- 1Department of Psychiatry, College of Medicine, University of Kentucky, Lexington, KY, United States
- 2Department of Psychology, College of Arts & Sciences, University of Kentucky, Lexington, KY, United States
- 3Brain Imaging and Analyses Center (BIAC), Duke University Medical Center, Durham, NC, United States
- 4Department of Behavioral Sciences, College of Medicine, University of Kentucky, Lexington, KY, United States
- 5Department of Psychiatry, University of Texas Southwestern Medical School, Dallas, TX, United States
Background: People living with HIV (PLWHA) smoke at three times the rate of the general population and respond poorly to cessation strategies. Previous studies examined repetitive transcranial magnetic stimulation (rTMS) over left dorsolateral prefrontal cortex (L. dlPFC) to reduce craving, but no studies have explored rTMS among PLWHA who smoke. The current pilot study compared the effects of active and sham intermittent theta-burst stimulation (iTBS) on resting state functional connectivity (rsFC), cigarette cue attentional bias, and cigarette craving in PLWHA who smoke.
Methods: Eight PLWHA were recruited (single-blind, within-subject design) to receive one session of iTBS (n=8) over the L. dlPFC using neuronavigation and, four weeks later, sham iTBS (n=5). Cigarette craving and attentional bias assessments were completed before and after both iTBS and sham iTBS. rsFC was assessed before iTBS (baseline) and after iTBS and sham iTBS.
Results: Compared to sham iTBS, iTBS enhanced rsFC between the L. dlPFC and bilateral medial prefrontal cortex and pons. iTBS also enhanced rsFC between the right insula and right occipital cortex compared to sham iTBS. iTBS also decreased cigarette craving and cigarette cue attentional bias.
Conclusion: iTBS could potentially offer a therapeutic option for smoking cessation in PLWHA.
1 Introduction
People living with HIV/AIDS (PLWHA) smoke at three times the rate of the general population (1). These elevated smoking rates are associated with greater morbidity and mortality (2). Smoking impacts the progression and outcome of HIV disease and has been identified as the leading contributor to premature mortality among PLWHA (3). Smoking cessation may be the single most important behavioral health change for PLWHA who smoke (4, 5).
Pharmacotherapy is a pillar of smoking cessation treatment (6). Evidence regarding pharmacological smoking cessation strategies such as nicotine replacement therapy, bupropion, and varenicline in PLWHA is mixed and limited (7, 8). Although nicotine replacement therapy has been shown to be effective compared to placebo in people without HIV for smoking cessation for up to six months (9), controlled trials comparing nicotine replacement therapy to placebo are lacking in PLWHA who smoke. There have been no RCTs (Randomized Clinical Trial) examining efficacy of bupropion for smoking cessation in PLWHA who smoke. Additionally, bupropion’s pharmacokinetic interactions with antiretroviral therapy pose a barrier in PLWHA who smoke (10). While more effective than placebo, quit rates observed with varenicline remain troublingly low in PLWHA who smoke (11–14). Elevated rates of smoking in PLWHA, suboptimal treatment response rates, and lack of adherence to cessation strategies (15) underscore a significant need for novel or adjunct interventions for smoking cessation in PLWHA who smoke.
Multiple studies have demonstrated efficacy of repetitive transcranial magnetic stimulation (rTMS) in decreasing craving for cigarettes and for smoking cessation (16). Fourteen studies have tested the effects of TMS (including rTMS and deep TMS) in people who smoke (17–30). The majority (12) applied excitatory high frequency rTMS ranging from 10-20 Hz (17–28). The number of sessions in these studies ranged from 1-18 and cortical targets were heterogenous. Nine studies targeted the left dorsolateral prefrontal cortex (L. dlPFC), one study targeted the left superior frontal gyrus, and two studies targeting the right dlPFC. Moreover, two deep TMS studies targeted the bilateral dLPFC and insula using H coil. All studies, save for one (30), reported reductions in clinically relevant smoking metrics (e.g., subjective craving, number of cigarettes smoked, abstinence using exhaled CO or cotinine). All nine studies targeting the L. dlPFC showed reduction in clinically relevant smoking metrics (17–19, 21–26).
Theta burst stimulation (TBS) was approved by the Food and Drug Administration (FDA) for treatment of major depressive disorder (MDD) (31). Intermittent theta burst stimulation (iTBS) has been shown to be excitatory and 1800 pulses of iTBS has been seminal to a novel accelerated treatment protocol for MDD (Major Depressive Disorder) called Stanford Neuromodulation Treatment (32, 33). To the best of our knowledge, there has been only a single clinical study that utilized iTBS for smoking cessation (29), which found that four sessions of iTBS (600 pulses) with cognitive-behavioral therapy (CBT) was associated with a significantly greater reduction in smoking urges when compared to sham iTBS with CBT (29).
Previous studies have shown resting state functional connectivity (rsFC) changes following iTBS between the target site and other brain regions (34–40). Three studies have shown rsFC changes when iTBS was applied over L. dlPFC (41–43). The application of three trains of iTBS (600 pulses at 80% resting motor threshold [RMT]) at five-minute intervals reduced L. dlPFC to right anterior insula rsFC when compared to sham iTBS in healthy controls (n=28) (41). Another study applied a single train of iTBS (600 pulses at 90-120% RMT) over L. dlPFC to 18 healthy controls and found that rsFC increased (from baseline) between the L. dlPFC and bilateral caudate (42). iTBS in ten healthy volunteers (600 pulses at 80% RMT) over the L. dlPFC revealed increased rsFC between the bilateral superior frontal gyri, and bilateral, middle frontal, inferior frontal, and orbitofrontal gyri immediately after iTBS, compared to rsFC before iTBS (43).
Although neuroimaging correlates of iTBS in people who smoke cigarettes have not been explored, two published studies investigated neural correlates of 10Hz rTMS in people who smoke cigarettes (44, 45). One study delivered one session of 10Hz rTMS (3000 pulses) over the L. dlPFC in eleven people who smoked cigarettes and showed reductions in blood oxygen level dependent (BOLD) activity during a cigarette cue reactivity task in the left nucleus accumbens and right medial orbitofrontal cortex (OFC) when compared to sham rTMS (45). The other study compared one session of 10Hz rTMS versus sham rTMS over the L. dlPFC in ten participants who smoked cigarettes and showed decreased resting fractional amplitude of low frequency fluctuation (fALFF) in the right insula (44), which suggests reduced activity in this region.
Cigarette cue attentional bias (AB) offers a behavioral paradigm to monitor rTMS effects in smokers (46, 47). This bias (AB) is quantified using fixation time on cigarette and neutral cues, via an eye tracker. It can predict the severity of cigarette smoking as well as the chances of relapse (48–50). Two previous studies comparing 3600 pulses of continuous theta burst stimulation (cTBS) and sham cTBS over the left medial prefrontal cortex (MPFC) when viewing alcohol and cocaine cues, showed decreased seed based functional connectivity (MPFC as seed) with cTBS compared to sham cTBS (51, 52). In recent years, fMRI studies have also shown the right insula to be important for interoceptive awareness, cigarette cue reactivity and craving in participants who smoke (53–55).
To the best of our knowledge, no published studies have examined the effects of iTBS on rsFC in participants who smoke cigarettes. No studies have examined the effects of iTBS of the L. dlPFC on cigarette cue attentional bias, and no studies have evaluated the effects of iTBS among PLWHA who smoke. The following pilot study aimed to examine within subject effects of a single session of iTBS (1800 pulses at 120% RMT) administered to L. dlPFC using individualized neuronavigation on rsFC, cigarette cue attentional bias, and cigarette craving. In line with previous studies, our study hypotheses were as follows: 1) iTBS would alter rsFC between L. dlPFC and the right insula when compared to sham iTBS; 2) iTBS would alter rsFC between right insula and the dorsal cingulate cortex when compared to sham iTBS; 3) iTBS would decrease fixation time on cigarette cues and cigarette cue AB when compared to sham iTBS, and; 4) iTBS would decrease craving for cigarettes when compared to sham iTBS.
2 Methods
2.1 Participants
Eight participants were recruited from the Bluegrass Care Clinic within the University of Kentucky Medical Center. The average age of participants (comprising seven males and one female) was 42.88 years (standard error of the mean [SEM] 3.45 years). All five participants who returned to receive sham iTBS were male. Average Fagerstrom Test for Nicotine Dependence (FTND) score was 5.75 (SEM 0.96) for the sample and participants endorsed smoking an average of 22.75 cigarettes daily (SEM 2.4). All participants were right-handed.
[Table 1 – Demographics]
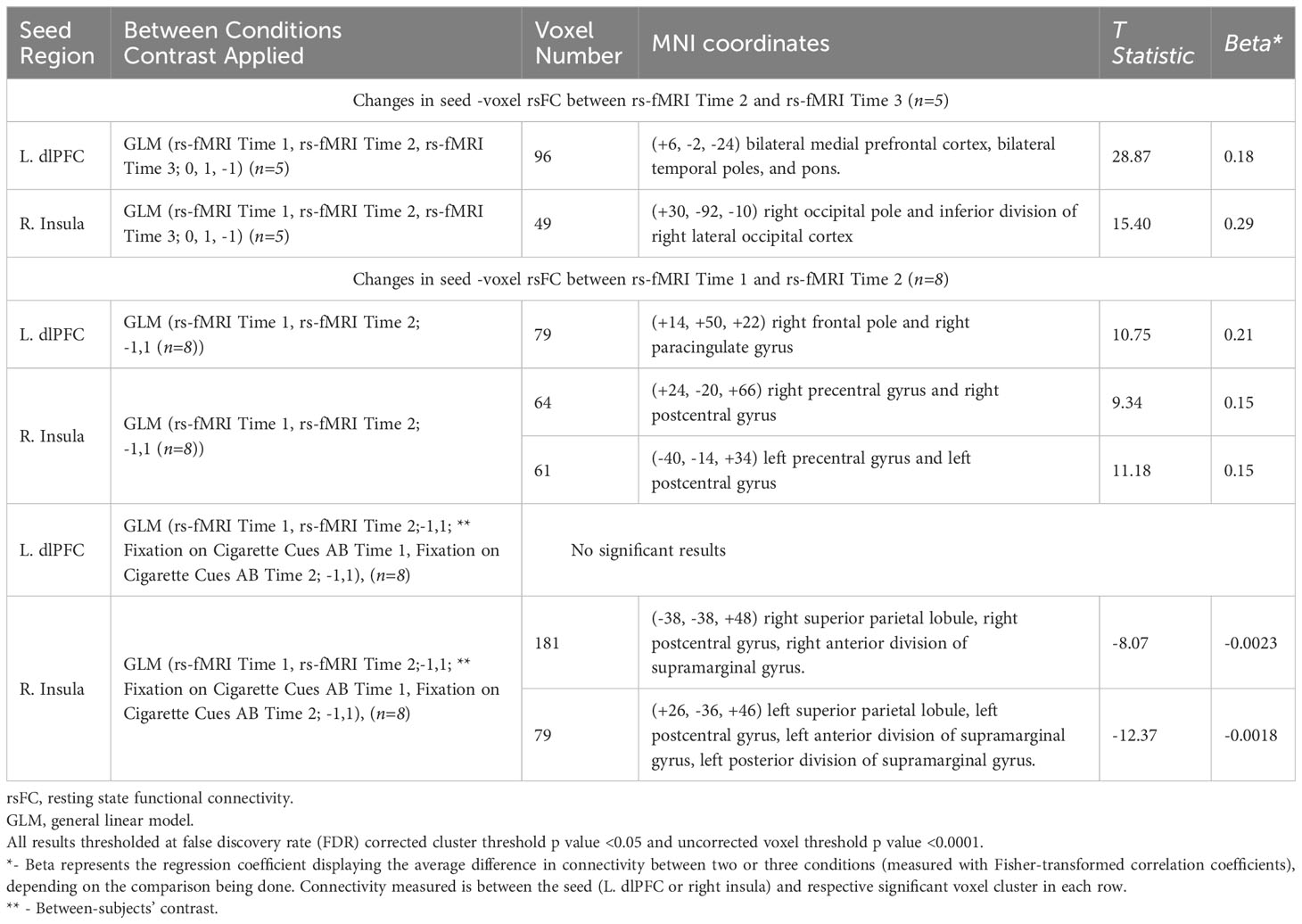
Table 1 Resting state functional connectivity (rsFC) changes with iTBS/Sham iTBS (two seeds - left dorsolateral prefrontal cortex or L. dlPFC and right insular cortex to all voxels).
2.2 Experimental protocol
The study protocol was approved by Medical Institutional Review Board (IRB) at University of Kentucky (IRB#64473) and was registered on clinical trials.gov (NCT04936594). Participants were recruited from the Bluegrass Care Clinic affiliated with University of Kentucky Medical Center. Supplementary Material Figure 1 summarizes the three-day experimental protocol. See Supplementary Material Section 1 for details regarding the number of patients who screened eligible for the study or dropped out. For a list of inclusion/exclusion criteria, please see Supplementary Material Section 2. After completing a phone screen to determine eligibility, participants were scheduled for Day 1 procedures, which consisted of informed consent, collection of demographic details, measuring carbon monoxide (CO) using a Smokerlyzer Breath CO Monitor (Bedfont Scientific Ltd., Rochester, England), measuring blood alcohol level (BAL) using a Breathalyzer, administering Fagerstrom Test for Nicotine Dependence (FTND) and an attentional bias screen (AB Screen). All study participants were scheduled to receive iTBS a week from Day 1 (Day 2) and sham iTBS five weeks from Day 1 (Day 3). iTBS and sham iTBS sessions were separated by four weeks to limit carryover effects. Day 2 consisted of 1) resting state fMRI brain scans before and after iTBS (rs-fMRI Time 1 and rs-fMRI Time 2 respectively), 2) attentional bias before and after iTBS (AB Time 1 and AB Time 2 respectively) and 3) cigarette craving assessment using the short form version of tobacco craving questionnaire before and after iTBS (TCQ-SF Time 1 and TCQ-SF Time 2 respectively). Day 3 was procedurally the same as Day 2 except sham iTBS was administered instead of iTBS and rs-fMRI scan were only completed after sham iTBS (rs-fMRI Time 3). All participants received iTBS first and sham iTBS subsequently but were blinded regarding treatment.
2.3 Attentional bias
We used a visual probe task to measure AB, adapted for cigarette cues (56, 57)(Supplementary Material Figure 2). We used a Tobii Pro Fusion 120 Hz eye tracker (Tobii Technology, Sweden) to monitor fixation time on cigarette and neutral cues, which helped calculate cigarette cue AB. For each AB trial, images of a cigarette I and a matched neutral (N) cue were presented on a laptop screen, 3 cm (about 1.18 in) apart. Upon offset of the image pairs, a visual probe (X) appeared on either the left side or right side of the screen, in the same location as one of the previously presented images. Firstly, twenty trials showed images of cues encompassing people smoking cigarettes and matched neutral cues for 2000 milliseconds (ms) (about 2 seconds). Secondly, twenty trials showed images of cues encompassing cigarette paraphernalia and matched neutral cues for 2000 ms.
For each set of twenty cigarette cue AB trials, twenty filler trials consisting of twenty pairs of additional neutral cue images (N-N) were intermixed to generate a total of 40 trials. Filler trials were also presented for 2000 ms. Intervals between C-N and N-N cue pairs were 2000 ms. Average fixation time was calculated separately for each set of AB trials by summing the total fixation time for each cue type across all trials and then dividing by the total number of critical trials (i.e., 20). Cigarette cue AB for each set of AB trials was calculated by average fixation time on cigarette cues minus average fixation time on neutral cues (56).
fMRI: Neuroimaging acquisition was completed with a 3T Siemens Magnetom PRISMA Scanner. Structural MRI images (MPRAGE) were acquired before iTBS. Multi-slice gradient echoplanar imaging (EPI) resting state images were acquired before iTBS (rs-fMRI Time 1), within an hour after iTBS (rs-fMRI Time 2) and within an hour after sham iTBS (rs-fMRI Time 3). Specifics of scan sequences are elucidated in Supplementary Material Section 4.
iTBS and Neuronavigation for Targeting: Structural MRI images were input into Brainsight software (Rogue Solutions, Montreal, Canada). The images were then registered to Montreal Neurological Institute (MNI) space by identifying the anterior commissure and posterior commissure in Brainsight software (Rogue Solutions, Montreal, Canada). The registered images were consequently used to generate a three-dimensional curvilinear brain model for each subject. This was used to identify the L. dlPFC (Brodmann area 46, MNI coordinate -44, 40,29) (58, 59). During delivery of iTBS, nine minutes eleven seconds (1800 pulses) of iTBS (biphasic bursts) at 120% of resting motor threshold (RMT) was administered to the L. dlPFC using MagVenture MagPro x100 with MagOption and B65 active/placebo (A/P) coil (MagVenture A/S, Denmark). The coil position was stabilized using a holder, with guidance from Brainsight Neuronavigation (Rogue Solutions, Montreal, Canada). Sham iTBS was administered using the sham interface of the B65 A/P coil. Two stimulation electrodes (Ambu Neuroline 710) were connected to the coil and placed on the left side of the scalp to mimic the somatosensory stimulation associated with iTBS. RMT was measured prior to iTBS and sham iTBS (RMT procedure described in Section 3 of Supplementary Material).
2.4 Clinical assessments
Tobacco Craving Questionnaire-Short Form (TCQ-SF). The Tobacco Craving Questionnaire-short form (TCQ-SF) consists of 12 items rated on an 84-point visual analogue scale. Validity and reliability of the TCQ-SF is comparable to the original 47-item version (60). The TCQ-SF was administered before and after iTBS on Day 2 (TCQ-SF Time 1 and TCQ-SF Time 2 in Figure 1) and sham iTBS on Day 3 (TCQ-SF Time 3 and TCQ-SF Time 4 in Figure 1).
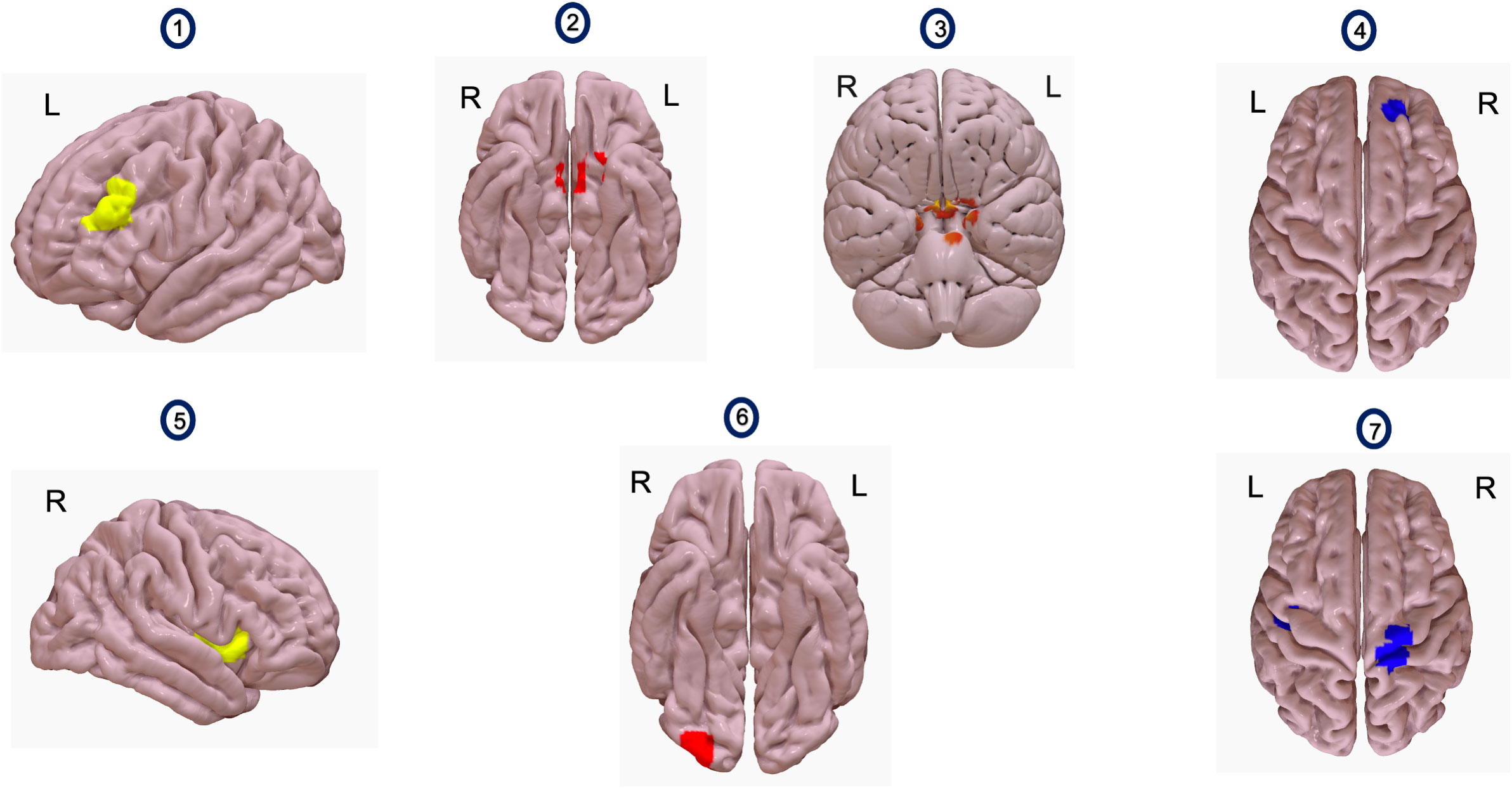
Figure 1 Images 1 and 5 show seeds used for analyses. Image 1 shows the left dlPFC and image 5 shows the right insula. Images 2 and 3 show a voxel cluster where resting state functional connectivity (rsFC) with L. dlPFC as a seed increased, with iTBS compared to sham iTBS (n=5). This cluster (red) encompasses bilateral medial prefrontal cortex (Image 2), bilateral temporal poles and pons (Image 3). Image 4 shows a voxel cluster where rsFC with L.dlPFC as a seed increased, after receiving iTBS, compared to before (n=8). The voxel cluster (blue) is situated over right frontal pole and right paracingulate gyrus. Image 6 shows a voxel cluster where rsFC with right insula as a seed increased, after receiving iTBS compared to sham iTBS (n=5). The voxel cluster (red) is situated in the right occipital pole and right lateral occipital cortex. Image 7 shows a voxel cluster where rsFC with right insula as a seed changed, after receiving iTBS, compared to before (n=8). The figure shows a voxel cluster (blue) situated over bilateral precentral and bilateral postcentral gyri.
Fagerstrom Test for Nicotine Dependence (FTND). The FTND is a six-item self-report measure of nicotine dependence severity. Three yes(1)/no(0) items and three multiple choice items (scored from 0 to 3) are used to calculate total severity (0-10) (61).
2.5 Data analytic strategy
2.5.1 Resting state functional connectivity analyses
CONN functional connectivity toolbox (version 21.a) was used for all preprocessing and neuroimaging analyses using standard procedures (see Supplementary Material Section 4). Firstly, general linear model (GLM) analyses explored if rsFC changed from two seeds (L. dlPFC and right insula) to all voxels following iTBS (rs-fMRI Time 2) versus sham iTBS (rs-fMRI Time 3) relative to baseline rsFC (rs-fMRI Time 1) in the five participants who completed all three days of the study. In first level analyses, a seed map was created for every subject using respective seeds (L. dlPFC or right insula). In second level analyses, a between conditions contrast of (0,1,-1) was used to assess rsFC within-subject differences across the three time points (rs-fMRI Time 1, rs-fMRI Time 2, rs-fMRI Time 3). All second level analyses used cluster level inferences based on Gaussian Random Field Theory (62). This was done using general linear models (GLM) to generate a statistical map of t-values, that were subsequently thresholded to reveal non overlapping clusters (https://web.conn-toolbox.org/fmri-methods/cluster-level-inferences). Two-sided p-values were generated using cluster-level false discovery rate (FDR) correction (63).
Subsequently, rsFC change from two seeds (L. dlPFC and right insula) to all voxels across from rs-fMRI Time 1 to rs-fMRI Time 2 was examined among the eight participants who only received iTBS. In first level analyses, a seed map was created for every subject using respective seeds (L. dlPFC or right insula). In second level analyses, a GLM with between conditions contrast of (1,-1) for scans (rs-fMRI Time 1, rs-fMRI Time 2) was used. In these eight participants, associations between changes in seed based rsFC following iTBS (rs-fMRI Time 1 versus rs-fMRI Time 2) and changes in fixation time on cues of people smoking cigarette (AB Time 1 versus AB Time 2) were explored. A GLM was specified with between conditions contrast of (-1,1) for rs-fMRI Time 1 and rs-fMRI Time 2, respectively, and a between subjects’ contrast of (-1,1) for fixation time on cues encompassing people smoking cigarettes acquired as part of AB Session 1 and AB Session 2 respectively.
2.5.2 Attentional bias analyses
Cigarette cue AB was calculated separately for each set of AB trials, by subtracting the average fixation time on neutral cues from the average fixation time on cigarette cues. Fixation time on cigarette cues for each session and resultant cigarette cue attentional bias score were entered into separate linear fixed effects regression models. Dependent variables for these models were fixation time on cigarette cue and cigarette cue AB, respectively. Fixed effects for both models were type of cigarette cues (people smoking cigarettes versus cigarette paraphernalia), time (before or iTBS/sham iTBS), intervention arm (iTBS versus sham iTBS) and FTND scores. Subject ID was a grouping variable in both models. Further details on regression models can be found in Supplementary Material Section 5.
2.5.3 Cigarette craving analyses
TCQ-SF scores were entered into a linear fixed effects regression model. Outcome variables for this model was TCQ-SF score. Predictor variables for this model were time (before or after iTBS/sham iTBS), intervention arm (iTBS versus sham iTBS), and FTND scores. Further details on regression models can be found in Supplementary Material Section 5.
3 Results
3.1 Seed-based resting state functional connectivity
General linear models (GLMs) comparing differences in rsFC between iTBS (rs-fMRI Time 2) and sham iTBS (rs-fMRI Time 3), relative to rs-fMRI Time 1, suggested that rsFC significantly increased between the L. dlPFC and a cluster comprising bilateral medial prefrontal cortex (MPFC), bilateral temporal poles, and pons [t(4)=28.87, p=0.0007] (Figure 1, Table 1) and significantly increased between the right insula and a cluster comprising the right occipital pole and inferior division of right lateral occipital cortex [t(4)=15.40, p=0.04] (Figure 1, Table 1). GLMs comparing change in rsFC from pre-to-post iTBS (rs-fMRI Time 1 and Time 2), suggested that rsFC significantly increased between the L. dlPFC and a cluster comprising the right frontal pole and right paracingulate gyrus [t(7)=10.75, p=0.02] (Figure 1, Table 1) and significantly increased between the right insula and in two voxel clusters – one comprising the right precentral gyrus and right postcentral gyrus, [t(7)=9.34, p=0.04] and one comprising the left precentral gyrus and left postcentral gyrus [t(7)=11.18, p=0.04] (Figure 1, Table 1).
[Table 1- Resting State Functional Connectivity (rsFC) Changes with iTBS/Sham iTBS]
[Figure 1 - Comparing resting state functional connectivity (rsFC) changes between iTBS and sham iTBS (Seeds – L. dlPFC, right insula)]
3.2 Attentional bias and self-reported craving
Linear fixed effects models revealed that, across both attention bias cigarette cues, the severity of nicotine dependence (FTND) was significantly associated with changes in fixation time on cigarette cues with iTBS and sham iTBS [t (43) = 2.27, p=0.03] (Supplementary Material Table 1). FTND was also significantly associated with changes cigarette cue AB with iTBS and sham iTBS [t (43) = 17.14, p=0.01] (Supplementary Material Table 2). Severity of cigarette cravings (TCQ-SF) was not significantly associated with fixation time on cigarette cues or cigarette cue AB (Supplementary Material Table 3).
Paired t-tests showed that fixation time and attentional bias to cues of people smoking cigarettes significantly reduced following iTBS, [t (15) =4.20, p=0.04] and [t (15) =3.14, p=0.02], respectively. See Table 2, Figure 2. Similarly, nicotine craving scores reduced from a mean of 55.88 (SEM 3.95) to 43.13 (SEM 4.68) with iTBS [t (15) =3.02, p=0.02]. With sham iTBS it reduced from a mean of 53.2 (SEM 4.77) to 48.40 (SEM 6.84) [t (9) =1.24, p=0.28].
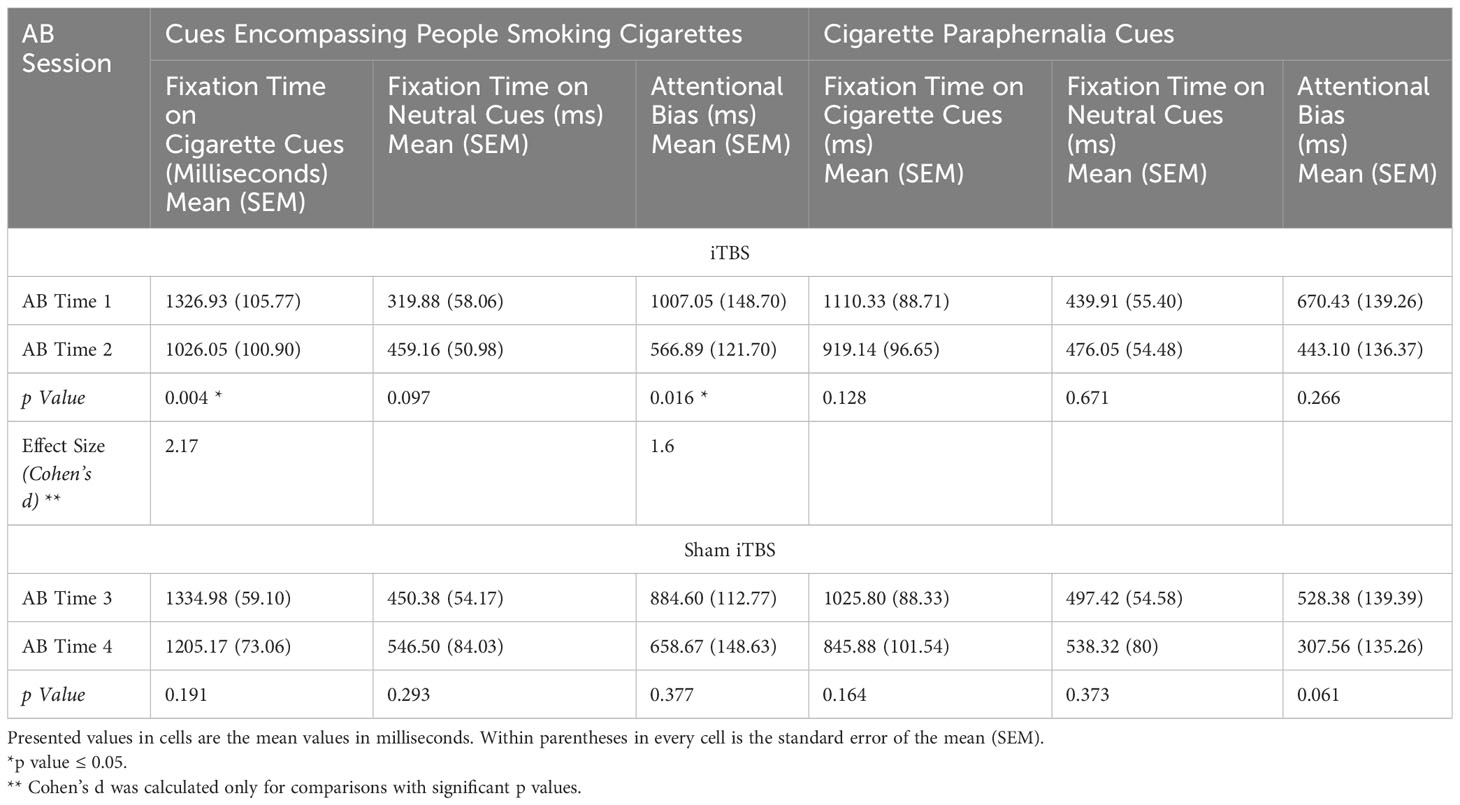
Table 2 Ad hoc t-tests to compare changes in fixation time and attentional bias with iTBS/Sham iTBS.
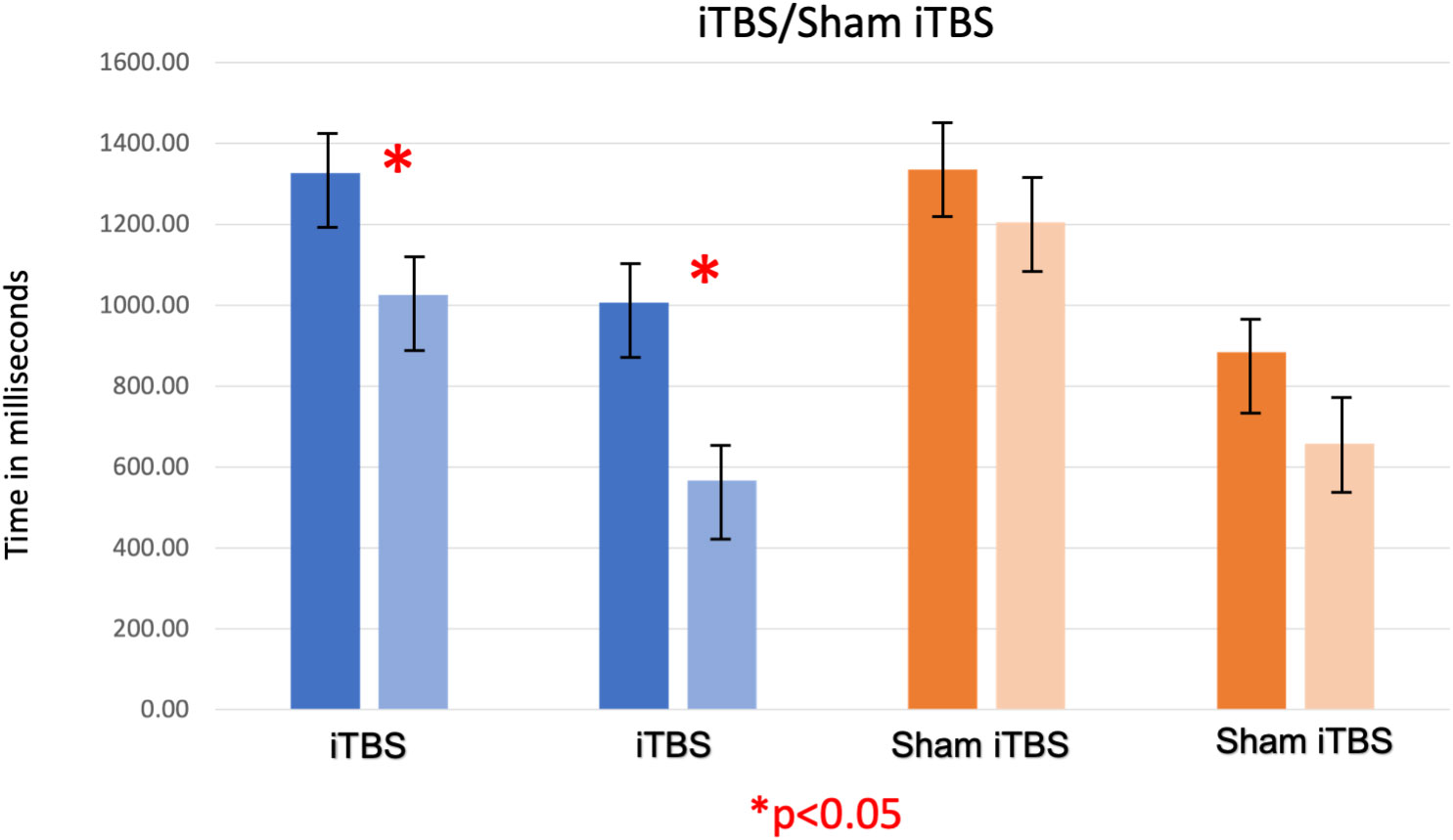
Figure 2 The first two bars (shades of blue) represent gaze fixation on cues encompassing people smoking cigarettes, acquired before and after iTBS (AB Time 1 and AB Time 2). The third and fourth bars (shades of blue) represent attentional bias for on cues encompassing people smoking cigarettes (measured by subtracting gaze fixation on neutral cues from gaze fixation on smoking cues) acquired before and after iTBS. The fifth and sixth bars (shades of orange) represent gaze fixation on the same cues, acquired before and after sham iTBS (AB Time 3 and AB Time 4). The seventh and eighth bars represent attentional bias for on cues encompassing people smoking cigarettes acquired before and after sham iTBS. As observed, iTBS showed a significant decrease in gaze fixation on on cues encompassing people smoking cigarettes and consequently on attentional bias also, which was not seen with sham iTBS.
[Figure 2 – Changes in Fixation Time and Cigarette Cue Attentional Bias (AB) with iTBS/Sham iTBS]
[Table 2 – Ad Hoc T-tests to Compare Changes in Fixation Time and Attentional Bias with iTBS/Sham iTBS]
3.3 Correlation between fixation time on cigarette cues and seed-based rsFC
A GLM suggested that decrease in fixation time to cues of people smoking cigarettes was significantly associated with increases in rsFC between the right insula and two clusters comprising right postcentral gyrus [t (7) = -8.07, p=0.000005] and left postcentral gyrus [t (7) = -12.37, p=0.005] respectively following iTBS (rs-fMRI 1 and rs-fMRI 2) (Table 1).
4 Discussion
This is the first study to examine the effects of iTBS on rsFC, cigarette craving, and cigarette cue attentional bias in PLWHA who smoke cigarettes. We found that, compared to sham iTBS, rsFC between the L. dlPFC and a voxel cluster comprising the mPFC and pons increased more following iTBS of the L. dlPFC. Although unexpected, we also found that rsFC between the right insula and right occipital cortex increased more following iTBS compared to sham iTBS.
With iTBS, we also saw increased rsFC between the L. dlPFC and the right frontal pole, right paracingulate gyrus, as well as between the right insula and bilateral precentral and postcentral gyri (somatosensory cortices). Cigarette craving and cigarette cue attentional bias significantly reduced following iTBS but not following sham iTBS, though the magnitude of these changes was not significantly different across the two experimental groups. Lastly, reductions in cigarette cue fixation time following iTBS were associated with increases in rsFC between the right insula and the right and left superior parietal lobules, the right and left postcentral gyri (somatosensory cortices) and the right and left supramarginal gyri.
Our results extend literature on functional activation changes following rTMS of the L.dlPFC in people who smoke. Two previous studies reported that rTMS of the L.dlPFC in smokers reduced BOLD activity in the right medial orbitofrontal cortex (OFC) (45), and right insula (44). The present findings suggest that rsFC of the mPFC, which included the medial OFC, and right insula may also be effected by rTMS of the L.dlPFC; though the present study utilized iTBS instead of high frequency TMS. Consistent with prior research (41–43), the present iTBS findings and past TMS research (including rTMS and deep TMS studies) with people who smoke suggest that brain stimulation can modulate functional connectivity and functional activation of regions and networks distal from the site of stimulation.
Although measurement of cigarette cue attentional bias provided a behavioral measure to compare effects of iTBS versus sham iTBS, it is possible that this facet may have unintentionally increased craving secondary to cue presentation. Since cigarette cue attentional bias was measured immediately before and after iTBS/sham iTBS session, it is possible that cigarette cue presentation may have influenced the brain state at the time of stimulation (64, 65). In this context, the finding that rsFC between the right insula and right lateral occipital cortex increased after iTBS is consistent with cigarette cue reactivity task studies, which reported that functional activation of the occipital cortex was positively associated with reactivity towards smoking cues in people who smoke cigarettes (54, 66). Increased rsFC between L. dlPFC and right frontal pole and right paracingulate gyrus following iTBS extends results from a meta-analysis examining neural correlates of cigarette cue reactivity. This meta-analysis showed the left anterior cingulate gyrus, paracingulate gyrus and dorsal cingulate cortex to be instrumental when examining fMRI correlates of reactivity towards smoking cues in people who smoke (67).
Our regression models suggested that changes in fixation time (Supplementary Material Table 1), cigarette cue attentional bias (Supplementary Material Table 2), and cigarette craving (Supplementary Material Table 3) were not significantly different between iTBS and sham iTBS. Nonetheless, we showed a significant reduction in craving, cigarette cue fixation time and attentional bias following iTBS and not following sham iTBS. The decrease in cigarette craving has been shown in previous TMS studies (16) and one iTBS study (29). However, our study is the first to show decrease in cigarette cue attentional bias with iTBS. The decrease in cigarette cue fixation time to cues of people smoking cigarettes following iTBS was also significantly associated with increases in rsFC between the right insula and clusters comprising the right and left somatosensory cortices in addition to the right and left posterior parietal cortices. This extends the literature on the role of right insula and parietal cortex in craving. Structural white matter connectivity between posterior parietal cortex and right insula can modulate craving (68). Right anterior insula is crucial for interceptive awareness and salience (69, 70). The posterior parietal cortices modulate selective attention, in addition to the somatosensory cortices being important for perceptual awareness (71, 72). When viewing cigarette and neutral cues for cigarette cue attentional bias measurement, the lateral posterior parietal cortex in conjunction with the right anterior insula integrates perceptual awareness of cues with internal physiological awareness, consequently modulating sustained attention (73, 74). These facets become crucial to craving and attentional bias as well (75).
We delivered 1800 pulses of iTBS to L.dlPFC, based on two seminal studies in major depressive disorder (MDD) which used the same dosing strategy in an accelerated fashion and showed a remission rate of approximately 90% in treatment resistant MDD (32, 33). We used a stimulus intensity of 120% of RMT based on a previous iTBS trial (31). Prior to this study, neither of these TBS dosing parameters have been applied to TMS studies in people who smoke cigarettes (regardless of HIV status). Moreover, the present study was the first to use neuronavigational targeting with structural MRI in PLWHA who smoke cigarettes.
We delivered actual and sham iTBS in a single blind fashion. Although patient expectations and placebo effects could have influenced results, we separated active and sham iTBS sessions by four weeks, which should have limited carryover effects (76). Nonetheless, replication and extension efforts should include double blinding. In an ideal design, we would have acquired a resting state fMRI scan before sham iTBS too. This is a limitation of our study. Although we measured cigarette cue attentional bias immediately after iTBS/sham iTBS, there was an average of an hour’s delay before they got to the scanner, and this may have influenced our results as well.
Future research using online (with cue presentation) vs. offline (at rest without prior provocation) iTBS could explore the potential moderating effects of cue presentation on iTBS in people who smoke cigarettes.
5 Conclusions and limitations
iTBS to the L. dlPFC in PLWHA who smoke increased rsFC between L.dlPFC and bilateral medial prefrontal cortex and pons, and increased rsFC between right insula and right occipital cortex. Despite a small sample size, iTBS decreased cigarette cue fixation time, cigarette cue attentional bias and cigarette craving. We also showed a negative association between change in cigarette cue fixation time to cues of people smoking cigarettes and rsFC between the right insula and clusters comprising the right and left somatosensory cortices in addition to the right and left posterior parietal cortices.
Our preliminary results are limited by our small sample size and within group comparisons (77–79). We would like to emphasize that our pilot study is the first attempt at delivering a high frequency paradigm like iTBS to PLWHA who smoke at elevated rates. Albeit preliminary, our results do warrant a powered prospective trial evaluating iTBS as a therapeutic intervention for smoking cessation in PLWHA who smoke, along with an optimal rsFC biomarker to guide treatment response.
Data availability statement
The raw data supporting the conclusions of this article will be made available by the authors, without undue reservation.
Ethics statement
The studies involving humans were approved by University of Kentucky College of Medicine IRB. The studies were conducted in accordance with the local legislation and institutional requirements. Written informed consent for participation in this study was provided by the participants’ legal guardians/next of kin. Written informed consent was obtained from the individual(s) for the publication of any potentially identifiable images or data included in this article.
Author contributions
GR: Conceptualization, Data curation, Formal analysis, Funding acquisition, Investigation, Methodology, Project administration, Software, Writing – original draft. TA: Conceptualization, Formal analysis, Investigation, Writing – review & editing. RM: Conceptualization, Formal analysis, Writing – review & editing. JA: Data curation, Formal analysis, Writing – review & editing. RK: Investigation, Writing – review & editing. AS: Investigation, Writing – review & editing. SH: Conceptualization, Writing – review & editing. CR: Conceptualization, Data curation, Formal analysis, Methodology, Project administration, Software, Supervision, Validation, Writing – review & editing.
Funding
The author(s) declare financial support was received for the research, authorship, and/or publication of this article. This work was supported by the National Institutes of Health (grant numbers TR001997, CA225419) and by the Junior Scholars' Track Award from the Department of Psychiatry, University of Kentucky College of Medicine.
Acknowledgments
The manuscript is available as a preprint on medrxiv https://www.medrxiv.org/content/10.1101/2023.05.08.23289662v1.
Conflict of interest
The authors declare that the research was conducted in the absence of any commercial or financial relationships that could be construed as a potential conflict of interest.
Publisher’s note
All claims expressed in this article are solely those of the authors and do not necessarily represent those of their affiliated organizations, or those of the publisher, the editors and the reviewers. Any product that may be evaluated in this article, or claim that may be made by its manufacturer, is not guaranteed or endorsed by the publisher.
Supplementary material
The Supplementary Material for this article can be found online at: https://www.frontiersin.org/articles/10.3389/fpsyt.2024.1315854/full#supplementary-material
References
1. Lifson AR, Lando HA. Smoking and HIV: prevalence, health risks, and cessation strategies. Curr HIV/AIDS Rep (2012) 9:223–30. doi: 10.1007/s11904-012-0121-0
2. Shirley DK, Kaner RJ and Glesby MJ. Effects of smoking on non-AIDS-related morbidity in HIV-infected patients. Clin Infect Dis (2013) 57:275–82. doi: 10.1093/cid/cit207
3. Helleberg M, Afzal S, Kronborg G, Larsen CS, Pedersen G, Pedersen C, et al. Mortality attributable to smoking among HIV-1-infected individuals: a nationwide, population-based cohort study. Clin Infect Dis (2013) 56:727–34. doi: 10.1093/cid/cis933
4. Drach L, Holbert T, Maher J, Fox V, Schubert S, Saddler LC, et al. Integrating smoking cessation into HIV care. AIDS Patient Care STDS (2010) 24:139–40. doi: 10.1089/apc.2009.0274
5. Lubitz SF, Flitter A, Ashare RL, Thompson M, Leone F, Gross R, et al. Improved clinical outcomes among persons with HIV who quit smoking. AIDS Care (2020) 32:1217–23. doi: 10.1080/09540121.2019.1703891
6. Lindson N, Klemperer E, Hong B, Ordonez-Mena JM, Aveyard P. Smoking reduction interventions for smoking cessation. Cochrane Database Syst Rev (2019) 9:CD013183. doi: 10.1002/14651858.CD013183.pub2
7. Ledgerwood DM, Yskes R. Smoking cessation for people living with HIV/AIDS: A literature review and synthesis. Nicotine Tob Res (2016) 18:2177–84. doi: 10.1093/ntr/ntw126
8. Pacek LR, Cioe PA. Tobacco use, use disorders, and smoking cessation interventions in persons living with HIV. Curr HIV/AIDS Rep (2015) 12:413–20. doi: 10.1007/s11904-015-0281-9
9. Cepeda-Benito A, Reynoso JT and Erath S. Meta-analysis of the efficacy of nicotine replacement therapy for smoking cessation: differences between men and women. J Consult Clin Psychol (2004) 72:712–22. doi: 10.1037/0022-006X.72.4.712
10. Thompson A, Silverman B, Dzeng L. Psychotropic medications and HIV. Clin Infect Dis (2006) 42:1305–10. doi: 10.1086/501454
11. Cui Q, Robinson L, Elston D, Cohen J, Quan C, McFarland N, et al. Safety and tolerability of varenicline tartrate (Champix((R))/Chantix((R))) for smoking cessation in HIV-infected subjects: a pilot open-label study. AIDS Patient Care STDS (2012) 26:12–9. doi: 10.1089/apc.2011.0199
12. Tornero C, Mafe C. Varenicline and antiretroviral therapy in patients with HIV. J Acquir Immune Defic Syndr (2009) 52:656. doi: 10.1097/QAI.0b013e3181ba1beb
13. Ferketich AK, Diaz P, Browning KK, Lu B, Koletar SL, Reynolds NR, et al. Safety of varenicline among smokers enrolled in the lung HIV study. Nicotine Tob Res (2013) 15:247–54. doi: 10.1093/ntr/nts121[doi
14. Ashare RL, Thompson M, Serrano K, Leone F, Metzger D, Frank I, et al. Placebo-controlled randomized clinical trial testing the efficacy and safety of varenicline for smokers with HIV. Drug Alcohol Depend (2019) 200:26–33. doi: 10.1016/j.drugalcdep.2019.03.011
15. Pacek LR, McClernon FJ, Bosworth HB. Adherence to pharmacological smoking cessation interventions: A literature review and synthesis of correlates and barriers. Nicotine Tob Res (2018) 20:1163–72. doi: 10.1093/ntr/ntx210
16. Hauer L, Scarano GI, Brigo F, Golaszewski S, Lochner P, Trinka E, et al. Effects of repetitive transcranial magnetic stimulation on nicotine consumption and craving: A systematic review. Psychiatry Res (2019) 281:112562. doi: 10.1016/j.psychres.2019.112562
17. Abdelrahman AA, Noaman M, Fawzy M, Moheb A, Karim AA, Khedr EM. A double-blind randomized clinical trial of high frequency rTMS over the DLPFC on nicotine dependence, anxiety and depression. Sci Rep (2021) 11:1640. doi: 10.1038/s41598-020-80927-5
18. Amiaz R, Levy D, Vainiger D, Grunhaus L, Zangen A. Repeated high-frequency transcranial magnetic stimulation over the dorsolateral prefrontal cortex reduces cigarette craving and consumption. Addiction (2009) 104:653–60. doi: 10.1111/j.1360-0443.2008.02448.x
19. Chang D, Zhang J, Peng W, Shen Z, Gao X, Du Y, et al. Smoking cessation with 20 hz repetitive transcranial magnetic stimulation (rTMS) applied to two brain regions: A pilot study. Front Hum Neurosci (2018) 12:344. doi: 10.3389/fnhum.2018.00344
20. Dinur-Klein L, Dannon P, Hadar A, Rosenberg O, Roth Y, Kotler M, et al. Smoking cessation induced by deep repetitive transcranial magnetic stimulation of the prefrontal and insular cortices: a prospective, randomized controlled trial. Biol Psychiatry (2014) 76:742–9. doi: 10.1016/j.biopsych.2014.05.020
21. Eichhammer P, Johann M, Kharraz A, Binder H, Pittrow D, Wodarz N, et al. High-frequency repetitive transcranial magnetic stimulation decreases cigarette smoking. J Clin Psychiatry (2003) 64:951–3. doi: 10.4088/jcp.v64n0815
22. Li X, Hartwell KJ, Henderson S, Badran BW, Brady KT, George MS. Two weeks of image-guided left dorsolateral prefrontal cortex repetitive transcranial magnetic stimulation improves smoking cessation: A double-blind, sham-controlled, randomized clinical trial. Brain Stimul (2020) 13:1271–9. doi: 10.1016/j.brs.2020.06.007
23. Li X, Hartwell KJ, Owens M, Lematty T, Borckardt JJ, Hanlon CA, et al. Repetitive transcranial magnetic stimulation of the dorsolateral prefrontal cortex reduces nicotine cue craving. Biol Psychiatry (2013) 73:714–20. doi: 10.1016/j.biopsych.2013.01.003
24. Pripfl J, Tomova L, Riecansky I, Lamm C. Transcranial magnetic stimulation of the left dorsolateral prefrontal cortex decreases cue-induced nicotine craving and EEG delta power. Brain Stimul (2014) 7(2):226–33. doi: 10.1016/j.brs.2013.11.003
25. Sheffer CE, Bickel WK, Brandon TH, Franck CT, Deen D, Panissidi L, et al. Preventing relapse to smoking with transcranial magnetic stimulation: Feasibility and potential efficacy. Drug Alcohol Depend (2018) 182:8–18. doi: 10.1016/j.drugalcdep.2017.09.037
26. Sheffer CE, Mennemeier M, Landes RD, Bickel WK, Brackman S, Dornhoffer J, et al. Neuromodulation of delay discounting, the reflection effect, and cigarette consumption. J Subst Abuse Treat (2013) 45:206–14. doi: 10.1016/j.jsat.2013.01.012
27. Trojak B, Meille V, Achab S, Lalanne L, Poquet H, Ponavoy E, et al. Transcranial magnetic stimulation combined with nicotine replacement therapy for smoking cessation: A randomized controlled trial. Brain Stimul (2015) 8:1168–74. doi: 10.1016/j.brs.2015.06.004
28. Zangen A, Moshe H, Martinez D, Barnea-Ygael N, Vapnik T, Bystritsky A, et al. Repetitive transcranial magnetic stimulation for smoking cessation: a pivotal multicenter double-blind randomized controlled trial. World Psychiatry (2021) 20:397–404. doi: 10.1002/wps.20905
29. Dieler AC, Dresler T, Joachim K, Deckert J, Herrmann MJ, Fallgatter AJ, et al. Can intermittent theta burst stimulation as add-on to psychotherapy improve nicotine abstinence? Results from a pilot study. Eur Addict Res (2014) 20:248–53. doi: 10.1159/000357941
30. Rose JE, McClernon FJ, Froeliger B, Behm FM, Preud'homme X, Krystal AD, et al. Repetitive transcranial magnetic stimulation of the superior frontal gyrus modulates craving for cigarettes. Biol Psychiatry (2011) 70:794–9. doi: 10.1016/j.biopsych.2011.05.031
31. Blumberger DM, Vila-Rodriguez F, Thorpe KE, Feffer K, Noda Y, Giacobbe P, et al. Effectiveness of theta burst versus high-frequency repetitive transcranial magnetic stimulation in patients with depression (THREE-D): a randomised non-inferiority trial. Lancet (2018) 391:1683–92. doi: 10.1016/S0140-6736(18)30295-2
32. Cole EJ, Phillips AL, Bentzley BS, Stimpson KH, Nejad R, Barmak F, et al. Stanford neuromodulation therapy (SNT): A double-blind randomized controlled trial. Am J Psychiatry (2021) 2021:appiajp202120101429. doi: 10.1176/appi.ajp.2021.20101429
33. Cole EJ, Stimpson KH, Bentzley BS, Gulser M, Cherian K, Tischler C, et al. Stanford accelerated intelligent neuromodulation therapy for treatment-resistant depression. Am J Psychiatry (2020) 177:716–26. doi: 10.1176/appi.ajp.2019.19070720
34. Abellaneda-Perez K, Vaque-Alcazar L, Vidal-Pineiro D, Jannati A, Solana E, Bargallo N, et al. Age-related differences in default-mode network connectivity in response to intermittent theta-burst stimulation and its relationships with maintained cognition and brain integrity in healthy aging. Neuroimage (2019) 188:794–806. doi: 10.1016/j.neuroimage.2018.11.036
35. Agnew ZK, Banissy MJ, McGettigan C, Walsh V, Scott SK. Investigating the neural basis of theta burst stimulation to premotor cortex on emotional vocalization perception: A combined TMS-fMRI study. Front Hum Neurosci (2018) 12:150. doi: 10.3389/fnhum.2018.00150
36. Bation R, Magnin C, Poulet E, Mondino M, Brunelin J. Intermittent theta burst stimulation for negative symptoms of schizophrenia-A double-blind, sham-controlled pilot study. NPJ Schizophr (2021) 7:10. doi: 10.1038/s41537-021-00138-3
37. Halko MA, Farzan F, Eldaief MC. Intermittent theta-burst stimulation of the lateral cerebellum increases functional connectivity of the default network. J Neurosci (2014) 34:12049–56. doi: 10.1523/JNEUROSCI.1776-14.2014
38. Nettekoven C, Volz LJ, Kutscha M, Pool EM, Rehme AK, Eickhoff SB, et al. Dose-dependent effects of theta burst rTMS on cortical excitability and resting-state connectivity of the human motor system. J Neurosci (2014) 34:6849–59. doi: 10.1523/JNEUROSCI.4993-13.2014
39. Nettekoven C, Volz LJ, Leimbach M, Pool EM, Rehme AK, Eickhoff SB, et al. Inter-individual variability in cortical excitability and motor network connectivity following multiple blocks of rTMS. Neuroimage (2015) 118:209–18. doi: 10.1016/j.neuroimage.2015.06.004
40. Vidal-Pineiro D, Martin-Trias P, Arenaza-Urquijo EM, Sala-Llonch R, Clemente IC, Mena-Sanchez I, et al. Task-dependent activity and connectivity predict episodic memory network-based responses to brain stimulation in healthy aging. Brain Stimul (2014) 7:287–96. doi: 10.1016/j.brs.2013.12.016
41. Iwabuchi SJ, Raschke F, Auer DP, Liddle PF, Lankappa ST, Palaniyappan L. Targeted transcranial theta-burst stimulation alters fronto-insular network and prefrontal GABA. Neuroimage (2017) 146:395–403. doi: 10.1016/j.neuroimage.2016.09.043
42. Alkhasli I, Sakreida K, Mottaghy FM, Binkofski F. Modulation of fronto-striatal functional connectivity using transcranial magnetic stimulation. Front Hum Neurosci (2019) 13:190. doi: 10.3389/fnhum.2019.00190
43. Tang Y, Jiao X, Wang J, Zhu T, Zhou J, Qian Z, et al. Dynamic functional connectivity within the fronto-limbic network induced by intermittent theta-burst stimulation: A pilot study. Front Neurosci (2019) 13:944. doi: 10.3389/fnins.2019.00944
44. Li X, Du L, Sahlem GL, Badran BW, Henderson S, George MS, et al. Repetitive transcranial magnetic stimulation (rTMS) of the dorsolateral prefrontal cortex reduces resting-state insula activity and modulates functional connectivity of the orbitofrontal cortex in cigarette smokers. Drug Alcohol Depend (2017) 174:98–105. doi: 10.1016/j.drugalcdep.2017.02.002
45. Li X, Sahlem GL, Badran BW, McTeague LM, Hanlon CA, Hartwell KJ, et al. Transcranial magnetic stimulation of the dorsal lateral prefrontal cortex inhibits medial orbitofrontal activity in smokers. Am J Addict (2017) 26:788–94. doi: 10.1111/ajad.12621
46. Christiansen P, Schoenmakers TM, Field M. Less than meets the eye: reappraising the clinical relevance of attentional bias in addiction. Addict Behav (2015) 44:43–50. doi: 10.1016/j.addbeh.2014.10.005
47. Field M, Marhe R and Franken IH. The clinical relevance of attentional bias in substance use disorders. CNS Spectr (2014) 19:225–30. doi: 10.1017/S1092852913000321
48. Marks KR, Pike E, Stoops WW, Rush CR. The magnitude of drug attentional bias is specific to substance use disorder. Psychol Addict Behav (2015) 29:690–5. doi: 10.1037/adb0000084
49. Robinson JD, Versace F, Engelmann JM, Cui Y, Gilbert DG, Waters AJ, et al. Attentional bias to smoking and other motivationally relevant cues is affected by nicotine exposure and dose expectancy. J Psychopharmacol (2016) 30:627–40. doi: 10.1177/0269881116642879
50. Waters AJ, Shiffman S, Sayette MA, Paty JA, Gwaltney CJ, Balabanis MH, et al. Attentional bias predicts outcome in smoking cessation. Health Psychol (2003) 22:378–87. doi: 10.1037/0278-6133.22.4.378
51. Hanlon CA, Dowdle LT, Correia B, Mithoefer O, Kearney-Ramos T, Lench D, et al. Left frontal pole theta burst stimulation decreases orbitofrontal and insula activity in cocaine users and alcohol users. Drug Alcohol Depend (2017) 178:310–7. doi: 10.1016/j.drugalcdep.2017.03.039
52. Kearney-Ramos TE, Dowdle LT, Lench DH, Mithoefer OJ, Devries WH, George MS, et al. Transdiagnostic effects of ventromedial prefrontal cortex transcranial magnetic stimulation on cue reactivity. Biol Psychiatry Cognit Neurosci Neuroimaging (2018) 3:599–609. doi: 10.1016/j.bpsc.2018.03.016
53. Janes AC, Gilman JM, Radoman M, Fava M, Evins AE. Revisiting the role of the insula and smoking cue-reactivity in relapse: A replication and extension of neuroimaging findings. Drug Alcohol Depend (2017) 179:8–12. doi: 10.1016/j.drugalcdep.2017.06.012
54. Moran-Santa Maria MM, Hartwell KJ, Hanlon CA, Canterberry M, Lematty T, Owens M, et al. Right anterior insula connectivity is important for cue-induced craving in nicotine-dependent smokers. Addict Biol (2015) 20:407–14. doi: 10.1111/adb.12124
55. Morales AM, Ghahremani D, Kohno M, Hellemann GS, London ED. Cigarette exposure, dependence, and craving are related to insula thickness in young adult smokers. Neuropsychopharmacology (2014) 39:1816–22. doi: 10.1038/npp.2014.48
56. Marks KR, Roberts W, Stoops WW, Pike E, Fillmore MT, Rush CR, et al. Fixation time is a sensitive measure of cocaine cue attentional bias. Addiction (2014) 109:1501–8. doi: 10.1111/add.12635
57. Rakesh G, Alcorn JL 3rd, Khanal R, Himelhoch SS, Rush CR. Comparing cigarette-cue attentional bias between people with HIV/AIDS and people with opioid use disorder who smoke. Health Psychol Behav Med (2023) 11(1):2255028. doi: 10.1101/2022.09.30.22280501
58. Rajkowska G, Goldman-Rakic PS. Cytoarchitectonic definition of prefrontal areas in the normal human cortex: II. Variability in locations of areas 9 and 46 and relationship to the Talairach Coordinate System. Cereb Cortex (1995) 5:323–37. doi: 10.1093/cercor/5.4.323
59. Fox MD, Buckner RL, White MP, Greicius MD, Pascual-Leone A. Efficacy of transcranial magnetic stimulation targets for depression is related to intrinsic functional connectivity with the subgenual cingulate. Biol Psychiatry (2012) 72:595–603. doi: 10.1016/j.biopsych.2012.04.028
60. Heishman SJ, Singleton EG, Pickworth WB. Reliability and validity of a short form of the tobacco craving questionnaire. Nicotine Tob Res (2008) 10:643–51. doi: 10.1080/14622200801908174
61. Heatherton TF, Kozlowski LT, Frecker RC, Fagerstrom KO. The fagerstrom test for nicotine dependence: a revision of the fagerstrom tolerance questionnaire. Br J Addict (1991) 86:1119–27. doi: 10.1111/j.1360-0443.1991.tb01879.x
62. Worsley KJ, Cao J, Paus T, Petrides M, Evans AC. Applications of random field theory to functional connectivity. Hum Brain Mapp (1998) 6:364–7. doi: 10.1002/(SICI)1097-0193(1998)6:5/6<364::AID-HBM6>3.0.CO;2-T
63. Chumbley J, Worsley K, Flandin G. Topological FDR for neuroimaging. Neuroimage (2010) 49:3057–64. doi: 10.1016/j.neuroimage.2009.10.090
64. Silvanto J, Muggleton NG. Testing the validity of the TMS state-dependency approach: targeting functionally distinct motion-selective neural populations in visual areas V1/V2 and V5/MT+. Neuroimage (2008) 40:1841–8. doi: 10.1016/j.neuroimage.2008.02.002
65. Hanlon CA. Treating cue-reactivity with brain stimulation: a new (transdiagnostic) approach. Neuropsychopharmacology (2019) 44:232–3. doi: 10.1038/s41386-018-0215-5
66. Janes AC, Pizzagalli DA, Richardt S, Frederick Bde B, Holmes AJ, Sousa J, et al. Neural substrates of attentional bias for smoking-related cues: an FMRI study. Neuropsychopharmacology (2010) 35:2339–45. doi: 10.1038/npp.2010.103
67. Lin X, Deng J, Shi L, Wang Q, Li P, Li H. Neural substrates of smoking and reward cue reactivity in smokers: a meta-analysis of fMRI studies. Transl Psychiatry (2020) 10:97. doi: 10.1038/s41398-020-0775-0
68. Uddin LQ, Supekar K, Amin H, Rykhlevskaia E, Nguyen DA, Greicius MD, et al. Dissociable connectivity within human angular gyrus and intraparietal sulcus: evidence from functional and structural connectivity. Cereb Cortex (2010) 20:2636–46. doi: 10.1093/cercor/bhq011
69. Menon V, Uddin LQ. Saliency, switching, attention and control: a network model of insula function. Brain Struct Funct (2010) 214:655–67. doi: 10.1007/s00429-010-0262-0
70. Uddin LQ, Nomi JS, Hebert-Seropian B, Ghaziri J, Boucher O. Structure and function of the human insula. J Clin Neurophysiol (2017) 34:300–6. doi: 10.1097/WNP.0000000000000377
71. Behrmann M, Geng JJ and Shomstein S. Parietal cortex and attention. Curr Opin Neurobiol (2004) 14:212–7. doi: 10.1016/j.conb.2004.03.012
72. Han S, Jiang Y, Gu H, Rao H, Mao L, Cui Y, et al. The role of human parietal cortex in attention networks. Brain. (2004) 127:650–9. doi: 10.1093/brain/awh071
73. Hartwell KJ, Johnson KA, Li X, Myrick H, LeMatty T, George MS, et al. Neural correlates of craving and resisting craving for tobacco in nicotine dependent smokers. Addict Biol (2011) 16:654–66. doi: 10.1111/j.1369-1600.2011.00340.x
74. Hartwell KJ, Lematty T, McRae-Clark AL, Gray KM, George MS, Brady KT. Resisting the urge to smoke and craving during a smoking quit attempt on varenicline: results from a pilot fMRI study. Am J Drug Alcohol Abuse (2013) 39:92–8. doi: 10.3109/00952990.2012.750665
75. Zhao Q, Li H, Hu B, Li Y, Gillebert CR, Mantini D, et al. Neural correlates of drug-related attentional bias in heroin dependence. Front Hum Neurosci (2017) 11:646. doi: 10.3389/fnhum.2017.00646
76. Sibbald B, Roberts C. Understanding controlled trials. Crossover trials. BMJ (1998) 316:1719. doi: 10.1136/bmj.316.7146.1719
77. Szucs D, Ioannidis JP. Sample size evolution in neuroimaging research: An evaluation of highly-cited studies (1990-2012) and of latest practices (2017-2018) in high-impact journals. Neuroimage (2020) 221:117164. doi: 10.1016/j.neuroimage.2020.117164
78. Button KS, Ioannidis JP, Mokrysz C. Power failure: why small sample size undermines the reliability of neuroscience. Nat Rev Neurosci (2013) 14:365–76. doi: 10.1038/nrn3475
Keywords: rTMS, hiv/aids, smoking, cessation, cigarette cue attentional bias, fMRI
Citation: Rakesh G, Adams TG, Morey RA, Alcorn JL III, Khanal R, Su AE, Himelhoch SS and Rush CR (2024) Intermittent theta burst stimulation and functional connectivity in people living with HIV/AIDS who smoke tobacco cigarettes: a preliminary pilot study. Front. Psychiatry 15:1315854. doi: 10.3389/fpsyt.2024.1315854
Received: 10 October 2023; Accepted: 30 January 2024;
Published: 04 March 2024.
Edited by:
Mauro Ceccanti, Sapienza University of Rome, ItalyReviewed by:
Weronika Dębowska, Medical University of Warsaw, PolandMichael Kritzer-Cheren, Massachusetts General Hospital and Harvard Medical School, United States
Copyright © 2024 Rakesh, Adams, Morey, Alcorn, Khanal, Su, Himelhoch and Rush. This is an open-access article distributed under the terms of the Creative Commons Attribution License (CC BY). The use, distribution or reproduction in other forums is permitted, provided the original author(s) and the copyright owner(s) are credited and that the original publication in this journal is cited, in accordance with accepted academic practice. No use, distribution or reproduction is permitted which does not comply with these terms.
*Correspondence: Gopalkumar Rakesh, Z29wYWxrdW1hci5yYWtlc2hAdWt5LmVkdQ==