- 1Department of Psychiatry, Faculty of Medicine, Masaryk University, Brno, Czechia
- 2Department of Computer Graphics and Multimedia, Faculty of Information Technologies, Brno University of Technology, Brno, Czechia
Introduction: Virtual reality (VR) holds significant promise for psychiatric research, treatment, and assessment. Its unique ability to elicit immersion and presence is important for effective interventions. Immersion and presence are influenced by matching—the alignment between provided sensory information and user feedback, and self-presentation—the depiction of a user’s virtual body or limbs. Discrepancies between real and virtual hands can affect the sense of presence and thus treatment efficacy. However, the precise impact of positional offsets in healthy individuals remains under-explored. This review assesses how various factors influence the detection thresholds for positional offsets in VR among healthy subjects.
Methods: A comprehensive database search targeted English-language studies on the detection thresholds of virtual hand positional offsets using head-mounted displays (HMDs) with specific tracking capabilities. Data on methodologies, participant demographics, and VR system specifics were extracted.
Results: Thirteen studies met the inclusion criteria, revealing significant variability in detection thresholds—from a few millimeters to 42 cm for linear shifts and from 2° to 45° for angular shifts. Sensitivity to these offsets was affected by hand movement direction and magnitude, hand representation realism, and the presence of distractions. VR system specifications, such as resolution and tracking accuracy, also played a significant role. Methodological issues included small sample sizes, inadequate demographic reporting, and inconsistent presence or avatar embodiment measures.
Conclusion: The results highlight the need to consider identified influencing factors to maximize user presence in VR-based therapies. Variability in VR device capabilities also emphasizes the need for detailed reporting of device properties in research. The individual variability in offset detection further illustrates VR’s potential as a tool for studying body ownership and multisensory integration.
1 Introduction
Virtual reality (VR) is a technology that has been attracting the attention of researchers in psychiatry and neuroscience since the 1990s (1), soon after Jaron Lanier first used the term in 1987 (2). Interest in this technology was increasing hand in hand with advances in computer graphics capabilities and, in recent years, also with increased availability of cost-effective VR hardware. The main feature distinguishing this human-computer interaction method from others is the ability to elicit a sense of presence in the user (3). However, this is only one of its advantages, which further include options to present various multi-modal stimuli, manipulate them and measure the activity of the user in a simulated environment (4). VR thus represents an unparalleled tool for ecologically valid treatment interventions and assessment.
Presence is mediated by but distinct from the concept of immersion. While presence is a subjective feeling of ‘being there’ within the virtual environment, immersion is an objective measure of the hardware’s capabilities (5). The degree of immersion—and consequently, the sense of presence—is influenced by several facets of VR equipment. These aspects include matching, which refers to the concordance between provided sensory information and the user’s feedback, and self-presentation, involving the representation of the participant’s virtual body or limbs (5). The main focus of this review will be on matching, with self-presentation discussed primarily as an influencing factor.
To achieve a satisfactory sense of presence, the position of the user’s virtual self-representation needs to align closely with that of their real body or limbs. However, phenomena such as the rubber hand illusion (6), indicate that this alignment does not need to be perfect. There appears to exist a perceptible margin within which discrepancies between the virtual and actual limb positions do not significantly disrupt the user’s sense of immersion and presence. For the purpose of this review, the discrepancy between the virtual and actual limb positions will be termed positional offset.
The ability to detect and respond to positional offsets of a virtual hand can significantly affect a user’s sense of immersion and presence. In the following text, the term detection threshold will refer to the minimum spatial difference between the virtual and real hands that can be detected with a predefined probability. Understanding detection thresholds can help optimize VR environments to enhance immersion, making therapeutic interventions more effective. Additionally, detection thresholds can serve as a practical, technology-agnostic proxy measure to address standardization of various VR hardware in mental-health research. Lastly, variability in positional offset detection offers a promising research tool for exploring alterations in bodily self-consciousness and related constructs.
Bodily self-consciousness represents the integration of various sensory inputs to construct the physical self (7). It outlines three main components: sense of ownership, self-location, and first-person perspective (8). Sense of ownership refers to the feeling that a body or body part belongs to oneself (9) and self-location is the perceived spatial location of oneself (10). The first-person perspective is the viewpoint from which one interacts with the world (11), although some argue it cannot be distinctly separated from self-location (8).
Disturbances in body ownership and self-location are prevalent in several mental disorders, indicating altered bodily self-consciousness. Notably, this includes schizophrenia spectrum disorders (12), borderline personality disorder (13), eating disorders (14), social anxiety (15) post-traumatic stress disorder (16) and autism spectrum disorders (17). Exploring interindividual variability in positional offset detection could illuminate underlying mechanisms of bodily self-consciousness alterations and contribute to a development of diagnostic tools and targeted interventions.
While basic concepts such as immersion and presence in VR are well-established, the precise impact of positional offsets on these experiences in healthy individuals has not yet been thoroughly quantified. Understanding the detection thresholds and influences of positional offsets is essential for creating immersive VR environments and for body-consciousness research. This review seeks to fill this gap by systematically assessing how various factors affect the perception of positional shifts, thereby providing a reliable baseline from which deviations in clinical populations can be identified and addressed.
The aim of this review therefore is to assess the detection thresholds for positional offsets in virtual reality among healthy individuals, examining how factors such as the direction of movement, temporal characteristics, visual representation, and environmental distractions influence these thresholds.
2 Methods
2.1 PRISMA checklist
This systematic review was conducted with regard to the Preferred Reporting Items for Systematic reviews and Meta-Analyses (PRISMA) (18). The PRISMA 2020 checklist, along with item locations, is provided in the Supplementary Materials.
2.2 Eligibility criteria
This systematic review included research articles, proceeding papers, and book chapters published in English from 1998 to 2024. The starting year marks the first mention of the Virtual Research V8 VR set in a published study. Only completed studies that presented original data were considered. Exclusions were made for pilot studies, reviews, abstracts, and studies involving neurologically or psychiatrically impaired participants.
For inclusion, studies had to employ immersive virtual reality technology, characterized by the immersion of participants in a 3D virtual environment equipped with a head-mounted display and a hand-tracking system. Technologies involving mixed reality, shutter glasses, and virtual scenarios displayed on a computer screen were excluded.
Eligible studies had to involve a positional shift applied to a virtual hand. These shifts could be angular or linear, and either fixed or continuously increasing. Studies utilizing gain displacements were excluded. Participants must have seen a representation of their hands in the form of a virtual hand, finger, or fingertip depicted as a geometric shape; studies focusing on unseen hands, other body parts, or tools were excluded.
Finally, included studies were required to measure and precisely define detection thresholds for positional shifts, with estimated values clearly stated and described.
2.3 Information sources and search strategy
Literature searches were conducted using two databases: Scopus and Web of Science. The most recent search was completed on May 15, 2024. Research areas included Computer Science, Engineering, Neurology, Psychiatry, Neuroscience, and Psychology, with searches performed on titles, abstracts, and keywords.
Given the technological focus of this review, terms like proprioception and multisensory integration were excluded to avoid limiting the search to medical literature. Instead, broader, general terms were selected to maximize the retrieval of relevant studies. Search terms were grouped into five categories: virtual reality, detection threshold, hand, shift, and participants. The search string is listed in full in the Supplementary Materials.
2.4 Data items
Data items were chosen to answer the following research questions:
1. Does the direction of positional offsets influence the detection?
Direction of positional offsets refers to the specific spatial relationship between virtual and real hands. In relation to real hands location, the virtual hands might be shifted in a horizontal manner (X-axis), vertical manner (Y-axis) or sagittal manner (Z-axis).
2. Is the sensitivity to positional offsets influenced by their character in time?
The magnitude of displayed positional offsets might remain temporally constant or evolve (i.e. increase or decrease). This attribute of positional offsets is expressed as character in time.
3. Does the distance of the hand and the direction of hand reach influence the detection of positional offsets?
The first part of this research question asks whether the distance between the hand and the body influences the ability to detect positional offsets of virtual hands. This systematic review also investigates whether the position of the target and thus the direction of a moving hand influences the offset detection.
4. Does the depiction of the hand in virtual reality influence the detection of positional offsets?
The virtual extremities might be depicted as realistic hand-shaped objects or as abstract objects lacking the resemblance to real hands.
5. Can a distraction influence the detection threshold of positional offsets?
For the purpose of this systematic review, distractions refer to additional sensory signals that tend to either mislead or divert attention.
6. Are detection thresholds of positional offsets influenced by participant’s gender?
7. Does handedness influence the detection of positional offsets?
3 Results
After the database search and records screening, 13 studies were identified as relevant based on the eligibility criteria, as illustrated in the PRISMA flow diagram (see Figure 1). These studies employed various methodologies and terminology; related terms and concepts are explained in Table 1. Basic information about the included studies and their samples is summarized in Table 2, while technical characteristics and paradigm details are outlined in Tables 3, 4, respectively. Table 5 provides a summary of the results, focusing on the extent of offset detection. For clarity, the term higher sensitivity is used to describe a smaller detection threshold in outcome comparisons. The text below offers a concise summary of the findings, addressing the research questions outlined earlier.
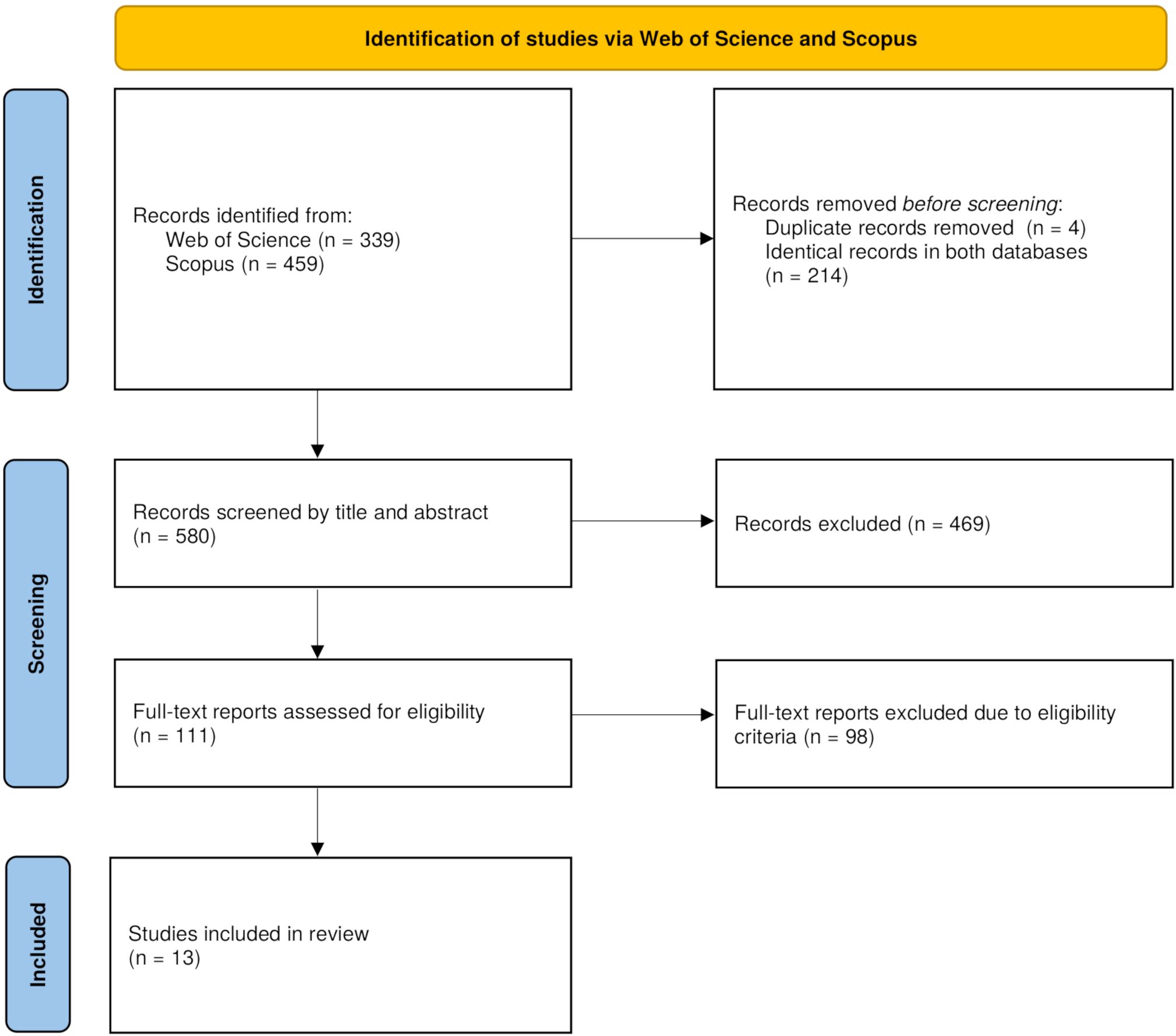
Figure 1. PRISMA diagram (18).
3.1 Sample sizes and demographic characteristics of included studies
Most of the reviewed studies (ten in total) included 20 or fewer participants. Two of the assessed studies included 22 participants. One study recruited 40 participants.
The number of male participants exceeded the number of female participants in a total of seven studies. In two reviewed studies, the pattern inverted with a predominance of female participants. In three of the reviewed studies, the number of male and female participants corresponded. One study was performed only in male participants.
Six studies were performed only in right-handed participants. Other six studies recruited both right- and left-handed participants. In five of these studies, right-handed participants prevailed, only one study was performed on corresponding numbers of left- and right-handed participants. One study did not report handedness.
In nine studies, the mean age of the participants falls under the age group 20-30, standard deviation is reported in seven of these studies. Although mere age intervals were reported in three studies, they indicate that the predominant age group of the participants will not significantly differ from other studies. One study did not report the participants’ age.
The demographic characteristics of samples in the eligible studies are summarized in Table 2.
3.2 Research question 1: does the direction of positional offsets influence the detection?
Shifts can be categorized based on the axis related to the hand-target trajectory: X-axis (right-left), Y-axis (up-down), and Z-axis (farther-closer). In studies examining the X-axis, participants were more sensitive to rightward shifts in two studies (19, 20), with statistical significance reported in one (19). However, two other studies found no significant differences within the X-axis (21, 22). One study noted greater noticeability of leftward shifts but did not report statistical significance (23). Of note, the overwhelming majority of the subjects were right-handed.
For the Y-axis, studies reported mixed findings: one study found higher sensitivity to upward shifts (20), and another to downward shifts (24), neither reporting p-values. Two other studies observed no significant differences along the Y-axis (21, 22).
Shifts towards the body were detected more easily than those away from the body in two studies (19, 22). Comparatively, participants consistently showed higher sensitivity to shifts along the X-axis than those along the Y-axis (20, 22, 25) and Z-axis (19, 22). One study outlined an order of sensitivity from highest to lowest as X-axis, Z-axis, then Y-axis (22).
3.3 Research question 2: is the sensitivity to positional offsets influenced by their character in time?
Shifts can be categorized based on their temporal characteristics into fixed and continuously increasing types. Zenner et al. (25) explored two specific forms of shifts: instantaneously increasing shifts (fixed shifts that increase after blinks) and continuously increasing shifts. The study found that participants were most sensitive to instantaneously increasing shifts. Detection thresholds significantly rose when continuous shifts were introduced. Furthermore, continuous shifts, without preliminary exposure to fixed shifts, exhibited the lowest detection sensitivity.
3.4 Research question 3: does the distance of the hand and the direction of hand reach influence the detection of positional offsets?
This question was explored in two studies. Deligiannidis et al. (19) analyzed shifts within a workspace divided into three rows at varying distances from the participant and three columns along the sagittal plane. Detection was most effective in the row closest to the participant, and in the median plane. However, statistical analysis showed only a significant effect of the column.
In a second study by Clarence et al. (23), participants interacted with eight targets at different distances. The results showed significantly higher sensitivity to discrepancies when reaching towards the body compared to reaching away from the body.
3.5 Research question 4: does the depiction of the hand in virtual reality influence the detection of positional offsets?
A single study investigated the effect of virtual hand depiction on the sensitivity to positional shifts (26). The findings indicated that the detection threshold for positional shifts was significantly lower when abstract objects were used compared to realistic virtual hands, but this was only the case for leftward shifts. For rightward shifts, there was no significant difference in detection thresholds between the realistic and abstract avatars.
3.6 Research question 5: can a distraction influence the detection threshold of positional offsets?
Three studies explored the impact of distractions on detection thresholds. In the first study, Lee et al. (27) found that false haptic feedback on an index finger increased the just noticeable difference, although the statistical significance was not reported. The second study by Zenner et al. (20) used audio-vibrational distractions mimicking a flying bee, along with visual distractions coupled with a heightened cognitive load, reporting only minor differences between distracted and undistracted conditions. In the third study, Ogawa et al. (28) applied noisy tendon electrical stimulation, which significantly increased the detection threshold.
3.7 Research question 6: are detection thresholds of positional offsets influenced by participant’s gender?
Three studies examined the potential effect of participant gender on detection thresholds, yielding mixed results. Clarence et al. (23) found no significant differences between genders. Conversely, Ogawa et al. (28) reported that men were significantly more sensitive to displacements. Meanwhile, Yang et al. (29) observed higher sensitivity among women, although they did not report a p-value for this finding.
3.8 Research question 7: does handedness influence the detection of positional offsets?
In a single study (19), right-handed subjects were more sensitive than left-handed subjects. According to the authors, the difference was ‘marginally significant’.
3.9 Hand tracking accuracy and technological considerations
The accuracy of hand tracking in VR devices is a factor that significantly influences the assessment of results. Hand tracking systems vary between inside-out tracking, where sensors are built into the headset facing outward, and outside-in tracking, which uses external sensors directed towards the headset. Insideout devices can have maximum measurement deviations exceeding 12.0 mm, whereas outside-in systems generally show measurement errors as low as 0.4 mm (30). Among the studies reviewed, nine employed outside-in tracking systems, in contrast to four using the less accurate inside-out tracking. Notably, two of these studies utilized the Meta Quest’s built-in hand tracking, potentially introducing an additional positional error of up to 1.1 cm in fingertip location (31), significantly impacting results as demonstrated by Kohm et al., where positional offset results ranged from -2.1 to 0.5 cm (32).
The HTC Vive Tracker, an outside-in tracking system, was used in four studies, where it was noted that the maximum absolute distance error in a constrained area could reach up to 14.9 mm (33).
Various technological complications impacting data integrity and results accuracy were reported. Burns et al. experienced the loss of six datasets due to software malfunctions (21). Ogawa et al. attributed biases in their results to the absolute error in hand position estimation by the inside-out Leap Motion Controller (26). Kohm et al. relied solely on the headset’s gyroscopes to approximate gaze direction, operating the HMD without a tether, which could affect immersion and outcomes (32). Clarence et al. speculated that the VR setup and specific methods of hand redirection might influence the reported detection limits (23).
Further, technological issues extended beyond VR tracking. Ogawa et al. observed an unintended hint given to participants estimating their pulse due to the pressure from a pulse oximeter, which might have influenced their actual results (26). A second study from the same laboratory reported varied results based on gender, speculating that the increased impact of electrical stimulation on women could explain differences in offset tolerance, without accounting for potential variations in VR experience by gender (28).
4 Discussion
The purpose of this systematic review was to explore the current knowledge about the threshold of the virtual hand positional offset, i.e. the limits in which the user perceives the virtual hand as their own and factors influencing it, including the effect of various hardware setups. Matching between actual and virtual limbs is, together with optimal self-presentation, a crucial aspect of VR immersion and, therefore, presence. Understanding offset detection limits may help to create more immersive VR interventions and set a baseline for research on body ownership in various clinical populations, which could open new avenues for diagnostic VR paradigms.
The most important finding of this review is that reported detection thresholds for a positional discrepancy between a virtual and a physical hand are very heterogeneous and depend on several factors. It seems that even in healthy individuals, awareness of physical hand boundaries is not rigid, depends on several factors, and can probably be successfully manipulated.
Regarding absolute values, the threshold for detecting positional offsets ranges from a few millimeters to 42 cm for linear shifts and from 2° to 45°for angular offsets. However, these values likely overestimate true inter-individual variability, as they are influenced by multiple factors, including experimental setups, multisensory integration mechanisms (34), individual participant characteristics, and hardware specifications.
Firstly, misalignment detection seems to be influenced by the direction of discrepancies and their changes over time. Sensitivity appears to be highest within the X-axis (horizontal left-right), followed by the Z-axis (horizontal farther-closer) and the Y-axis (vertical). Additionally, continuously increasing shifts were found to be less detectable than those that increase instantaneously (25). Additionally, higher sensitivity to positional offsets was found for a dominant hand. Furthermore, shifts closer to the body are more noticeable than those farther away. Consequently, there is greater leeway for matching between the virtual and real limb when reaching outward. This implies that requirements for matching should be more stringent in experimental paradigms or interventions focusing on actions close to the body, as compared to applications like VR fitness or VR exercise programs, that were lately utilized to improve quality of life in elderly (35) or in depression and anxiety (36).
The second group of influencing factors underscores the well-established role of multisensory integration in self-location (37). These factors should be considered when designing paradigms that explore body ownership or agency, as well as in VR interventions. Visually, a more accurate representation of a human hand allowed for a greater tolerance to positional offsets compared to abstract shapes. However, the role of haptic feedback is more complex. Intriguingly, corresponding haptic feedback reduced the detection threshold—meaning it necessitated a more precise match between the hand and its virtual representation. Conversely, sensory distractions such as false haptic feedback and noisy tendon electrical stimulation seemed to increase the threshold for offset detection. Unfortunately, the role of passive haptics remains unclear, as it was implemented in only three studies. It is plausible to hypothesize that while the use of physical props can enhance VR presence (38), their impact might be similar to that of matching haptic feedback, thereby heightening the matching requirements. While multisensory integration is important in the neuroscientific understanding of this topic, it was not a primary focus in the reviewed studies. Therefore, the correlation between the characteristics of positional offset detection and multisensory integration should be considered putative rather than established.
The third set of variables influencing offset sensitivity relates to inter-individual variability. Unfortunately, the effect of gender remains unclear due to ambiguous results. Similarly, the influences of age and VR experience cannot be clearly established; only a single study explored these factors, finding that while age and VR experience have a statistically significant influence, they account for only minimal variability among individuals (23). Concerning handedness, right-handed individuals appeared to be more sensitive to positional shifts than left-handed individuals. However, the representation of left-handed individuals in studies was exceptionally low, ranging from 1 to 4, and they constituted a negligible fraction of participants, if included at all (in 5 out of 13 studies). Consequently, drawing any meaningful conclusions about the influence of handedness is challenging.
An interesting finding was reported by Kohm et al., suggesting that sensitivity to positional offsets seems to be stable over time (32). This stability implies that the sensitivity to detecting misalignment between virtual and real hands may be a trait characteristic of an individual. This aligns with observations of an aberrant sense of body ownership and agency in certain neuropsychiatric disorders, notably schizophrenia (12). If confirmed, and if it varies with the clinical state, this observation could pave the way for using positional offset detection as a diagnostic tool.
Other factors might also influence the detection of positional offsets, though their impacts have not been thoroughly investigated in the reviewed studies. One such example is the complexity of a task. Studies generally employed simple paradigms such as target-touching tasks, grasping and placing objects, or following along virtual shapes. Only a single study introduced a more complex setup: Burns et al. required participants to engage in a game demanding high concentration, which could have affected their final detection thresholds—these were notably higher compared to other studies (21). It is important to mention that the applied offsets were constantly increasing, and therefore more challenging to detect (25).
An essential factor to consider is the accuracy and technological capabilities of VR equipment, which have dramatically improved over time. The evolution from the early 2000s’ Virtual Research Systems V8 HMD, featuring a resolution of 640x480 pixels per eye and a 60° field of view, to the more recent Meta Quest 2 HMD, with its 1832x1920 pixels per eye and 90°field of view, underscores the rapid technological progress in this field. This advancement is detailed in Table 6, summarizing the technological parameters of HMDs used in the reviewed studies. Results should be evaluated within this context of continual technological improvement.
4.1 Limitations of the reviewed studies
Several limitations within the presented studies may have impacted the quality and, consequently, the reliability of the results.
A notable portion of the source material comprises conference papers, with 10 out of 13 research studies reported in this format. However, the majority of these conference papers (7 out of 10) underwent peer review. This aspect adds a layer of credibility, though the consistency of review processes for conference papers can vary compared to journal publications.
A fundamental issue is the lack of a uniform definition of the offset detection threshold across studies. Most research employed the concept of the point of subjective equality, defined as the minimum difference between two stimuli recognizable as distinct with a 50% accuracy rate (39). However, not all reviewed studies adhered to this definition, introducing variability in how offset detection thresholds were quantified.
From a methodological perspective, the sample sizes in most studies were small, with only two studies conducting a power analysis (26, 28). Additionally, in several studies examining differences between various conditions, p-values were insufficiently reported. Reporting on demographic variables was also often incomplete; key characteristics such as handedness or age were missing in seven studies. Predominantly right-handed subjects were recruited, and most experiments focused on the dominant hand. The lack of assessment concerning technological familiarity due to previous VR experience might also significantly influence the results. Although methodological and reporting issues are common in the field of VR utilization in psychiatry (40), these factors collectively hinder the ability to generalize or draw firm conclusions about the factors influencing offset detection.
Furthermore, while matching and self-presentation are recognized as crucial factors in immersion and presence, measures of presence or avatar embodiment were employed inconsistently. Only five studies utilized a measure of presence or avatar embodiment, three tested the feeling of presence within the virtual environment, and only three included embodiment questionnaires. A proprioceptive drift self-localization task was performed in just two studies. Both presence and embodiment measures were included in only two studies. This scarcity of data represents a missed opportunity to explore the interrelations between offset detection, immersion, and presence, which are vital aspects of VR interventions.
Lastly, the impact of technological and methodological factors on experimental results should not be overlooked. These factors include, but are not limited to, errors in positional tracking, data losses due to software malfunctions, biases arising from incorrect equipment placement or assembly, and inconsistencies in spatial anchoring of participants with data cables. To illustrate these challenges, in a study by Burns et al. (21), complete data were available for only 19 out of 40 participants, highlighting the significant effect such issues can have on the integrity and interpretability of research findings.
4.2 Limitations of the systematic review
The limitations of this systematic review should be acknowledged. The review is confined to reports published in English and was conducted across only two databases, with the search query limited to titles, abstracts, and keywords. Despite the intention to use the most general terms, it is possible that some relevant reports were not identified. Furthermore, during the screening of results, there is a chance that some reports meeting the eligibility criteria may have been inadvertently overlooked. Additionally, the diversity of methods used across the reported studies complicates the task of standardizing the limits for acceptable positional offsets.
4.3 Recommendations for experimental design
The insights from studies on offset detection can significantly inform and enhance the experimental designs and reporting practices for VR assessments in mental health research. Utilizing detection thresholds as a practical, technology-agnostic proxy measure allows for the comparison of results across various VR headsets, essential due to the rapid advancements and frequent updates in VR technology. This approach not only addresses the variability introduced by differing technologies but also improves the robustness and applicability of research findings in real-world clinical settings.
In studies exploring positional offsets, body ownership and multisensory integration, it may be recommended to utilize more precise outside-in detection systems than built-in inside-out systems. Calibration of equipment for each individual user, as recommended by Zenner et al. (25) and implemented by Benda et al. (22), is advised to enhance data reliability. Additionally, procedural unification could be further advanced by incorporating standardized questionnaires such as the Virtual Embodiment Questionnaire (VEQ) or the Igroup Presence Questionnaire (IPQ), which provide a foundation for comparable data across different studies.
5 Conclusion
Proper alignment between a virtual and a real hand is critical for immersion and presence in virtual reality (VR), which in turn could influence the efficacy of VR mental health interventions. This systematic review reveals a surprisingly wide tolerance for detecting positional offsets within a virtual environment among healthy individuals. While the full extent of this tolerance is not yet completely understood, it appears to be significantly shaped by the design of the application itself, such as the nature of misalignment and the depiction of the virtual hand, and to a lesser extent, by individual factors. Additionally, the accuracy of VR devices plays a crucial role in influencing perception and the requirements for appropriate matching.
The identified influencing factors must be carefully considered to ensure the highest possible user presence in forthcoming therapeutic approaches. Furthermore, the variation in VR device capabilities underscores the importance of reporting device properties, particularly tracking sensitivity, in future research and VR interventions.
Lastly, the existence of individual variability in detecting positional offsets underscores the potential of VR as an optimal tool for researching body ownership and multisensory integration. This has significant implications for developing diagnostic applications, particularly for disorders like schizophrenia, where such research can provide valuable insights.
Data availability statement
The original contributions presented in the study are included in the article/Supplementary Material. Further inquiries can be directed to the corresponding author.
Author contributions
DA: Conceptualization, Data curation, Investigation, Methodology, Visualization, Writing – original draft, Writing – review & editing. TŠ: Data curation, Formal analysis, Investigation, Writing – original draft, Writing – review & editing. JH: Validation, Writing – original draft, Writing – review & editing. EB: Data curation, Formal analysis, Funding acquisition, Supervision, Validation, Writing – original draft, Writing – review & editing.
Funding
The author(s) declare financial support was received for the research, authorship, and/or publication of this article. Supported by the Ministry of Health, Czech Republic - conceptual development of research organization (FNBr, 65269705) and by Masaryk University, Brno, Czech Republic - Specific research - Support for student projects (MUNI/A/1609/2023).
Acknowledgments
The authors acknowledge the assistance of generative AI technology (Grammarly and ChatGPT 4o model) exclusively for grammar and style editing. No AI tools were used in the data extraction, data summarization or initial text drafting processes.
Conflict of interest
JH reports grants from the Ministry of Health of the Czech Republic and the Ministry of Education, Youth and Sports of the Czech Republic and honoraria from Angelini Pharma S.p.a. nd clinical research for Ketabon GmbH. EB reports grants from the Ministry of Health of the Czech Republic and Ministry of Education, Youth and Sports of the Czech Republic, honoraria from Angelini Pharma S.p.a., H. Lundbeck A/S., consultations and lecturing for Johnson & Johnson and clinical research for Janssen Pharmaceuticals and Ketabon GmbH.
The remaining authors declare that the research was conducted in the absence of any commercial or financial relationships that could be construed as a potential conflict of interest.
Publisher’s note
All claims expressed in this article are solely those of the authors and do not necessarily represent those of their affiliated organizations, or those of the publisher, the editors and the reviewers. Any product that may be evaluated in this article, or claim that may be made by its manufacturer, is not guaranteed or endorsed by the publisher.
Supplementary material
The Supplementary Material for this article can be found online at: https://www.frontiersin.org/articles/10.3389/fpsyt.2024.1455495/full#supplementary-material
References
1. Rothbaum BO, Hodges L, Watson BA, Kessler GD, Opdyke D. Virtual reality exposure therapy in the treatment of fear of flying: A case report. Behav Res Ther. (1996) 34:477–81. doi: 10.1016/0005-7967(96)00007-1
2. Berkman MI. History of Virtual Reality. New York City - USA: Springer International Publishing (2024) p. 873–81. doi: 10.1007/978-3-031-23161-2169
3. Witmer BG, Singer MJ. Measuring presence in virtual environments: A presence questionnaire. Presence: Teleoperators Virtual Environments. (1998) 7:225–40. doi: 10.1162/105474698565686
4. Segawa T, Baudry T, Bourla A, Blanc JV, Peretti CS, Mouchabac S, et al. Virtual reality (vr) in assessment and treatment of addictive disorders: A systematic review. Front Neurosci. (2020) 13:1409. doi: 10.3389/fnins.2019.01409
5. Slater M. Measuring presence: A response to the witmer and singer presence questionnaire. Presence: Teleoperators Virtual Environments. (1999) 8:130–44. doi: 10.1162/105474699566477
6. Botvinick M, Cohen J. Rubber hands ‘feel’ touch that eyes see. Nature (1998) 8:756. doi: 10.1038/35784
7. Blanke O. Multisensory brain mechanisms of bodily self-consciousness. Nat Rev Neurosci. (2012) 13:556–71. doi: 10.1038/nrn3292
8. Serino A, Alsmith A, Costantini M, Mandrigin A, Tajadura-Jiménez A, Lopez C. Bodily ownership and self-location: Components of bodily self-consciousness. Consciousness Cogn. (2013) 22:1239–52. doi: 10.1016/j.concog.2013.08.013
9. Gallagher S. Philosophical conceptions of the self: Implications for cognitive science. Trends Cogn Sci. (2000) 4:14–21. doi: 10.1016/S1364-6613(99)01417-5
10. Blanke O, Metzinger T. Full-body illusions and minimal phenomenal selfhood. Trends Cogn Sci. (2009) 13:7–13. doi: 10.1016/j.tics.2008.10.003
11. Vogeley K, Fink GR. Neural correlates of the first-person-perspective. Trends Cogn Sci. (2003) 7:38–42. doi: 10.1016/S1364-6613(02)00003-7
12. Baum K, Hackmann J, Pakos J, Kannen K, Wiebe A, Selaskowski B, et al. Body transfer illusions in the schizophrenia spectrum: a systematic review. Schizophrenia (2022) 8:103. doi: 10.1038/s41537-022-00314-z
13. Bekrater-Bodmann R, Chung BY, Foell J, Gescher DM, Bohus M, Flor H. Body plasticity in borderline personality disorder: A link to dissociation. Compr Psychiatry. (2016) 69:36–44. doi: 10.1016/j.comppsych.2016.05.002
14. Eshkevari E, Rieger E, Longo MR, Haggard P, Treasure J. Increased plasticity of the bodily self in eating disorders. psychol Med. (2012) 42:819–28. doi: 10.1017/S0033291711002091
15. Macpherson MC, Birladeanu A, Miles LK. Examining the relationship between subclinical levels of social anxiety and the rubber hand illusion. Acta Psychologica. (2021) 212:103209. doi: 10.1016/j.actpsy.2020.103209
16. Rabellino D, Burin D, Harricharan S, Lloyd C, Frewen PA, McKinnon MC, et al. Altered sense of body ownership and agency in posttraumatic stress disorder and its dissociative subtype: A rubber hand illusion study. Front Hum Neurosci. (2018) 12:163. doi: 10.3389/fnhum.2018.00163
17. Cascio CJ, Foss-Feig JH, Burnette CP, Heacock JL, Cosby AA. The rubber hand illusion in children with autism spectrum disorders: Delayed influence of combined tactile and visual input on proprioception. Autism. (2012) 16:406–19. doi: 10.1177/1362361311430404
18. Page MJ, McKenzie JE, Bossuyt PM, Boutron I, Hoffmann TC, Mulrow CD, et al. The prisma 2020 statement: An updated guideline for reporting systematic reviews. BMJ (2021) 2021:372. doi: 10.1136/bmj.n71
19. Deligiannidis L, McConnell DS, Vallee C. Visual and proprioceptive integration of the virtual and real fingertips. 2009 2nd Conference on Human System Interactions (2009) 2:190–5. doi: 10.1109/HSI.2009.5090977
20. Zenner A, Kruqer A. Estimating detection thresholds for desktop-scale hand redirection in virtual reality. 2019 IEEE Conference on Virtual Reality and 3D User Interfaces (VR) (2019) 2019:47–55. doi: 10.1109/VR.2019.8798143
21. Burns E, Razzaque S, Panter AT, Whitton MC, McCallus MR, Brooks FP. The hand is more easily fooled than the eye: Users are more sensitive to visual interpenetration than to visual-proprioceptive discrepancy. Presence: Teleoperators Virtual Environ. (2006) 15:1–15. doi: 10.1162/pres.2006.15.1.1
22. Benda B, Esmaeili S, Ragan ED. Determining detection thresholds for fixed positional offsets for virtual hand remapping in virtual reality. 2020 IEEE International Symposium on Mixed and Augmented Reality (ISMAR) (2020) 2020:269–78. doi: 10.1109/ISMAR50242.2020.00050
23. Clarence A, Knibbe J, Cordeil M, Wybrow M. Investigating the effect of direction on the limits of haptic retargeting. 2022 IEEE International Symposium on Mixed and Augmented Reality (ISMAR) (2022) 2022:612–21 doi: 10.1109/ISMAR55827.2022.00078
24. Gonzalez EJ, Follmer S. Investigating the detection of bimanual haptic retargeting in virtual reality. VRST '19: Proceedings of the 25th ACM Symposium on Virtual Reality Software and Technology (2019) 25:1–5. doi: 10.1145/3359996.3364248
25. Zenner A, Heqitz KP, Kruger A. Blink-suppressed hand redirection. 2021 IEEE Virtual Reality and 3D User Interfaces (VR) (2021) 2021:75–84. doi: 10.1109/VR50410.2021.00028
26. Ogawa N, Narumi T, Hirose M. Effect of avatar appearance on detection thresholds for remapped hand movements. IEEE Trans Visualization Comput Graphics. (2021) 27:3182–97. doi: 10.1109/TVCG.2020.2964758
27. Lee Y, Jang I, Lee D. Enlarging just noticeable differences of visual-proprioceptive conflict in vr using haptic feedback. 2015 IEEE World Haptics Conference (WHC) (2015) 2015:19–24. doi: 10.1109/WHC.2015.7177685
28. Ogawa M, Matsumoto K, Aoyama K, Narumi T. Expansion of detection thresholds for hand redirection using noisy tendon electrical stimulation. 2023 IEEE International Symposium on Mixed and Augmented Reality (ISMAR) (2023) 2023:1026–35. doi: 10.1109/ISMAR59233.2023.00119
29. Yang X, Zhang Y, Yang X. Redirected placement: Evaluating the redirection of passive props during reach-to-place in virtual reality. VRST '23: Proceedings of the 29th ACM Symposium on Virtual Reality Software and Technology (2023) 29:1–11. doi: 10.1145/3611659.3615688
30. Fellbach VDCV. Werkstattbericht 2: Head-mounted displays - messung räumlicher präzision bei vr-trackingsystemen. Fellbach/Stuttgart - Germany: Tech. rep., Virtual Dimension Center (VDC) Fellbach (2021).
31. Abdlkarim D, Luca MD, Aves P, Maaroufi M, Yeo SH, Miall RC, et al. A methodological framework to assess the accuracy of virtual reality hand-tracking systems: A case study with the meta quest 2. Behav Res Methods. (2024) 56:1052–63. doi: 10.3758/s13428-022-02051-8
32. Kohm K, Porter J, Robb A. Sensitivity to hand offsets and related behavior in virtual environments over tim. ACM Trans Appl Percept. (2022) 19:1–15. doi: 10.1145/3561055
33. Luckett E. A quantitative evaluation of the htc vive for virtual reality research. (Honors Theses). Department Comput Inf Sci. (2018) 331. Available online at: https://egrove.olemiss.edu/hon_thesis/331 (Accessed June 26, 2024).
34. Tsakiris M. The multisensory basis of the self: From body to identity to others. Q J Exp Psychol. (2017) 70. doi: 10.1080/17470218.2016.1181768
35. Wang LT. Effects of semi-immersive virtual reality exercise on the quality of life of communitydwelling older adults: Three-month follow-up of a randomized controlled trial. Digital Health. (2024) 10. doi: 10.1177/20552076241237391
36. Turoń-Skrzypińska A, Tomska N, Mosiejczuk H, Rył A, Szylińska A, Marchelek-Myśliwiec M, et al. Impact of virtual reality exercises on anxiety and depression in hemodialysis. Sci Rep. (2023) 13:12435. doi: 10.1038/s41598-023-39709-y
37. Ionta S, Heydrich L, Lenggenhager B, Mouthon M, Fornari E, Chapuis D, et al. Multisensory mechanisms in temporo-parietal cortex support self-location and first-person perspective. Neuron. (2011) 70:363–74. doi: 10.1016/j.neuron.2011.03.009
38. Insko B, Insko B. Passive haptics significantly enhances virtual environments. (PhD Thesis). Computer. (2001). doi: 10.5555/933178
39. Allin S, Matsuoka Y, Klatzky R. Measuring just noticeable differences for haptic force feedback: Implications for rehabilitation. Proceedings 10th Symposium on Haptic Interfaces for Virtual Environment and Teleoperator Systems. HAPTICS 2002 (2002) 10:299–302. doi: 10.1109/HAPTIC.2002.998972
40. Bartečků E, Hořínková J. Virtual reality in psychiatry: Literature review — virtuální realita v psychiatrii: Přehled literatury. Ceska Slovenska Psychiatr. (2020) 116:150–8. Available online at: http://www.cspsychiatr.cz/detail.php?stat=1334 (Accessed June 26, 2024).
41. Gescheider G. Psychophysics: Method, Theory, and Application. Abingdon - UK: Taylor & Francis Group (1985).
42. Zenner A, Karr C, Feick M, Ariza O, Krüger A. The detectability of saccadic hand offset in virtual reality. VRST '23: Proceedings of the 29th ACM Symposium on Virtual Reality Software and Technology (2023) 29:1–2. doi: 10.1145/3611659.3617223
43. Slater M, Steed A, Usoh M. Depth of presence in immersive virtual environments article in presence teleoperators & virtual environments. Presence: Teleoperators Virtual Environments (1994) 3:130–44. doi: 10.1162/pres.1994.3.2.130
44. Gonzalez-Franco M, Peck TC. Avatar embodiment. towards a standardized questionnaire. Front Robot AI. (2018) 5:74. doi: 10.3389/frobt.2018.00074
45. Cheng LP, Ofek E, Holz C, Benko H, Wilson AD. Sparse haptic proxy: Touch feedback in virtual environments using a general passive prop. CHI '17: Proceedings of the 2017 CHI Conference on Human Factors in Computing Systems (2017) 2017:3718–28. doi: 10.1145/3025453.3025753
46. Roth D, Latoschik ME. Construction of the virtual embodiment questionnaire (veq). IEEE Trans Visualization Comput Graphics. (2020) 26:3546–56. doi: 10.1109/TVCG.2020.3023603
Keywords: virtual reality, body ownership, hand redirection, bodily self-consciousness, self-location, just noticable difference, point of subjective equality, detection threshold
Citation: Antoš D, Švec T, Hořínková J and Bartečková E (2025) Borders of physical self in virtual reality: a systematic review of virtual hand position discrepancy detection. Front. Psychiatry 15:1455495. doi: 10.3389/fpsyt.2024.1455495
Received: 26 June 2024; Accepted: 27 November 2024;
Published: 06 January 2025.
Edited by:
Ana Manzano-León, University of Almeria, SpainReviewed by:
Maria Bianca Amadeo, Italian Institute of Technology (IIT), ItalyPaula Rodríguez-Rivera, University of Vigo, Spain
Copyright © 2025 Antoš, Švec, Hořínková and Bartečková. This is an open-access article distributed under the terms of the Creative Commons Attribution License (CC BY). The use, distribution or reproduction in other forums is permitted, provided the original author(s) and the copyright owner(s) are credited and that the original publication in this journal is cited, in accordance with accepted academic practice. No use, distribution or reproduction is permitted which does not comply with these terms.
*Correspondence: Eliška Bartečková, QmFydGVja292YS5FbGlza2FAZm5icm5vLmN6