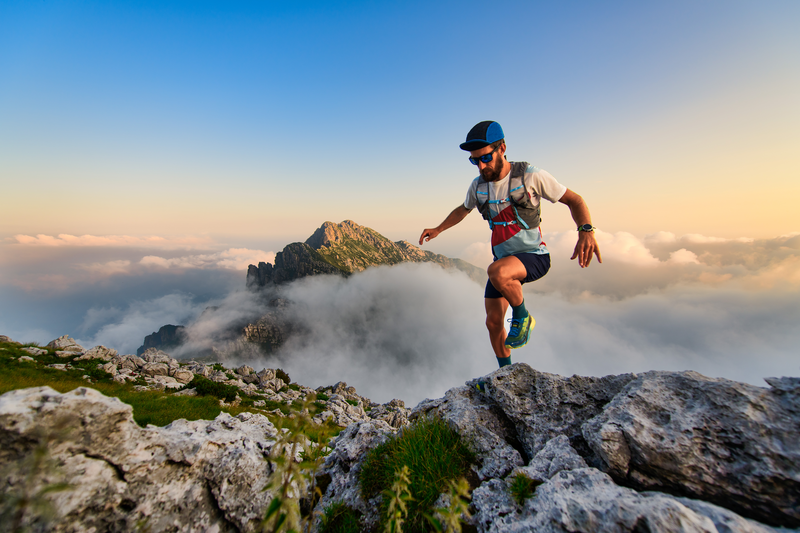
95% of researchers rate our articles as excellent or good
Learn more about the work of our research integrity team to safeguard the quality of each article we publish.
Find out more
ORIGINAL RESEARCH article
Front. Psychiatry , 23 April 2024
Sec. Schizophrenia
Volume 15 - 2024 | https://doi.org/10.3389/fpsyt.2024.1361997
This article is part of the Research Topic Unveiling the Neurobiological Underpinnings of Cognitive Dysfunction in Patients with Schizophrenia View all 11 articles
Introduction: Membrane phospholipid abnormalities are considered a pathophysiological background for schizophrenia. The aim of the study was to explore in detail the fatty acid (FA) composition in patients with antipsychotic-free schizophrenia and its association with clinical symptoms and cognitive function.
Materials and methods: Erythrocyte membrane FAs were measured in 29 antipsychotic-free patients with schizophrenia (male/female = 11/18; mean [standard deviation] age=26.7 [7.9] years) and age and sex-matched 32 healthy volunteers. Clinical symptoms and cognitive function were assessed using the Positive and Negative Syndrome Scale (PANSS), Brief Assessment of Cognition in Schizophrenia (BACS), and the Schizophrenia Cognition Rating Scale (SCoRS).
Results: Eicosapentaenoic acid levels were lower in the schizophrenia group than in the healthy control group. In contrast, arachidonic acid and nervonic acid levels were higher in the schizophrenia group than in the control group. Nervonic acid levels were significantly associated with depression scores as measured by the PANSS. No FA levels were correlated with BACS score; however, oleic acid levels were significantly related to cognitive dysfunction, as measured by the SCoRS.
Conclusion: These findings suggest that depressive symptoms along with cognitive dysfunction in daily living in schizophrenia may be linked to the FA composition abnormalities. Further studies will be needed to examine potential longitudinal FA changes during the course of schizophrenia as well as disease specificity.
It has been suggested that the altered composition of phospholipids (composed of the sphingosine skeleton, fatty acid (FA), phosphoric acid, and alcohol), a major component of neural membranes, may be related to the pathophysiology of schizophrenia (1–4). As the phospholipid components likely influence the fluidity and elasticity of cell membranes (5, 6), their changes may lead to an increased membrane rigidity and consequently alter the conformation and function of proteins, receptors, and ion channels (7). Among the constituents of phospholipids, saturated FA e.g. palmitic acid (PA) and stearic acid (SA), as well as monounsaturated FAs (MUFAs), e.g. oleic acid (OA) and nervonic acid (NA), can be synthesized de novo. Conversely, some polyunsaturated fatty acids (PUFAs), e.g. n-3 (omega-3) and n-6 (omega-6) PUFAs are called essential unsaturated fatty acids because they must be acquired from the diet. n-3 PUFAs contain eicosapentaenoic acid (EPA), docosapentaenoic acid (DPA), and docosahexaenoic acid (DHA). n-6 PUFAs contain linoleic acid (LA), dihomogammalinolenic acid (DGLA), and arachidonic acid (AA). PUFAs play important roles in the regulation of neuronal migration, pruning, and synaptic plasticity (8). Moreover, n-6 PUFAs are pro-inflammatory bioactive lipids, whereas n-3 PUFAs are anti-inflammatory bioactive lipids (9–11). In this context, n-6/n-3 ratio has been frequently used, since it has been found to be related to several negative health consequences, such as promotion of chronic inflammation (12, 13). Meanwhile, OA can also exert anti-inflammatory effects (14) and NA can activate the antioxidant system (15), these are considered to compensate for deficiencies in essential FAs and their metabolites.
While not consistently replicated, decreased n-3 PUFAs, alteration of n-6 PUFAs and increased n-9 MUFAs in the erythrocyte membrane have been observed in patients with schizophrenia and related conditions (4, 7, 16–20). Several but no all studies also demonstrated an association between PUFAs deficiency and the severity of the negative symptoms of schizophrenia (21–23). Sumiyoshi et al. reported that a decrease in n-3 and n-6 PUFA levels was associated with impaired social cognition in chronically ill patients with schizophrenia (24), while we failed to replicate such relationship in anti-psychotic free first-episode patients (25). Recent studies suggested similar FA abnormalities in at-risk mental state (ARMS) individuals (8, 25) irrespective of outcome (i.e., later psychosis onset), where FA levels were associated with prodromal symptomatology and global functioning. These findings may suggest the role of erythrocyte membrane FA abnormalities as a biomarker associated with vulnerability to psychopathology, but reported FA findings in schizophrenia might also be affected by other factors such as environmental factors after the onset (e.g., dietary habits, physical condition), antipsychotic medication, and chronic oxidative stress. Therefore, further research in patients with less confounding factors is needed to better understand the pathophysiological role of membrane FA composition and its relation to clinical characteristics in schizophrenia.
As human brain is generally inaccessible for direct FA analysis in vivo, alternative approaches such as peripheral cell models and neuroimaging techniques have been used as surrogate biomarkers of membrane FAs in the central nervous system (16). Peripheral cell models, especially those of erythrocyte membranes, are frequently used to study brain lipid metabolism due to high correlation between peripheral erythrocyte membrane and brain tissue FA levels (r = 0.86) (26). Erythrocyte membrane FA composition is, as compared to plasma FA levels, less affected by food intake prior to blood sampling as a marker of long-term lipid storage (27–29) and also better reflects neural cell membrane FAs in human (30–32) and animals (33, 34).
This study comprehensively measured erythrocyte membrane FAs in antipsychotic-free patients with schizophrenia and healthy controls. The FAs assessed in this study included saturated FAs (PA and SA), n-9 MUFAs (OA and NA), n-3 PUFAs (EPA, DPA, and DHA), and n-6 PUFAs (LA, DGLA, and AA). These FAs were essential for a comprehensive analysis because of their importance as major constituents, accounting for more than 90% of the total FA content in erythrocytes and the brain (5, 18). Based on previous findings, we predicted that patient group would have an altered FA composition (especially decreased n-3 PUFA and increased n-9 MUFA) and that such alterations would contribute to their symptom severity and cognitive functions.
Twenty-nine Japanese patients with schizophrenia recruited from the University of Toyama Hospital, participated in this study. None of the patients took antipsychotic medications within the two weeks before blood sampling, and 20 of the 29 patients were antipsychotic-naïve. Eleven of the 29 patients had first-episode schizophrenia, defined as an illness duration of fewer than two years with a single psychotic episode. Thirty-two healthy volunteers were recruited from university students, hospital staff, and acquaintances. The patients diagnosed with schizophrenia underwent diagnostic interviews using the Structured Clinical Interview for DSM-IV Axis I Disorders (SCID-I) Patient Edition (35). Eleven of the 29 patients with schizophrenia and all the healthy volunteers overlapped with the subjects in our previous report (25).
Information on clinical history was collected through interviews with the patients, their families, or medical records. Physical examinations and standard laboratory tests confirmed that the participants were physically healthy. The exclusion criteria included being 40 years of age or older, a history of substance abuse or dependence, seizures, head injury, and an estimated premorbid IQ of less than 70 based on the Japanese Adult Reading Test (JART) (36). The JART is the Japanese version of The National Adult Reading Test (Nelson, 1982). It comprises several irregular Japanese words, and the participants’ premorbid IQ is estimated based on their reading performance (36). Additional criteria for healthy controls were; i) no Axis I disorders based on the SCID-I Non-patient Edition (35), and ii) no personal or family (within first-degree relatives) history of psychiatric disorders.
This study was conducted in accordance with the principles of the Declaration of Helsinki and approved by the Committee on Medical Ethics of Toyama University (no. I2013006) on February 5, 2014. Written informed consent was obtained from all participants after a full explanation of the purpose and procedure of the study was provided. Written consent was obtained from the parents or guardians of participants under 20 years of age.
Experienced psychiatrists or psychologists evaluated clinical symptoms, cognitive function, and social function using the Positive and Negative Syndrome Scale (PANSS) (37), Brief Assessment of Cognition in Schizophrenia (BACS) (38, 39), Schizophrenia Cognition Rating Scale (SCoRS) (40, 41), and the modified Global Assessment of Functioning (mGAF) (42). The BACS composite scores were obtained by averaging the z-scores of the six subtests (39). Socio-economic status (SES) was measured by the Hollingshead-Redlich scale (43). Clinical assessments were performed on the same day as or within two weeks of blood collection.
Blood samples were collected from the study participants between 08:30 and 10:00 after at least two hours of fasting for FA measurements and general blood and biochemical examinations. Erythrocyte membrane FA levels were analyzed by gas chromatography based on an established method (18, 24, 25, 44). Briefly, 1 mL of red blood cells obtained from the subjects was collected in a 15-mL screw cap vial. Erythrocytes and plasma were separated by centrifugation and only erythrocytes were extracted and washed with saline. The vial was filled with 4.0 mL of 0.6 N methanolic HCl containing 4 μL of 0.5% butyl hydroxytoluene (BHT) as an internal standard and was then sealed and incubated at 80°C for two hours. Methylated FAs were extracted twice with hexane and the layers were separated by centrifugation in a swinging rotor at 3000 g for 15 min at room temperature. The hexane layer was carefully removed and the residue was collected in separate vials. The hexane extract was dried entirely by passing it through argon and it was then stored at -40°C until use. The methylated FAs were resuspended in 150 μL hexane, and aliquots (1 μL) were used for FA analysis by a Shimadzu gas chromatograph (Model GC-2010, Japan), using a capillary column with dimensions 30 m×0.32 mm×0.20 μm (Supelco, USA). A flame ionization detector was used with a column oven temperature of 160°C for 10 min, programmed at 10°C rise/min up to 175°C, and held at 220°C for 10 min was used. The injector and detector temperatures were set to 240°C and 275°C, respectively. The column was calibrated by injecting standard FA mixtures in approximately equal proportions. Peaks in the recorded data were identified based on the retention times of standard FAs under identical conditions.
The FA data were categorized into four groups: i) saturated FAs (PA and SA), ii) n-9 series MUFAs (OA and NA), iii) n-3 PUFAs (EPA, DPA, and DHA), and iv) n-6 series PUFAs (LA, DGLA, and AA). The FA levels were expressed as relative values measured as 100% of the 11 FAs, which included the 10 FAs mentioned above, with BHT as an internal standard (5). We calculated the following parameters as indices to assess the inflammatory response: i) n-6/n-3 ratio (AA/[EPA + DHA]) and ii) omega-3 index (EPA + DHA), based on previous literature (8, 45, 46).
Statistical analyses were performed using the Statistical Package for Social Sciences version 25 (SPSS Japan Inc.) and Jamovi Software (https://www.jamovi.org). The analyses covered FA composition and the PANSS, BACS and SCoRS scores and their subscale scores. The mGAF was measured as it is a well-known tool for determining the level of functioning of patients with general mental illness, however was not included in the present analysis due to its strong correlation with SCoRS. As most demographic/clinical data (age, PANSS subscale scores, BACS, SCoRS) had skewed distributions, the nonparametric Mann-Whitney U test was used to compare group differences. Similarly, nonparametric tests were employed to determine group differences in the FA composition, which had non-normal distributions. Spearman’s rho with semi-partial correlation was used to calculate the correlation between FA composition and clinical data, with only FA indices being controlled for by age, because age significantly affected FA composition in previous literature (47, 48) as well as our data (data not shown). For correlation analyses between FA composition and clinical variables, the Benjamini–Hochberg false discovery rate (FDR) procedure was used because there were many items to be compared (49). Statistical significance was set at p less than 0.05.
Demographic data are shown in Table 1. The age and sex ratios of the groups were matched; however, there were significant differences in personal/parental socioeconomic status (controls > patients with schizophrenia). Body mass index (BMI) was higher in the schizophrenia than in the healthy group; however, it was within the normal range (18.5–25) in both groups. The estimated premorbid IQ measured by the JART was within the normal range. Some patients with schizophrenia were taking anxiolytics (n=2) and hypnotics (n=2). These medications did not affect clinical or cognitive indices, or the FAs composition (data not shown). None were taking antidepressant. Regarding cognitive function, patients with schizophrenia performed approximately one standard deviation lower on the composite score calculated from the BACS than those in the healthy group. SCoRS scores were high in the current dataset and exceeded the reported average of first-episode and chronic schizophrenia (3.8 ± 1.8 and 4.4 ± 1.9, respectively) (41). Social functioning measured by the mGAF was approximately 30 points, indicating major impairment in several areas of functioning (42).
Table 2 and Figure 1 present the results of FA composition analysis. The NA levels were significantly higher in the schizophrenia group than in the healthy group. EPA levels were lower and AA levels were higher in the schizophrenia group than in healthy controls. There was a significant difference in DPA levels and the n-6/n-3 ratio between controls and patients with schizophrenia; however, this did not persist after post-hoc analysis for multiple comparisons. These findings remained consistent even when we analyzed only antipsychotic-naïve or only first-episode patients (data not shown).
Figure 1 Scatter and violin plots of fatty acid composition. (A), (B), and (C) represent NA, EPA and AA compositions for H and Sch, respectively. The y-axis shows the percentage of each fatty acid when all (ten) fatty acids plus the internal standard, are adjusted to 100%. Values indicate false discovery rate adjusted p value (q). AA, arachidonic acid (20:4 n-6); EPA, eicosapentaenoic acid (20:5 n-3); H, healthy control; NA, nervonic acid (24:1 n-9); Sch, schizophrenia.
No correlations were found between the FAs and PANSS total or positive/negative scores. Only NA was weakly correlated with PANSS general psychopathology scores (rho=0.47, p=0.017), however this correlation did not survive after multiple comparisons (Table 3A). Regarding the subscales, a significant positive correlation was found between NA level and “depression” score (G6) as presented in Table 3B and Figure 2. NA level was also correlated with “anxiety” (G2) and “poor attention” (G11) scores, but they did not survive post-hoc analysis for multiple comparisons. There were no significant correlations between the other FAs or the summary values (the n6/n3 ratio and n-3 index) and PANSS subscale scores (data not shown).
Figure 2 The relationship between nervonic acid (NA) and PANSS_G6 (depression) score in schizophrenia. The y-axis shows the percentage of each fatty acid when all (ten) fatty acids plus the internal standard, are adjusted to 100%. Values represent Spearman’s rho and false discovery rate adjusted p value (q). The shaded area represents 95% confidence interval. NA, nervonic acid (24:1 n-9), PANSS, Positive and Negative Syndrome Scale.
The relationships between FAs and cognitive scales are presented in Table 4. A significant positive correlation was found between OA level and SCoRS score. The BACS composite score correlated with SA level; however, this correlation did not persist after post-hoc analysis for multiple comparisons. There were no significant correlations between the other FAs or summary values (the n6/n3 ratio and n-3 index) and cognitive scales.
Table 4 Relationships between fatty acid composition and cognitive and social functions in schizophrenia.
The correlation results between the OA and SCoRS subscales are presented in Table 5 and Figure 3. There were significant positive correlations between OA level and items 8 (remembering what you were going to say)?, 11 (concentrating sufficiently to read newspapers or books)?, and 20 (following conversations in a group)?.
Figure 3 The relationships between oleic acid (OA) and SCoRS subscales in schizophrenia. SCoRS_8; ‘Remembering what you were going to say?’; SCoRS_11, ‘Concentrating well enough to read a newspaper or a book?’; SCoRS_20, ‘Following conversations in a group?’. The y-axis shows the percentage of each fatty acid when all (ten) fatty acids plus the internal standard, are adjusted to 100%. Values represent Spearman’s rho and false discovery rate adjusted p value (q). The shaded areas represent 95% confidence interval. OA, oleic acid (18:1 n-9); SCoRS, Schizophrenia Cognition Rating Scale.
The present study investigated the erythrocyte membrane FA composition and its relationship with symptom severity, social, and cognitive functioning in antipsychotic-free patients with schizophrenia. Our findings showed that patients with schizophrenia had decreased levels of EPA (an n-3 PUFA), increased levels of AA (an n-6 PUFA), and a markedly increased level of NA (an n-9 MUFA), compared to controls. We also found that NA levels were predominantly associated with depression and related symptoms in patients with schizophrenia. Moreover, OA, an n-9 PUFA, was significantly and positively related to cognitive dysfunction related to daily living, as measured by the SCoRS in schizophrenia. These results suggest an association with specific aspects of symptomatology, suggesting FA composition may contribute.
The decreased EPA level in the current schizophrenia group is consistent with previous research (4, 7, 18), indicating that this change may be a characteristic trait of schizophrenia. FA levels including EPA composition are known to be affected by various confounding factors, such as antipsychotics (5, 7, 23, 50–53), aging (47, 48), dietary intake (54), and smoking (54). For this reason, this study examined only antipsychotic-free schizophrenia patients with early illness stages matched for age with control subjects, who likely have fewer confounding factors compared to previous studies. We also observed increased levels of AA, an n-6 PUFA. Previous studies consistently found an Increased n-6/n-3 ratio in schizophrenia (4, 5, 20). AA-derived eicosanoids have more prominent inflammatory activity than n-3 PUFAs, and an imbalance between n-6 and n-3 PUFAs, as indicated by the increased n-6/n-3 ratio, may cause neuroinflammatory pathology in neuropsychiatric disorders (55, 56). In other words, AA is a precursor of inflammatory bioactive lipids such as prostaglandins and thromboxane, which act in a pro-inflammatory direction (9, 10). Conversely, EPA is a known precursor of anti-inflammatory bioactive lipids, particularly resolvins E1/2, which are potent anti-inflammatory mediators and act in the direction of converging inflammation (11). Our findings suggest that patients with schizophrenia may have an excess of induced inflammation, resulting in damage to neurons or other organs. Furthermore, animal and experimental studies have suggested that PUFA abnormalities affect membrane properties in the central nervous system (e.g., fluidity, elasticity, and thickness) (6) and dopaminergic transmission (57), which might underlie vulnerability to psychosis (58). A combination of these factors may shape the pathophysiology of schizophrenia.
The role of PUFAs in the pathophysiology of schizophrenia led to the hypothesis that n-3 PUFAs (EPA and DHA) could be administered to patients with ARMS to prevent the onset of overt psychosis. Accordingly, the worldwide multicenter RCT, NEURAPRO, was conducted (59). However, n-3 PUFA did not provide additional benefits for psychosis prevention. Considering this finding, the role of n-3 PUFAs in schizophrenia remain unclear, suggesting that the changes observed in the present study also might be a secondary phenomenon rather than pathophysiology of schizophrenia. Conversely, a recently published network meta-analysis concluding that n-3 PUFAs helped in preventing transitions to psychosis as compared to in controls (60). Currently, Other large-scale studies have been conducted (https://clinicaltrials.gov/ct2/show/NCT01429454; https://clinicaltrials.gov/ct2/show/record/NCT02597439), and their conclusions are expected to further our understanding of the role of FAs in schizophrenia.
Another important finding in this study was a markedly high level of NA, an n-9 MUFA, in the schizophrenia group. This finding is consistent with previous studies that indicated increased NA levels in patients with schizophrenia and ARMS (16, 20, 25). We also found a positive correlation between NA and depressive symptoms as measured by the PANSS subscale. Anxiety and poor attention, which are common symptoms with patients with depression (61, 62) also showed trend-level correlation with an increased NA level. NA is an essential molecule for the growth and maintenance of integrity in the white matter and peripheral nervous tissue enriched by sphingomyelin (63) and is suggested to be related to psychiatric disorders (64). Our results may be partly in line with the neuroimaging evidence that abnormalities in brain connectivity contribute to the trait characteristics of schizophrenia (65), such as negative symptomatology (66) and cognitive deficits (67). Because the impaired integrity of white matter is also reported in depression (68–70), it may be possible that NA abnormalities commonly contribute to the pathophysiology of both schizophrenia and depression.
In this study, we found for the first time that OA and n-9 MUFA levels were significantly related to cognitive dysfunction related to daily living, as measured by the SCoRS, in patients with schizophrenia. As far as we know, no previous study has shown significant association between OA levels and schizophrenia; however, previous OA studies have shown associations with depression (71, 72), Parkinson’s disease (73) and Alzheimer’s disease (74). Notably, a recent large-scale study on depression (n=4459) (75) suggested that serum OA levels were positively associated with depression and that for every 1 mmol/L increase in OA levels, the prevalence of depression increased by 40%. OA is an important FA as the most abundant FA in plasma, accounting for approximately 80% of the plasma phospholipid MUFAs (76). Since stearoyl-CoA desaturase, the rate-limiting enzyme of OA, has been found to cause neurotoxicity by producing MUFAs and impairing microglia and macrophages (77), the possibility exists that this mechanism affects cognitive function in patients with schizophrenia.
The present study had several potential limitations. First, the sample size of the study was rather small by including only antipsychotic-free patients, which may have limited the generalizability and statistical power of our results. Second, we did not control for participants’ dietary habits and cigarette smoking that could affect FA results. All participants had normal blood test results and standard BMIs; however, the BMIs were higher in the patients than in the controls, whereas the SES was higher in the controls than in the patients. The SES score did not always reflect the exact nutritional status of the young patients included in this study; thus, we should have conducted a nutritional questionnaire survey in addition to the assessments of SES, BMI, and blood chemistry. Differences in the nutritional status potentially led to differences in the lifestyle habits and physical conditions between the groups. The possibility that this further affected the results of the symptom/cognition measures in each group cannot be ruled out. Third, as our study was cross-sectional, future longitudinal studies are needed to confirm the role of FA changes as a trait marker and to investigate the influence of illness stage. Finally, as FA abnormalities and their contribution to clinical symptoms were reported also in other neuropsychiatric disorders, such as depression [63], disease specificity of our findings should be tested in future studies.
In conclusion, this study expanded our previous FA findings in schizophrenia and confirmed the increase in EPA levels and decrease in AA levels in the affected patients. It further showed that erythrocyte membrane level of NA, an n-9 MUFA elevated in patients with possibility of impaired white matter integrity (65, 68–70), was significantly correlated with the severity of depressive symptoms in a cohort of antipsychotic free patients with relatively early stages. We also demonstrated for the first time that membrane OA, an n-9 MUFA abundantly exists in the human body, is associated with cognitive dysfunction in schizophrenia. Further studies on FA abnormalities in various illness stages of schizophrenia, potential influencing factors, and disease specificity will be needed to clarify the role of FA abnormalities in the pathophysiology of schizophrenia.
The raw data supporting the conclusions of this article will be made available by the authors, without undue reservation.
The studies involving humans were approved by The Committee on Medical Ethics of the University of Toyama. The studies were conducted in accordance with the local legislation and institutional requirements. Written informed consent for participation in this study was provided by the participants’ legal guardians/next of kin.
YH: Conceptualization, Data curation, Formal analysis, Funding acquisition, Investigation, Methodology, Project administration, Resources, Software, Supervision, Validation, Visualization, Writing – original draft, Writing – review & editing. TT: Data curation, Funding acquisition, Investigation, Project administration, Resources, Supervision, Writing – review & editing. HI: Data curation, Investigation, Writing – review & editing. DS: Data curation, Funding acquisition, Investigation, Project administration, Resources, Supervision, Writing – review & editing. TS: Conceptualization, Data curation, Formal analysis, Investigation, Methodology, Project administration, Resources, Supervision, Writing – review & editing. MS: Conceptualization, Formal analysis, Funding acquisition, Investigation, Methodology, Project administration, Resources, Supervision, Writing – review & editing, Data curation, Validation.
The author(s) declare financial support was received for the research, authorship, and/or publication of this article. This study was supported by the Japan Society for the Promotion of Science KAKENHI (grant numbers 16K10205, 18K07550, 20H03598, 26461739, 22K07554, and 22K15745), Japan Agency for Medical Research and Development (grant numbers JP19dk0307029 and JP22dk0307103h0002), 47th SEISHIN Medical Research Foundation (2014), and 10th Research Group for Schizophrenia (2014). The funding sources were not involved in the study design, data collection, data analyses, interpretation of results, writing of the report, or decision to submit the article for publication.
The authors would like to thank all participants involved in this study. We thank Yoichiro Takayanagi for their recruitment and patient support. We also thank Shimako Nishiyama for the clinical assessment and supporting the subjects, and Yuko Mizukami and Yukiko Akasaki for their clinical and neuropsychological assessments. The authors also greatly appreciate Aki Sugano’s contribution to the statistical analyses. We would like to thank Editage (www.editage.com) for the English language editing.
The authors declare that the research was conducted in the absence of any commercial or financial relationships that could be construed as a potential conflict of interest.
All claims expressed in this article are solely those of the authors and do not necessarily represent those of their affiliated organizations, or those of the publisher, the editors and the reviewers. Any product that may be evaluated in this article, or claim that may be made by its manufacturer, is not guaranteed or endorsed by the publisher.
1. Horrobin DF. The membrane phospholipid hypothesis as a biochemical basis for the neurodevelopmental concept of schizophrenia. Schizophr Res. (1998) 30:193–208. doi: 10.1016/s0920-9964(97)00151-5
2. Bennett CN, Horrobin DF. Gene targets related to phospholipid and fatty acid metabolism in schizophrenia and other psychiatric disorders: an update. Prostaglandins Leukot Essent Fatty Acids. (2000) 63:47–59. doi: 10.1054/plef.2000.0191
3. Fenton WS, Hibbeln J, Knable M. Essential fatty acids, lipid membrane abnormalities, and the diagnosis and treatment of schizophrenia. Biol Psychiatry. (2000) 47:8–21. doi: 10.1016/S0006-3223(99)00092-X
4. Reddy RD, Keshavan MS, Yao JK. Reduced red blood cell membrane essential polyunsaturated fatty acids in first episode schizophrenia at neuroleptic-naive baseline. Schizophr Bull. (2004) 30:901–11. doi: 10.1093/oxfordjournals.schbul.a007140
5. Khan MM, Evans DR, Gunna V, Scheffer RE, Parikh VV, Mahadik SP. Reduced erythrocyte membrane essential fatty acids and increased lipid peroxides in schizophrenia at the never-medicated first-episode of psychosis and after years of treatment with antipsychotics. Schizophr Res. (2002) 58:1–10. doi: 10.1016/s0920-9964(01)00334-6
6. Baccouch R, Shi Y, Vernay E, Mathelie-Guinlet M, Taib-Maamar N, Villette S, et al. The impact of lipid polyunsaturation on the physical and mechanical properties of lipid membranes. Biochim Biophys Acta Biomembr. (2023) 1865:184084. doi: 10.1016/j.bbamem.2022.184084
7. van der Kemp WJ, Klomp DW, Kahn RS, Luijten PR, Hulshoff Pol HE. A meta-analysis of the polyunsaturated fatty acid composition of erythrocyte membranes in schizophrenia. Schizophr Res. (2012) 141:153–61. doi: 10.1016/j.schres.2012.08.014
8. Kim SW, Jhon M, Kim JM, Smesny S, Rice S, Berk M, et al. Relationship between erythrocyte fatty acid composition and psychopathology in the vienna omega-3 study. PloS One. (2016) 11:e0151417. doi: 10.1371/journal.pone.0151417
9. Choque B, Catheline D, Rioux V, Legrand P. Linoleic acid: between doubts and certainties. Biochimie. (2014) 96:14–21. doi: 10.1016/j.biochi.2013.07.012
10. Tilley SL, Coffman TM, Koller BH. Mixed messages: modulation of inflammation and immune responses by prostaglandins and thromboxanes. J Clin Invest. (2001) 108:15–23. doi: 10.1172/JCI13416
11. Arita M, Bianchini F, Aliberti J, Sher A, Chiang N, Hong S, et al. Stereochemical assignment, antiinflammatory properties, and receptor for the omega-3 lipid mediator resolvin E1. J Exp Med. (2005) 201:713–22. doi: 10.1084/jem.20042031
12. Simopoulos AP. Evolutionary aspects of the dietary omega-6:omega-3 fatty acid ratio: medical implications. World Rev Nutr Diet. (2009) 100:1–21. doi: 10.1159/000235706
13. Calder PC. The relationship between the fatty acid composition of immune cells and their function. Prostaglandins Leukot Essent Fatty Acids. (2008) 79:101–8. doi: 10.1016/j.plefa.2008.09.016
14. Santa-Maria C, Lopez-Enriquez S, Montserrat-de la Paz S, Geniz I, Reyes-Quiroz ME, Moreno M, et al. Update on anti-inflammatory molecular mechanisms induced by oleic acid. Nutrients. (2023) 15(1):224. doi: 10.3390/nu15010224
15. Ling C, Li F, Zhao J, Wen M, Han X. Research progress of nervonic acid biosynthesis. J Oleo Sci. (2023) 72:889–900. doi: 10.5650/jos.ess23039
16. Alqarni A, Mitchell TW, McGorry PD, Nelson B, Markulev C, Yuen HP, et al. Comparison of erythrocyte omega-3 index, fatty acids and molecular phospholipid species in people at ultra-high risk of developing psychosis and healthy people. Schizophr Res. (2020) 226:44–51. doi: 10.1016/j.schres.2019.06.020
17. Su YA, Si T. Progress and challenges in research of the mechanisms of anhedonia in major depressive disorder. Gen Psychiatr. (2022) 35:e100724. doi: 10.1136/gpsych-2021-100724
18. Ranjekar PK, Hinge A, Hegde MV, Ghate M, Kale A, Sitasawad S, et al. Decreased antioxidant enzymes and membrane essential polyunsaturated fatty acids in schizophrenic and bipolar mood disorder patients. Psychiatry Res. (2003) 121:109–22. doi: 10.1016/s0165-1781(03)00220-8
19. Rice SM, Schafer MR, Klier C, Mossaheb N, Vijayakumar N, Amminger GP. Erythrocyte polyunsaturated fatty acid levels in young people at ultra-high risk for psychotic disorder and healthy adolescent controls. Psychiatry Res. (2015) 228:174–6. doi: 10.1016/j.psychres.2015.04.036
20. Medema S, Mocking RJ, Koeter MW, Vaz FM, Meijer C, de Haan L, et al. Levels of red blood cell fatty acids in patients with psychosis, their unaffected siblings, and healthy controls. Schizophr Bull. (2016) 42:358–68. doi: 10.1093/schbul/sbv133
21. Glen AI, Glen EM, Horrobin DF, Vaddadi KS, Spellman M, Morse-Fisher N, et al. A red cell membrane abnormality in a subgroup of schizophrenic patients: evidence for two diseases. Schizophr Res. (1994) 12:53–61. doi: 10.1016/0920-9964(94)90084-1
22. Bentsen H, Solberg DK, Refsum H, Bohmer T. Clinical and biochemical validation of two endophenotypes of schizophrenia defined by levels of polyunsaturated fatty acids in red blood cells. Prostaglandins Leukot Essent Fatty Acids. (2012) 87:35–41. doi: 10.1016/j.plefa.2012.05.005
23. Sethom MM, Fares S, Bouaziz N, Melki W, Jemaa R, Feki M, et al. Polyunsaturated fatty acids deficits are associated with psychotic state and negative symptoms in patients with schizophrenia. Prostaglandins Leukot Essent Fatty Acids. (2010) 83:131–6. doi: 10.1016/j.plefa.2010.07.001
24. Sumiyoshi T, Matsui M, Itoh H, Higuchi Y, Arai H, Takamiya C, et al. Essential polyunsaturated fatty acids and social cognition in schizophrenia. Psychiatry Res. (2008) 157:87–93. doi: 10.1016/j.psychres.2006.05.025
25. Le ATP, Higuchi Y, Sumiyoshi T, Itoh H, Sasabayashi D, Takahashi T, et al. Analysis of polyunsaturated fatty acids in antipsychotic-free individuals with at-risk mental state and patients with first-episode schizophrenia. Front Psychiatry. (2023) 14:1188452. doi: 10.3389/fpsyt.2023.1188452
26. Kuratko CN, Salem N Jr. Biomarkers of DHA status. Prostaglandins Leukot Essent Fatty Acids. (2009) 81:111–8. doi: 10.1016/j.plefa.2009.05.007
27. Harris WS, Sands SA, Windsor SL, Ali HA, Stevens TL, Magalski A, et al. Omega-3 fatty acids in cardiac biopsies from heart transplantation patients: correlation with erythrocytes and response to supplementation. Circulation. (2004) 110:1645–9. doi: 10.1161/01.CIR.0000142292.10048.B2
28. Arab L. Biomarkers of fat and fatty acid intake. J Nutr. (2003) 133 Suppl 3:925S–32S. doi: 10.1093/jn/133.3.925S
29. Harris WS, Thomas RM. Biological variability of blood omega-3 biomarkers. Clin Biochem. (2010) 43:338–40. doi: 10.1016/j.clinbiochem.2009.08.016
30. Yao J, Stanley JA, Reddy RD, Keshavan MS, Pettegrew JW. Correlations between peripheral polyunsaturated fatty acid content and in vivo membrane phospholipid metabolites. Biol Psychiatry. (2002) 52:823–30. doi: 10.1016/s0006-3223(02)01397-5
31. McNamara RK, Jandacek R, Rider T, Tso P, Hahn CG, Richtand NM, et al. Abnormalities in the fatty acid composition of the postmortem orbitofrontal cortex of schizophrenic patients: gender differences and partial normalization with antipsychotic medications. Schizophr Res. (2007) 91:37–50. doi: 10.1016/j.schres.2006.11.027
32. Hamazaki K, Maekawa M, Toyota T, Dean B, Hamazaki T, Yoshikawa T. Fatty acid composition of the postmortem corpus callosum of patients with schizophrenia, bipolar disorder, or major depressive disorder. Eur Psychiatry. (2017) 39:51–6. doi: 10.1016/j.eurpsy.2016.05.007
33. Connor WE, Neuringer M, Lin DS. Dietary effects on brain fatty acid composition: the reversibility of n-3 fatty acid deficiency and turnover of docosahexaenoic acid in the brain, erythrocytes, and plasma of rhesus monkeys. J Lipid Res. (1990) 31:237–47. doi: 10.1016/S0022-2275(20)43209-2
34. Carlson SE, Carver JD, House SG. High fat diets varying in ratios of polyunsaturated to saturated fatty acid and linoleic to linolenic acid: a comparison of rat neural and red cell membrane phospholipids. J Nutr. (1986) 116:718–25. doi: 10.1093/jn/116.5.718
35. First MB, Gibbon M, Spitzer RL, Williams JBW. Structured clinical interview for DSM-IV Axis I disorders. Washington DC: American Psychiatric Press (1997).
36. Matsuoka K, Uno M, Kasai K, Koyama K, Kim Y. Estimation of premorbid IQ in individuals with Alzheimer's disease using Japanese ideographic script (Kanji) compound words: Japanese version of National Adult Reading Test. Psychiatry Clin Neurosci. (2006) 60:332–9. doi: 10.1111/j.1440-1819.2006.01510.x
37. Kay SR, Fiszbein A, Opler LA. The positive and negative syndrome scale (PANSS) for schizophrenia. Schizophr Bull. (1987) 13:261–76. doi: 10.1093/schbul/13.2.261
38. Keefe RS, Goldberg TE, Harvey PD, Gold JM, Poe MP, Coughenour L. The Brief Assessment of Cognition in Schizophrenia: reliability, sensitivity, and comparison with a standard neurocognitive battery. Schizophr Res. (2004) 68:283–97. doi: 10.1016/j.schres.2003.09.011
39. Kaneda Y, Sumiyoshi T, Keefe R, Ishimoto Y, Numata S, Ohmori T. Brief assessment of cognition in schizophrenia: validation of the Japanese version. Psychiatry Clin Neurosci. (2007) 61:602–9. doi: 10.1111/j.1440-1819.2007.01725.x
40. Keefe RS, Poe M, Walker TM, Kang JW, Harvey PD. The Schizophrenia Cognition Rating Scale: an interview-based assessment and its relationship to cognition, real-world functioning, and functional capacity. Am J Psychiatry. (2006) 163:426–32. doi: 10.1176/appi.ajp.163.3.426
41. Higuchi Y, Sumiyoshi T, Seo T, Suga M, Takahashi T, Nishiyama S, et al. Associations between daily living skills, cognition, and real-world functioning across stages of schizophrenia; a study with the Schizophrenia Cognition Rating Scale Japanese version. Schizophr Res Cogn. (2017) 7:13–8. doi: 10.1016/j.scog.2017.01.001
42. Hall RC. Global assessment of functioning. A modified scale. Psychosomatics. (1995) 36:267–75. doi: 10.1016/S0033-3182(95)71666-8
43. Hollingshead AB, Redlich FC. Social class and mental illness: a community study. 1958. Am J Public Health. (2007) 97:1756–7. doi: 10.2105/ajph.97.10.1756
44. Sumiyoshi T, Higuchi Y, Matsui M, Itoh H, Uehara T, Itoh T, et al. Membrane fatty acid levels as a predictor of treatment response in chronic schizophrenia. Psychiatry Res. (2011) 186:23–7. doi: 10.1016/j.psychres.2010.07.049
45. Mansara PP, Deshpande RA, Vaidya MM, Kaul-Ghanekar R. Differential ratios of omega fatty acids (AA/EPA+DHA) modulate growth, lipid peroxidation and expression of tumor regulatory MARBPs in breast cancer cell lines MCF7 and MDA-MB-231. PloS One. (2015) 10:e0136542. doi: 10.1371/journal.pone.0136542
46. Harris WS, Von Schacky C. The Omega-3 Index: a new risk factor for death from coronary heart disease? Prev Med. (2004) 39:212–20. doi: 10.1016/j.ypmed.2004.02.030
47. Misharina TA, Burlakova EB, Fatkullina LD, Terenina MB, Krikunova NI, Vorob'eva AK, et al. [Changes in fatty acid composition in the brain and liver in aging mice of high cancer risk AKR strain and effect of savory essential oil administration on leukemic process]. BioMed Khim. (2011) 57:604–14. doi: 10.18097/PBMC
48. Lishinevs'ka V, Briuzhina TS, Korkushko OV. [Age-related changes in fatty acid composition of the erythrocyte membrane]. Fiziol Zh (1994). (2003) 49:47–51.
49. Benjamini Y, Drai D, Elmer G, Kafkafi N, Golani I. Controlling the false discovery rate in behavior genetics research. Behav Brain Res. (2001) 125:279–84. doi: 10.1016/s0166-4328(01)00297-2
50. McEvoy J, Baillie RA, Zhu H, Buckley P, Keshavan MS, Nasrallah HA, et al. Lipidomics reveals early metabolic changes in subjects with schizophrenia: effects of atypical antipsychotics. PloS One. (2013) 8:e68717. doi: 10.1371/journal.pone.0068717
51. Chung YC, Cui Y, Sumiyoshi T, Kim MG, Lee KH. Associations of fatty acids with cognition, psychopathology, and brain-derived neurotrophic factor levels in patients with first-episode schizophrenia and related disorders treated with paliperidone extended release. J Psychopharmacol. (2017) 31:1556–63. doi: 10.1177/0269881117731169
52. Evans DR, Parikh VV, Khan MM, Coussons C, Buckley PF, Mahadik SP. Red blood cell membrane essential fatty acid metabolism in early psychotic patients following antipsychotic drug treatment. Prostaglandins Leukot Essent Fatty Acids. (2003) 69:393–9. doi: 10.1016/j.plefa.2003.08.010
53. Li N, Yang P, Tang M, Liu Y, Guo W, Lang B, et al. Reduced erythrocyte membrane polyunsaturated fatty acid levels indicate diminished treatment response in patients with multi- versus first-episode schizophrenia. Schizophr (Heidelb). (2022) 8:7. doi: 10.1038/s41537-022-00214-2
54. Hibbeln JR, Makino KK, Martin CE, Dickerson F, Boronow J, Fenton WS. Smoking, gender, and dietary influences on erythrocyte essential fatty acid composition among patients with schizophrenia or schizoaffective disorder. Biol Psychiatry. (2003) 53:431–41. doi: 10.1016/s0006-3223(02)01549-4
55. Kirkbride JB, Susser E, Kundakovic M, Kresovich JK, Davey Smith G, Relton CL. Prenatal nutrition, epigenetics and schizophrenia risk: can we test causal effects? Epigenomics. (2012) 4:303–15. doi: 10.2217/epi.12.20
56. Lankinen M, Uusitupa M, Schwab U. Genes and dietary fatty acids in regulation of fatty acid composition of plasma and erythrocyte membranes. Nutrients. (2018) 10(11):1785. doi: 10.3390/nu10111785
57. Ducrocq F, Walle R, Contini A, Oummadi A, Caraballo B, van der Veldt S, et al. Causal link between n-3 polyunsaturated fatty acid deficiency and motivation deficits. Cell Metab. (2020) 31:755–72.e7. doi: 10.1016/j.cmet.2020.02.012
58. Maekawa M, Watanabe A, Iwayama Y, Kimura T, Hamazaki K, Balan S, et al. Polyunsaturated fatty acid deficiency during neurodevelopment in mice models the prodromal state of schizophrenia through epigenetic changes in nuclear receptor genes. Transl Psychiatry. (2017) 7:e1229. doi: 10.1038/tp.2017.182
59. Nelson B, Amminger GP, Yuen HP, Markulev C, Lavoie S, Schafer MR, et al. NEURAPRO: a multi-centre RCT of omega-3 polyunsaturated fatty acids versus placebo in young people at ultra-high risk of psychotic disorders-medium-term follow-up and clinical course. NPJ Schizophr. (2018) 4:11. doi: 10.1038/s41537-018-0052-x
60. Chen C, Deng Y, Li Y, Zhang M, Yu T, Xie K, et al. Network meta-analysis indicates superior effects of omega-3 polyunsaturated fatty acids in preventing the transition to psychosis in individuals at clinical high-risk. Int J Neuropsychopharmacol. (2024) 27(3):pyae014. doi: 10.1093/ijnp/pyae014
62. Roca M, Vives M, Lopez-Navarro E, Garcia-Campayo J, Gili M. Cognitive impairments and depression: a critical review. Actas Esp Psiquiatr. (2015) 43:187–93.
63. Martinez M, Mougan I. Fatty acid composition of human brain phospholipids during normal development. J Neurochem. (1998) 71:2528–33. doi: 10.1046/j.1471-4159.1998.71062528.x
64. Amminger GP, Schafer MR, Klier CM, Slavik JM, Holzer I, Holub M, et al. Decreased nervonic acid levels in erythrocyte membranes predict psychosis in help-seeking ultra-high-risk individuals. Mol Psychiatry. (2012) 17:1150–2. doi: 10.1038/mp.2011.167
65. Fitzsimmons J, Kubicki M, Shenton ME. Review of functional and anatomical brain connectivity findings in schizophrenia. Curr Opin Psychiatry. (2013) 26:172–87. doi: 10.1097/YCO.0b013e32835d9e6a
66. Hochstrasser L, Studerus E, Riecher-Rossler A, Schimmelmann BG, Lambert M, Lang UE, et al. Latent state-trait structure of BPRS subscales in clinical high-risk state and first episode psychosis. Sci Rep. (2022) 12:6652. doi: 10.1038/s41598-022-10207-x
67. Mark W, Toulopoulou T. Cognitive intermediate phenotype and genetic risk for psychosis. Curr Opin Neurobiol. (2016) 36:23–30. doi: 10.1016/j.conb.2015.08.008
68. Nobuhara K, Okugawa G, Sugimoto T, Minami T, Tamagaki C, Takase K, et al. Frontal white matter anisotropy and symptom severity of late-life depression: a magnetic resonance diffusion tensor imaging study. J Neurol Neurosurg Psychiatry. (2006) 77:120–2. doi: 10.1136/jnnp.2004.055129
69. Li L, Ma N, Li Z, Tan L, Liu J, Gong G, et al. Prefrontal white matter abnormalities in young adult with major depressive disorder: a diffusion tensor imaging study. Brain Res. (2007) 1168:124–8. doi: 10.1016/j.brainres.2007.06.094
70. van Velzen LS, Kelly S, Isaev D, Aleman A, Aftanas LI, Bauer J, et al. White matter disturbances in major depressive disorder: a coordinated analysis across 20 international cohorts in the ENIGMA MDD working group. Mol Psychiatry. (2020) 25:1511–25. doi: 10.1038/s41380-019-0477-2
71. Zhou X, Liu L, Lan X, Cohen D, Zhang Y, Ravindran AV, et al. Polyunsaturated fatty acids metabolism, purine metabolism and inosine as potential independent diagnostic biomarkers for major depressive disorder in children and adolescents. Mol Psychiatry. (2019) 24:1478–88. doi: 10.1038/s41380-018-0047-z
72. Durand AC, Gentile S, Gerbeaux P, Alazia M, Kiegel P, Luigi S, et al. Be careful with triage in emergency departments: interobserver agreement on 1,578 patients in France. BMC Emerg Med. (2011) 11:19. doi: 10.1186/1471-227X-11-19
73. Tardiff DF, Lucas M, Wrona I, Chang B, Chung CY, Le Bourdonnec B, et al. Non-clinical pharmacology of YTX-7739: a clinical stage stearoyl-coA desaturase inhibitor being developed for parkinson's disease. Mol Neurobiol. (2022) 59:2171–89. doi: 10.1007/s12035-021-02695-1
74. Hamilton LK, Moquin-Beaudry G, Mangahas CL, Pratesi F, Aubin M, Aumont A, et al. Stearoyl-CoA Desaturase inhibition reverses immune, synaptic and cognitive impairments in an Alzheimer's disease mouse model. Nat Commun. (2022) 13:2061. doi: 10.1038/s41467-022-29506-y
75. Yin J, Li S, Li J, Gong R, Jia Z, Liu J, et al. Association of serum oleic acid level with depression in American adults: a cross-sectional study. BMC Psychiatry. (2023) 23:845. doi: 10.1186/s12888-023-05271-0
76. Staiger H, Staiger K, Stefan N, Wahl HG, Machicao F, Kellerer M, et al. Palmitate-induced interleukin-6 expression in human coronary artery endothelial cells. Diabetes. (2004) 53:3209–16. doi: 10.2337/diabetes.53.12.3209
Keywords: cognitive function, depression, erythrocyte membrane, monounsaturated fatty acid, polyunsaturated fatty acid, nervonic acid, oleic acid, schizophrenia
Citation: Higuchi Y, Takahashi T, Itoh H, Sasabayashi D, Sumiyoshi T and Suzuki M (2024) Relationships between erythrocyte membrane mono- and poly- unsaturated fatty acid composition and clinical/cognitive indices in antipsychotic-free schizophrenia patients. Front. Psychiatry 15:1361997. doi: 10.3389/fpsyt.2024.1361997
Received: 27 December 2023; Accepted: 26 March 2024;
Published: 23 April 2024.
Edited by:
Wing Chung Chang, The University of Hong Kong, Hong Kong SAR, ChinaReviewed by:
Gregor E. Berger, Psychiatric University Hospital Zurich, SwitzerlandCopyright © 2024 Higuchi, Takahashi, Itoh, Sasabayashi, Sumiyoshi and Suzuki. This is an open-access article distributed under the terms of the Creative Commons Attribution License (CC BY). The use, distribution or reproduction in other forums is permitted, provided the original author(s) and the copyright owner(s) are credited and that the original publication in this journal is cited, in accordance with accepted academic practice. No use, distribution or reproduction is permitted which does not comply with these terms.
*Correspondence: Yuko Higuchi, eWhpZ3VjaGlAbWVkLnUtdG95YW1hLmFjLmpw
Disclaimer: All claims expressed in this article are solely those of the authors and do not necessarily represent those of their affiliated organizations, or those of the publisher, the editors and the reviewers. Any product that may be evaluated in this article or claim that may be made by its manufacturer is not guaranteed or endorsed by the publisher.
Research integrity at Frontiers
Learn more about the work of our research integrity team to safeguard the quality of each article we publish.