes. Bull Environ Contam Toxicol (1991) 47:316–20. doi:10.1007/BF01688658
152. Chen HH, Sirianni SR, Huang CC. Sister chromatid exchanges in Chinese hamster cells treated with seventeen organophosphorus compounds in the presence of a metabolic activation system. Environ Mutagen (1982) 4:621–4. doi:10.1002/em.2860040513
154. Bai CL, Qiao CB, Zhang WD, Chen YL, Qu SX. A study of the pesticide fenthion: toxicity, mutagenicity, and influence on tissue enzymes. Biomed Environ Sci (1990) 3:262–75.
156. Herrera A, Laborda E. Mutagenic activity in synthetic pyrethroids in Salmonella typhimurium. Mutagenesis (1988) 3:509–14. doi:10.1093/mutage/3.6.509
157. Giri A, Giri S, Sharma GD. Malathion and fenvalerate induce micronuclei in mouse bone marrow cells. Environ Mol Mutagen (2011) 52:607–13. doi:10.1002/em.20649
158. Caballo C, Herrera A, Barrueco C, Santa-María A, Sanz F, de la Peña E. Analysis of cytogenetic damage induced in CHO cells by the pyrethroid insecticide fenvalerate. Teratog Carcinog Mutagen (1992) 12:243–9. doi:10.1002/tcm.1770120602
160. Parker CM, Patterson DR, Van Gelder GA, Gordon EB, Valerio MG, Hall WC. Chronic toxicity and carcinogenicity evaluation of fenvalerate in rats. Toxicol Environ Health (1984) 13:83–97. doi:10.1080/15287398409530483
161. Parker CM, Mccullough CB, Gellatly JBM, Johnston CD. Toxicologic and carcinogenic evaluation of fenvalerate in the B6C3F1 mouse. Fundam Appl Toxicol (1983) 3:114–20. doi:10.1016/S0272-0590(83)80066-9
163. Çelik A, Ekinci SY, Güler G, Yildirim S. In vitro genotoxicity of fipronil sister chromatid exchange, cytokinesis block micronucleus test, and comet assay. DNA Cell Biol (2014) 33:148–54. doi:10.1089/dna.2013.2158
164. Ghisi NC, Ramsdorf WA, Ferraro MV, de Almeida MI, Ribeiro CA, Cestari MM. Evaluation of genotoxicity in Rhamdia quelen (Pisces, Siluriformes) after sub-chronic contamination with fipronil. Environ Monit Assess (2011) 180:589–99. doi:10.1007/s10661-010-1807-7
165. Sinsheimer JE, Giri AK, Osorio S, Wise DS, Townsend LB. Comparative mutagenicity and genotoxicity of the antiparasitic drugs, mebendazole, flubendazole and flubendazole oxime. Prog Clin Biol Res (1990) 340:225–34.
166. Bradley MO, Bhuyan B, Francis MC, Langenbach R, Peterson A, Huberman E. Mutagenesis by chemical agents in V79 Chinese hamster cells: a review and analysis of the literature, a report of the Gene–Tox Program. Mutat Res (1981) 87:81–142. doi:10.1016/0165-1110(81)90029-4
167. Sturdik E, Rosenberg M, Stibranyi L, Balaz S, Chreno O, Ebringer L, et al. Structure-mutagenicity relationships of 5-nitro-2-furylethylenes in Salmonella typhimurium TA98. Chem Biol Interact (1985) 53:145–53. doi:10.1016/S0009-2797(85)80092-2
168. Brockman HE, Serres FJ, Ong TM, De Marini DM, Katz AJ, Griffiths AJ, et al. Mutation tests in Neurospora crassa, a report of the U.S. Environmental Protection Agency Gene–Tox Program. Mutat Res (1984) 133:87–134. doi:10.1016/0165-1110(84)90004-6
170. Ni YC, Shao BR. Comparison of the mutagenicity of 4 nitrofurans in Salmnella/microsome system. Zhongguo Yao Li Xue Bao (1985) 6:134–7.
171. Karabay NU, Oguz MG. Cytogenetic and genotoxic effects of the insecticides, imidacloprid and methamidophos. Genet Mol Res (2005) 4:653–62.
172. Jia Q, Guo M, Xie L, Liu YZ, Sai LL. A experimental mutagenesis study on active compound of imidacloprid. Chin J Ind Med (2008) 21(2):114–5.
173. Feng S, Kong Z, Wang X, Zhao L, Peng P. Acute toxicity and genotoxicity of two novel pesticides on amphibian, Rana N. Hallowell. Chemosphere (2004) 56:457–63. doi:10.1016/j.chemosphere.2004.02.010
174. Demsia G, Vlastos D, Goumenou M, Matthopoulos DP. Assessment of the genotoxicity of imidacloprid and metalaxyl in cultured human lymphocytes and rat bone-marrow. Mutat Res (2007) 634:32–9. doi:10.1016/j.mrgentox.2007.05.018
175. Costa C, Silvari V, Melchini A, Catania S, Heffron JJ, Trovato A, et al. Genotoxicity of imidacloprid in relation to metabolic activation and composition of the commercial product. Mutat Res (2009) 672:40–4. doi:10.1016/j.mrgentox.2008.09.018
176. Feng S, Kong Z, Wang X, Peng P, Zeng EY. Assessing the genotoxicity of imidacloprid and RH-5849 in human peripheral blood lymphocytes in vitro with comet assay and cytogenetic tests. Ecotoxicol Environ Saf (2005) 61:239–46. doi:10.1016/j.ecoenv.2004.10.005
177. Lin A, Zhu Y, Tong Y. Evaluation of genotoxicity of combined soil pollution by cadmium and imidacloprid. Sci China C Life Sci (2005) 48:7–13. doi:10.1007/BF02889796
178. Pérez–Iglesias JM, Ruiz de Arcaute C, Nikoloff N, Dury L, Soloneski S, Natale GS, et al. The genotoxic effects of the imidacloprid-based insecticide formulation Glacoxan Imida on Montevideo tree frog Hypsiboas pulchellus tadpoles (Anura, Hylidae). Ecotoxicol Environ Saf (2014) 104:120–6. doi:10.1016/j.ecoenv.2014.03.002
179. Kong ZM, Zang Y, Shen JP, Luo Y, Zhong Y. Effect of two new pesticides on sperm morphology of earthworms (Eisenia foetida). Carcinog Teratog Mutagen (1999) 11(1).
180. Calderón-Segura ME, Gómez-Arroyo S, Villalobos-Pietrini R, Martínez-Valenzuela C, Carbajal-López Y, Calderón-Ezquerro Mdel C, et al. Evaluation of genotoxic and cytotoxic effects in human peripheral blood lymphocytes exposed in vitro to neonicotinoid insecticides news. J Toxicol (2012) 2012:612647. doi:10.1155/2012/612647
182. O’Connor PJ, MacNaught F, Butler WH, Cooper DP, Margison GP, Povey AC. Increased pathology incidence in the forestomach of rats maintained on a diet containing ivermectin and given a single dose of N-methyl-N1-nitro-N-nitrosoguanidine. J Environ Pathol Toxicol Oncol (2001) 20:223–7. doi:10.1615/JEnvironPatholToxicolOncol.v20.i3.60
183. Kalantzi OI, Hewitt R, Ford KJ, Cooper L, Alcock RE, Thomas GO, et al. Low dose induction of micronuclei by lindane. Carcinogenesis (2004) 25:613–22. doi:10.1093/carcin/bgh048
184. Rupa DS, Reddy PP, Reddi OS. Genotoxic effect of benzene hexachloride in cultured human lymphocytes. Hum Genet (1989) 83:271–3. doi:10.1007/BF00285170
185. Bhunya SP, Jena GB. Genotoxic potential of the organochlorine insecticide lindane (g–BHC): an in vivo study in chicks. Mutat Res (1992) 272:175–81. doi:10.1016/0165-1161(92)90045-N
186. Constantin MJ, Nilan RA. Chromosome aberration assays in barley (Hordeum vulgare): a report of the U.S. Environmental Protection Agency Gene–Tox Program. Mutat Res (1982) 99:11–36. doi:10.1016/0165-1110(82)90029-X
187. Ma TH. Tradescantia cytogenetic tests (root-tip mitosis, pollen mitosis, pollen mother-cell meiosis); a report of the U.S. Environmental Protection Agency Gene–Tox Program. Mutat Res (1982) 99:293–302. doi:10.1016/0165-1110(82)90047-1
188. Tisch M, Bergenthal S, Maier H. Genotoxic effect of PCP and lindane on human epithelial tonsil cells. HNO (2002) 50:920–7. doi:10.1007/s00106-001-0621-5
189. Weisse I, Herbst M. Carcinogenicity study of lindane in the mouse. Toxicology (1977) 7:233–8. doi:10.1016/0300-483X(77)90069-5
190. Wolff GL, Suber RL. Hepatic glutathione S-transferase activity in mice: effects of Avy/-genotype, obesity, lindane treatment, and sex. Proc Soc Exp Biol Med (1986) 181:535–41. doi:10.3181/00379727-181-42289
191. Pednekar MD, Gandhi SR, Netrawali MS. Evaluation of mutagenic activities of endosulfan, phosalone, malathion, and permethrin, before and after metabolic activation in the ames salmonella test. Bull Environ Contam Toxicol (1987) 38:925–33. doi:10.1007/BF01609074
192. Amer SM, Fahmy MA. Genotoxicity studies on the organophosphorus insecticide “malathion” in mouse bone-marrow. Bull Natl Res Cent (2004) 29:93–108.
193. Galloway SM, Armstrong MJ, Reuben C, Colman S, Brown B, Cannon C, et al. Chromosome aberrations and sister chromatid exchanges in Chinese hamster ovary cells: evaluations of 108 chemicals. Environ Mol Mutagen (1987) 10:1–175. doi:10.1002/em.2850100502
194. Dulout FN, Olivero OA, Guradze Von H, Pastori MC. Cytogenetic effect of malathion assessed by the micronucleus test. Mutat Res (1982) 105:413–6. doi:10.1016/0165-7992(82)90186-5
195. Titenko–Holland N, Windham G, Kolachana P, Reinisch F, Parvatham S, Osorio AM, et al. Genotoxicity of malathion in human lymphocytes assessed using the micronucleus assay in vitro and in vivo: a study of malathion-exposed workers. Mutat Res (1997) 388:85–95. doi:10.1016/S1383-5718(96)00140-4
196. Réus GZ, Valvassori SS, Nuernberg H, Comim CM, Stringari RB, Padilha PT, et al. DNA damage after acute and chronic treatment with malathion in rats. J Agric Food Chem (2008) 56:7560–5. doi:10.1021/jf800910q
197. Batzinger RP, Ou SY, Bueding E. Antimutagenic effects of 2(3)-tert-butyl-4-hydroxyanisole and of antimicrobial agents. Cancer Res (1978) 38:4478–85.
198. Hennig UG, Galindo-Prince OC, Cortinas de Nava C, Savage EA, Von Borstel RC. A comparison of the genetic activity of pyrvinium pamoate with that of several other anthelmintic drugs in Saccharomyces cerevisiae. Mutat Res (1987) 187:79–89. doi:10.1016/0165-1218(87)90120-0
199. CDER. Center for Drug Evaluation and Research (CDER). Available from: http://www.fda.gov
200. Connor TH, Stoeckel M, Evrard J, Legator MS. The contribution of metronidazole and two metabolites to the mutagenic activity detected in urine of treated humans and mice. Cancer Res (1977) 37:629–33.
201. Nagasawa HT, Muldoon WP, Shirota FN. Nitramino acids. Synthesis and biological evaluation of 1-nitroproline, 1-nitropipecolic acid, and N-nitrosarcosine. J Med Chem (1977) 20:1588–91. doi:10.1021/jm00222a010
202. Chacko M, Nagabhushan M, Sarkar S, Bhide SV. Studies on the possible mutagenic action of metronidazole. Indian J Exp Boil (1987) 25:240–3.
203. Ehlhardt WJ, Beaulieu BB Jr, Goldman P. Mammalian cell toxicity and bacterial mutagenicity of nitrosoimidazoles. Biochem Pharmacol (1988) 37:2603–6. doi:10.1016/0006-2952(88)90252-3
204. Zeiger E, Anderson B, Haworth S, Lawlor T, Mortelmans K. Salmonella mutagenicity tests: V. Results from the testing of 311 chemicals. Environ Mol Mutagen (1992) 19:2–141. doi:10.1002/em.2850190603
205. Gupta RL, Vats V, Juneja TR. Activation of tinidazole, an antiprotozoal drug to a mutagen by mammalian liver S9. Mutat Res (1996) 370:195–201. doi:10.1016/S0165-1218(96)00076-6
206. Dubini F, Riviera L, Scaltrito MM, Bellotti MG. Susceptibility of Helicobacter pylori and pharmacokinetic properties in mice of new 5-nitroimidazole derivatives devoid of mutagenic activity in the Ames test. J Chemother (1993) 5:168–73. doi:10.1080/1120009X.1993.11739228
207. Grzybowska E, Szala S. Mutagenic activity of two nitroimidazole derivatives in the WP2 repair test. Bull Pol Acad Sci (1985) 33:73–8.
208. Das Roy L, Giri S, Singh S, Giri A. Effects of radiation and vitamin C treatment on metronidazole genotoxicity in mice. Mutat Res (2013) 753:65–71. doi:10.1016/j.mrgentox.2013.02.001
209. Neal SB, Probst GS. Chemically-induced sister-chromatid exchange in vivo in bone marrow of Chinese hamsters, An evaluation of 24 compounds. Mutat Res (1983) 113:33–43.
210. Wulf HC. Sister chromatid exchanges in lymphocytes treated with metronidazole, preliminary communication. Dan Med Bull (1980) 27:38–40.
211. Mudry MD, Carballo M, Labal de Vinuesa M, Gonzalez Cid M, Larripa I. Mutagenic bioassay of certain pharmacological drugs: III. Metronidazole (MTZ). Mutat Res (1994) 305:127–32. doi:10.1016/0027-5107(94)90230-5
212. IARC. IARC Monographs on the Evaluation of the Carcinogenic Risk of Chemicals to Humans. Lyon: IARC (1977). 113 p.
213. Kelly BE, Meikle WD, Moore DE. Enhancement of UV-induced skin carcinogenesis by azathioprine: role of photochemical sensitization. Photochem Photobiol (1989) 49:59–65. doi:10.1111/j.1751-1097.1989.tb04078.x
214. Espinosa-Aguirre JJ, Reyes RE, Cortinas de Nava C. Mutagenic activity of 2-chloro-4-nitroaniline and 5-chlorosalicylic acid in Salmonella typhimurium: two possible metabolites of niclosamide. Mutat Res (1991) 264:139–45. doi:10.1016/0165-7992(91)90131-M
215. Ostrosky-Wegman P, García G, Montero R, Pérez Romero B, Alvarez Chacón R, Cortinas de Nava C. Susceptibility to genotoxic effects of niclosamide in human peripheral lymphocytes exposed in vitro and in vivo. Mutat Res (1986) 173:81–7. doi:10.1016/0165-7992(86)90015-1
216. Gupta RL, Kaur IP, Juneja TR. Genotoxicity of potential metabolites of nitroscanate-an antischistosomal drug. Mutat Res (1995) 335:235–43. doi:10.1016/0165-1161(95)90045-4
217. E-Makawy A, Radwan HA, Ghaly IS, El-Raouf AA. Genotoxical, teratological and biochemical effects of anthelmintic drug oxfendazole maximum residue limit (MRL) in male and female mice. Reprod Nutr Dev (2006) 46:139–56. doi:10.1051/rnd:2006007
218. Stauffert I, Paulini H, Steinmann U, Sippel H, Estler CJ. Investigations on mutagenicity and genotoxicity of pentamidine and some related trypanocidal diamidines. Mutat Res (1990) 245:93–8. doi:10.1016/0165-7992(90)90006-6
219. Mori Y, Hasegawa K, Utsunomiya A, Setsuda S, Goto S, Onodera S, et al. Ames mutagenicity of pyrethroid pesticides (tetramethrin, permethrin, empenthrin, and allethrin) and 1,1’-oxybis(2,3,3,3-tetrachloropropane) as a synergist. Kankyo hen’igen Kenkyu (1998) 20:29–33.
220. Nishi K, Tatsuo N, Nishioka H. DNA damaging capacity and mutagenicity of pyrethroid compounds in E. coli DNA repair and salmonella/microsome tests. Sci Eng Rev Doshisha Univ (1985) 26:93–9.
221. Haworth S. Salmonella mutagenicity test results for 250 chemicals. Environ Mutagen (1983) 5:1–142. doi:10.1002/em.2860050703
222. Kahashi K, Ono H, Yoshida T, Tuboi S. Integration of porin into the outer mitochondrial membrane through the common import machinery located in contact sites. Biochem Mol Biol Int (1993) 29:429–34.
223. Obermeier J, Frohberg H. Mutagenicity studies with praziquantel, a new anthelmintic drug: tissue-, host-, and urine-mediated mutagenicity assays. Arch Toxicol (1977) 38:149–61. doi:10.1007/BF00293649
224. Suling WJ, Struck RF, Woolley CW, Shannon WM. Comparative disposition of phosphoramide mustard and other cyclophosphamide metabolites in the mouse using the Salmonella/mutagenesis assay. Cancer Treat Rep (1978) 62:1321–8.
225. Loprieno N, Barale R, Von Halle ES, Von Borstel RC. Testing of chemicals for mutagenic activity with Schizosaccharomyces pombe. Mutat Res (1983) 115:215–23. doi:10.1016/0165-1110(83)90004-0
226. Machemer L, Lorke D. Mutagenicity studies with praziquantel, a new anthelmintic drug, in mammalian systems. Arch Toxicol (1978) 39:187–97. doi:10.1007/BF00368227
227. Thamavit W, Moore MA, Ruchirawat S, Ito N. Repeated exposure to Opisthorchis viverrini and treatment with the antihelminthic Praziquantel lacks carcinogenic potential. Carcinogenesis (1992) 13:309–11. doi:10.1093/carcin/13.2.309
228. Vijayalaxmi KK, Vishalakshi M. Evaluation of the genotoxic effects of pyrimethamine, an antimalarial drug in the in vivo mouse. Teratog Carcinog Mutagen (2000) 20:65–71. doi:10.1002/(SICI)1520-6866(2000)20:2<65::AID-TCM2>3.0.CO;2-K
229. Tsuda S, Kosaka Y, Matsusaka N, Sasaki YF. Detection of pyrimethamine-induced DNA damage in mouse embryo and maternal organs by the modified alkaline single cell gel electrophoresis assay. Mutat Res (1998) 415:69–77. doi:10.1016/S1383-5718(98)00057-6
230. Sasaki YF, Izumiyama F, Nishidate E, Ohta T, Ono T, Matsusaka N, et al. Simple detection of in vivo genotoxicity of pyrimethamine in rodents by the modified alkaline single-cell gel electrophoresis assay. Mutat Res (1997) 392:251–9. doi:10.1016/S1383-5718(97)00079-X
231. Migliore L, Nieri M. Evaluation of twelve potential aneuploidogenic chemicals by the in vitro human lymphocyte micronucleus assay. Toxicol In Vitro (1991) 5:325–36. doi:10.1016/0887-2333(91)90009-3
232. Ji L, Shanguan XL, Cen JJ, Xu YZ, Liu YL, Xia YE. A mutagenesis, acute and subchronic study of RH-5849. China Acad J Electron Publish House (2005) 36(11).
233. Kafer E, Scott BR, Dorn GL, Stafford R. Aspergillus nidulans: systems and results of tests for chemical induction of mitotic segregation and mutation, I. Diploid and duplication assay systems, a report of the US EPA Gene–Tox Program. Mutat Res (1982) 98:1–48. doi:10.1016/0165-1110(82)90002-1
234. Watanabe–Akanuma M, Ohta T, Sasaki YF. A novel genotoxic aspect of thiabendazole as a photomutagen in bacteria and cultured human cells. Toxicol Lett (2005) 158:213–9. doi:10.1016/j.toxlet.2005.03.013
235. Mudry de Pargament MD, Labal de Vinuesa M, Larripa I. Mutagenic bioassay of certain pharmacological drugs. I. Thiabendazole (TBZ). Mutat Res (1987) 188:1–6. doi:10.1016/0165-1218(87)90107-8
236. Von der Hude W, Kalweit S, Engelhardt G, McKiernan S, et al. In vitro micronucleus assay with Chinese hamster V79 cells – results of a collaborative study with in situ exposure to 26 chemical substances. Mutat Res (2000) 468:137–63. doi:10.1016/S1383-5718(00)00045-0
237. Tada Y, Fujitani T, Yano N, Yuzawa K, Nagasawa A, Yoneyam M. Thiabendazole induces urinary tract toxicity in male ICR mice. Toxicology (2001) 162(1):1–10. doi:10.1016/S0300-483X(00)00460-1
238. Fujii T, Mikuriya H, Sasaki M. Chronic oral toxicity and carcinogenicity study of thiabendazole in rats. Food Chem Toxicol (1991) 29:771–5. doi:10.1016/0278-6915(91)90186-B
239. Ahmed S, Othman OE. Clastogenic effects of the fasciolicide drug fasinex on river buffalo lymphocyte cultures in vitro. Mutat Res (2003) 541:115–21. doi:10.1016/S1383-5718(03)00185-2
240. Kasper P, Uno Y, Mauthe R, Asano N, Douglas G, Matthews E, et al. Follow-up testing of rodent carcinogens not positive in the standard genotoxicity testing battery: IWGT workgroup report. Mutat Res (2007) 627:106. doi:10.1016/j.mrgentox.2006.10.007
241. Benigni R. Analysis of the National Toxicology Program data on in vitro genetic toxicity tests using multivariate statistical methods. Mutagenesis (1989) 4:412–9. doi:10.1093/mutage/4.6.412
242. Zeiger E, Haseman JK, Shelby MD, Margolin BH, Tennant RW. Evaluation of four in vitro genetic toxicity tests for predicting rodent carcinogenicity: confirmation of earlier results with 41 additional chemicals. Environ Mol Mutagen (1990) 16:1–14. doi:10.1002/em.2850160502
243. Zeiger E. Mutagens that are not carcinogens: faulty theory or faulty tests? Mutat Res (2001) 492:29–38. doi:10.1016/S1383-5718(01)00153-X
244. Kirkland DJ, Hayashi M, Jacobson-Kram D, Kasper P, MacGregor JT, Muller L, et al. Summary of major conclusions from the 4th IWGT, San Francisco. Mutat Res (2005) 627:5–9. doi:10.1016/j.mrgentox.2006.08.009
245. Thybaud V, Aardema M, Clements J, Dearfield K, Galloway S, Hayashi M, et al. Strategy for genotoxicity testing: hazard identification and risk assessment in relation to in vitro testing. Mutat Res (2007) 627:41–58. doi:10.1016/j.mrgentox.2006.10.003
246. Müller L, Kikuchi Y, Probst G, Schechtman L, Shimada H, Sofuni T, et al. ICH-harmonised guidances on genotoxicity testing of pharmaceuticals: evolution, reasoning and impact. Mutat Res (1999) 436:195–225. doi:10.1016/S1383-5742(99)00004-6
247. Kirkland DJ, Müller L. Interpretation of the biological relevance of genotoxicity test results: the importance of thresholds. Mutat Res (2000) 464:137–47. doi:10.1016/S1383-5718(99)00175-8
248. Müller L, Kasper P. Human biological relevance and the use of threshold-arguments in regulatory genotoxicity assessment: experience with pharmaceuticals. Mutat Res (2000) 464:19–34. doi:10.1016/S1383-5718(99)00163-1
249. Hilliard CA, Armstrong MJ, Bradt CI, Hill RB, Greenwood SK, Galloway SM. Chromosome aberrations in vitro related to cytotoxicity of non-mutagenic chemicals and metabolic poisons. Environ Mol Mutagen (1998) 31:316–26. doi:10.1002/(SICI)1098-2280(1998)31:4<316::AID-EM3>3.0.CO;2-G
250. Galloway S. Cytotoxicity and chromosome aberrations in vitro: experience in industry and the case for an upper limit on toxicity in the aberration assay. Environ Mol Mutagen (2000) 35:191–201. doi:10.1002/(SICI)1098-2280(2000)35:3<191::AID-EM6>3.0.CO;2-4
251. Parry JM, Parry E, Phrakonkha P, Corvi R. Analysis of published data for top concentration considerations in mammalian cell genotoxicity testing. Mutagenesis (2010) 25:531–8. doi:10.1093/mutage/geq046
252. Tweats DJ, Blakey D, Heflich RH, Jacobs A, Jacobsen SD, Morita T, et al. Report of the IWGT working group on strategies and interpretation of regulatory in vivo tests. I. Increases in micronucleated bone marrow cells in rodents that do not indicate genotoxic hazards. Mutat Res (2007) 627:78–91. doi:10.1016/j.mrgentox.2006.10.005
253. Brambilla G, Martelli A. Genotoxic and carcinogenic risk to humans of drugnitrite interaction products. Mutat Res (2007) 635:17–52. doi:10.1016/j.mrrev.2006.09.003
254. Zeiger E. Identification of rodent carcinogens and non-carcinogens using genetic toxicity tests: premises, promises, and performance. Regul Toxicol Pharmacol (1998) 28:85–95. doi:10.1006/rtph.1998.1234
255. Brambilla G, Mattioli F, Martelli A. Genotoxic and carcinogenic effects of antipsychotics and antidepressants. Toxicology (2009) 261:77–88. doi:10.1016/j.tox.2009.04.056
256. Brambilla G, Mattioli F, Robbiano L, Martelli A. Genotoxicity and carcinogenicity testing of pharmaceuticals: correlations between induction of DNA lesions and carcinogenic activity. Mutat Res (2010) 705:20–39. doi:10.1016/j.mrrev.2010.02.004
257. Cooper JA, Saracci R, Cole P. Describing the validity of carcinogen screening tests. Br J Cancer (1979) 39:87–9. doi:10.1038/bjc.1979.10
258. Brambilla G, Martelli A. Update on genotoxicity and carcinogenicity testing of 472 marketed pharmaceuticals. Mutat Res (2009) 681:209–29. doi:10.1016/j.mrrev.2008.09.002
259. Kirkland D, Aardema M, Muller L, Hayashi M. Evaluation of the ability of a battery of three in vitro genotoxicity tests to discriminate rodent carcinogens and non-carcinogens II. Further analysis of mammalian cell results, relative predictivity and tumour profiles. Mutat Res (2006) 608:29–42. doi:10.1016/j.mrgentox.2006.04.017
260. Trosko JE, Upham BL. The emperor wears no clothes in the field of carcinogen risk assessment: ignored concepts in cancer risk assessment. Mutagenesis (2005) 20:81–92. doi:10.1093/mutage/gei017
261. LeBarona MJ, Rasoulpoura RJ, Klapacza J, Ellis–Hutchings RG, Hollnagel HM, Gollapudi BB. Epigenetics and chemical safety assessment. Mutat Res (2010) 705:83–95. doi:10.1016/j.mrrev.2010.04.003
262. Friedrich A, Olejniczak K. Evaluation of carcinogenicity studies of medicinal products for human use authorised via the European Centralised Procedure (1995–2009). Regul Toxicol Pharmacol (2011) 60:225–48. doi:10.1016/j.yrtph.2011.04.001
Keywords: genotoxicity, carcinogenicity, antiparasitics, risk evaluation, DNA lesions
Citation: Liu Q, Lei Z, Zhu F, Ihsan A, Wang X and Yuan Z (2017) A Novel Strategy to Predict Carcinogenicity of Antiparasitics Based on a Combination of DNA Lesions and Bacterial Mutagenicity Tests. Front. Public Health 5:288. doi: 10.3389/fpubh.2017.00288
Received: 21 March 2017; Accepted: 16 October 2017;
Published: 09 November 2017
Edited by:
Alberto Mantovani, Istituto Superiore di Sanità, ItalyReviewed by:
Iddya Karunasagar, Nitte University, IndiaMonica Catarina Botelho, Istituto Nacional de Saúde, Portugal
Copyright: © 2017 Liu, Lei, Zhu, Ihsan, Wang and Yuan. This is an open-access article distributed under the terms of the Creative Commons Attribution License (CC BY). The use, distribution or reproduction in other forums is permitted, provided the original author(s) or licensor are credited and that the original publication in this journal is cited, in accordance with accepted academic practice. No use, distribution or reproduction is permitted which does not comply with these terms.
*Correspondence: Xu Wang, d2FuZ3h1QG1haWwuaHphdS5lZHUuY24=;
Zonghui Yuan, eXVhbjU4MDJAbWFpbC5oemF1LmVkdS5jbg==