- 1Department of Neonatology, The Second Affiliated Hospital and Yuying Children's Hospital of Wenzhou Medical University, Wenzhou, China
- 2Department of Pediatric Neurology, The Second Affiliated Hospital and Yuying Children's Hospital of Wenzhou Medical University, Wenzhou, China
Objective: While low-dose lamotrigine has shown effectiveness in managing paroxysmal kinesigenic dyskinesia (PKD) in pediatric populations, the cognitive consequences of extended use are yet to be fully elucidated. This study seeks to assess the evolution of cognitive functions and the amelioration of attention deficit and hyperactivity disorder (ADHD) symptoms following a two-year lamotrigine treatment in children.
Methods: This investigation employed an open-label, uncontrolled trial design. Between January 2008 and December 2021, thirty-one participants, ranging in age from 6.5 to 14.1 years, were enrolled upon receiving a new diagnosis of PKD, as defined by the clinical diagnostic criteria set by Bruno in 2004. Comprehensive evaluation of PRRT2 variants and 16p11.2 microdeletion was achieved using whole-exome sequencing (WES) and bioinformatics analysis of copy number variant (CNV) for all subjects. Immediately after diagnosis, participants commenced treatment with low-dose lamotrigine. Cognitive function was assessed using the Wechsler Intelligence Scale for Children-Chinese Revised (WISC-CR) at baseline and after 2 years, with ADHD diagnoses and symptom severity simultaneously assessed by experts in accordance with the DSM-IV diagnostic criteria for ADHD and the ADHD Rating Scale-IV (ADHD-RS-IV).
Results: Initially, twelve out of 31 patients (38.7%) presented with comorbid ADHD. The latency to treatment initiation was notably longer in PKD patients with ADHD (30.75 ± 12.88 months) than in those without ADHD (11.66 ± 9.08 months), t = 4.856, p<0.001. Notably, patients with a latency exceeding 2 years exhibited a heightened risk for comorbid ADHD (OR = 4.671, P=0.015) in comparison to those with shorter latency. Out of the cohort, twenty-five patients saw the clinical trial to its completion. These individuals demonstrated a marked elevation in WISC-CR scores at the 2-year mark relative to the outset across FSIQ (baseline mean: 108.72 ± 10.45 vs 24 months: 110.56 ± 10.03, p=0.001), VIQ (baseline mean: 109.44 ± 11.15 vs 24 months: 110.80 ± 10.44, p=0.028), and PIQ domains (baseline mean: 106.52 ± 9.74 vs 24 months: 108.24 ± 9.38, p=0.012). Concurrently, a substantial mitigation was observed in ADHD inattention at 2 years compared to baseline (p<0.001), with an average total subscale scores decrement from 9.04 ± 4.99 to 6.24 ± 4.05.
Conclusion: Prolonged duration of untreated PKD in children may elevate the risk of ADHD comorbidity. Notably, following a 2-year lamotrigine regimen, enhancements were observed in both cognitive test outcomes and ADHD symptomatology.
1 Introduction
Paroxysmal kinesigenic dyskinesia (PKD; MIM: 128200) falls within a spectrum of movement disorders. It is typified by sporadic episodes of involuntary movements triggered by sudden voluntary actions-especially those involving alterations in speed and amplitude, such as abrupt running or rising from a seated position. Attack frequencies can reach over 100 times daily, with the majority lasting under a minute (1, 2). The proline-rich transmembrane protein 2 (PRRT2) gene emerges as a significant causative factor in PKD, accounting for 77%-93% of familial PKD cases (3). While certain medications effectively manage these episodes, discontinuation frequently results in relapse. Contemporary studies have highlighted potential intellectual and behavioral comorbidities in PKD patients, encompassing developmental delay, intellectual disability, attention deficit hyperactivity disorder (ADHD), anxiety and depression (4–7). However, the evidence remains constrained by small study cohorts and based on PRRT2 gene mutation.
As a commonly used anti-epileptic drug (AED) that appears to be associated with fewer cognitive and behavioral changes than are many other AEDs (8). Existing data suggest that the cognitive deficits are rarely observed in patients receiving Lamotrigine (LTG) monotherapy (9). LTG is as well endorsed as a second-line recommended medication for PKD treatment as per guidelines, yet its influence on cognitive function development in affected children remains understudied. Notably, multiple studies involving epilepsy patients on lamotrigine have indicated an absence of clinically significant cognitive side effects, and even demonstrated enhancements in quality of life (10–12). However, this may be attributed to lamotrigine’s potential in suppressing epileptiform EEG discharges, which could subsequently lead to positive alterations in cognitive function. This study aims to address the critical gap in knowledge by focusing on the effects of long-term low-dose lamotrigine treatment on cognitive functions, specifically ADHD and intelligence, in children with PKD.
2 Methods
2.1 Study design
This was an open-label, uncontrolled trial in which all PKD participants underwent video-EEG and genetic testing. Evaluations of cognitive function and ADHD symptoms were conducted pre-lamotrigine treatment (baseline) and after 2 years on treatment. The trial protocol encompassed clinical visits for initial screening, baseline assessment, followed by Visits 1-6 at intervals of 1, 2, 6, 12, 18, and 24 months for monitoring.
2.2 Patient consent and enrolment
This study was conducted at Department of Pediatric Neurology, The Second Affiliated Hospital and Yuying Children’s Hospital of Wenzhou Medical University. The research adhered to the latest version of the Declaration of Helsinki and secured approval from the institution’s Ethical Committee (2021-K-166-02). Prior to enrollment, written informed consent was procured from the patients or their guardians. The study is registered with the Chinese Clinical Trial Registry (ChiCTR-OPC-15006294).
From January 2008 to December 2021, we enrolled 31 participants, aged between 6.5 and 14.1 years, with newly diagnosed PKD and no biological relationships. All patients were of Han Chinese descent and hailed from Wenzhou city. The inclusion criteria were as follows: 1) No administration of anticonvulsant or ADHD drugs within the past year; 2) Diagnosis of PKD based on Bruno’s 2004 clinical diagnostic criteria (13): (a) Presence of a kinesigenic trigger for the attacks; (b) Short duration of attacks (<1 min); (c) No loss of consciousness or pain during attacks; (d) Exclusion of other organic diseases with normal neurologic examination results; (e) Onset age between 1 and 20 years, in the absence of a family history of PKD.
2.3 Treatment of lamotrigine
Upon diagnosis of PKD, all patients were recommended to commence standardized lamotrigine therapy promptly. The lamotrigine dosage was initiated with escalating doses, incrementally adjusted weekly until the attacks were managed. Once effective doses were attained, maintenance doses were administered, confirmed by two consecutive attack-free outpatient visits. Since the commonly used dose of lamotrigine for epilepsy is 1-4mg/kg.d, here, we defined maintenance doses less than 1.5mg/kg.d as low-dose.
2.4 Measurements
A video-EEG of over 4 hours was conducted prior to medication initiation. During this procedure, patients were instructed to fall asleep naturally. Upon awakening, patients were prompted to execute their individual trigger actions, such as abrupt movements, to induce an attack. Subsequently, Whole-exome sequencing (WES) and a bioinformatics analysis of copy number variant (CNV) were executed on all patients to analyze PRRT2 variants and the 16p11.2 microdeletion.
Patients’ cognitive abilities were assessed using the Wechsler Intelligence Scale for Children-Chinese Revised Edition (WISC-CR) at both baseline and after 2 years of treatment. The WISC is a recognized and extensively employed intelligence scale tailored for children. The WISC-CR is designed for Chinese children aged between 6 and 16 years, encompassing 12 domains: common sense, analogies, arithmetic, vocabulary, comprehension, digit span, missing picture completion, picture arrangement, block design, object collocation, decoding, and mazes. Scores for each patient were determined based on the operational manual and the respective patient’s age. The first six domains contribute to the verbal intelligence quotient (VIQ), whereas the latter six yield the performance intelligence quotient (PIQ). The cumulative results then allow calculation of the full-scale intelligence quotient (FSIQ) (14).
ADHD evaluations were conducted by investigators following the DSM-IV diagnostic criteria for ADHD at consistent intervals. According to the DSM-IV, ADHD can be categorized into three nominal subtypes based on a specific number of symptoms in two dimensions: inattention and hyperactivity-impulsivity (15). The Predominantly Inattentive Type (ADHD-I) is characterized by individuals exhibiting six or more symptoms of inattention but fewer than six of hyperactivity-impulsivity. The Predominantly Hyperactive-Impulsive Type (ADHD-H) pertains to individuals with six or more hyperactivity-impulsivity symptoms and fewer than six inattention symptoms. Meanwhile, the Combined Type (ADHD-C) encompasses individuals manifesting six or more symptoms across both dimensions (16).
Parent-reports on the ADHD-Rating Scale (ADHD-RS-IV) was administered at baseline and at 2years. It has 18 items covering the symptom criteria for ADHD as outlined in DSM-IV. Parents rated the frequency of each symptom within the past six months on a four-point Likert scale ranging from “Never or rarely” (0), “Sometimes” (1), “Often” (2) to “Very often” (3) with higher scores indicating greater degree of perceived ADHD symptomatology and severity in the child. According to the scale developers, nine items cover deficits in attention and can be summed to generate an Inattention subscale (range: 0–27), whereas the remaining nine items cover hyperactivity and impulsivity and can be summed to yield a Hyperactivity/impulsivity subscale (range: 0–27). A total score can be derived by summing all 18 items (range: 0–54) (17).
2.5 Data collection and analysis
Data were analyzed using SPSS software (version 23.0). Alterations in WISC-CR scores and ADHD symptom-items from baseline to the 2-year treatment mark were evaluated with the paired t-test. Group comparisons employed independent samples t-tests and the chi-square test, while simple correlations determined associations between variables. A p-value of < 0.05 was set as the threshold for statistical significance.
3 Results
Twenty-one males and 10 females were enrolled, with the mean age 9.6 ± 2.4 years old (range from 6.5 to 14.1 years old). Their mean age of attack onset was 8.0 ± 2.9 years old (range from 3.3 to 13.2 years old) and the mean period before drug administration was 1.6 ± 1.2 years (range from 3 months to 4.1 years).
Of the 31 participants, all exhibited normal results on video-EEGs as well as brain CT or MRI scans. Genetic testing revealed mutations in the PRRT2 gene in 21 patients (67.7%). Three distinct causative heterozygous variants were discerned: the c.649dupC variant (17/21), the c.291delC variant (2/21), and the c.649C>T variant (2/21). Of these, nineteen had a familial history of the disorder, with the criterion being the presence of at least one affected relative within three preceding generations, while 12 emerged as sporadic instances. A notable finding was that five patients had previously encountered afebrile infantile seizures. Parental accounts indicated that attack durations consistently remained under 30 seconds, with frequencies varying from fewer than once daily to in excess of 10 instances per day. In this cohort, 14 patients were sensitive to multiple triggers, while the remaining 17 patients had a singular trigger, namely, sudden movement. The three most prevalent triggers included sudden movement (31/31), intention to move (15/31), and stress (6/31).
Initially, the cognitive abilities of all 31 participants were assessed using WISC-CR. The group’s average FSIQ, VIQ, and PIQ scores were 106.6 ± 11.2, 107.6 ± 11.2, and 104.6 ± 10.5, respectively. None of the participants recorded a FSIQ score below 80. Using the DSM-IV diagnostic criteria, ADHD was diagnosed in 12 participants (38.7%). Meanwhile, ADHD symptoms severity of the participants were assessed according to ADHD-RS-IV. Demographic characteristics of the participants were presented in Table 1.
Comparing PKD patients with ADHD to those without, there were no significant differences in trigger causes (p=0.212), duration of attacks (p=0.475), frequency of attacks (p=0.565), PRRT2 variants (p=0.611), family history of paroxysmal dyskinesia (p=0.541), history of afebrile infantile seizure (p=0.342), age of onset (p=0.244), FSIQ score (0.654), VIQ score (0.749), and PIQ score (0.584). The only significant difference between the two groups was that PKD patients with ADHD comorbidity experienced a longer duration before medication initiation (30.75 ± 12.88 months) than those without comorbid ADHD (11.66 ± 9.08 months, t=4.856, p<0.001). Patients waiting over 2 years before initiating therapy exhibited a significantly higher risk for ADHD comorbidity (OR = 4.671, P=0.015) than those with a waiting period less than 2 years. Potential risk factors for ADHD comorbidity in PKD patients can be found in Table 2.
By the end of the fourth week of lamotrigine therapy, all patients reached an attack-free state. During the follow-up, six patients withdrew from the clinical trial, of whom five took medications for ADHD (cases 1, 2, 3, 14, and 19) and one patient experienced relapses due to poor compliance (case 17). The 25 patients who completed the study underwent reassessment of cognitive ability and ADHD symptoms at the two-year mark (Visit 6).
A notable increase in WISC-CR scores was observed in the cohort of 25 patients following two years of treatment compared to baseline, with significant improvements in FSIQ (mean at baseline 108.72 ± 10.45 vs. at two years 110.56 ± 10.03, p=0.001), VIQ (mean at baseline 109.44 ± 11.15 vs. at two years 110.80 ± 10.44, p=0.028), and PIQ (mean at baseline 106.52 ± 9.74 vs. at two years 108.24 ± 9.38, p=0.012). Concurrently, a pronounced improvement was observed in ADHD inattention symptoms (p<0.001), as reflected by a decline in the mean total subscale scores from 9.04 ± 4.99 to 6.24 ± 4.05. In contrast, ADHD symptoms related to hyperactivity-impulsivity remained statistically unaltered (p=0.328). Moreover, robust correlations were noted between baseline and two-year follow-up assessments in terms of both cognitive scores and ADHD symptoms, specifically in FSIQ (r=0.974, p<0.001), VIQ (r=0.966, p<0.001), PIQ (r=0.946, p<0.001), total inattention subscale scores (r=0.945, p<0.001), and total hyperactivity-impulsivity subscale scores (r=0.955, p<0.001), see Table 3. However, We found no statistical differences (all p>0.05) in our study between daily dosage per kilogram and improvements in WISC-CR scores or ADHD symptoms, see Table 4.
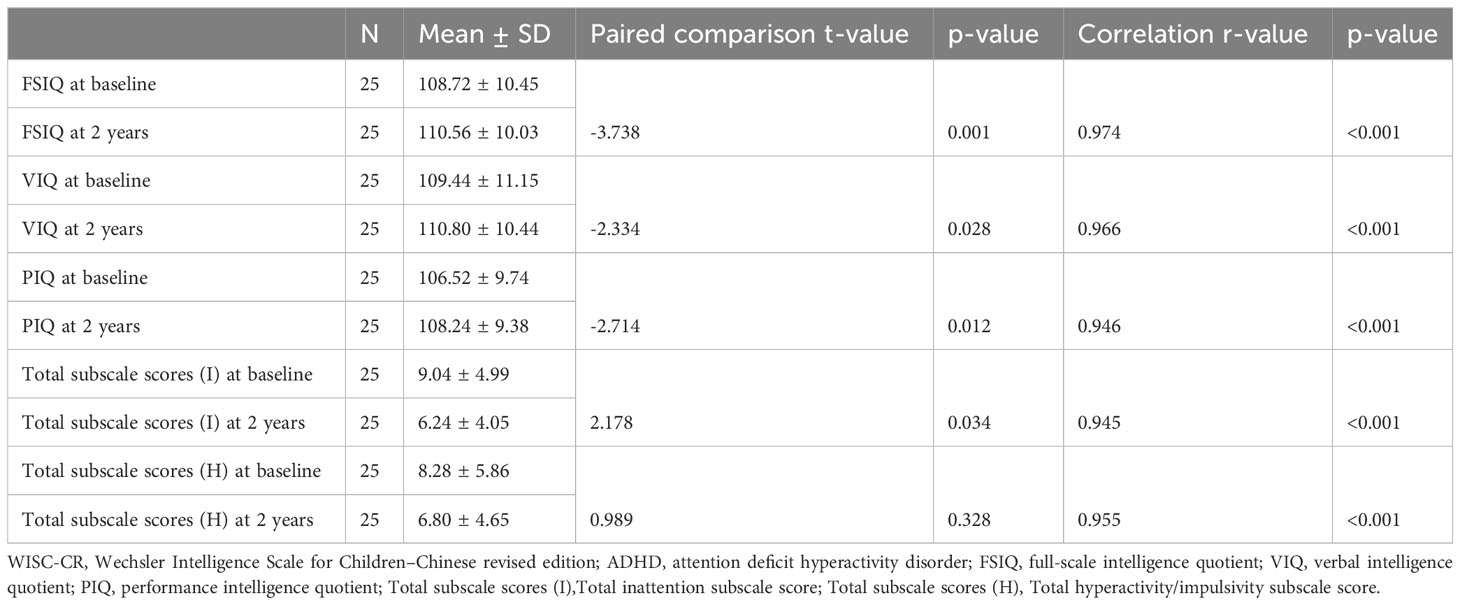
Table 3 Longitudinal Changes in WISC-CR Scores and ADHD Symptomatology Over a 2-Year Treatment Period.
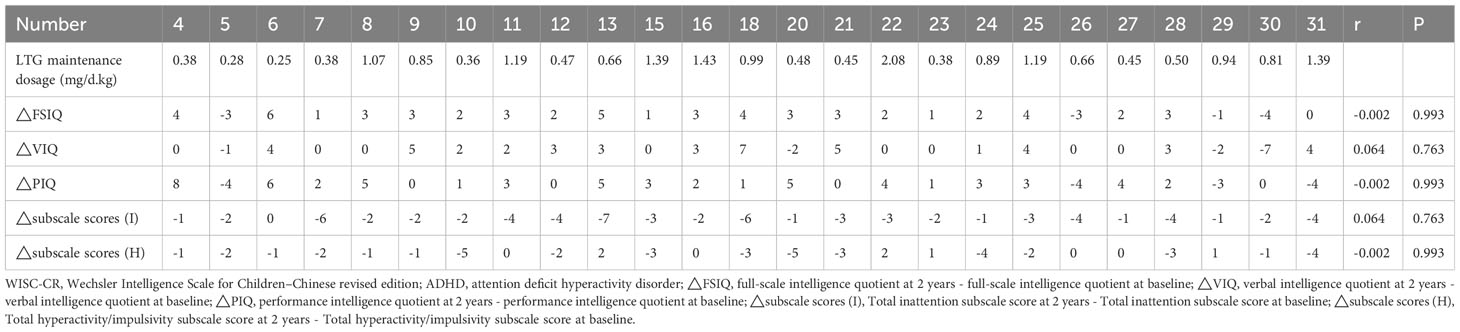
Table 4 Relationship between dosage per kilogram and improvements in WISC-CR Scores or ADHD Symptomatology.
4 Discussion
Paroxysmal kinesigenic dyskinesia (PKD) is the most common form of paroxysmal dyskinesia. Typically triggered by sudden voluntary movements, PKD manifests as brief episodes of movement disorders such as bilateral chorea, ballism, or dystonia, with patients maintaining consciousness during these episodes (18, 19). Over 40% of patients are initially misdiagnosed upon their first medical consultation. Patients often spend years, sometimes decades, seeking an accurate diagnosis and appropriate treatment (20). In addition there is still a very lack of research on cognitive function in children with PKD patients, only very few studies found individuals carrying either homozygous or compound-heterozygous present with a higher risk of intellectual disability and learning difficulties than those with heterozygous PRRT2 mutations (6, 21). This suggests that PRRT2 mutations have a gene-dosage effect and that other factors critically influence PRRT2-PKD symptoms.
Approximately more than half of global PKD cases are linked to PRRT2 mutations, underscoring their close association (22–25). Although not much is known about the PRRT2 protein, it is known to interact with SNAP25, a pre-synaptic membrane protein, which has led to the theory that the disease pathogenesis may be related to synaptic dysfunction (26, 27). In addition, PRRT2 mutations are hypothesized to interfere with the glutamatergic signal, leading to neuronal hyperexcitability by glutamate (28). Findings from animal model studies of PKD support the theory that hyperexcitability in the basal ganglia underlies these symptoms (29, 30). Most studies indicate that γ-aminobutyric acid acts as the principal inhibitory neurotransmitter in the basal ganglia, dominating information processing across its various regions (20, 31). A recent study highlighted that PRRT2 mutations might directly impact synaptic vesicle cycling (32–35). Notably, this cycling process is associated with a spectrum of neurological symptoms such as movement disorders and epilepsies, cognitive impairments including developmental delay and intellectual disabilities, as well as mental health challenges like autism and ADHD (4, 36, 37).
ADHD is the most common neurobehavioral disorder in children, marked by inattention, impulse and anger control problems, influenced by genetic and environmental factors. The worldwide-pooled prevalence of ADHD is 5.29% (38). The mechanisms underlying ADHD are still unclear, monoamine neurotransmitters are still most valued (39, 40), but an imbalance of glutamic acid/γ-aminobutyric acid may also be present (41). As is mentioned above, glutamate is the major excitatory neurotransmitter, meanwhile, γ-aminobutyric acid is the major inhibitory neurotransmitter in the brain. A study has demonstrated hyperactivity can be produced by pharmacologically increasing the activity of the nucleus accumbens core using glutamatergic (42). And another study has showed children with ADHD had lower γ-aminobutyric acid levels in a region including parts of the primary somatosensory and motor cortices (43).
To the best of our understanding, limited studies have been conducted on the outcomes of cognitive functions and behavioral impairments in children with PKD, both in China and internationally. An earlier study with a limited sample size revealed that psychiatric comorbidities such as obsessive-compulsive disorder, depression, Autism spectrum disorder, tic disorder, and attention deficit hyperactivity disorder (ADHD) were observed in 6/14 patients with PKD (42.9%) (44). A cross-sectional survey of 165 primary PKD patients highlighted that depression, anxiety, and low levels of quality of life were prevalent among these patients (45, 46). Certain research suggests that the PRRT2 loss of function in the hippocampus and frontal lobe of the cerebral cortex might induce neuronal hyperexcitability and synaptic deregulation, leading to compromised cognitive performance (47).
Our findings align with previous studies (37), highlighting a higher prevalence of ADHD (12/31) in children with PKD compared to the general population (5.32%). However, we noted that the average cognitive function in PKD patients did not deviate from the normal range, and PRRT2 variants did not contribute to the prevalence of ADHD. We hypothesize that genetic modifiers, age-dependent transcriptional profiles, or distinct environmental factors might influence the condition of neurodevelopment. Interestingly, the only significant predictor for comorbid ADHD in patients with PKD was a relatively longer period before drug therapy, indicating that it is potentially a consequence of psychosocial disadvantages associated with enduring PKD symptoms or the cumulative negative impacts of living with the illness. We posit that attacks in PKD are more likely a dysfunction of the basal ganglia, which do not lead to neurobiological consequences of plasticity or apoptosis. Nonetheless, the causal relationship among ADHD, FSIQ, and PKD remains inconclusive.
Moreover, our study revealed that patients who had never received specialized treatment for ADHD experienced significant alleviation of ADHD symptoms after two years of consistent medication for PKD. They also achieved significantly higher scores on FSIQ and VIQ. This significant alleviation of ADHD symptoms might lead to improved academic achievement and reduce the risk of several negative outcomes. The practical implications of minor improvements in IQ scores remain uncertain. Still, a plausible explanation could be that the medication reduced disturbances from both PKD and ADHD symptoms, thereby enabling the children to tap into more of their cognitive potential.
The effect of lamotrigine on cognition remains a topic of debate (10). Several studies have indicated that lamotrigine is not associated with impaired cognitive function and is effective in treating bipolar disorder (48). Moreover, it may enhance cognitive function and health-related quality of life (9, 49). Conversely, other studies have suggested that long-term lamotrigine treatment might lead to types of cognitive impairments not observed during short-term treatment (10). Nevertheless, no conclusive evidence exists that lamotrigine therapy directly affects cognitive function or ADHD symptoms.
Our study indicates Prolonged duration of untreated PKD in children may elevate the risk of ADHD comorbidity and provides evidence of significant cognitive improvement and ADHD symptom mitigation in children with PKD following a two-year treatment with low-dose lamotrigine. These findings recommended clinicians to screen all children with PKD for ADHD-related symptoms, meanwhile, a proactive and comprehensive treatment s is probably necessary.
Our study had several limitations, including the open uncontrolled design, which suggests that factors other than the treatment of lamotrigine might have influenced the results, and the relatively small sample size. Despite neither the patients nor their parents reporting specific ADHD symptoms, neuropsychological tests were conducted as screening tests for all newly diagnosed PKD patients. This might have led parents to respond more positively on the questionnaires.
Data availability statement
The original contributions presented in the study are included in the article/Supplementary Material. Further inquiries can be directed to the corresponding authors.
Ethics statement
The studies involving humans were approved by The Second Affiliated Hospital and Yuying Children’s Hospital of Wenzhou Medical University. The studies were conducted in accordance with the local legislation and institutional requirements. Written informed consent for participation was not required from the participants or the participants’ legal guardians/next of kin in accordance with the national legislation and institutional requirements. Written informed consent was obtained from the minor(s)’ legal guardian/next of kin for the publication of any potentially identifiable images or data included in this article.
Author contributions
FL: Resources, Writing – original draft. D-DY: Data curation,Investigation, Writing–original draft. Y-MH: Data curation,Funding acquisition, Methodology, Resources, Writing–original draft, Writing – review & editing. X-YW: Data curation, Investigation, Methodology, Writing – review & editing. WL: Data curation, Writing – original draft, Writing – review & editing.
Acknowledgments
This work was supported by the grants from Science and Technology Program of Wenzhou (Y20210273) and Science and Technology Program of Wenzhou (Y20210284).
Conflict of interest
The authors declare that the research was conducted in the absence of any commercial or financial relationships that could be construed as a potential conflict of interest.
Publisher’s note
All claims expressed in this article are solely those of the authors and do not necessarily represent those of their affiliated organizations, or those of the publisher, the editors and the reviewers. Any product that may be evaluated in this article, or claim that may be made by its manufacturer, is not guaranteed or endorsed by the publisher.
Supplementary material
The Supplementary Material for this article can be found online at: https://www.frontiersin.org/articles/10.3389/fpsyt.2024.1368289/full#supplementary-material
References
2. Jankovic J, Demirkiran M. Classification of paroxysmal dyskinesias and ataxias. Adv Neurol. (2002) 89:387–400.
3. Li J, Zhu X, Wang X, Sun W, Feng B, Du T, et al. Targeted genomic sequencing identifies PRRT2 mutations as a cause of paroxysmal kinesigenic choreoathetosis. J Med Genet. (2012) 49:76–8. doi: 10.1136/jmedgenet-2011-100635
4. Najmabadi H, Hu H, Garshasbi M, Zemojtel T, Abedini SS, Chen W, et al. Deep sequencing reveals 50 novel genes for recessive cognitive disorders. Nature. (2011) 478:57–63. doi: 10.1038/nature10423
5. Zhao G, Liu X, Zhang Q, Wang K. PRRT2 mutations in a cohort of Chinese families with paroxysmal kinesigenic dyskinesia and genotype-phenotype correlation reanalysis in literatures. Int J Neurosci. (2018) 128:751–60. doi: 10.1080/00207454.2017.1418345
6. Labate A, Tarantino P, Viri M, Mumoli L, Gagliardi M, Romeo A, et al. Homozygous c.649dupC mutation in PRRT2 worsens the BFIS/PKD phenotype with mental retardation, episodic ataxia, and absences. Epilepsia. (2012) 53:e196-9. doi: 10.1111/epi.12009
7. Delcourt M, Riant F, Mancini J, Milh M, Navarro V, Roze E, et al. Severe phenotypic spectrum of biallelic mutations in PRRT2 gene. J Neurol Neurosurg Psychiatry. (2015) 86:782–5. doi: 10.1136/jnnp-2014-309025
8. Park SP, Kwon SH. Cognitive effects of antiepileptic drugs. J Clin Neurol. (2008) 4:99–106. doi: 10.3988/jcn.2008.4.3.99
9. D Blum 1, Meador K, Biton V, Fakhoury T, Shneker B, Chung S, et al. Cognitive effects of lamotrigine compared with topiramate in patients with epilepsy. Neurology. (2006) 67:400–6. doi: 10.1212/01.wnl.0000232737.72555.06
10. Aldenkamp AP, Baker G. A systematic review of the effects of lamotrigine on cognitive function and quality of life. Epilepsy Behav. (2001) 2:85–91. doi: 10.1006/ebeh.2001.0168
11. Kwan P, Brodie MJ. Neuropsychological effects of epilepsy and antiepileptic drugs. Lancet. (9251) 2001:357. doi: 10.1016/S0140-6736(00)03600-X
12. Pitkänen A, Sutula TP. Is epilepsy a progressive disorder? Prospects for new therapeutic approaches in temporal-lobe epilepsy. Lancet Neurol. (2002) 1:173–81. doi: 10.1016/S1474-4422(02)00073-X
13. Bruno MK, Hallett M, Gwinn-Hardy K, Sorensen B, Considine E, Tucker S, et al. Clinical evaluation of idiopathic paroxysmal kinesigenic dyskinesia: new diagnostic criteria. Neurology. (2004) 63:2280–7doi: 10.1212/01.wnl.0000147298.05983.50
14. Wechsler D. The wechsler intelligence scale for children. 3rd ed.San Antonio, TX: The Psychological Corporation (1991).
15. American Psychiatric Association. DSM-IV: Diagnostic and statistical manual of mental disorders. Washington, DC: American Psychiatric Association (2000).
16. Chao RCL, Manita J. DSM-IV-TR: Diagnostic and statistical manual of mental disorders. Hoboken, NJ: Wiley (2000). doi: 10.1002/9781118339893.wbeccp180
17. DuPaul GJ, Reid R, Anastopoulos AD, Lambert MC, Watkins MW, Power TJ. Parent and teacher ratings of attention-deficit/hyperactivity disorder symptoms: Factor structure and normative data. Psychol Assess. (2016) 28:214–25. doi: 10.1037/pas0000166
18. Demirkiran M, Jankovic J. Paroxysmal dyskinesias: clinical features and classification. Ann Neurol. (1995) 38:571–9. doi: 10.1002/ana.410380405
19. Lance JW. Familial paroxysmal dystonic choreoathetosis and its differentiation from related syndromes. Ann Neurol. (1977) 2:285–93. doi: 10.1002/ana.410020405
20. Pan G, Zhang L, Zhou S. Clinical features of patients with paroxysmal kinesigenic dyskinesia, mutation screening of PRRT2 and the effects of morning draughts of oxcarbazepine. BMC Pediatr. (2019) 19:439. doi: 10.1186/s12887-019-1798-7
21. Dale RC, Gardiner A, Antony J, Houlden H. Familial PRRT2 mutation with heterogeneous paroxysmal disorders including paroxysmal torticollis and hemiplegic migraine. Dev Med Child Neurol. (2012) 54:958–60. doi: 10.1111/j.1469-8749.2012.04394.x
22. Chen WJ, Lin Y, Xiong ZQ, Wei W, Ni W, Tan GH, et al. Exome sequencing identifies truncating mutations in PRRT2 that cause paroxysmal kinesigenic dyskinesia. Nat Genet. (2011) 43:1252–5. doi: 10.1038/ng.1008
23. Youn J, Kim JS, Lee M, Lee J, Roh H, Ki CS, et al. Clinical manifestations in paroxysmal kinesigenic dyskinesia patients with proline-rich transmembrane protein 2 gene mutation. J Clin Neurol. (2014) 10:50–4. doi: 10.3988/jcn.2014.10.1.50
24. Méneret A, Grabli D, Depienne C, Gaudebout C, Picard F, Dürr A, et al. PRRT2 mutations: a major cause of paroxysmal kinesigenic dyskinesia in the European population. Neurology. (2012) 79:170–4. doi: 10.1212/WNL.0b013e31825f06c3
25. Huang XJ, Wang T, Wang JL, Liu XL, Che XQ, Li J, et al. Paroxysmal kinesigenic dyskinesia: Clinical and genetic analyses of 110 patients. Neurology. (2015) 85:1546–53. doi: 10.1212/WNL.0000000000002079
26. Lee H-Y, Huang Y, Bruneau N, Roll P, Roberson EDO, Hermann M, et al. Mutations in the novel protein PRRT2 cause paroxysmal kinesigenic dyskinesia with infantile convulsions. . Cell Rep. (2012) 26:2–12. doi: 10.1016/j.celrep.2011.11.001
27. Gizer IR, Ficks C, Waldman ID. Candidate gene studies of ADHD: a meta-analytic review. Hum Genet. (2009) 126:51–90. doi: 10.1007/s00439-009-0694-x
28. Li M, Niu F, Zhu X, Wu X, Shen N, Peng X, et al. PRRT2 mutant leads to dysfunction of glutamate signaling. Int J Mol Sci. (2015) 16:9134–51. doi: 10.3390/ijms16059134
29. Yoo D, Kim HJ, Choi JH, Lim JH, Jeon B. Tics in paroxysmal kinesigenic dyskinesia. Mov Disord Clin Pract. (2019) 6:502–3. doi: 10.1002/mdc3.12779
30. Mir P, Huang YZ, Gilio F, Edwards MJ, Berardelli A, Rothwell JC, et al. Abnormal cortical and spinal inhibition in paroxysmal kinesigenic dyskinesia. Brain. (2005) 128:291–9. doi: 10.1093/brain/awh342
31. Margari L, Presicci A, Ventura P, Margari F, Perniola T. Channelopathy: hypothesis of a common pathophysiologic mechanism in different forms of paroxysmal dyskinesia. Pediatr Neurol. (2005) 32:229–35. doi: 10.1016/j.pediatrneurol.2004.12.004
32. Jarvis SE, Zamponi GW. Masters or slaves? Vesicle release machinery and the regulation of presynaptic calcium channels. Cell Calcium. (2005) 37:483–8. doi: 10.1016/j.ceca.2005.01.017
33. Lamperti C, Invernizzi F, Solazzi R, Freri E, Carella F, Zeviani M, et al. Clinical and genetic features of paroxysmal kinesigenic dyskinesia in Italian patients. Eur J Paediatr Neurol. (2016) 20:152–7. doi: 10.1016/j.ejpn.2015.08.006
34. Valtorta F, Benfenati F, Zara F, Meldolesi J. PRRT2: from paroxysmal disorders to regulation of synaptic function. Trends Neurosci. (2016) 39:668–79. doi: 10.1016/j.tins.2016.08.005
35. Erro R, Bhatia KP, Espay AJ, Striano P. The epileptic and nonepileptic spectrum of paroxysmal dyskinesias: Channelopathies, synaptopathies, and transportopathies. Mov Disord. (2017) 32:310–8. doi: 10.1002/mds.26901
36. Long Z, Xu Q, Miao HH, Yu Y, Ding MP, Chen H, et al. Thalamocortical dysconnectivity in paroxysmal kinesigenic dyskinesia: Combining functional magnetic resonance imaging and diffusion tensor imaging. Mov Disord. (2017) 32:592–600. doi: 10.1002/mds.26905
37. Dale RC, Grattan-Smith P, Nicholson M, Peters GB. Microdeletions detected using chromosome microarray in children with suspected genetic movement disorders: a single-centre study. Dev Med Child Neurol. (2012) 54:618–23. doi: 10.1111/j.1469-8749.2012.04287.x
38. Polanczyk G, de Lima MS, Horta BL, Biederman J, Rohde LA. The worldwide prevalence of ADHD: a systematic review and metaregression analysis. Am J Psychiatry. (2007) 164:942–8. doi: 10.1176/ajp.2007.164.6.942
39. Sharma A, Couture J. A review of the pathophysiology, etiology, and treatment of attention-deficit hyperactivity disorder (ADHD). Ann Pharmacother. (2014) 48:209–25. doi: 10.1177/1060028013510699
40. Matheus FC, Rial D, Real JI, Lemos C, Ben J, Guaita GO, et al. Decreased synaptic plasticity in the medial prefrontal cortex underlies short-term memory deficits in 6-OHDA-lesioned rats. Behav Brain Res. (2016) 15:43–54. doi: 10.1016/j.bbr.2015.12.011
41. Purkayastha P, Malapati A, Yogeeswari P, Sriram D. A review on GABA/glutamate pathway for therapeutic intervention of ASD and ADHD. Curr Med Chem. (2015) 22:1850–59. doi: 10.2174/0929867322666150209152712
42. Hayes DJ, Jupp B, Sawiak SJ, Merlo E, Caprioli D, Dalley JW. Brain γ-aminobutyric acid: a neglected role in impulsivity. Eur J Neurosci. (2014) 39:1921–32. doi: 10.1111/ejn.12485
43. Edden RA, Crocetti D, Zhu H, Gilbert DL, Mostofsky SH. Reduced GABA concentration in attention-deficit/hyperactivity disorder. Arch Gen Psychiatry. (2012) 69:750–3. doi: 10.1001/archgenpsychiatry.2011.2280
44. Balint B, Wiethoff S, Martino D, Del Gamba C, Latorre A, Ganos C, et al. Quick flicks: association of paroxysmal kinesigenic dyskinesia and tics. Mov Disord Clin Pract. (2018) 5:317–20. doi: 10.1002/mdc3.12615
45. Lee HY, Huang Y, Bruneau N, Roll P, Roberson ED, Hermann M, et al. Mutations in the gene PRRT2 cause paroxysmal kinesigenic dyskinesia with infantile convulsions. Cell Rep. (2012) 1:2–12. doi: 10.1016/j.celrep.2011.11.001
46. Ebrahimi-Fakhari D, Saffari A, Westenberger A, Klein C. The evolving spectrum of PRRT2-associated paroxysmal diseases. Brain. (2015) 138:3476–95. doi: 10.1093/brain/awv317
47. Kim SY, Lee JS, Kim WJ, Kim H, Choi SA, Lim BC, et al. Paroxysmal dyskinesia in children: from genes to the clinic. J Clin Neurol. (2018) 14:492–7. doi: 10.3988/jcn.2018.14.4.492
48. Meador KJ, Loring DW, Vahle VJ, Ray PG, Werz MA, Fessler AJ, et al. Cognitive and behavioral effects of lamotrigine and topiramate in healthy volunteers. Neurology. (2005) 64:2108–14. doi: 10.1212/01.WNL.0000165994.46777.BE
Keywords: PKD, ADHD, intelligence, PRRT2, follow-up, lamotrigine
Citation: You D-d, Huang Y-m, Wang X-y, Li W and Li F (2024) Long-term low-dose lamotrigine for paroxysmal kinesigenic dyskinesia: a two-year investigation of cognitive function in children. Front. Psychiatry 15:1368289. doi: 10.3389/fpsyt.2024.1368289
Received: 10 January 2024; Accepted: 27 February 2024;
Published: 11 March 2024.
Edited by:
Ernest Pedapati, Cincinnati Children’s Hospital Medical Center, United StatesReviewed by:
Kotaro Yuge, Kurume University Hospital, JapanWong Shi Bing, Taipei Tzu Chi Hospital, Taiwan
Copyright © 2024 You, Huang, Wang, Li and Li. This is an open-access article distributed under the terms of the Creative Commons Attribution License (CC BY). The use, distribution or reproduction in other forums is permitted, provided the original author(s) and the copyright owner(s) are credited and that the original publication in this journal is cited, in accordance with accepted academic practice. No use, distribution or reproduction is permitted which does not comply with these terms.
*Correspondence: Feng Li, bGlsaWZlZmVAMTYzLmNvbQ==; Wei Li, d3psdzE5NzMxMDIwQHlhaG9vLmNvbS5jbg==
†These authors have contributed equally to this work