- 1Department of Psychiatry, Seoul National University Hospital, Seoul, Republic of Korea
- 2Department of Psychological and Brain Sciences, Texas A&M University, College Station, TX, United States
- 3Department of Psychiatry, Seoul National University College of Medicine and Institute of Human Behavioral Medicine, Seoul National University-Medical Research Center (SNU-MRC), Seoul, Republic of Korea
Introduction: While abnormal responses to threat, including overgeneralization to conditioned fear, have been postulated to play a critical role in pathological anxiety, the relevance of previous findings to social anxiety disorder (SAD) is unclear. We investigated conditioned and generalized fear responses in patients with SAD using socially relevant stimuli.
Methods: A total of 26 patients with SAD and 25 healthy controls participated in a fear conditioning and generalization paradigm consisting of two neutral faces as conditioned stimuli (CS+ or CS−) and an angry face with contemptuous comments as unconditioned stimuli. Eight morphed faces of two conditioned stimuli in each continuum were given to test generalization. Behavioral data and physiological responses were acquired.
Results: Successful conditioning was observed in the risk ratings for both groups, while only a marginal indication of conditioning was noted in physiological measures. During the generalization phase, patients rated the risk higher than CS− when the stimuli close to CS− contained a portion of CS+ features. Larger skin conductance responses to this stimulus were linked to higher fear of negative evaluation. In addition, patients spent a longer time evaluating safe and ambiguous stimuli than healthy controls and exhibited consistently high levels of subjective arousal.
Discussion: Taken together, our findings suggest that SAD patients may exhibit a tendency towards overgeneralization of fear responses and show distinct patterns in processing generalized threat stimuli compared to healthy controls. Even though overgeneralization was not evident in physiological measures, it is necessary to consider this behavioral characteristic in the clinical management of patients with SAD.
1 Introduction
Social anxiety disorder (SAD) is one of the most common mental disorders, with 13% life prevalence in the United States, 8% in Canada, and 8.4% in Australia (1–3). SAD is characterized by an intense and persistent fear of being judged, criticized, or embarrassed in social situations, leading to extreme distress and/or avoidance of social situations (4). A diagnosis of SAD is associated with an increased risk of developing other mental disorders such as mood disorders, other anxiety disorders, substance use disorders, and independently predicts suicidal ideation, attempts, and completion (5–7). The co-occurring mental health challenges linked to SAD not only pose significant individual burdens but also contribute to a broader societal impact, increasing indirect costs, such as reduced workplace productivity (8).
Fear conditioning has been widely employed as a laboratory model to investigate the development and maintenance of fear and anxiety (9–13). Classical fear conditioning represents the learning process by which a neutral stimulus, through repetitive association with an unconditioned aversive stimulus (US), comes to induce fear responses and consequently becomes a conditioned stimulus (CS+). Individuals with anxiety disorders have been reported to exhibit maladaptive characteristics in distinct mechanisms of fear conditioning: 1) impaired fear extinction and 2) overgeneralization of conditioned fear (11–14). Fear extinction typically involves a decrease in conditioned fear response after repeated exposure to the CS+ in the absence of the US. However, findings from previous meta-analyses have shown that during extinction trials, patients with anxiety-related disorders tend to display heightened autonomic fear responses to the CS+ that is no longer predictive of the US (9, 10). Furthermore, compared to controls, patients demonstrate increased differentiation between the CS+ and the CS− (the safe stimulus that was never paired with the US) during extinction (9, 10). These results suggest that conditioned fear responses to the CS+ are more resistant to extinction in patients compared to controls. In addition to impaired fear extinction, patients with anxiety disorders often exhibit maladaptive fear generalization, where conditioned fear responses extend beyond the original CS+ to other stimuli resembling it. While fear generalization serves as an adaptive mechanism that protects an individual from potential harm, it becomes maladaptive when fear extends to a broader range of harmless stimuli. Emerging evidence indicates heightened fear generalization in anxiety disorders, as measured by stronger conditioned fear responses to generalization stimuli resembling the CS+ but never paired with the US, compared to healthy controls (14).
Conceptually, fear generalization can play a crucial role not only in the acquisition of fear but also in the maintenance of SAD (15). For instance, an individual may develop fear after experiencing the humiliation of being criticized during a public speech in a specific location. The fear response stemming from this negative feedback may generalize to different environments or other social situations, subsequently leading to avoidance behavior and, thus, impeding safety learning. Difficulties in distinguishing between danger and safety cues have been experimentally demonstrated in previous studies in SAD (16–18). However, given that anxiety-evoking stimuli outside laboratory setting are generally more uncertain and vague, a fear generalization paradigm using ambiguous generalization stimuli is likely to better reflect clinical anxiety compared to tests involving simple discrimination between CS+ and CS− (14). In addition, anxiety-inducing stimuli vary across different psychopathological dysfunctions. When applying the fear conditioning paradigm to social anxiety subjects, researchers have found that socially relevant stimuli such as emotional facial expressions and verbal feedback induce greater fear responses in patients (19) and highly socially anxious individuals (20). Such patterns, however, have not been observed in experiments using other nonspecific aversive stimuli such as odor or painful pressure (18, 21). This underscores the significance of incorporating disorder-relevant stimuli in the fear conditioning paradigm.
To date, few studies have investigated the fear generalization in SAD. Ahrens et al. employed two faces as CS+ and CS−, along with a loud scream and a fearful face as the US (17). They assessed generalization by presenting both CSs and ambiguous generalization stimuli comprising four morphs of the two faces. The researchers concluded that there is no solid and robust evidence of overgeneralization in SAD and suggested that, while socially relevant, the screaming sound employed as the US might not have been disorder-specific enough to elicit an overgeneralization pattern in patients (17). Given that the core fear of SAD is related to social or performance situations wherein negative judgment is anticipated (4), stimuli such as angry faces and contemptuous comments may be more appropriate as US (17, 19, 20). Incorporating these disorder-relevant stimuli into the fear generalization paradigm could offer a more detailed and clinically relevant understanding of social anxiety.
The main objective of this study was to investigate whether an ecologically enhanced fear conditioning paradigm using disorder-specific stimuli (i.e., negative evaluation) can elicit a maladaptive pattern of overgeneralization in social anxiety. To this aim, we employed a social conditioning paradigm (19) using pictures of male/female faces as the CSs and social anxiety-related aversive stimulus (contemptuous auditory comment) as the US. In the paradigm, the generalization phase following fear acquisition involved generalization stimuli (GS), which were eight morphed faces along a continuum between CS+ and CS−, none of which were paired with the US. Fear responses were assessed by behavioral (risk rating and reaction times), physiological (skin conductance and heart rate), and fear-potentiated startle (electromyography, EMG) responses. Based on the generalization paradigm, we anticipated that, as the presented GSs decrease in resemblance to CS+, subjects would exhibit a decrease in risk ratings, skin conductance, and startle EMG but an increase in heart rate (due to more pronounced heart rate deceleration, i.e., fear bradycardia, to stimuli closer to CS+). As an indicator of stimulus discrimination, reaction times were expected to be slower for ambiguous GSs resembling both CS+ and CS− to some extent. Based on previous findings that socially anxious individuals have difficulties in discriminating threat and safety cues (16–20), we hypothesized that patients with SAD, compared to controls, would generalize their conditioned fear responses to a wider range of GSs. Specifically, this would manifest as heightened fear responses to GSs closer to CS− than to CS+ in patients (but not in controls).
2 Materials and methods
2.1 Participants and measurements
A total of 28 patients with SAD and 39 healthy controls were recruited from the psychiatric outpatient clinic and the community through an advertisement. One patient and two controls dropped out of the study, as the loud acoustic startle probe was intolerable for them. Physiological data acquired from one patient was missing. A total 12 controls were excluded due to high levels of social anxiety and/or depressive symptoms. These participants had an average Liebowitz Social Anxiety Scale score of 42.9 (standard deviation=10.9), which was higher than the mean of 18.0 (standard deviation=9.6) for the included controls. However, they volunteered as controls, did not report social anxiety symptoms, and did not meet the full diagnostic criteria for SAD. Ultimately, 26 patients with SAD and 25 controls were included in the statistical analyses. As presented in Table 1, the demographic variables, including age, sex, and education, did not differ significantly between the two groups.
Patients were diagnosed with SAD when they met the full criteria for SAD according to the Diagnostic and Statistical Manual of Mental Disorders Fifth Edition through an intensive and open-ended clinical interview with a psychiatrist. When SAD was the primary diagnosis, patients who had related depressive disorder were also included. Through an additional structured interview, specifically the Mini-International Neuropsychiatric Interview (22), a psychologist assessed patients’ comorbidities and excluded control participants with past or current psychiatric disorders. Exclusion criteria for both groups included a past or current history of neurological disorders, pregnancy, or a past or current diagnosis of psychosis. The numbers of patients with past or current comorbid psychiatric disorders were as follows: major depression (n = 20), panic disorder (n = 3), agoraphobia (n = 7), general anxiety disorder (n = 6), obsessive–compulsive disorder (n = 1), eating disorder (n = 3), and premenstrual dysphoric disorder (n = 8).
To measure the severity of clinical symptoms, participants completed self-reported questionnaires, including the Liebowitz Social Anxiety Scale (LSAS) (23), the Social Interaction Anxiety Scale (SIAS) and the Social Phobia Scale (SPS) (24), a brief version of the Fear of Negative Evaluation scale (B-FNE) (25), the State-Trait Anxiety Inventory (STAI) (26), the Beck Anxiety Inventory (BAI) (27), and the Beck Depression Inventory (BDI) (28). Participants were also assessed using the Hamilton Anxiety Scale (HAS) (29), Montgomery–Åsberg Depression Rating Scale (MADRS) (30), and Sheehan Disability Scale (31). Patients showed overall higher clinical symptom scores than the controls, including the scores for general anxiety (STAI, BAI, and HAS) and depressed mood (BDI and MADRS), and social anxiety severity (LSAS, SIAS, SPS, and B-FNE) (Table 1).
The study protocol was approved by the Institutional Review Board of Seoul National University Hospital and was conducted in accordance with the Declaration of Helsinki. Written informed consent was obtained from all participants.
2.2 Stimulus
Two pictures of actors with neutral facial expressions were selected from the Korean Facial Expressions of Emotion (32) as the CS. An audiovisual stimulus comprising an angry facial expression of a threat cue (CS+) paired with a simultaneously presented contemptuous comment (e.g., “you are good for nothing!”) served as the US. The other CS face was included as a safety cue (CS−) and was associated with the neutral content (e.g., “banana is yellow”). Details about the selection of the US are described in Supplementary Material. Given the clinical findings that the level of social anxiety increases when dealing with the opposite sex (33), the participants were conditioned with pictures of the opposite sex. Female participants were presented with CS featuring male faces and US with a male voice. Conversely, male participants were exposed to CS featuring female faces and US with a female voice. The order of the stimuli was pseudo-randomized, and the allocation of which actor was assigned as being the CS+ was also balanced out across the participants.
Eight morphed faces of the CS faces in each continuum were given to test generalization (GS); GS1 refers to the one closest to CS+, and GS8 was closest to CS–. Then, we grouped the two adjacent stimuli as one class, i.e., GS1 and GS2 as Class 1 (C1), GS3 and GS4 as C2, and so forth. Hence, C1 was the most similar to CS+, and C4 showed the greatest difference with respect to CS+ (Figure 1).
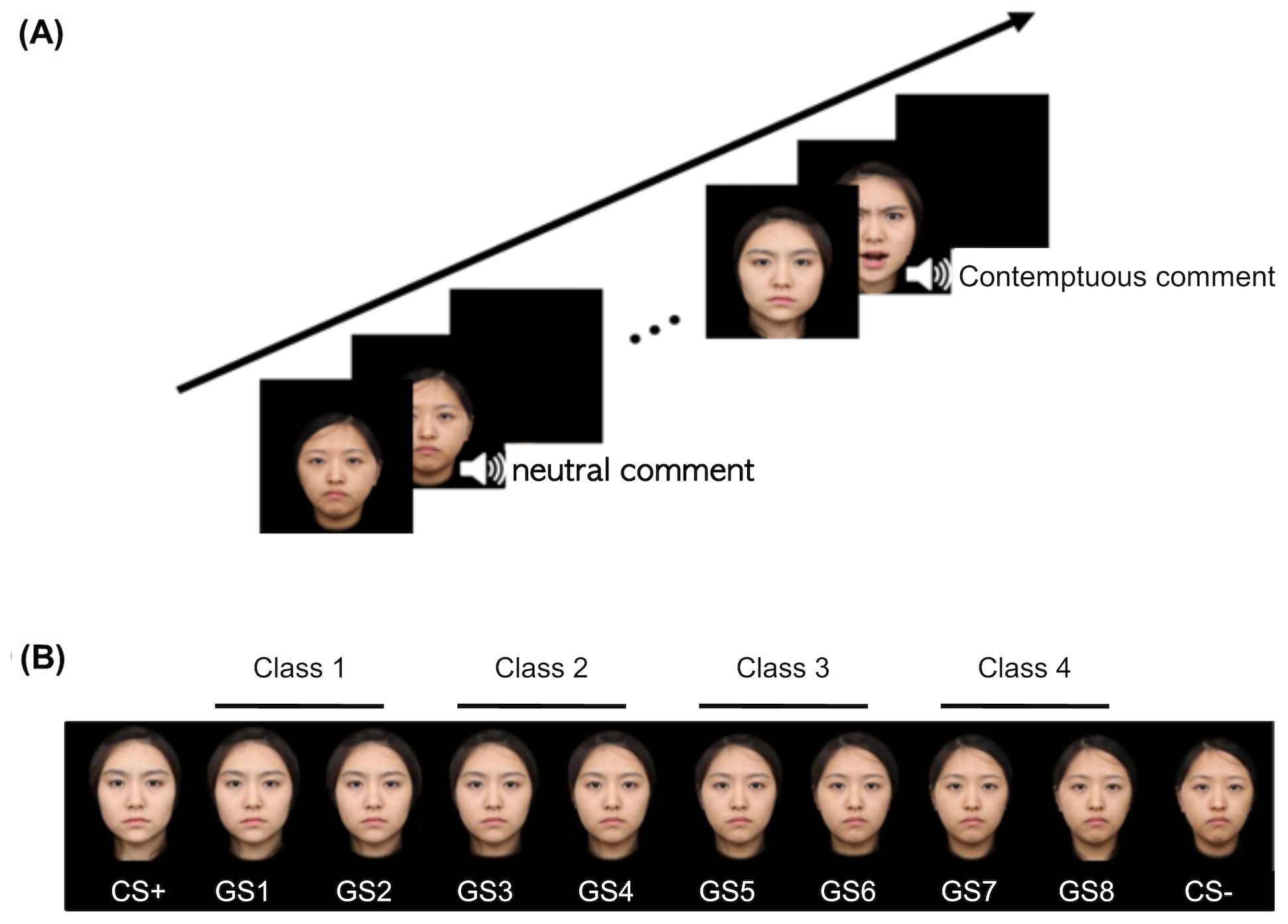
Figure 1. An example of conditioning and generalization stimuli. (A) A safe cue (CS−) was paired only with a neutral comment and neutral face, while a threat cue (CS+) was paired with a contemptuous comment and an angry face. (B) Generalization stimuli comprise eight morphed faces of two conditioning stimuli, which were collapsed into four different classes such that Class 1 is the closest to the CS+ and Class 4 to the CS−. Each item of GSs differs from the other by 12.5%. CS, conditioned stimuli; GS, generalized stimuli.
The startle probe, a burst of white noise (103 dB, 50 ms) was given binaurally through headphones (34).
2.3 Design and procedure
The experiment paradigm comprises the habituation of the startle sound, pre-acquisition, acquisition, and generalization phases. In the habituation phase, four trials of the white noise were presented with a blank screen. This phase was introduced to facilitate participants’ adaptation to the startle probe, and no specific instructions were provided. After the habituation phase, the pre-acquisition, acquisition, and generalization phases were presented sequentially. Throughout each phase, participants were instructed to provide risk ratings indicating the likelihood of the US occurring while observing the presented facial stimuli. At the end of each phase, participants were directed to rate the valence, arousal, and threat associated with the presented facial stimuli. Detailed explanations regarding the ratings will be provided below. The pre-acquisition phase consisted of six CS+ and six CS− with no subsequent US presentation. During the acquisition phase, 12 of each of the CS faces were presented with 83% of the reinforcement schedule, i.e., 10 of each CS trial were followed by either a neutral comment with a neutral facial expression (CS−) or a contemptuous comment with an angry facial expression (CS+). Similarly, in the generalization phase, a total of 12 trials of each CS and six trials per GSs were given. To prevent early extinction, 50% of the reinforcement schedule was given during the generalization phase. All CSs and GSs over a black background were presented for 6 s on the center of a screen. The US, either neutral or contemptuous, followed immediately for 3 s. A blank screen with a black background was presented for either 9 s or 11 s as an interval inter-trial to minimize the expectancy effect. The startle probe was given in half of the trials at either 4 s or 5 s after the onset of the CS.
Participants’ subjective risk ratings and reaction times (RTs) for these risk ratings were measured as behavior indices. During half of the CS and GS trials, the risk rating scale appeared at the bottom of the screen 3 s after stimulus onset. The scale ranged from 1 (not at all) to 3 (highly probable). Participants were instructed to rate the likelihood of hearing a contemptuous comment using three buttons on a numeric keyboard. Subjects were told to respond as quickly as possible to each stimulus. At the end of each pre-acquisition, acquisition, and generalization phase, participants were instructed to rate the valence, arousal, and threat for CS+ and CS−. Each stimulus was presented on the screen, followed by three 9-point Likert scales, each reflecting levels of valence (“How negative or positive was the stimulus?,” 1 = negative, 9 = positive), arousal (“How arousing was the stimulus?,” 1 = low, 9 = high), and threat (“How threatening was the stimulus?,” 1 = not at all, 9 = threatening). Participants were instructed to respond using the nine buttons on a numeric keyboard.
The paradigm was delivered by Presentation® software (Neurobehavioral Systems Inc., Berkeley, CA, USA) on a monitor at approximately 60 cm.
2.4 Physiological data acquisition and analysis
The participants underwent the study individually in a temperature- and humidity-controlled room (23°C ± 3°C and 40% ± 5%, respectively). Skin conductance level (SCL), heart rate (HR) as a physiological response, and EMG as the startle response were acquired with an MP-150 system (BIOPAC Systems Inc., Goleta, CA, USA) at a sample rate of 1,000 kHz and analyzed using AcqKnowledge software (BIOPAC Systems Inc.). For all measurements, data with less than two valid responses per stimuli for each phase were excluded (35).
Skin conductance level was recorded using two Ag/AgCl electrodes filled with an isotonic electrode gel from the distal phalanges of the second and third digits of the non-dominant hand. The acquired skin conductance recording was filtered by a 50-Hz notch filter and a 1-Hz cutoff filter. Responses lower than 0.02 μS were filtered out. Based on previous studies that applied a range correction to SCL to account for individual differences (36–39), the SCL values were calculated by subtracting the minimum SCL (within 0–2 s before the stimulus picture onset) from the stimulus-specific onset SCL and then dividing by the difference between the maximum [within 1–6 s following the stimulus picture onset (40)] and the minimum SCL. The results of the analyses using different time windows are presented in Supplementary Material.
HR was measured using a photoplethysmography transducer at 50 Hz from the fourth digits of the non-dominant hand. First, the estimated beats per minute for each stimulus (1–6 s from the stimulus picture onset) and the baseline HR of 2 s before each onset was automatically driven by the AcqKnowledge software and then averaged out. Then, we deducted the baseline HR estimate from the averaged HR.
Eyeblink magnitude to the startle was measured using EMG from the orbicularis oculi with two Ag/AgCl electrodes (diameter, 4 mm) placed on the lower eyelids centrally located in line with the pupil and under the lateral canthus of the left eye. In addition, the isolated ground electrode was placed at the mastoid. Impedance was kept below 5 kΩ. The acquired signal was amplified and filtered with a 50-Hz notch filter and band-pass at 28–500 Hz. Then, it was rectified and smoothed with a 1,000-Hz sample rate. Baseline correction was performed for over 50 ms before the startle onset. The peak EMG per stimuli was calculated with a time window of 50–200 ms after the startle onset. Artifact rejection was completed manually with an exclusion criterion of responses with baseline shifts above or below 5 μV. Trial-irrelevant movements occurring at the end of the session were eliminated, and the percentage fell within the range of 3%–5%. No trials were excluded due to movement artifacts. Within-subject T-score standardization was applied to the EMG magnitudes across all phases.
2.5 Statistical analysis
Statistical analyses were conducted using SPSS (version 26; SPSS Inc., Chicago, IL, USA) and R (R Foundation for Statistical Computing, Vienna, Austria, 2012). In pre-acquisition, we applied 2 (Group: controls, SAD) × 2 (Condition: CS+, CS−) rmANOVA. To assess the acquisition of conditioning over time, the acquisition phase was analyzed by dividing it into early and late phases. Each phase was defined as the first (6 CS+ and 6 CS−) and the second halves (6 CS+ and 6 CS−) of the experiment. Then, we carried out 2 (Group: controls, SAD) × 2 (Condition: CS+, CS−) × 2 (Phase: early, late) rmANOVA. In the generalization phase, we carried out 2 (Group: controls, SAD) × 6 (Stimulus: CS+, C1, C2, C3, C4, and CS−) rmANOVA. Dependent variables were risk rating, RT, SCL, HR, and startle EMG. No SCL data were available for CS− during the late acquisition phase for one participant in the CON group. Additionally, no startle EMG data were available for CS+ during the early acquisition phase for another participant in the CON group. As a result, each participant was excluded from the SCL and startle EMG analysis of the acquisition phase, respectively, but was included in all other analyses.
To test across-phase changes in subjective ratings and physiological measures for CS+ and CS−, 2 (Group: controls, SAD) × 2 (Time: acquisition, generalization) × 2 (Condition: CS+, CS−) rmANOVA was conducted. Dependent variables include subjective ratings of valence, arousal, and threat and physiological outcomes such as SCL, HR, and startle EMG. Lastly, we investigated whether these behavioral and physiological outcomes are correlated with social anxiety symptoms such as LSAS, SIAS, SPS, and FNE. Pearson correlation analysis was conducted followed by partial correlation analysis to control for the effect of general anxiety, with trait anxiety serving as a control variable.
For analyses of variance, the Greenhouse–Geisser correction (ϵ) was applied when the assumption of sphericity was violated. Analyses incorporating BDI and BAI as covariates were also conducted, and the results can be found in Supplementary Material. The Benjamini–Hochberg (B–H) correction was used to correct the alpha level of multiple comparisons for post-hoc t-tests (30) except for correlation analysis. A significance level for the p-value of 0.05 was applied across the analyses, and the effect sizes were reported using partial eta-squared (η2p).
Sample size was determined by power calculations for repeated measures ANOVA, using an effect size of 0.77 from a previous fear conditioning study in social anxiety disorder (17). With an alpha of 0.05 and a statistical power of 0.95, the sample size of 20 for each group is required.
3 Results
3.1 Pre-acquisition phase
The rmANOVA results with two factors (i.e., Group as a between-subject factor and Condition as a within-subject factor) revealed no significant main or interaction effects on risk rating (all, p >.05). For RT, the main effect of Condition was the only significant result [F(1,44) = 4.087, p =.049, η2p =.085]; RT of CS− was longer than that of CS+. Results regarding physiological outcomes indicated that there was no significant main or interaction effect on SCL, HR, or startle EMG (all, p >.05).
3.2 Acquisition phase
For risk rating, a significant Condition × Time interaction was observed [F(1,45) = 19.375, p <.001, η2p=.301] (Table 2). Post-hoc t-tests indicated that the risk rating for CS+ increased significantly from 2.34 to 2.71 over time (t = −5.429, p <.001), whereas there was no significant change for CS− (t = 1.510, p =.130) (early: 1.23, late: 1.12). Further examination of changes in risk rating for CS− within each group revealed a significant decrease from 1.30 to 1.02 in controls (t = 2.658, p = .015). However, risk rating for CS− did not change in SAD (t = −0.334, p =.741) (early: 1.18, late: 1.21), indicating that SAD did not adjust their threat evaluation for CS− even after repeated learning that CS− is not associated with US. The main effects of Condition [F(1,45) = 375.908, p <.001, η2p=.893] and Time [F(1,45) = 7.027, p =.011, η2p =.135] were significant. No other significant main or interaction effect was found.
For RT, there was no significant interaction effect (all, p >.05). However, a significant main effect of Condition suggests longer RT for CS+ compared to CS− [F(1,43) = 5.226, p =.027, η2p=.108]. Additionally, the main effect of Time indicates that participants judged faster in the late phase (128.36 ms) compared to the early phase (140.54 ms) [F(1,43) = 4.194, p =.047, η2p=.089]. No other significant main or interaction effect was found.
For SCL, there was a marginal main effect of Condition [F(1,44) = 3.445, p =.070, η2p=.073], which suggests a slightly larger SCL for CS+. Subsequent paired t-tests revealed that this marginal difference in SCL was observed in the early acquisition phase (t = 1.835, p =.073) but not in the late acquisition phase (t = 0.779, p =.440). No other significant main or interaction effects were found.
For HR, no significant main or interaction effect was found (all, p >.05).
For startle EMG, there was a marginal main effect of Condition [F(1,44) = 3.491, p =.068, η2p=.074], indicating a slightly larger startle response for CS+. Further examination through paired t-tests disclosed this marginal difference in the late acquisition phase (t = 1.946, p =.058) with no such difference observed in the early acquisition phase (t = 1.687, p =.099). No other significant main or interaction effects were found.
3.3 Generalization phase
In the generalized phase, the rmANOVA on risk rating revealed the significant main effects of Condition [F(2.035,95.650) = 157.811, p <.001, η2p =.771]. The rmANOVA on RT revealed the significant interaction effect [F(5,225) = 2.396, p =.038, η2p =.051] and main effects of Group [F(1,45) = 6.023, p =.018, η2p =.118] and Condition [F(5.225) = 4.770, p <.001, η2p =.096]. Further analyses on the interaction effect revealed a slower RT for C2, C3, C4, and CS− in patients compared to controls (Figure 2B), although the differences were marginal for C3 (CS+: t = −.733, p =.467; C1: t = −1.576, p =.122; C2: t = −3.149, p =.003; C3: t = −1.938, p =.059; C4: t = −2.403, p =.020, CS−: t = 2.90, p =.006), indicating patients’ difficulties in evaluating risk for both safe and ambiguous stimuli. To further examine the difference in stimuli generalization between SAD and control groups, subsequent planned comparisons between morphed stimuli (C1–4) and CS− were conducted for four pairs with the B–H correction in each group. The controls showed significantly higher ratings for C1 and C2 than CS− [for C1, mean difference (MD) = 1.22, t(22) = 8.537, p <.001; for C2, MD = 0.64, t(22) = 4.723, p <.001]. On the other hand, the patient group showed significantly higher ratings for C1–3 than CS− [for C1, MD = 1.40, t(25) = 11.915, p <.001; for C2, MD = 0.78, t(25) = 5.690, p <.001; for C3, MD = 0.21, t(25) = 3.065, p =.005], indicating that the patient group assessed the risk higher than CS− when the stimuli close to CS− contained a portion of the CS+ features (Figure 2A). While the controls showed comparable RTs in all classes of morphed stimuli compared to CS−, the patient group showed significantly slower RTs for C2 than CS− [MD = 18.31 ms, t(23) = 3.164, p =.004], indicating that the patient group took longer to assess the risk for ambiguous stimuli (Figure 2B).
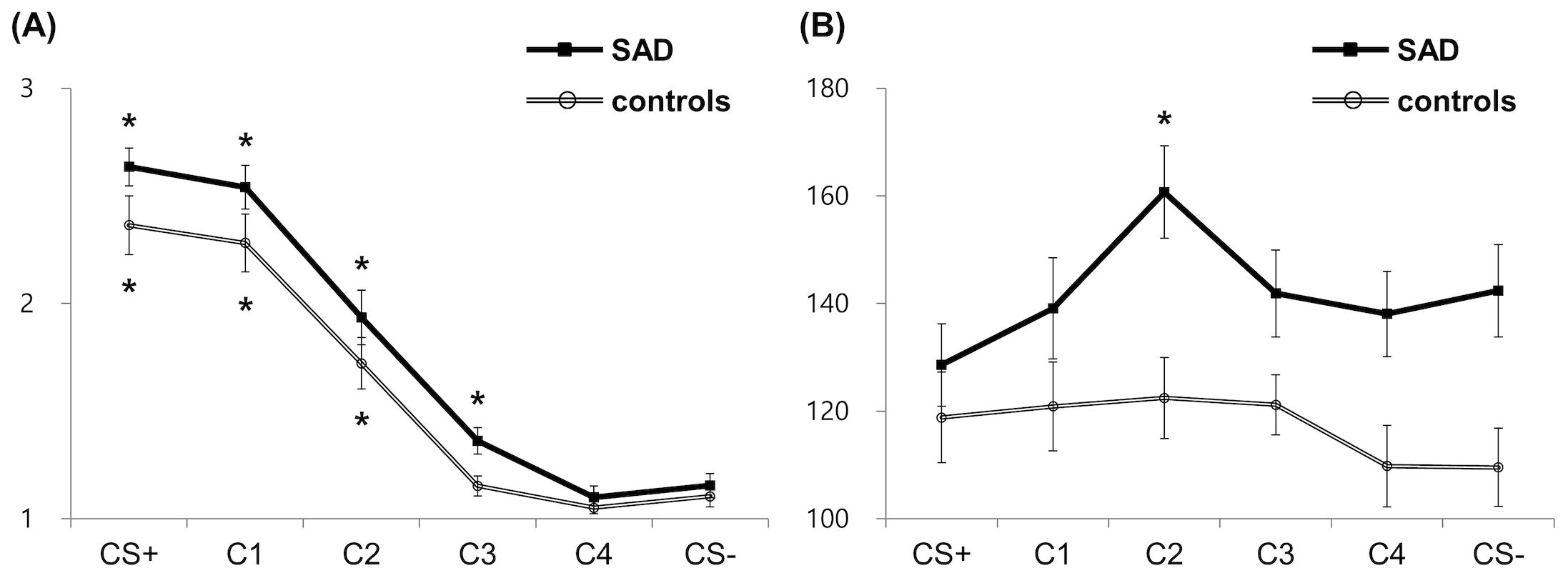
Figure 2. Behavioral results for risk rating (A) and response time (B). Asterisks indicate significant differences from the reference condition (CS−) in each group (corrected p <.05). SAD, social anxiety disorder; CS+, threat cue; CS-, safety cue; C1-4, class 1-4.
The ANOVA on SCL, HR, and EMG yielded no significant main or interaction effect (all, p >.05) (Figure 3). For the SCL, although the effect of Condition falls short of significance [F(2.815,112.598) = 2.176, p = .099, η2p =.052], there was an observable tendency of higher SCL in response to safe signals (i.e., C4 and CS−) in patients (Figure 3A). Subsequent independent t-tests on group difference showed that patients exhibited significantly higher SCL compared to controls for C4 [MD = −0.17, t(42) = −2.211, p =.033] and marginally higher SCL compared to controls for CS− [MD = −0.15, t(40) = −1.845, p =.073].
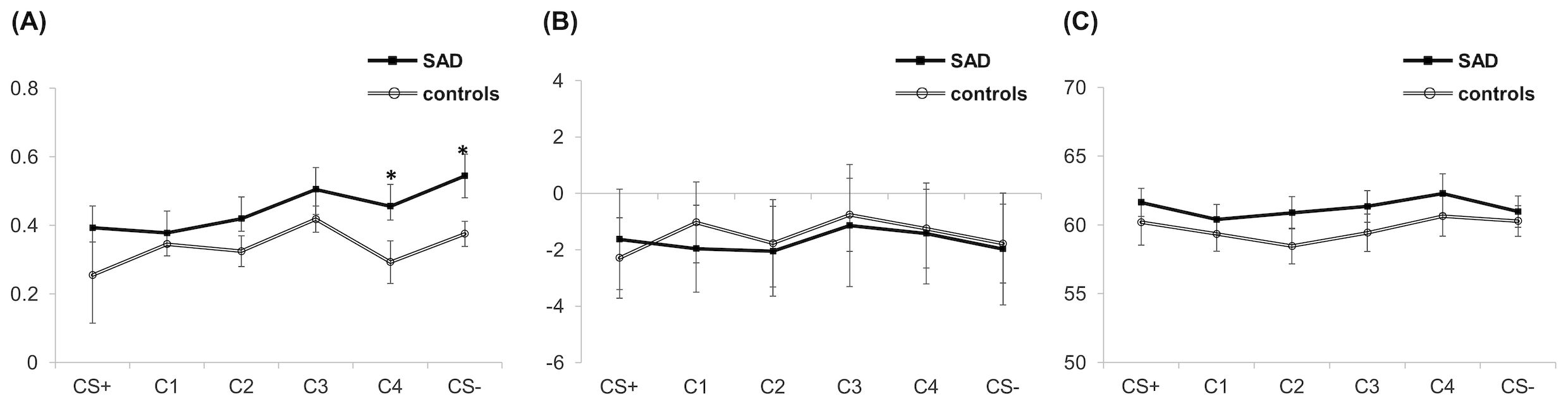
Figure 3. Physiological responses including SCL (A), HR (B), and startle EMG (C) during the generalization. Asterisks indicate significant differences between the two groups. (corrected p < .05). SAD, social anxiety disorder; CS+, threat cue; CS-, safety cue; C1-4, class 1-4; SCL: skin conductance level; HR: heart rate; EMG: electromyography.
3.4 Changes across phase
Subsequent analyses were conducted on subjective ratings of valence, arousal, and threat that were measured at the end of each phase (Figure 4). The rmANOVA on the valence rating revealed a significant main effect of Phase [F(1,47) = 4.129, p =.048, η2p =.081], participants rated the valence of CSs more negatively in the acquisition phase than in the generalization phase (Figure 4A). No other main or interaction effects were significant (all, 3p >.05). The rmANOVA on the arousal rating revealed the significant main effects of Group [F(1,47) = 7.809, p =.008, η2p =.142] and Phase [F(1,47) = 10.573, p =.002, η2p =.184] and the interaction effects of Group × Phase [F(1,47) = 5.964, p =.018, η2p =.113] and Group × Phase × Condition [F(1,47) = 4.260, p =.045, η2p =.083]. While controls showed lower arousal ratings for CSs in the generalization phase compared to the acquisition phase [CS+, t(22) = 3.869, p =.001; CS−, t(22) = 1.811 p =.084], patients did not show a decrease in the arousal rating in the generalization phase [CS+, t(25) = −.547, p =.589; CS−, t(25) = 1.247, p =.224], and the difference was particularly noticeable in CS+ (Figure 4B). This contrasting pattern shows normal habituation to CSs in controls but failed habituation in patients. The rmANOVA on the threat rating revealed the significant main effect of Group [F(1,47) = 15.431, p <.001, η2p =.247]; patients rated CSs as more threatening than controls across the acquisition and generalization phases (Figure 4C). The significant interaction effect of Phase × Condition [F(1,47) = 8.629, p =.005, η2p =.155] indicated that participants tended to rate CS+ as more threatening compared to CS− in the acquisition phase [t(48) = 1.823, p =.074], whereas ratings were comparable for CS+ and CS− in the generalization phase [t(48) =.343, p =.733].
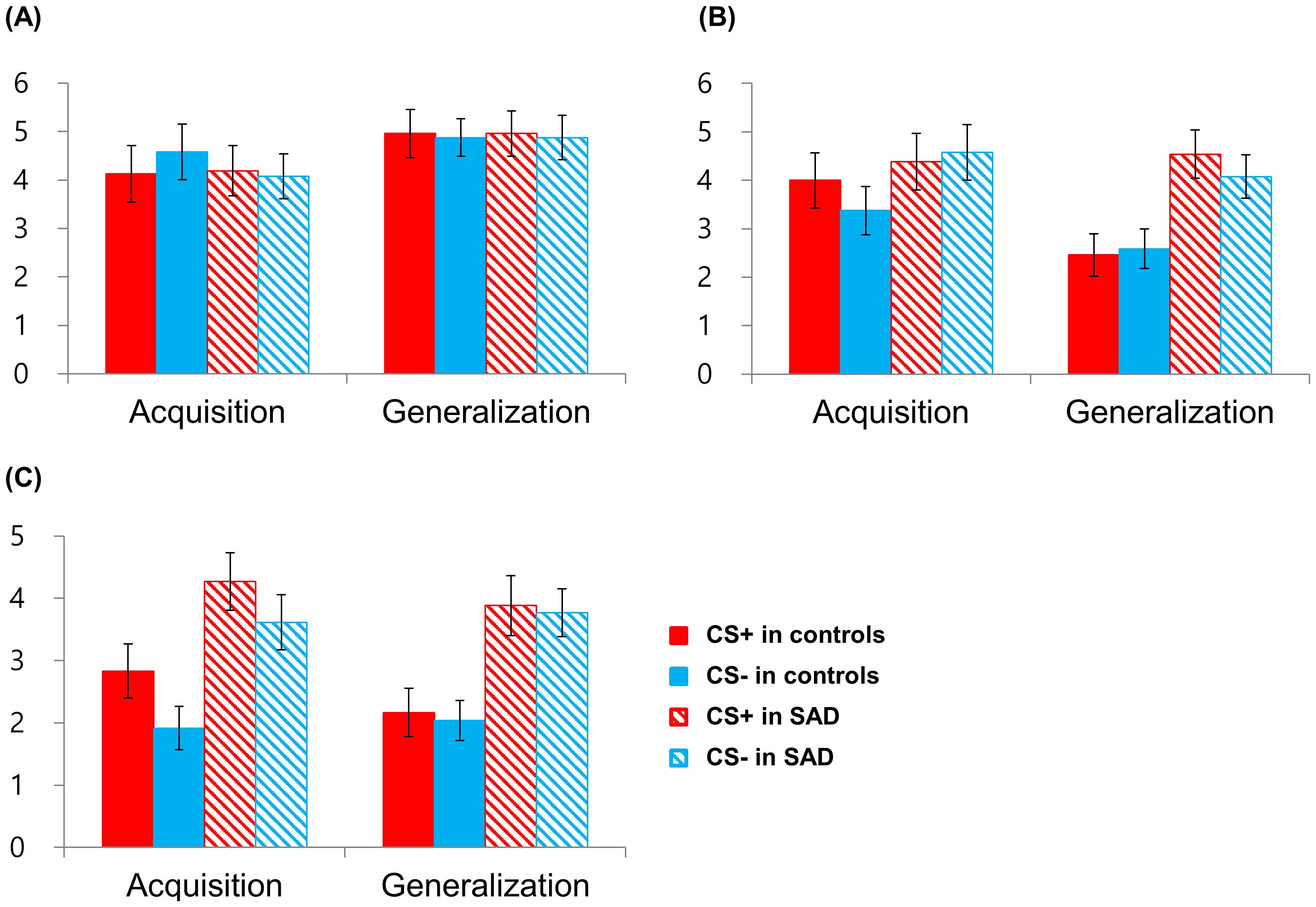
Figure 4. Retrospective subjective ratings of valence (A), arousal (B), and threat (C) for the threat (CS+) and the safety cue (CS−) after the acquisition and generalization phases. SAD, social anxiety disorder.
We further investigated whether the observed pattern of habituation would also be evident in physiological dimension. The rmANOVA on EMG revealed the significant main effect of Phase [F(1,44) = 28.502, p <.001, η2p = .393]. In both groups, startle response decreased from the acquisition (for CS+: mean, 64.49; for CS−: mean, 63.57) to the generalization phase (for CS+: mean, 60.47; for CS−: mean, 60.66) regardless of Condition. The rmANOVA on SCL and HR showed no significant main or interaction effect (all, p >.05).
3.5 Correlations
In patients during the acquisition phase, risk ratings for CS− showed a significant correlation with SIAS (r = 0.420, p =.033) and SPS (r = 0.510, p =.008), a trend-level correlation with FNE (r = 0.369, p =.064), and a non-significant correlation with LSAS (r = 0.249, p =.220). In contrast, risk rating for CS+ was not associated with any of social anxiety scores (all, p >.05). In the generalization phase, no significant correlation was found in both behavioral and physiological outcomes (all, p >.05).
After controlling for trait anxiety, the correlation between risk ratings for CS− and social anxiety symptoms weakened, showing a significant correlation with SPS (r = 0.506, p = .019) and a trend-level correlation with SIAS (r = 0.417, p =.060), with no significant correlations with LSAS and FNE (all, p >.05). Notably, significant correlations emerged in the generalization phase, including associations between risk ratings for C4 and LSAS (r = 0.447, p = .042) and SPS (r = 0.446, p = .043), along with marginal correlations for CS- with LSAS (r = 0.417, p = .060) and SPS (r = 0.418, p = .059). Risk ratings for CS+ remained unassociated with any social anxiety scores (all, p >.05). In terms of physiological measures, the SCL for C4 significantly correlated with FNE (r = 0.495, p = .026), with no other significant correlations found (all, p >.05).
4 Discussion
Using an ecologically enhanced paradigm, we investigated whether patients with SAD differ from healthy controls in the processes of conditioned fear acquisition and generalization. During both the early and late acquisition phases, a higher risk rate for CS+ indicated that fear conditioning was successful irrespective of the group. While there was a trend for conditioning in a similar direction for SCL and startle EMG, it did not reach statistical significance. We found no group differences in risk rating, RT, physiological, or startle responses, except that patients reported higher subjective arousal levels and threat ratings than healthy controls. By contrast, group differences were apparent in the generalization phase. While healthy controls generalized their conditioned fear response (i.e., risk rating) from the CS+ to two generalization stimuli (C1 and C2), patients’ fear response was transferred further to C3, which is closer to CS− than CS+. In addition, for ambiguous and safe stimuli, patients spent a much longer time evaluating the risk and exhibited higher SCL compared to controls. Interestingly, patients with a high level of fear of negative evaluation showed larger SCL in response to C4. In addition, within-group differences were also observed in the subjective arousal levels between phases; while controls experienced a decrease in arousal levels from the acquisition to the generalization phase, patients consistently demonstrated heightened arousal throughout the study. Taken together, although there were no typical and robust patterns of overgeneralization of physiological and startle responses in patients in the present study, our results imply that SAD patients have lower thresholds for provoking a conditioned fear response and exhibit less habituation.
In line with previous studies (17, 41), we did not find evidence for enhanced conditionability in patients during acquisition. Our behavioral results, including RT and risk rating, imply heightened fear generalization in SAD patients. The observed patient-group differences in RT, i.e., slower RT for safe (but not for threat) stimuli in patients, are consistent with previous findings that anxiety patients and controls show different fear responses to CS−, but not to CS+ [for a review, see (9)]. Greater fear responses to CS− have been interpreted as reflecting fear overgeneralization or impaired fear inhibition in patients (9). Notably, within patients, higher risk ratings for safe and ambiguous stimuli were positively correlated with social anxiety symptoms after controlling for trait anxiety. Specifically, higher ratings for C4 positively correlated with the LSAS and SPS, while ratings for CS− showed marginal positive correlations. In addition, patients with a higher level of fear of negative evaluation exhibited significantly larger SCL for C4. Inter-individual differences including trait anxiety have been intensively investigated in relation to fear learning (42), and trait anxiety has been reported to influence fear generalization in healthy controls (43). Our results indicate that even after controlling for these inter-individual differences, there were still significant associations between the level of social anxiety and overgeneralization for social threat. Together with risk rating results, our RT data suggest that patients have difficulties in evaluating the risks of safe and ambiguous signals in an aversive context, implying an associative learning deficit in patients with SAD. In addition, within-group differences in subjective reporting across phases also suggest maladaptive non-associative learning in SAD. While controls showed a decrease in subjective arousal from the acquisition to the generalization phase, patients’ arousal state was consistently higher throughout the two phases. According to the theoretical framework, non-associative learning includes persistent or increasing fear reactivity to novel stimuli, which derives from pathological failure to habituate or sensitization (44). To summarize, SAD patients experience difficulties distinguishing between threat and safety cues and adapting to novel stimuli. We assume that failure to habituate makes patients persistently anxious during an aversive context, making fear extinction more difficult.
While the behavioral dimension (i.e., risk rating and RT) showed a significant group difference in fear generalization, physiological responses were comparable between the two groups. The lack of physiological discrimination between CS+ and CS− in the generalization phase may be explained separately in controls and SAD. For the controls, we observed a general decrease in both subjective arousal and fear-potentiated startle responses across phases, suggesting a habituation effect despite occasional presentations of the US in the generalization phase. This overall reduction in physiological reactivity likely contributed to the diminished discrimination between CS+ and CS−. In contrast, the patient group maintained high levels of subjective arousal from the acquisition to the generalization phase, indicating a lack of habituation. Notably, patients exhibited elevated SCL to CS− compared to controls in the generalization phase. This heightened response to the safety cue might have obscured the physiological discrimination between CS+ and CS−. The dissociation between behavioral and physiological responses in fear conditioning has been reported in a previous lesion study (45). Bechara et al. demonstrated that declarative associative learning, represented by risk rating, is impaired in patients with bilateral hippocampal damage, whereas implicit learning, as reflected by autonomic nervous system responses, is compromised in patients with bilateral amygdala damage (45). This suggests the possibility that potentially distinct neural mechanisms may be involved in implicit and explicit learning. Similarly, dissociation between self-reported arousal/anxiety and the physiological response to emotional stimuli has often been reported in studies of clinical and subclinical samples of anxiety (46–50). For example, socially anxious individuals reported feeling more anxious during public speaking compared to controls despite equal patterns of physiological arousal, which suggests that increased awareness of or sensitivity to arousal, rather than the level of physiological arousal per se, may be a more important factor in characterizing SAD. One possible explanation for similar phenomena in this study is that socially relevant US was aversive enough to elicit fear conditioning as defined by cognitive awareness and arousal but not sufficient to generate a physiological response, as is often seen in human fear conditioning paradigms. It has been suggested that studies using a startle probe should use a more highly aversive US (19, 51, 52).
Alternatively, some have attempted to attribute this discrepancy to the idiosyncrasies of the socially anxious sample. When they encounter a social threat, they presumably show avoidance and submissiveness rather than a fight-or-flight response and thus exhibit less autonomic activation (48). In addition, inward-directed attention (which makes an external startle probe less effective) and a high comorbidity rate with major depression in social anxiety are also considered major causes of the lack of physiological activation (53, 54). Previous studies on fear conditioning in social anxiety have revealed nonsignificant or weak physiological evidence of fear (over)generalization (17, 41) leading to the conclusion that social anxiety may not be characterized by strong fear (over)generalization. On the other hand, a recent meta-analysis (14) and the Hierarchical Taxonomy of Psychopathology (HiTOP) model (55) advocate for a transdiagnostic approach in understanding fear generalization. The meta-analysis revealed heightened fear generalization across anxiety-related disorders, emphasizing its transdiagnostic nature. Similarly, the HiTOP model proposes a dimensional framework that transcends traditional taxonomies, providing a cross-cutting perspective on psychopathological syndromes. Both studies highlight the importance of adopting a unified view, moving beyond disorder-specific boundaries to better comprehend fear-related phenomena like generalization across various anxiety-related disorders. In this context, intolerance of uncertainty (IU), a transdiagnostic construct encompassing both personality and cognitive biases that assesses the inclination to perceive uncertainty as aversive, has emerged as a crucial factor in classical threat conditioning mechanisms. It has been found that a high level of self-reported IU, over trait anxiety and worry, was related to greater generalization (42, 56, 57). As we have not measured subjective IU or manipulated outcome uncertainty as in previous studies (58), further discussion on these aspects is not feasible within the scope of our research. However, it appears crucial to incorporate uncertainty-related factors in future fear learning studies, considering the potential significance of such elements.
The primary strength of this study is that we employed ecologically enhanced social-anxiety-related stimuli, such as angry faces and contemptuous comments. Given the prior neuroimaging studies reporting that directly facing angry and contemptuous facial expressions provokes strong neural responses in participants with SAD (59, 60), our study design seemed suitable for investigating fear acquisition and generalization in SAD patients who are sensitive to others’ evaluations. However, it is important to note that the use of disorder-relevant US generally offers both methodological advantages and challenges (61). On the one hand, this approach enhances ecological validity by providing conditions more closely aligned with the real-world experiences of patients with SAD. Such an experimental design may facilitate the capture of more clinically relevant behavioral and physiological patterns, potentially yielding deeper insights into the mechanisms of fear acquisition and generalization in patients. On the other hand, although angry faces or verbal rejection are a social threat signal eliciting fear responses in healthy adults (20, 62), the inherent aversiveness of the US may potentially vary between patients with SAD and healthy controls (59, 60). This difference makes it challenging to discern whether group differences in fear responses stem from variations in fear acquisition and generalization processes or from disparities in the perceived aversiveness of the stimuli themselves. Although these two factors cannot be disentangled in the present study, future studies could use both disorder-relevant and irrelevant USs to evaluate their differential effects in clinical populations. Furthermore, future research could benefit from the inclusion of diverse comparison groups. In this study, the use of psychiatrically healthy adults as a control group might not have been optimal for fully capturing the effects of employing disorder-specific stimuli. A more relevant control group might consist of individuals in recovery from SAD or subclinical populations that are likely to have a similar baseline sensitivity to SAD-specific stimuli. This shared characteristic could provide a more sophisticated understanding of the differences in fear acquisition and generalization specifically attributable to current SAD symptoms, rather than to differences in the perceived relevance or salience of the stimuli.
The present study had several limitations. First, since our power calculation was based on a previously reported effect size of fear acquisition, the sample size of 25 per group might not be sufficient to robustly capture the interaction between the stimulus type and group during the generalization phase. Second, the self-reported risk rating that we used to evaluate participants’ perceived likelihood of a person on the screen making contemptuous comments consisted of a simple 1–3 Likert scale, which was too simple to reveal the variability of the fear response. The use of a three-point Likert scale can limit the ability to analyze the results of a measurement. As it only categorizes three possible responses to the risk rating, it is difficult to get information about the intensity or degree of the response. When a subject selects “2,” the interpretation can be ambiguous because it can have multiple meanings. Therefore, it may not accurately reflect the risk expectancy of the subjects, which limits the interpretation of the results of this study. Third, it should be noted that the present study recruited only an Asian population, which has a low prevalence of anxiety disorders presumably due to cultural characteristics such as a high level of emotional control (63). For example, a previous study investigating the effect of ethnicity on startle response showed that Asians demonstrate less emotional expression (64), smaller fear-potentiated startle response in the baseline period (65), and reduced sensitivity to unpredictable threats (66). This emphasizes that biomarkers of emotional response should be interpreted with caution. Consequently, the consideration of challenges and biases in recording electrodermal activity in different ethnicities underscores the critical need for a more inclusive approach in psychophysiological research (67). In this vein, the exclusive recruitment of Asian participants can be seen as a strength in the current study. Many research studies predominantly feature participants from Western populations, leading to a gap in cross-cultural representation. By focusing on Asian participants, our research contributes to a more comprehensive understanding of fear generalization across diverse cultural contexts. Finally, we did not measure participants’ subjective rating for startle stimuli. Due to the possibility that the startle probe could make participants feel CS aversive as well, it is recommended that studies using startle probes include a larger number of trials or a rating for startle probes (68).
5 Conclusion
This study examined whether an ecologically enhanced fear conditioning paradigm using more disorder-specific stimuli can elicit a distinct pattern of overgeneralization in patients with SAD. Using social-anxiety-related stimuli such as an angry face and contemptuous comments, we found that SAD patients assessed the risk higher for ambiguous stimuli, spent more time than healthy controls when evaluating safe and ambiguous stimuli, and showed consistently high levels of subjective arousal. Although we did not find a typical and robust pattern of overgeneralization of physiological and startle responses in patients, our results imply that SAD patients have lower thresholds for provoking a conditioned fear response, making it more difficult for them to discriminate between threat and safety cues.
Data availability statement
The raw data supporting the conclusions of this article will be made available by the authors, without undue reservation.
Ethics statement
The studies involving humans were approved by Institutional Review Board of Seoul National University Hospital. The studies were conducted in accordance with the local legislation and institutional requirements. The participants provided their written informed consent to participate in this study.
Author contributions
YL: Data curation, Investigation, Methodology, Visualization, Writing – original draft. DL: Formal analysis, Investigation, Methodology, Visualization, Writing – original draft, Writing – review & editing. HK: Investigation, Methodology, Writing – review & editing. MK: Investigation, Writing – original draft. HJ: Investigation, Writing – original draft. DK: Formal analysis, Investigation, Writing – original draft. EG-S: Formal analysis, Methodology, Writing – original draft. S-HC: Conceptualization, Formal analysis, Funding acquisition, Project administration, Supervision, Writing – original draft, Writing – review & editing.
Funding
The author(s) declare financial support was received for the research, authorship, and/or publication of this article. This work was supported by the Ministry of Science and ICT (2019R1A2C1086581), the SNUH Research Fund (04-2018-0480), and Korea Institute of Science and Technology (KIST) Institutional Program (2E32190).
Acknowledgments
We would like to thank Professor Juyoen Hur at Yonsei University for her insightful comments on this paper.
Conflict of interest
The authors declare that the research was conducted in the absence of any commercial or financial relationships that could be construed as a potential conflict of interest.
Publisher’s note
All claims expressed in this article are solely those of the authors and do not necessarily represent those of their affiliated organizations, or those of the publisher, the editors and the reviewers. Any product that may be evaluated in this article, or claim that may be made by its manufacturer, is not guaranteed or endorsed by the publisher.
Supplementary material
The Supplementary Material for this article can be found online at: https://www.frontiersin.org/articles/10.3389/fpsyt.2024.1415135/full#supplementary-material
References
1. Kessler RC, Petukhova M, Sampson NA, Zaslavsky AM, Wittchen HU. Twelve-month and lifetime prevalence and lifetime morbid risk of anxiety and mood disorders in the United States. Int J Methods Psychiatr Res. (2012) 21:169–84. doi: 10.1002/mpr.1359
2. Shields M. Social anxiety disorder: Much more than shyness. Can Soc Trends. (2005) 11:22–8. doi: 10.1117/12.601817
3. Australian Bureau of Statistics. National Survey of Mental Health and Wellbeing: Summary of Results. ABS (2007). Available at: https://www.abs.gov.au/statistics/health/mental-health/national-study-mental-health-and-wellbeing/2007. [Accessed August 9, 2024].
4. American Psychiatric Association, DSM-5 Task Force. Diagnostic and statistical manual of mental disorders: DSM-5™ (5th ed.). Washington, DC: American Psychiatric Publishing, Inc. (2013).
5. Bolton JM, Cox BJ, Afifi TO, Enns MW, Bienvenu OJ, Sareen J. Anxiety disorders and risk for suicide attempts: findings from the Baltimore Epidemiologic Catchment area follow-up study. Depression anxiety. (2008) 25:477–81. doi: 10.1002/(ISSN)1520-6394
6. Cougle JR, Keough ME, Riccardi CJ, Sachs-Ericsson N. Anxiety disorders and suicidality in the National Comorbidity Survey-Replication. J Psychiatr Res. (2009) 43:825–9. doi: 10.1016/j.jpsychires.2008.12.004
7. Sareen J, Cox BJ, Afifi TO, De Graaf R, Asmundson GJ, Ten Have M, et al. Anxiety disorders and risk for suicidal ideation and suicide attempts: a population-based longitudinal study of adults. Arch Gen Psychiatry. (2005) 62:1249–57. doi: 10.1001/archpsyc.62.11.1249
8. Stuhldreher N, Leibing E, Leichsenring F, Beutel ME, Herpertz S, Hoyer J, et al. The costs of social anxiety disorder: the role of symptom severity and comorbidities. J Affect Disord. (2014) 165:87–94. doi: 10.1016/j.jad.2014.04.039
9. Duits P, Cath DC, Lissek S, Hox JJ, Hamm AO, Engelhard IM, et al. Updated meta-analysis of classical fear conditioning in the anxiety disorders. Depression anxiety. (2015) 32:239–53. doi: 10.1002/da.2015.32.issue-4
10. Lissek S, Powers AS, McClure EB, Phelps EA, Woldehawariat G, Grillon C, et al. Classical fear conditioning in the anxiety disorders: a meta-analysis. Behav Res Ther. (2005) 43:1391–424. doi: 10.1016/j.brat.2004.10.007
11. Mineka S, Zinbarg R. A contemporary learning theory perspective on the etiology of anxiety disorders: it's not what you thought it was. Am Psychol. (2006) 61:10. doi: 10.1037/0003-066X.61.1.10
12. Pittig A, Treanor M, LeBeau RT, Craske MG. The role of associative fear and avoidance learning in anxiety disorders: Gaps and directions for future research. Neurosci Biobehav Rev. (2018) 88:117–40. doi: 10.1016/j.neubiorev.2018.03.015
13. Scheveneels S, Boddez Y, Hermans D. Predicting clinical outcomes via human fear conditioning: A narrative review. Behav Res Ther. (2021) 142:103870. doi: 10.1016/j.brat.2021.103870
14. Cooper SE, van Dis EA, Hagenaars MA, Krypotos A-M, Nemeroff CB, Lissek S, et al. A meta-analysis of conditioned fear generalization in anxiety-related disorders. Neuropsychopharmacology. (2022) 47:1652–61. doi: 10.1038/s41386-022-01332-2
15. Fraunfelter L, Gerdes A, Alpers GW. Fear one, fear them all: A systematic review and meta-analysis of fear generalization in pathological anxiety. Neurosci Biobehav Rev. (2022) 139:104707. doi: 10.1016/j.neubiorev.2022.104707
16. Ahrens LM, Mühlberger A, Pauli P, Wieser MJ. Impaired visuocortical discrimination learning of socially conditioned stimuli in social anxiety. Soc Cogn Affect Neurosci. (2015) 10:929–37. doi: 10.1093/scan/nsu140
17. Ahrens LM, Pauli P, Reif A, Mühlberger A, Langs G, Aalderink T, et al. Fear conditioning and stimulus generalization in patients with social anxiety disorder. J Anxiety Disord. (2016) 44:36–46. doi: 10.1016/j.janxdis.2016.10.003
18. Hermann C, Ziegler S, Birbaumer N, Flor H. Psychophysiological and subjective indicators of aversive pavlovian conditioning in generalized social phobia. Biol Psychiatry. (2002) 52:328–37. doi: 10.1016/S0006-3223(02)01385-9
19. Lissek S, Levenson J, Biggs AL, Johnson LL, Ameli R, Pine DS, et al. Elevated fear conditioning to socially relevant unconditioned stimuli in social anxiety disorder. Am J Psychiatry. (2008) 165:124–32. doi: 10.1176/appi.ajp.2007.06091513
20. Reichenberger J, Pfaller M, Forster D, Gerczuk J, Shiban Y, Mühlberger A. Men scare me more: gender differences in social fear conditioning in virtual reality. Front Psychol. (2019) 10:1617. doi: 10.3389/fpsyg.2019.01617
21. Veit R, Flor H, Erb M, Hermann C, Lotze M, Grodd W, et al. Brain circuits involved in emotional learning in antisocial behavior and social phobia in humans. Neurosci letters. (2002) 328:233–6. doi: 10.1016/S0304-3940(02)00519-0
22. Sheehan DV, Lecrubier Y, Sheehan KH, Amorim P, Janavs J, Weiller E, et al. The Mini-International Neuropsychiatric Interview (MINI): the development and validation of a structured diagnostic psychiatric interview for DSM-IV and ICD-10. J Clin Psychiatry. (1998) 59:22–33.
24. Mattick RP, Clarke JC. Development and validation of measures of social phobia scrutiny fear and social interaction anxiety. Behav Res Ther. (1998) 36:455–70. doi: 10.1016/S0005-7967(97)10031-6
25. Leary MR. A brief version of the Fear of Negative Evaluation Scale. Pers Soc Psychol bulletin. (1983) 9:371–5. doi: 10.1177/0146167283093007
26. Spielberger C, Gorsuch R, Lushene R, Vagg P, Jacobs G. Manual for the State-Trait Anxiety Inventory. Palo Alto CA, editor. Columbia, MO, USA: Consulting Psychologists Press, Inc (1983).
27. Beck AT, Epstein N, Brown G, Steer RA. An inventory for measuring clinical anxiety: psychometric properties. J consulting Clin Psychol. (1988) 56:893. doi: 10.1037/0022-006X.56.6.893
28. Beck AT, Ward CH, Mendelson M, Mock J, Erbaugh J. An inventory for measuring depression. Arch Gen Psychiatry. (1961) 4:561–71. doi: 10.1001/archpsyc.1961.01710120031004
29. Hamilton M. The assessment of anxiety states by rating. Br J Med Psychol. (1959) 32:50–5. doi: 10.1111/j.2044-8341.1959.tb00467.x
30. Montgomery SA, Åsberg M. A new depression scale designed to be sensitive to change. Br J Psychiatry. (1979) 134:382–9. doi: 10.1192/bjp.134.4.382
31. Sheehan DV, Harnett-Sheehan K, Raj BA. The measurement of disability. Int Clin Psychopharmacol. (1996) 3:89–95. doi: 10.1097/00004850-199606003-00015
32. Park JY, Oh JM, Kim SY, Lee M, Lee C, Kim BR, et al. Korean facial expressions of emotion (KOFEE). Seoul, Korea: Section of Affect & Neuroscience, Institute of Behavioral Science in Medicine, Yonsei University College of Medicine (2011).
33. Wieser MJ, Pauli P, Alpers GW, Mühlberger A. Is eye to eye contact really threatening and avoided in social anxiety?—An eye-tracking and psychophysiology study. J Anxiety Disord. (2009) 23:93–103. doi: 10.1016/j.janxdis.2008.04.004
34. Vrana SR. Emotional modulation of skin conductance and eyeblink responses to a startle probe. Psychophysiology. (1995) 32:351–7. doi: 10.1111/j.1469-8986.1995.tb01217.x
35. Glotzbach-Schoon E, Andreatta M, Reif A, Ewald H, Tröger C, Baumann C, et al. Contextual fear conditioning in virtual reality is affected by 5HTT LPR and NPSR1 polymorphisms: effects on fear-potentiated startle. Front Behav Neurosci. (2013) 7:31. doi: 10.3389/fnbeh.2013.00031
36. Creaser JL, Storr J, Karl A. Brain responses to a self-compassion induction in trauma survivors with and without post-traumatic stress disorder. Front Psychol. (2022) 13:765602. doi: 10.3389/fpsyg.2022.765602
37. Lykken D. Range correction applied to heart rate and to GSR data. Psychophysiology. (1972) 9:373–9. doi: 10.1111/j.1469-8986.1972.tb03222.x
38. Powell PS, Travers BG, Klinger LG, Klinger MR. Difficulties with multi-sensory fear conditioning in individuals with autism spectrum disorder. Res Autism Spectr Disord. (2016) 25:137–46. doi: 10.1016/j.rasd.2016.02.008
39. Suarez-Jimenez B, Bisby JA, Horner AJ, King JA, Pine DS, Burgess N. Linked networks for learning and expressing location-specific threat. Proc Natl Acad Sci. (2018) 115:E1032–E40. doi: 10.1073/pnas.1714691115
40. Morato C, Guerra P, Bublatzky F. A partner's smile is not per se a safety signal: Psychophysiological response patterns to instructed threat and safety. Psychophysiology. (2023) 60:e14273. doi: 10.1111/psyp.14273
41. Tinoco-González D, Fullana MA, Torrents-Rodas D, Bonillo A, Vervliet B, Blasco MJ, et al. Conditioned fear acquisition and generalization in generalized anxiety disorder. Behav Ther. (2015) 46:627–39. doi: 10.1016/j.beth.2014.12.004
42. Lonsdorf TB, Merz CJ. More than just noise: Inter-individual differences in fear acquisition, extinction and return of fear in humans-Biological, experiential, temperamental factors, and methodological pitfalls. Neurosci Biobehav Rev. (2017) 80:703–28. doi: 10.1016/j.neubiorev.2017.07.007
43. Sep MS, Steenmeijer A, Kennis M. The relation between anxious personality traits and fear generalization in healthy subjects: A systematic review and meta-analysis. Neurosci Biobehav Rev. (2019) 107:320–8. doi: 10.1016/j.neubiorev.2019.09.029
44. Lissek S, van Meurs B. Learning models of PTSD: Theoretical accounts and psychobiological evidence. Int J psychophysiology. (2015) 98:594–605. doi: 10.1016/j.ijpsycho.2014.11.006
45. Bechara A, Tranel D, Damasio H, Adolphs R, Rockland C, Damasio AR. Double dissociation of conditioning and declarative knowledge relative to the amygdala and hippocampus in humans. Science. (1995) 269:1115–8. doi: 10.1126/science.7652558
46. Beidel DC, Turner SM, Dancu CV. Physiological, cognitive and behavioral aspects of social anxiety. Behav Res Ther. (1985) 23:109–17. doi: 10.1016/0005-7967(85)90019-1
47. Eckman PS, Shean GD. Habituation of cognitive and physiological arousal and social anxiety. Behav Res Ther. (1997) 35:1113–21. doi: 10.1016/S0005-7967(97)80005-8
48. Mauss I, Wilhelm F, Gross J. Is there less to social anxiety than meets the eye? Emotion experience, expression, and bodily responding. Cogn emotion. (2004) 18:631–42. doi: 10.1080/02699930341000112
49. Raskin M. Decreased skin conductance response habituation in chronically anxious patients. Biol Psychol. (1975) 2:309–19. doi: 10.1016/0301-0511(75)90039-3
50. Rosebrock LE, Hoxha D, Norris C, Cacioppo JT, Gollan JK. Skin conductance and subjective arousal in anxiety, depression, and comorbidity. J Psychophysiol. (2016) 31:145–57. doi: 10.1027/0269-8803/a000176
51. Hamm AO, Vaitl D. Affective learning: Awareness and aversion. Psychophysiology. (1996) 33:698–710. doi: 10.1111/j.1469-8986.1996.tb02366.x
52. Lipp OV, Sheridan J, Siddle DA. Human blink startle during aversive and nonaversive Pavlovian conditioning. J Exp Psychology: Anim Behav Processes. (1994) 20:380. doi: 10.1037/0097-7403.20.4.380
53. McTeague LM, Lang PJ, Laplante M-C, Cuthbert BN, Strauss CC, Bradley MM. Fearful imagery in social phobia: generalization, comorbidity, and physiological reactivity. Biol Psychiatry. (2009) 65:374–82. doi: 10.1016/j.biopsych.2008.09.023
54. Panayiotou G, Vrana SR. Effect of self-focused attention on the startle reflex, heart rate, and memory performance among socially anxious and nonanxious individuals. Psychophysiology. (1998) 35:328–36. doi: 10.1017/S0048577298960875
55. Kotov R, Krueger RF, Watson D, Achenbach TM, Althoff RR, Bagby RM, et al. The Hierarchical Taxonomy of Psychopathology (HiTOP): A dimensional alternative to traditional nosologies. J Abnormal Psychol. (2017) 126:454. doi: 10.1037/abn0000258
56. Morriss J, Macdonald B, Van Reekum CM. What is going on around here? Intolerance of uncertainty predicts threat generalization. PLoS One. (2016) 11:e0154494. doi: 10.1371/journal.pone.0154494
57. Morriss J, Zuj DV, Mertens G. The role of intolerance of uncertainty in classical threat conditioning: Recent developments and directions for future research. Int J Psychophysiology. (2021) 166:116–26. doi: 10.1016/j.ijpsycho.2021.05.011
58. Zika O, Wiech K, Reinecke A, Browning M, Schuck NW. Trait anxiety is associated with hidden state inference during aversive reversal learning. Nat Commun. (2023) 14:4203. doi: 10.1038/s41467-023-39825-3
59. Phan KL, Fitzgerald DA, Nathan PJ, Tancer ME. Association between amygdala hyperactivity to harsh faces and severity of social anxiety in generalized social phobia. Biol Psychiatry. (2006) 59:424–9. doi: 10.1016/j.biopsych.2005.08.012
60. Stein MB, Goldin PR, Sareen J, Zorrilla LTE, Brown GG. Increased amygdala activation to angry and contemptuous faces in generalized social phobia. Arch Gen Psychiatry. (2002) 59:1027–34. doi: 10.1001/archpsyc.59.11.1027
61. Lonsdorf TB, Menz MM, Andreatta M, Fullana MA, Golkar A, Haaker J, et al. Don’t fear ‘fear conditioning’: Methodological considerations for the design and analysis of studies on human fear acquisition, extinction, and return of fear. Neurosci Biobehav Rev. (2017) 77:247–85. doi: 10.1016/j.neubiorev.2017.02.026
62. Grosbras M-H, Paus T. Brain networks involved in viewing angry hands or faces. Cereb cortex. (2006) 16:1087–96. doi: 10.1093/cercor/bhj050
63. Tsai JL, Levenson RW. Cultural influences on emotional responding: Chinese American and European American dating couples during interpersonal conflict. J Cross-Cultural Psychol. (1997) 28:600–25. doi: 10.1177/0022022197285006
64. Soto JA, Levenson RW, Ebling R. Cultures of moderation and expression: emotional experience, behavior, and physiology in Chinese Americans and Mexican Americans. Emotion. (2005) 5:154. doi: 10.1037/1528-3542.5.2.154
65. Swerdlow NR, Talledo JA, Braff DL. Startle modulation in Caucasian-Americans and Asian-Americans: a prelude to genetic/endophenotypic studies across the ‘Pacific Rim’. Psychiatr Genet. (2005) 15:61–5. doi: 10.1097/00041444-200503000-00010
66. Nelson BD, Bishop JR, Sarapas C, Kittles RA, Shankman SA. Asians demonstrate reduced sensitivity to unpredictable threat: a preliminary startle investigation using genetic ancestry in a multiethnic sample. Emotion. (2014) 14:615. doi: 10.1037/a0035776
67. Bradford DE, DeFalco A, Perkins ER, Carbajal I, Kwasa J, Goodman FR, et al. Whose signals are being amplified? Toward a more equitable clinical psychophysiology. Clin psychol Sci. (2022) 12:237–52. doi: 10.31234/osf.io/c2naf
Keywords: social anxiety disorder, fear conditioning, overgeneralization, threat appraisal, arousal
Citation: Lee YI, Lee D, Kim H, Kim MJ, Jeong H, Kim D, Glotzbach-Schoon E and Choi S-H (2024) Overgeneralization of conditioned fear in patients with social anxiety disorder. Front. Psychiatry 15:1415135. doi: 10.3389/fpsyt.2024.1415135
Received: 10 April 2024; Accepted: 29 July 2024;
Published: 23 August 2024.
Edited by:
Adam Gonzalez, Stony Brook University, United StatesReviewed by:
Jayne Morriss, University of Southampton, United KingdomSeth Davin Norrholm, Wayne State University, United States
Copyright © 2024 Lee, Lee, Kim, Kim, Jeong, Kim, Glotzbach-Schoon and Choi. This is an open-access article distributed under the terms of the Creative Commons Attribution License (CC BY). The use, distribution or reproduction in other forums is permitted, provided the original author(s) and the copyright owner(s) are credited and that the original publication in this journal is cited, in accordance with accepted academic practice. No use, distribution or reproduction is permitted which does not comply with these terms.
*Correspondence: Soo-Hee Choi, c29vaGNob2lAc251LmFjLmty
†Present address: Evelyn Glotzbach-Schoon, Department of Clinical Ethics, University Hospital Erlangen, Erlangen, Germany
‡These authors share first authorship