- 1School of Health Preservation and Rehabilitation, Chengdu University of Traditional Chinese Medicine, Chengdu, China
- 2Department of Rehabilitation, Mianyang Hospital of Traditional Chinese Medicine, Mianyang, China
- 3Department of Endocrinology and Metabolism, The Affiliated Hospital of Southwest Medical University, Luzhou, China
Background: Depression is a common mental illness that is widely recognized by its lack of pleasure, fatigue, low mood, and, in severe cases, even suicidal tendencies. Photobiomodulation (PBM) is a non-invasive neuromodulation technique that could treat patients with mood disorders such as depression.
Methods: A systematic search of ten databases, including randomized controlled trials (RCTs) for depression, was conducted from the time of library construction to September 25, 2023. The primary outcome was depression. The secondary outcome was sleep. Meta-analysis was performed using RevMan (version 5.4) and Stata (version 14.0). Subgroup analyses were performed to identify sources of heterogeneity. The certainty of the evidence was assessed using the Grading of Recommendations Assessment, Development, and Evaluation (GRADE).
Results: Three thousand two hundred and sixty-five studies were retrieved from the database and screened for inclusion in eleven trials. The forest plot results demonstrated that PBM alleviated depression (SMD = −0.55, 95% CI [−0.75, −0.35], I2 = 46%). But it is not statistically significant for patients’ sleep outcomes (SMD = −0.82, 95% CI [−2.41, 0.77], I2 = 0%, p > 0.05). Subgroup analysis showed that s-PBM was superior to t-PBM in relieving symptoms of depression. The best improvement for t-PBM was achieved using a wavelength of 823 nm, fluence of 10–100 J/cm2, irradiance of 50–100 mW/cm2, irradiance time of 30 min, treatment frequency < 3/week, and number of treatments >15 times. The best improvement for s-PBM was achieved using a wavelength of 808 nm, fluence ≤1 J/cm2, irradiance of 50–100 mW/cm2, irradiance time ≤ 5 min, treatment frequency ≥ 3/week, number of treatments >15 times. All results had evidence quality that was either moderate or very low, and there was no bias in publication.
Conclusion: We conclude that PBM is effective in reducing depression symptoms in patients. However, the current number of studies is small, and further studies are needed to extend the current analysis results.
Systematic Review Registration: https://www.crd.york.ac.uk/PROSPERO/, CRD42023444677.
1. Introduction
Depression is a common mental illness that is widely recognized by its lack of pleasure, fatigue, low mood, and, in severe cases, even suicidal tendencies (1). As reported in 2017 statistics released by the World Health Organization (WHO), 322 million people worldwide suffer from depression, with an overall prevalence rate of 4.4%, including 5.1% for women and 3.6% for men (2). Depression can be a significant burden on an individual’s life, and those who suffer from it are more susceptible to developing cardiovascular disease, long-term diseases such as obesity, and other illnesses caused by lifestyle behaviors (e.g., smoking alcohol abuse) (3, 4). Furthermore, depression is a burden on society due to the 20 times higher than usual suicide rate (5, 6). Sleeping disorders, which are often caused by depression, are often positively correlated with mortality and have a negative impact on patients’ quality of life as the disease progresses (7–9). Pharmacological treatments such as tricyclic antidepressant treatment are still the mainstay of treatment for depression today. However, with less than half of all patients worldwide receiving antidepressant treatment [possibly due to side effects and low patient compliance (10, 11)], treating depression remains difficult and challenging (12).
Photobiomodulation (PBM), also known as low-level laser therapy (LLLT), represents a device-based intervention employing visible and/or near-infrared (NIR) light emitted by lasers or light-emitting diodes (LEDs) to regulate physiological processes (13–16). Our review examines the impact of PBM on brain physiology, with a specific focus on its effects on depression and sleep. The research on PBM’s influence on brain physiology can be broadly categorized by where the light is applied within the body: transcranial PBM (t-PBM) and systemic PBM (s-PBM) (15). t-PBM directly modulates brain physiology by delivering light to the brain (17). At the same time, s-PBM indirectly influences brain physiology by applying light to other body areas, such as acupoints or pain points, thereby inducing systemic physiological changes that subsequently affect brain physiology (13–16). The mechanism of action (MoA) for t-PBM has partially been elucidated - transcranial-delivered light is absorbed by mitochondria in brain cells, normalizing mitochondrial function and restoring energy homeostasis (18–20). Mitochondrial dysfunction has been liked to conditions such as depression (21), sleep disturbances (22), and cognitive impairment (23, 24). Under appropriate light irradiance and luminous flux, red and NIR photons (ranging from 600 nm to 1,164 nm), absorbed by cytochrome c oxidase (CCO) in brain cells, contribute to the normalization of mitochondrial function (25) and an increase in ATP synthesis (18, 26, 27). This, in turn, has beneficial effects on enhancing cognitive and emotional brain functioning (28–31). The precise mechanism (s) by which s-PBM could affect brain physiology is still unclear. However, the beneficial effects of s-PBM on neurons have been well established, even if it is applied to distant locations and not directly to neurons (32). s-PBM can activate circulating immune cells (13, 33–35) or stem cells (36), the mitochondria (37), and the cardiovascular or the lymphatic system, which then leads to an overall increase in the mitochondrial activity to increase (similar to direct stimulation with t-PBM), resulting in a neuroprotective effect (32). In summary, both t-PBM and s-PBM possess neuroprotective effects, and when comparing the two, direct stimulation with t-PBM is more effective, at least in animal models of Parkinson’s (38).
PBM is currently garnering considerable attention in neuropsychiatric disorders (39). Evidence indicates that t-PBM can ameliorate depressive symptoms (40), with potential mediation through enhanced cerebral blood flow (31). For instance, Schiffer et al. (31) found that people with major depressive disorder (MDD) complicated by anxiety experienced significant reductions in depression scores after 2 weeks of t-PBM treatment. Clinical studies have demonstrated that s-PBM irradiation of the back and thighs alleviates depressive symptoms in patients with low back pain (41). s-PBM irradiation of the neck and acupuncture points in patients with alcohol addiction also relieves depressive symptoms (42). The use of s-PBM has been shown to relieve depression in patients with low back pain.
The FDA has cleared PBM for peripheral pain, and it is gaining popularity as a neuromodulation technique (13). Specifically, PBM was utilized to target emotional disturbance in animal models and humans, and it was demonstrated to modify the neural inflammatory profile of rat brains (43–45).
Given the current global disease burden of depression and sleep disorders, few systematic review and Meta-analyses have explored the effects of PBM on depression (46, 47), and even fewer have explored the optimal dose of PBM. Therefore, we attempted to summarize the most compelling evidence from randomized controlled trials using meta-analysis to explore the feasibility of PBM for the treatment of depressive symptoms and to summarize the optimal dose of PBM for the treatment of depressive symptoms.
2. Methods
This study has been registered with PROSPERO, registration number CRD42023444677. It was carried out in accordance with the Preferred Reporting Items for Systematic Reviews and Meta-analyses (PRISMA) (Supplementary Appendix 1) (48).
2.1. Search strategy
From their inceptions to September 25, 2023, two reviewers independently searched PubMed, Embase, the Cochrane Library, Web of Science, ScienceDirect, Psyclnfo, CNKI, VIP, Sinomed, and Wanfang Database. Search terms included Low-Level Light Therapy, Photobiomodulation Therapy, transcranial photobiomodulation, near-infrared light, depression, and randomized controlled trials. In Supplementary Appendix 2, the search formula is displayed in detail. In addition, we manually checked all reference lists of the retrieved papers and questioned specialists to find any potential relevant research.
2.2. Inclusion and exclusion criteria
Studies were chosen if they met the following inclusion criteria: (1) the study design was either a randomized or crossover study; (2) participants had depressive symptoms; (3) patients were being treated using PBM (t-PBM or s-PBM); (4) the studies used widely recognized depression and sleep scales, with a primary outcome of depression and the secondary outcome of sleep.
Excluded were studies that met the following criteria: (1) conference reports, abstracts, animal trials, and replicated research; (2) the experimental group received both PBM and other treatments; (3) the objective data was either missing or could not be extracted, and the full text was unavailable after contacting the corresponding author; (4) studies in which other nondepressive outcomes interfere with the observation of depression efficacy; (5) non-Chinese and English literature.
2.3. Study selection
Endnote 20 was utilized to manage the search record. Two reviewers independently screened titles and abstracts for potentially qualified studies based on the inclusion criteria after deleting duplicate results. They then read the full text of potentially eligible studies to identify the final included literature. In the event of a disagreement, a third reviewer is invited to discuss and make a decision.
2.4. Data collection and extraction
Two reviewers (Q-pJ and S-cY) used the data extraction form to extract the required data from the included studies. The data to be extracted includes first author, time of publication, irradiance, irradiation site, wavelength, fluence, light source, treatment frequency, number of treatments, time of intervention, and outcomes. A third reviewer was asked to discuss and make a judgment call in case of disagreement.
2.5. Risk-of-bias assessment
Using the Cochrane assessment of the risk of bias, two reviewers (Q-pJ and S-cY) independently evaluate the bias risk. The tool divides studies into three categories according to their risk of bias: low, high, or unclear. These categories are selection bias (random sequence generation and allocation concealment), performance bias (blinding of participants and staff), detection bias (blinding of the outcome assessment), attrition bias (incomplete outcome data), reporting bias (selective reporting bias), and other biases. In the event of a dispute, a third reviewer was brought in.
2.6. Certainty of the evidence
The rating of recommendations is grounded in the GRADE (Grading of Recommendations Assessment, Development, and Evaluation) methodology, in which the certainty of evidence is categorized as “very low,” “low,” “medium,” or “high.” High” (49). The quality of randomized controlled trials is high, while the quality of observational studies is low. Research quality can be lowered by five factors: limitations, inconsistency, indirectness, imprecision, and publication bias (50).
2.7. Statistical analysis
The statistical significance threshold was set at p < 0.05, and the data was integrated with RevMan 5.4 and Stata 14.0. Results for this study were continuous variables, and for each effect size, the researchers calculated 95% confidence intervals (CIs). For outcomes assessed using the same scale, the data were pooled using the mean difference (MD), while for outcomes assessed using different scales, the standardized mean difference (SMD) was calculated. Statistical heterogeneity of the included studies was assessed using the chi-square test and the I2 statistical measure. Low, medium, and high heterogeneity existed according to the I2 statistic (25, 50, and 75%). A fixed-effects model was used when p > 0.1 or I2 < 50%. Otherwise, a random effects model was used. Forest plots are used to display the pooled estimations. The estimates were analyzed in subgroups based on age, light source, intervention duration, wavelength, irradiance time, irradiation site, fluence, irradiance, treatment frequency, and number of treatments. Sensitivity analyses for exploring the stability of the study results were present.
2.8. Publication bias
When there were more than ten included studies for the outcome, possible publication bias was assessed by funnel plot, Egger’s regression, and Begg’s regression (p < 0.05 indicates publication bias) (51, 52). If there was a significant publication bias, a Duvaland and Tweedie trim and fill method was used to provide possible missing trials.
3. Results
3.1. Selection and inclusion of studies
An initial search of 3,265 studies was conducted through ten databases. Eleven research studies (53–63) were identified for meta-analysis through multiple screening steps, such as excluding duplicates, selecting the type of literature, systematically reviewing title and abstract contests, and reviewing the full text (Figure 1).
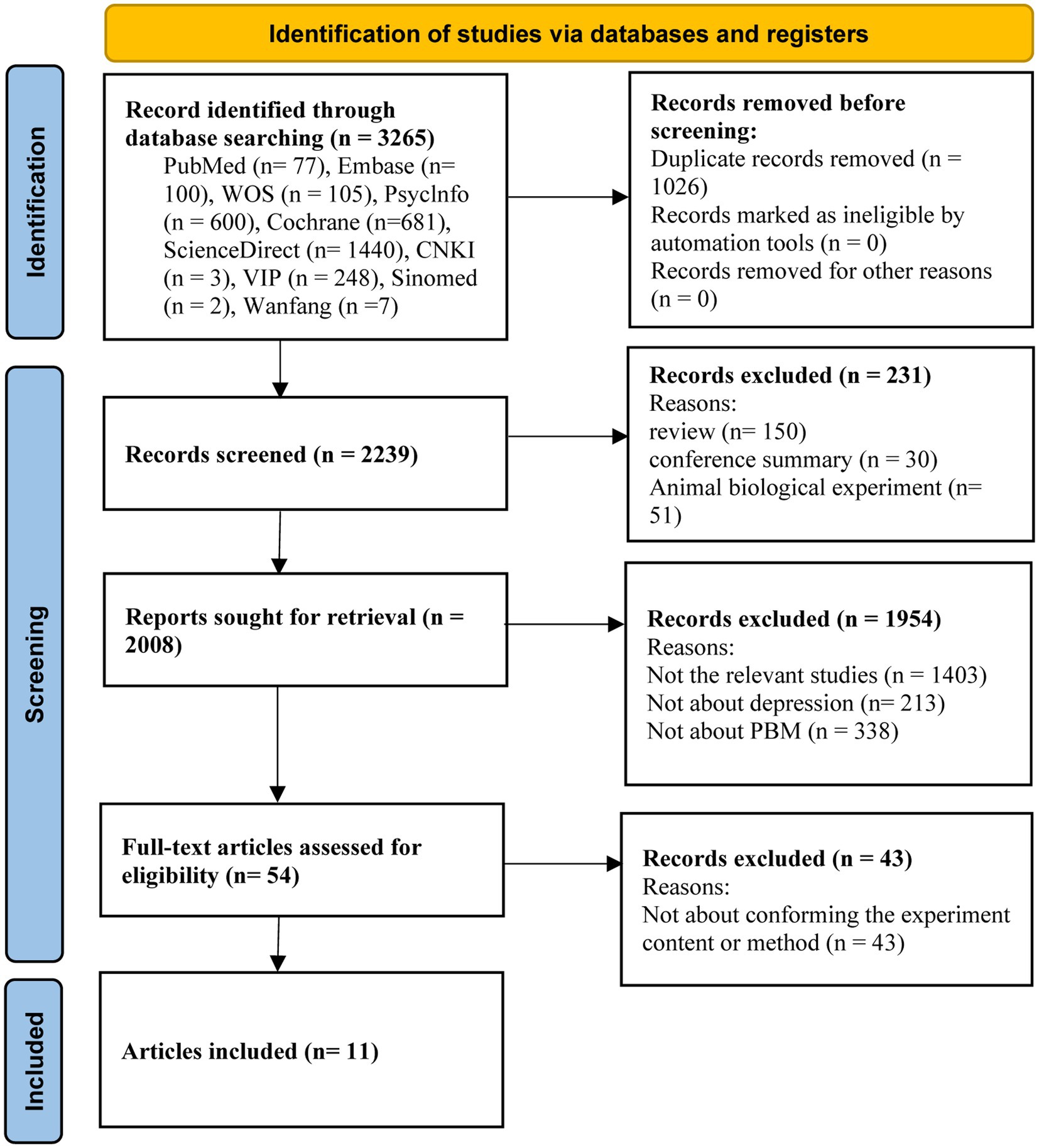
Figure 1. PRISMA flowchart of the literature search for the effects of photobiomodulation on depression.
3.2. Characteristics of included studies
Table 1 presents the characterization of the included trials. A total of eleven studies met our inclusion and exclusion criteria. The study areas were mainly in the United States, Australia, and Turkey, and most participants suffered from varying degrees of depression or other mood-affecting disorders. The participants totaled 407, including 200 in the experimental group and 207 in the control group. Most of the participants were between 30 and 50 years old.
Among the eleven studies, the light interventions differed in light source, Irradiation site, intervention duration, Irradiance, wavelength, fluence, treatment frequency, and number of treatments. The light source was LED in 5 studies (53, 54, 56, 57, 60, 61) and laser in 6 (55, 58, 59, 61–63). Regarding the duration of the interventions, five studies (53, 54, 56, 61, 62) were within 4 weeks, five studies (53–55, 57, 63) were within 6–8 weeks, and one study was within 9–12 weeks (60). The wavelengths of the light ranged from 808 nm to 1,064 nm. By irradiating site, PBM can be categorized into t-PBM and s-PBM, where five studies (53, 54, 56, 60, 61) treated the brain directly (t-PBM) exposing the DIPFC, three (57–59) treated at a tender acupoint, and three in different acupoint (55, 62, 63). Based on treatment frequency, six (53–57, 60) studies treated <3/week and five studies ≥3/week (58, 59, 61–63). Based on the number of treatments, six (55–57, 60–62) studies treated ≤15 times and five studies >15 (53, 54, 58, 59, 63). Regarding the fluence, two studies were within ≤1 J/cm2 (62, 63), three studies within 1–10 J/cm2 (57–59), four studies within 10–100 J/cm2 (53, 54, 56, 60), and two study within 100–1,000 J/cm2 (55, 61). For the irradiance, four studies were within ≤50 mW/cm2 (53, 54, 58, 59), five were within 50–100 mW/cm2 (57, 60–63), and one was 250 mW/cm2 (56).
3.3. Risk of bias in studies
The risk of bias graph for each inclusion study is shown in Figure 2, and the percentages for each study are shown in Figure 3. Of the eleven included studies, all mentioned random sequence generation. Regarding allocation concealment, three studies were assessed as having some problems because details of assignment concealment were not mentioned, and eight studies reported details of allocation concealment. Nine studies mentioned blinding in their studies. Although the studies used different types of interventions, participants and staff were not informed whether they were in the experimental or control group. Three studies did not mention blinding of participants and staff. In addition, incomplete outcome data and selective reporting were not mentioned in any of the studies. Regarding other biases, seven studies were considered to be at low risk, and the remaining four were considered to have some problems.
3.4. Results of the meta-analysis
3.4.1. Depression
A total of eleven trials (53–63) reported on 407 patients with depression. Meta-analysis of fixed effects showed that PBM improved patients’ depressive symptoms compared with controls (SMD = −0.55, 95% CI [−0.75, −0.35], I2 = 46%; Figure 4). Sensitivity analyses showed that exclusion of each study did not affect the stability of our trial (Figure 5).
3.4.1.1. Subgroup analysis
Subgroups were analyzed as shown in Table 2 (supplementing the forest plot in Appendix 4). The light was broadly categorized according to where it was applied in the body: transcranial PBM (t-PBM) and systemic PBM (s-PBM). In terms of improvement in depression, s-PBM was superior to t-PBM. A subgroup analysis of the efficacy of t-PBM on depression showed that adults under 40 years of age showed more significant improvement in depression than adults over 40 years of age; the light source was more effective with LED than with a laser; and an irradiance time of 30 min provided the best improvement in depression; Light with a wavelength of 823 nm is best; fluence of 10–100 J/cm2 is better than 100–1,000 J/cm2 for depression; irradiance ≤50 mW/cm2 is best; treatment frequency < 3/week is best; number of treatments >15 times is the best.
Subgroup analyses of the efficacy of s-PBM on depression showed that s-PBM improved depression better in adults under 40 years old; irradiation site was more effective at the acupoint than at the tender point; light source was more effective at the laser; and irradiance time was best when it was ≤5 min; Wavelength of 808 nm is best for depression; fluence at ≤1 J/cm2 best improves depression; irradiance of 50–100 mW/cm2 is best; treatment frequency ≥ 3/week is best; number of treatments >15 times is best.
3.4.1.2. Publication bias
As shown in Figure 6, publication bias was examined by funnel plot and Egger’s and Begg’s tests. The funnel plot showed basic symmetry with p = 0.547 in Egger’s test and p = 0.533 in Begg’s test, indicating no publication bias (p > 0.05).
3.4.2. Sleep
A total of two trials (55, 57) with 75 patients reported sleep. Meta-analysis of fixed effects showed no statistically significant difference in patient sleep in the PBM (s-PBM) group compared to the control group (SMD = −0.82, 95% CI [−2.41, 0.77], I2 = 0%; p > 0.05; Figure 7).
3.5. Certainty of the evidence
The GRADE results are presented in Supplementary Appendix 3. The level of evidence certainty was rated as “moderate” for depression and ‘very low’ for sleep. The leading causes of the sample size of the included study were too small, and the confidence interval was too broad.
4. Discussion
4.1. The effect of PBM on depression and sleep
A total of eleven randomized controlled trials was included in this meta-analysis, and the combined data suggest that PBM may be an efficacious depression treatment. Neurophysiological studies have confirmed that important pathological causes of depression include neuroinflammation, reduced levels of monoamine neurotransmitters, and mitochondrial dysfunction (64–67). PBM has been increased for the treatment of depression, and it stimulates neuronal processes in the brain as well as CCO, the excitation of which leads to an increase in neuronal ATP synthesis (68). These low-level lasers can modulate nitric oxide (NO) release (68, 69) thereby increasing brain oxygenation and blood flow. In addition to this, PBM modulates neurotransmitter metabolism, reduces neuroinflammation and oxidative stress, and thus acts as a neuroprotective agent (70). Many clinical trials have shown that PBM can be effective in alleviating depressive symptoms. Schiffer et al. (31) used the t-PBM to treat MDD patients suffering from anxiety disorders and traumatic stress disorders, and the research showed a significant improvement in depression and anxiety in the second and fourth weeks.
Regarding the sleep aspect, the results of this study showed no statistically significant effect of PBM on sleep. Therefore, more randomized controlled trials may be needed to determine the role of PBM in sleep. The current study suggests that PBM may improve PSQI scores and sleep quality by modulating the circadian rhythm of cortisol levels (71, 72). Several studies have also reported that PBM improves sleep quality in patients, but the exact mechanism is still under investigation (73–75).
4.2. The prognosis of PBM for the treatment of depression
According to subgroup analyses, t-PBM and s-PBM showed opposite effects on the improvement of depression in terms of age. t-PBM showed better effects in patients aged 40 years or older; the opposite was true for s-PBM. Previous studies have shown that t-PBM affects prefrontal cortical CCO, which plays a key role in mitochondrial respiration (76). Whereas changes in CCO increase with age, older adults may benefit more from t-PBM treatment, which is consistent with our findings (77). Interestingly, s-PBM appeared to be more beneficial for younger participants in our study. This may be because fewer studies included middle-aged and older patients. Few studies have focused on the effects of PBM on depressed patients of different ages, so more research is needed to validate this finding.
PBM has started experimenting with different light sources (laser and LED). Our findings suggest that s-PBM has more dramatic improvements in depression with laser as the light source. This may be because lasers have greater penetration and irradiance than LEDs (78). t-PBM was best with LEDs as a light source, but the effect was insignificant. Although it is debated whether lasers are superior to LEDs, there is evidence that LEDs are comparable to lasers (15) and are safer and less costly (79, 80). t-PBMs are typically available at wavelengths in the 600–1,164 nm region, and wavelengths in this range can stimulate CCO activity more (81). Our results validate this theory, and subgroup analysis showed that PBM at 823 nm and 808 nm wavelengths showed the best improvement in depression. Several studies have confirmed that t-PBM at 808 nm is superior to t-PBM at other wavelengths regarding brain tissue penetration (82). However, according to Mie’s theory, light at 1064 nm scatters more, penetrates more, and may excite more CCOs than at 600–900 nm (82, 83). Therefore, more research is needed to explore the PBM wavelengths most suitable for treating depression.
It has been indicated that depression is associated with the metabolism of the ventral-lateral and dorsal-medial prefrontal cortex (84). In our included study, t-PBM could irradiate the DLPFC region in F3 F4 on the patient’s scalp. This suggests that DLPF may be associated with mechanisms that modulate antidepressant effects. At the same time, s-PBM may have systemic anti-inflammatory, antioxidant, and pro-metabolic effects (85, 86). In our study, s-PBM irradiated patients’ acupoints and tender points. A case–control trial mentioned the possible antidepressant effect of s-PBM on the back and thighs of patients with low back pain combined with depression (41). It is difficult to establish a causal relationship when pain and depression coexist because chronic low back pain may lead to depression, and somatic pain is a common symptom of depression (87). In this trial, five patients with LBP comorbid with depression were treated with physical therapy and s-PBM and retrospectively matched with five control patients who received physical therapy alone. Depression scores decreased significantly more in the s-PBM group than in the control group after treatment. This case–control study demonstrated that s-PBM possesses antidepressant effects. It has been proposed that the systemic effects of s-PBM through the mechanism of blood irradiation can also ultimately be neuroprotective in the brain (88). The brain will benefit from PBM’s ability to down-regulate pro-inflammatory factors and to increase anti-inflammatory cytokines irrespective of the site of treatment (88).
4.3. The dose-dependent effects of PBM
Longer treatment times and higher irradiance PBMs may lead to longer-lasting results (74). In our study, t-PBM with irradiance time of 30 min, treatment frequency < 3/week, and number of treatments >15 times showed the best improvement in depressive symptoms, which is consistent with the above findings; however, subgroup analysis of s-PBM showed that s-PBM was best at irradiance time ≤ 5 min, treatment frequency ≥ 3/week, and number of treatments >15 times. This may be related to the biphasic dose response of PBM, i.e., PBM regimens based on excessive energy density (J/cm2), excessive irradiance (mW/cm2), or excessive number of repetitions with longer duration are significantly less effective than t-PBM regimens based on lower doses (89, 90). The biphasic response has been demonstrated several times in PBM studies (91, 92). Fluence-based subgroup analyses suggest that t-PBM (10–100 J/cm2) and s-PBM (1–10 J/cm2) may have higher antidepressant effects. This may be because most of the photons of t-PBM are absorbed or dispersed by the scalp, skull, and cerebrospinal fluid, and only a small portion may reach the neural tissues (93). Thus, a greater fluence is required to achieve an antidepressant effect. t-PBM at 0–50 mW/cm2 possesses a more substantial antidepressant effect than t-PBM at 50–100 mW/cm2, which the biphasic response of the PBM can also explain. The biphasic response of PBM has been verified in various in vitro and in vivo studies and animal models (94). This phenomenon suggests that excessive irradiance and excessively long irradiation times may have inhibitory effects. Various studies have shown that low to moderate doses of PBM promote cell growth, whereas high intensities counteract the beneficial effects of PBM on various cell types (90).
In summary, the dose of t-PBM can be considered: irradiance time of 30 min, fluence of 10–100 J/cm2, irradiance ≤50 mW/cm2, treatment frequency < 3/week, number of treatments >15 times; Dose of s-PBM can be considered: irradiance time ≤ 5 min, fluence of ≤1 J/cm2, irradiance of 50–100 mW/cm2, treatment frequency ≥ 3/week, number of treatments >15 times. However, due to the limited number of studies, the optimal regimen and potential influences of PBM for depression have not been determined, and more rigorously designed randomized controlled trials are needed to address these issues.
4.4. Limitations of this study
There are some potential limitations of our study. Firstly, the small sample size of the included studies may affect the accuracy of the results. Second, some of the included studies did not explicitly report the blinding method, which may present a risk of bias. Finally, significant heterogeneity may result from the different locations, doses, and wavelengths of PBM irradiation in the studies we included.
5. Conclusion
In conclusion, we conclude that PBM effectively improves depression in patients. Although it is not possible to determine the specific parameters for obtaining the best results, the following can be considered in this study: t-PBM wavelength selection 823 nm, fluence selection 10–100 J/cm2, irradiance selection 50–100 mW/cm2, irradiance time selection 30 min, treatment frequency < 3/week, number of treatments >15 times; s-PBM wavelength selection 808 nm, fluence selection ≤1 J/cm2, irradiance selection 50–100 mW/cm2, irradiance time ≤ 5 min, treatment frequency ≥ 3/week, number of treatments >15 times. However, the current number of studies is low, and further studies are needed to extend the current analysis. More rigorous randomized controlled trials are used to understand PBM’s physiological mechanisms and beneficial effects on depression.
Data availability statement
The original contributions presented in the study are included in the article/Supplementary material, further inquiries can be directed to the corresponding authors.
Author contributions
QJ: Writing – original draft, Writing – review & editing. SY: Writing – review & editing. JD: Writing – review & editing. XZ: Writing – review & editing. ZL: Writing – original draft. TZ: Writing – review & editing. ZW: Writing – review & editing. WW: Writing – review & editing. HL: Writing – review & editing. SL: Writing – review & editing. SA: Writing – original draft, Writing – review & editing.
Funding
The author(s) declare financial support was received for the research, authorship, and/or publication of this article. This study was supported by the Youth Fund Program of the Sichuan Provincial Science and Technology Department (2023NSFSC1827), the Sichuan Province Science and Technology Department key research and development project (2023YFS0337), and Chengdu University of Traditional Chinese Medicine 2020 “Apricot Grove Scholars” Hospital Special (YYZX2020034).
Conflict of interest
The authors declare that the research was conducted in the absence of any commercial or financial relationships that could be construed as a potential conflict of interest.
Publisher’s note
All claims expressed in this article are solely those of the authors and do not necessarily represent those of their affiliated organizations, or those of the publisher, the editors and the reviewers. Any product that may be evaluated in this article, or claim that may be made by its manufacturer, is not guaranteed or endorsed by the publisher.
Supplementary material
The Supplementary material for this article can be found online at: https://www.frontiersin.org/articles/10.3389/fpsyt.2023.1267415/full#supplementary-material
References
1. Mirzaei, M, Yasini Ardekani, SM, Mirzaei, M, and Dehghani, A. Prevalence of depression, anxiety and stress among adult population: results of Yazd health study. Iran J Psychiatry. (2019) 14:137–46. doi: 10.18502/ijps.v14i2.993
2. Global, Regional, and National Incidence. Prevalence, and years lived with disability for 354 diseases and injuries for 195 countries and territories, 1990-2017: a systematic analysis for the global burden of disease study 2017. Lancet. (2018) 392:1789–858. doi: 10.1016/s0140-6736(18)32279-7
3. Cohen, BE, Edmondson, D, and Kronish, IM. State of the art review: depression, stress, anxiety, and cardiovascular disease. Am J Hypertens. (2015) 28:1295–302. doi: 10.1093/ajh/hpv047
4. Strine, TW, Mokdad, AH, Balluz, LS, Gonzalez, O, Crider, R, Berry, JT, et al. Depression and anxiety in the United States: findings from the 2006 behavioral risk factor surveillance system. Psychiatr Serv. (2008) 59:1383–90. doi: 10.1176/ps.2008.59.12.1383
5. Li, H, Chen, Z, Gong, Q, and Jia, Z. Voxel-wise Meta-analysis of task-related brain activation abnormalities in major depressive disorder with suicide behavior. Brain Imaging Behav. (2020) 14:1298–308. doi: 10.1007/s11682-019-00045-3
6. Otte, C, Gold, SM, Penninx, BW, Pariante, CM, Etkin, A, Fava, M, et al. Major depressive disorder. Nat Rev Dis Primers. (2016) 2:16065. doi: 10.1038/nrdp.2016.65
7. Li, Y, Zhang, X, Winkelman, JW, Redline, S, Hu, FB, Stampfer, M, et al. Association between insomnia symptoms and mortality: a prospective study of U.S. Men Circulation. (2014) 129:737–46. doi: 10.1161/circulationaha.113.004500
8. Mc Carthy, CE. Sleep disturbance, sleep disorders and co-morbidities in the Care of the Older Person. Med Sci (Basel). (2021) 9:31. doi: 10.3390/medsci9020031
9. Rod, NH, Kumari, M, Lange, T, Kivimäki, M, Shipley, M, and Ferrie, J. The joint effect of sleep duration and disturbed sleep on cause-specific mortality: results from the Whitehall ii cohort study. PLoS One. (2014) 9:e91965. doi: 10.1371/journal.pone.0091965
10. Locke, AB, Kirst, N, and Shultz, CG. Diagnosis and management of generalized anxiety disorder and panic disorder in adults. Am Fam Physician. (2015) 91:617–24.
11. Guideline Development Panel for the Treatment of Depressive Disorders. Summary of the clinical practice guideline for the treatment of depression across three age cohorts. Am Psychol. (2022) 77:770–80. doi: 10.1037/amp0000904
12. Sowa-Kućma, M, Pańczyszyn-Trzewik, P, Misztak, P, Jaeschke, RR, Sendek, K, Styczeń, K, et al. Vortioxetine: a review of the pharmacology and clinical profile of the novel antidepressant. Pharmacol Rep. (2017) 69:595–601. doi: 10.1016/j.pharep.2017.01.030
13. Chung, H, Dai, T, Sharma, SK, Huang, YY, Carroll, JD, and Hamblin, MR. The nuts and bolts of low-level laser (light) therapy. Ann Biomed Eng. (2012) 40:516–33. doi: 10.1007/s10439-011-0454-7
14. Gonzalez-Lima, F, and Barrett, DW. Augmentation of cognitive brain functions with transcranial lasers. Front Syst Neurosci. (2014) 8:36. doi: 10.3389/fnsys.2014.00036
15. Hamblin, MR. Shining light on the head: photobiomodulation for brain disorders. BBA Clin. (2016) 6:113–24. doi: 10.1016/j.bbacli.2016.09.002
16. Wong-Riley, MT, Liang, HL, Eells, JT, Chance, B, Henry, MM, Buchmann, E, et al. Photobiomodulation directly benefits primary neurons functionally inactivated by toxins: role of cytochrome C oxidase. J Biol Chem. (2005) 280:4761–71. doi: 10.1074/jbc.M409650200
17. Salehpour, F, Khademi, M, Bragin, DE, and DiDuro, JO. Photobiomodulation therapy and the Glymphatic system: promising applications for augmenting the brain lymphatic drainage system. Int J Mol Sci. (2022) 23:2975. doi: 10.3390/ijms23062975
18. Mochizuki-Oda, N, Kataoka, Y, Cui, Y, Yamada, H, Heya, M, and Awazu, K. Effects of near-infra-red laser irradiation on adenosine triphosphate and adenosine diphosphate contents of rat brain tissue. Neurosci Lett. (2002) 323:207–10. doi: 10.1016/s0304-3940(02)00159-3
19. Oron, A, Oron, U, Streeter, J, de Taboada, L, Alexandrovich, A, Trembovler, V, et al. Low-level laser therapy applied Transcranially to mice following traumatic brain injury significantly reduces long-term neurological deficits. J Neurotrauma. (2007) 24:651–6. doi: 10.1089/neu.2006.0198
20. Yu, W, Naim, JO, McGowan, M, Ippolito, K, and Lanzafame, RJ. Photomodulation of oxidative metabolism and Electron chain enzymes in rat liver mitochondria. Photochem Photobiol. (1997) 66:866–71. doi: 10.1111/j.1751-1097.1997.tb03239.x
21. Tripathi, A, Scaini, G, Barichello, T, Quevedo, J, and Pillai, A. Mitophagy in depression: pathophysiology and treatment targets. Mitochondrion. (2021) 61:1–10. doi: 10.1016/j.mito.2021.08.016
22. Andreazza, AC, Andersen, ML, Alvarenga, TA, De-Oliveira, MR, Armani, F, Ruiz, FS, et al. Impairment of the mitochondrial electron transport chain due to sleep deprivation in mice. J Psychiatr Res. (2010) 44:775–80. doi: 10.1016/j.jpsychires.2010.01.015
23. Mutisya, EM, Bowling, AC, and Beal, MF. Cortical cytochrome oxidase activity is reduced in Alzheimer's disease. J Neurochem. (1994) 63:2179–84. doi: 10.1046/j.1471-4159.1994.63062179.x
24. Parker, WD Jr. Cytochrome oxidase deficiency in Alzheimer's disease. Ann N Y Acad Sci. (1991) 640:59–64. doi: 10.1111/j.1749-6632.1991.tb00191.x
25. Yang, F, Qi, X, Gao, Z, Yang, X, Zheng, X, Duan, C, et al. Homocysteine injures vascular endothelial cells by inhibiting mitochondrial activity. Exp Ther Med. (2016) 12:2247–52. doi: 10.3892/etm.2016.3564
26. de Freitas, LF, and Hamblin, MR. Proposed mechanisms of Photobiomodulation or low-level light therapy. IEEE J Sel Top Quantum Electron. (2016) 22:348–64. doi: 10.1109/jstqe.2016.2561201
27. Lapchak, PA, and De Taboada, L. Transcranial near infrared laser treatment (Nilt) increases cortical Adenosine-5′-triphosphate (Atp) content following embolic strokes in rabbits. Brain Res. (2010) 1306:100–5. doi: 10.1016/j.brainres.2009.10.022
28. Nizamutdinov, D, Qi, X, Berman, MH, Dougal, G, Dayawansa, S, Wu, E, et al. Transcranial near infrared light stimulations improve cognition in patients with dementia. Aging Dis. (2021) 12:954–63. doi: 10.14336/ad.2021.0229
29. Rojas, JC, and Gonzalez-Lima, F. Neurological and psychological applications of transcranial lasers and Leds. Biochem Pharmacol. (2013) 86:447–57. doi: 10.1016/j.bcp.2013.06.012
30. Barrett, DW, and Gonzalez-Lima, F. Transcranial infrared laser stimulation produces beneficial cognitive and emotional effects in humans. Neuroscience. (2013) 230:13–23. doi: 10.1016/j.neuroscience.2012.11.016
31. Schiffer, F, Johnston, AL, Ravichandran, C, Polcari, A, Teicher, MH, Webb, RH, et al. Psychological benefits 2 and 4 weeks after a single treatment with near infrared light to the forehead: a pilot study of 10 patients with major depression and anxiety. Behav Brain Funct. (2009) 5:46. doi: 10.1186/1744-9081-5-46
32. Moro, C, Valverde, A, Dole, M, Hoh Kam, J, Hamilton, C, Liebert, A, et al. The effect of photobiomodulation on the brain during wakefulness and sleep. Front Neurosci. (2022) 16:942536. doi: 10.3389/fnins.2022.942536
33. Byrnes, KR, Waynant, RW, Ilev, IK, Wu, X, Barna, L, Smith, K, et al. Light promotes regeneration and functional recovery and alters the immune response after spinal cord injury. Lasers Surg Med. (2005) 36:171–85. doi: 10.1002/lsm.20143
34. Muili, KA, Gopalakrishnan, S, Meyer, SL, Eells, JT, and Lyons, JA. Amelioration of experimental autoimmune encephalomyelitis in C57bl/6 mice by photobiomodulation induced by 670 nm light. PLoS One. (2012) 7:e30655. doi: 10.1371/journal.pone.0030655
35. Saliba, A, Du, Y, Liu, H, Patel, S, Roberts, R, Berkowitz, BA, et al. Photobiomodulation mitigates diabetes-induced retinopathy by direct and indirect mechanisms: evidence from intervention studies in pigmented mice. PLoS One. (2015) 10:e0139003. doi: 10.1371/journal.pone.0139003
36. Farfara, D, Tuby, H, Trudler, D, Doron-Mandel, E, Maltz, L, Vassar, RJ, et al. Low-level laser therapy ameliorates disease progression in a mouse model of Alzheimer's disease. J Mol Neurosci. (2015) 55:430–6. doi: 10.1007/s12031-014-0354-z
37. Al Amir Dache, Z, Otandault, A, Tanos, R, Pastor, B, Meddeb, R, Sanchez, C, et al. Blood contains circulating cell-free respiratory competent mitochondria. FASEB J. (2020) 34:3616–30. doi: 10.1096/fj.201901917RR
38. Johnstone, DM, el Massri, N, Moro, C, Spana, S, Wang, XS, Torres, N, et al. Indirect application of near infrared light induces neuroprotection in a mouse model of parkinsonism – an Abscopal neuroprotective effect. Neuroscience. (2014) 274:93–101. doi: 10.1016/j.neuroscience.2014.05.023
39. Hamblin, MR. Photobiomodulation and the brain – has the light dawned? Biochemist. (2016) 38:24–8. doi: 10.1042/bio03806024
40. Cassano, P, Cusin, C, Mischoulon, D, Hamblin, MR, De Taboada, L, Pisoni, A, et al. Near-infrared transcranial radiation for major depressive disorder: proof of concept study. Psychiatry J. (2015) 2015:352979:1–8. doi: 10.1155/2015/352979
41. Gabel, CP, Petrie, SR, Mischoulon, D, Hamblin, MR, Yeung, A, Sangermano, L, et al. A case control series for the effect of photobiomodulation in patients with low back pain and concurrent depression. Laser Ther. (2018) 27:167–73. doi: 10.5978/islsm.27_18-OR-18
42. Zalewska-Kaszubska, J, and Obzejta, D. Use of low-energy laser as adjunct treatment of alcohol addiction. Lasers Med Sci. (2004) 19:100–4. doi: 10.1007/s10103-004-0307-9
43. Cardoso, FS, De Souza Oliveira Tavares, C, BHS, A, Mansur, F, RÁB, L-M, and Gomes Da Silva, S. Improved spatial memory and neuroinflammatory profile changes in aged rats submitted to photobiomodulation therapy. Cell Mol Neurobiol. (2022) 42:1875–86. doi: 10.1007/s10571-021-01069-4
44. Rhie, SJ, Jung, EY, and Shim, I. The role of neuroinflammation on pathogenesis of affective disorders. J Exerc Rehabil. (2020) 16:2–9. doi: 10.12965/jer.2040016.008
45. Zhang, D, Shen, Q, Wu, X, and Xing, D. Photobiomodulation therapy ameliorates glutamatergic dysfunction in mice with chronic unpredictable mild stress-induced depression. Oxidative Med Cell Longev. (2021) 2021:6678276–20. doi: 10.1155/2021/6678276
46. Cassano, P, Petrie, SR, Hamblin, MR, Henderson, TA, and Iosifescu, DV. Review of transcranial photobiomodulation for major depressive disorder: targeting brain metabolism, inflammation, oxidative stress, and neurogenesis. Neurophotonics. (2016) 3:031404. doi: 10.1117/1.NPh.3.3.031404
47. Cho, Y, Tural, U, and Iosifescu, DV. Efficacy of transcranial photobiomodulation on depressive symptoms: a Meta-analysis. Photobiomodul Photomed Laser Surg. (2023) 41:460–6. doi: 10.1089/photob.2023.0041
48. Page, MJ, McKenzie, JE, Bossuyt, PM, Boutron, I, Hoffmann, TC, Mulrow, CD, et al. The Prisma 2020 statement: an updated guideline for reporting systematic reviews. BMJ. (2021) 372:n71. doi: 10.1136/bmj.n71
49. Balshem, H, Helfand, M, Schünemann, HJ, Oxman, AD, Kunz, R, Brozek, J, et al. Grade guidelines: 3. Rating the quality of evidence. J Clin Epidemiol. (2011) 64:401–6. doi: 10.1016/j.jclinepi.2010.07.015
50. Guyatt, GH, Oxman, AD, Schünemann, HJ, Tugwell, P, and Knottnerus, A. Grade guidelines: a new series of articles in the journal of clinical epidemiology. J Clin Epidemiol. (2011) 64:380–2. doi: 10.1016/j.jclinepi.2010.09.011
51. Begg, CB, and Mazumdar, M. Operating characteristics of a rank correlation test for publication Bias. Biometrics. (1994) 50:1088–101. doi: 10.2307/2533446
52. Irwig, L, Macaskill, P, Berry, G, and Glasziou, P. Bias in meta-analysis detected by a simple, graphical test. Graphical test is itself biased. BMJ. (1998) 316:469. doi: 10.1136/bmj.316.7129.469
53. Cassano, P, Dording, C, Thomas, G, Foster, S, Yeung, A, Uchida, M, et al. Effects of transcranial photobiomodulation with near-infrared light on sexual dysfunction. Lasers Surg Med. (2019) 51:127–35. doi: 10.1002/lsm.23011
54. Cassano, P, Petrie, SR, Mischoulon, D, Cusin, C, Katnani, H, Yeung, A, et al. Transcranial photobiomodulation for the treatment of major depressive disorder. The Elated-2 pilot trial. Photomed Laser Surg. (2018) 36:634–46. doi: 10.1089/pho.2018.4490
55. Chen, CK, Lin, YC, Cheng, JW, Pei, YC, Liu, GH, Chen, YL, et al. Effectiveness of laser acupuncture in alleviating chronic insomnia: a single-blinded randomized controlled trial. Evid Based Complement Alternat Med. (2019) 2019:8136967–9. doi: 10.1155/2019/8136967
56. Disner, SG, Beevers, CG, and Gonzalez-Lima, F. Transcranial laser stimulation as neuroenhancement for attention bias modification in adults with elevated depression symptoms. Brain Stimul. (2016) 9:780–7. doi: 10.1016/j.brs.2016.05.009
57. Ferrandez-Pujante, A, Pons-Fuster, E, and López-Jornet, P. Efficacy of photobiomodulation in reducing symptomatology and improving the quality of life in patients with xerostomia and hyposalivation: a randomized controlled trial. J Clin Med. (2022) 11:3414. doi: 10.3390/jcm11123414
58. Gür, A, Karakoc, M, Nas, K, Cevik, R, Sarac, J, and Ataoglu, S. Effects of low power laser and low dose amitriptyline therapy on clinical symptoms and quality of life in fibromyalgia: a single-blind, placebo-controlled trial. Rheumatol Int. (2002) 22:188–93. doi: 10.1007/s00296-002-0221-z
59. Gur, A, Sarac, AJ, Cevik, R, Altindag, O, and Sarac, S. Efficacy of 904 nm gallium arsenide low level laser therapy in the management of chronic myofascial pain in the neck: a double-blind and randomize-controlled trial. Lasers Surg Med. (2004) 35:229–35. doi: 10.1002/lsm.20082
60. Iosifescu, DV, Norton, RJ, Tural, U, Mischoulon, D, Collins, K, McDonald, E, et al. Very low-level transcranial photobiomodulation for major depressive disorder: the Elated-3 multicenter, randomized, sham-controlled trial. J Clin Psychiatry. (2022) 83:21m14226. doi: 10.4088/JCP.21m14226
61. Kheradmand, A, Tabeie, F, Seif, P, Rezaei, O, and Yasamy, MT. Effect of low-level laser therapy (LLLT) on cognitive impairment among patients with chronic schizophrenia: a double-blind randomized placebo-controlled clinical trial. Lasers Med Sci. (2022) 37:2717–25. doi: 10.1007/s10103-022-03545-9
62. Quah-Smith, I, Smith, C, Crawford, JD, and Russell, J. Laser acupuncture for depression: a randomised double blind controlled trial using low intensity laser intervention. J Affect Disord. (2013) 148:179–87. doi: 10.1016/j.jad.2012.11.058
63. Quah-Smith, JI, Tang, WM, and Russell, J. Laser acupuncture for mild to moderate depression in a primary care setting – a randomised controlled trial. Acupunct Med. (2005) 23:103–11. doi: 10.1136/aim.23.3.103
64. Baldessarini, RJ. The basis for amine hypotheses in affective disorders. A critical evaluation. Arch Gen Psychiatry. (1975) 32:1087–93. doi: 10.1001/archpsyc.1975.01760270019001
65. Dantzer, R, O'Connor, JC, Freund, GG, Johnson, RW, and Kelley, KW. From inflammation to sickness and depression: when the immune system subjugates the brain. Nat Rev Neurosci. (2008) 9:46–56. doi: 10.1038/nrn2297
66. Maes, M, Yirmyia, R, Noraberg, J, Brene, S, Hibbeln, J, Perini, G, et al. The inflammatory & neurodegenerative (I&Nd) hypothesis of depression: leads for future research and new drug developments in depression. Metab Brain Dis. (2009) 24:27–53. doi: 10.1007/s11011-008-9118-1
67. Wang, B, Shi, H, Yang, B, Miao, Z, Sun, M, Yang, H, et al. The mitochondrial Ahi1/GR participates the regulation on mtDNA copy numbers and brain ATP levels and modulates depressive behaviors in mice. Cell Commun Signal. (2023) 21:21. doi: 10.1186/s12964-022-01034-8
68. Karu, TI, and Kolyakov, SF. Exact action spectra for cellular responses relevant to phototherapy. Photomed Laser Surg. (2005) 23:355–61. doi: 10.1089/pho.2005.23.355
69. Morries, LD, Cassano, P, and Henderson, TA. Treatments for traumatic brain injury with emphasis on transcranial near-infrared laser phototherapy. Neuropsychiatr Dis Treat. (2015) 11:2159–75. doi: 10.2147/ndt.S65809
70. Salehpour, F, Farajdokht, F, Cassano, P, Sadigh-Eteghad, S, Erfani, M, Hamblin, MR, et al. Near-infrared photobiomodulation combined with coenzyme Q(10) for depression in a mouse model of restraint stress: reduction in oxidative stress, neuroinflammation, and apoptosis. Brain Res Bull. (2019) 144:213–22. doi: 10.1016/j.brainresbull.2018.10.010
71. Figueiro, MG, Plitnick, BA, Lok, A, Jones, GE, Higgins, P, Hornick, TR, et al. Tailored lighting intervention improves measures of sleep, depression, and agitation in persons with Alzheimer's disease and related dementia living in long-term care facilities. Clin Interv Aging. (2014) 9:1527–37. doi: 10.2147/cia.S68557
72. Ortuño-Lizarán, I, Esquiva, G, Beach, TG, Serrano, GE, Adler, CH, Lax, P, et al. Degeneration of human photosensitive retinal ganglion cells may explain sleep and circadian rhythms disorders in Parkinson's disease. Acta Neuropathol Commun. (2018) 6:90. doi: 10.1186/s40478-018-0596-z
73. Maiello, M, Losiewicz, OM, Bui, E, Spera, V, Hamblin, MR, Marques, L, et al. Transcranial photobiomodulation with near-infrared light for generalized anxiety disorder: a pilot study. Photobiomodul Photomed Laser Surg. (2019) 37:644–50. doi: 10.1089/photob.2019.4677
74. Naeser, MA, Zafonte, R, Krengel, MH, Martin, PI, Frazier, J, Hamblin, MR, et al. Significant improvements in cognitive performance post-transcranial, red/near-infrared light-emitting diode treatments in chronic, mild traumatic brain injury: open-protocol study. J Neurotrauma. (2014) 31:1008–17. doi: 10.1089/neu.2013.3244
75. Saltmarche, AE, Naeser, MA, Ho, KF, Hamblin, MR, and Lim, L. Significant improvement in cognition in mild to moderately severe dementia cases treated with transcranial plus intranasal photobiomodulation: case series report. Photomed Laser Surg. (2017) 35:432–41. doi: 10.1089/pho.2016.4227
76. Gonzalez-Lima, F, Barksdale, BR, and Rojas, JC. Mitochondrial respiration as a target for neuroprotection and cognitive enhancement. Biochem Pharmacol. (2014) 88:584–93. doi: 10.1016/j.bcp.2013.11.010
77. Saucedo, CL, Courtois, EC, Wade, ZS, Kelley, MN, Kheradbin, N, Barrett, DW, et al. Transcranial laser stimulation: mitochondrial and cerebrovascular effects in younger and older healthy adults. Brain Stimul. (2021) 14:440–9. doi: 10.1016/j.brs.2021.02.011
78. Pruitt, T, Carter, C, Wang, X, Wu, A, and Liu, H. Photobiomodulation at different wavelengths boosts mitochondrial redox metabolism and hemoglobin oxygenation: lasers vs. light-emitting diodes in vivo. Metabolites. (2022) 12:103. doi: 10.3390/metabo12020103
79. Buendía, D, Guncay, T, Oyanedel, M, Lemus, M, Weinstein, A, Ardiles, ÁO, et al. The transcranial light therapy improves synaptic plasticity in the Alzheimer's disease mouse model. Brain Sci. (2022) 12:1272. doi: 10.3390/brainsci12101272
80. Hamblin, MR. Photobiomodulation for Alzheimer's disease: has the light dawned? Photo-Dermatology. (2019) 6:77. doi: 10.3390/photonics6030077
81. Kolyva, C, Tachtsidis, I, Ghosh, A, Moroz, T, Cooper, CE, Smith, M, et al. Systematic investigation of changes in oxidized cerebral cytochrome C oxidase concentration during frontal lobe activation in healthy adults. Biomed Opt Express. (2012) 3:2550–66. doi: 10.1364/boe.3.002550
82. Jacques, SL. Optical properties of biological tissues: a review. Phys Med Biol. (2013) 58:R37–61. doi: 10.1088/0031-9155/58/11/r37
83. Tedford, CE, DeLapp, S, Jacques, S, and Anders, J. Quantitative analysis of transcranial and Intraparenchymal light penetration in human cadaver brain tissue. Lasers Surg Med. (2015) 47:312–22. doi: 10.1002/lsm.22343
84. Kennedy, SH, Konarski, JZ, Segal, ZV, Lau, MA, Bieling, PJ, McIntyre, RS, et al. Differences in brain glucose metabolism between responders to Cbt and venlafaxine in a 16-week randomized controlled trial. Am J Psychiatry. (2007) 164:778–88. doi: 10.1176/ajp.2007.164.5.778
85. Hamper, M, Cassano, P, and Lombard, J. Treatment of Kleine-Levin syndrome with intranasal Photobiomodulation and methylene blue. Cureus. (2021) 13:e18596. doi: 10.7759/cureus.18596
86. Salehpour, F, Gholipour-Khalili, S, Farajdokht, F, Kamari, F, Walski, T, Hamblin, MR, et al. Therapeutic potential of intranasal photobiomodulation therapy for neurological and neuropsychiatric disorders: a narrative review. Rev Neurosci. (2020) 31:269–86. doi: 10.1515/revneuro-2019-0063
87. Kamper, SJ, Apeldoorn, AT, Chiarotto, A, Smeets, RJ, Ostelo, RW, Guzman, J, et al. Multidisciplinary biopsychosocial rehabilitation for chronic low back pain. Cochrane Database Syst Rev. (2014) 9:Cd000963. doi: 10.1002/14651858.CD000963.pub3
88. Hennessy, M, and Hamblin, MR. Photobiomodulation and the brain: a new paradigm. J Opt. (2017) 19:013003. doi: 10.1088/2040-8986/19/1/013003
89. Huang, YY, Chen, AC, Carroll, JD, and Hamblin, MR. Biphasic dose response in low level light therapy. Dose Response. (2009) 7:358–83. doi: 10.2203/dose-response.09-027.Hamblin
90. Huang, YY, Sharma, SK, Carroll, J, and Hamblin, MR. Biphasic dose response in low level light therapy - an update. Dose Response. (2011) 9:602–18. doi: 10.2203/dose-response.11-009.Hamblin
91. Lanzafame, RJ, Stadler, I, Kurtz, AF, Connelly, R, Peter, TA Sr, Brondon, P, et al. Reciprocity of exposure time and irradiance on energy density during photoradiation on wound healing in a murine pressure ulcer model. Lasers Surg Med. (2007) 39:534–42. doi: 10.1002/lsm.20519
92. Oron, U, Yaakobi, T, Oron, A, Hayam, G, Gepstein, L, Rubin, O, et al. Attenuation of infarct size in rats and dogs after myocardial infarction by low-energy laser irradiation. Lasers Surg Med. (2001) 28:204–11. doi: 10.1002/lsm.1039
93. Jagdeo, JR, Adams, LE, Brody, NI, and Siegel, DM. Transcranial red and near infrared light transmission in a cadaveric model. PLoS One. (2012) 7:e47460. doi: 10.1371/journal.pone.0047460
Keywords: photobiomodulation, t-PBM, s-PBM, low-level laser therapy, depression, sleep, meta-analysis
Citation: Ji Q, Yan S, Ding J, Zeng X, Liu Z, Zhou T, Wu Z, Wei W, Li H, Liu S and Ai S (2024) Photobiomodulation improves depression symptoms: a systematic review and meta-analysis of randomized controlled trials. Front. Psychiatry. 14:1267415. doi: 10.3389/fpsyt.2023.1267415
Edited by:
Yuanjun Xie, Fourth Military Medical University, ChinaReviewed by:
Zhidan Wang, Jiangsu Normal University, ChinaZhaowen Liu, Massachusetts General Hospital and Harvard Medical School, United States
Luis De Taboada, Jelikalite, Inc., United States
Copyright © 2024 Ji, Yan, Ding, Liu, Liu, Zeng, Zhou, Wei, Wu, Li and Ai. This is an open-access article distributed under the terms of the Creative Commons Attribution License (CC BY). The use, distribution or reproduction in other forums is permitted, provided the original author(s) and the copyright owner(s) are credited and that the original publication in this journal is cited, in accordance with accepted academic practice. No use, distribution or reproduction is permitted which does not comply with these terms.
*Correspondence: Shuangchun Ai, aishuangchun@163.com; Shuangyue Liu, shuangyue_liu@hotmail.com;
†These authors have contributed equally to this work and share first authorship