- 1Mosquito Section, Division of Livestock and Human Diseases Vector Control, Tropical Pesticides Research Institute, Arusha, Tanzania
- 2Department of Medical Parasitology and Entomology, Catholic University of Health and Allied Sciences, Mwanza, Tanzania
- 3Centre for Global Health Research, Kenya Medical Research Institute, Kisumu, Kenya
Background: African highlands were known to be free of malaria for the past 50 years. However, the ever growing human population in the highlands of Africa has led to the deforestation and land coverage changes to create space for more land for cultivation, grazing, and house construction materials needs. This has lead to the creation of suitable breeding habitats, which are in open places. Decrease of canopy and forest cover has led to increased temperature both in outdoors and indoors in deforested areas. This increased temperature has resulted in the shortening of developmental stages of aquatic stages of mosquitoes and sporogony development in adult mosquitoes.
Method: Assessment of the effects of deforestation and land coverage changes (decrease), which leads to temperature changes and subsequently increases survivorship of adults and sporogony development in adult mosquitoes’ body was gathered from previous data collected from 2003 to 2012 using different analysis techniques. Habitats productivity, species dynamics and abundance, mosquitoes feeding rates, and sporogony development are presented in relation to temperature changes.
Results: The effects of temperature rise due to land cover changes in highlands of western Kenya on larval developmental rates, adult sporogony developments, and malaria risk in human population were derived. Vector species dynamics and abundance in relation to land use changes have been found to change with time.
Conclusion: This study found that, land cover changes is a key driver for the temperature rise in African highlands and increases the rate of malaria vectors Anopheles gambiae ssp., An. Funestus, and An. arabiensis colonizing the highlands. It has also significantly enhanced sporogony development rate and adult vector survival and therefore the risk of malaria transmission in the highlands.
Introduction
In the recent past, the African highland sites were known to be malaria-free zones. Only sporadic malaria outbreaks were recorded earlier between 1920 and 1950 in the East African highlands (1, 2). However, since 1988, malaria epidemics occurred nearly every year at different sites of African highlands and are a great concern of public health (3–7). These frequent outbreaks have raised concerns that highland malaria has been on the rise (8–12) in the recent past.
The highlands have come under intense pressure from increased population growth. This has increased the demand resulting in tremendous changes in land use practices (13–15). In western Kenya highlands, forests have been cleared to create farmlands, pasture, and homesteads, while swamps have been cultivated to increase agricultural production. These environmental degradation processes, which mainly occur as a result of anthropogenic activities, change the ecological balance and context thus providing suitable habitats for disease vectors to breed, develop, and transmit disease.
Each species occupies a particular ecological niche, and vector species subpopulations are distinct behaviorally and genetically as they adapt to man-made environments (16, 17). However, the major causes of spatial differences in vector distribution and abundance in such heterogeneous environments has not been investigated in the highlands. In lowland areas around Lake Victoria in western Kenya, Minakawa and others detected significant spatial heterogeneity in species composition of anopheline larvae composition in Suba district (18). However, they did not identify environmental variables that determine the occurrence and relative abundance of anopheline larvae.
Several postulates have been put forward to explain increase in malaria epidemics in high altitude areas: land use changes (4, 9, 10), changes in climate (4, 19), and demographic patterns (5, 17). Land cover changes have been suggested to cause increased risks of malaria transmission in high altitude areas in several parts of the world (9, 10, 12, 20). Hoof prints in newly cleared areas form puddles during rains and are conducive for breeding of Anopheles gambiae (18). Replacement of natural swamp vegetation with agricultural crops also lead to increased temperatures, which was suggested to have been responsible for elevated malaria transmission risk in cultivated areas in high altitude areas in Uganda, central Africa, and Madagascar (5, 9). The destruction of tropical forests in west Africa lead to increased risks of malaria transmission and other diseases (17).
Although there is great diversity of anopheline species in the highland areas of Africa members of the An. gambiae complex and Anopheles funestus are the principal vectors of malaria, as is true for most of the continent (21–24). This study explored the effect of deforestation and land cover changes on effect of mosquitoes’ life cycle and infectivity influences.
Materials and Methods
Study Site Location
The study area is situated at Iguhu, a highland area in Kakamega and Vihiga districts of Western Kenya Province (Figure 1). The study sites were located within a grid of 4 × 4 km2 and at 0°17′N, 34°74′E and densely populated with approximately 3000 inhabitants. The area has an average population growth rate of 2.98% per annum. Iguhu was selected as a highland area based on previous work that had been done in the area during 1997/1998 El Nino episode in Kenya (25). Like many highland areas in western Kenya, increase in human settlements has led to rapid changes on land use and land cover. These changes in land use practices continue to create more space to meet other human needs such as pasture fields and farmland.
Estimation of Relative Habitat Productivity (Emerging Adults)
Relative larval habitat productivity in terms of emerging adult mosquitoes was estimated monthly in different land cover types (cultivated and natural swamps, and forest) using collapsible emergence traps (26, 27) monthly from July 2002 to July 2003 and other part on 2008–2010. The traps had a surface area of 1 and 0.5 m2 (Figure 2). The traps were placed over 30 randomly selected habitats (10 habitats in each land cover type) for a period of 7 days while being moved daily within the habitat to maintain dynamics of the habitats. Emerging adults were collected the following day by aspirators, preserved, and identified using morphological keys. Members of the An. gambiae complex were identified by rDNA–PCR (28). Productivity of each land use type was calculated as number of emerging adults per square meter per week. The following habitats parameters were also measured: depth, width, size, canopy cover, algae, grass, and debris cover. Surface area of the larval habitat was calculated as a function of width and length. Canopy cover was measured by taking four readings at right angles at each habitat using a densitometer. Water temperature was recorded using Stowaway tidbit data logger (Onset Computers, MA, USA) at hourly interval.
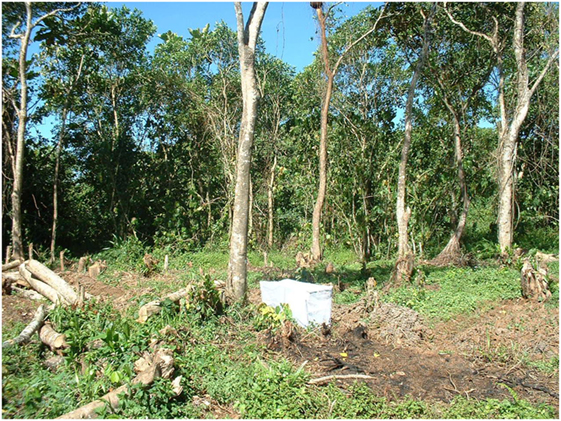
Figure 2. Remnants of indigenous forest undergoing deforestation, in the foreground is a drainage ditch with an emergence trap for estimating relative habitat productivity.
Indoor Microclimate Changes Induced by Land Use and Land Cover
Microclimate is defined as the climate of a very small or restricted area, especially when this differs from the climate of the surrounding area. Houses were selected in the forest and deforested areas of Iguhu and in the lowland site of Kisian, Kisumu. Houses selected in both the highland and lowland areas were those that had corrugated iron roofing. Four houses were selected at each of the three sites for the study. The minimum distance between houses in the forest and deforested areas was about 2.5 km. Houses within the same area (forest, deforested, or the lowland sites) were selected to be over 300 m apart to allow the capture of the microclimate of houses of a larger area within the study sites. Microclimate data were collected using HOBO® data loggers placed inside and outside the houses in Stevenson’s screens all placed 2 m from the ground.
Effects of Land Use and Land Cover on Gonotrophic Cycle Duration
Mosquitoes used in this experiment were An. gambiae from Iguhu, western Kenya. Standard cages of sizes 30 cm × 30 cm × 30 cm containing hundred 4-day-old virgin females of An. gambiae were hanged inside the house. Each site had three houses selected, and inside each a cage was hung. The mosquitoes were fed on human blood for 30 min (a volunteer). Unfed mosquitoes were taken out of the cage. Similar numbers of An. gambiae ssp. males from insectary were introduced in the cage with fully fed females for mating purposes. In 24-h time, each blood-fed and mated female was transferred into a cup for oviposition. Each female was provided with oviposition substrates and placed on the table inside a sleeping room. Each paper cup was covered with polystyrene material. Sugar solution was dispensed using wet cotton placed on top of each cup. The eggs laid in each gonotrophic cycle were counted. Successfully oviposited mosquitoes were given a second blood meal. Microclimate data were collected using HOBO® data loggers placed in the bedrooms of the houses and outside the houses in Stevenson’s screens all placed 2 m from the ground.
Deforestation Effects on the Survivorship and Reproductive Fitness of Anopheles gambiae
Three houses each in the forest and deforested area were used for this study during the dry season, and four houses each were used during the rainy season in all the forest, deforest, and lowland area. Also, 100 newly emerged female and 100 male An. gambiae raised in the insectary in Kisian were placed in a 30 cm × 30 cm × 30 cm metal-framed cage covered with polystyrene netting. Cages were suspended from the roof, 2 m above the ground, in the bedrooms of the selected houses. Human blood from the hand was provided to the mosquitoes for about 15 min every 2 days in the mornings. An oviposition substrate consisting of a petri dish lined with a Whatman filter paper was provided. Sugar solution 10% was dispensed using cotton wool in each cage. Number of eggs laid was counted daily. All dead mosquitoes were taken off the cages. Each cage had a HOBO® data logger to record indoor temperature and humidity.
Data Analysis
Effect of different land use types on larvae abundance was done using one-way analysis of variance (ANOVA) for comparisons of larval abundances in different land use, cropping season, vegetation cover, seasonality, cropping season, and breeding habitat type. Where significant differences were observed, the means were separated by Tukey–HSD test.
Estimation of relative habitat productivity (emerging adults) was analyzed using stepwise multiple logistic regressions. The effect of land use and seasons on the habitat productivity was analyzed by two-way ANOVA. Comparison of the habitats’ productivity between wet and dry seasons was computed by Student’s t-test. Effects of land use and land cover on gonotrophic cycle duration of female mosquitoes and indoor microclimate changes induced by land use and land cover was done using land use cover. The number of eggs laid in each type of land cover and the proportion of mosquitoes that laid eggs was compared using the Chi-square (χ2) test. Non-parametric Kruskal–Wallis rank sum tests were used to determine the effects of land cover (forest vs. deforested) on the mean duration of the gonotrophic cycle of An. gambiae. The analysis of the effect of land cover types on fecundity in different season was done by t-test.
Analysis of variance with repeated measure was used to assess the effects of deforestation on the survivorship and reproductive fitness of An. gambiae in western Kenya highland on outdoor and indoor temperature and relative humidity. The age-dependent mortality comparison was calculated using t-test between forest and deforested and between seasons. The difference was considered significant when the p-value was below 0.05.
Ethics Statement
The Kenya Medical Research Institute, National Ethical Review Committee, and University of California, Irvine ethical review board, approved the ethical clearance for this study under the main project “Ecology of African highland malaria (II), with SSC No. 1382.”
Results
Effect of Different Land Use Types, Seasonality, and Agriculture on Larval Abundance
Vegetation Cover and Larval Abundance
The abundance of plants vegetation cover in habitats influenced significantly An. gambiae s.l. larvae abundance (F15.18, 2, p ≤ 0.001), and other anopheline species and other anopheles species (F3.40, 2, p = 0.034). Mosquito larval abundance associate negatively with increased habitat vegetations, Culicine species (F1.58, 2, p = 0.207), and An. funestus (F2.69, 2, p = 0.069). In grouping all larvae species together, the increase in grass cover associated highly with the decrease of larvae abundance (F15.25, 2, p ≤ 0.001).
Cropping Cycles and Larvae Abundance
In all three crop cycle (land preparation, crops weeding, and plant flowering) An. gambiae s.l. (F8.14, 2, p ≤ 0.001), other anopheline (F12.6, 2, p ≤ 0.001) and Culicines (F21.1, 2, p ≤ 0.001) larvae abundance were significantly different between cropping cycle, while An. funestus (F30.2, 2, p = 0.277) were not significantly different.
Seasonality and Larvae Abundance
In different seasons (rainy, short rainy, and dry seasons) have found that, An. gambiae s.l. had no significant difference (F2.14, 2, p = 0.119), while other anopheline (F13.6, 2, p = 0.003), Culicines (F18.7, 2, p = 0.007), and An. funestus (F6.38, 2, p = 0.046) were statistically different among seasons.
Habitats Types and Larval Abundance
In all habitat types, the larvae abundance was significantly higher in An. gambiae s.l. (F = 2.80, DF = 3, p = 0.004), An. funestus (p ≤ 0.001), other anopheline (p ≤ 0.001), and Culicine (p ≤ 0.001) among the habitats.
Land Use Types and Larval Abundance
Pasture had statistically significant higher abundance of An. gambiae s.l. (F4.23, 1, p = 0.040), other anophelines (F13.44, 1, p ≤ 0.001), and Culicines species (F4.52, 1, p = 0.034) than in farmland, while An. funestus (F0.83, 1, p = 0.363) had no statistically significant differences in larvae abundance between the land use types. In grouping all larvae species together, pasture land use type had significantly higher larval abundance than farmland (F10.05, 1, p = 0.002).
Estimation of Relative Habitat Productivity (Emerging Adults)
The principal malaria vector An. gambiae ssp. were exclusively found to breed in cultivated swamps with the other species of Anopheles squamosus, Anopheles coustani, Anopheles Implexus, and Anopheles maculipalpis being recovered from emergence traps both in the forested and natural swamp habitats. Final model stepwise multiple logistic regression of the productivity and the environmental variables measured did not explain much of the productivity of An. gambiae. Variables such as debris cover land use, which is associated with canopy cover and season, were found to explain in small proportion productivity of this species.
Effects of Land Use and Cover on Gonotrophic Cycle Duration of Female Mosquitoes
Impact of Deforestation on Relative Humidity, Outdoors, and Indoor Temperature
It was found that, temperature outdoor was 0.4°C higher during dry and rainy seasons in deforested area than in forested area. The indoor average temperature was 1.2°C higher in deforested area than forested areas during dry season (F151.04, 1, p < 0.001), while the outdoor temperature variations between forested and deforested area was 0.7°C higher during the rainy season (F20.26, 1, p < 0.001). The average outdoor temperature in the lowland area was 3.1°C higher than the highland deforested area during the rainy season (F225.34, 1, p < 0.001), while the average indoor temperature was 2.8°C higher (F252.95, 1, p < 0.001). Land cover types also affected indoor relative humidity in the highland.
Deforestation Effects on Mosquito Survivorship
The life span of mosquitoes was found to be longer in forested areas than those in deforested areas of western Kenya highlands. During the rainy season, the survival rates were higher in forested area, than in deforested area (χ2 = 73.54, p < 0.001), similar scenario was observed during the dry season (χ2 = 13.11, p < 0.001). The survival period in deforested lowlands area was shorter than in deforested area of the highlands (χ2 = 27.16, p < 0.001).
Effects of Microclimatic Changes Due to Deforestation on the Survivorship and Reproductive Fitness of Anopheles gambiae
Effects of Deforestation on Gonotrophic Cycle Duration
During the dry season, mosquitoes (42.7%) in the forest area laid significantly fewer eggs than those in the deforested area (χ2 = 7.27, DF = 1, p < 0.01). The average duration of the first gonotrophic cycle in the forest area was 4.6 days post blood feeding, 1.7 days (59%) longer than those in the deforested area (2.9 days) (Z = 7.72, p < 0.001). The average duration of the second gonotrophic cycle in the forest area was 3.0 days post second blood meal, 0.9 days (43%) longer than those in the deforested area (Z = 3.62, p < 0.001). The number of eggs laid during first gonotrophic cycle in the rainy season was not statistically different between forested and deforested areas (χ2 = 1.97, p > 0.05). During all the seasons, the gonotrophic cycle was longer in forested area than in deforested area (Z = 3.24, p < 0.001).
Discussion
Understanding the effects of land use, and land cover changes, and succession is important in designing an effective targeted malaria control program. This helps in knowing if a larval habitat is most productive and when it should be targeted for maximum reduction of adult population. Currently, there is growing interest of investing on mosquito larval control and the feasibility of reducing malaria vector populations through environmental management, which has been under investigation in different ecological settings in malaria endemic countries (29–34).
Four larval habitat types in two land use types were studied to understand the variation in larval abundance with relation to crop cycle and land use management. This study has demonstrated dominance of An. gambiae s.l. over other species for 85 weeks of the larval habitats follow-up in both land use and habitat types. The Simpson diversity index model revealed species homogeneity and heterogeneity in different weeks within the study period. In land use type, pasture land use had higher number An. gambiae s.l. larvae abundance than found in farmland as habitats are exposed to sunlight most. These findings have shown similar outcomes as previous studies did in different ecology (13, 24, 35–40). Surveyed larval habitats found that, habitats colonization are species dependant where as An. funestus and Culicines spp. colonized swamps and large open disused goldmines which has been reported by previous studies (13, 24, 35, 40–43). An. gambiae s.l. larvae abundance dominated in hoof print, in small open disused goldmines, and in swamps (13, 24, 35, 40–43).
In farmland, the different cropping cycles’ habitats were dominated by An. gambiae s.l. larvae abundance and reduced toward plant flowering season, which is contrary to what other studies found in farmlands that plants’ flowering was the cycle associated with the abundance of An. gambiae s.l. in the habitats (44–47). The findings of this study suggest that, the food sources in larval habitats in farmlands are more complex and larvae might have other source of food apart of flowering maize pollens. Hence, the prediction of the food sources for mosquito larvae in this study could not be ascertained by cropping cycles alone. In nature, larvae habitats have been found to have varieties of food sources to support different mosquito species larvae at a point of time (44–47). In different agro-ecosystem it was found that nitrogenous fertilizers’ applications lowers the turbidity of water, which is correlated and proved to associate with the abundance of mosquito larvae (48–52). Studies by McCrae found that, the An. gambiae s.l. gravid females prefer to oviposit on turbid water rather than on clear water (53). The lower larval density in early short rainy and in long rainy seasons might be attributed and explained with unexpected flush effect (washing of eggs and larvae from habitats), which was similar with the other studies in western Kenya (24, 54).
The Knowledge of estimation of relative larval habitat productivity and survivorship of An. gambiae would be important in planning and implementing larval control measures in high altitude areas in western Kenya. The current study has demonstrated the effect of land cover on larval habitat productivity (emerging adults) and survivorship at a highland area in western Kenya. We have demonstrated that land cover affects the productivity of and larval survivorship of Anopheles gambaie. These results confirm the hypothesis that land cover changes in western Kenya highlands alter larval breeding habitat conditions and increases larval survivorship thus accelerating the development rate. Reducing canopy cover may significantly increase productivity of larval breeding habitats in two ways: one is by increasing the availability of open and sunlit pools, which are preferred by An. gambiae and two by accelerating the development rate and increasing the survivorship. This may be critical for malaria transmission and may partly explain the upsurge in outbreaks of malaria in the high altitude areas in western Kenya. There were seasonal variations in productivity of An. Gambiae, which were likely to have been caused by the amount of rainfall. Higher productivity was recorded during months of high rainfall. These findings are similar to previous studies (55) in rainfall and coincided with abundance of adult mosquitoes. However during the period between September and December 2003, an increase in rainfall did not show a corresponding increase in productivity of An. gambiae in the cultivated swamps. During this period, grass cover in these drainage ditches in the cultivated swamps had increased, and habitats may have become unsuitable for breeding of An. gambiae even though rainfall levels seemed high to sustain production. At the same time, the dry season marked the end of growing season in the cultivated swamps when the drainage ditches are unattended to by the farmers. Flush effect of rains is also likely to have washed away the larvae. An increase in productivity for the period beginning February to July 2003 may be attributed to clearing of the drainage ditches in the cultivated swamps in preparation for the growing season by the local inhabitants. This may have created suitable breeding habitats for An. gambiae. Results of stepwise multiple regression indicated that canopy cover, maximum and minimum temperature, and algae cover affect the productivity of this malaria vector in western Kenya highland. Canopy cover however is highly linked to land cover as forests have higher canopy cover, making the environmental inimical to breeding of this species. Habitats in the open areas receive a lot of sunshine and are likely to have abundant larval food resources such as bacteria and algae (56). Unlike habitats in the open areas, habitats in forest and natural swamp had an average canopy cover of up to 92–97% as estimated by densitometer and visual estimates, respectively. In such shaded habitats, algal growth is likely to be minimal (57). Thus growth and survivorship of An. gambiae larvae is likely to be retarded. In the current study, higher productivity of An. gambiae was associated with algae (23, 24). Our results are consistent with previous findings in lowland and highland areas in western Kenya in which occurrence of An. gambiae and Anopheles arabiensis were associated with small, temporary habitats with algae and little aquatic vegetation (36, 42). In the natural conditions, these results of larval habitat productivity may have implications for malaria transmission in western Kenya highlands. Maize is the staple food grown in cultivated swamps, An. gambiae larvae may feed on pollen grains and thus emerge as bigger adults with higher vectorial capacity and increase the risk of malaria transmission to a community with low immunity. Previous observations in Ethiopia (58) have demonstrated that larvae of An. arabiensis, which fed on pollen grains emerged as larger adults and are capable of imbibing more blood, properties that would be crucial for malaria transmission in the high altitude areas. Also, this study has revealed that, there is an increase in An. arabiensis to 33.7% from formerly reported 3.5% in An. gambiae sibling species (24), which was implicated by the land use changes and deforestation.
Our results on survivorship of An. gambiae larvae under different land cover types in western Kenya must be the first to do so. In the current study, larvae of this malaria vector survived much better in the open areas compared to other land cover type. Density and land cover significantly affected survivorship of mosquito larvae. Larvae in the forested and natural swamp habitats took longer to develop into pupae in the artificial habitats in which larvae were provided with food. In their study, Gimnig et al. (59) suggested that implications for extending development time may include larvae being stranded as the habitat dries up. This suggests that the changes in land cover types in the area may increase the development time and size of mosquitoes. The current study has clearly demonstrated that land cover and density can impact the survivorship and growth of An. gambiae under artificial conditions. This may be critical for malaria transmission in this area of unstable malaria. It has been suggested that though density-dependent processes can regulate populations of An. gambiae under artificial conditions, it is not known how important these factors can be in natural conditions (59).
The effects of land use and land cover on gonotrophic cycle duration of female mosquitoes demonstrated that, the average ambient temperature in the deforested area in western Kenya highlands was about 0.5°C higher than the forest area over a 10-month period. The average indoor temperature of houses located in the deforested area was 1.8 and 1.2°C higher than those inside the forest area during the dry and rainy seasons, respectively. The relative humidity in the deforested area fluctuated substantially, while the humidity in the forest area was more stable. As a consequence of the indoor temperature increase, the average duration of the first gonotrophic cycle of An. gambiae was shorter in both seasons for deforested than forested.
There was significant association between gonotrophic cycle and indoor temperature that could make clear that, temperature is the driving force for the gonotrophic time. The findings suggest that, an increase of temperature by 1°C would decrease the gonotrophic cycle of An. gambiae indoors. Similar results have been found by Stern and others that, when temperature increases in highlands of Kericho, more malaria incidences were expected as the effect of better mosquito developmental; conditions including gonotrophic cycle (60).
The results of this study are consistent with those of earlier studies using the mark–release–recapture technique. Rodriguez and others carried out studies on the pacific coast of Mexico and found the gonotrophic cycle of An. albimanus to be 4 days for nulliparous mosquitoes and 2 days for parous mosquitoes (61), while Charlwood et al. reported durations of 2.4–3.2 days for An. koliensis, 2.7–3.7 for An. punctulatus, and 2.1–3.0 days for An. farauti in Papua New Guinea (62). The capture–mark–release–recapture technique is unsuitable for study of the gonotrophic cycle duration in the highlands due to low vector densities, which would result in extremely low recapture rates.
The daily man-biting rates affect the two indices used to measure malaria transmission: that is, the vectorial capacity and the entomological inoculation rate (EIR) (63). The vectorial capacity varies as a square of the daily man-biting rates, and EIR varies linearly with the daily man-biting rates. Simple calculations indicated that during the dry season, deforestation caused a net change of the daily man-biting rate from 0.22 to 0.33, which represents a 50% increase in transmission as would be indicated by EIR. However, during the rainy season, the effects of deforestation are lower as the daily biting rate increased from 0.1 to 0.12, representing a 20% increase in transmission assuming all other transmission variables remain the same in the two areas. During the main transmission period, as a result of longer durations of the gonotrophic cycle, the average daily man-biting rate in the highlands was 44% lower compared to the lowlands.
The effect of deforestation on the microclimatic have affected the survivorship and reproductive fitness of An. gambiae, it was demonstrated that in the increase in malaria vector reproductive rate was associated with increased temperature due to deforestation and land clearance. Changes in both microclimatic conditions and vector biology depend on land cover types and vary between seasons. Blood meal digestion rate have been found directly proportional to the temperature (64). In addition, lower humidity in houses located in the deforested area may have negatively affected mosquito survival because An. gambiae mosquitoes are adapted to humid environment (21, 65). Although mosquitoes in the highland deforested area exhibited a reduced survivorship, they showed a significantly higher net reproductive rate and per-capita intrinsic growth rate than those in the forest area. This may primarily be due to the higher fecundity and shorter generation time exhibited by mosquitoes in the deforested area. A combination of sugar and blood feeding in mosquitoes seems to affect their longevity (66).
The results presented here have implications for understanding the effect of land cover changes on malaria transmission in African highlands. Earlier studies found that deforestation can increase larval productivity, accelerate larval development time, and increase larval pupation rate as well as adult emergence rate (40). In this study, it was found that adult survival time was shorter in the deforested area than in the forest area but the net reproductive rate was greater in the deforested area than that in the forest area. Putting them all together, it was found that deforestation can, first, greatly reduce vector generation time, thus, there will be more generations of vector in this area; second, increase reproductive rate together with increased larval–pupa survival rate, thus more offspring will survive to produce even more larvae; more generations and more offspring will greatly enhance the vector population. Furthermore, increased temperatures as a result of deforestation can shorten the gonotrophic cycle length, increase biting rates, and fasten the sporogonic development of the Plasmodium parasite in mosquitoes (64). In terms of malaria transmission, this could result in a great increase in the vectorial capacity and, consequently, of malaria transmission.
Conclusion
This study concludes that, the land cover changes and deforestation are the main drivers for the temperature rise in African highlands and escalates the rate of potential malaria vectors An. gambiae ssp., An. funestus, and An. arabiensis colonizing the highlands. It is also enhancing significantly sporogony development rate and adult vector survivorship and therefore malaria transmission risk in the highlands.
Author Contributions
EJK and SM conceived and designed the experiments, performed the experiments, and analyzed the data. EJK, SM, and EEK wrote the paper and also read and approved the final manuscript.
Conflict of Interest Statement
The authors declare that the research was conducted in the absence of any commercial relationships that could be construed as a potential conflict of interest.
Acknowledgments
Authors wish to thank the community of Iguhu village for their initial support to get this research started. We also thank the community members for their understanding and having allowed us to work in their farms during the larval sampling. We are grateful to the field assistants, Wilberforce Muyeso, Josephine Shikholwa (RIP), and Amos Wabwile, a project technician, for their tireless support in the field and laboratory. Drs. Andrew Githeko and Afrane Asare Yaw are highly appreciated for logistics, human resource supervision, and project activities coordination. Dr. Afrane Asare Yaw is appreciated for conducting experiments on microclimate monitoring, gonotrophic cycles. and mosquito feeding. This paper is published with the permission of the Director, Kenya Medical Research Institute (KEMRI).
Funding
This work received financial support from American National Institute of Health (D43 TW01505 and R01 AI-50243). The funding body had no role in the study design, data collection and analysis, decision to publish, or preparation of the manuscript.
References
2. Roberts J. The control of epidemic malaria in the highlands of western Kenya. Part II. The campaign. J Trop Med Hyg (1964) 67(8):191–9.
3. Midekisa A, Beyene B, Mihretie A, Bayabil E, Wimberly MC. Seasonal associations of climatic drivers and malaria in the highlands of Ethiopia. Parasit Vectors (2015) 8(1):1–11. doi:10.1186/s13071-015-0954-7
4. Himeidan YE-S, Kweka E. Malaria in east African highlands during the past 30 years: impact of environmental changes. Front Physiol (2012) 3:315. doi:10.3389/fphys.2012.00315
5. Mouchet J, Manguin S, Sircoulon J, Laventure S, Faye O, Onapa AW, et al. Evolution of malaria in Africa for the past 40 years: impact of climatic and human factors. J Am Mosq Control Assoc (1998) 14(2):121–30.
6. Protopopoff N, Van Herp M, Maes P, Reid T, Baza D, D’Alessandro U, et al. Vector control in a malaria epidemic occurring within a complex emergency situation in Burundi: a case study. Malar J (2007) 6(1):1–9. doi:10.1186/1475-2875-6-93
7. Pascual M, Ahumada JA, Chaves LF, Rodó X, Bouma M. Malaria resurgence in the East African highlands: temperature trends revisited. Proc Natl Acad Sci U S A (2006) 103(15):5829–34. doi:10.1073/pnas.0508929103
8. Lindblade KA, O’Neill DB, Mathanga DP, Katungu J, Wilson ML. Treatment for clinical malaria is sought promptly during an epidemic in a highland region of Uganda. Trop Med Int Health (2000) 5(12):865–75. doi:10.1046/j.1365-3156.2000.00651.x
9. Lindblade KA, Walker ED, Onapa AW, Katungu J, Wilson ML. Land use change alters malaria transmission parameters by modifying temperature in a highland area of Uganda. Trop Med Int Health (2000) 5(4):263–74. doi:10.1046/j.1365-3156.2000.00551.x
10. Lindblade KA, Walker ED, Wilson ML. Early warning of malaria epidemics in African highlands using Anopheles (Diptera: Culicidae) indoor resting density. J Med Entomol (2000) 37(5):664–74. doi:10.1603/0022-2585-37.5.664
11. Maïga-Ascofaré O, Rakotozandrindrainy R, Girmann M, Hahn A, Randriamampionona N, Poppert S, et al. Molecular epidemiology and seroprevalence in asymptomatic Plasmodium falciparum infections of Malagasy pregnant women in the highlands. Malar J (2015) 14(1):1–9. doi:10.1186/s12936-015-0704-5
12. Malakooti MA, Biomndo K, Shanks GD. Reemergence of epidemic malaria in the highlands of western Kenya. Emerg Infect Dis (1998) 4(4):671–6. doi:10.3201/eid0404.980422
13. Munga S, Minakawa N, Zhou G, Mushinzimana E, Barrack OO, Githeko AK, et al. Association between land cover and habitat productivity of malaria vectors in western Kenyan highlands. Am J Trop Med Hyg (2006) 74(1):69–75.
14. Minakawa N, Munga S, Atieli F, Mushinzimana E, Zhou G, Githeko AK, et al. Spatial distribution of anopheline larval habitats in Western Kenyan highlands: effects of land cover types and topography. Am J Trop Med Hyg (2005) 73(1):157–65.
15. Munga S, Yakob L, Mushinzimana E, Zhou G, Ouna T, Minakawa N, et al. Land use and land cover changes and spatiotemporal dynamics of anopheline larval habitats during a four-year period in a highland community of Africa. Am J Trop Med Hyg (2009) 81(6):1079–84. doi:10.4269/ajtmh.2009.09-0156
16. Patz JA, Graczyk TK, Geller N, Vittor AY. Effects of environmental change on emerging parasitic diseases. Int J Parasitol (2000) 30(12–13):1395–405. doi:10.1016/S0020-7519(00)00141-7
17. Mouchet J, Carnevale P. Impact of environmental changes on vector-borne diseases. Sante (1997) 7:263–70.
18. Minakawa N, Mutero CM, Githure JI, Beier JC, Yan G. Spatial distribution and habitat characterization of anopheline mosquito larvae in Western Kenya. Am J Trop Med Hyg (1999) 61(6):1010–6.
19. Githeko AK, Lindsay SW, Confalonieri UE, Patz JA. Climate change and vector-borne diseases: a regional analysis. Bull World Health Organ (2000) 78(9):1136–47.
20. Lindsay SW, Bodker R, Malima R, Msangeni HA, Kisinza W. Effect of 1997-98 El Nino on highland malaria in Tanzania. Lancet (2000) 355(9208):989–90. doi:10.1016/S0140-6736(00)90022-9
21. Lindsay SW, Martens WJ. Malaria in the African highlands: past, present and future. Bull World Health Organ (1998) 76(1):33–45.
22. Kweka EJ, Kamau L, Munga S, Lee MC, Githeko AK, Yan G. A first report of Anopheles funestus sibling species in western Kenya highlands. Acta Trop (2013) 128(1):158–61. doi:10.1016/j.actatropica.2013.06.006
23. Kweka EJ, Zhou G, Munga S, Lee MC, Atieli HE, Nyindo M, et al. Anopheline larval habitats seasonality and species distribution: a prerequisite for effective targeted larval habitats control programmes. PLoS One (2012) 7(12):e52084. doi:10.1371/journal.pone.0052084
24. Kweka EJ, Zhou G, Lee MC, Gilbreath TM, Mosha F, Munga S, et al. Evaluation of two methods of estimating larval habitat productivity in western Kenya highlands. Parasit Vectors (2011) 4:110. doi:10.1186/1756-3305-4-110
25. Githeko AK, Ayisi JM, Odada PK, Atieli FK, Ndenga BA, Githure JI, et al. Topography and malaria transmission heterogeneity in western Kenya highlands: prospects for focal vector control. Malar J (2006) 5(1):1–9. doi:10.1186/1475-2875-5-107
26. Karanja D, Githeko A, Vulule J. Small-scale field evaluation of the monomolecular surface film ‘Arosurf MSF’ against Anopheles arabiensis Patton. Acta Trop (1994) 56(4):365–9. doi:10.1016/0001-706X(94)90107-4
27. WHO. Manual on Practical Entomology in Malaria. Part II. Methods and Techniques. Geneva, Switzerland: World Health Organization (1975).
28. Scott JA, Brogdon WG, Collins FH. Identification of single specimens of the Anopheles gambiae complex by the polymerase chain reaction. Am J Trop Med Hyg (1993) 49(4):520–9.
29. Chaki PP, Govella NJ, Shoo B, Hemed A, Tanner M, Fillinger U, et al. Achieving high coverage of larval-stage mosquito surveillance: challenges for a community-based mosquito control programme in urban Dar es Salaam, Tanzania. Malar J (2009) 8:311. doi:10.1186/1475-2875-8-311
30. Fillinger U, Lindsay SW. Suppression of exposure to malaria vectors by an order of magnitude using microbial larvicides in rural Kenya. Trop Med Int Health (2006) 11(11):1629–42. doi:10.1111/j.1365-3156.2006.01733.x
31. Fillinger U, Sonye G, Killeen GF, Knols BG, Becker N. The practical importance of permanent and semipermanent habitats for controlling aquatic stages of Anopheles gambiae sensu lato mosquitoes: operational observations from a rural town in western Kenya. Trop Med Int Health (2004) 9(12):1274–89. doi:10.1111/j.1365-3156.2004.01335.x
32. Geissbuhler Y, Kannady K, Chaki PP, Emidi B, Govella NJ, Mayagaya V, et al. Microbial larvicide application by a large-scale, community-based program reduces malaria infection prevalence in urban Dar es Salaam, Tanzania. PloS One (2009) 4(3):e5107. doi:10.1371/journal.pone.0005107
33. Mwangangi JM, Shililu J, Muturi EJ, Muriu S, Jacob B, Kabiru EW, et al. Anopheles larval abundance and diversity in three rice agro-village complexes Mwea irrigation scheme, central Kenya. Malar J (2010) 9:228. doi:10.1186/1475-2875-9-228
34. Yasuoka J, Levins R. Impact of deforestation and agricultural development on anopheline ecology and malaria epidemiology. Am J Trop Med Hyg (2007) 76(3):450–60.
35. Minakawa N, Omukunda E, Zhou G, Githeko A, Yan G. Malaria vector productivity in relation to the highland environment in Kenya. Am J Trop Med Hyg (2006) 75(3):448–53.
36. Minakawa N, Sonye G, Mogi M, Yan G. Habitat characteristics of Anopheles gambiae s.s. larvae in a Kenyan highland. Med Vet Entomol (2004) 18(3):301–5. doi:10.1111/j.0269-283X.2004.00503.x
37. Minakawa N, Sonye G, Yan G. Relationships between occurrence of Anopheles gambiae s.l. (Diptera: Culicidae) and size and stability of larval habitats. J Med Entomol (2005) 42(3):295–300. doi:10.1093/jmedent/42.3.295
38. Munga S, Minakawa N, Zhou G, Barrack OO, Githeko AK, Yan G. Oviposition site preference and egg hatchability of Anopheles gambiae: effects of land cover types. J Med Entomol (2005) 42(6):993–7. doi:10.1093/jmedent/42.6.993
39. Munga S, Minakawa N, Zhou G, Githeko AK, Yan G. Survivorship of immature stages of Anopheles gambiae s.l. (Diptera: Culicidae) in natural habitats in western Kenya highlands. J Med Entomol (2007) 44(5):758–64. doi:10.1603/0022-2585(2007)44[758:SOISOA]2.0.CO;2
40. Tuno N, Okeka W, Minakawa N, Takagi M, Yan G. Survivorship of Anopheles gambiae sensu stricto (Diptera: Culicidae) larvae in western Kenya highland forest. J Med Entomol (2005) 42(3):270–7. doi:10.1603/0022-2585(2005)042[0270:SOAGSS]2.0.CO;2
41. Gillies TM, Coetzee M. Supplement of the Anopheles of Africa South of Sahara (Afrotropical Region). Johannesburg: Republic of South Africa Publication of the South African Institute for Medical Research (1987).
42. Gimnig JE, Ombok M, Kamau L, Hawley WA. Characteristics of larval anopheline (Diptera: Culicidae) habitats in Western Kenya. J Med Entomol (2001) 38(2):282–8. doi:10.1603/0022-2585-38.2.282
43. Mutuku FM, Bayoh MN, Gimnig JE, Vulule JM, Kamau L, Walker ED, et al. Pupal habitat productivity of Anopheles gambiae complex mosquitoes in a rural village in western Kenya. Am J Trop Med Hyg (2006) 74(1):54–61.
44. Kebede A, McCann JC, Kiszewski AE, Ye-Ebiyo Y. New evidence of the effects of agro-ecologic change on malaria transmission. Am J Trop Med Hyg (2005) 73(4):676–80.
45. Ye-Ebiyo Y, Pollack RJ, Kiszewski A, Spielman A. A component of maize pollen that stimulates larval mosquitoes (Diptera: Culicidae) to feed and increases toxicity of microbial larvicides. J Med Entomol (2003) 40(6):860–4. doi:10.1603/0022-2585-40.6.860
46. Ye-Ebiyo Y, Pollack RJ, Kiszewski A, Spielman A. Enhancement of development of larval Anopheles arabiensis by proximity to flowering maize (Zea mays) in turbid water and when crowded. Am J Trop Med Hyg (2003) 68(6):748–52.
47. Ye-Ebiyo Y, Pollack RJ, Spielman A. Enhanced development in nature of larval Anopheles arabiensis mosquitoes feeding on maize pollen. Am J Trop Med Hyg (2000) 63(1–2):90–3.
48. Mutero CM, Ng’ang’a PN, Wekoyela P, Githure J, Konradsen F. Ammonium sulphate fertiliser increases larval populations of Anopheles arabiensis and culicine mosquitoes in rice fields. Acta Trop (2004) 89(2):187–92. doi:10.1016/j.actatropica.2003.08.006
49. Muturi EJ, Mwangangi J, Shililu J, Muriu S, Jacob B, Kabiru E, et al. Mosquito species succession and physicochemical factors affecting their abundance in rice fields in Mwea, Kenya. J Med Entomol (2007) 44(2):336–44. doi:10.1093/jmedent/44.2.336
50. Mwangangi J, Shililu J, Muturi E, Gu W, Mbogo C, Kabiru E, et al. Dynamics of immature stages of Anopheles arabiensis and other mosquito species (Diptera: Culicidae) in relation to rice cropping in a rice agro-ecosystem in Kenya. J Vector Ecol (2006) 31(2):245–51. doi:10.3376/1081-1710(2006)31[245:DOISOA]2.0.CO;2
51. Mwangangi JM, Muturi EJ, Shililu J, Muriu SM, Jacob B, Kabiru EW, et al. Survival of immature Anopheles arabiensis (Diptera: Culicidae) in aquatic habitats in Mwea rice irrigation scheme, central Kenya. Malar J (2006) 5:114. doi:10.1186/1475-2875-5-114
52. Victor TJ, Reuben R. Effects of organic and inorganic fertilisers on mosquito populations in rice fields of southern India. Med Vet Entomol (2000) 14(4):361–8. doi:10.1046/j.1365-2915.2000.00255.x
53. McCrae AW. Oviposition by African malaria vector mosquitoes. II. Effects of site tone, water type and conspecific immatures on target selection by freshwater Anopheles gambiae Giles, sensu lato. Ann Trop Med Parasitol (1984) 78(3):307–18.
54. Paaijmans KP, Wandago MO, Githeko AK, Takken W. Unexpected high losses of Anopheles gambiae larvae due to rainfall. PLoS One (2007) 2(11):e1146. doi:10.1371/journal.pone.0001146
55. Grillet ME. Factors associated with distribution of Anopheles aquasalis and Anopheles oswaldoi (Diptera: Culicidae) in a malarious area, northeastern Venezuela. J Med Entomol (2000) 2:231–8. doi:10.1603/0022-2585-37.2.231
56. Merrit RW, Dadd RH, Walker ED. Feeding behaviour, natural food and nutritional relationships of larval mosquitoes. Annu Rev Entomol (1992) 37:349–76. doi:10.1146/annurev.en.37.010192.002025
57. Rejmankova E, Harbin-Ireland A, Lege M. Bacterial abundance in larval habitats of four species of Anopheles (Diptera: Culicidae) in Belize, Central America. J Vector Ecol (2000) 25(2):229–38.
58. Yemane Y, Pollack RJ, Spielman A. Enhanced development in nature of larval Anopheles arabiensis mosquitoes feeding on maize pollen. Am J Trop Med Hyg (2000) 63:90–3.
59. Gimnig JE, Ombok M, Otieno S, Kaufman MG, Vulule JM, Walker ED. Density-dependent development of Anopheles gambiae (Diptera: Culicidae) larvae in artificial habitats. J Med Entomol (2002) 39(1):162–72. doi:10.1603/0022-2585-39.1.162
60. Stern DI, Gething PW, Kabaria CW, Temperley WH, Noor AM, Okiro EA, et al. Temperature and malaria trends in highland East Africa. PLoS One (2011) 6(9):e24524. doi:10.1371/journal.pone.0024524
61. Rodriguez MH, Chavez B, Orozco A, Loyola EG, Martinez-Palomo A. Scanning electron microscopic observations of Anopheles albimanus (Diptera: Culicidae) eggs. J Med Entomol (1992) 29(3):400–6. doi:10.1093/jmedent/29.5.887
62. Charlwood J, Graves P, Birley M. Capture-recapture studies with mosquitoes of the group of Anopheles punctulatus Dönitz (Diptera: Culicidae) from Papua New Guinea. Bull Entomol Res (1986) 76(2):211–27. doi:10.1017/S000748530001470X
63. Garrett-Jones C, Shidrawi G. Malaria vectorial capacity of a population of Anopheles gambiae: an exercise in epidemiological entomology. Bull World Health Organ (1969) 40(4):531.
64. Afrane YA, Lawson BW, Githeko AK, Yan G. Effects of microclimatic changes caused by land use and land cover on duration of gonotrophic cycles of Anopheles gambiae (Diptera: Culicidae) in Western Kenya Highlands. J Med Entomol (2005) 42(6):974–80. doi:10.1093/jmedent/42.6.974
65. White G. Anopheles gambiae complex and disease transmission in Africa. Trans R Soc Trop Med Hyg (1974) 68(4):278–98. doi:10.1016/0035-9203(74)90035-2
Keywords: highland, Anopheles gambiae ssp., habitat productivity, land use, topography, temperature, gonotrophic cycle
Citation: Kweka EJ, Kimaro EE and Munga S (2016) Effect of Deforestation and Land Use Changes on Mosquito Productivity and Development in Western Kenya Highlands: Implication for Malaria Risk. Front. Public Health 4:238. doi: 10.3389/fpubh.2016.00238
Received: 07 May 2016; Accepted: 12 October 2016;
Published: 26 October 2016
Edited by:
Mohiuddin Md. Taimur Khan, Washington State University, USAReviewed by:
Robert Canales, University of Arizona, USAManoj M. Veleeparambil, Cleveland Clinic Lerner College of Medicine, USA
Copyright: © 2016 Kweka, Kimaro and Munga. This is an open-access article distributed under the terms of the Creative Commons Attribution License (CC BY). The use, distribution or reproduction in other forums is permitted, provided the original author(s) or licensor are credited and that the original publication in this journal is cited, in accordance with accepted academic practice. No use, distribution or reproduction is permitted which does not comply with these terms.
*Correspondence: Eliningaya J. Kweka, kwekae@tpri.or.tz