- 1Department of Neuropsychiatry, Graduate School of Medical Sciences, Kyushu University, Fukuoka, Japan
- 2Department of Neuropsychiatry, Kyushu University Hospital, Fukuoka, Japan
- 3Graduate School of Human Environment Studies, Kyushu University, Fukuoka, Japan
- 4Department of Clinical Radiology, Graduate School of Medical Sciences, Kyushu University, Fukuoka, Japan
Background: Hoarding disorder (HD) is characterized by cognitive control impairments and abnormal brain activity in the insula and anterior cingulate cortex (ACC) during disposal of personal items or certain executive function tasks. However, whether there are any changes in resting-state functional connectivity of the insula and ACC remains unclear.
Methods: A total of 55 subjects, including 24 patients with HD and 31 healthy controls (HCs), participated in the study. We acquired resting-state functional magnetic resonance imaging data and examined group differences in functional connectivity from the insula and ACC in whole-brain voxels.
Results: In patients with HD, functional connectivity was significantly lower between the right insula and right inferior frontal gyrus (IFG) and left superior temporal gyrus (STG) compared to HCs. There was no correlation between these connectivities and HD symptoms.
Conclusions: Although the clinical implication is uncertain, our results suggest that patients with HD have resting-state functional alterations between the insula and IFG and STG, corresponding with the results of previous fMRI studies. These findings provide new insight into the neurobiological basis of HD.
1 Introduction
Hoarding disorder (HD) is a mental disorder characterized by difficulty disposing of one’s own possessions, regardless of their actual value. HD was previously considered a subtype of obsessive-compulsive disorder (OCD) but is now considered a distinct disorder in the Diagnostic and Statistical Manual of Mental Disorders fifth edition (DSM-5) (1). According to a meta-analysis of epidemiological studies, the prevalence of HD is estimated at approximately 2.5% (2), higher than OCD at 1–2% (3). However, many individuals with HD do not receive help because of the lack of treatment methods and medical interventions as well as their poor insight into their hoarding behavior and its impact on their living condition (4). Moreover, the neurobiological features of HD remain unclear.
Previous studies have shown that patients with HD could have impairments in some domains of cognitive control function, including response inhibition, working memory, attention, memory, categorization (5–8), emotional dysregulation such as intolerance of uncertainty (9, 10), and biased decision-making patterns such as inefficient choices to maximize value (11, 12). Task functional magnetic resonance imaging (fMRI) studies using a go/no-go task, stop-signal task, or switch-signal task have shown abnormal brain activity in a wide range of brain regions, including the orbitofrontal cortex, prefrontal cortex, insula, anterior cingulate cortex (ACC), precentral gyrus, fusiform cortex, visual cortex, striatum, and cerebellum, compared to healthy controls or patients with OCD, although the results vary among studies (13–15). Compared to patients with OCD and healthy individuals, patients with HD showed greater brain activity of the right insula when making decisions about personal possessions and lower activity in the ACC and left insula when making decisions about another person’s possessions (16). Similar results have been reported previously (17). Stevens et al. evaluated brain activity in patients with HD and controls during acquisition and discarding of objects, as hoarding symptoms, and a semantic processing task as a nonrelated executive function (17). They showed that there was greater brain activity in the insula, cingulate gyrus, and inferior frontal gyrus (IFG) during the acquisition and discarding tasks than during the semantic processing task in the HD group compared with the non-HD group. Recent fMRI studies examining the effect of cognitive behavioral therapy in patients with HD have shown abnormal brain activity in several regions, including the insula and ACC, during simulated decisions on whether to keep or discard objects (18). These studies indicate that HD is associated with neurophysiological abnormalities and abnormal activation of the insula and ACC during both executive control and decision-making tasks. However, it is unclear whether the altered activity of the insula and ACC exists during the resting state, and not only during cognitive tasks.
Resting-state functional MRI (rsfMRI) is a method to explore the neurophysiological basis of resting-state functional connectivity (rsFC), which is defined as the temporal correlation of blood-oxygen-level-dependent (BOLD) signals between spatially distinct brain regions at rest (19). The advantages of rsfMRI are that there is no need to concurrently administer and monitor tasks, and task performance does not affect the interpretation of the results. Although the biological background of rsfMRI is not fully understood compared with that of task-related fMRI, rsfMRI has the advantages of being performed in patients who are unable to perform tasks accurately and has a low burden of data acquisition. These characteristics allow for broad sampling of the patient population. In other psychiatric disorders, mega-analyses using a large amount of rsfMRI data have been conducted (20, 21). Many studies have focused on resting-state functional connectivity and reported abnormalities that may lead to the discovery of biomarkers related to psychiatric disorders (21–25). Up to date, there is only one study focusing rsfMRI in HD, and that study using independent component analysis (ICA) (26). ICA is a data-driven approach that can assess general connectivity patterns in the entire brain without prior hypotheses. In their experiment, they measured the resting-state brain activity in patients with HD, HD and major depressive disorder (MDD), MDD, and healthy subjects. The participants were separated into two groups according to the presence or absence of an HD diagnosis. They found that participants with HD diagnosis have shown abnormalities in major brain networks, including the salience network (SN), default mode network (DMN), and frontoparietal network (FPN) compared to participants without HD diagnosis. However, there were no common abnormal networks in the HD and HD+MDD groups. These results may have been influenced by the small sample size in each group (8 in the MDD group and 10 in the HD+MDD group), and the fact that the non-HD group included some patients with MDD. It remains unclear whether there are any alterations in resting-state functional connectivity of the insula and ACC. Seed-based analysis can test hypotheses focusing specific brain areas by measuring the functional connectivity between specific regions of interest (ROIs) and the whole brain (27), and the interpretation of the results is straightforward compared with the ICA approach (28). The aim of this study was to clarify the rsFC, using seed-based analysis with ROIs, related to insula and ACC, which may be involved in the neurobiological basis of HD. The aim of this study was to clarify the rsFC, using seed-based analysis with ROIs, related to insula and ACC, which may be involved in the neurobiological basis of HD. Based on previous literature on HD, we hypothesized that patients with HD may have abnormalities in the rsFC of the insula and ACC. Specifically, the IFG, orbitofrontal cortex, dorsolateral prefrontal cortex, and superior temporal gyrus (STG) are anatomically connected to the insula and ACC and have shown abnormalities along with the insula and ACC in previous task-related fMRI on HD. Therefore, we expected that patients with HD may show abnormalities in the rsFC between the insula and ACC and these regions.
2 Methods
2.1 Participants
A total of 56 subjects, including 25 patients with HD and 31 healthy controls (HCs) matched for age, sex, and dominant hand, were enrolled. One patient with HD was excluded because of a high rate of invalid volumes, as described below. A total of 24 patients with HD and 31 HCs were included in this study. All patients were recruited from the Department of Neuropsychiatry at Kyushu University Hospital, Japan. HCs were recruited from the local community and interviewed using the Structured Clinical Interview for DSM-IV non-patient edition (SCID-I/NP) (29). None of the HCs had Axis I disorders. Participants were excluded if they had a neurological disorder, head injury, serious medical conditions, a history of drug or alcohol dependence, or comorbidities of major psychiatric disorders such as schizophrenia or bipolar disorder. All participants provided written informed consent before enrollment in the study. This study was conducted in accordance with the Declaration of Helsinki and approved by the Ethics Committee of Kyushu University.
2.2 Clinical assessments
All participants were evaluated for OCD severity, anxiety, and depression symptoms using the Yale-Brown Obsessive-Compulsive Scale (Y-BOCS) (30, 31), Hamilton Rating Scale for Anxiety (HAM-A), and Hamilton Rating Scale for Depression (HAM-D:17-item version) (32, 33). In the HD group, the Structured Interview for Hoarding Disorders (SIHD) (34) was used to confirm they fulfilled the DSM-5 criteria for HD as the primary diagnosis and to exclude secondary hoarding symptoms to OCD and autism spectrum disorder. The HD group was further evaluated for the severity of hoarding symptoms using the Hoarding Rating Scale-Interview (HRS-I) (35), Clutter Imaging Rating (CIR) (36), and Saving Inventory-Revised (SI-R) (37). Current comorbid disorders were also evaluated, including attention deficit hyperactivity disorder (ADHD), using the Structured Clinical Interview for DSM-IV Axis I Disorders-Patient Edition (SCID-I/P) (38) and Conners’ Adult ADHD Diagnostic Interview for DSM-IV (CAADID) (39). In addition, the severity of ADHD symptoms was evaluated using the Conners’ Adult ADHD Rating Scales-Self-Report: Long Version (CAARS-S:L) (40).
2.3 Brain image acquisition
Brain imaging was performed using a 3.0-Tesla MRI scanner (Achieva TX,Phillips Healthcare,Best, Netherlands) at the Department of Neuropsychiatry, Kyushu University Hospital, Japan. All the participants underwent fMRI sequencing. Prior to the resting-state fMRI scan, the participants were instructed to relax and keep their eyes opened and fixed on a gray cross symbol on the screen. First, we acquired a T2*-weighted gradient-echo echo-planar imaging (EPI) sequence (echo time [TE], 30 ms; repetition time [TR], 2500 ms; field of view [FOV], 212×212 mm; matrix, 64×64; slice thickness=3.2 mm; flip angle, 80°) from each participant. After an initial 10-s dummy scan, 240 real scans during a 10-min period were performed. High-resolution T1-weighted anatomical images (TE=3.8 ms; TR=8.2 ms; FOV 240×240 mm; flip angle 8°; slice thickness, 1 mm; inversion time=1026 ms) were also acquired for each participant after the EPI sequence.
2.4 MRI data preprocessing
The preprocessing steps were the same as those described in our previous studies (41, 42). CONN Toolbox 17.f (http://www.nitrc.org/projects/conn) was used for MRI data preprocessing. After removal of the first four volumes, 236 volumes were preprocessed using default preprocessing parameters of the CONN toolbox. Anatomical and functional images were realigned and normalized according to the standard Montreal Neurological Institute (MNI) template with slice timing corrected according to the slice order. Spatial smoothing was conducted with a 6-mm full-width-at-half-maximum Gaussian kernel. For temporal filtering, the fMRI data were band-pass filtered using the default settings in CONN (0.008–0.09 Hz). Twelve potential noise components, including six rigid-body parameters, were estimated for each participant. Outlier volumes were detected using Artifact Detection Tools (ART) software with a setting to detect volumes that had greater head motion than 0.9 mm from the previous image, and a z-score of 5 for scan-to-scan changes in the global signal. Participants with a high rate of outlier volume (>30%) were excluded from the analysis (43). There was no significant difference between HD and HC groups in motion parameters (mean FD [t =-0.384, p >0.05]).
2.5 MRI data analysis
After preprocessing, BOLD signal time-series correlations between each source pair for each participant were calculated over the resting time series, and Fisher z-transformation was applied. Seed-based connectivity maps were generated for each seed ROI of each participant. We created the seed ROIs as an 8 mm radius sphere with MNI coordinates in the right insula (30, 15, –3), left insula (-36, 12, -3), and ACC (0, 30, 21) based on a prior task-related functional MRI study of HD (16). Covariates included age, dummy-coded sex, and dummy-coded psychological medication use. We examined differences in functional connectivity from seed ROIs to whole-brain voxels between the HD and HC groups using a two-sample t-test. The significance level was set at individual voxel p < 0.001, and a cluster size threshold of p < 0.05 was corrected for false discovery rate. Furthermore, within the HD group, we investigated the correlations between the rsFC changes detected in group comparisons and the clinical symptoms. For detail, from the results of analysis of covariance group comparison between groups, we extracted the beta values, and transformed them to z-values, and then we conducted a correlation analysis between these altered rsFC and some clinical scores (HRS-I SI-R Total, SI-R Discarding, SI-R Clutter, SI-R Acquiring, CIR Mean, Y-BOCS, HAM-A, HAM-D, and CAARS-S:L).
3 Results
3.1 Demographic and clinical characteristics
The characteristics of patients with HD and HCs are shown in Table 1. The groups did not differ significantly in age (t (53) = 0.0244, p = 0.98 > 0.05), sex (χ2 (1) =0.017, p = 0.99 > 0.05), or handedness (χ2 (1) =0.293, p = 0.98 > 0.05). The HD group had hoarding symptoms above the cutoff value of the SI-R, and the severity of these symptoms was moderate or higher in the HRS-I. The HD group had significantly higher Y-BOCS (t (53) = 11.1, p < 0.001), HAM-D (t (53) = 4.18, p < 0.001), and HAM-A (t (53) = 3.85, p < 0.001) scores than HCs. Six participants in the HD group were diagnosed with HD only, and 18 participants had a variety of comorbidities, including major depressive disorder (n = 4), OCD (n = 8), ADHD (n = 10), post-traumatic stress disorder (PTSD) (n = 3), and anxiety disorder (n = 5), including specific phobia (n = 3), social anxiety disorder (n= 2), panic disorder (n = 1), and generalized anxiety disorder (n = 2). According to the SIHD, SCID-I/P, and CAADID, all participants in the HD group met the criteria for primary HD and did not show hoarding symptoms secondary to comorbidities. Twelve patients in the HD group were psychotropic medication-free, whereas the other 12 were taking antidepressants (n = 10), major tranquilizers (n = 1), non-stimulant ADHD medications (n = 3), and other medications. None of the participants in the HC group had taken psychotropic drugs during their lifetime.
3.2 Between-group differences in resting-state functional connectivity from ROI to whole brain voxels between HD and HCs
Connectivity comparisons between the groups revealed that patients with HD had significantly lower functional connectivity between the right insula and right IFG (peak MNI coordinates [52, 34, 4]) and between the right insula and left STG (peak MNI coordinates [-38, 0, -12]) compared to HCs (Table 2, Figure 1). There was no significant group difference in functional connectivity of the ACC and left insula in whole-brain voxels. When we included FD as a covariate of no interest, no changes were observed in our results. Furthermore, functional connectivity, which was abnormal in the comparison between groups, did not correlate significantly with the clinical assessments of the correlation coefficient (p <0.05) (Supplementary Table 1).
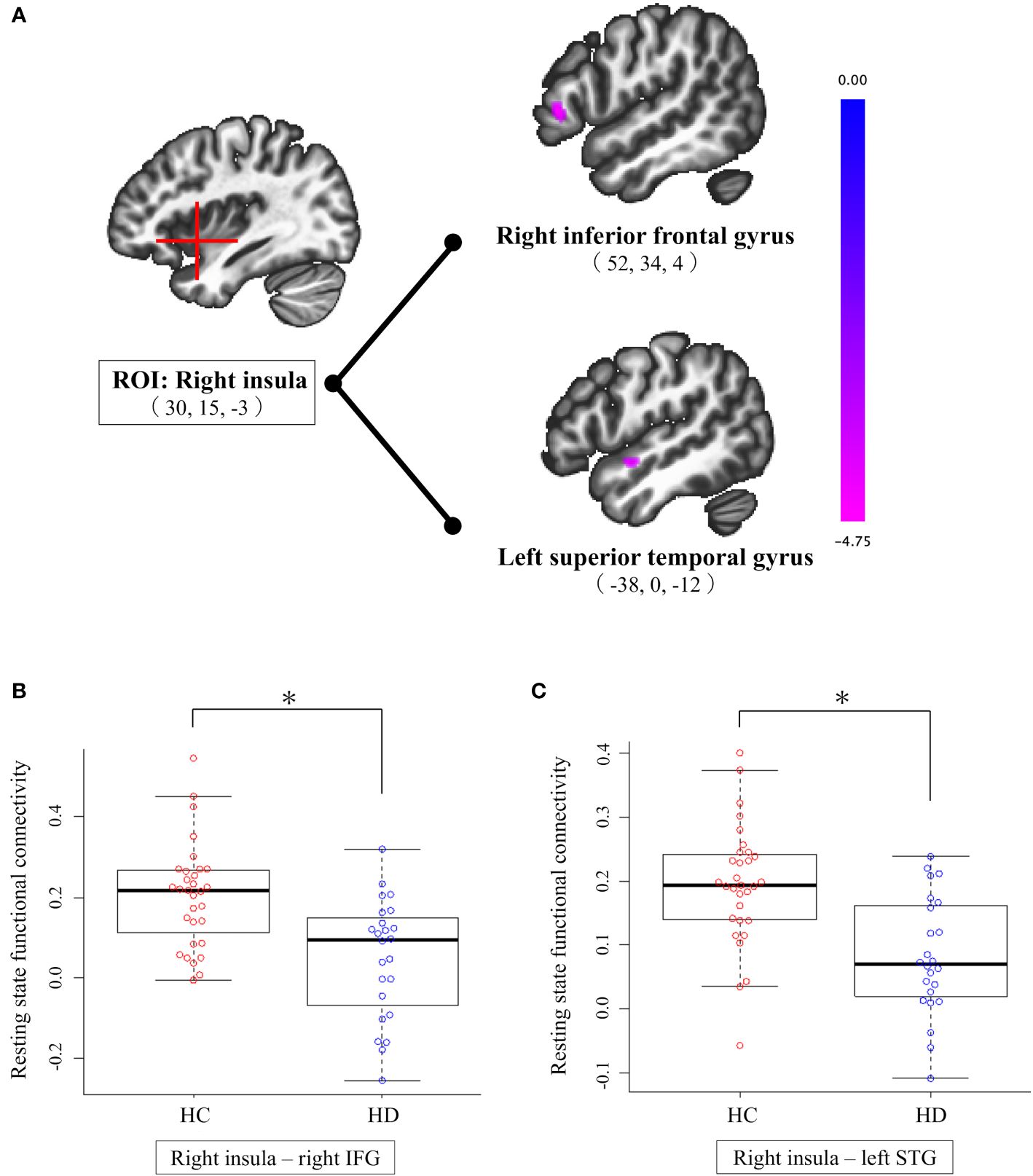
Figure 1 Results of seed-based analysis comparing right insula-right IFG and right insula-left STG connectivity between individuals with HD and HCs. (A) Patients with HD showed significantly lower functional connectivity from the right insula to the right IFG and left STG compared to HCs (cluster size corrected significance p < 0.05, after applying a per-voxel height threshold of p < 0.001). Peak coordinates are given in MNI space. (B) Group difference in right insula-right IFG connectivity; compared to HCs, individuals with HD exhibit significantly lower connectivity between right insula and right IFG. (C) Group difference in right insula-left STG connectivity; compared to HCs, individuals with HD exhibit significantly lower connectivity between right insula and left STG. HC, healthy control; HD, hoarding disorder; IFG, inferior frontal gyrus; MNI, Montreal Neurological Institute; ROI, region of interest; STG, superior temporal gyrus. *p-FDR <.05 (representing a Holm-corrected p-value adjusted for three ROIs comparisons after cluster-level FDR correction).
4 Discussion
We found that patients with HD had lower rsFC between the right insula and right IFG and left STG compared to HCs. Given the lack of correlations between rsFC abnormalities and clinical symptoms of HD, the clinical implications of the present findings are not clear. However, considering the functional roles of these regions and previous findings on HD, we speculated that lower functional connectivity between the right insula and right IFG could be associated with the pathophysiology of HD. The right insula is known to identify salient stimuli from a variety of stimuli, including emotional responses, and facilitate information processing (44, 45). The IFG plays an important role in cognitive control (46–48), particularly relevant to emotional control through reappraisal strategies that regulate emotions by changing the interpretation of situations, stimuli, and mental states (49, 50). The IFG and insula are implicated in various cognitive control functions (45, 49–53), and functional connectivity between these regions has also been suggested to be related to emotional regulation (51, 52). Lower functional connectivity between the right insula and right IFG in the present study show an important overlap with those of previous findings on HD. In a study of HD for the disposal task, which we referenced in our seed ROIs, in addition to biphasic changes in brain activity in the insula and ACC, the activation of the insula and IFG in patients with HD was positively correlated with the degree of indecisiveness when considering the disposal of their own possessions (16). Another fMRI study using a disposal task suggested that patients with HD have semantic processing impairments based on the lower brain activity of the IFG during semantic processing tasks (17). Indecisiveness and semantic processing impairments can lead to hoarding symptoms, as they make it more difficult to make decisions about the disposal of their own possessions. It is hypothesized that changes in brain activity of the insula and IFG during hoarding symptom-induced tasks is related to the index of cognitive control in the context of indecisiveness and semantic processing in HD. Therefore, lower rsFC between the right insula and right IFG in the present study indicates that there are functional alterations in these regions, even in the resting state. As we did not perform neuropsychological tests of cognitive control function in this study, it is not possible to associate these results directly with impaired cognitive control in HD. However, it is interesting that regions identified in our study overlapped with the brain areas identified in previous task-related fMRI studies on HD. Alterations in brain regions that occur both during cognitive tasks and in the resting state may provide important insights into the neurobiological basis of HD.
In the present study, patients with HD had lower functional connectivity between the right insula and left STG compared to HCs. The abnormalities we observed in the STG match the results of several previous task-related fMRI studies on HD. Specifically, when patients with HD decided to keep or dispose of their personal possessions instead of the experimenter’s possessions, the brain activity in the left STG was positively correlated with self-reported sadness and “not just right” feelings (16). In another fMRI study, the brain activity in the left STG during both decisions to acquire and to discard possessions was significantly correlated with the severity of difficulty in discarding (17). Also, atrophy of the left temporal lobe, including the STG and insula, was associated with the prevalence of hoarding symptoms in frontotemporal dementia, particularly semantic dementia (54). The STG is hypothesized to be involved in semantic processing, underlying various cognitive control functions (46, 55–57). Previous studies showed that the STG is associated with the neurobiological basis of HD or hoarding symptoms. Our observation of lower rsFC between the right insula and left STG adds new evidence that patients with HD have abnormalities in the STG even in the resting state. However, because there was no correlation between the resting state functional change and the clinical symptoms, we cannot propose clinical applications of our results. Future studies are needed to examine the relationship between these rsFC changes in the STG and clinical implications for HD.
In contrast to our prior hypothesis, there was no significant difference in functional connectivity from the ACC between patients with HD and HCs, although previous neuroimaging studies reported characteristic ACC activities in patients with HD (14, 16, 17). The ACC and insula are key components of the salience network which regulates cognitive function and facilitates information processing (44, 45). Moreover, the ACC can be anatomically divided into dorsal and ventral regions which play different roles (Dixon et al., 2017, Etkin et al., 2011, Heilbronner and Hayden, 2016, Kolling et al., 2016, Lockwood and Wittmann, 2018). The dorsal ACC has been suggested to exhibit a central role in integrating information about errors, conflicts, negative feedback, and rewards, and assigning the appropriate cognitive control needed for action and decision making (53, 58, 59). The ventral ACC has been associated with the appraisal of interoceptive sensations related to subjective emotions (53, 60, 61). Previous neuroimaging studies on HD have shown that most ACC abnormalities are located in the dorsal regions which are often hyperactive during symptom-inducing tasks and hypoactive during non-symptom-inducing tasks (14, 16, 17). These abnormalities in the dorsal ACC may prevent patients with HD from correctly assessing the value of stimuli and may cause their abnormally high estimates of the risk of making errors, which lead to hoarding symptoms. Therefore, we selected an ROI in the dorsal part of the ACC and expected patients with HD to show abnormalities in the dorsal ACC and insula, even at rest. However, the present results suggest that rsFC changes in patients with HD are not engaging the dorsal ACC. While the interpretation of this discrepancy of the results is unclear, it should be noted that our study evaluated resting-state functional connectivity from ACC, not the regional brain activity of ACC itself. Moreover, owing to the following limitations of this study, further studies are needed to draw definite conclusions about the abnormalities of the ACC in patients with HD.
This study had several limitations. First, because of the small sample size and large number of comorbidities, it was not possible to analyze group differences according to comorbidity. In addition, the HD group had a high rate of psychotropic medication use because of comorbidities. HD is known to have a high comorbidity rate of approximately 75% (62), and the HD group in this study also had a similar rate of comorbidity. As a result, psychotropic drugs were used more frequently in the HD group. To examine the influence of comorbidities in the present study, we evaluated the correlation coefficient between the severity of comorbidities and abnormalities of functional connectivity. However, there was no significant correlation between altered functional connectivity and comorbidity severity. To test the effect of medication, we divided HD group into those with and without medication, and we investigated group difference from each ROIs using age and gender as covariate of no interest. As a result, there were no significant group differences between the group under medication and without medication. Second, we did not evaluate any cognitive functions in this study; therefore, we cannot predict the clinical implications of altered functional connectivity in HD. To assess whether the present findings are related to impairments in cognitive control in patients with HD, it is necessary to investigate the relationship between neuropsychological testing for cognitive control and brain activity during tasks or in the resting state. Future studies should increase the sample size and adjust groups according to the typical comorbidities of HD, such as depression, ADHD, and OCD, to test whether abnormal rsFC is a specific feature of HD. Third, methodological issues could have affected our results. In this study, we used seed-based analysis from the insula and ACC to whole-brain voxels. We selected the insula and ACC as ROIs with reference to a previous study on HD using symptom provocation paradigm task fMRI (16); therefore, we may have missed abnormalities in other regions that we did not evaluate. Furthermore, we determined the ROI coordinates based on that of previous fMRI studies, so a subtle difference in ROI coordinates could partially affect our results. Despite these limitations, this study has certain significance. It is the first to demonstrate that abnormalities related to the insula, which have been observed in task-related fMRI studies on HD, may also be present in the resting state.
5 Conclusion
We found lower rsFC between the right insula and right IFG and left STG in patients with HD compared to HCs. These brain regions overlap with those identified in previous task-related fMRI studies on HD. These results suggest that patients with HD have functional alterations in these brain areas, even in the resting state. Future studies are needed to clarify the clinical implication of these changes in resting-state functional connectivity in patients with HD.
Data availability statement
The original contributions presented in the study are included in the article/Supplementary Material. Further inquiries can be directed to the corresponding author.
Ethics statement
The studies involving humans were approved by The Ethics Committee of Kyushu University. The studies were conducted in accordance with the local legislation and institutional requirements. The participants provided their written informed consent to participate in this study.
Author contributions
KK: Conceptualization, Formal analysis, Investigation, Visualization, Writing – original draft, Writing – review & editing. HT: Formal analysis, Funding acquisition, Methodology, Writing – review & editing. KeM: Funding acquisition, Writing – review & editing. TM: Investigation, Writing – review & editing. AM: Investigation, Writing – review & editing. NN: Investigation, Writing – review & editing. KoM: Investigation, Writing – review & editing. MK: Data curation, Writing – review & editing. KS: Data curation, Writing – review & editing. KfK: Investigation, Writing – review & editing. OT: Investigation, Writing – review & editing. TN: Funding acquisition, Supervision, Writing – review & editing.
Funding
The author(s) declare that financial support was received for the research, authorship, and/or publication of this article. This work was supported by JSPS KAKENHI Grant Number (C) JP24K10681, JP21K07547, JP22K07598, and JP22K15766.
Conflict of interest
The authors declare that the research was conducted in the absence of any commercial or financial relationships that could be construed as a potential conflict of interest.
Publisher’s note
All claims expressed in this article are solely those of the authors and do not necessarily represent those of their affiliated organizations, or those of the publisher, the editors and the reviewers. Any product that may be evaluated in this article, or claim that may be made by its manufacturer, is not guaranteed or endorsed by the publisher.
Supplementary material
The Supplementary Material for this article can be found online at: https://www.frontiersin.org/articles/10.3389/fpsyt.2024.1399062/full#supplementary-material
References
1. American Psychiatric Association. Diagnostic and Statistical Manual of Mental Disorders. 5th ed. Washington, DC: American Psychiatric Publishing, Inc. (2013). doi: 10.1176/appi.books.9780890425596
2. Postlethwaite A, Kellett S, Mataix-Cols D. Prevalence of hoarding disorder: A systematic review and meta-analysis. J Affect Disord. (2019) 256:309–16. doi: 10.1016/j.jad.2019.06.004
3. Ruscio AM, Stein DJ, Chiu WT, Kessler RC. The epidemiology of obsessive-compulsive disorder in the national comorbidity survey replication. Mol Psychiatry. (2010) 15:53–63. doi: 10.1038/mp.2008.94
4. Bodryzlova Y, O'Connor K. Factors affecting the referral rate of the hoarding disorder at primary mental health care in Quebec. Community Ment Health J. (2018) 54:773–81. doi: 10.1007/s10597-018-0234-z
5. Grisham JR, Norberg MM, Williams AD, Certoma SP, Kadib R. Categorization and cognitive deficits in compulsive hoarding. Behav Res Ther. (2010) 48:866–72. doi: 10.1016/j.brat.2010.05.011
6. Ayers CR, Wetherell JL, Schiehser D, Almklov E, Golshan S, Saxena S. Executive functioning in older adults with hoarding disorder. Int J Geriatr Psychiatry. (2013) 28:1175–81. doi: 10.1002/gps.3940
7. McMillan SG, Rees CS, Pestell C. An investigation of executive functioning, attention and working memory in compulsive hoarding. Behav Cognit Psychother. (2013) 41:610–25. doi: 10.1017/S1352465812000835
8. Nakao T, Kanba S. Pathophysiology and treatment of hoarding disorder. Psychiatry Clin Neurosci. (2019) 73:370–5. doi: 10.1111/pcn.12853
9. Tolin DF, Levy HC, Wootton BM, Hallion LS, Stevens MC. Hoarding disorder and difficulties in emotion regulation. J Obsessive Compuls Relat Disord. (2018) 16:98–103. doi: 10.1016/j.jocrd.2018.01.006
10. Castriotta N, Dozier ME, Taylor CT, Mayes T, Ayers CR. Intolerance of uncertainty in hoarding disorder. J Obsessive Compuls Relat Disord. (2019) 21:97–101. doi: 10.1016/j.jocrd.2018.11.005
11. Pushkarskaya H, Tolin DF, Henick D, Levy I, Pittenger C. Unbending mind: individuals with hoarding disorder do not modify decision strategy in response to feedback under risk. Psychiatry Res. (2018) 259:506–13. doi: 10.1016/j.psychres.2017.11.001
12. Wheaton MG, Topilow K. Maximizing decision-making style and hoarding disorder symptoms. Compr Psychiatry. (2020) 101:152187. doi: 10.1016/j.comppsych.2020.152187
13. Tolin DF, Witt ST, Stevens MC. Hoarding disorder and obsessive-compulsive disorder show different patterns of neural activity during response inhibition. Psychiatry Res. (2014) 221:142–8. doi: 10.1016/j.pscychresns.2013.11.009
14. Hough CM, Luks TL, Lai K, Vigil O, Guillory S, Nongpiur A, et al. Comparison of brain activation patterns during executive function tasks in hoarding disorder and non-hoarding Ocd. Psychiatry Res Neuroimaging. (2016) 255:50–9. doi: 10.1016/j.pscychresns.2016.07.007
15. Sunol M, Martinez-Zalacain I, Pico-Perez M, Lopez-Sola C, Real E, Fullana MA, et al. Differential patterns of brain activation between hoarding disorder and obsessive-compulsive disorder during executive performance. Psychol Med. (2020) 50:666–73. doi: 10.1017/S0033291719000515
16. Tolin DF, Stevens MC, Villavicencio AL, Norberg MM, Calhoun VD, Frost RO, et al. Neural mechanisms of decision making in hoarding disorder. Arch Gen Psychiatry. (2012) 69:832–41. doi: 10.1001/archgenpsychiatry.2011.1980
17. Stevens MC, Levy HC, Hallion LS, Wootton BM, Tolin DF. Functional neuroimaging test of an emerging neurobiological model of hoarding disorder. Biol Psychiatry Cognit Neurosci Neuroimaging. (2020) 5:68–75. doi: 10.1016/j.bpsc.2019.08.010
18. Tolin DF, Levy HC, Hallion LS, Wootton BM, Jaccard J, Diefenbach GJ, et al. Changes in neural activity following a randomized trial of cognitive behavioral therapy for hoarding disorder. J Consult Clin Psychol. (2023) 91:242–50. doi: 10.1037/ccp0000804
19. Lee MH, Smyser CD, Shimony JS. Resting-state Fmri: A review of methods and clinical applications. AJNR Am J Neuroradiol. (2013) 34:1866–72. doi: 10.3174/ajnr.A3263
20. van den Heuvel OA, Boedhoe PSW, Bertolin S, Bruin WB, Francks C, Ivanov I, et al. An overview of the first 5 years of the enigma obsessive-compulsive disorder working group: the power of worldwide collaboration. Hum Brain Mapp. (2022) 43:23–36. doi: 10.1002/hbm.24972
21. Bruin WB, Abe Y, Alonso P, Anticevic A, Backhausen LL, Balachander S, et al. The functional connectome in obsessive-compulsive disorder: resting-state mega-analysis and machine learning classification for the enigma-Ocd consortium. Mol Psychiatry. (2023) 28:4307–19. doi: 10.1038/s41380–023-02077–0
22. Zhang X, Yang X, Wu B, Pan N, He M, Wang S, et al. Large-scale brain functional network abnormalities in social anxiety disorder. Psychol Med. (2023) 53:6194–204. doi: 10.1017/S0033291722003439
23. Muratore AF, Foerde K, Lloyd EC, Touzeau C, Uniacke B, Aw N, et al. Reduced dorsal fronto-striatal connectivity at rest in anorexia nervosa. Psychol Med. (2024), 1–10. doi: 10.1017/S003329172400031X
24. Russman Block S, Norman LJ, Zhang X, Mannella KA, Yang H, Angstadt M, et al. Resting-state connectivity and response to psychotherapy treatment in adolescents and adults with ocd: A randomized clinical trial. Am J Psychiatry. (2023) 180:89–99. doi: 10.1176/appi.ajp.21111173
25. Mason L, Peters E, Kumari V. Functional connectivity predictors and mechanisms of cognitive behavioural therapies: A systematic review with recommendations. Aust N Z J Psychiatry. (2016) 50:311–21. doi: 10.1177/0004867415624970
26. Levy HC, Stevens MC, Glahn DC, Pancholi K, Tolin DF. Distinct resting state functional connectivity abnormalities in hoarding disorder and major depressive disorder. J Psychiatr Res. (2019) 113:108–16. doi: 10.1016/j.jpsychires.2019.03.022
27. Biswal B, Yetkin FZ, Haughton VM, Hyde JS. Functional connectivity in the motor cortex of resting human brain using Echo-Planar Mri. Magn Reson Med. (1995) 34:537–41. doi: 10.1002/mrm.1910340409
28. Wu L, Caprihan A, Bustillo J, Mayer A, Calhoun V. An approach to directly link Ica and seed-based functional connectivity: application to Schizophrenia. Neuroimage. (2018) 179:448–70. doi: 10.1016/j.neuroimage.2018.06.024
29. First MB, Spitzer RL, Miriam G, Williams JB. Structured Clinical Interview for Dsm-Iv-Tr Axis I Disorders, Research Version, Non-Patient Edition (Scid-I/Np). New York, NY: Biometrics Research Department (2002).
30. Goodman WK, Price LH, Rasmussen SA, Mazure C, Fleischmann RL, Hill CL, et al. The Yale-Brown obsessive compulsive scale. I. Development, use, and reliability. Arch Gen Psychiatry. (1989) 46:1006–11. doi: 10.1001/archpsyc.1989.01810110048007
31. Goodman WK, Price LH, Rasmussen SA, Mazure C, Delgado P, Heninger GR, et al. The yale-brown obsessive compulsive scale. Ii. Validity. Arch Gen Psychiatry. (1989) 46:1012–6. doi: 10.1001/archpsyc.1989.01810110054008
32. Hamilton M. The assessment of anxiety-states by rating. Br J Med Psychol. (1959) 32:50–5. doi: 10.1111/j.2044-8341.1959.tb00467.x
33. Hamilton M. A rating scale for depression. J Neurol Neurosurg Psychiatry. (1960) 23:56–62. doi: 10.1136/jnnp.23.1.56
34. Nordsletten AE, Fernandez de la Cruz L, Pertusa A, Reichenberg A, Hatch SL, Mataix-Cols D. The structured interview for hoarding disorder (Sihd): development, usage and further validation. J Obsessive-Compulsive Related Disord. (2013) 2:346–50. doi: 10.1016/j.jocrd.2013.06.003
35. Tolin DF, Frost RO, Steketee G. A brief interview for assessing compulsive hoarding: the hoarding rating scale-interview. Psychiatry Res. (2010) 178:147–52. doi: 10.1016/j.psychres.2009.05.001
36. Frost RO, Steketee G, Tolin DF, Renaud S. Development and validation of the clutter image rating. J Psychopathol Behav Assess. (2007) 30:193–203. doi: 10.1007/s10862–007-9068–7
37. Frost RO, Steketee G, Grisham J. Measurement of compulsive hoarding: saving inventory-revised. Behav Res Ther. (2004) 42:1163–82. doi: 10.1016/j.brat.2003.07.006
38. First MB, Spitzer RL, Gibbon M, Williams JB. Structured clinical interview for axis I Dsm-Iv disorders-patient edition (Scid-I/P). New York: Biometrics Res Department New York State Psychiatr Institute. (1995) 531:1987–95. doi: 10.1037/t07827-000
39. Epstein JN, Kollins SH. Psychometric properties of an adult adhd diagnostic interview. J attention Disord. (2006) 9:504–14. doi: 10.1177/1087054705283575
40. Conners CK, Erhardt D, Sparrow E. Conner's adult adhd rating scales: technical manual: multi-health systems incorporated (MHS). Arch Clin Neuropsychol. (1999) 18(4):431–7. doi: 10.1016/S0887-6177(03)00021-0
41. Tomiyama H, Murayama K, Nemoto K, Tomita M, Hasuzawa S, Mizobe T, et al. Increased functional connectivity between presupplementary motor area and inferior frontal gyrus associated with the ability of motor response inhibition in obsessive-compulsive disorder. Hum Brain Mapp. (2022) 43:974–84. doi: 10.1002/hbm.25699
42. Murayama K, Tomiyama H, Tsuruta S, Ohono A, Kang M, Hasuzawa S, et al. Aberrant resting-state cerebellar-cerebral functional connectivity in unmedicated patients with obsessive-compulsive disorder. Front Psychiatry. (2021) 12:659616. doi: 10.3389/fpsyt.2021.659616
43. Paul AK, Bose A, Kalmady SV, Shivakumar V, Sreeraj VS, Parlikar R, et al. Superior temporal gyrus functional connectivity predicts transcranial direct current stimulation response in Schizophrenia: A machine learning study. Front Psychiatry. (2022) 13:923938. doi: 10.3389/fpsyt.2022.923938
44. Menon V, Uddin LQ. Saliency, switching, attention and control: A network model of insula function. Brain Struct Funct. (2010) 214:655–67. doi: 10.1007/s00429–010-0262–0
45. Pavuluri M, May A. I feel, therefore, I am: the insula and its role in human emotion, cognition and the sensory-motor system. AIMS Neurosci. (2015) 2:18–27. doi: 10.3934/Neuroscience.2015.1.18
46. Noonan KA, Jefferies E, Visser M, Lambon Ralph MA. Going beyond inferior prefrontal involvement in semantic control: evidence for the additional contribution of dorsal angular gyrus and posterior middle temporal cortex. J Cognit Neurosci. (2013) 25:1824–50. doi: 10.1162/jocn_a_00442
47. Liakakis G, Nickel J, Seitz RJ. Diversity of the inferior frontal gyrus–a meta-analysis of neuroimaging studies. Behav Brain Res. (2011) 225:341–7. doi: 10.1016/j.bbr.2011.06.022
48. Hartwigsen G, Neef NE, Camilleri JA, Margulies DS, Eickhoff SB. Functional segregation of the right inferior frontal gyrus: evidence from coactivation-based parcellation. Cereb Cortex. (2019) 29:1532–46. doi: 10.1093/cercor/bhy049
49. Grecucci A, Giorgetta C, Bonini N, Sanfey AG. Reappraising social emotions: the role of inferior frontal gyrus, temporo-parietal junction and insula in interpersonal emotion regulation. Front Hum Neurosci. (2013) 7:523. doi: 10.3389/fnhum.2013.00523
50. Pan WG, Wang T, Wang XP, Hitchman G, Wang LJ, Chen AT. Identifying the core components of emotional intelligence: evidence from amplitude of low-frequency fluctuations during resting state. PloS One. (2014) 9. doi: 10.1371/journal.pone.0111435
51. Li W, Yang P, Ngetich RK, Zhang J, Jin Z, Li L. Differential involvement of frontoparietal network and insula cortex in emotion regulation. Neuropsychologia. (2021) 161:107991. doi: 10.1016/j.neuropsychologia.2021.107991
52. Li W, Xie K, Ngetich RK, Zhang J, Jin Z, Li L. Inferior frontal gyrus-based resting-state functional connectivity and medium dispositional use of reappraisal strategy. Front Neurosci. (2021) 15:681859. doi: 10.3389/fnins.2021.681859
53. Dixon ML, Thiruchselvam R, Todd R, Christoff K. Emotion and the prefrontal cortex: an integrative review. Psychol Bull. (2017) 143:1033–81. doi: 10.1037/bul0000096
54. Mitchell E, Tavares TP, Palaniyappan L, Finger EC. Hoarding and obsessive-compulsive behaviours in frontotemporal dementia: clinical and neuroanatomic associations. Cortex. (2019) 121:443–53. doi: 10.1016/j.cortex.2019.09.012
55. Jefferies E. The neural basis of semantic cognition: converging evidence from neuropsychology, neuroimaging and Tms. Cortex. (2013) 49:611–25. doi: 10.1016/j.cortex.2012.10.008
56. Jackson RL, Hoffman P, Pobric G, Lambon Ralph MA. The semantic network at work and rest: differential connectivity of anterior temporal lobe subregions. J Neurosci. (2016) 36:1490–501. doi: 10.1523/JNEUROSCI.2999–15.2016
57. Petrides M. On the evolution of polysensory superior temporal sulcus and middle temporal gyrus: A key component of the semantic system in the human brain. J Comp Neurol. (2023) 531:1987-1995.. doi: 10.1002/cne.25521
58. Heilbronner SR, Hayden BY. Dorsal anterior cingulate cortex: A bottom-up view. Annu Rev Neurosci. (2016) 39:149–70. doi: 10.1146/annurev-neuro-070815–013952
59. Kolling N, Wittmann MK, Behrens TE, Boorman ED, Mars RB, Rushworth MF. Dorsal anterior cingulate cortex and the value of control. Nat Neurosci. (2016) 19:1280–5. doi: 10.1038/nn.4382
60. Lockwood PL, Wittmann MK. Ventral anterior cingulate cortex and social decision-making. Neurosci Biobehav Rev. (2018) 92:187–91. doi: 10.1016/j.neubiorev.2018.05.030
61. Etkin A, Egner T, Kalisch R. Emotional processing in anterior cingulate and medial prefrontal cortex. Trends Cognit Sci. (2011) 15:85–93. doi: 10.1016/j.tics.2010.11.004
Keywords: hoarding disorder, resting-state, functional connectivity, seed-based analysis, cognitive control
Citation: Kato K, Tomiyama H, Murayama K, Mizobe T, Matsuo A, Nishida N, Matukuma K, Kang M, Sashikata K, Kikuchi K, Togao O and Nakao T (2024) Reduced resting-state functional connectivity between insula and inferior frontal gyrus and superior temporal gyrus in hoarding disorder. Front. Psychiatry 15:1399062. doi: 10.3389/fpsyt.2024.1399062
Received: 11 March 2024; Accepted: 03 June 2024;
Published: 19 June 2024.
Edited by:
Gerd Wagner, University Hospital Jena, GermanyReviewed by:
Aki Tsuchiyagaito, Laureate Institute for Brain Research, United StatesLuke Norman, National Institute of Mental Health (NIH), United States
Copyright © 2024 Kato, Tomiyama, Murayama, Mizobe, Matsuo, Nishida, Matukuma, Kang, Sashikata, Kikuchi, Togao and Nakao. This is an open-access article distributed under the terms of the Creative Commons Attribution License (CC BY). The use, distribution or reproduction in other forums is permitted, provided the original author(s) and the copyright owner(s) are credited and that the original publication in this journal is cited, in accordance with accepted academic practice. No use, distribution or reproduction is permitted which does not comply with these terms.
*Correspondence: Keitaro Murayama, murayama.keitaro.003@m.kyushu-u.ac.jp