- 1Medical Imaging College, North Sichuan Medical College, Nanchong, China
- 2Department of Radiology, The Third Hospital of Mianyang, Sichuan Mental Health Center, Mianyang, China
- 3Department of Neurosurgery, The Third Hospital of Mianyang, Sichuan Mental Health Center, Mianyang, China
- 4Department of Psychiatry, The Third Hospital of Mianyang, Sichuan Mental Health Center, Mianyang, China
Background: Previous studies based on resting-state functional magnetic resonance imaging(rs-fMRI) and voxel-based morphometry (VBM) have demonstrated significant abnormalities in brain structure and resting-state functional brain activity in patients with early-onset schizophrenia (EOS), compared with healthy controls (HCs), and these alterations were closely related to the pathogenesis of EOS. However, previous studies suffer from the limitations of small sample sizes and high heterogeneity of results. Therefore, the present study aimed to effectively integrate previous studies to identify common and specific brain functional and structural abnormalities in patients with EOS.
Methods: The PubMed, Web of Science, Embase, Chinese National Knowledge Infrastructure (CNKI), and WanFang databases were systematically searched to identify publications on abnormalities in resting-state regional functional brain activity and gray matter volume (GMV) in patients with EOS. Then, we utilized the Seed-based d Mapping with Permutation of Subject Images (SDM-PSI) software to conduct a whole-brain voxel meta-analysis of VBM and rs-fMRI studies, respectively, and followed by multimodal overlapping on this basis to comprehensively identify brain structural and functional abnormalities in patients with EOS.
Results: A total of 27 original studies (28 datasets) were included in the present meta-analysis, including 12 studies (13 datasets) related to resting-state functional brain activity (496 EOS patients, 395 HCs) and 15 studies (15 datasets) related to GMV (458 EOS patients, 531 HCs). Overall, in the functional meta-analysis, patients with EOS showed significantly increased resting-state functional brain activity in the left middle frontal gyrus (extending to the triangular part of the left inferior frontal gyrus) and the right caudate nucleus. On the other hand, in the structural meta-analysis, patients with EOS showed significantly decreased GMV in the right superior temporal gyrus (extending to the right rolandic operculum), the right middle temporal gyrus, and the temporal pole (superior temporal gyrus).
Conclusion: This meta-analysis revealed that some regions in the EOS exhibited significant structural or functional abnormalities, such as the temporal gyri, prefrontal cortex, and striatum. These findings may help deepen our understanding of the underlying pathophysiological mechanisms of EOS and provide potential biomarkers for the diagnosis or treatment of EOS.
Introduction
Schizophrenia is a severe psychiatric disorder characterized by high disabling and progressive development, and the main clinical symptoms are positive symptoms (e.g. hallucinations, delusions, etc.), negative symptoms (e.g. affective apathy, impoverished thinking, etc.), and cognitive impairment (1). The World Health Organization (WHO) reported that schizophrenia affected approximately 24 million people or 1 in 300 people (0.32%) worldwide in 2022 (2). Schizophrenia has become a major public health problem worldwide, causing serious harm to patients and their families, as well as a heavy economic burden on society and government. Specifically, compared with the general population, patients with schizophrenia have a 2.08 times increased risk of death (3), and their mean life expectancy is shortened by about 15 years (4). On the other hand, patients with schizophrenia often suffer from social exclusion and human rights violations, which may extend to their family members (5). In addition, the combined direct and indirect economic burden of schizophrenia in the United States exceeded $340 billion (6). As mentioned above, the dangers of schizophrenia are obvious, over the past decades, despite extensive basic and clinical studies on schizophrenia by many researchers, the pathophysiological mechanisms of schizophrenia remain unclear, and effective treatments are still lacking. In response to the above problems, many researchers have put forward different hypotheses in an attempt to reveal the pathophysiological mechanisms of schizophrenia, among which the widely accepted view is that schizophrenia is related to alterations in the neural developmental trajectory of the brain due to abnormalities in the action of genetic, environmental and other factors (7). Early-onset schizophrenia (EOS) is a subgroup of schizophrenia that is defined as the first onset of symptoms of schizophrenia before the age of 18. EOS accounts for approximately 5% of all schizophrenia cases and is characterized by higher genetic susceptibility, more atypical and severe symptomatology, and poorer therapeutic response to antipsychotic medications compared with adult-onset schizophrenia (8–10). Notably, EOS is less affected by potential confounders such as antipsychotic medications, life events, and the social environment (11). Therefore, conducting studies on patients with EOS provides a unique perspective on schizophrenia research and may be helpful in the exploration of the pathophysiological mechanisms of schizophrenia.
With the development of neuroimaging techniques, resting-state functional magnetic resonance imaging (rs-fMRI) and voxel-based morphometry (VBM) have been widely used in the studies of functional and structural brain abnormalities in patients with EOS. Rs-fMRI can be used to detect the spontaneous activity of neurons in the brain when subjects are not performing any specific task or receiving any external stimulation, and it can effectively avoid instability caused by subjects performing specific tasks (12–14). Hence, rs-fMRI provides a powerful tool for the exploration of resting-state regional functional brain activity in patients with EOS. The alterations of resting-state regional functional brain activity can be represented by indicators such as amplitude of low-frequency fluctuation (ALFF), fractional ALFF (fALFF), regional homogeneity (ReHo) and cerebral blood flow (CBF). Among which, ALFF indirectly reflects regional functional brain activity by measuring the total power of blood oxygenation level-dependent (BOLD) signals in the low frequency range (15). fALFF reflects the relative contribution of oscillations in the low frequency range to the overall detectable frequency range (16). ReHo is an assessment of the synchronization between a given voxel and a neighboring voxel time-series through the consistency of the Kendall’s coefficient of concordance (17). In addition, CBF can be quantified by fMRI, single-photon emission computed tomography (SPECT) and positron emission tomography (PET) using the arterial spin labelling (ASL) technique (18). Previous studies based on the above methodologies had identified significant abnormalities in resting-state regional functional brain activity in patients with EOS versus healthy controls (HCs). However, there was high heterogeneity in these results. For example, some studies have reported significantly increased resting-state regional functional brain activity in prefrontal cortex (PFC) in patients with EOS (19–21), however, other studies have observed significant decreased resting-state functional activity in PFC (22, 23). In addition, it has been identified that patients with EOS have both regions of increased and decreased resting-state regional functional brain activity in the PFC (24).
In addition to the abnormalities in resting-state functional brain activity, previous studies had revealed significant anatomical abnormalities in patients with EOS.VBM is an automated whole-brain technique capable of assessing regional grey matter volume (GMV) alterations without bias (25). GMV, as a structural marker that is relatively stable over time, can reflect to a certain extent the structural basis behind alterations in functional brain activity (26). Similarly, there were discrepancies in the results of the VBM studies. For instance, some previous VBM studies shown decreased regional GMV in the inferior temporal gyrus in patients with EOS (27–29), yet another study reported increased GMV in the inferior temporal gyrus in patients with EOS (30). The inconsistency in the results of the VBM and rs-fMRI studies described above may be attributed to the limited sample size of the studies, the heterogeneity of the study methodology (e.g., different inclusion and exclusion criteria, different methods of data analysis, etc.), and the heterogeneity of the study subjects (e.g., different duration of the disease, severity of the disease, and medication status, etc.).
Hence, the present study aimed to explore the most reliable and consistent structural and functional brain abnormalities in EOS by systematically reviewing and effectively integrating previous studies on resting-state regional functional brain activity and GMV conducted on EOS patients. Alterations in functional brain activity and structural abnormalities are closely related, so another aim of the present study was to explore whether there is a corresponding structural basis for brain regions with abnormal resting-state functional brain activity in patients with EOS. In brief, the present study began with separate meta-analysis of rs-fMRI studies and VBM studies, followed by multimodal overlapping on this basis. We then conducted a validation subgroup meta-analysis after excluding uncorrected for statistics to test the robustness of the results of the main meta-analyses. We also performed subgroup meta-analyses of the rs-fMRI studies and the VBM studies, respectively, to investigate whether there are different functional and structural abnormalities in patients with EOS in different disease statuses. Specifically, two subgroup meta-analyses were included (studies of patients with first-episode EOS and studies of patients with drug-naive EOS). Finally, we conducted meta-regression analyses to explore potential associations of clinical variables (age, sex, years of education, duration of illness, and symptom severity scores) with the results of the main meta-analyses. Based on the evidence from previous studies, we speculated that the brain regions in which EOS patients develop abnormalities in GMV and resting-state regional functional brain activity are mainly located in the PFC, temporal gyrus and striatum.
Methods
Literature search
This study followed the Preferred Reporting Items for Systematic Reviews and Meta-Analyses (PRISMA) guidelines (31). The current meta-analysis was registered with PROSPERO (registration number: CRD42024544361). In the present study, we conducted a systematic and comprehensive search for studies on VBM and rs-fMRI in patients with EOS published in PubMed, Web of Science, Embase, Chinese National Knowledge Infrastructure (CNKI) and WanFang databases through April 30, 2024, combined with the following keywords:(“schizophrenia” OR “schizophrenics” OR “schizophrenic disorder”) AND (“functional magnetic resonance imaging” OR “fMRI” OR “resting-state” OR “amplitude of low-frequency fluctuation” OR “ALFF” OR “fractional ALFF” OR “fALFF” OR “regional homogeneity” OR “ReHo” OR “cerebral blood flow” OR “CBF” OR “positron emission tomography” OR “PET” OR “single photon emission computed tomography” OR “SPECT” OR “arterial spin labeling” OR “ASL” OR “voxel-based morphometry” OR “gray matter” OR “VBM”) AND (“adolescent” OR “child” OR “early-onset”). In addition, the references of the included studies and relevant review literature were examined to avoid the omission of other relevant studies.
Study selection
Studies were included in the meta-analysis if they satisfied the following criteria: (1) the study was original (rather than a review or abstract, etc.) that was peer-reviewed for publication in English or Chinese language journal; (2) the study subjects were formally diagnosed with schizophrenia before the age of 18 according to DSM, ICD or other criteria; (3) they analyzed resting-state functional brain activity or GMV at the whole-brain level; (4) they compared regional resting-state functional brain activity or GMV between patients with EOS and HCs; (5) peak coordinates based on whole-brain analysis were reported in three-dimensional stereotactic coordinates [Talairach or Montreal Neurological Institute (MNI)]; (6) If the study was a longitudinal or intervention trial, only baseline data were included for analysis.
Exclusion criteria were: (1) patients with EOS were diagnosed with comorbid neurological or other psychiatric disorders; (2) they had fewer than 10 samples in a single group; (3) three-dimensional stereotactic coordinates of the peak of the activation point were unavailable, even after contacting the corresponding author by email or telephone; (4) the baseline data were unavailable; (5) the full-text could not be accessed; (6) If the data of different studies partially or completely overlapped, only the study with larger sample sizes and higher quality were included.
Data extraction
For each included study, we extracted the following information: (1) peak coordinates and effect values (e.g., t-values, etc.) of brain regions significantly different between patients with EOS and HCs; (2) the demographic and clinical characteristics, including sample size, gender, mean age, years of education, diagnostic criteria, medication status, duration of the illness, and PANSS scores; and (3) the imaging characteristics, including MRI scanner, method of analysis, data processing and analysis software used, the full width at half maximum (FWHM) parameter of the smoothing kernel, slice thickness and statistical thresholds used in brain imaging preprocessing.
Quality assessment
We used a 10-point checklist based on previous meta-analyses to assess the quality of each study included in the current meta-analysis (32–34), which consisted of three modules including demographic and clinical characteristics of patients and HCs, methods of image acquisition and analysis, and quality of results and conclusions. Specifically, scores of 0, 0.5, and 1 were assigned to each item based on whether the criteria were not satisfied, partially satisfied, or fully satisfied, with a total score of no more than 10 (Supplementary Table S1). The literature search, study selection, data extraction, and quality assessment were performed independently by two researchers (Lu Wang and Ruishan Liu). Any discrepancies were resolved jointly by the third and fourth researchers (Hongwei Li and Lihua Zhuo) for a final decision.
Data analyses
Voxel-wise meta-analyses for functional and structural differences
We performed separate meta-analyses of brain regions with significant differences in resting-state regional functional brain activity (i.e., ALFF, fALFF, ReHo, and CBF) and structure (i.e., gray matter volume) between patients with EOS and HCs, using the Seed-based d Mapping with Permutation of Subject Images (SDM-PSI, version 6.23) (https://www.sdmproject.com/) software following standard procedures. The procedures have been described in detail in previous studies (35, 36). Briefly, we initially created the corresponding text for each study and then entered the peak coordinates and effect sizes of the extracted brain regions that were significantly different between patients with EOS and HCs into the corresponding text files. If the effect sizes reported in the original studies were p-values or Z-values, we could convert them to t-values using the SDM online converter. Meanwhile, if the coordinates reported in Talairach space could be uniformly transformed to MNI space by matrix transformation so that all peak coordinates were in the same normalized space. We then recreated the standardized MNI-based effect size maps (Hedges’ effect size) of contrast results for each dataset separately using an anisotropic non-normalized Gaussian kernel. Next, the mean maps were computed using the random-effects model, weighted by sample size, intra-study variability, and between-study heterogeneity, and multiple imputations were pooled using Rubin’s rules. Finally, the maps were visualized by MRIcron software (www.mricro.com/mricron/). In addition, the meta-analyses were conducted with the default Gaussian kernel size and thresholds of the SDM-PSI software [i.e., FWHM = 20 mm, peak height Z > 1, p < 0.005 (uncorrected), cluster extent > 10 voxels], which have been validated to balance false positives and false negatives optimally and to be approximate to the corrected results (37–39).
Multimodal meta-analysis
We overlapped the thresholded meta-analytic results-maps of resting-state regional functional brain activity and brain GMV alterations to localize brain regions presenting abnormalities both at the functional and structural level in patients with EOS (i.e., examine the convergence of the results from different modalities). It is worth noting that in the multimodal analysis, we used a more stringent probability threshold (i.e., p < 0.0025) (40).
Subgroup meta-analyses
In the current study, to verify the reliability of the results of the main meta-analyses, we conducted a validation subgroup analysis after excluding studies with uncorrected statistics. Furthermore, to explore the structural and functional changes in the brain of EOS patients in different statuses, we performed the following two subgroup analyses (1): the studies were conducted in patients with drug-naive EOS; (2) the studies were conducted in patients with first-episode EOS. The same thresholds were applied as for the subgroup meta-analyses [i.e., peak height Z > 1, cluster range > 10 voxels, p < 0.005 (uncorrected)].
Analyses of jackknife sensitivity, heterogeneity, and publication bias
In the present study, we used the whole-brain voxel-based Jackknife sensitivity analysis, i.e. iteratively repeating the same analysis after excluding one dataset at a time to evaluate the stability and reproducibility of the main meta-analyses results (41, 42). If an abnormal brain region remains significant in all or most studies, the result is considered highly reproducible and stable. We used the I2 statistic to assess between-study heterogeneity of the results, with I2 < 50% commonly indicating low heterogeneity (43). In addition, to evaluate potential publication bias, in the present study, funnel plots were created for visual inspection, and publication bias was quantified by Egger’s test. Significant publication bias was considered to exist if the p-value < 0.05 of Egger’s test and the funnel plots were asymmetric (44, 45).
Meta-regression analyses
To explore the potential impact of clinical variables (including mean age, percentage of females, years of education, illness duration, and PANSS scores) on the results of the meta-analyses, linear regression analyses were performed in the current study within the EOS. We applied the more conservative thresholds (i.e., p < 0.0005 and cluster extent > 10 voxels) recommended by previous studies to minimize the reporting of spurious relationships (46, 47).
Results
Included studies and sample characteristics
The flow chart for identifying and excluding studies is shown in Figure 1. Through a systematic and comprehensive search and review, we finally included 12 studies (13 datasets) for resting-state regional functional brain activity and 15 studies (15 datasets) for GMV. The total sample sizes of the functional meta-analysis were 891 subjects, including 496 EOS patients (57.06% females, mean age =14.81 years) and 395 HCs (55.44% females, mean age =14.74 years), and there was no statistically significant difference between the two groups on sex (χ2= 0.778, p=0.378) or age (t=0.172, p=0.865). In patients with EOS, the mean duration of illness was 5.74 months (range: 1.50-9.60 months), 86.49% were drug-naive patients, and 83.87% were first-episode patients. In addition, the mean quality score of the included studies was 9.04 (range:7.5-10.0).
The total sample sizes of the structural meta-analysis were 989 subjects, including 458 EOS patients (50.22% females, mean age =16.30 years) and 531 HCs (46.14% females, mean age =16.11 years), and there was no statistically significant difference between the two groups on sex (χ2 = 1.639, p=0.200) or age (t=0.177, p=0.861). In patients with EOS, the mean duration of illness was 5.76 months (range: 1.20-16.00 months), 50.66% were drug-naive patients, and 77.29% were first-episode patients. Furthermore, the mean quality score of the included studies was 8.80 (range:7.0-9.5). The demographic and clinical characteristics, imaging characteristics, and quality scores of the included studies are presented in Supplementary Tables S2, S3.
Main voxel-wise meta-analyses
In the functional meta-analysis, resting-state functional brain activity was significantly increased in the left middle frontal gyrus (extending to the triangular part of the left inferior frontal gyrus) and the right caudate nucleus in patients with EOS, compared with HCs. However, no brain regions with significantly decreased resting-state brain functional activity were observed (Figure 2, Supplementary Table S4).
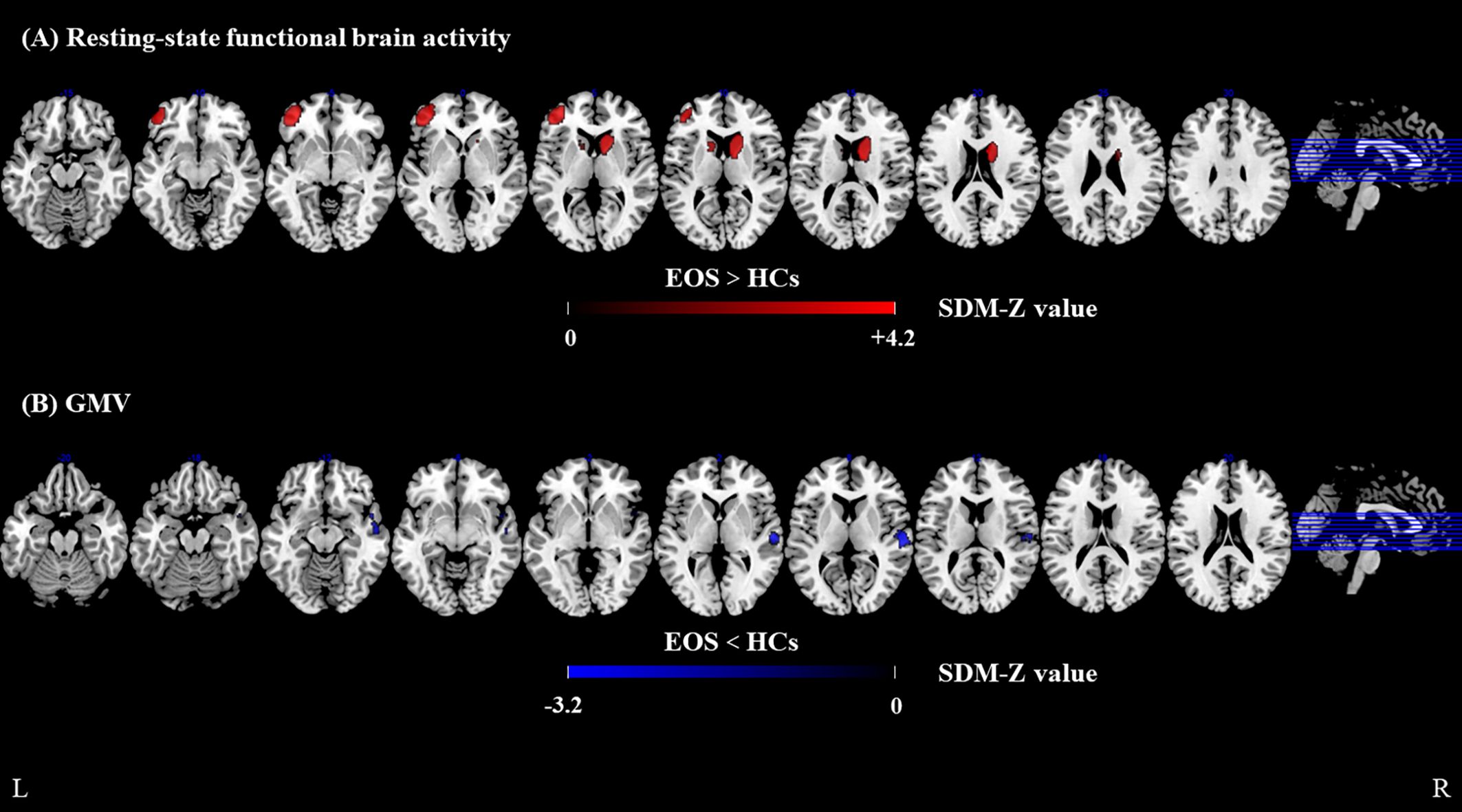
Figure 2. Meta-analyses results of difference between EOS and HCs. (A) resting-state regional functional activity difference between EOS and HCs, (B) GMV difference between EOS and HCs. Regions with decreased resting-state regional functional activity or GMV are displayed in blue, and regions with increased resting-state regional functional activity or GMV are displayed in red. The color bar indicates the maximum and minimum SDM-Z values. EOS, early-onset schizophrenia; HCs, healthy controls; SDM, Seed-based d mapping; GMV, gray matter volume.
In the structural meta-analysis, GMV was significantly decreased in the right superior temporal gyrus (extending to the right rolandic operculum), the right middle temporal gyrus, and the right temporal pole (superior temporal gyrus) in patients with EOS, compared with HCs. No significantly increased GMV was observed in patients with EOS (Figure 2, Supplementary Table S5).
Multimodal meta-analysis
Brain regions with conjoint alterations in resting-state functional brain activity and GMV were not observed in patients with EOS, compared with HCs.
Subgroup meta-analyses
First, we performed subgroup analyses after excluding studies of uncorrected for statistics. To be specific, for resting-state functional brain activity analysis (10 datasets), compared with HCs, patients with EOS showed significantly increased resting-state functional brain activity in the left middle frontal gyrus (extending to the orbital part of the left inferior frontal gyrus), and right caudate nucleus, while resting-state functional brain activity was significantly decreased in the left superior temporal gyrus (Supplementary Table S6, Supplementary Figure S1). For GMV analysis (12 datasets), GMV was significantly decreased in the right superior temporal gyrus in patients with EOS, compared with HCs (Supplementary Table S7, Supplementary Figure S1).
Subgroup analyses of patients with first-episode EOS revealed that compared with HCs, patients with first-episode EOS had significantly increased resting-state regional functional brain activity in the triangular part of the left inferior frontal gyrus (extending to the left middle frontal gyrus) and significantly decreased in the left postcentral gyrus (Supplementary Table S8, Supplementary Figure S2). However, no brain regions with significantly increased or decreased GMV were observed between patients with first-episode EOS and HCs.
In addition, Subgroup analyses of patients with drug-naive EOS indicated that patients with drug-naive EOS had significantly increased resting-state regional functional brain activity in the left middle frontal gyrus (extending to the triangular part of the left inferior frontal gyrus and the orbital part of the left inferior frontal gyrus) and right caudate nucleus, compared with HCs (Supplementary Table S9, Supplementary Figure S2). Likewise, no brain regions with significant increased or decreased GMV were observed in patients with drug-naive EOS.
Analyses of jackknife sensitivity, heterogeneity, and publication bias
The jackknife sensitivity analysis revealed high reliability and reproducibility of the results of the functional and structural meta-analysis. In the functional meta-analysis, jackknife sensitivity analysis showed that alterations (i.e., decreased resting-state functional brain activity) in the left middle frontal gyrus (extending to the triangular part of the inferior frontal gyrus) and the right caudate nucleus remained significant in all combinations. For the structural meta-analysis, jackknife sensitivity analysis indicated that the most robust data was the decreased GMV in the right superior temporal gyrus (extending to the right rolandic operculum), which could be cross-validated in at least 13 of all 15 datasets. In addition, the other results were relatively robust, in which the right middle temporal gyrus could be cross-validated in 8 of the 15 data sets, and the right temporal pole could be cross-validated in 7 of the 15 data sets.
In both functional and structural meta-analyses, no significant between-study heterogeneity (I2 < 50%) or publication bias (Egger’s test, p > 0.05) was observed in all brain regions with significant abnormal alterations (Supplementary Tables S4, S5).
Meta-regression analyses
Meta-regression analyses demonstrated that there were no significant linear associations between abnormal alterations in resting-state regional functional brain activity or GMV and clinical variables (including age, percentage of females, years of education, illness duration, and disease severity scores) in patients with EOS.
Discussion
To our knowledge, this is the first multimodal neuroimaging meta-analysis of EOS patients applying the SDM-PSI meta-analysis method. The main findings of the present study are as follows (1): compared with HCs, patients with EOS had significantly increased resting-state regional functional brain activity in the left middle frontal gyrus (extending to the triangular part of the left inferior frontal gyrus) and the right caudate nucleus; (2) the brain regions with significantly decreased GMV in patients with EOS were mainly located in the right superior temporal gyrus (extending to the rolandic operculum), the right middle temporal gyrus, and the right temporal pole (superior temporal gyrus). (3) In the multimodal meta-analysis, we failed to identify brain regions with conjoint abnormalities in resting-state regional functional brain activity and GMV in patients with EOS; (4) The results of jackknife sensitivity analyses, heterogeneity analyses, and validation subgroup analyses showed relatively high reliability and reproducibility of the results of the main meta-analyses; (5) Meta-regression analyses showed no significant linear associations between the major structural and functional alterations in EOS patients and age, gender, years of education, duration of illness, or severity of disease.
In the functional meta-analysis, we identified a significantly increased regional spontaneous brain activity in the left middle frontal gyrus in patients with EOS, which was consistent with the results of the previous studies (48, 49). The middle frontal gyrus is one of the important constituent brain regions of the PFC, which is closely related to working memory (50). Previous studies have shown that working memory processes are often impaired in patients with schizophrenia and their unaffected first-degree relatives (51–53). In addition, abnormal activation patterns in the middle frontal gyrus are frequently associated with attentional control (54), and the top-down attentional control processes of patients with schizophrenia are likewise particularly susceptible to being impaired (55). On the other hand, neural activity in the left middle frontal gyrus was significantly increased in patients with schizophrenia during the processing of fearful faces (56). Athanassiou et al. reported that abnormal activation of the left middle frontal gyrus in suicidal patients with schizophrenia also during emotional processing tasks (57), and significant cortical thinning of the PFC was also observed in suicidal patients with schizophrenia (58), suggesting that suicidal behaviors in patients with schizophrenia may be related to the abnormal anatomical structure and neural activity of the PFC. In summary, the PFC is involved in the regulation of decision-making and executive control, and in appropriate behaviors by integrating feeling and emotional information (59, 60). The hyperactivation of functional activity in the PFC found in the present study we speculate may be due to a functional compensatory neural mechanism that the organism develops after cognitive, emotional processing and behavioral processes are impaired in patients with EOS, and genetic factors may also influence some of these processes. However, it is noteworthy that some studies reported significantly decreased regional spontaneous functional activity in the PFC in patients with EOS (23, 61). The possible reasons for this discrepancy are: (i) the irregular involvement of the PFC may not be fully explained by an explicit failure of function in this region, but perhaps reflects a dysfunction in the processes of cognitive control, affective processing, and behavioral regulation themselves; (ii) heterogeneity of the samples, moderating variables, and performance on the task (62).
The triangular part of the inferior frontal gyrus belongs to Broca’s area, the area where the motor speech center is located and is closely related to the processing of verbal information and the production of discourse (63, 64). Patients with schizophrenia often suffer from extensive language disorders (65). Previous studies revealed that abnormal activation of the left inferior frontal gyrus was negatively correlated with increased use of transitive verbs in continuous speech in patients with schizophrenia (66). It was also found that patients with schizophrenia had hyperactivation of the left inferior frontal gyrus during a speech task (67). In addition, a significantly increased spontaneous neural activity in the triangular part of the left inferior frontal gyrus was positively correlated with the polygenic risk score (PRS) in patients with non-chronic schizophrenia, and furthermore, glutamatergic-related genes involved in synaptic organization and transmission were found to be highly enriched in the PRS-schizophrenia genes (68). A previous study revealed that glutamatergic neurotransmission may play an important role in regulating speech processing and production (69). On the other hand, the left inferior frontal gyrus of young people at family high risk (FHR) for schizophrenia also showed hyperenhancement due to semantic associations (70). Moreover, significantly increased ReHo in the left inferior frontal gyrus showed high sensitivity and specificity in distinguishing treatment-resistant schizophrenia (TRS) from non-treatment-resistant schizophrenia (NTRS) (71). Previous studies also revealed that a significantly increased functional activity in the triangular part of the left inferior frontal gyrus also played an important role in auditory verbal hallucinations (AVH) in the schizophrenia (72). Besides that, a meta-analysis also showed that a significant decreased in GMV in the left inferior frontal gyrus was associated with the development of the AVH (73). In the present study, we identified a significantly increased regional spontaneous neural activity in the triangular part of the left inferior frontal gyrus in patients with EOS, which is consistent with previous studies, suggesting that abnormal neural activity in this region may be involved in the pathogenesis of EOS, which may lead to language deficits and hallucinations and may be a potential biomarker for distinguishing among TRS, NTRS, and HCs and for predicting neurobiological risk of the schizophrenia.
In addition, we also identified the presence of abnormally increased spontaneous neural activity in the right caudate nucleus in patients with EOS, which was consistent with previous findings in patients with adult schizophrenia (74, 75). The caudate nucleus is an important component of the striatum, the vast majority of which is involved in constituting the dorsal striatum, while its ventral portion is one of the components of the ventral striatum. Several studies suggested that striatum dysfunction played a central role in the pathophysiological mechanisms of schizophrenia (76–78). The striatum mainly received dopaminergic neural projections from the midbrain (especially the substantia nigra and ventral tegmental area (79). Previous studies showed that striatal dopamine synthesis and release were significantly enhanced in patients with schizophrenia and were associated with positive symptoms of schizophrenia (80, 81). And the increased volume of the striatum may be the structural basis for the hyperfunction of the striatal dopaminergic system in the schizophrenia (82). Sorg et al. revealed that increased intrinsic neural activity in the striatum of patients with schizophrenia corresponded to symptom dimensions and disorder states, and further identified a potential link between intrinsic neural activity in the striatum and signaling in the dopamine pathway (83). An animal study also found that intrinsic neural activity in the striatum was modulated by dopamine levels (84). In addition, abnormalities of spontaneous functional activity in the striatum may cause dysfunction of the frontal cortex-thalamus-striatum-midbrain circuit, leading to imbalances in the signaling of neurotransmitters, such as dopamine, which can reduce the signal-to-noise ratio of neural activity and impair cortical and basal ganglia function resulting in the development of psychotic symptoms (85, 86). However, it is worth noting that direct evidence of the association of intrinsic neural activity in the striatum with dopamine signaling is still lacking, which awaits further studies in the future. Our findings similarly indicated that striatal dysfunction may play a key role in the pathogenesis of schizophrenia and provided evidence for the EOS aspect of the schizophrenia dopamine hypothesis.
In the structural meta-analysis, the present study revealed that compared with HCs, the regions with significantly decreased GMV in patients with EOS were mainly located in the right superior temporal gyrus (extending to the right rolandic operculum), the right middle temporal gyrus, and the right temporal pole (superior temporal gyrus). superior temporal gyrus plays a crucial role in auditory information processing, language processing and auditory memory (87). Several previous studies showed a strong association between GMV changes in superior temporal gyrus and auditory verbal hallucinations (AVH) (88–90). To be specific, Zhang et al. reported a negative correlation between decreased GMV in superior temporal gyrus and the severity of AVH symptoms (91). Some studies also revealed functional sub-regions of the superior temporal gyrus, e.g. Heschl’s gyrus (primary auditory cortex) and planum temporale were similarly altered, and further found that both were associated with AVH and delusional behaviors in the schizophrenia (92–94). In addition, in the present study, we also identified that GMV abnormalities in the right superior temporal gyrus extended to the right rolandic operculum. The rolandic operculum is located in the frontal lobe and also plays a role in auditory feedback processing, suggesting that decreased GMV in the right rolandic operculum may be associated with abnormal auditory feedback processing behind AVH (95). On the other hand, previous VBM studies in adult schizophrenia patients with first-episode and antipsychotic-naive also revealed significantly decreased GMV in the right superior temporal gyrus (96, 97), suggesting that GMV alterations in the right superior temporal gyrus may be a stable biomarker for schizophrenia less susceptible to time of onset. A structural meta-analysis showed that a significantly decreased GMV in the right superior temporal gyrus was similarly found in a population at high risk of clinical psychosis (and later converted to psychosis) (98). Moreover, decreased GMV in superior temporal gyrus was strongly associated with the severity of negative and psychotic symptoms in schizophrenia patients (99). However, unfortunately, the present study didn’t find a significant association (at least not linearly) between GMV alterations and schizophrenia symptoms severity, which may be explained by the fact that most of the patients with EOS included in the present study were first-episode patients (with a relatively short duration of illness), the relatively small sample size or the inherent limitations of cross-sectional studies as opposed to longitudinal studies (i.e., additional timepoints per subject may strengthen the observed associations) (100). Previous studies also identified a positive correlation between GMV alterations in the right superior temporal gyrus and the PANSS total scores reduction ratio in patients with schizophrenia after treatment with antipsychotic medication (101, 102), suggesting that GMV alterations in the right superior temporal gyrus may be a potential biomarker for predicting the efficacy of schizophrenia drug therapy. Furthermore, significant abnormalities in GMV of the right temporal pole (superior temporal gyrus) were found in the present study. The temporal pole is mainly involved in the integration of facial information processing and emotional information processing (103). Previous studies indicated lower GMV in the temporal pole region in schizophrenia patients with a history of violent behaviors compared to schizophrenia patients without a history of violent behaviors (104). Therefore, we hypothesized that decreased GMV in the temporal pole in schizophrenia may contribute to a higher risk of violence by disturbing the process of integration of facial and emotional information processing.
The middle temporal gyrus is also one of the vulnerable brain regions in patients with schizophrenia (105), and the middle temporal gyrus plays a key role in language comprehension, semantic reasoning, and integrating information from different semantic systems (106, 107). Thus, patients with schizophrenia often have extensive social cognitive deficits, especially in interpersonal communication (108). In this study, we found that patients with EOS also presented with a GMV deficit in the middle temporal gyrus, which was similar to the results of previous studies (109, 110). Moreover, it was also found that both patients with schizophrenia and their first-degree unaffected siblings had significantly decreased regional GMV in the left middle temporal gyrus relative to HCs and that the mean GMV in the left middle temporal gyrus was a good predictor in distinguishing patients/siblings from HCs (111). Another study identified a correlation between genetically determined IL-6 levels and decreased GMV in the middle temporal gyrus (112). Furthermore, the association of decreased GMV in the right middle temporal gyrus with the Val158Met polymorphism in the COMT gene was also reported (113, 114). The above-mentioned studies suggested that decreased GMV in the middle temporal gyrus may be a potential endophenotype of schizophrenia with some heritability and specificity. In addition, decreased GMV in the middle temporal gyrus was associated with a poor prognosis in the schizophrenia (115).
Limitation
The present study had some limitations. First, the present meta-analysis was based on the peak coordinates and effect sizes of significantly abnormal brain regions reported in the original studies rather than the original statistical maps, which resulted in the loss of some information and may have decreased the accuracy of the results of the present study to some extent. Second, the majority of subjects included in the present study were from the Chinese population, which somewhat limited the generalizability of the results to other populations. Third, because the vast majority of the included studies were cross-sectional and we included only baseline data for longitudinal studies, we were not able to determine whether alterations in brain anatomical structure and function were part of the pathogenesis of EOS or a consequence of the disease. Fourth, since the original studies included in the present study were limited and didn’t meet the criteria for subgroup analysis, we didn’t perform a subgroup analysis of the different functional imaging methods in the rs-fMRI studies, and more high-quality studies will need to be included in the future to explore the impact of functional imaging methods on the results of the main meta-analyses. Furthermore, we conducted separate subgroup analyses of first-episode EOS patients and drug-naive EOS patients which indicated that alterations of resting-state regional functional brain activity in patients with EOS seemed to be related not to the time of onset, or the medication status, but to the disease itself. Finally, Finally, in the subgroup analyses, some of the results were inconsistent with those of the main meta-analyses, and we failed to identify brain regions with significantly increased or decreased regional GMV either in the subgroup analysis of patients with first-episode EOS or in the subgroup analysis of patients with drug-naive EOS, possibly due to the presence of some heterogeneities that were not captured by the present meta-analysis (e.g., type of antipsychotic medication used, etc.), the limited inclusion of the sample sizes, the between-subjects heterogeneity, and methodological heterogeneity among the included studies (e.g., scanning methods and parameters, methods of data processing and analysis, etc.). Hence, in the future, studies with larger and more homogeneous samples are needed to further validate the findings of the present study.
Conclusion
In summary, EOS showed significant abnormalities in resting-state functional brain activity in the left middle frontal gyrus (extending to the triangular part of the left inferior frontal gyrus) and the right caudate nucleus, and significant gray matter structural deficits in the right superior temporal gyrus (extending to the right rolandic operculum), the right middle temporal gyrus, and the right temporal pole (superior temporal gyrus). These findings may contribute to our more comprehensive understanding of the underlying pathophysiological mechanisms of schizophrenia and provide potential biomarkers for the diagnosis, differential diagnosis and treatment of schizophrenia.
Data availability statement
The original contributions presented in the study are included in the article/Supplementary Material. Further inquiries can be directed to the corresponding authors.
Author contributions
LW: Writing – original draft, Software, Methodology, Investigation, Formal analysis, Conceptualization. RL: Writing – review & editing, Validation, Investigation, Data curation. JL: Writing – review & editing, Validation, Investigation, Data curation. XX: Writing – review & editing, Validation, Software, Investigation. LX: Writing – review & editing, Software, Investigation. WW: Writing – review & editing, Software, Project administration. JQL: Writing – review & editing, Investigation, Formal analysis. FZ: Writing – review & editing, Investigation, Data curation. LZ: Writing – review & editing, Supervision, Resources, Methodology, Funding acquisition, Conceptualization. HL: Writing – review & editing, Resources, Methodology, Funding acquisition, Conceptualization.
Funding
The author(s) declare financial support was received for the research, authorship, and/or publication of this article. This study was supported by the National Key Research and Development Program of China (No. 2022YFC2009901/2022YFC2009900) and the Chengdu Municipal Science and Technology Program of China (No. 2022-YF09-00062-SN).
Conflict of interest
The authors declare that the research was conducted in the absence of any commercial or financial relationships that could be construed as a potential conflict of interest.
Publisher’s note
All claims expressed in this article are solely those of the authors and do not necessarily represent those of their affiliated organizations, or those of the publisher, the editors and the reviewers. Any product that may be evaluated in this article, or claim that may be made by its manufacturer, is not guaranteed or endorsed by the publisher.
Supplementary material
The Supplementary Material for this article can be found online at: https://www.frontiersin.org/articles/10.3389/fpsyt.2024.1465758/full#supplementary-material
References
1. McCutcheon RA, Reis Marques T, Howes OD. Schizophrenia-an overview. JAMA Psychiatry. (2020) 77:201–10. doi: 10.1001/jamapsychiatry.2019.3360
2. WHO. Schizophrenia (2022). Accessed 10 January 2022. Available online at: https://www.who.int/news-room/fact-sheets/detail/schizophrenia. (Accessed January 10, 2022)
3. Hayes JF, Marston L, Walters K, King MB, Osborn DPJ. Mortality gap for people with bipolar disorder and schizophrenia: uk-based cohort study 2000-2014. Br J psychiatry: J Ment Sci. (2017) 211:175–81. doi: 10.1192/bjp.bp.117.202606
4. Hjorthøj C, Stürup AE, McGrath JJ, Nordentoft M. Years of potential life lost and life expectancy in schizophrenia: A systematic review and meta-analysis. Lancet Psychiatry. (2017) 4:295–301. doi: 10.1016/s2215-0366(17)30078-0
5. Faden J, Citrome L. Schizophrenia: one name, many different manifestations. Med Clinics North America. (2023) 107:61–72. doi: 10.1016/j.mcna.2022.05.005
6. Kadakia A, Catillon M, Fan Q, Williams GR, Marden JR, Anderson A, et al. The economic burden of schizophrenia in the United States. J Clin Psychiatry. (2022) 83(6):22m14458. doi: 10.4088/JCP.22m14458
7. Wahbeh MH, Avramopoulos D. Gene-environment interactions in schizophrenia: A literature review. Genes. (2021) 12(12):1850. doi: 10.3390/genes12121850
8. Ioakeimidis V, Haenschel C, Fett AK, Kyriakopoulos M, Dima D. Functional neurodevelopment of working memory in early-onset schizophrenia: A longitudinal fmri study. Schizophr Res Cogn. (2022) 30:100268. doi: 10.1016/j.scog.2022.100268
9. Priol AC, Denis L, Boulanger G, Thépaut M, Geoffray MM, Tordjman S. Detection of morphological abnormalities in schizophrenia: an important step to identify associated genetic disorders or etiologic subtypes. Int J Mol Sci. (2021) 22(17):9464. doi: 10.3390/ijms22179464
10. Coulon N, Godin O, Bulzacka E, Dubertret C, Mallet J, Fond G, et al. Early and very early-onset schizophrenia compared with adult-onset schizophrenia: french face-sz database. Brain Behav. (2020) 10:e01495. doi: 10.1002/brb3.1495
11. Hu X, Wang S, Zhou H, Li N, Zhong C, Luo W, et al. Altered functional connectivity strength in distinct brain networks of children with early-onset schizophrenia. J Magnetic Resonance Imaging. (2023) 58:1617–23. doi: 10.1002/jmri.28682
12. Zhang D, Raichle ME. Disease and the brain's dark energy. Nat Rev Neurol. (2010) 6:15–28. doi: 10.1038/nrneurol.2009.198
13. Bennett CM, Miller MB. How reliable are the results from functional magnetic resonance imaging? Ann New York Acad Sci. (2010) 1191:133–55. doi: 10.1111/j.1749-6632.2010.05446.x
14. Biswal B, Yetkin FZ, Haughton VM, Hyde JS. Functional connectivity in the motor cortex of resting human brain using echo-planar mri. Magnetic resonance Med. (1995) 34:537–41. doi: 10.1002/mrm.1910340409
15. Zang YF, He Y, Zhu CZ, Cao QJ, Sui MQ, Liang M, et al. Altered baseline brain activity in children with adhd revealed by resting-state functional mri. Brain Dev. (2007) 29:83–91. doi: 10.1016/j.braindev.2006.07.002
16. Zou QH, Zhu CZ, Yang Y, Zuo XN, Long XY, Cao QJ, et al. An improved approach to detection of amplitude of low-frequency fluctuation (Alff) for resting-state fmri: fractional alff. J Neurosci Methods. (2008) 172:137–41. doi: 10.1016/j.jneumeth.2008.04.012
17. Zang Y, Jiang T, Lu Y, He Y, Tian L. Regional homogeneity approach to fmri data analysis. NeuroImage. (2004) 22:394–400. doi: 10.1016/j.neuroimage.2003.12.030
18. Yang Z, Xiao S, Su T, Gong J, Qi Z, Chen G, et al. A multimodal meta-analysis of regional functional and structural brain abnormalities in obsessive-compulsive disorder. Eur Arch Psychiatry Clin Neurosci. (2024) 274:165–80. doi: 10.1007/s00406-023-01594-x
19. Zheng J, Zhang Y, Guo X, Duan X, Zhang J, Zhao J, et al. Disrupted amplitude of low-frequency fluctuations in antipsychotic-naïve adolescents with early-onset schizophrenia. Psychiatry Res Neuroimaging. (2016) 249:20–6. doi: 10.1016/j.pscychresns.2015.11.006
20. Wang S, Zhang Y, Lv L, Wu R, Fan X, Zhao J, et al. Abnormal regional homogeneity as a potential imaging biomarker for adolescent-onset schizophrenia: A resting-state fmri study and support vector machine analysis. Schizophr Res. (2018) 192:179–84. doi: 10.1016/j.schres.2017.05.038
21. Yan W, Zhang R, Zhou M, Lu S, Li W, Xie S, et al. Relationships between abnormal neural activities and cognitive impairments in patients with drug-naive first-episode schizophrenia. BMC Psychiatry. (2020) 20:283. doi: 10.1186/s12888-020-02692-z
22. Jiang SA, Zhou B, Liao YH, Liu WQ, Tian CL, Chen XG, et al. Primary study of resting state functional magnetic resonance imaging in early onset schizophrenia using reho. J Cent South Univ (Med Sci). (2010) 35:947–51. doi: 10.3969/j.issn.1672-7347.2010.09.008
23. Li YL, Li YD, Zhang H, Gao ZT, Xia YH, Liang YH, et al. [Relationship between auditory hallucination and regional homogeneity of functional magnetic resonance imaging in first-episode childhood and adolescence-onset schizophrenia]. Zhonghua yi xue za zhi. (2021) 101:1915–20. doi: 10.3760/cma.j.cn112137-20201126-03195
24. Fan YS, Xu Y, Bayrak Ş, Shine JM, Wan B, Li H, et al. Macroscale thalamic functional organization disturbances and underlying core cytoarchitecture in early-onset schizophrenia. Schizophr Bull. (2023) 49(5):1375–86. doi: 10.1093/schbul/sbad048
25. Ashburner J, Friston KJ. Voxel-based morphometry–the methods. NeuroImage. (2000) 11:805–21. doi: 10.1006/nimg.2000.0582
26. Honey CJ, Kötter R, Breakspear M, Sporns O. Network structure of cerebral cortex shapes functional connectivity on multiple time scales. Proc Natl Acad Sci United States America. (2007) 104:10240–5. doi: 10.1073/pnas.0701519104
27. Zhang Y, Zheng J, Fan X, Guo X, Guo W, Yang G, et al. Dysfunctional resting-state connectivities of brain regions with structural deficits in drug-naive first-episode schizophrenia adolescents. Schizophr Res. (2015) 168:353–9. doi: 10.1016/j.schres.2015.07.031
28. Li Q, Liu S, Cao X, Li Z, Fan YS, Wang Y, et al. Disassociated and concurrent structural and functional abnormalities in the drug-naïve first-episode early onset schizophrenia. Brain Imaging Behav. (2022) 16:1627–35. doi: 10.1007/s11682-021-00608-3
29. Shen Y, Gao X, Huang C, Luo X, Ge R. Decreased gray matter volume is associated with theory of mind deficit in adolescents with schizophrenia. Brain Imaging Behav. (2022) 16:1441–50. doi: 10.1007/s11682-021-00591-9
30. Fang JC, Li DM, Chen XD, Nie BB, Liu H, Wang JJ, et al. Morphological abnormities in early-onset and late-onset schizophrenia patients. Chin J Clin Psychol. (2015) 23:839–42. doi: 10.16128/j.cnki.1005-3611.2015.05.019
31. Moher D, Liberati A, Tetzlaff J, Altman DG. Preferred reporting items for systematic reviews and meta-analyses: the prisma statement. PloS Med. (2009) 6:e1000097. doi: 10.1371/journal.pmed.1000097
32. Shepherd AM, Matheson SL, Laurens KR, Carr VJ, Green MJ. Systematic meta-analysis of insula volume in schizophrenia. Biol Psychiatry. (2012) 72:775–84. doi: 10.1016/j.biopsych.2012.04.020
33. Chen ZQ, Du MY, Zhao YJ, Huang XQ, Li J, Lui S, et al. Voxel-wise meta-analyses of brain blood flow and local synchrony abnormalities in medication-free patients with major depressive disorder. J Psychiatry neuroscience: JPN. (2015) 40:401–11. doi: 10.1503/jpn.140119
34. Yan H, Xiao S, Fu S, Gong J, Qi Z, Chen G, et al. Functional and structural brain abnormalities in substance use disorder: A multimodal meta-analysis of neuroimaging studies. Acta psychiatrica Scandinavica. (2023) 147:345–59. doi: 10.1111/acps.13539
35. Gao X, Zhang W, Yao L, Xiao Y, Liu L, Liu J, et al. Association between structural and functional brain alterations in drug-free patients with schizophrenia: A multimodal meta-analysis. J Psychiatry neuroscience: JPN. (2017) 43:160219. doi: 10.1503/jpn.160219
36. Radua J, Mataix-Cols D, Phillips ML, El-Hage W, Kronhaus DM, Cardoner N, et al. A new meta-analytic method for neuroimaging studies that combines reported peak coordinates and statistical parametric maps. Eur psychiatry: J Assoc Eur Psychiatrists. (2012) 27:605–11. doi: 10.1016/j.eurpsy.2011.04.001
37. Radua J, Rubia K, Canales-Rodríguez EJ, Pomarol-Clotet E, Fusar-Poli P, Mataix-Cols D. Anisotropic kernels for coordinate-based meta-analyses of neuroimaging studies. Front Psychiatry. (2014) 5:13. doi: 10.3389/fpsyt.2014.00013
38. Lim L, Radua J, Rubia K. Gray matter abnormalities in childhood maltreatment: A voxel-wise meta-analysis. Am J Psychiatry. (2014) 171:854–63. doi: 10.1176/appi.ajp.2014.13101427
39. Zhang M, Huang X, Li B, Shang H, Yang J. Gray matter structural and functional alterations in idiopathic blepharospasm: A multimodal meta-analysis of vbm and functional neuroimaging studies. Front Neurol. (2022) 13:889714. doi: 10.3389/fneur.2022.889714
40. Radua J, Borgwardt S, Crescini A, Mataix-Cols D, Meyer-Lindenberg A, McGuire PK, et al. Multimodal meta-analysis of structural and functional brain changes in first episode psychosis and the effects of antipsychotic medication. Neurosci Biobehav Rev. (2012) 36:2325–33. doi: 10.1016/j.neubiorev.2012.07.012
41. Alegria AA, Radua J, Rubia K. Meta-analysis of fmri studies of disruptive behavior disorders. Am J Psychiatry. (2016) 173:1119–30. doi: 10.1176/appi.ajp.2016.15081089
42. Ruiz-Torras S, Gudayol-Ferré E, Fernández-Vazquez O, Cañete-Massé C, Peró-Cebollero M, Guàrdia-Olmos J. Hypoconnectivity networks in schizophrenia patients: A voxel-wise meta-analysis of rs-fmri. Int J Clin Health psychology: IJCHP. (2023) 23:100395. doi: 10.1016/j.ijchp.2023.100395
43. Egger M, Davey Smith G, Schneider M, Minder C. Bias in meta-analysis detected by a simple, graphical test. BMJ (Clinical Res ed). (1997) 315:629–34. doi: 10.1136/bmj.315.7109.629
44. Gong J, Wang J, Luo X, Chen G, Huang H, Huang R, et al. Abnormalities of intrinsic regional brain activity in first-episode and chronic schizophrenia: A meta-analysis of resting-state functional mri. J Psychiatry neuroscience: JPN. (2020) 45:55–68. doi: 10.1503/jpn.180245
45. Sterne JA, Sutton AJ, Ioannidis JP, Terrin N, Jones DR, Lau J, et al. Recommendations for examining and interpreting funnel plot asymmetry in meta-analyses of randomised controlled trials. BMJ (Clinical Res ed). (2011) 343:d4002. doi: 10.1136/bmj.d4002
46. Xiao S, Yang Z, Su T, Gong J, Huang L, Wang Y. Functional and structural brain abnormalities in posttraumatic stress disorder: A multimodal meta-analysis of neuroimaging studies. J Psychiatr Res. (2022) 155:153–62. doi: 10.1016/j.jpsychires.2022.08.010
47. Wang Y, Gao Y, Tang S, Lu L, Zhang L, Bu X, et al. Large-scale network dysfunction in the acute state compared to the remitted state of bipolar disorder: A meta-analysis of resting-state functional connectivity. EBioMedicine. (2020) 54:102742. doi: 10.1016/j.ebiom.2020.102742
48. Lü D, Shao RR, Liang YH, Xia YH, Guo SQ. [Fractional amplitude of low-frequency fluctuations in childhood and adolescence-onset schizophrenia: A resting state fmri study]. Zhonghua yi xue za zhi. (2016) 96:3479–84. doi: 10.3760/cma.j.issn.0376-2491.2016.43.008
49. Koeda M, Takahashi H, Matsuura M, Asai K, Okubo Y. Cerebral responses to vocal attractiveness and auditory hallucinations in schizophrenia: A functional mri study. Front Hum Neurosci. (2013) 7:221. doi: 10.3389/fnhum.2013.00221
50. Kikinis Z, Fallon JH, Niznikiewicz M, Nestor P, Davidson C, Bobrow L, et al. Gray matter volume reduction in rostral middle frontal gyrus in patients with chronic schizophrenia. Schizophr Res. (2010) 123:153–9. doi: 10.1016/j.schres.2010.07.027
51. Weinberger DR, Berman KF, Zec RF. Physiologic dysfunction of dorsolateral prefrontal cortex in schizophrenia. I. Regional cerebral blood flow evidence. Arch Gen Psychiatry. (1986) 43:114–24. doi: 10.1001/archpsyc.1986.01800020020004
52. Cannon TD, Glahn DC, Kim J, Van Erp TG, Karlsgodt K, Cohen MS, et al. Dorsolateral prefrontal cortex activity during maintenance and manipulation of information in working memory in patients with schizophrenia. Arch Gen Psychiatry. (2005) 62:1071–80. doi: 10.1001/archpsyc.62.10.1071
53. Meda SA, Bhattarai M, Morris NA, Astur RS, Calhoun VD, Mathalon DH, et al. An fmri study of working memory in first-degree unaffected relatives of schizophrenia patients. Schizophr Res. (2008) 104:85–95. doi: 10.1016/j.schres.2008.06.013
54. Curtin A, Sun J, Zhao Q, Onaral B, Wang J, Tong S, et al. Visuospatial task-related prefrontal activity is correlated with negative symptoms in schizophrenia. Sci Rep. (2019) 9:9575. doi: 10.1038/s41598-019-45893-7
55. Luck SJ, Ford JM, Sarter M, Lustig C. Cntrics final biomarker selection: control of attention. Schizophr Bull. (2012) 38:53–61. doi: 10.1093/schbul/sbr065
56. Li HJ, Chan RC, Gong QY, Liu Y, Liu SM, Shum D, et al. Facial emotion processing in patients with schizophrenia and their non-psychotic siblings: A functional magnetic resonance imaging study. Schizophr Res. (2012) 134:143–50. doi: 10.1016/j.schres.2011.10.019
57. Athanassiou M, Dumais A, Iammatteo V, De Benedictis L, Dubreucq JL, Potvin S. The processing of angry faces in schizophrenia patients with a history of suicide: an fmri study examining brain activity and connectivity. Prog Neuropsychopharmacol Biol Psychiatry. (2021) 107:110253. doi: 10.1016/j.pnpbp.2021.110253
58. Besteher B, Wagner G, Koch K, Schachtzabel C, Reichenbach JR, Schlösser R, et al. Pronounced prefronto-temporal cortical thinning in schizophrenia: neuroanatomical correlate of suicidal behavior? Schizophr Res. (2016) 176:151–7. doi: 10.1016/j.schres.2016.08.010
59. Bicks LK, Koike H, Akbarian S, Morishita H. Prefrontal cortex and social cognition in mouse and man. Front Psychol. (2015) 6:1805. doi: 10.3389/fpsyg.2015.01805
60. Nelson EE, Guyer AE. The development of the ventral prefrontal cortex and social flexibility. Dev Cogn Neurosci. (2011) 1:233–45. doi: 10.1016/j.dcn.2011.01.002
61. Feng A, Luo N, Zhao W, Calhoun VD, Jiang R, Zhi D, et al. Multimodal brain deficits shared in early-onset and adult-onset schizophrenia predict positive symptoms regardless of illness stage. Hum Brain Mapp. (2022) 43:3486–97. doi: 10.1002/hbm.25862
62. Pujol N, Penadés R, Rametti G, Catalán R, Vidal-Piñeiro D, Palacios E, et al. Inferior frontal and insular cortical thinning is related to dysfunctional brain activation/deactivation during working memory task in schizophrenic patients. Psychiatry Res. (2013) 214:94–101. doi: 10.1016/j.pscychresns.2013.06.008
63. Liakakis G, Nickel J, Seitz RJ. Diversity of the inferior frontal gyrus–a meta-analysis of neuroimaging studies. Behav Brain Res. (2011) 225:341–7. doi: 10.1016/j.bbr.2011.06.022
64. Greenlee JD, Oya H, Kawasaki H, Volkov IO, Severson MA 3rd, Howard MA 3rd, et al. Functional connections within the human inferior frontal gyrus. J Comp Neurol. (2007) 503:550–9. doi: 10.1002/cne.21405
65. Shenton ME, Dickey CC, Frumin M. McCarley RW. A review of mri findings in schizophrenia. Schizophr Res. (2001) 49:1–52. doi: 10.1016/s0920-9964(01)00163-3
66. Hahn W, Domahs F, Straube B, Kircher T, Nagels A. Neural processing of nouns and verbs in spontaneous speech of patients with schizophrenia. Psychiatry Res Neuroimaging. (2021) 318:111395. doi: 10.1016/j.pscychresns.2021.111395
67. Pilon F, Boisvert M, Potvin S. Losing the chain of thought: A meta-analysis of functional neuroimaging studies using verbal tasks in schizophrenia. J Psychiatr Res. (2024) 169:238–46. doi: 10.1016/j.jpsychires.2023.11.013
68. Kang J, Jiao Z, Qin Y, Wang Y, Wang J, Jin L, et al. Associations between polygenic risk scores and amplitude of low-frequency fluctuation of inferior frontal gyrus in schizophrenia. J Psychiatr Res. (2022) 147:4–12. doi: 10.1016/j.jpsychires.2021.12.043
69. Li W, Kutas M, Gray JA, Hagerman RH, Olichney JM. The role of glutamate in language and language disorders - evidence from erp and pharmacologic studies. Neurosci Biobehav Rev. (2020) 119:217–41. doi: 10.1016/j.neubiorev.2020.09.023
70. Thermenos HW, Whitfield-Gabrieli S, Seidman LJ, Kuperberg G, Juelich RJ, Divatia S, et al. Altered language network activity in young people at familial high-risk for schizophrenia. Schizophr Res. (2013) 151:229–37. doi: 10.1016/j.schres.2013.09.023
71. Gao S, Lu S, Shi X, Ming Y, Xiao C, Sun J, et al. Distinguishing between treatment-resistant and non-treatment-resistant schizophrenia using regional homogeneity. Front Psychiatry. (2018) 9:282. doi: 10.3389/fpsyt.2018.00282
72. Jardri R, Pouchet A, Pins D, Thomas P. Cortical activations during auditory verbal hallucinations in schizophrenia: A coordinate-based meta-analysis. Am J Psychiatry. (2011) 168:73–81. doi: 10.1176/appi.ajp.2010.09101522
73. Romeo Z, Spironelli C. Hearing voices in the head: two meta-analyses on structural correlates of auditory hallucinations in schizophrenia. NeuroImage Clin. (2022) 36:103241. doi: 10.1016/j.nicl.2022.103241
74. Turner JA, Damaraju E, van Erp TG, Mathalon DH, Ford JM, Voyvodic J, et al. A multi-site resting state fmri study on the amplitude of low frequency fluctuations in schizophrenia. Front Neurosci. (2013) 7:137. doi: 10.3389/fnins.2013.00137
75. Meda SA, Wang Z, Ivleva EI, Poudyal G, Keshavan MS, Tamminga CA, et al. Frequency-specific neural signatures of spontaneous low-frequency resting state fluctuations in psychosis: evidence from bipolar-schizophrenia network on intermediate phenotypes (B-snip) consortium. Schizophr Bull. (2015) 41:1336–48. doi: 10.1093/schbul/sbv064
76. Howes OD, Kapur S. The dopamine hypothesis of schizophrenia: version iii–the final common pathway. Schizophr Bull. (2009) 35:549–62. doi: 10.1093/schbul/sbp006
77. Carlsson A. Neurocircuitries and neurotransmitter interactions in schizophrenia. Int Clin Psychopharmacol. (1995) 10 Suppl 3:21–8. doi: 10.1097/00004850-199509003-00004
78. Ehrlich S, Yendiki A, Greve DN, Manoach DS, Ho BC, White T, et al. Striatal function in relation to negative symptoms in schizophrenia. psychol Med. (2012) 42:267–82. doi: 10.1017/s003329171100119x
79. Haber SN. The place of dopamine in the cortico-basal ganglia circuit. Neuroscience. (2014) 282:248–57. doi: 10.1016/j.neuroscience.2014.10.008
80. McCutcheon R, Beck K, Jauhar S, Howes OD. Defining the locus of dopaminergic dysfunction in schizophrenia: A meta-analysis and test of the mesolimbic hypothesis. Schizophr Bull. (2018) 44:1301–11. doi: 10.1093/schbul/sbx180
81. Agid O, Mamo D, Ginovart N, Vitcu I, Wilson AA, Zipursky RB, et al. Striatal vs extrastriatal dopamine D2 receptors in antipsychotic response–a double-blind pet study in schizophrenia. Neuropsychopharmacology: Off Publ Am Coll Neuropsychopharmacol. (2007) 32:1209–15. doi: 10.1038/sj.npp.1301242
82. Oertel-Knöchel V, Knöchel C, Matura S, Rotarska-Jagiela A, Magerkurth J, Prvulovic D, et al. Cortical-basal ganglia imbalance in schizophrenia patients and unaffected first-degree relatives. Schizophr Res. (2012) 138:120–7. doi: 10.1016/j.schres.2012.02.029
83. Sorg C, Manoliu A, Neufang S, Myers N, Peters H, Schwerthöffer D, et al. Increased intrinsic brain activity in the striatum reflects symptom dimensions in schizophrenia. Schizophr Bull. (2013) 39:387–95. doi: 10.1093/schbul/sbr184
84. Rolls ET, Thorpe SJ, Boytim M, Szabo I, Perrett DI. Responses of striatal neurons in the behaving monkey. 3. Effects of iontophoretically applied dopamine on normal responsiveness. Neuroscience. (1984) 12:1201–12. doi: 10.1016/0306-4522(84)90014-9
85. Howes OD, Bukala BR, Beck K. Schizophrenia: from neurochemistry to circuits, symptoms and treatments. Nat Rev Neurol. (2024) 20:22–35. doi: 10.1038/s41582-023-00904-0
86. Rolls ET, Loh M, Deco G, Winterer G. Computational models of schizophrenia and dopamine modulation in the prefrontal cortex. Nat Rev Neurosci. (2008) 9:696–709. doi: 10.1038/nrn2462
87. Xu X, Li Q, Qian Y, Cai H, Zhang C, Zhao W, et al. Genetic mechanisms underlying gray matter volume changes in patients with drug-naive first-episode schizophrenia. Cereb Cortex (New York NY: 1991). (2023) 33:2328–41. doi: 10.1093/cercor/bhac211
88. Sun J, Maller JJ, Guo L, Fitzgerald PB. Superior temporal gyrus volume change in schizophrenia: A review on region of interest volumetric studies. Brain Res Rev. (2009) 61:14–32. doi: 10.1016/j.brainresrev.2009.03.004
89. Modinos G, Costafreda SG, van Tol MJ, McGuire PK, Aleman A, Allen P. Neuroanatomy of auditory verbal hallucinations in schizophrenia: A quantitative meta-analysis of voxel-based morphometry studies. Cortex. (2013) 49:1046–55. doi: 10.1016/j.cortex.2012.01.009
90. Barta PE, Pearlson GD, Powers RE, Richards SS, Tune LE. Auditory hallucinations and smaller superior temporal gyral volume in schizophrenia. Am J Psychiatry. (1990) 147:1457–62. doi: 10.1176/ajp.147.11.1457
91. Zhang M, Xiang H, Yang F, Fan H, Fan F, Song J, et al. Structural brain imaging abnormalities correlate with positive symptom in schizophrenia. Neurosci Lett. (2022) 782:136683. doi: 10.1016/j.neulet.2022.136683
92. Sumich A, Chitnis XA, Fannon DG, O'Ceallaigh S, Doku VC, Faldrowicz A, et al. Unreality symptoms and volumetric measures of heschl's gyrus and planum temporal in first-episode psychosis. Biol Psychiatry. (2005) 57:947–50. doi: 10.1016/j.biopsych.2004.12.041
93. Yamasaki S, Yamasue H, Abe O, Yamada H, Iwanami A, Hirayasu Y, et al. Reduced planum temporale volume and delusional behaviour in patients with schizophrenia. Eur Arch Psychiatry Clin Neurosci. (2007) 257:318–24. doi: 10.1007/s00406-007-0723-5
94. Bandeira ID, Barouh JL, Bandeira ID, Quarantini L. Analysis of the superior temporal gyrus as a possible biomarker in schizophrenia using voxel-based morphometry of the brain magnetic resonance imaging: A comprehensive review. CNS spectrums. (2021) 26:319–25. doi: 10.1017/s1092852919001810
95. Chen C, Huang H, Qin X, Zhang L, Rong B, Wang G, et al. Reduced inter-hemispheric auditory and memory-related network interactions in patients with schizophrenia experiencing auditory verbal hallucinations. Front Psychiatry. (2022) 13:956895. doi: 10.3389/fpsyt.2022.956895
96. Lui S, Deng W, Huang X, Jiang L, Ma X, Chen H, et al. Association of cerebral deficits with clinical symptoms in antipsychotic-naive first-episode schizophrenia: an optimized voxel-based morphometry and resting state functional connectivity study. Am J Psychiatry. (2009) 166:196–205. doi: 10.1176/appi.ajp.2008.08020183
97. Chua SE, Cheung C, Cheung V, Tsang JT, Chen EY, Wong JC, et al. Cerebral grey, white matter and csf in never-medicated, first-episode schizophrenia. Schizophr Res. (2007) 89:12–21. doi: 10.1016/j.schres.2006.09.009
98. Fortea A, Batalla A, Radua J, van Eijndhoven P, Baeza I, Albajes-Eizagirre A, et al. Cortical gray matter reduction precedes transition to psychosis in individuals at clinical high-risk for psychosis: A voxel-based meta-analysis. Schizophr Res. (2021) 232:98–106. doi: 10.1016/j.schres.2021.05.008
99. Kim JJ, Crespo-Facorro B, Andreasen NC, O'Leary DS, Magnotta V, Nopoulos P. Morphology of the lateral superior temporal gyrus in neuroleptic nai;Ve patients with schizophrenia: relationship to symptoms. Schizophr Res. (2003) 60:173–81. doi: 10.1016/s0920-9964(02)00299-2
100. Makowski C, Nichols TE, Dale AM. Quality over quantity: powering neuroimaging samples in psychiatry. Neuropsychopharmacology. (2024). doi: 10.1038/s41386-024-01893-4
101. Guo F, Zhu YQ, Li C, Wang XR, Wang HN, Liu WM, et al. Gray matter volume changes following antipsychotic therapy in first-episode schizophrenia patients: A longitudinal voxel-based morphometric study. J Psychiatr Res. (2019) 116:126–32. doi: 10.1016/j.jpsychires.2019.06.009
102. Cui X, Deng Q, Lang B, Su Q, Liu F, Zhang Z, et al. Less reduced gray matter volume in the subregions of superior temporal gyrus predicts better treatment efficacy in drug-naive, first-episode schizophrenia. Brain Imaging Behav. (2021) 15:1997–2004. doi: 10.1007/s11682-020-00393-5
103. Lu J, Gou N, Sun Q, Huang Y, Guo H, Han D, et al. Brain structural alterations associated with impulsiveness in male violent patients with schizophrenia. BMC Psychiatry. (2024) 24:281. doi: 10.1186/s12888-024-05721-3
104. Kuroki N, Kashiwagi H, Ota M, Ishikawa M, Kunugi H, Sato N, et al. Brain structure differences among male schizophrenic patients with history of serious violent acts: an mri voxel-based morphometric study. BMC Psychiatry. (2017) 17:105. doi: 10.1186/s12888-017-1263-9
105. Weng Y, Lin J, Ahorsu DK, Tsang HWH. Neuropathways of theory of mind in schizophrenia: A systematic review and meta-analysis. Neurosci Biobehav Rev. (2022) 137:104625. doi: 10.1016/j.neubiorev.2022.104625
106. Parola A, Brasso C, Morese R, Rocca P, Bosco FM. Understanding communicative intentions in schizophrenia using an error analysis approach. NPJ Schizophr. (2021) 7:12. doi: 10.1038/s41537-021-00142-7
107. Morese R, Brasso C, Stanziano M, Parola A, Valentini MC, Bosco FM, et al. Efforts for the correct comprehension of deceitful and ironic communicative intentions in schizophrenia: A functional magnetic resonance imaging study on the role of the left middle temporal gyrus. Front Psychol. (2022) 13:866160. doi: 10.3389/fpsyg.2022.866160
108. Green MF, Horan WP, Lee J. Nonsocial and social cognition in schizophrenia: current evidence and future directions. World psychiatry: Off J World Psychiatr Assoc (WPA). (2019) 18:146–61. doi: 10.1002/wps.20624
109. Onitsuka T, Shenton ME, Salisbury DF, Dickey CC, Kasai K, Toner SK, et al. Middle and inferior temporal gyrus gray matter volume abnormalities in chronic schizophrenia: an mri study. Am J Psychiatry. (2004) 161:1603–11. doi: 10.1176/appi.ajp.161.9.1603
110. Tang J, Liao Y, Zhou B, Tan C, Liu W, Wang D, et al. Decrease in temporal gyrus gray matter volume in first-episode, early onset schizophrenia: an mri study. PloS One. (2012) 7:e40247. doi: 10.1371/journal.pone.0040247
111. Guo W, Hu M, Fan X, Liu F, Wu R, Chen J, et al. Decreased gray matter volume in the left middle temporal gyrus as a candidate biomarker for schizophrenia: A study of drug naive, first-episode schizophrenia patients and unaffected siblings. Schizophr Res. (2014) 159:43–50. doi: 10.1016/j.schres.2014.07.051
112. Williams JA, Burgess S, Suckling J, Lalousis PA, Batool F, Griffiths SL, et al. Inflammation and brain structure in schizophrenia and other neuropsychiatric disorders: A mendelian randomization study. JAMA Psychiatry. (2022) 79:498–507. doi: 10.1001/jamapsychiatry.2022.0407
113. Ohnishi T, Hashimoto R, Mori T, Nemoto K, Moriguchi Y, Iida H, et al. The association between the val158met polymorphism of the catechol-O-methyl transferase gene and morphological abnormalities of the brain in chronic schizophrenia. Brain: J Neurol. (2006) 129:399–410. doi: 10.1093/brain/awh702
114. Ira E, Zanoni M, Ruggeri M, Dazzan P, Tosato S. Comt, neuropsychological function and brain structure in schizophrenia: A systematic review and neurobiological interpretation. J Psychiatry neuroscience: JPN. (2013) 38:366–80. doi: 10.1503/jpn.120178
Keywords: early-onset schizophrenia, voxel-based morphometry, gray matter volume, resting state functional imaging, multimodal, meta-analysis
Citation: Wang L, Liu R, Liao J, Xiong X, Xia L, Wang W, Liu J, Zhao F, Zhuo L and Li H (2024) Meta-analysis of structural and functional brain abnormalities in early-onset schizophrenia. Front. Psychiatry 15:1465758. doi: 10.3389/fpsyt.2024.1465758
Received: 16 July 2024; Accepted: 06 August 2024;
Published: 23 August 2024.
Edited by:
Massimo Tusconi, University of Cagliari, ItalyReviewed by:
Cristiano Chaves, Queen’s University, CanadaSerdar M. Dursun, University of Alberta, Canada
Copyright © 2024 Wang, Liu, Liao, Xiong, Xia, Wang, Liu, Zhao, Zhuo and Li. This is an open-access article distributed under the terms of the Creative Commons Attribution License (CC BY). The use, distribution or reproduction in other forums is permitted, provided the original author(s) and the copyright owner(s) are credited and that the original publication in this journal is cited, in accordance with accepted academic practice. No use, distribution or reproduction is permitted which does not comply with these terms.
*Correspondence: Hongwei Li, bGh3NjMyMUAxNjMuY29t; Lihua Zhuo, MTAyOTUxMTEwNEBxcS5jb20=