- 1School of Nursing, Southern Medical University, Guangzhou, Guangdong, China
- 2Women and Children Medical Research Center, Foshan Women and Children Hospital, Foshan, Guangdong, China
- 3Clinical Epidemiology Program, Ottawa Hospital Research Institute, Ottawa, ON, Canada
- 4School of Epidemiology and Public Health, University of Ottawa, Ottawa, ON, Canada
- 5Department of Obstetrics and Gynecology, University of Ottawa, Ottawa, ON, Canada
- 6Risk Science International, Ottawa, ON, Canada
Introduction: Understanding the mechanisms underlying maternal postpartum depression (PPD) and its effects on offspring development is crucial. However, research on the association between maternal PPD, gut microbiota, and offspring neurodevelopment remains limited. This study aimed to examine the association of maternal PPD symptoms with early gut microbiome, gut metabolome, and neurodevelopment in infants at 6 months.
Methods: Maternal PPD symptoms were assessed using the Edinburgh Postpartum Depression Scale (EPDS) at 42 days postpartum. Infants stool samples collected at 42 days after birth were analyzed using 16S rRNA sequencing and liquid chromatography–mass spectrometry (LC–MS) detection. Infant neurodevelopment was measured at 6 months using the Ages and Stages Questionnaire, Third Edition (ASQ-3). Correlations between gut microbiota, metabolites and neurodevelopment were identified through co-occurrence network analysis. Finally, mediation analyses were conducted to determine potential causal pathways.
Results: A total of 101 mother-infant dyads were included in the final analysis. Infants born to mothers with PPD symptoms at 42 days postpartum had lower neurodevelopmental scores at 6 months. These infants also had increased alpha diversity of gut microbiota and were abundant in Veillonella and Finegoldia, while depleted abundance of Bifidobacterium, Dialister, Cronobacter and Megasphaera. Furthermore, alterations were observed in metabolite levels linked to the Alanine, aspartate, and glutamate metabolic pathway, primarily characterized by decreases in N-Acetyl-L-aspartic acid, L-Aspartic acid, and L-Asparagine. Co-occurrence network and mediation analyses revealed that N-Acetyl-L-aspartic acid and L-Aspartic acid levels mediated the relationship between maternal PPD symptoms and the development of infant problem-solving skills.
Conclusions: Maternal PPD symptoms are associated with alterations in the gut microbiota and neurodevelopment in infants. This study provides new insights into potential early intervention for infants whose mother experienced PPD. Further research is warranted to elucidate the biological mechanisms underlying these associations.
Introduction
Postpartum depression (PPD) is a prevalent mental health issue in the perinatal period, affecting approximately 17.2% of mothers globally, with rates varying from 6.5% to 60.9% across different populations (1). This condition may emerge during pregnancy or persist beyond the first postpartum month, possibly lasting several years. PPD not only jeopardizes the physical and mental well-being of mothers, but is also linked to adverse impacts on the development and behavior of infants (2). Previous studies have shown that postpartum depressive symptoms were associated with delayed early child development (3) and reduced verbal Intelligence Quotient (IQ) in offspring (4). Similar findings have been observed in animal models, where offspring from stressed dams had increased anxiety-like behavior and behavioral deficits compared to those from non-stressed mothers (5, 6). Elucidating the potential biological mechanisms behind these associations is the key to the development of strategies for early intervention in at-risk infants.
The early stages of life represent a critical window for gut microbial colonization, influencing infant health in terms of growth, neurodevelopment, and temperament (7, 8). Factors such as maternal emotional status can shape the composition of the infant’s early-life gut microbiota. Research indicates that infants born to mothers with higher reported levels of anxiety and perceived stress had less alpha diversity and lower abundance of Bifidobacterium dentium (9). Furthermore, maternal depressive symptoms have been linked to reduced fecal Immunoglobulin A concentrations in infants (10). However, longitudinal studies examining the infant gut microbiota in mediating the effects of maternal PPD on infant health outcomes are lacking.
Therefore, we hypothesize that infants born to mothers with postpartum depressive symptoms are subject to early gut microbiota composition and influencing neurodevelopment. Leveraging data from our previous randomized controlled trial (11), this study aims to investigate the association of maternal PPD symptoms with infant gut microbiota and neurodevelopment.
Materials and methods
Study participants
This study was derived from a triple-blinded randomized controlled trial investigating the effects of vaginal microbiota transfer on the gut microbiome and early neurodevelopment of infants born by cesarean delivery (11). Vaginal delivery cases during the same period served as natural controls. Pregnant women were enrolled from December 2020 to July 2021 at a tertiary teaching hospital in Guangdong, China. Eligible participants were women aged 18 years or older, with singleton intrauterine pregnancies, and newborns who were alive with a gestational age of over 37 weeks. Women were excluded if they were infected with sexually transmitted diseases (STDs), including syphilis, HIV, gonorrhea, and chlamydia. This study enrolled mothers regardless of their delivery mode. Newborns with Apgar scores less than seven at one minute after birth, as well as those with any congenital anomalies or intrauterine infections, were excluded. Given that the focus of this study was to explore the effects of maternal PPD symptoms on infant gut microbiome and neurodevelopment, pregnant women with a history of mental disorders before or during pregnancy, or those using psychiatric medications in the preceding 6 months were also excluded. Informed consent was obtained from all participants. A total of 101 participants were enrolled in the study and completed baseline assessments, irrespective of their group allocations in the randomized trial.
Outcome measures
We assessed infant development using the Ages and Stages Questionnaire, Third Edition (ASQ-3) (12) at 6 months after birth. The assessment covers five domains: communication, problem-solving, gross motor, fine motor, and personal social skills, for a total of 30 age-appropriate items. Each item is assigned a score of 10 if the child has mastered the skill, 5 if the skill is emerging or occasional, and 0 if the child cannot master the skill. The individual domain scores are obtained by summing scores of 6 items, resulting in a range of 0 to 60 for each domain. The total ASQ-3 score is the sum of the five domain scores, yielding a total score range from 0 to 300.
Exposure measures
The primary exposure was maternal PPD symptoms at 42 days postpartum, assessed using the Edinburgh Postpartum Depression Scale (EPDS) (13), thereby establishing two distinct groups: the PPD group and the non-PPD group. Depressive symptoms were measured using the 10-item EPDS, which describes depression as cognitive and affective features that last for at least one week (13). Each item has four possible responses and is scored from 0 to 3; individual item scores are then summed to generate a total score ranging from 0 to 30. Consistent with prior research validating a cutoff score of 11 for better sensitivity to major or minor depression (13–15), scores of 11 or higher were considered indicative of depressive symptoms.
Potential confounders
Potential confounders evaluated included maternal and infant characteristics that have an established or potential associations with infants’ neurodevelopment and/or maternal PPD symptoms. According to previous reports, we considered several maternal and infant characteristics as covariates, including maternal pre-pregnancy BMI (16), weeks of gestation (1, 17), birth mode (vaginal delivery, cesarean section, cesarean section exposed to maternal vaginal fluids) (18), neonatal intensive care unit (NICU) care (19), infant feeding (exclusive breastfeeding, formula feeding, and partial breastfeeding at 42 days postpartum, along with the introduction of complementary foods at 6 months) (20). Demographic and clinical information about the study participants was collected from the transcribed medical records and obtained through a self-administered questionnaire.
Sample collection and gut microbiota sequencing
Infant fecal samples were collected at 42 days after birth. A sterile applicator stick was used to collect stool from a soiled diaper, placing it directly into a sterile collection tube. Samples were then refrigerated until transferred to a -80 °C freezer, where they were stored until sequencing procedures were performed. Genomic DNA was extracted from infant stool using MoBio PowerSoil DNA Isolation Kits (MoBio Laboratories, Carlsbad, CA, USA) in accordance with the manufacturer’s instructions. Amplicon sequencing of the V3-V4 gene region of the 16S ribosomal RNA was conducted, following the procedures detailed elsewhere (11).
Metabolomics profiling
Targeted liquid chromatography–mass spectrometry (LC–MS) was used to characterize the fecal metabolomic signatures as our previously published study (11). Briefly, dried fecal samples were homogenized, extracted, and derivatized. Subsequently, supernatant aliquots underwent ultra-performance liquid chromatography coupled to tandem mass spectrometry. The LC–MS system, controlled by MassLynx 4.1 software, employed an ACQUITY BEH C18 column. A mixed standard solution calibrated the absolute concentrations of metabolites, processed using Targeted Metabolome Batch Quantification (TMBQ) software.
Statistical analyses
Participant characteristics in the PPD and non-PPD groups were compared at 42 days postpartum using appropriate statistical tests. Linear regression analyses were conducted to explore the associations between maternal PPD symptoms and neurodevelopmental outcomes in infants. In the univariate linear regression model, maternal PPD symptoms was the independent variable and ASQ-3 total score and the scores for its 5 domains were the dependent variables. The multivariable linear regression model considered confounders including pre-pregnancy BMI, gestational weeks, delivery mode, NICU care, and complementary food at 6 months. The delivery mode covariate adjusted in this study included categories from the original trial (vaginal delivery, cesarean section, cesarean section with exposure to vaginal fluids) (11). To reduce potential bias from missing data, particularly regarding complementary food intake at 6 months and ASQ-3 scores, we employed a random forest imputation method to estimate and impute the missing values in the dataset. A sensitivity analysis was conducted to assess the robustness of the association between maternal PPD symptoms and infant neurodevelopment after imputation.
Microbiome alpha diversity evenness was assessed using the Shannon diversity index and compared between the PPD and non-PPD groups through the Wilcoxon rank-sum test. Differences in community-level composition, as measured by unweighted UniFrac distances matrices between the two groups, were assessed using permutation multivariate analysis of variance (PERMANOVA) with the adonis2 function (vegan package) (21).
The Linear Discriminant Analysis Effect Size (LEfSe) algorithm was used to identify differentially abundant taxa between groups with a logarithmic linear discriminant analysis (LDA) score cutoff of 2. Multivariate linear regression was used to identify statistically significant associations between microbial features and maternal PPD symptoms. The analyses were adjusted for mode of delivery (including original intervention for CS) and feeding type at 42 days. The discriminative fecal metabolites between the two groups were identified using the Wilcoxon rank-sum test. Pathway enrichment analysis was carried out based on the Kyoto Encyclopedia of Genes and Genomes (KEGG) Pathway Database (http://www.genome.jp/kegg/) and MetaboAnalyst database (http://www.metaboanalyst.ca/).
A co-occurrence network, comprising discriminative fecal genera, metabolites, and neurodevelopmental domain scores, was constructed using Spearman’s correlation analysis and visualized in Cytoscape (Version 3.2.1, http://www.cytoscape.org). Causal mediation analysis was used to test whether maternal PPD symptoms at 42 days postpartum influenced infant neurodevelopment at 6 months through intermediate gut microbiota or metabolites (22) with the above mentioned confounders adjusted. P-values were adjusted based on the Benjamini–Hochberg false discovery rate (FDR) method, with the significance level and FDR threshold set at 0.05 and 0.25, respectively. All analyses were performed using R version 4.2.1.
Results
Maternal and infant characteristics
A total of 101 mothers who met the inclusion criteria were enrolled in the study, and all of them completed the depression assessment online through a web-based platform using the EPDS at 42 days postpartum. Among them, 4 mother-infant dyads were temporarily residing outside our local area, leaving 97 infant fecal samples being were collected at 42 days after birth. However, within the first 6 months, a total of 15 infants were lost to follow-up, leaving 86 infants available for neurodevelopmental evaluation using ASQ-3 (Figure 1).
A total of 41 mothers experienced PPD at 42 days after delivery, based on a cutoff score of 11 on the EPDS. Of these, 40 mothers contributed infant fecal samples for subsequent gut microbiota analysis. The demographic and clinical characteristics of the mothers and infants are presented in Table 1. The participants were divided into two groups based on their EPDS scores at 42 days after delivery (PPD group vs. non-PPD group), representing mothers with and without postpartum depression. The age range of mothers was 19 to 42 years old, with the majority being multiparous women. No infants were received antibiotic within the first 42 days of life due to physical illness. No significant differences were observed in occupation, education, income, pre-pregnancy BMI, gestational age, mode of delivery, and feeding types between the two groups. A comparable number of infants in both the non-PPD (24 infants) and PPD (8 infants) groups had received maternal vaginal microbiota transplantation at birth as part of our previous RCT (11). At the 6-month follow-up, 34 infants in the PPD group and 52 in the non-PPD group completed the neurodevelopment assessment using ASQ-3.
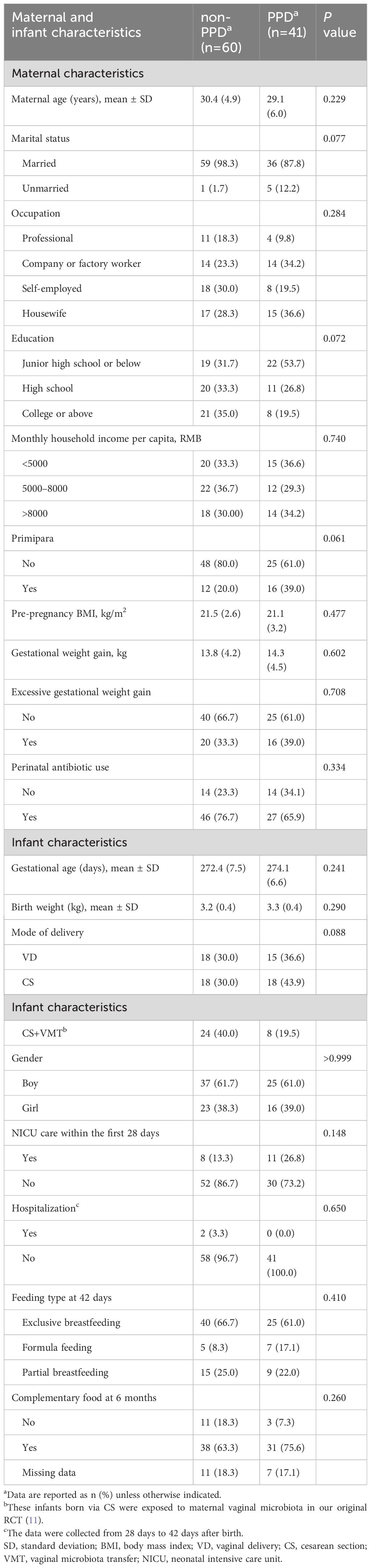
Table 1 Maternal and infant demographic and clinical characteristics between the non-PPD and PPD groups.
Association between maternal PPD symptoms and infant neurodevelopment at 6 months
The linear regression results showed that infants in the PPD group had significantly lower scores in ASQ-3 total score (MD = -21.2; 95% CI = -36.6 to -5.9; P=0.007), fine motor (MD = -4.2; 95% CI = -7.4 to -0.9; P=0.012), problem-solving (MD = -7.5; 95% CI = -11.5 to -3.4; P<0.001) and personal-social domains (MD = -6.2; 95% CI = -11.6 to -0.7; P=0.028). These differences remained statistically significant in multiple linear regression models after adjusting for confounders, including maternal pre-pregnancy BMI, gestational age, mode of delivery (including original CS intervention factor), NICU care of infants within 42 days of birth, and introduction of complementary food at 6 months after birth (Figure 2). After imputing the missing data with random forest methods, the significant results of the multiple regression model remained.
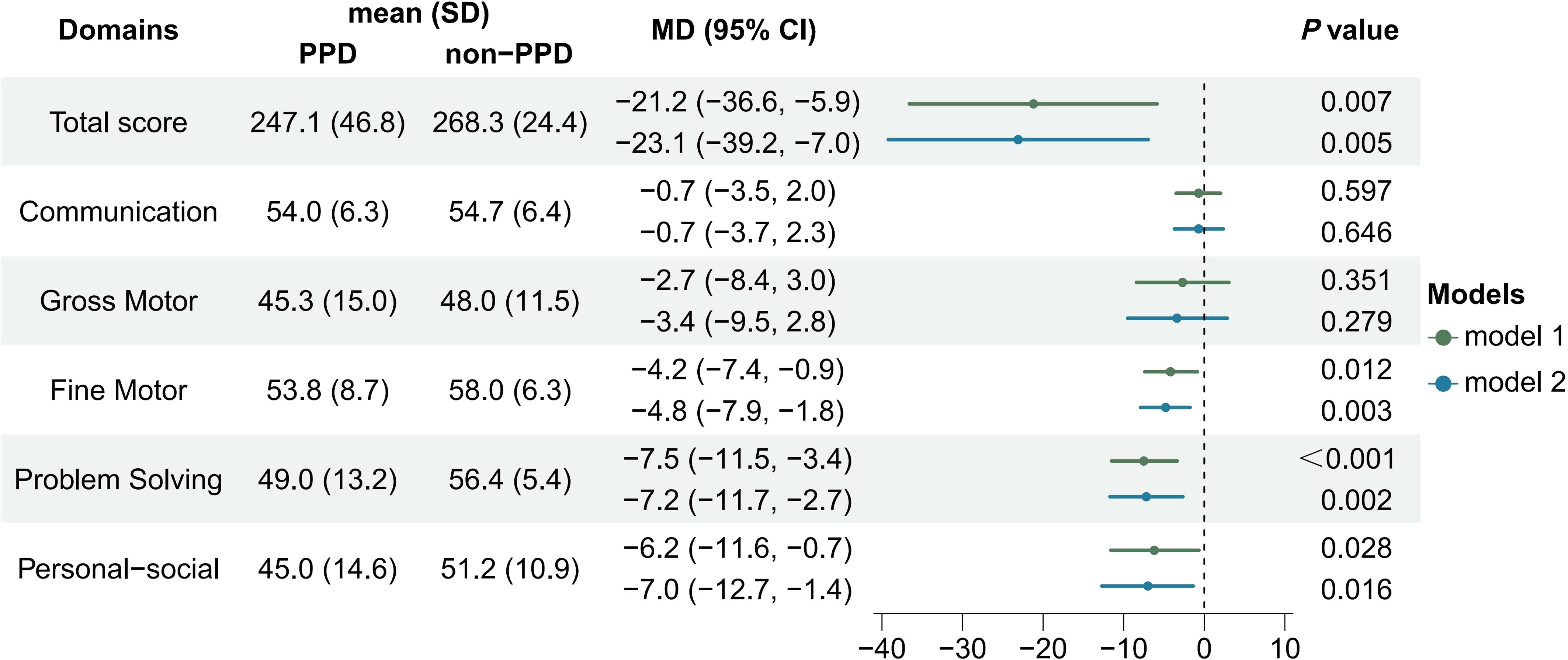
Figure 2 Comparison of infant neurodevelopment between PPD and non-PPD groups based on ASQ-3 scores at 6 months after birth. [Model 1 was the crude model, model 2 was adjusted for pre-pregnancy BMI, gestational age, NICU care, mode of delivery (intervention factor included), and introduction of complementary food at 6 months.].
Association between maternal PPD symptoms and infant gut microbiota
We examined the associations between infant gut microbiota and maternal PPD symptoms using infant stool samples collected at 42 days after birth. Our findings revealed that infants exposed to maternal PPD symptoms had higher Shannon diversity than those in the non-PPD group at 42 days (P=0.013, Figure 3A); the maternal EPDS score at day 42 was also positively associated with the Shannon diversity index (r=0.22, P=0.032). However, the overall microbial community composition did not significantly differ between the PPD and non-PPD groups (PERMANOVA test, P=0.332). The predominant phyla across all samples were Proteobacteria, Firmicutes, Actinobacteria, and Bacteroidetes. At the genera level, 14 dominant genera, constituting over 90% of the total microbial abundance, were listed (Figure 3B). Among these, Escherichia, Bifidobacterium, and Klebsiella were the three most abundant genera in infants. A total of 10 taxa showed significant changes in abundance with the criteria LDA>2.0 and P<0.05 (Figure 3C). Notably, the abundance of genera Bifidobacterium, Dialister, Cronobacter and Megasphaera was higher in the non-PPD group, while Veillonella and Finegoldia were enriched in the PPD group. When performing multiple linear regression after adjusting for mode of delivery (including original CS intervention factor) and feeding type at day 42 to identify differential abundant taxa between groups, we observed that Dialister (P=0.047) and Blautia (P=0.038) were significantly enriched in infants exposed to maternal PPD symptoms, while Bifidobacterium (P=0.048) was significantly depleted.
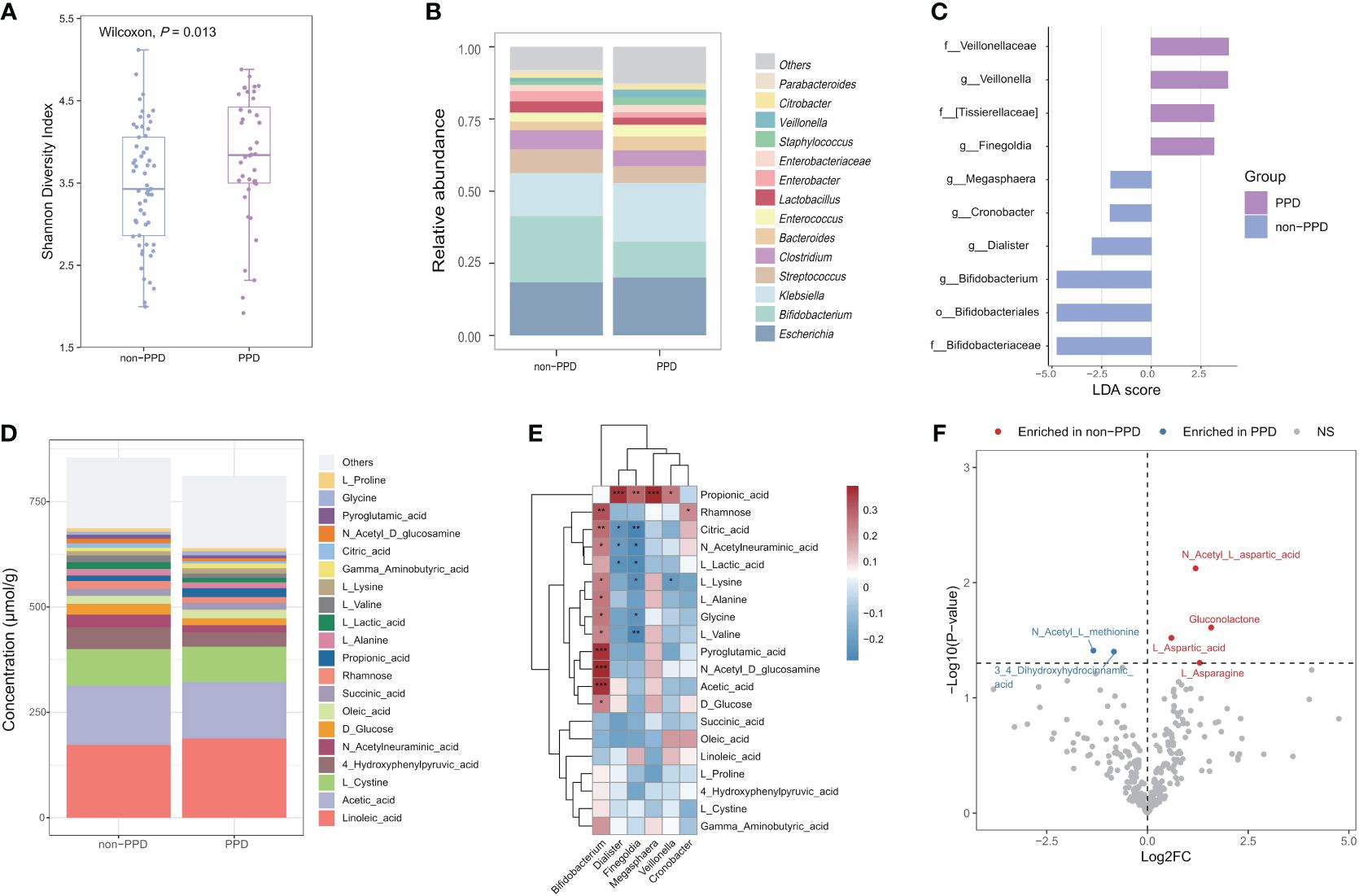
Figure 3 Associations between maternal PPD symptoms at 42 days and infant gut microbiota and metabolome at 42 days after birth. (A) Comparison of Shannon diversity index between infants in the PPD and non-PPD groups at 42 days using Wilcoxon test. (B) Relative abundances of the 14 predominant genera between groups. Dissimilarities in gut microbiota composition at genus level between PPD and non-PPD groups at 42 days. (C) LEfSe (LDA>2) illustrating taxa that are significantly different in infants between PPD and non-PPD groups. (D) Metabolite composition of infants in non-PPD and PPD groups. The metabolites with the top 20 concentrations are shown. (E) Heatmap of associations between differentially abundant genera and the top 20 concentrations metabolites (Spearman’s rank correlation analysis). *** P<0.001, **P<0.01, *P<0.05 (FDR<0.25). (F) Volcano chart of fold changes in metabolites in stool samples of infants in the PPD and non-PPD groups. The dotted line represents P = 0.05. Red dots show enriched metabolites in non-PPD group, while blue dots show opposite. Notable metabolites are labeled.
Association between maternal PPD symptoms and infant gut metabolites
Next, we performed targeted metabolomic profiling of fecal samples from infants in the non-PPD and PPD groups by using liquid chromatograph-mass spectrometry (LC–MS). Infants in the non-PPD group harbored a number of richer and higher-concentration metabolites in stool (Figure 3D). The top 3 metabolites in infants at 42 days were Linoleic acid, Acetic acid and L-Cystine. Next, we focused on the correlations between differential microbial features and the top 20 metabolites (Figure 3E). Impressively, positive correlations were identified between the abundance of Bifidobacterium and the majority of fecal metabolites, whereas the abundance of Finegoldia exhibited negative correlations. By using the Wilcoxon test, six discriminative metabolites between PPD and non-PPD groups were identified (Figure 3F). Specifically, compared to those in the non-PPD group, the PPD group displayed enrichment of 2 metabolites, 3,4-Dihydroxyhydrocinnamic acid and N-Acetyl-L-methionine, and depletion of 4 metabolites, including N-Acetyl-L-aspartic acid, Gluconolactone, L-Aspartic acid, L-Asparagine. Moreover, pathway-based differential abundance analysis highlighted that the metabolic pathway of Alanine, aspartate and glutamate metabolism was significantly downregulated in the PPD group.
Association of infant gut microbiota and gut metabolites with neurodevelopment at 6 months of age
To explore the potential relevance between bacterial composition and metabolomic phenotypes, we calculated Spearman’s correlation matrices and constructed a co-occurrence network of differential microbial genera, metabolites and neurodevelopment (Figure 4A). Co-occurrence network analysis revealed a total of 36 co-occurrence relationships, with 16 connections found in microbiota-metabolite. Of these, 5 were negative, with Finegoldia and 3,4-Dihydroxyhydrocinnamic acid being the major contributors to this finding, which were also enriched in the PPD group. Interestingly, Bifidobacterium had positive relationships with Gluconolactone and L-Asparagine, while Finegoldia showed opposite. When linking these differential genera and metabolites with infant neurodevelopment, the Bifidobacterium was the only taxa showing positive correlation with infant fine motor skill, while Finegoldia showed opposite. Moreover, the metabolites involved with Alanine, aspartate and glutamate metabolism were positively correlated with neurodevelopment. For example, L-Aspartic acid and L-Asparagine were positively associated with personal-social score, L-Aspartic acid and N-Acetyl-L-aspartic acid were positively associated with problem solving score, L-Aspartic acid was positively associated with fine motor.
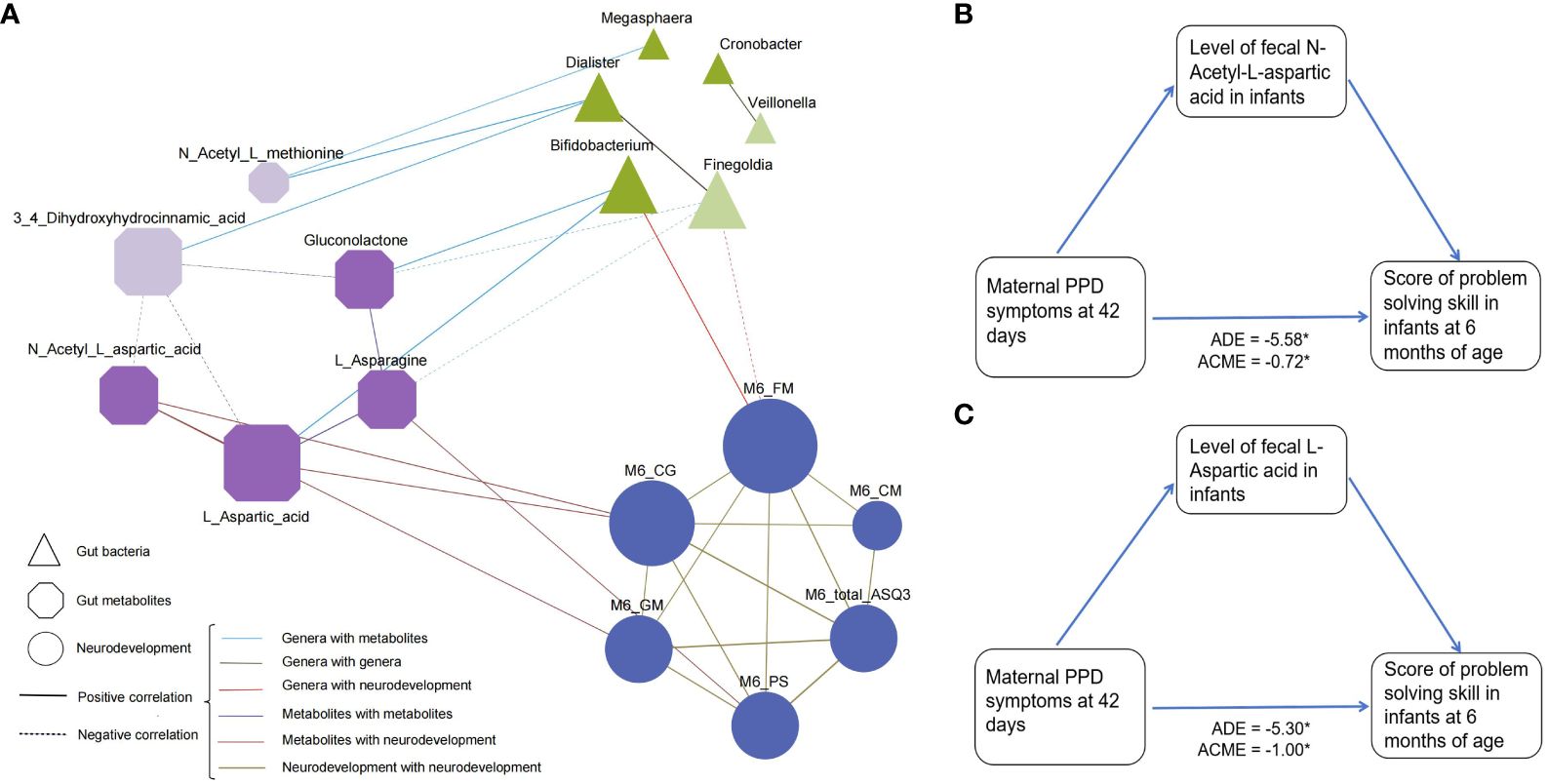
Figure 4 Association between differentially abundant genera, metabolites, and infant neurodevelopment at 6 months of age. (A) A co-occurrence network constructed from the relative abundances of differential microbial genus, fecal metabolites as well as neurodevelopment score in infants in PPD group and non-PPD group. The spearman correlation analysis was used to explore the co-occurrence network. The node size signifies its degree, representing the number of network connections. The shape of node denotes the components (ellipse: neurodevelopment domains, triangle: microbial genera, and cut-rectangle: metabolites). Deep and light colors indicate increased and decreased relative abundances in non-PPD compared to PPD, respectively. Solid and dashed edges represent positive and negative correlations, respectively. The colors and shapes of nodes indicate the categories of nodes. Edges thickness indicate the magnitude of correlation. FM, fine motor; GM, gross motor; CM, communication; CG, problem solving skills; PS, personal-social skills. (B, C) Mediation analysis assessing the role of (B) N-Acetyl-L-aspartic acid and (C) L-Aspartic acid in the association between maternal PPD symptoms and infant problem-solving skill scores. The model was adjusted for gestational age, mode of delivery, feeding type at day 42, NICU care, and maternal pre-pregnancy BMI.
Furthermore, to evaluate whether metabolites can mediate the microbial impact on the association between maternal PPD symptoms at day 42 postpartum and infant neurodevelopment, we performed mediation analyses using the above identified discriminative metabolites. Results indicated that the level of fecal N-Acetyl-L-aspartic acid and L-Aspartic acid mediated the relationships between maternal PPD symptoms at 42 days postpartum and infant problem-solving skill scores, with an effect size of -0.72 (95% CI = -1.59 to -0.11, Figure 4B) and -0.34 (95% CI = -2.42 to -0.08, Figure 4C), respectively.
Discussion
The prevalence of PPD in this study population was approximately 40%, which is higher than in other studies (1, 23, 24). Previous research has indicated that cesarean section delivery could elevate the risk of PPD (25, 26). In this study, there were twice as many women who delivered by cesarean section compared to those who delivered vaginally. Furthermore, our definition of PPD differs from those of other studies due to variations in the use of the EPDS tool and its cut-off points. Even so, we cannot ignore the global trend of increasing PPD incidence and its harm. With escalating rates of maternal perinatal depression, we face not only the risk of maternal mental disorders but also growing concerns about the potential long-term impacts on infant health and well-being. A particular emphasis in research on infant neurodevelopment has been on the early development of gut microbiota (27–29). It is therefore crucial to explore the associations among maternal PPD, infant gut microbiota, gut metabolites, and neurodevelopment. In this study, we found that maternal PPD symptoms at day 42 postpartum were negatively associated with infant neurodevelopment at 6 months of age. This association could be partially explained by alterations in the infant’s gut microbiota and gut metabolites.
Maternal psychiatric disorders and stress-induced disturbances in bacterial community structure have been confirmed in both human and preclinical animal studies (5, 30–32). Our data further indicated that maternal PPD symptoms were correlated with changes in the microbial composition of offspring within the first 6 weeks after birth. Infants born to mothers with depressive symptoms had higher alpha diversity in their gut microbiota and were characterized by a lower relative abundance of Bifidobacterium. These findings were consistent with a recent study reporting that both maternal prenatal and PPD could affect offspring gut microbiome diversity and Bifidobacterial abundances (9). However, the measurement of maternal PPD and the collection of infant fecal samples were conducted simultaneously in our study. Despite this synchrony, it is essential to recognize that the EPDS evaluates depressive symptoms over the past week, whereas PPD typically manifests within the first 30 days postpartum (2, 33). Consequently, it is conceivable that maternal alterations in mood and behavior may have already exerted an influence on the developing infant gut microbiota prior to the measurement at 42 days. Future studies with longitudinal designs are warranted to elucidate the causal relationships between maternal PPD and infant gut microbiota dynamics.
The mechanism by which early-life exposure to maternal PPD influences infant gut microbiota and neurodevelopment is still unclear. One significant factor is that exposure to maternal depression can affect the bacterial composition of breast milk at both the phyla and genera levels (34), an important observation considering that breast milk is a primary dietary and microbial source for the offspring. Moreover, PPD may affect infant immunity through breast milk. Changes in the offspring’s immune systems, with potential effects on antimicrobial peptide and antibody production and quantity, could lead to dysregulated shaping of the microbiome in early life. We previously observed lower levels of TGF-β in the colostrum of mothers with PPD (35), which is crucial for modulating infant inflammatory responses and promoting the development of the infant’s gastrointestinal tract (36, 37). A prior study also showed that maternal depressive symptoms were linked to reduced fecal IgA concentrations in infants (10). Further studies could focus on the vertical transmission of maternal and infant microbiota in mother–child dyads with PPD. Conversely, reduced mother–infant interaction could perturb the development of infant microbiota due to reduced horizontal transmission. Maternal depression has been shown to affect the skin microbiome in offspring (38). The elucidation of the pathway by which PPD affects the offspring’s microbiome is an ongoing and crucial issue, requiring further research in both humans and animals. In addition to factors related to mother–infant bacterial transmission, the Integrative Body-Mind-Spirit (IBMS) model potentially provides an explanation for this phenomenon. From the IBMS model, which emphasize the importance of achieving balance and harmony between the body, emotions, and spirit (39, 40), a mother’s emotional status could influence the emotional and spiritual status of her infant. For example, PPD affects a mother’s emotions and life attitude, thereby diminishing her caregiving capacity and impacting the mental and neural development of her infant. Therefore, promoting the well-being of postpartum women in from Body-Mind-Spirit perspective could help mitigate the effects of PPD on their offspring.
Early-life microbial colonization plays a key role in neuropsychological development (41). Our study revealed a positive correlation between the relative abundance of Bifidobacterium and infant neurodevelopment. Conversely, Finegoldia was found to be negatively associated with infant fine motor development. These findings align with prior studies (36, 42, 43). For example, alterations in gut microbial composition have been identified in children with autism (44). Additionally, sterile mice presented more pronounced short-term cognitive and working memory impairments compared to mice with normal gut microbiota (45). These results may be linked to the brain-gut-microbiome axis, where the gut microbiome collaborates with its host to regulate the development and function of the nervous system by producing and modifying various metabolic, immunological and neurochemical factors (46). Within our co-occurrence network analysis, we observed that Bifidobacterium and Finegoldia formed co-occurring relationships with fecal L-Aspartic acid and its derivative, N-Acetyl-L-aspartic acid. Bifidobacterium has the potential to elevate L-Aspartic acid levels by incorporating aspartate into its peptidoglycan structure, alongside its immunomodulatory effects on brain metabolism and the synthesis of related substances within the same pathway (47–49). However, the pathophysiological mechanism linking Finegoldia and L-Aspartic acid remains unknown and requires further research. These findings imply synergistic relationships between altered gut microbes and the host’s metabolism in infants with maternal PPD. N-Acetyl-L-aspartic acid is a contributor to energy production from the amino acid glutamate in neuronal mitochondria, and could function as a neurotransmitter in the brain through its interaction with metabotropic glutamate receptors (50). The levels of L-Aspartic acid and N-Acetyl-L-aspartic acid have been reported to decrease in various neuropathological conditions, such as brain injury, Alzheimer’s disease, and autism (51, 52). Higher level of N-Acetyl-L-aspartic acid in the hippocampus have been associated with enhanced working memory performance in humans (53). In our study, infants whose mother had PPD exhibited a reduction in Bifidobacterium and L-Aspartic acid, aligning with lower neurodevelopmental scores. It is therefore important to monitor and maintain an early gut microbiota balance in infants with mothers experiencing PPD. Based on these findings, animal experiments to further identify the key gut microbial strains and their functional metabolism associated with neurodevelopment would be of paramount importance and may lead to new therapeutic strategies for mothers with PPD and their infants.
To the best of our knowledge, this study is the first to demonstrate that maternal postpartum depression symptoms may impact offspring neurodevelopment by influencing the composition of infant fecal microbiota and metabolites. This impact includes an increase in alpha diversity and a reduction in the relative abundance of beneficial bacteria, such as Bifidobacterium, as well as a decrease in the neurotransmitter N-Acetyl aspartic acid. Notably, our mediation analysis provided causal evidence regarding the connection between changes in the level of N-Acetyl aspartic acid and the development of infant problem-solving skills (54–56). Nevertheless, our study has several limitations. First, we did not evaluate or clinically confirm maternal depression during pregnancy and other perinatal emotional disorders such as anxiety; instead, we relied on self-reported history of depression or psychiatric diseases before or during pregnancy to exclude women with these conditions. Different mood disorders can coexist or be interrelated, and previous emotional symptoms might be associated with the occurrence of PPD. Further research could be initiated, encompassing the period from pregnancy through the postnatal phase, with a focus on gathering diagnostic data. Second, this was a single-center study encompassing approximately 100 participants, whether and to what extent the results can be generalized to other populations require confirmation by future studies in diverse populations. Third, the persistence of early PPD symptoms and how the gut microbiota evolves with age remain unclear. Therefore, longitudinal studies with larger sample sizes, multicenter designs and measuring emotional disorders before, during, and after pregnancy up to 6 months are needed to draw more definitive causal conclusions. Finally, our study did not include an analysis of maternal samples such as stool, blood, and breast milk, limiting our ability to explore potential pathways through which maternal PPD might affect maternal inflammatory and microbiome profiles, and subsequently disrupt the offspring’s microbiome. Future studies incorporating both maternal and infant microbiome samples, along with assessment of mood and behavior in both cohorts, are essential to advance our understanding of maternal influence on the infant microbiome and health.
Conclusions
This study suggests that symptoms of maternal postpartum depression could affect infant neurodevelopment, potentially involving alterations in infant fecal aspartate metabolism. Future research focusing on mother–infant dyads over an extended period across diverse populations may reveal more direct relationships between postnatal maternal mental health and the offspring microbiome, providing further insights into how these alterations may affect offspring health outcomes.
Data availability statement
All sequencing data associated with this study have been made publicly available. The 16S rRNA bacterial profiling data generated in this study have been deposited in the NCBI database with accession number SRA: PRJNA945351 (https://www.ncbi.nlm.nih.gov/sra). All data supporting the findings of this study are available within the paper.
Ethics statement
The studies involving humans were approved by Ethics Committee of the Seventh Affiliated Hospital, Southern Medical University. The studies were conducted in accordance with the local legislation and institutional requirements. Written informed consent for participation in this study was provided by the participants’ legal guardians/next of kin.
Author contributions
LZ: Conceptualization, Data curation, Formal analysis, Methodology, Writing – original draft, Writing – review & editing. LT: Formal analysis, Writing–original draft, Writing – review & editing. CZ: Writing – review & editing, Formal analysis. SW: Writing – review & editing. DK: Writing – review & editing. RX: Conceptualization, Project administration, Resources, Supervision, Writing – review & editing.
Funding
The author(s) declare financial support was received for the research, authorship, and/or publication of this article. This study is in part supported by the Canadian Institute of Health Research (P14-175351, PJT-178049), the Clinical Research Startup Program of Southern Medical University by High-level University Construction Funding of Guangdong Provincial Department of Education (LC2019ZD019), the Top Talent Program of Affiliated Foshan Maternity & Child Healthcare Hospital (FY2022011), and Natural Science Foundation of Guangdong Province, China (2024A1515010343).
Acknowledgments
We thank all the participants for their time and efforts.
Conflict of interest
The authors declare that the research was conducted in the absence of any commercial or financial relationships that could be construed as a potential conflict of interest.
The reviewer ZH declared a shared affiliation with the authors SW, DK to the handling editor at the time of review.
Publisher’s note
All claims expressed in this article are solely those of the authors and do not necessarily represent those of their affiliated organizations, or those of the publisher, the editors and the reviewers. Any product that may be evaluated in this article, or claim that may be made by its manufacturer, is not guaranteed or endorsed by the publisher.
References
1. Wang Z, Liu J, Shuai H, Cai Z, Fu X, Liu Y, et al. Mapping global prevalence of depression among postpartum women. Transl Psychiatry. (2021) 11:543. doi: 10.1038/s41398–021-01663–6
2. Pearlstein T, Howard M, Salisbury A, Zlotnick C. Postpartum depression. Am J Obstet Gynecol. (2009) 200:357–64. doi: 10.1016/j.ajog.2008.11.033
3. Lubotzky-Gete S, Ornoy A, Grotto I, Calderon-Margalit R. Postpartum depression and infant development up to 24 months: A nationwide population-based study. J Affect Disord. (2021) 285:136–43. doi: 10.1016/j.jad.2021.02.042
4. Evans J, Melotti R, Heron J, Ramchandani P, Wiles N, Murray L, et al. The timing of maternal depressive symptoms and child cognitive development: a longitudinal study. J Child Psychol Psychiatry. (2012) 53:632–40. doi: 10.1111/j.1469-7610.2011.02513.x
5. Gur TL, Shay L, Palkar AV, Fisher S, Varaljay VA, Dowd S, et al. Prenatal stress affects placental cytokines and neurotrophins, commensal microbes, and anxiety-like behavior in adult female offspring. Brain Behav Immun. (2017) 64:50–8. doi: 10.1016/j.bbi.2016.12.021
6. Scarborough J, Mueller FS, Weber-Stadlbauer U, Mattei D, Opitz L, Cattaneo A, et al. A novel murine model to study the impact of maternal depression and antidepressant treatment on biobehavioral functions in the offspring. Mol Psychiatry. (2021) 26:6756–72. doi: 10.1038/s41380–021-01145–7
7. Carlson AL, Xia K, Azcarate-Peril MA, Goldman BD, Ahn M, Styner MA, et al. Infant gut microbiome associated with cognitive development. Biol Psychiatry. (2018) 83:148–59. doi: 10.1016/j.biopsych.2017.06.021
8. Christian LM, Galley JD, Hade EM, Schoppe-Sullivan S, Kamp Dush C, Bailey MT. Gut microbiome composition is associated with temperament during early childhood. Brain Behav Immun. (2015) 45:118–27. doi: 10.1016/j.bbi.2014.10.018
9. Galley JD, Mashburn-Warren L, Blalock LC, Lauber CL, Carroll JE, Ross KM, et al. Maternal anxiety, depression and stress affects offspring gut microbiome diversity and bifidobacterial abundances. Brain Behav Immun. (2023) 107:253–64. doi: 10.1016/j.bbi.2022.10.005
10. Kang LJ, Koleva PT, Field CJ, Giesbrecht GF, Wine E, Becker AB, et al. Maternal depressive symptoms linked to reduced fecal Immunoglobulin A concentrations in infants. Brain Behavior Immun. (2018) 68:123–31. doi: 10.1016/j.bbi.2017.10.007
11. Zhou L, Qiu W, Wang J, Zhao A, Zhou C, Sun T, et al. Effects of vaginal microbiota transfer on the neurodevelopment and microbiome of cesarean-born infants: A blinded randomized controlled trial. Cell Host Microbe. (2023) 31(7):1232–47.e5. doi: 10.1016/j.chom.2023.05.022
12. Squires J, Bricker DD, Twombly E. Ages & Stages questionnaires, third edition. (ASQ-3) Baltimore: Paul H Brookes. (2009). doi: 10.1037/t11523-000
13. Cox JL, Holden JM, Sagovsky R. Detection of postnatal depression. Development of the 10-item Edinburgh Postnatal Depression Scale. Br J Psychiatry. (1987) 150:782–6. doi: 10.1192/bjp.150.6.782
14. Lee DT, Yip SK, Chiu HF, Leung TY, Chan KP, Chau IO, et al. Detecting postnatal depression in Chinese women. Validation of the Chinese version of the Edinburgh Postnatal Depression Scale. Br J Psychiatry. (1998) 172:433–7. doi: 10.1192/bjp.172.5.433
15. Levis B, Negeri Z, Sun Y, Benedetti A, Thombs BD, He C, et al. Accuracy of the Edinburgh Postnatal Depression Scale (EPDS) for screening to detect major depression among pregnant and postpartum women: systematic review and meta-analysis of individual participant data. BMJ. (2020) 371:m4022. doi: 10.1136/bmj.m4022
16. Widen EM, Kahn LG, Cirillo P, Cohn B, Kezios KL, Factor-Litvak P. Prepregnancy overweight and obesity are associated with impaired child neurodevelopment. Matern Child Nutr. (2018) 14:e12481. doi: 10.1111/mcn.12481
17. Gale-Grant O, Fenn-Moltu S, França LGS, Dimitrova R, Christiaens D, Cordero-Grande L, et al. Effects of gestational age at birth on perinatal structural brain development in healthy term-born babies. Hum Brain Mapp. (2022) 43:1577–89. doi: 10.1002/hbm.25743
18. Sun L, Wang S, Li X-Q. Association between mode of delivery and postpartum depression: A systematic review and network meta-analysis. Aust N Z J Psychiatry. (2021) 55:588–601. doi: 10.1177/0004867420954284
19. Zhang X, Spear E, Hsu H-HL, Gennings C, Stroustrup A. NICU-based stress response and preterm infant neurobehavior: exploring the critical windows for exposure. Pediatr Res. (2022) 92:1470–8. doi: 10.1038/s41390–022-01983–3
20. Xiang X, Yang T, Chen J, Chen L, Dai Y, Zhang J, et al. Association of feeding patterns in infancy with later autism symptoms and neurodevelopment: a national multicentre survey. BMC Psychiatry. (2023) 23:174. doi: 10.1186/s12888–023-04667–2
21. Oksanen J, Simpson GL, Blanchet FG, Kindt R, Legendre P, Minchin PR, et al. vegan: Community Ecology Package . Available online at: https://CRAN.R-project.org/package=vegan (Accessed March 18, 2023).
22. Tingley D, Yamamoto T, Hirose K, Keele L, Imai K. mediation: R package for causal mediation analysis. J Stat Softw. (2014) 59:1–38. doi: 10.18637/jss.v059.i05
23. Liu Y, Zhang L, Guo N, Jiang H. Postpartum depression and postpartum post-traumatic stress disorder: prevalence and associated factors. BMC Psychiatry. (2021) 21:487. doi: 10.1186/s12888–021-03432–7
24. Liu Y, Guo N, Li T, Zhuang W, Jiang H. Prevalence and associated factors of postpartum anxiety and depression symptoms among women in Shanghai, China. J Affect Disord. (2020) 274:848–56. doi: 10.1016/j.jad.2020.05.028
25. Xie R, Lei J, Wang S, Xie H, Walker M, Wen SW. Cesarean section and postpartum depression in a cohort of Chinese women with a high cesarean delivery rate. J Womens Health (Larchmt). (2011) 20:1881–6. doi: 10.1089/jwh.2011.2842
26. Xu H, Ding Y, Ma Y, Xin X, Zhang D. Cesarean section and risk of postpartum depression: A meta-analysis. J Psychosom Res. (2017) 97:118–26. doi: 10.1016/j.jpsychores.2017.04.016
27. Sherwin E, Bordenstein SR, Quinn JL, Dinan TG, Cryan JF. Microbiota and the social brain. Science. (2019) 366:eaar2016. doi: 10.1126/science.aar2016
28. Sharon G, Sampson TR, Geschwind DH, Mazmanian SK. The central nervous system and the gut microbiome. Cell. (2016) 167:915–32. doi: 10.1016/j.cell.2016.10.027
29. Wang Q, Yang Q, Liu X. The microbiota-gut-brain axis and neurodevelopmental disorders. Protein Cell. (2023) 14:762–75. doi: 10.1093/procel/pwad026
30. Dawson SL, O’Hely M, Jacka FN, Ponsonby A-L, Symeonides C, Loughman A, et al. Maternal prenatal gut microbiota composition predicts child behaviour. EBioMedicine. (2021) 68:103400. doi: 10.1016/j.ebiom.2021.103400
31. Kelly JR, Borre Y, O’ Brien C, Patterson E, El Aidy S, Deane J, et al. Transferring the blues: Depression-associated gut microbiota induces neurobehavioural changes in the rat. J Psychiatr Res. (2016) 82:109–18. doi: 10.1016/j.jpsychires.2016.07.019
32. Jašarević E, Howerton CL, Howard CD, Bale TL. Alterations in the vaginal microbiome by maternal stress are associated with metabolic reprogramming of the offspring gut and brain. Endocrinology. (2015) 156:3265–76. doi: 10.1210/en.2015–1177
33. Battle DE. Diagnostic and statistical manual of mental disorders: DSM-5TM. 5th ed. Arlington, VA, US: American Psychiatric Publishing, Inc (2013). p. xliv, 947. doi: 10.1176/appi.books.9780890425596
34. Browne PD, Aparicio M, Alba C, Hechler C, Beijers R, Rodríguez JM, et al. Human milk microbiome and maternal postnatal psychosocial distress. Front Microbiol. (2019) 10:2333. doi: 10.3389/fmicb.2019.02333
35. Xiong Z, Zhou L, Chen J, Li M, Xie R. Association between postpartum depression and concentrations of transforming growth factor-β in human colostrum: a nested cohort study. Nan Fang Yi Ke Da Xue Xue Bao. (2022) 42:1426–30. doi: 10.12122/j.issn.1673–4254.2022.09.21
36. Maheshwari A, Kelly DR, Nicola T, Ambalavanan N, Jain SK, Murphy-Ullrich J, et al. TGF-β2 suppresses macrophage cytokine production and mucosal inflammatory responses in the developing intestine. Gastroenterology. (2011) 140:242–53. doi: 10.1053/j.gastro.2010.09.043
37. Oddy WH, Rosales F. A systematic review of the importance of milk TGF-beta on immunological outcomes in the infant and young child. Pediatr Allergy Immunol. (2010) 21:47–59. doi: 10.1111/j.1399-3038.2009.00913.x
38. Mutic AD, Jordan S, Edwards SM, Ferranti EP, Thul TA, Yang I. The postpartum maternal and newborn microbiomes. MCN Am J Matern Child Nurs. (2017) 42:326–31. doi: 10.1097/NMC.0000000000000374
39. Chan C, Ho PS, Chow E. A body-mind-spirit model in health: an Eastern approach. Soc Work Health Care. (2001) 34:261–82. doi: 10.1300/j010v34n03_02
40. Fung YL, Lau BHP, Tam MYJ, Xie Q, Chan CLW, Chan CHY. Protocol for psychosocial interventions based on Integrative Body-Mind-Spirit (IBMS) model for children with eczema and their parent caregivers. J Evid Based Soc Work. (2019) 16:36–53. doi: 10.1080/23761407.2018.1545618
41. Acuña I, Cerdó T, Ruiz A, Torres-Espínola FJ, López-Moreno A, Aguilera M, et al. Infant gut microbiota associated with fine motor skills. Nutrients. (2021) 13:1673. doi: 10.3390/nu13051673
42. Chen X, Yan Z, Liu L, Zhang R, Zhang X, Peng C, et al. Characteristics of gut microbiota of term small gestational age infants within 1 week and their relationship with neurodevelopment at 6 months. Front Microbiol. (2022) 13:912968. doi: 10.3389/fmicb.2022.912968
43. Aatsinki A-K, Lahti L, Uusitupa H-M, Munukka E, Keskitalo A, Nolvi S, et al. Gut microbiota composition is associated with temperament traits in infants. Brain Behav Immun. (2019) 80:849–58. doi: 10.1016/j.bbi.2019.05.035
44. Dan Z, Mao X, Liu Q, Guo M, Zhuang Y, Liu Z, et al. Altered gut microbial profile is associated with abnormal metabolism activity of Autism Spectrum Disorder. Gut Microbes. (2020) 11:1246–67. doi: 10.1080/19490976.2020.1747329
45. Gareau MG, Wine E, Rodrigues DM, Cho JH, Whary MT, Philpott DJ, et al. Bacterial infection causes stress-induced memory dysfunction in mice. Gut. (2011) 60:307–17. doi: 10.1136/gut.2009.202515
46. Jian C, Carpén N, Helve O, de Vos WM, Korpela K, Salonen A. Early-life gut microbiota and its connection to metabolic health in children: Perspective on ecological drivers and need for quantitative approach. EBioMedicine. (2021) 69:103475. doi: 10.1016/j.ebiom.2021.103475
47. Yamashita T, Ashiuchi M, Ohnishi K, Kato S, Nagata S, Misono H. Molecular identification of monomeric aspartate racemase from Bifidobacterium bifidum. Eur J Biochem. (2004) 271:4798–803. doi: 10.1111/j.1432-1033.2004.04445.x
48. Laue HE, Karagas MR, Coker MO, Bellinger DC, Baker ER, Korrick SA, et al. Sex-specific relationships of the infant microbiome and early-childhood behavioral outcomes. Pediatr Res. (2022) 92:580–91. doi: 10.1038/s41390-021-01785-z
49. Naspolini NF, Schüroff PA, Figueiredo MJ, Sbardellotto GE, Ferreira FR, Fatori D, et al. The gut microbiome in the first one thousand days of neurodevelopment: A systematic review from the microbiome perspective. Microorganisms. (2024) 12:424. doi: 10.3390/microorganisms12030424
50. Yan H-D, Ishihara K, Serikawa T, Sasa M. Activation by N-acetyl-L-aspartate of acutely dissociated hippocampal neurons in rats via metabotropic glutamate receptors. Epilepsia. (2003) 44:1153–9. doi: 10.1046/j.1528-1157.2003.49402.x
51. Saleem TH, Shehata GA, Toghan R, Sakhr HM, Bakri AH, Desoky T, et al. Assessments of amino acids, ammonia and oxidative stress among cohort of Egyptian autistic children: correlations with electroencephalogram and disease severity. Neuropsychiatr Dis Treat. (2020) 16:11–24. doi: 10.2147/NDT.S233105
52. Yan Z, Li R, Shi W, Yao L. Role of the gut-microbiota-metabolite axis in the rotenone model of early-stage Parkinson’s Disease. Metab Brain Dis. (2022) 37:2511–20. doi: 10.1007/s11011–022-01004–6
53. Bertolino A, Sciota D, Brudaglio F, Altamura M, Blasi G, Bellomo A, et al. Working memory deficits and levels of N-acetylaspartate in patients with schizophreniform disorder. Am J Psychiatry. (2003) 160:483–9. doi: 10.1176/appi.ajp.160.3.483
54. Kato T, Nishina M, Matsushita K, Hori E, Mito T, Takashima S. Neuronal maturation and N-acetyl-L-aspartic acid development in human fetal and child brains. Brain Dev. (1997) 19:131–3. doi: 10.1016/s0387–7604(96)00496–2
55. Moffett JR, Ross B, Arun P, Madhavarao CN, Namboodiri AMA. N-Acetylaspartate in the CNS: from neurodiagnostics to neurobiology. Prog Neurobiol. (2007) 81:89–131. doi: 10.1016/j.pneurobio.2006.12.003
Keywords: postpartum depression, neurodevelopment, gut microbiome, gut metabolome, gut-brain axis
Citation: Zhou L, Tang L, Zhou C, Wen SW, Krewski D and Xie R-h (2024) Association of maternal postpartum depression symptoms with infant neurodevelopment and gut microbiota. Front. Psychiatry 15:1385229. doi: 10.3389/fpsyt.2024.1385229
Received: 12 February 2024; Accepted: 06 May 2024;
Published: 21 May 2024.
Edited by:
Laura Orsolini, Marche Polytechnic University, ItalyReviewed by:
Jun Lei, Central South University, ChinaZhiyong Hong, University of Ottawa, Canada
Silvia Bellagamba, Marche Polytechnic University, Italy
Copyright © 2024 Zhou, Tang, Zhou, Wen, Krewski and Xie. This is an open-access article distributed under the terms of the Creative Commons Attribution License (CC BY). The use, distribution or reproduction in other forums is permitted, provided the original author(s) and the copyright owner(s) are credited and that the original publication in this journal is cited, in accordance with accepted academic practice. No use, distribution or reproduction is permitted which does not comply with these terms.
*Correspondence: Ri-hua Xie, eGllcmlodWE5MjhAMTYzLmNvbQ==
†These authors have contributed equally to this work and share first authorship