- 1Laboratorio de Neurociencias, Subdirección de Medicina Experimental, Instituto Nacional de Pediatría, Ciudad de México, Mexico
- 2Departamento de Fisiología “Mauricio Russek”, Escuela Nacional de Ciencias Biológicas, Instituto Politécnico Nacional, Ciudad de México, Mexico
- 3Laboratorio de Psicoinmunología, Instituto Nacional de Psiquiatría Ramón de la Fuente Muñíz, Ciudad de México, Mexico
Hypothyroidism is a prevalent thyroid condition in which the thyroid gland fails to secrete an adequate amount of thyroid hormone into the bloodstream. This condition may develop due to genetic or acquired factors. The most frequent cause of acquired hypothyroidism is chronic autoimmune thyroiditis, also known as Hashimoto’s disease. Acquired hypothyroidism is diagnosed when patients present with overt hypothyroidism (also known as clinical hypothyroidism), as they exhibit increased TSH and decreased T3 and T4 serum levels. This article examines the prevalence of psychiatric disorders among patients diagnosed with acquired hypothyroidism with or without Levothyroxine treatment. We discuss the available evidence indicating that acquired hypothyroidism may be a risk factor for psychiatric disorders, and the effectiveness of thyroid treatment in relieving psychiatric symptoms. Additionally, we provide critical details on thyroid hormone cutoff values reported in the literature, their potential clinical importance, and their correlation with psychiatric symptoms. Finally, we examined the various mechanisms by which acquired hypothyroidism can lead to depression. The high rate of comorbidity between hypothyroidism and psychiatric disorders deserves special attention, indicating the importance of consistent monitoring and timely identification of psychiatric symptoms to prevent disease exacerbation and facilitate therapeutic management. On the other hand, several mechanisms underlie the strong association between depression and acquired hypothyroidism. Deeper research into these mechanisms will allow knowledge of the pathophysiology of depression in patients with acquired hypothyroidism and will provide clues to design more precise therapeutic strategies for these patients.
Highlights
● Acquired hypothyroidism increases the risk of depression and anxiety in adult patients with or without L-T4 treatment.
● Acquired hypothyroidism may lead to depression by multiple mechanisms, including alterations in serotonergic neurotransmission or the HPA axis, changes in adult hippocampal neurogenesis or brain region integrity, activation of systemic pro-inflammatory processes or neuroinflammation, or triggering of the kynurenine pathway.
● Several lines of research should be undertaken to investigate whether acquired hypothyroidism is a risk factor for the development of schizophrenia or bipolar disorder, or for the development of psychiatric disorders in children and adolescents.
1 Introduction
Hypothyroidism is a medical condition resulting from inadequate production of thyroid hormones (THs), often caused by thyroid gland dysfunction. According to Usman et al. (2023), hypothyroidism is diagnosed when thyroid stimulating hormone (TSH), triiodothyronine (T3), and thyroxine (T4) are >4.94 μIU/mL, <1.71 pg/mL, and <0.7 ng/dL, respectively (1). This condition results in clinical complications and disturbances in the physiological maintenance of bodily functions (2). Hypothyroidism can manifest because of either genetic or acquired factors. The latter can affect individuals during childhood, adolescence, or adulthood, and develops from sources other than genetic ones (2, 3). The most frequent cause of acquired hypothyroidism is chronic autoimmune thyroiditis, more commonly known as Hashimoto’s disease. In addition to other causes, such as iodine deficiency, thyroidectomy, radioactive iodine therapy, radiation (4), viral infections (5), and drug use (6). In low-income countries, iodine deficiency is the leading cause of acquired hypothyroidism, whereas, in more developed countries (where foods are enriched and fortified with iodine), autoimmune thyroiditis is the predominant cause (6).
Acquired hypothyroidism is diagnosed when patients present with overt hypothyroidism (also known as clinical hypothyroidism), as they exhibit increased TSH and decreased T3 and T4 serum levels (TSH >4.94 μIU/mL; T3 <1.71 pg/mL; T4 <0.7 ng/dL). The prevalence of this disease may differ based on gender, age, or geographic location (7, 8). In the United States, in adolescent and adult populations, the prevalence of acquired hypothyroidism ranged between 2.1 to 6.1% depending on the criteria for defining hypothyroidism. Moreover, a higher occurrence in women was reported (8). In European countries, this prevalence was 0.37% with predominance in females (9). In Mexico, it was reported between 1.2–1.8% in the adult population (10, 11).
The close relationship between hypothyroidism and psychiatric symptoms has been the subject of extensive research. The evidence indicates an association between thyroid function and psychiatric disorders, including anxiety, depression, bipolar disorder, and schizophrenia (12, 13). Most clinical studies and previous reviews have focused on describing thyroid alterations in patients with psychiatric disorders, and the effects of psychiatric drugs on the thyroid endocrine system (12, 14, 15). In contrast, there are fewer scientific studies regarding the prevalence of psychiatric disorders in patients initially diagnosed with acquired hypothyroidism. Additionally, only a minority of investigations examine the effectiveness of Levothyroxine (L-T4) treatment in mitigating or alleviating psychiatric disorders in these kinds of patients.
A pioneering study, published in the 60´s decade, showed that hypothyroidism could be causing psychiatric disorders. The study reported eight patients with both concurrent hypothyroidism and mental illness; six of them recovered with treatment consisting of consumption of thyroid gland or dried thyroid tissue and showed normal mental status in the medium term (from 2 to 12 years after the event) (16). It was suggested that hypothyroidism acts as an inducer of mental changes and that thyroid medication could be relevant to the recovery of mental health. Recent evidence continues supporting this notion, the studies were compiled in this review to answer whether the acquired hypothyroidism acts as a risk factor for psychiatric disorders in patients with or without thyroid medication, and whether the L-T4 treatment is successful in alleviating the psychiatric illness. In addition, we briefly address the possible mechanisms underlying the establishment of depression in patients with acquired hypothyroidism providing key points that should be considered for future research.
2 Methods
The search criteria, including the literature inclusion and exclusion criteria, as well as the limitations, are described in detail in the Supplementary Material.
3 Depression in patients with acquired hypothyroidism
Depression is classified as a mood disorder that presents with persistent sadness, emptiness or irritable mood, loss of interest in daily activities, hopelessness, difficulty experiencing pleasure, as well as disturbances in sleeping and eating (17, 18). According to the World Health Organization (WHO), one in eight people worldwide has a mental disorder, and depression affects 280 million people (19).
Depressive symptoms have a high prevalence in patients with hypothyroidism, and the study of this close relationship between the two conditions has aroused great interest in both the psychiatric and endocrinological fields. One of the greatest concerns in patients with acquired hypothyroidism is the imminent risk of developing depression, which will worsen the patient’s condition and its therapeutic management. Based on different studies (8–11), the prevalence of acquired hypothyroidism is reported between 0.3 and 6.1%, whereas depression can afflict up to 18% of the population, according to Lee’s findings (2023) (20). Even more interestingly, patients with acquired hypothyroidism have a high prevalence of depression reaching 79.2% in patients with hypothyroidism without treatment (21), between 12.1 and 36.7% in patients under L-T4 treatment (1, 22, 23), and between 33.3 and 66.7% in patients who had developed an euthyroid state after L-T4 treatment (See Table 1) (24, 25). This high prevalence of depression in patients with acquired hypothyroidism, with or without thyroid treatment, is in line with a higher risk of developing this psychiatric illness.
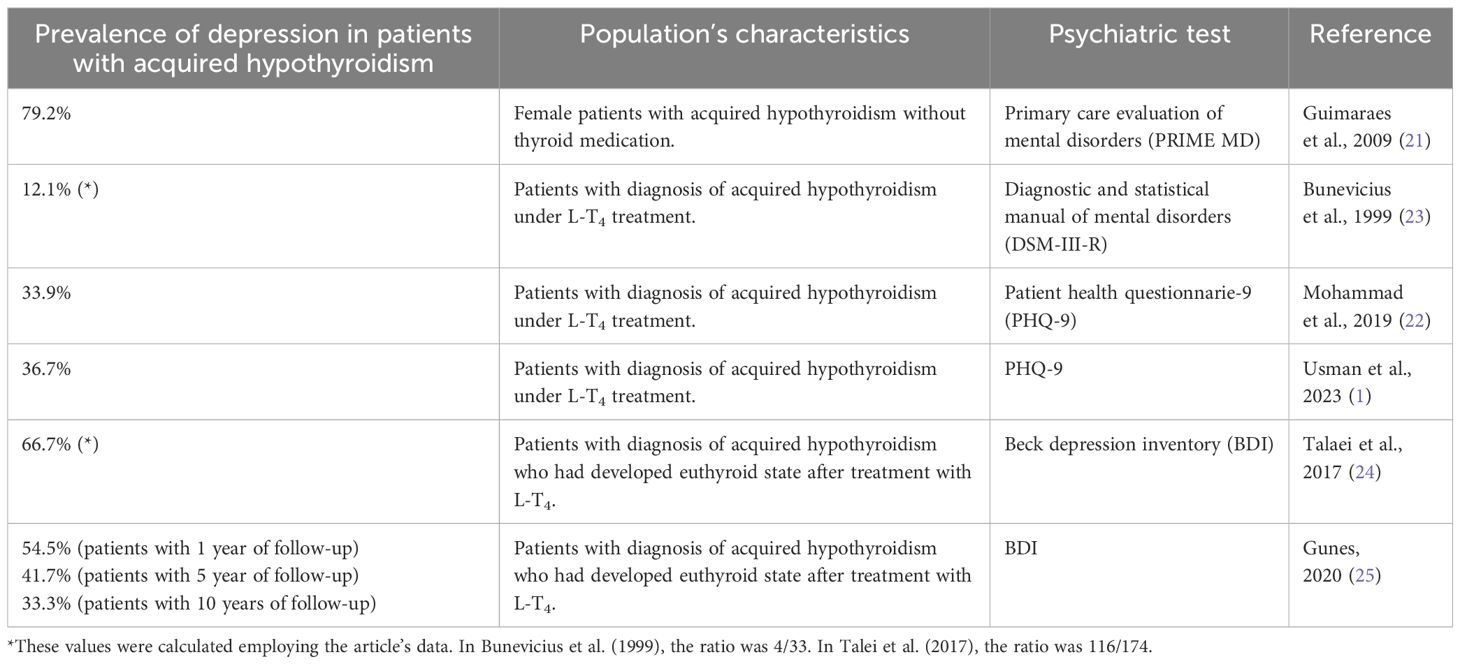
Table 1 Prevalence of depression in patients with acquired hypothyroidism without or with L-T4 treatment.
3.1 Depression in patients with acquired hypothyroidism without thyroid medication
Ittermann et al. (2015) showed that patients with acquired hypothyroidism, not taking medication, have 2.10 (95% CI, 0.86–5.11) or 2.32 (95% CI, 1.28–4.21) times higher risk of developing major depressive disorder according to data obtained by the Munich composite international diagnostic interview (M-CIDI) or Beck depression inventory II (BDI-II), respectively (26). Interestingly, this risk seems to be more if only women are evaluated. Guimaraes’ research in women with overt hypothyroidism (TSH>4 μIU/mL and free T4<0.7 ng/dL) found that they face 8.7 times (95% CI, 2.56–29.50) higher risk of experiencing depression than euthyroid women (21). The authors used Spitzer et al.’s (1994) PRIME-MD test (27) instead of the Beck depression inventory (BDI) for the assessment of depressive symptoms (21). Regarding the severity of depression, the literature shows that depressive symptoms were more severe in patients with overt hypothyroidism than control subjects or patients evaluated after receiving L-T4 treatment, based on elevated scores in the Hamilton depression rating scale (HDRS) and BDI test (28, 29).
3.2 Depression in patients with acquired hypothyroidism with thyroid medication
Regarding patients with acquired hypothyroidism under thyroid medication, Thvilum et al. (2014) found that, after the diagnosis of hypothyroidism, these patients were more likely to develop depression and require antidepressant treatment (HR: 1.30, 95% CI: 1.15–1.47) (30). The severity of their depressive stage was predominantly mild or moderate-severe. Mohammad et al. (2019) showed that depression was moderate in 10.7%, moderate-severe in 19.6%, and severe in 3.6% of these patients (22). In line, Usman et al. (2023) showed that such patients experienced mild, moderate, moderate-severe, and severe depressive symptoms in 44.4%, 33.3%, 16.7%, and 5.6% of cases, respectively (1). Both authors determined the severity of depression using the patient health questionnaire-9 (PHQ-9) (1, 22).
Clinical studies in patients receiving L-T4 treatment have been also useful to study the relationship between TSH levels and depression severity, suggesting a positive correlation between elevated TSH levels and more severe depression (21, 24). Talaei et al. (2017) proposed a cut-off value of TSH for the evaluation and consideration of antidepressant treatment in patients with hypothyroidism treated with L-T4 (24). They showed that a TSH cut-off point of ≥2.5 mIU/L is associated with a depression diagnosis, displaying 89.6% sensitivity (95% CI, 82.6–94.5) and 87.9% specificity (95% CI, 76.7–95.0). Additionally, the study found that a cut-off point of ≥4mIU/L indicated severe depression with 80.5% (95% CI, 64.0–91.8) and 95% (95.6% CI, 90.8–98.4) sensitivity and specificity, respectively. It’s worth noting that the patients with hypothyroidism in Talaei et al.’s (2017) study were under treatment with L-T4, with a mean age of 45.5 ± 11.7 years, and had mean T3 and T4 values of 1.2 ng/mL and 8.4 pg/dL, respectively. In addition, BDI score above 10 was used as a criterion for depression diagnosis (24). A study conducted by Guimaraes et al. (2009) revealed that 65% of women (mean age of 53.6 years) with TSH levels ≥10 μIU/mL exhibited depressive symptoms and had a 3-fold (95% CI, 1.21–7.79) higher likelihood of developing depression compared to those with normal TSH levels (>0.3 and ≤4 μIU/mL) (21).
Finally, it can be inferred that there is a close association between thyroid dysfunction and the onset of depressive symptoms. Some studies have even delved into the connection between the hypothyroid condition and a higher incidence of suicidal behavior. In a meta-analysis conducted by Toloza et al. (2021), it was reported that patients with suicidal behavior have significantly lower levels of free T3 and total T4 as compared to patients without suicidal behavior (31). The authors clarify that research on the correlation between thyroid disorders and suicidal behavior is limited, warranting further investigation for improved understanding.
Thus, individuals with acquired hypothyroidism, whether treated or not, are more susceptible to depression, according to current evidence. It is important to consistently screen these patients for depressive symptoms to manage this comorbid psychiatric condition and prevent further complications.
4 Anxiety disorder in patients with acquired hypothyroidism
Anxiety disorder is characterized by excessive fear, anxiety, and related behavioral disturbances. Fear is the emotional response to a real or perceived imminent threat, whereas anxiety is the anticipation of future threats. Such anxious responses are disproportionate to the context or triggering stimuli and can significantly hinder a person’s daily life. Common symptoms of anxiety may comprise persistent worry, irritability, restlessness, difficulty concentrating, muscle tension, sleep disturbances, panic attacks, and avoidance of anxiety-provoking situations (18). Anxiety is a prevalent psychiatric disorder that affects 18.1% of people in the United States (32) and 301 million people worldwide (19). Interestingly, the presence of anxiety disorder has been reported in patients with acquired hypothyroidism, as evidenced by various studies in the adult population.
4.1 Anxiety in patients with acquired hypothyroidism without thyroid medication
Ittermann et al. (2015) found that individuals with a diagnosis of acquired hypothyroidism, with a mean age of 55 years and without L-T4 treatment, have a 3.98 (95% CI, 1.48–10.72) higher risk of experiencing anxiety (test: M-CIDI) (26). In addition, these patients had significantly higher scores for anxiety, as well as decreased attention and executive task performance, in comparison with control subjects (test: state-trait anxiety inventory (STAI)) (29, 33). These elevated scores for anxiety were also reported when patients with overt hypothyroidism were compared with patients under L-T4 treatment or with TSH values below 0.1 µIU/mL (test: Thyroid-related quality-of-life patient-reported outcome (ThyPRO)) (34).
4.2 Anxiety in patients with acquired hypothyroidism with thyroid medication
Thvilum et al. (2014) found that hypothyroid patients, even under L-T4 treatment, had an increased risk of developing anxiety and requiring anxiolytic treatment after diagnosis of hypothyroidism (HR: 1.27; 95% CI, 1.10–1.47) (30). Interestingly, it has been reported that even after becoming euthyroid with thyroid medication, patients had higher anxiety scores than controls at 1, 5, and 10 years of follow-up (test: Beck anxiety inventory (BAI)) (25). The patients experienced several symptoms, including hot flushes, weakness, tremors in the legs and hands, dizziness or drowsiness, palpitations, a feeling of loss of balance, becoming terrified, and flushing of the face (25). Additionally, it has been demonstrated that discontinuing thyroid medication can result in increased anxiety levels (29, 33).
The relationship between serum TSH levels and anxiety disorders has not been extensively studied. One report found that serum TSH levels and gender were the most significant predictive factors when anxiety was considered a dependent variable (34).
Overall, the literature suggests an association between hypothyroidism and anxiety, as supported by the higher risk of developing this disorder in patients with acquired hypothyroidism without or under L-T4 treatment. Discontinuation of thyroid medication in hypothyroid patients appears to be a risk factor for the development of anxiety in patients with this condition, making appropriate and timely therapeutic management and psychiatric care highly relevant.
5 Negative evidence about the relationship between acquired hypothyroidism and depression or anxiety
In contrast to other authors, Grabe et al. (2005) showed that patients with overt hypothyroidism did not differ from euthyroid controls in scores for anxiety and depression employing Zerssen complaint scale (2005) (35). The authors explain that hypothyroid patients may have a differential susceptibility to low thyroid hormone levels, with not all of them developing a psychiatric disorder.
In line, Gulseren et al. (2006) found that patients with overt hypothyroidism had similar scores for anxiety that control subjects, employing the Hamilton anxiety rating scale (HARS) (28). The authors explain that the causal relationship between thyroid dysfunction and anxiety is still speculative and that their findings need to be interpreted with caution due to the small sample size and that studies in larger populations are needed.
6 Schizophrenia or affective bipolar disorder in patients with acquired hypothyroidism
Schizophrenia is a mental disorder that is primarily characterized by a progressive decline in cognitive function and a range of psychiatric symptoms, such as hallucinations, delusions, and disorganized thinking (speech) (18, 36). On the other hand, bipolar disorder is a chronic mood disorder mainly characterized by a mixture of manic (bipolar mania), hypomanic, and depressive (bipolar depression) episodes (37, 38). Schizophrenia and bipolar disorder affect 24 and 40 million people worldwide, respectively (19), and both have been associated with hypothyroidism since the 1960s (39, 40).
In 2018, Sharif and colleagues found that individuals with hypothyroidism exhibited a significantly higher prevalence of schizophrenia (2.01%) compared to controls (1.25%). Nevertheless, the study was unable to establish a causal relationship between hypothyroidism and schizophrenia. One limitation of this study was that the etiology and type of hypothyroidism were not determined (41). In a separate study, Benros and colleagues (2011) found that a previous autoimmune disease increased the risk of schizophrenia by 29%, However, only a small number of patients presented with autoimmune thyroiditis (n=3), suggesting that there was no evidence of an association between autoimmune thyroiditis and the development of schizophrenia (42).
On the other hand, Thomsen et al. (2005) analyzed data from a Danish patient registry to investigate whether hospitalization for hypothyroidism increases the risk of developing bipolar disorder. They found that only 0.17% of hypothyroid patients were later hospitalized for bipolar disorder, which is comparable to the hospitalization rate for patients with nontoxic goiter or osteoarthritis (43).
Thus, there is insufficient evidence to conclude whether hypothyroidism is a risk factor for the development of schizophrenia or bipolar disorder. Therefore, longitudinal studies are needed to determine the incidence of these two disorders in patients initially diagnosed with acquired hypothyroidism.
7 Effectiveness of thyroid hormones for mitigating or alleviating psychiatric comorbidities in patients with acquired hypothyroidism
The role and use of THs in the treatment of psychiatric disorders have been well documented. While THs are prescribed to patients with hypothyroidism, they are also known to accelerate or enhance the efficacy of some psychiatric drugs, particularly in refractory patients without thyroid disorders (44, 45). Some of these therapeutic effects have been reported in patients who do not respond to antidepressant treatment, as well as in patients who require an accelerated effect of antidepressants. Multiple studies indicate that the administration of T3 hormone may benefit patients with depression (46–51). However, other reports suggest that the addition of L-T4 to antidepressant treatment may result in a more effective response to treatment (52–54).
Although depression is a disorder that often accompanies hypothyroidism, few reports have been conducted to determine whether the thyroid treatment reverses the depressive state in patients initially diagnosed with hypothyroidism. It has been reported that treatment of hypothyroidism with T4 alone does not improve mood (55). However, Gulseren et al. (2006) reported that hypothyroid patients with depression showed improvement after L-T4 administration, which reversed the hypothyroid state, without the need for psychiatric medication (28). Consistent with this, Rack and Makela (2000) reported a clinical case in which a patient with a long history of hypothyroidism treated with T4 maintained her depressed state, but when T3 was added to her treatment, her mood improved markedly (56). Several reports support the idea that the combination of T4+T3 prevents depression, although in some cases they report only a trend toward improvement (23, 57). In contrast, reports indicate that the combination of T4+T3 does not significantly improve depressive states in hypothyroid patients (58, 59).
Controversial evidence exists regarding the potential advantages of thyroid medication in reducing anxiety symptoms. The literature reports a benefit of adding L-T4 to anxiolytic treatment in hypothyroid patients with anxiety (28). Early initiation of L-T4 treatment is suggested to avoid anxiolytic administration (60). Monzani et al. (2023) reported that high doses of L-T4 were required to reverse anxiety in thyroidectomized patients (34). In contrast, Gunes et al. (2020) reported that anxiety may persist in hypothyroid patients even after achieving euthyroid status through thyroid medication (25).
Schizophrenia’s occurrence in hypothyroid patients, also known as myxedema psychosis, is rare. Thyroxine treatment’s efficacy has been reported in various clinical cases of this condition. A report indicates that T4 and T3 combination therapy (61) as well as T4 treatment alone improved symptoms in a woman with myxedema psychosis (62). Finally, there is no evidence indicating the effectiveness of L-T4 in treating bipolar disorder in patients who have been initially diagnosed with hypothyroidism.
Determining the efficacy of THs in psychiatric disorders is challenging due to the varying results reported in studies. This variability can be attributed to differences in the severity of thyroid disease, pharmacological treatments, and the severity of their psychiatric conditions. Additionally, factors such as gender, age, disease onset, and duration may also affect results. It is important to note that the T4 hormone must be converted to T3 in various tissues, which can be a significant limitation in some patients, increasing the variability of reported results. However, because of the strength of the reports indicating the benefit of THs in improving the symptoms of psychiatric illness present in hypothyroidism, consideration should be given to adjusting the treatment of hypothyroidism to improve psychiatric symptoms, even though the reports are scarce and conflicting. Figure 1; Table 1 summarize key information from this review.
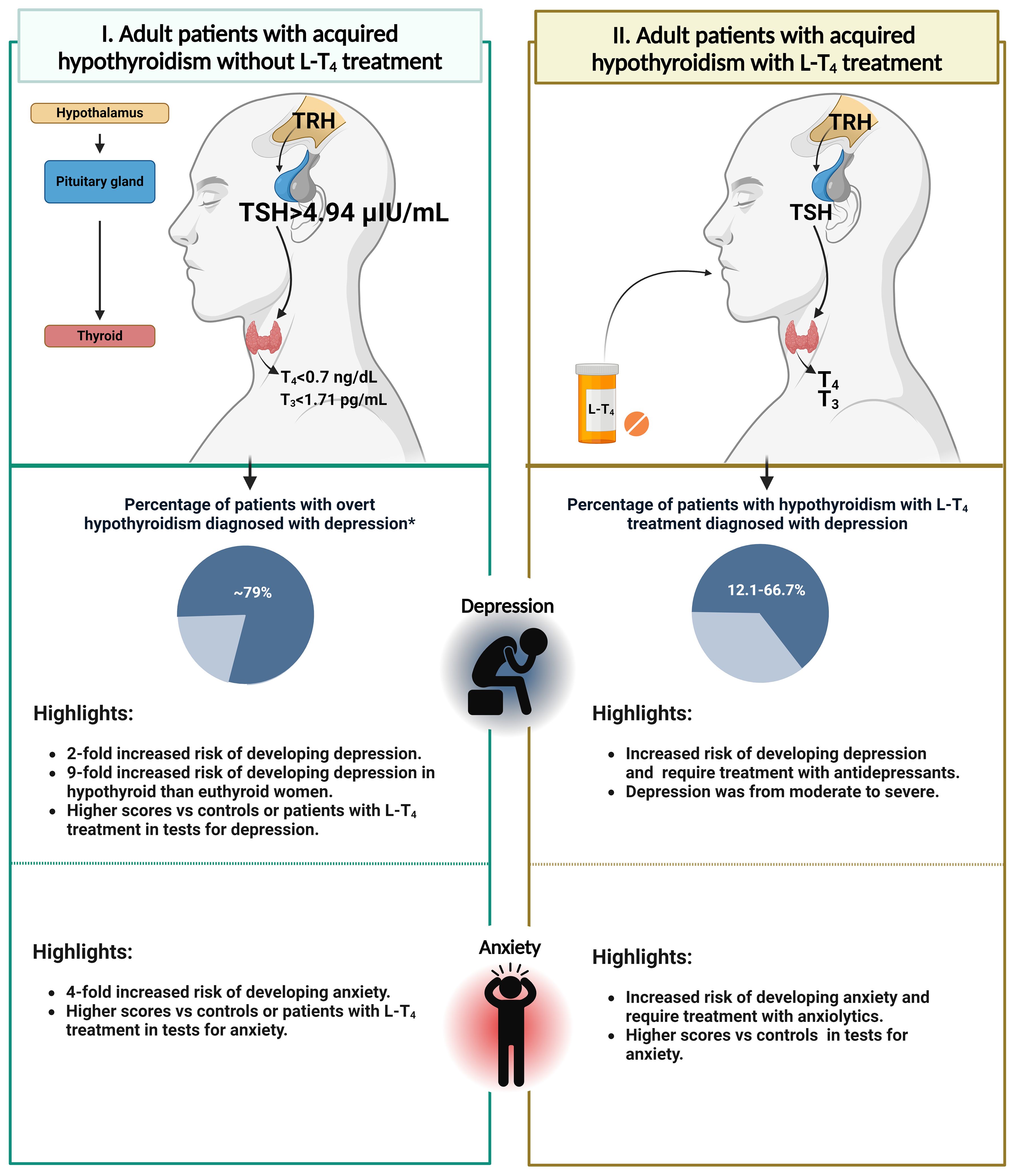
Figure 1 The association between acquired hypothyroidism and the subsequent development of depressive or anxiety disorders in adult patients. The figure on the left (I) depicts an adult patient with acquired hypothyroidism who has not received L-T4 treatment and their thyroid hormone levels (Usman et al., 2023). It is evident that there is a correlation between overt-acquired hypothyroidism and an increased risk of developing depression or anxiety. The figure on the right (II) shows an adult patient with acquired hypothyroidism who is undergoing L-T4 treatment. Furthermore, there is evidence indicating that depression or anxiety may be present despite the use of thyroid medication. The figure is based on clinical studies of the adult population (1, 21–26, 28–30, 33, 34). *Data obtained from the female population.
8 Acquired hypothyroidism and depression: key points on mechanisms underlaying this relationship
Previous evidence has shown that acquired hypothyroidism is associated with the onset of depression. This finding has been confirmed by studies in rodents showing that adult-onset hypothyroidism induces depression-like behavior, as indicated by increased immobility time in the forced swimming test (FST) or tail suspension test (TST) (63–65). The literature from animal models and clinical studies indicates that acquired hypothyroidism may lead to depression through several mechanisms, including alterations in serotonergic neurotransmission or the hypothalamic-pituitary-adrenal axis (HPA axis), changes in adult hippocampal neurogenesis or brain region integrity, or by activation of systemic pro-inflammatory processes or neuroinflammation, or by the triggering of the kynurenine pathway. The following sections will provide a brief overview of the various mechanisms by which acquired hypothyroidism may lead to the development of depression.
8.1 Acquired hypothyroidism and serotoninergic neurotransmission
The monoamine hypothesis proposes that a reduced availability of monoamine neurotransmitters, such as serotonin (5-HT), dopamine and norepinephrine, results in decreased neurotransmission and impaired cognitive performance, which may lead to depression (66, 67). Preclinical and clinical evidence supports the notion that monoaminergic alterations could partially explain the presence of depression in patients with acquired hypothyroidism.
Although some studies have evaluated alterations in brain dopamine and norepinephrine levels in hypothyroid adult rodents, the results are controversial, and it is difficult to draw conclusions about them (63, 68–70). In contrast, the findings are consistent showing that serotoninergic neurotransmission is the most affected by hypothyroidism. Hassan et al. (2013) reported lower 5-HT levels in the blood plasma of hypothyroid rats in comparison to controls (68). Interestingly, serotonergic deficits are also observed in the brain. Yu et al. (2015) reported reduced 5-HT concentrations in the whole brain of hypothyroid rodents (71). More specific studies have shown that 5-HT is reduced in brain regions implicated in depression, such as the cerebral cortex (68), hippocampus (63, 69), prefrontal cortex (63, 69), and dorsal raphe nucleus (65), in adult rodents with hypothyroidism. Similarly, clinical evidence has demonstrated that patients with acquired hypothyroidism (without L-T4 treatment) exhibit lower platelet 5-HT concentrations (72); this deficiency is also observed in serum samples from hypothyroid patients receiving L-T4 treatment (73). Additionally, patients with acquired hypothyroidism have reduced central 5-HT activity, as evaluated by the response to dexfenfluramine (74, 75).
The expression of 5-HT2A receptors in the prefrontal cortex (64, 76) and hippocampus (64) is lower in hypothyroid rats than in controls. Lee et al. (2017) reported that hypothyroidism provokes increased expression of 5-HT1A receptors in the hippocampus (77), while Kulikov and Jeanningro (2001) did not observe changes in this receptor (76).
A recent study reported a significant inverse correlation between depression-like behavior (as measured by increased immobility time in the forced swim test or tail suspension test) and 5-HT levels or the expression of 5-HT2A receptors in the prefrontal cortex and hippocampus of adult rodents with hypothyroidism (63, 64). At the clinical level, higher scores on the test for depression (test: HDRS) are correlated with reduced central 5-HT activity in patients with acquired hypothyroidism (75).
Thus, the evidence suggest that acquired hypothyroidism induces a deficit in serotoninergic neurotransmission by reducing the 5-HT concentration and the expression of postsynaptic 5HT2A receptors. Consequently, these changes could lead to the development of depressive symptoms in patients. More studies should be carried out to elucidate the role of dopamine and norepinephrine in the relationship between hypothyroidism and depression.
8.2 Acquired hypothyroidism and kynurenine pathway
The kynurenine pathway (KP) plays a pivotal role in the pathophysiology of depression and has also been linked to hypothyroidism. KP metabolites play important roles in various tissues, either as a neuroprotective agent (kynurenic acid) or a neurotoxic agent (quinolinic acid). Additionally, KP modulates the immune system and provides energy in the form of nicotinamide adenine dinucleotide (NAD) for immune system responses. In the context of psychiatric disorders, numerous KP metabolites exhibit neuroactive properties, modulating neuroplasticity and exerting neurotoxic effects, at least in part, through their influence on NMDA receptor signaling and glutamatergic neurotransmission. In consequence, these metabolites have been demonstrated to have a significant association with psychiatric illness in the context of inflammation (78).
The KP is initiated by the metabolism of tryptophan (TRP). It is noteworthy that TRP is also the precursor of 5-HT and NAD, which is a cofactor involved in various metabolic pathways. In the TRP metabolism, the enzyme that represents the limiting step is indoleamine 2,3-dioxygenase (IDO). This enzyme determines whether the kynurenine or serotonin pathway or NAD synthesis occurs. IDO catalyzes the conversion of TRP into kynurenine (KYN), which is converted into two metabolites: 3-hydroxykynurenine (3-HKYN) or kynurenic acid (KYNA). Subsequently, 3-HKYN is transformed into 3-hydroxyanthranilic acid (3-HAA), and it gives rise to quinolinic acid (QUIN), which acts as an agonist of NMDA receptors and may induce excitotoxicity and neuronal death (79).
A study of 57 young women with autoimmune thyroiditis revealed an abnormal activation of KP in these patients in comparison to healthy controls. The levels of KYN and anthranilic acid (AA) were elevated, while KYNA was reduced in autoimmune thyroiditis, resulting in an imbalance between AA and KYNA levels. In contrast, the other metabolites, including 3-HKYN and 3-HAA, remained unchanged, whereas QUIN exhibited a slight increase in patients with autoimmune thyroiditis (80).
The evidence from research in humans and animal models indicates that KYNA deficiency can result in neuronal loss (81, 82), while increased KYNA in the brain can induce cognitive dysfunction due to reduced signaling through NMDA receptors, which are critical for learning and memory (83, 84). Thyroid hormones modulate the synthesis of the tryptophan metabolite, KYNA. Experimental hypothyroidism is associated with an independent increase in cerebral KYNA levels. Some data indicates the existence of a new mechanism related to thyroid hormone deficiency. This suggests that high KYNA levels may play a role in cognitive impairment associated with hypothyroidism (85).
In pathological conditions, nitric oxide (NO) can cause oxidative damage through the formation of the highly reactive metabolite peroxynitrite. NO is synthesized from L-arginine by nitric oxide synthase (NOS). There are different isoforms of NOS, and both the endothelial (eNOS) and neuronal (nNOS) isoforms are calcium-dependent, while the inducible isoform (iNOS) is calcium-independent (86). It has been postulated that an elevation in reactive oxygen species (ROS) levels, resulting from the deficiency of thyroid hormones, may give rise to an oxidative stress condition in various organs, including the brain, with a subsequent lipid peroxidation response (85).
In a model of hypothyroidism induced by the administration of methimazole in adult rats, an increase in oxidative stress was found in the hippocampus and amygdala. This was accompanied by increased levels of free radicals, lipid peroxidation and nNOS activity (87). This oxidative stress was accompanied by the triggering of the apoptotic pathways and neuronal damage in all regions of the hippocampus (88), an effect that is mediated by the overactivation of NMDA receptors (89). These findings indicate that adult-onset hypothyroidism results in oxidative stress that leads to neuronal death in the hippocampus, where the nitrergic system is involved. This provides a potential explanation for the behavioral abnormalities observed during hypothyroidism.
The activation of the KP, the overproduction of NO, and the subsequent oxidative stress in hypothyroid subjects indicate that QUIN may be a potential mediator between acquired hypothyroidism, immunological and neurotransmitter alterations, and depression. This hypothesis is based on the observation that pro-inflammatory cytokines induce a change in 5-HT synthesis due to alterations in tryptophan metabolism by the activation of the kynurenine pathway in glial cells, which may ultimately lead to 5-HT depletion and increased production of the neurotoxic metabolite QUIN (90). Collectively, these processes may result in the onset of depression.
Based on the above, the potential causes of depressive symptoms in patients with acquired hypothyroidism include the triggering of the KP, induced oxidative stress, overstimulation of NMDA receptors, and neuronal apoptosis. It is possible that these events may be provoked by an overproduction of QUIN, although this hypothesis requires further investigation. Furthermore, the depletion of 5-HT caused by the activation of the kynurenine pathway also plays a significant role in the onset of depressive symptoms.
8.3 Acquired hypothyroidism and hypothalamic pituitary adrenal axis
The HPA axis is a vital neuroendocrine circuit responsible for regulating a variety of physiological processes and responses to stress (91). This axis coordinates the secretion of glucocorticoid hormones, such as cortisol, under normal conditions and in reaction to stressors (92). The HPA axis also facilitates the physiological adaptations that occur during stressful situations. Its activity is regulated through negative feedback mechanisms, which allow for self-regulation (93).
Dysregulation of the HPA axis has been documented in patients with acquired hypothyroidism, who have elevated serum cortisol levels (94, 95). Sinha et al. (2023) reported that patients with acquired hypothyroidism had significantly higher serum cortisol levels compared to healthy controls. They also found a positive correlation between raised serum cortisol and raised TSH levels; and a negative correlation between high serum cortisol and lower T3 and T4 levels. The authors explain that elevated cortisol levels in patients with hypothyroidism may be due to reduced cortisol elimination and negative cortisol feedback in the HPA axis. However, it could also be a possible compensatory mechanism triggered by the HPA axis with increased cortisol secretion to mitigate the metabolic consequences of thyroid hormone deficiency (94).
On the other hand, it has been reported that dysregulation of the HPA axis can lead to various physiological and psychological alterations, which in turn lead to the development of mood disorders (96). This is important because it shows that hypothyroidism affects the HPA axis and can cause depression. The HPA axis is implicated in the pathogenesis of conditions such as depression and post-traumatic stress disorder. Patients with both disorders exhibit abnormalities in the HPA axis regulation, including altered cortisol levels and a failure to suppress cortisol release in the dexamethasone suppression test (97). Hernandez et al. (2008) reported hypercortisolism (measured in urine) in patients with depression compared to healthy volunteers. Only after 52 weeks of treatment with selective serotonin reuptake inhibitors (SSRIs) did the patients achieve a significant reduction in their cortisol levels; however, the authors considered this reduction to be only a partial recovery of HPA axis function (98). Although patients improved clinically in terms of depressive symptoms, elevated cortisol levels resulting from HPA axis dysregulation kept potential relapse latent. Furthermore, in animal models, dysregulation of the HPA axis has been demonstrated to result in an increased depression-like behavior (99). In normal conditions, cortisol serves to reduce inflammation. However, if an individual is chronically stressed or exhibits a dysregulation of the HPA axis, the subjects will present chronic hypercortisolism. This results in a diminished capacity of the HPA axis to regulate itself, which can lead to the release of pro-inflammatory cytokines by immune cells (100). Elevated pro-inflammatory cytokines have been demonstrated to play a significant role in the pathophysiology of depression (101).
Collectively, the evidence indicates that the development of depression in patients with acquired hypothyroidism appears to be significantly correlated with dysregulation of the HPA axis. This is consistent with the high prevalence of depression in hypothyroid patients and the reports of patients with depression with alterations in the HPA axis. Further research in patients with acquired hypothyroidism, with particular attention to HPA axis function and depressive symptoms, is essential to fully understand this complex relationship between thyroid function, the HPA axis, and depression. In conclusion, chronic hypercortisolism due to HPA axis dysregulation in hypothyroid patients appears to be key to the development of depression in these patients.
8.4 Acquired hypothyroidism and pro-inflammatory cytokines
Patients with hypothyroidism frequently present alterations in proinflammatory cytokines. Studies have demonstrated that individuals with hypothyroidism exhibit elevated levels of proinflammatory cytokines, including TNF-α, IL-6, and C-reactive protein (CRP), when compared to healthy individuals (102). It has been observed that levothyroxine treatment can reduce these cytokine levels, although it is not always possible to achieve normalization (102).
A study conducted in 2006 on patients with Hashimoto thyroiditis demonstrated a significant positive correlation between elevated serum IL-6 levels (a pro-inflammatory cytokine) and the required L-T4 dose. Conversely, a significant negative correlation was observed between elevated IL-6 levels and serum T3 and T3/T4 ratio (103). Another study in patients with autoimmune thyroiditis found that the serum levels of IL-2, IFN-γ, and TNF-α were elevated. This increase in these three pro-inflammatory cytokines may be explained by a higher number of activated T cells, resulting from the recognition of thyroid autoantibodies (104). Figueroa-Vega et al. (2010) demonstrated that patients with Hashimoto thyroiditis exhibit elevated circulatory levels of T-helper 17 (Th17) cells, a subpopulation of proinflammatory lymphocytes. Furthermore, elevated serum levels of IL-6 and IL-15 were observed in these patients. Both cytokines are involved in the differentiation of Th17 cells and possess pro-inflammatory properties (105).
The presented evidence highlights the pro-inflammatory state observed in hypothyroid patients. Indeed, Lai et al. (2024) propose a bidirectional relationship between elevated pro-inflammatory cytokines and the observed alterations in thyroid function (102).
Therefore, it is inevitable to associate depression in this context, a disorder characterized predominantly by a proinflammatory state. Liu et al. observed that patients with depression presented elevated serum levels of IL-1β, which correlated positively with the severity of depressive symptoms. In addition, TNF-α emerged as a promising biomarker for predicting the elevated risk of suicidal behavior (106). It is well established that systemic inflammation can affect the permeability and normal function of the blood-brain barrier (107), allowing peripheral cytokines to access the brain and producing neuroinflammation and alterations in neurotransmitter function (108). Furthermore, cytokines may influence monoamine synthesis through the degradation of tetrahydrobiopterin (BH4), a cofactor essential for 5-HT synthesis (108).
In 2017, Tayde et al. (2017) raised the question of whether proinflammatory cytokines are the link between hypothyroidism and depression (109). In this intriguing study, the researchers observed that individuals with primary autoimmune hypothyroidism exhibited elevated levels of IL-6, TNF-α, and CRP. The study included patients with antithyroid antibodies and TSH levels ≥10 μIU/mL. Following six months of treatment with levothyroxine, 42% of patients achieved remission of depression and an euthyroid state. Moreover, the administration of levothyroxine resulted in a significant reduction in the levels of the three pro-inflammatory markers, yet the reduction did not reach baseline levels (109). The findings of Tayde et al. (2017) provide compelling evidence that restoring thyroid function through L-T4 treatment in hypothyroid patients can alleviate depressive symptoms while reducing the proinflammatory state in these patients. This study demonstrates that proinflammatory cytokines are crucial in the pathophysiology and mechanism of the development of depression.
It is crucial to acknowledge that most of the studies discussed in this section were conducted in patients with autoimmune thyroiditis. It would be valuable to ascertain whether the proinflammatory state observed in this form of hypothyroidism is also present in other types of hypothyroidism acquired through non-autoimmune causes.
8.5 Acquired hypothyroidism and adult hippocampal neurogenesis
Adult neurogenesis is the generation of new neurons in the mature brain (110). In the hippocampus, adult neurogenesis occurs in the subgranular zone of the dentate gyrus (111, 112) and is a complex process that involves the proliferation of neural stem cells and progenitor cells (Type 2a, 2b and 3 cells) (113–115), the differentiation of neuroblasts into granular neurons through the stadium of immature granular neurons (IGNs), and the integration of new neurons into preexisting circuits in the dentate gyrus (116, 117). It has been postulated that reduced adult hippocampal neurogenesis could be implicated in the pathophysiology of depression (118, 119), principally by leading to overactivation of the hypothalamus-pituitary-adrenal axis (120, 121) and by affecting the contextual encoding of emotions (119, 122).
Evidence from animal models has shown that adult-onset hypothyroidism provokes impaired neurogenesis, exerting a deleterious effect, particularly on postmitotic cells. Specifically, hypothyroidism significantly reduces the population of quiescent Type 2b and 3 cells, postmitotic neuroblasts and IGNs (123–126). This reduced neurogenesis appears to be mediated by TRα1 aporeceptors (unlinked receptors), which could be predominant in hypothyroidism and be capable of repressing the expression of proneural and cell survival genes (127–129).
Interestingly, the neurogenic deficit in hypothyroid adult rats is accompanied by decreased expression of brain derived neurotrophic factor (BDNF) in the whole hippocampus (130) and, particularly, in the dentate gyrus (126). This phenomenon has also been reported in patients. Bilous et al. (2020, 2021) reported decreased expression of BDNF and neurogenesis-regulated genes in patients with primary hypothyroidism (131, 132). Alterations in the expression of BDNF are relevant because of three reasons: 1) the BDNF promoter is responsive to thyroid hormones (133), 2) this neurotrophic factor plays a key role in the postmitotic phase of the neurogenic process, promoting the survival of newborn neurons (134, 135), and 3) several studies have highlighted associations between low levels of BDNF and the development of behavioral symptoms of depression (136). Thus, BDNF could be the link between adult hypothyroidism, reduced neurogenesis and depression.
The relationship between adult neurogenesis and depression-like behavior in hypothyroidism condition was explored by Montero-Pedrazuela et al. (2006), who reported that reduced neurogenesis is accompanied by increased immobility time in the FST in hypothyroid rats (125). In contrast, increased hippocampal neurogenesis, provoked by simultaneous treatment with T3 and fluoxetine, is associated with antidepressant behavior in rats (test: novelty suppressed feeding test) (137).
This evidence suggests that acquired hypothyroidism can induce depression by reducing adult neurogenesis and BDNF expression in the hippocampus. Deeper studies should be carried out to determine the causal relationships and molecular pathways involved in the associations between hypothyroidism, neurogenesis and depression.
8.6 Acquired hypothyroidism and structural changes in brain
Because thyroid hormones play an important role in the development of the nervous system, it is important to study whether structural changes are present in patients with acquired hypothyroidism. Although the adult brain has completed its development, thyroid hormones maintain different functions on neuronal physiology, so hypothyroidism may cause changes in the metabolism and structure of the nervous system, causing alterations in the functionality of different brain regions. Currently, with the development of various brain imaging techniques, it has been possible to study possible changes in brain structure in patients with untreated acquired hypothyroidism (138).
One structure that has received particular attention is the hippocampus, as this structure is associated with learning and memory functions as well as mood states, functions that are clearly altered in hypothyroid patients. There are reports in animal models of damage to the pyramidal neurons of the hippocampus as a result of decreased thyroid hormone levels (139, 140).
Using magnetic resonance imaging (MRI) in patients with untreated acquired hypothyroidism, a reduction in hippocampal volume has been observed (141), as well as a reduction specifically in the granular and molecular layers of the hippocampus (142). It has been proposed that the volume reduction correlates with a decrease in peripheral BDNF levels (143). In addition, using positron emission tomography (PET), it has been shown that hippocampal glucose utilization is reduced in hypothyroid patients (144).
Other structures associated with psychiatric disorders, such as the prefrontal cortex or the amygdala, have also been studied for changes due to the effects of acquired hypothyroidism. Although there are fewer studies on this topic, there is evidence of structural and metabolic changes, although the results are more variable.
Using voxel-based morphometry, a reduction in gray matter in the cerebellum as well as white matter in the cerebellum, frontal gyrus, temporal gyrus, and occipital gyrus has been reported (145), while using MRI, the group of Leyhe et al. (2014) observed decreased gray matter in the inferior frontal gyrus (146).
Regarding metabolic changes, decreased glucose utilization in the amygdala and anterior cingulate cortex has been reported (144), as well as decreased blood flow in the prefrontal, temporal and occipital lobes (147).
All these structural changes observed in hypothyroid patients could affect the proper functioning of the central nervous system and contribute to the development of psychiatric disorders.
9 Conclusion
Evidence supports that acquired hypothyroidism increases the risk of depression and anxiety in adult patients. Current data indicates that patients with acquired hypothyroidism without L-T4 treatment face a 2-fold increased risk of depression and a 4-fold elevated risk of anxiety, with a notably higher risk for depression in women (about 9-fold more risk). The prevalence of depression in these patients is 79%, and they show elevated scores in depression or anxiety tests when compared to controls or patients receiving L-T4 treatment. On the other hand, the literature shows that both depression and anxiety can be also present in patients with acquired hypothyroidism with L-T4 treatment. These patients are at a higher risk of developing depression or anxiety and therefore require treatment with antidepressants or anxiolytics. Depression affects between 12.1% and 66.7% of these patients and is often classified as moderate to severe. Regarding anxiety, these patients exhibit higher scores on tests for anxiety versus controls.
The significant prevalence of depression in patients with acquired hypothyroidism, coupled with the high susceptibility to developing depression and anxiety, highlights the need for enhanced monitoring of psychiatric symptoms. Consistent monitoring and timely recognition of psychiatric symptoms, especially in patients with TSH serum levels >2.5 µIU/mL, will prevent disease aggravation, facilitating more effective therapeutic management. On the other hand, there is no strong evidence suggesting that acquired hypothyroidism could be a risk factor for schizophrenia or bipolar disorder; however, more scientific research is necessary to address this matter. Finally, key points on the underlying mechanisms of the relationship between hypothyroidism and depression are presented. These evidence the different ways in which acquired hypothyroidism can lead to the onset of depression. These include disturbances in serotonergic neurotransmission or the HPA axis, alterations in adult hippocampal neurogenesis or brain region integrity, activation of systemic pro-inflammatory processes or neuroinflammation, or by the triggering of the kynurenine pathway.
Author contributions
NO-B: Writing – original draft. AV-P: Writing – original draft. DS: Writing – original draft. YG-M: Writing – original draft. JP-R: Writing – original draft. GP-S: Writing – review & editing, Supervision, Project administration, Conceptualization, Writing – original draft. KS-H: Writing – review & editing, Supervision, Project administration, Funding acquisition, Conceptualization, Writing – original draft.
Funding
The author(s) declare financial support was received for the research, authorship, and/or publication of this article. YG-M, JP-R, GP-S, and KS-H are SNII-CONAHCyT fellows. JP-R is fellow of DEDICT-COFFA and EDI, Instituto Politécnico Nacional. INP-027/2020-Program E022 also supported this work. Partially supported by Instituto Nacional de Psiquiatría Ramón de la Fuente Muñíz.
Acknowledgments
The authors would like to thank Julieta Mendoza Torreblanca for supporting in preparing figures. Figures were created with Biorender.com.
Conflict of interest
The authors declare that the research was conducted in the absence of any commercial or financial relationships that could be construed as a potential conflict of interest.
Publisher’s note
All claims expressed in this article are solely those of the authors and do not necessarily represent those of their affiliated organizations, or those of the publisher, the editors and the reviewers. Any product that may be evaluated in this article, or claim that may be made by its manufacturer, is not guaranteed or endorsed by the publisher.
Supplementary material
The Supplementary Material for this article can be found online at: https://www.frontiersin.org/articles/10.3389/fpsyt.2024.1429255/full#supplementary-material
Abbreviations
3-HAA, 3-hydroxyanthranilic acid; 3HKYN, 3-Hydroxy Kinurenine; 5-HT, serotonin; AA, anthranilic acid; BAI, Beck anxiety inventory; BDI, Beck depression inventory; BDI-II, Beck depression inventory-II; BDNF, Brain derived neurotrophic factor; CHS, Clalit health services; CI, confident interval; CRP, C-reactive protein; DSM-III-R, Diagnostic and statistical manual of mental disorders; eNOS, Endothelial NItric Oxide Synthase; FST, forced swimming test; HARS, Hamilton anxiety rating scale; HDRS, Hamilton depression rating scale; HPA-axis, Hypothalamic-Pituitary-Adrenal axis; HR, Hazard risk; IDO, Indolamine 2–3 Dioxygenase; IGNs, Immature granular neurons; IL, Interleukin; iNOS, Inducible Nitric Oxide Synthase; KP, Kynurenine Pathway; KYN, Kynurenine; KYNA, Kynurenic Acid; L-T4, Levothyroxine; M-CIDI, Munich composite international diagnostic interview; MRI, magnetic resonance imaging; NAD, Nicotinamide Adenine Dinucleotide; NMDA, N-Methyl-D-Aspartate; nNOS, Neuronal Nitric Oxide Synthase; NO, Nitric Oxide; NOS, Nitric Oxide Synthase; PET, positron emission tomography; PHQ-9, Patient health questionnaire-9; PRIME-MD Test, Primary care evaluation of mental disorders; QUIN, Quinolinic Acid; ROS, Reactive Oxygen Species; SciELO, Scientific electronic library online; SSRIs, selective serotonin reuptake inhibitors; SSRIs, selective serotonin reuptake inhibitors; STAI, state-trait anxiety inventory; T3, triiodothyronine; T4, thyroxine; Th17, T helper 17; THs, Thyroid hormones; ThyPRO, Thyroid-related quality-of-life patient-reported outcome; TNF, Tumor necrosis factor; TRP, Tryptophan; TRα1, Thyroid hormone receptor alpha 1; TSH, Thyroid stimulating hormone; TST, tail suspension test; WHO, World Health Organization.
References
1. Usman K, Kalra SK, Sarwar S, Ghaffar T, Zoak ZA, Sayed TM. Depression illness in patients with primary hypothyroidism presenting to endocrine clinic. Pakistan J Med Health Sci. (2023) 17:406. doi: 10.53350/pjmhs202317
2. Chaker L, Bianco AC, Jonklaas J, Peeters RP. Hypothyroidism. Lancet (London England). (2017) 390:1550–62. doi: 10.1016/S0140-6736(17)30703-1
3. Hanley P, Lord K, Bauer AJ. Thyroid disorders in children and adolescents: A review. JAMA Pediatr. (2016) 170:1008–19. doi: 10.1001/jamapediatrics.2016.0486
4. Kostoglou-Athanassiou I, Ntalles K. Hypothyroidism - new aspects of an old disease. Hippokratia. (2010) 14:82–7.
5. Naguib R. Potential relationships between COVID-19 and the thyroid gland: an update. J Int Med Res. (2022) 50:3000605221082898. doi: 10.1177/03000605221082898
6. Patil N, Rehman A, Anastasopoulou C, Jialal I. Hypothyroidism. Treasure Island (FL: StatPearls Publishing (2024).
7. Chiovato L, Magri F, Carlé A. Hypothyroidism in context: where we’ve been and where we’re going. Adv Ther. (2019) 36:47–58. doi: 10.1007/s12325-019-01080-8
8. Wyne KL, Nair L, Schneiderman CP, Pinsky B, Antunez Flores O, Guo D, et al. Hypothyroidism prevalence in the United States: A retrospective study combining national health and nutrition examination survey and claims data, 2009-2019. J Endocrine Soc. (2022) 7:bvac172. doi: 10.1210/jendso/bvac172
9. Garmendia Madariaga A, Santos Palacios S, Guillén-Grima F, Galofré JC. The incidence and prevalence of thyroid dysfunction in Europe: a meta-analysis. J Clin Endocrinol Metab. (2014) 99:923–31. doi: 10.1210/jc.2013-2409
10. Sánchez-Romero LM, Barquera S, Campos I, García E, Arellano S, González A, et al. Concentraciones séricas de hormona estimulante de la tiroides (TSH) en niños, adolescentes y adultos mexicanos. In: Resultados de la ENSANUT 2006. Instituto Nacional de Salud Pública, México (2012). p. 1–22.
11. Flores-Rebollar A, Pérez-Díaz I, Vega-Vega O, Rivera-Moscoso R, Fagundo-Sierra R, Carbajal-Morelos SL, et al. Prevalence of thyroid dysfunction in healthy adults according to the estimated iodine intake in 24-hour urine samples: The SALMEX cohort. Eur J Nutr. (2021) 60:399–409. doi: 10.1007/s00394-020-02254-9
12. Vargas Navarro P, Ibañez Pinilla EA, Galeano España A, Noguera Bravo AM, Milena Pantoja S, Suárez Acosta AM. [Prevalence of hypothyroidism in major psychiatric disorders in hospitalised patients in Montserrat hospital during the period march to october 2010]. Rev Colombiana psiquiatria. (2017) 46:140–6. doi: 10.1016/j.rcp.2016.06.006
13. Lekurwale V, Acharya S, Shukla S, Kumar S. Neuropsychiatric manifestations of thyroid diseases. Cureus. (2023) 15:e33987. doi: 10.7759/cureus.33987
14. Chakrabarti S. Thyroid functions and bipolar affective disorder. J Thyroid Res. (2011) 2011:306367. doi: 10.4061/2011/306367
15. Radhakrishnan R, Calvin S, Singh JK, Thomas B, Srinivasan K. Thyroid dysfunction in major psychiatric disorders in a hospital based sample. Indian J Med Res. (2013) 138:888–93.
16. Tonks CM. Mental Illnesses in hypothyroid patients. Br J psychiatry: J Ment Sci. (1964) 110:706–10. doi: 10.1192/bjp.110.468.706
17. Chand SP, Arif H. Depression. In: StatPearls. Treasure Island (FL): StatPearls Publishing. (2024).
18. American Psychiatric Association: Diagnostic and Statistical Manual of Mental Disorders, Fifth Edition. Arlington, VA, American Psychiatric Association, (2013).
19. World Health Organization. Mental Disorders . Available online at: https://www.who.int/news-room/fact-sheets/detail/mental-disorders.
20. Lee B, Wang Y, Carlson SA, Greenlund KJ, Lu H, Liu Y, et al. National, state-level, and county-level prevalence estimates of adults aged ≥18 years self-reporting a lifetime diagnosis of depression - United States, 2020. MMWR Morbidity mortality weekly Rep. (2023) 72:644–50. doi: 10.15585/mmwr.mm7224a1
21. Guimarães JMN, de Souza Lopes C, Baima J, Sichieri R. Depression symptoms and hypothyroidism in a population-based study of middle-aged Brazilian women. J Affect Disord. (2009) 117:120–3. doi: 10.1016/j.jad.2008.12.012
22. Mohammad MYH, Bushulaybi NA, AlHumam AS, AlGhamdi AY, Aldakhil HA, Alumair NA, et al. Prevalence of depression among hypothyroid patients attending the primary healthcare and endocrine clinics of King Fahad Hospital of the University (KFHU). J Family Med primary Care. (2019) 8:2708–13. doi: 10.4103/jfmpc.jfmpc_456_19
23. Bunevicius R, Kazanavicius G, Zalinkevicius R, Prange AJJ. Effects of thyroxine as compared with thyroxine plus triiodothyronine in patients with hypothyroidism. New Engl J Med. (1999) 340:424–9. doi: 10.1056/NEJM199902113400603
24. Talaei A, Rafee N, Rafei F, Chehrei A. TSH cut off point based on depression in hypothyroid patients. BMC Psychiatry. (2017) 17:327. doi: 10.1186/s12888-017-1478-9
25. Gunes NA. Evaluation of anxiety and depression in patients with thyroid function disorder. Rev da Associacao Med Bras (1992). (2020) 66:979–85. doi: 10.1590/1806-9282.66.7.979
26. Ittermann T, Völzke H, Baumeister SE, Appel K, Grabe HJ. Diagnosed thyroid disorders are associated with depression and anxiety. Soc Psychiatry Psychiatr Epidemiol. (2015) 50:1417–25. doi: 10.1007/s00127-015-1043-0
27. Spitzer RL, Williams JB, Kroenke K, Linzer M, deGruy FV 3rd, Hahn SR, et al. Utility of a new procedure for diagnosing mental disorders in primary care. The PRIME-MD 1000 study. JAMA. (1994) 272:1749–56. doi: 10.1001/jama.1994.03520220043029
28. Gulseren S, Gulseren L, Hekimsoy Z, Cetinay P, Ozen C, Tokatlioglu B. Depression, anxiety, health-related quality of life, and disability in patients with overt and subclinical thyroid dysfunction. Arch Med Res. (2006) 37:133–9. doi: 10.1016/j.arcmed.2005.05.008
29. Constant EL, Adam S, Seron X, Bruyer R, Seghers A, Daumerie C. Anxiety and depression, attention, and executive functions in hypothyroidism. J Int Neuropsychol Society: JINS. (2005) 11:535–44. doi: 10.1017/S1355617705050642
30. Thvilum M, Brandt F, Almind D, Christensen K, Brix TH, Hegedüs L. Increased psychiatric morbidity before and after the diagnosis of hypothyroidism: a nationwide register study. Thyroid: Off J Am Thyroid Assoc. (2014) 24:802–8. doi: 10.1089/thy.2013.0555
31. Toloza FJK, Mao Y, Menon L, George G, Borikar M, Thumma S, et al. Association of thyroid function with suicidal behavior: A systematic review and meta-analysis. Medicina (Kaunas Lithuania). (2021) 57(7):714. doi: 10.3390/medicina57070714
32. Kessler RC, Chiu WT, Demler O, Merikangas KR, Walters EE. Prevalence, severity, and comorbidity of 12-month DSM-IV disorders in the National Comorbidity Survey Replication. Arch Gen Psychiatry. (2005) 62:617–27. doi: 10.1001/archpsyc.62.6.617
33. Constant EL, Adam S, Seron X, Bruyer R, Seghers A, Daumerie C. Hypothyroidism and major depression: a common executive dysfunction? J Clin Exp Neuropsychol. (2006) 28:790–807. doi: 10.1080/13803390591000990
34. Monzani ML, Piccinini F, Boselli G, Corleto R, Margiotta G, Peeters RP, et al. Changes in quality of life after thyroidectomy in subjects with thyroid cancer in relation to the dose of levothyroxine. J endocrinological Invest. (2023) 46:319–26. doi: 10.1007/s40618-022-01903-6
35. Grabe HJ, Völzke H, Lüdemann J, Wolff B, Schwahn C, John U, et al. Mental and physical complaints in thyroid disorders in the general population. Acta psychiatrica Scandinavica. (2005) 112:286–93. doi: 10.1111/j.1600-0447.2005.00586.x
36. Girdler SJ, Confino JE, Woesner ME. Exercise as a treatment for schizophrenia: A review. Psychopharmacol Bull. (2019) 49:56–69.
37. Salvi V, Ribuoli E, Servasi M, Orsolini L, Volpe U. ADHD and bipolar disorder in adulthood: clinical and treatment implications. Medicina (Kaunas Lithuania). (2021) 57(5):466. doi: 10.3390/medicina57050466
38. McIntyre RS, Calabrese JR. Bipolar depression: the clinical characteristics and unmet needs of a complex disorder. Curr Med Res Opin. (2019) 35:1993–2005. doi: 10.1080/03007995.2019.1636017
39. Simpson GM, Cranswick EH, Blair JH. Thyroid indices in chronic schizophrenia. J nervous Ment Dis. (1963) 137:582–90. doi: 10.1097/00005053-196312000-00010
40. Rogers MP, Whybrow PC. Clinical hypothyroidism occurring during lithium treatment: two case histories and a review of thyroid function in 19 patients. Am J Psychiatry. (1971) 128:158–63. doi: 10.1176/ajp.128.2.158
41. Sharif K, Tiosano S, Watad A, Comaneshter D, Cohen AD, Shoenfeld Y, et al. The link between schizophrenia and hypothyroidism: a population-based study. Immunologic Res. (2018) 66:663–7. doi: 10.1007/s12026-018-9030-7
42. Benros ME, Nielsen PR, Nordentoft M, Eaton WW, Dalton SO, Mortensen PB. Autoimmune diseases and severe infections as risk factors for schizophrenia: a 30-year population-based register study. Am J Psychiatry. (2011) 168:1303–10. doi: 10.1176/appi.ajp.2011.11030516
43. Thomsen AF, Kvist TK, Andersen PK, Kessing LV. Increased risk of developing affective disorder in patients with hypothyroidism: a register-based study. Thyroid: Off J Am Thyroid Assoc. (2005) 15:700–7. doi: 10.1089/thy.2005.15.700
44. Danilo Q, Gloger S, Valdivieso S, Ivelic J, Fardella C. [Mood disorders, psychopharmacology and thyroid hormones]. Rev Med Chile. (2004) 132:1413–24.
45. Caneo C, Aedo I, Riquelme MJ, Fardella C. Disfunción tiroidea y trastornos del ánimo: revisión del estado del arte. Rev Médica Clínica Las Condes. (2020) 31:122–9. doi: 10.1016/j.rmclc.2020.01.003
46. Joffe RT. Refractory depression: treatment strategies, with particular reference to the thyroid axis. J Psychiatry neuroscience: JPN. (1997) 22:327–31.
47. Altshuler LL, Bauer M, Frye MA, Gitlin MJ, Mintz J, Szuba MP, et al. Does thyroid supplementation accelerate tricyclic antidepressant response? A review and meta-analysis of the literature. Am J Psychiatry. (2001) 158:1617–22. doi: 10.1176/appi.ajp.158.10.1617
48. Fardella CE, Artigas RA, Gloger S, Jiménez M, Carvajal CA, Krall PM, et al. Refractory depression in a patient with peripheral resistance to thyroid hormone (RTH) and the effect of triiodothyronine treatment. Endocrine. (2007) 31:272–8. doi: 10.1007/s12020-007-0042-7
49. Kelly TF, Lieberman DZ. Long term augmentation with T3 in refractory major depression. J Affect Disord. (2009) 115:230–3. doi: 10.1016/j.jad.2008.09.022
50. Hage MP, Azar ST. The link between thyroid function and depression. J Thyroid Res. (2012) 2012:590648. doi: 10.1155/2012/590648
51. Tundo A, de Filippis R, Proietti L. Pharmacologic approaches to treatment resistant depression: Evidences and personal experience. World J Psychiatry. (2015) 5:330–41. doi: 10.5498/wjp.v5.i3.330
52. Rudas S, Schmitz M, Pichler P, Baumgartner A. Treatment of refractory chronic depression and dysthymia with high-dose thyroxine. Biol Psychiatry. (1999) 45:229–33. doi: 10.1016/S0006-3223(98)00033-X
53. Moon JH, Han JW, Oh TJ, Choi SH, Lim S, Kim KW, et al. Effect of increased levothyroxine dose on depressive mood in older adults undergoing thyroid hormone replacement therapy. Clin Endocrinol. (2020) 93:196–203. doi: 10.1111/cen.14189
54. Bauer M, Whybrow PC. Role of thyroid hormone therapy in depressive disorders. J endocrinological Invest. (2021) 44:2341–7. doi: 10.1007/s40618-021-01600-w
55. Walsh JP, Ward LC, Burke V, Bhagat CI, Shiels L, Henley D, et al. Small changes in thyroxine dosage do not produce measurable changes in hypothyroid symptoms, well-being, or quality of life: results of a double-blind, randomized clinical trial. J Clin Endocrinol Metab. (2006) 91:2624–30. doi: 10.1210/jc.2006-0099
56. Rack SK, Makela EH. Hypothyroidism and depression: a therapeutic challenge. Ann pharmacotherapy. (2000) 34:1142–5. doi: 10.1345/aph.10022
57. Bunevicius R, Jakuboniene N, Jurkevicius R, Cernicat J, Lasas L, Prange AJJ. Thyroxine vs thyroxine plus triiodothyronine in treatment of hypothyroidism after thyroidectomy for Graves’ disease. Endocrine. (2002) 18:129–33. doi: 10.1385/ENDO:18:2:129
58. Sawka AM, Gerstein HC, Marriott MJ, MacQueen GM, Joffe RT. Does a combination regimen of thyroxine (T4) and 3,5,3’-triiodothyronine improve depressive symptoms better than T4 alone in patients with hypothyroidism? Results of a double-blind, randomized, controlled trial. J Clin Endocrinol Metab. (2003) 88:4551–5. doi: 10.1210/jc.2003-030139
59. Siegmund W, Spieker K, Weike AI, Giessmann T, Modess C, Dabers T, et al. Replacement therapy with levothyroxine plus triiodothyronine (bioavailable molar ratio 14: 1) is not superior to thyroxine alone to improve well-being and cognitive performance in hypothyroidism. Clin Endocrinol. (2004) 60:750–7. doi: 10.1111/j.1365-2265.2004.02050.x
60. Chan EC. Sustained remission of psychotic symptoms secondary to hypothyroidism (myxedema psychosis) after 6 months of treatment primarily with levothyroxine: a case report. J Med Case Rep. (2022) 16:378. doi: 10.1186/s13256-022-03626-x
61. Kaplan JL, Castro-Revoredo I. Severe hypothyroidism manifested as acute mania with psychotic features: A case report and review of the literature. J Psychiatr Pract. (2020) 26:417–22. doi: 10.1097/PRA.0000000000000497
62. Sardar S, Habib M-B, Sukik A, Tanous B, Mohamed S, Tahtouh R, et al. Myxedema psychosis: neuropsychiatric manifestations and rhabdomyolysis unmasking hypothyroidism. Case Rep Psychiatry. (2020) 2020:7801953. doi: 10.1155/2020/7801953
63. Bortolotto VC, Pinheiro FC, Araujo SM, Poetini MR, Bertolazi BS, de Paula MT, et al. Chrysin reverses the depressive-like behavior induced by hypothyroidism in female mice by regulating hippocampal serotonin and dopamine. Eur J Pharmacol. (2018) 822:78–84. doi: 10.1016/j.ejphar.2018.01.017
64. Jin Z, Ling J, Yu J, He M, Ni P, Zhang F, et al. Serotonin 2A receptor function and depression-like behavior in rats model of hypothyroidism. Exp Brain Res. (2021) 239:2435–44. doi: 10.1007/s00221-021-06129-1
65. Zhang Q, Feng JJ, Yang S, Liu XF, Li JC, Zhao H. Lateral habenula as a link between thyroid and serotoninergic system modiates depressive symptoms in hypothyroidism rats. Brain Res Bull. (2016) 124:198–205. doi: 10.1016/j.brainresbull.2016.05.007
66. Cui L, Li S, Wang S, Wu X, Liu Y, Yu W, et al. Major depressive disorder: hypothesis, mechanism, prevention and treatment. Signal transduction targeted Ther. (2024) 9:30. doi: 10.1038/s41392-024-01738-y
67. Jesulola E, Micalos P, Baguley IJ. Understanding the pathophysiology of depression: From monoamines to the neurogenesis hypothesis model - are we there yet? Behav Brain Res. (2018) 341:79–90. doi: 10.1016/j.bbr.2017.12.025
68. Hassan WA, Aly MS, Rahman TA, Shahat AS. Impact of experimental hypothyroidism on monoamines level in discrete brain regions and other peripheral tissues of young and adult male rats. Int J Dev neuroscience: Off J Int Soc Dev Neurosci. (2013) 31:225–33. doi: 10.1016/j.ijdevneu.2013.02.001
69. Wahman LF, Abd-Rabo MM, Youseef MHM, Abdel-Hameed UK. The effect of Hordeum vulgare on the monoaminergic system modulating neural-thyroid dysfunction in hypothyroid female rats. Cell Mol Biol (Noisy-le-Grand France). (2019) 65:53–62. doi: 10.14715/cmb/2019.65.4.9
70. Hosny EN, El-Gizawy MM, Sawie HG, Abdel-Wahhab KG, Khadrawy YA. Neuroprotective effect of ashwagandha extract against the neurochemical changes induced in rat model of hypothyroidism. J dietary Suppl. (2021) 18:72–91. doi: 10.1080/19390211.2020.1713959
71. Yu D, Zhou H, Yang Y, Jiang Y, Wang T, Lv L, et al. The bidirectional effects of hypothyroidism and hyperthyroidism on anxiety- and depression-like behaviors in rats. Hormones Behav. (2015) 69:106–15. doi: 10.1016/j.yhbeh.2015.01.003
72. Stipcevic T, Kusacic-Kuna S, Dezeljin M, Dodig D, Korsic M, Pivac N, et al. Platelet serotonin concentration and monoamine oxidase activity in hypothyroid patients. Hormone Res. (2009) 71:207–12. doi: 10.1159/000201109
73. Raij T, Raij K. Association between fatigue, peripheral serotonin, and L-carnitine in hypothyroidism and in chronic fatigue syndrome. Front Endocrinol. (2024) 15:1358404. doi: 10.3389/fendo.2024.1358404
74. Cleare AJ, McGregor A, O’Keane V. Neuroendocrine evidence for an association between hypothyroidism, reduced central 5-HT activity and depression. Clin Endocrinol. (1995) 43:713–9. doi: 10.1111/j.1365-2265.1995.tb00540.x
75. Cleare AJ, McGregor A, Chambers SM, Dawling S, O’Keane V. Thyroxine replacement increases central 5-hydroxytryptamine activity and reduces depressive symptoms in hypothyroidism. Neuroendocrinology. (1996) 64:65–9. doi: 10.1159/000127099
76. Kulikov AV, Jeanningro R. The effects of hypothyroidism on 5-HT1A and 5-HT2A receptors and the serotonin transporter protein in the rat brain. Neurosci Behav Physiol. (2001) 31:445–9. doi: 10.1023/A:1010401015064
77. Lee J-H, Lee M, Park J-A, Ryu YH, Lee KC, Kim KM, et al. Effects of hypothyroidism on serotonin 1A receptors in the rat brain. Psychopharmacology. (2018) 235:729–36. doi: 10.1007/s00213-017-4799-y
78. Savitz J. The kynurenine pathway: a finger in every pie. Mol Psychiatry. (2020) 25:131–47. doi: 10.1038/s41380-019-0414-4
79. Hazari N, Bhad R. Kynurenine pathway (KP) inhibitors: Novel agents for the management of depression. J Psychopharmacol (Oxford England). (2015) 29:1133–4. doi: 10.1177/0269881115599386
80. Krupa A, Łebkowska A, Kondraciuk M, Kaminski KA, Kowalska I. Alteration in kynurenine pathway metabolites in young women with autoimmune thyroiditis. Sci Rep. (2024) 14:6851. doi: 10.1038/s41598-024-57154-3
81. Schwarcz R, Bruno JP, Muchowski PJ, Wu H-Q. Kynurenines in the mammalian brain: when physiology meets pathology. Nat Rev Neurosci. (2012) 13:465–77. doi: 10.1038/nrn3257
82. Urbańska EM, Chmiel-Perzyńska I, Perzyński A, Derkacz M, Owe-Larsson B. Endogenous Kynurenic Acid and Neurotoxicity. In: Kostrzewa RM, editor. Handbook of Neurotoxicity. Springer New York, New York, NY (2014). p. 421–53. doi: 10.1007/978-1-4614-5836-4_92
83. Pocivavsek A, Elmer GI, Schwarcz R. Inhibition of kynurenine aminotransferase II attenuates hippocampus-dependent memory deficit in adult rats treated prenatally with kynurenine. Hippocampus. (2019) 29:73–7. doi: 10.1002/hipo.23040
84. Kozak R, Campbell BM, Strick CA, Horner W, Hoffmann WE, Kiss T, et al. Reduction of brain kynurenic acid improves cognitive function. J neuroscience: Off J Soc Neurosci. (2014) 34:10592–602. doi: 10.1523/JNEUROSCI.1107-14.2014
85. Tomczyk T, Urbańska EM. Experimental hypothyroidism raises brain kynurenic acid - Novel aspect of thyroid dysfunction. Eur J Pharmacol. (2020) 883:173363. doi: 10.1016/j.ejphar.2020.173363
86. Brown LA, Key BJ, Lovick TA. Bio-imaging of nitric oxide-producing neurones in slices of rat brain using 4,5-diaminofluorescein. J Neurosci Methods. (1999) 92:101–10. doi: 10.1016/S0165-0270(99)00098-9
87. Cano-Europa E, Pérez-Severiano F, Vergara P, Ortiz-Butrón R, Ríos C, Segovia J, et al. Hypothyroidism induces selective oxidative stress in amygdala and hippocampus of rat. Metab Brain Dis. (2008) 23:275–87. doi: 10.1007/s11011-008-9099-0
88. Alva-Sanchez C, Sanchez-Huerta K, Arroyo-Helguera O, Anguiano B, Aceves C, Pacheco-Rosado J. The maintenance of hippocampal pyramidal neuron populations is dependent on the modulation of specific cell cycle regulators by thyroid hormones. Brain Res. (2009) 1271:27–35. doi: 10.1016/j.brainres.2009.02.043
89. Alva-Sanchez C, Rodriguez A, Villanueva I, Anguiano B, Aceves C, Pacheco-Rosado J. The NMDA receptor antagonist MK-801 abolishes the increase in both p53 and Bax/Bcl2 index induced by adult-onset hypothyroidism in rat. Acta neurobiologiae experimentalis. (2014) 74:111–7. doi: 10.55782/ane-2014-1978
90. Steiner J, Walter M, Gos T, Guillemin GJ, Bernstein H-G, Sarnyai Z, et al. Severe depression is associated with increased microglial quinolinic acid in subregions of the anterior cingulate gyrus: evidence for an immune-modulated glutamatergic neurotransmission? J Neuroinflamm. (2011) 8:94. doi: 10.1186/1742-2094-8-94
91. Mormede P, Foury A, Barat P, Corcuff J-B, Terenina E, Marissal-Arvy N, et al. Molecular genetics of hypothalamic-pituitary-adrenal axis activity and function. Ann New York Acad Sci. (2011) 1220:127–36. doi: 10.1111/j.1749-6632.2010.05902.x
92. Hill MN, Tasker JG. Endocannabinoid signaling, glucocorticoid-mediated negative feedback, and regulation of the hypothalamic-pituitary-adrenal axis. Neuroscience. (2012) 204:5–16. doi: 10.1016/j.neuroscience.2011.12.030
93. Evanson NK, Tasker JG, Hill MN, Hillard CJ, Herman JP. Fast feedback inhibition of the HPA axis by glucocorticoids is mediated by endocannabinoid signaling. Endocrinology. (2010) 151:4811–9. doi: 10.1210/en.2010-0285
94. Sinha SR, Prakash P, Keshari JR, Kumari R, Prakash V. Assessment of serum cortisol levels in hypothyroidism patients: A cross-sectional study. Cureus. (2023) 15:e50199. doi: 10.7759/cureus.50199
95. Wahab Al-Shaban SA, Hassan Al-Bayati AA, Shaker A, Ali KA-W. Evaluation of Vitamin D3 and cortisol hormone levels in overt hypothyroidism sample of Iraqi patients visiting specialized National diabetes and endocrine centre. JPMA J Pakistan Med Assoc. (2021) 71:S67–71.
96. Berardelli I, Serafini G, Cortese N, Fiaschè F, O’Connor RC, Pompili M. The involvement of hypothalamus-pituitary-adrenal (HPA) axis in suicide risk. Brain Sci. (2020) 10(5):466. doi: 10.3390/brainsci10090653
97. Almeida FB, Pinna G, Barros HMT. The role of HPA axis and allopregnanolone on the neurobiology of major depressive disorders and PTSD. Int J Mol Sci. (2021) 22(11):5495. doi: 10.3390/ijms22115495
98. Hernández ME, Mendieta D, Martínez-Fong D, Loría F, Moreno J, Estrada I, et al. Variations in circulating cytokine levels during 52 week course of treatment with SSRI for major depressive disorder. Eur neuropsychopharmacology: J Eur Coll Neuropsychopharmacol. (2008) 18:917–24. doi: 10.1016/j.euroneuro.2008.08.001
99. Boyle MP, Brewer JA, Funatsu M, Wozniak DF, Tsien JZ, Izumi Y, et al. Acquired deficit of forebrain glucocorticoid receptor produces depression-like changes in adrenal axis regulation and behavior. Proc Natl Acad Sci United States America. (2005) 102:473–8. doi: 10.1073/pnas.0406458102
100. Hassamal S. Chronic stress, neuroinflammation, and depression: an overview of pathophysiological mechanisms and emerging anti-inflammatories. Front Psychiatry. (2023) 14:1130989. doi: 10.3389/fpsyt.2023.1130989
101. Pérez-Sánchez G, Becerril-Villanueva E, Arreola R, Martínez-Levy G, Hernández-Gutiérrez ME, Velasco-Velásquez MA, et al. Inflammatory profiles in depressed adolescents treated with fluoxetine: an 8-week follow-up open study. Mediators Inflammation. (2018) 2018:4074051. doi: 10.1155/2018/4074051
102. Lai R, Yin B, Feng Z, Deng X, Lv X, Zhong Y, et al. The causal relationship between 41 inflammatory cytokines and hypothyroidism: bidirectional two-sample Mendelian randomization study. Front Endocrinol (Lausanne). (2024) 14:1332383. doi: 10.3389/fendo.2023.1332383
103. Papanas N, Papazoglou D, Papatheodorou K, Antonoglou C, Kotsiou S, Maltezos E. Thyroxine replacement dose in patients with Hashimoto disease: A potential role for interleukin-6. Cytokine. (2006) 35:166–70. doi: 10.1016/j.cyto.2006.07.017
104. Drugarin D, Negru S, Koreck A, Zosin I, Cristea C. The pattern of a T(H)1 cytokine in autoimmune thyroiditis. Immunol Lett. (2000) 71:73–7. doi: 10.1016/S0165-2478(99)00156-X
105. Figueroa-Vega N, Alfonso-Pérez M, Benedicto I, Sánchez-Madrid F, González-Amaro R, Marazuela M. Increased circulating pro-inflammatory cytokines and Th17 lymphocytes in Hashimoto’s thyroiditis. J Clin Endocrinol Metab. (2010) 95:953–62. doi: 10.1210/jc.2009-1719
106. Liu F, Yang Y, Fan XW, Zhang N, Wang S, Shi YJ, et al. Impacts of inflammatory cytokines on depression: a cohort study. BMC Psychiatry. (2024) 24:1–9. doi: 10.1186/S12888-024-05639-W/TABLES/4
107. Elwood E, Lim Z, Naveed H, Galea I. The effect of systemic inflammation on human brain barrier function. Brain Behav Immun. (2017) 62:35–40. doi: 10.1016/j.bbi.2016.10.020
108. Felger JC, Lotrich FE. Inflammatory cytokines in depression: neurobiological mechanisms and therapeutic implications. Neuroscience. (2013) 246:199. doi: 10.1016/j.neuroscience.2013.04.060
109. Tayde PS, Bhagwat NM, Sharma P, Sharma B, Dalwadi PP, Sonawane A, et al. Hypothyroidism and depression: are cytokines the link? Indian J Endocrinol Metab. (2017) 21:886. doi: 10.4103/ijem.IJEM_265_17
110. Balu DT, Lucki I. Adult hippocampal neurogenesis: regulation, functional implications, and contribution to disease pathology. Neurosci Biobehav Rev. (2009) 33:232–52. doi: 10.1016/j.neubiorev.2008.08.007
111. Altman J. Autoradiographic investigation of cell proliferation in the brains of rats and cats. Anatomical Rec. (1963) 145:573–91. doi: 10.1002/ar.1091450409
112. Gould E, Cameron HA, Daniels DC, Woolley CS, McEwen BS. Adrenal hormones suppress cell division in the adult rat dentate gyrus. J neuroscience: Off J Soc Neurosci. (1992) 12:3642–50. doi: 10.1523/JNEUROSCI.12-09-03642.1992
113. Kronenberg G, Reuter K, Steiner B, Brandt MD, Jessberger S, Yamaguchi M, et al. Subpopulations of proliferating cells of the adult hippocampus respond differently to physiologic neurogenic stimuli. J Comp Neurol. (2003) 467:455–63. doi: 10.1002/cne.10945
114. Seri B, Garcia-Verdugo JM, McEwen BS, Alvarez-Buylla A. Astrocytes give rise to new neurons in the adult mammalian hippocampus. J neuroscience: Off J Soc Neurosci. (2001) 21:7153–60. doi: 10.1523/JNEUROSCI.21-18-07153.2001
115. Steiner B, Klempin F, Wang L, Kott M, Kettenmann H, Kempermann G. Type-2 cells as link between glial and neuronal lineage in adult hippocampal neurogenesis. Glia. (2006) 54:805–14. doi: 10.1002/glia.20407
116. Zhao C, Teng EM, Summers RGJ, Ming G-L, Gage FH. Distinct morphological stages of dentate granule neuron maturation in the adult mouse hippocampus. J neuroscience: Off J Soc Neurosci. (2006) 26:3–11. doi: 10.1523/JNEUROSCI.3648-05.2006
117. Toni N, Sultan S. Synapse formation on adult-born hippocampal neurons. Eur J Neurosci. (2011) 33:1062–8. doi: 10.1111/j.1460-9568.2011.07604.x
118. Chaudhury D, Liu H, Han M-H. Neuronal correlates of depression. Cell Mol Life sciences: CMLS. (2015) 72:4825–48. doi: 10.1007/s00018-015-2044-6
119. Baptista P, Andrade JP. Adult hippocampal neurogenesis: regulation and possible functional and clinical correlates. Front Neuroanat. (2018) 12:44. doi: 10.3389/fnana.2018.00044
120. Schloesser RJ, Manji HK, Martinowich K. Suppression of adult neurogenesis leads to an increased hypothalamo-pituitary-adrenal axis response. Neuroreport. (2009) 20:553–7. doi: 10.1097/WNR.0b013e3283293e59
121. Snyder JS, Soumier A, Brewer M, Pickel J, Cameron HA. Adult hippocampal neurogenesis buffers stress responses and depressive behaviour. Nature. (2011) 476:458–61. doi: 10.1038/nature10287
122. Sahay A, Wilson DA, Hen R. Pattern separation: a common function for new neurons in hippocampus and olfactory bulb. Neuron. (2011) 70:582–8. doi: 10.1016/j.neuron.2011.05.012
123. Desouza LA, Ladiwala U, Daniel SM, Agashe S, Vaidya RA, Vaidya VA. Thyroid hormone regulates hippocampal neurogenesis in the adult rat brain. Mol Cell Neurosci. (2005) 29:414–26. doi: 10.1016/j.mcn.2005.03.010
124. Ambrogini P, Cuppini R, Ferri P, Mancini C, Ciaroni S, Voci A, et al. Thyroid hormones affect neurogenesis in the dentate gyrus of adult rat. Neuroendocrinology. (2005) (4):244–53. doi: 10.1159/000087648
125. Montero-Pedrazuela A, Venero C, Lavado-Autric R, Fernández-Lamo I, García-Verdugo JM, Bernal J, et al. Modulation of adult hippocampal neurogenesis by thyroid hormones: Implications in depressive-like behavior. Mol Psychiatry. (2006) (4):361–71. doi: 10.1038/sj.mp.4001802
126. Sanchez-Huerta K, Garcia-Martinez Y, Vergara P, Segovia J, Pacheco-Rosado J. Thyroid hormones are essential to preserve non-proliferative cells of adult neurogenesis of the dentate gyrus. Mol Cell Neurosci. (2016) 76:1–10. doi: 10.1016/j.mcn.2016.08.001
127. Fanibunda SE, Desouza LA, Kapoor R, Vaidya RA, Vaidya VA. Thyroid hormone regulation of adult neurogenesis. Vitamins hormones. (2018) 106:211–51. doi: 10.1016/bs.vh.2017.04.006
128. Kapoor R, Van Hogerlinden M, Wallis K, Ghosh H, Nordstrom K, Vennstrom B, et al. Unliganded thyroid hormone receptor α1 impairs adult hippocampal neurogenesis. FASEB J. (2010) 24(12):4793–805. doi: 10.1096/fj.10-161802
129. Kapoor R, Fanibunda SE, Desouza LA, Guha SK, Vaidya VA. Perspectives on thyroid hormone action in adult neurogenesis. J Neurochemistry. (2015) 133(5):599–616. doi: 10.1111/jnc.13093
130. da Conceição RR, Laureano-Melo R, Oliveira KC, de Carvalho Melo MC, Kasamatsu TS, de Barros Maciel RM, et al. Antidepressant behavior in thyroidectomized Wistar rats is induced by hippocampal hypothyroidism. Physiol Behav. (2016) 157:158–64. doi: 10.1016/j.physbeh.2016.02.005
131. Bilous II, Korda MM, Krynytska IY, Kamyshnyi AM. Nerve impulse transmission pathway-focused genes expression analysis in patients with primary hypothyroidism and autoimmune thyroiditis. Endocrine regulations. (2020) 54:109–18. doi: 10.2478/enr-2020-0013
132. Bilous II, Pavlovych LL, Kamyshnyi AM. Primary hypothyroidism and autoimmune thyroiditis alter the transcriptional activity of genes regulating neurogenesis in the blood of patients. Endocrine regulations. (2021) 55:5–15. doi: 10.2478/enr-2021-0002
133. Sui L, Ren W-W, Li B-M. Administration of thyroid hormone increases reelin and brain-derived neurotrophic factor expression in rat hippocampus. vivo. Brain Res. (2010) 1313:9–24. doi: 10.1016/j.brainres.2009.12.010
134. Linnarsson S, Willson CA, Ernfors P. Cell death in regenerating populations of neurons in BDNF mutant mice. Brain Res Mol Brain Res. (2000) 75:61–9. doi: 10.1016/S0169-328X(99)00295-8
135. Scharfman H, Goodman J, Macleod A, Phani S, Antonelli C, Croll S. Increased neurogenesis and the ectopic granule cells after intrahippocampal BDNF infusion in adult rats. Exp Neurol. (2005) 192:348–56. doi: 10.1016/j.expneurol.2004.11.016
136. Porter GA, O’Connor JC. Brain-derived neurotrophic factor and inflammation in depression: Pathogenic partners in crime? World J Psychiatry. (2022) 12:77–97. doi: 10.5498/wjp.v12.i1.77
137. Eitan R, Landshut G, Lifschytz T, Einstein O, Ben-Hur T, Lerer B. The thyroid hormone, triiodothyronine, enhances fluoxetine-induced neurogenesis in rats: possible role in antidepressant-augmenting properties. Int J Neuropsychopharmacol. (2010) 13:553–61. doi: 10.1017/S1461145709990769
138. Pilhatsch M, Marxen M, Winter C, Smolka MN, Bauer M. Hypothyroidism and mood disorders: integrating novel insights from brain imaging techniques. Thyroid Res. (2011) 4 Suppl 1:S3. doi: 10.1186/1756-6614-4-S1-S3
139. Alva-Sánchez C, Ortiz-Butrón R, Pacheco-Rosado J. Kainic acid does not affect CA3 hippocampal region pyramidal cells in hypothyroid rats. Brain Res Bull. (2004) 63:167–71. doi: 10.1016/j.brainresbull.2004.02.002
140. Madeira MD, Sousa N, Lima-Andrade MT, Calheiros F, Cadete-Leite A, Paula-Barbosa MM. Selective vulnerability of the hippocampal pyramidal neurons to hypothyroidism in male and female rats. J Comp Neurol. (1992) 322:501–18. doi: 10.1002/cne.903220405
141. Cooke GE, Mullally S, Correia N, O’Mara SM, Gibney J. Hippocampal volume is decreased in adults with hypothyroidism. Thyroid. (2014) 24(3):433–40. doi: 10.1089/thy.2013.0058
142. Zhang T, Zhao L, Chen C, Yang C, Zhang H, Su W, et al. Structural and functional alterations of hippocampal subfields in patients with adult-onset primary hypothyroidism. J Clin Endocrinol Metab. (2024). doi: 10.1210/clinem/dgae070
143. Toll A, Blanco-Hinojo L, Berge D, Manzano A, El Abidi K, Perez-Solà V, et al. Relationship between thyroid-stimulating hormone, BDNF levels, and hippocampal volume in antipsychotic-naïve first-episode psychosis patients. Front Psychiatry. (2023) 14:1301714. doi: 10.3389/fpsyt.2023.1301714
144. Bauer M, Silverman DHS, Schlagenhauf F, London ED, Geist CL, Van Herle K, et al. Brain glucose metabolism in hypothyroidism: A positron emission tomography study before and after thyroid hormone replacement therapy. J Clin Endocrinol Metab. (2009) 94(8):2922–9. doi: 10.1210/jc.2008-2235
145. Singh S, Modi S, Bagga D, Kaur P, Shankar LR, Khushu S. Voxel-based morphometric analysis in hypothyroidism using diffeomorphic anatomic registration via an exponentiated lie algebra algorithm approach. J Neuroendocrinol. (2013) 25:229–34. doi: 10.1111/jne.12001
146. Leyhe T, Ethofer T, Bretscher J, Künle A, Säuberlich A-L, Klein R, et al. Low performance in attention testing is associated with reduced grey matter density of the left inferior frontal gyrus in euthyroid patients with Hashimoto’s thyroiditis. Brain behavior Immun. (2013) 27:33–7. doi: 10.1016/j.bbi.2012.09.007
Keywords: acquired hypothyroidism, depression, anxiety, TSH, triiodothyronine (T3), thyroxine (T4), levothyroxine, L-T4
Citation: Osnaya-Brizuela N, Valenzuela-Peraza A, Santamaría-del Ángel D, García-Martínez Y, Pacheco-Rosado J, Pérez-Sánchez G and Sánchez-Huerta K (2024) Is the acquired hypothyroidism a risk factor for developing psychiatric disorders? Front. Psychiatry 15:1429255. doi: 10.3389/fpsyt.2024.1429255
Received: 07 May 2024; Accepted: 20 June 2024;
Published: 19 July 2024.
Edited by:
Ali Saffet Gonul, Ege University, TürkiyeReviewed by:
Dimitar Bakalov, Medical University Sofia, BulgariaVladimir Janjic, University of Kragujevac, Serbia
Maria Giuseppina Petruzzelli, University of Bari Aldo Moro, Italy
Copyright © 2024 Osnaya-Brizuela, Valenzuela-Peraza, Santamaría-del Ángel, García-Martínez, Pacheco-Rosado, Pérez-Sánchez and Sánchez-Huerta. This is an open-access article distributed under the terms of the Creative Commons Attribution License (CC BY). The use, distribution or reproduction in other forums is permitted, provided the original author(s) and the copyright owner(s) are credited and that the original publication in this journal is cited, in accordance with accepted academic practice. No use, distribution or reproduction is permitted which does not comply with these terms.
*Correspondence: Karla Sánchez-Huerta, a3NhbmNoZXpoQHBlZGlhdHJpYS5nb2IubXg=; Gilberto Pérez-Sánchez, Z2lsYmVydG8ucGVyZXouc2FuY2hlekBpbnByZi5nb2IubXg=
†ORCID: Norma Osnaya-Brizuela, orcid.org/0000-0003-0041-3190
Armando Valenzuela-Peraza, orcid.org/0000-0002-9293-186X
Daniel Santamaría del Ángel, orcid.org/0000-0003-3891-8055
Yuliana García-Martínez, orcid.org/0000-0002-9103-0154
Jorge Pacheco-Rosado, orcid.org/0000-0003-4531-8875
Gilberto Pérez-Sánchez, orcid.org/0000-0003-3878-0631
Karla Sánchez-Huerta, orcid.org/0000-0001-8745-8118