- Department of Neurobiology and Behavior, University of California, Irvine, Irvine, CA, United States
Electronic cigarette use has dramatically increased over the last decade. With this recent technological development and wide range of constituents in various products, putative adverse effects on the brain and body have been largely unexplored. Here, we review current evidence linking electronic nicotine cigarette use with potential health consequences and provide evidence supporting an association between drug use and depression in humans. We also examine the biological effects of individual constituents in electronic cigarette aerosols, which include labeled ingredients, such as propylene glycol, vegetable glycerin, nicotine, and flavorants, as well as unlabeled ingredients found in the aerosols, such as carbonyls and heavy metals. Lastly, we examine the effects of electronic cigarette use on endogenous metabolism via changes in cytochrome P450 enzymes, which can thereby impact therapeutic outcomes. While the current evidence offers insight into the potential effects of electronic cigarette use on biological processes, further studies are necessary to determine the long-term clinical relevance of aerosol inhalation.
Introduction
“We don’t think a lot about addiction here […] anything about health is not on our mind” – statement by a JUUL electronic cigarette engineer (1)
Since tobacco cigarette use continues to be a main cause of preventable death in the US and worldwide (2), significant efforts have been focused on developing smoking alternatives and therapeutics to support long-term cessation (3, 4). Initially developed as an innovative smoking cessation tool, electronic cigarettes have become increasingly popular among adult smokers, in addition to an unfortunate prevalence of use among adolescents and never-smokers (5, 6). This phenomenon may be attributed to how electronic cigarettes have evolved over time. Specifically, electronic cigarettes have become more inconspicuous, looking less like a tobacco cigarette, and their improved liquid solvents have been formulated to decrease the harshness of the smoking experience. Through a heating coil, electronic cigarettes aerosolize a liquid solvent, which is then inhaled by the user. Commercial liquids for electronic cigarettes contain nicotine and various constituents/flavorants dissolved in a vehicle solution, which is often propylene glycol and vegetable glycerin (7). However, additional constituents are also often present in electronic cigarette liquid and aerosol (7, 8), thus exposing the smoker to potentially numerous harmful substances.
In adult tobacco smokers, electronic cigarettes have been shown to be beneficial as cessation aids, but ultimately, the scientific evidence has indicated limited efficacy to reduce tobacco use over the long-term (9–14). It is important to note that electronic cigarette use among adolescents and never-smokers is increasingly prevalent, with reports indicating that use has more than doubled between 2017 and 2019 in Americans aged 16–19 (15). Commercial electronic cigarette products are continuing to evolve across time which may lead to increased intensity of use, for instance based on increasing nicotine levels, pH modifications, and potentially other design features. Importantly, electronic cigarette use has a high comorbidity with increased self-reported depression and anxiety symptoms (16, 17) Therefore, the association between electronic cigarettes and mental health will likely continue to evolve over time, especially given ease of inconspicuous use in home environments, school settings, and workplaces. It has become essential to understand how electronic cigarette use correlates with mental health, the impact on the brain’s neurobiology, and the potential impact on pharmaceutical therapies related to mental health. In this review, we examine the effects of individual constituents emitted from electronic cigarettes and the potential intersections with health. We further discuss how metabolic processes can be disrupted by constituents in electronic cigarettes, thereby impacting the effectiveness of pharmacological therapeutics used to treat mental health disorders, such as depression.
Factors influencing electronic cigarette use
Electronic cigarette harm perception
A multitude of factors influence the likelihood to initiate electronic cigarette use (18). Not surprisingly, both adolescent and adult electronic cigarette users are more likely to start smoking tobacco cigarettes within 2 years, as compared to non-users (19, 20). Because of this, it is especially important to understand factors that may predict the onset of electronic cigarette use in never-smokers. For instance, electronic cigarette perception was shown to be a predictor of smoking initiation amongst adolescents and adults (21, 22). Therefore, by deepening our understanding of how different sociodemographic factors and perception interrelate, vulnerable populations can be identified to prevent future initiation of drug use.
The relationship between sociodemographic factors and electronic cigarette use in adolescents is well established. In a recent survey, adolescents between 13 and 18 years of age were found to vary in their perceived harm of electronic cigarettes based on socioeconomic status, ethnic background, and parental educational attainment (23). Specifically, a lower perceived risk of electronic cigarettes was associated with a lower-income family, non-Hispanic Black ethnic background, or both parents having had no college degree (23). Furthermore, adolescents and young adult never users who were non-Hispanic Black reported a greater susceptibility to initiate disposable or reusable electronic cigarette use (24). As adolescents age, perceptions toward electronic cigarettes may persist and thus influence their likelihood of nicotine use. Not surprisingly, the internet and social media have become a powerful source of influence for both adolescents and young adults. Indeed, the advertisement of electronic cigarettes has been mainly found in online sources (25). For instance, a large number of electronic cigarette vaping-related videos are available on TikTok, a short form social media platform popular among adolescents and young adults (26). These vaping-related TikTok videos were largely found to positively depict electronic cigarette use (26). This is alarming given that advertising via social media significantly influences the perception of electronic cigarette harm in adolescents, even when controlling for exposure to warning labels and anti-tobacco advertising (27). Conversely, this highlights the potential by which social media can be used to communicate the harms of electronic cigarette use to these same adolescent and young adult populations.
Interestingly, a study in young adults found that advertising alone did not influence electronic cigarette use, but when individuals who had a low harm perception of electronic cigarettes viewed this advertising, they were then more likely to initiate electronic cigarette use (25). These findings are consistent with another study in adults, in which harm perception of electronic cigarettes in non-users could predict their status a year later (28). Specifically, increased harm perception of electronic cigarettes was significantly correlated with a lower incidence of future electronic cigarette use, whereas decreased harm perception was associated with increased likelihood of drug use (28). Given the recent regulations in over 30 states targeting electronic cigarette advertising, sales, and use (29), it will be interesting to see how electronic cigarette perceptions in adolescents and adults evolve to influence future use patterns.
Depression and anxiety
Over 20 million adults in the United States suffer from depression (30), and multiple studies have demonstrated comorbidity between depression and electronic cigarette use (16, 31–39). This association is also dose-dependent, where greater amounts of nicotine consumed were associated with greater self-reported depressive symptoms (40). Adolescents and adults who suffer from mental illness are more likely to be both electronic and tobacco cigarette users (14, 41–44), so these populations are disproportionately burdened with health consequences associated with both types of cigarettes. Interestingly, electronic cigarette use was associated with subsequent tobacco cigarette initiation, which then correlated with depression symptoms (39), illustrating that the initial use of electronic cigarettes in never-smokers can lead to adverse health consequences. Additionally, self-report studies reveal that never smokers and smokers who have quit report overall higher levels of positive affect and lower levels of negative affect compared to current tobacco smokers (45, 46). Although prior studies provide some insight for the intersection of nicotine use and depression, it had remained unclear as to whether symptoms related to depression led to the initiation of drug use, or if the effects of drug use (e.g., alterations in brain circuitry and/or induction of withdrawal symptoms during periods of abstinence) triggered the onset of depressive symptomology. Two longitudinal studies have investigated this question regarding electronic cigarette use, yielding somewhat conflicting results (18, 47). In the first study (47), college students were surveyed at two time points within 1 year, assessing for changes in electronic cigarette use and self-reported depressive symptoms. In this sample, reported symptoms of depression predicted electronic cigarette use at both 6 month and 1 year follow-ups, whereas electronic cigarette use did not predict depressive symptoms at either time point (47). These data suggest an unidirectional relationship, in which greater depressive symptomology increases the likelihood of electronic cigarette use (47). These data also support the “self-medication” hypothesis, in which people experiencing depression may seek out substances to ameliorate their symptoms. Given that nicotine in electronic cigarettes has been shown to induce transient effects of mild euphoria, increased energy, heightened arousal and relaxation, these effects can theoretically counteract an individual’s perceived depression-associated symptoms (48). Of note, this phenomenon has also been observed in tobacco cigarette users (49, 50). Contrary to these unidirectional effects (47), an interesting longitudinal study by Leventhal and colleagues provides evidence of a bidirectional relationship between nicotine use and depression (18). This study surveyed high school students for 1 year who reported never having used any nicotine products and assessed their self-reported depression symptoms and electronic cigarette use (18). Participants with a self-reported elevation of depression-associated symptoms at baseline were more likely to use both electronic cigarettes and tobacco cigarettes (18), consistent with the former study (47). However, the authors also found that sustained electronic cigarette use predicted an increase in self-reported depression symptoms at 12 months, thereby revealing a bidirectional relationship between depressive symptoms and electronic cigarettes (18). As these two studies suggest differences in directionality between depression and electronic cigarette use, it is important to consider factors mitigating these conclusions. For instance, differences in age, education, and geographic location have all been shown to affect nicotine use (4), and thus, the reported findings may be interdependent on other sociodemographic or developmental factors. In addition, the dose of nicotine contained in the electronic cigarettes or amount consumed by the participants was not recorded or standardized, which may have confounded the correlation with depressive symptoms. Finally, self-reported symptoms are subjective and may not meet the criteria for major depressive disorder (51).
Often closely associated with depression, electronic cigarette use is also positively correlated with both self-reported anxiety symptoms and generalized anxiety disorder (GAD) in humans (17, 52–56). While the directionality of this association is unclear, like depression, the relationship between anxiety and electronic cigarette use may also be bidirectional. Evidence demonstrates that people with a greater score as assessed with the GAD survey are more likely to initiate future electronic cigarette use (17). Further, adolescent populations self-report vaping for relaxation and stress and anxiety coping (54). Conversely, electronic cigarette use may increase the risk of anxiety disorders, including phobias, obsessive–compulsive disorder, or a panic disorder (57). Among adolescents and young adults, electronic cigarette use increased the likelihood of anxiety-related disorders by 37% (57). In addition to electronic cigarette initiation and continued use, increased anxiety is also evidenced during nicotine withdrawal (58). Interestingly, individuals that self-report greater anxiety sensitivity experience greater barriers for cessation (56) and individuals with depression diagnoses are more likely to experience more severe withdrawal symptoms (59). All in all, these findings suggest an intertwined relationship between electronic cigarette use, anxiety, and depression, which put those already experiencing these disorders at greater risk for electronic cigarette use, difficulties in cessation, and increased withdrawal symptomology.
Studying drug exposure in animal models
Preclinical research in animal models, particularly rodents, allows researchers to investigate the effects of drugs and chemical constituents in a controlled setting. A wide range of techniques are available to study drug exposure, including both passive and self-administration methods. Passive (a.k.a., experimenter-administered) exposure allows for the control of both dose and time of administration relative to other outcome measures (e.g., examination of brain activation after a discrete time period) (60). This method, however, eliminates the motivational aspects of dependence. In contrast, intravenous or vapor self-administration protocols allow for the examination of the motivational and reinforcing drug properties that lead to continued use and seeking behaviors, allowing researchers to investigate different aspects of addiction processes. Both intravenous nicotine and aerosolized nicotine self-administration assays have been established for both rats and mice (61–67). Intravenous nicotine self-administration is considered the most reliable and robust method to study nicotine dependence, craving, and withdrawal (68). Intravenous nicotine self-administration also allows for the precise quantification of the amount of nicotine infused by the animal (69), but there are translational limits with this approach as humans typically inhale most nicotine-containing products. Recently, vapor nicotine self-administration paradigms have been developed (61, 62, 70), in which animals inhale aerosolized nicotine to more closely mimic human nicotine consumption. However, measurement of the net amount of nicotine inhaled with vapor self-administration is not feasible; while blood samples can be used to examine nicotine and its metabolites, the time course of nicotine metabolism in rodents limits the accuracy of detection with exposure across a self-administration session duration (e.g., 1+ hour). However, electronic cigarette exposure can lead to clinically relevant pharmacokinetics that translate to human biology (71). To date, rodent vapor self-administration paradigms have been demonstrated to be less robust than intravenous self-administration; associations between drug reward and active versus inactive behavioral responding have been inconsistent across published experimental paradigms (62, 65). Nevertheless, both intravenous and vapor nicotine self-administration paradigms are valuable tools to investigate various aspects of nicotine dependence, and established protocols demonstrate blood levels of nicotine’s metabolites, cotinine, 5-hydroxycotinine and cotinine N-oxide, similar to that found in human smokers (60, 62, 65, 72–74).
Many rodent models have been used to assess a wide range of behaviors related to symptoms of psychiatric disorders (75). For instance, depression-like behaviors have been classically measured using behavioral despair tests, such as forced swim and tail suspension, where quicker immobilization times are inferred to be an indicator of behavioral despair (75). Anxiety-like behavior in rodents is often assessed using open field and elevated plus maze tests, in which more time spent in the periphery of open field or in the closed arms of the elevated plus maze are associated with anxiety-like effects (76). Behavioral assays can also be used to quantify motivational and consummatory behaviors, in which decreased reward consumption or motivation to obtain palatable food reward is considered an indicator of anhedonia (75). Intracranial self-stimulation (ICSS) can also serve as an assessment of anhedonia or aversive state, in which rodents in an aversive state have been found to press a lever or spin a wheel to obtain higher levels of brain electrical self-stimulation (77, 78). While these tools have proven useful in measuring depression- and anxiety-associated states in animals, translational limitations are present when trying to extrapolate to mental health disorders in humans, given complex cognitive and social factors contributing to the human psychological state. Therefore, behavioral assessments with rodent models can be used to examine the reinforcing, rewarding, and cognitive effects of a variety of constituents found in electronic cigarettes. For instance, data from rodents support findings from human studies associating electronic cigarette exposure with anxiety- and depression-associated behaviors (79–81). Specifically, withdrawal from nicotine was shown to induce persistent changes in anxiety-, depression- and compulsive-like behaviors following 7 weeks of electronic cigarette exposure (79, 80), and surprisingly, these behavioral changes persisted 90 days following the last electronic cigarette exposure in male mice (79). Behavioral changes have also been observed even in the absence of nicotine, as nicotine-free electronic cigarette vapor exposure was shown to result in anxiogenic phenotypes in both male and female mice (81). With these apparent behavioral changes associated with electronic cigarette exposure, additional animal model research studies are needed to specifically elucidate the neurobiological mechanisms driving, and resulting from, electronic cigarette use. In the following sections, we will review the impact of various constituents on biological processes with data derived from both in vitro and in vivo studies.
Health consequences and neurobiological effects of electronic cigarettes constituents
Electronic cigarettes have been promoted as a safer alternative to tobacco cigarettes, which has led to increased product use among various populations (25–28). However, electronic cigarette liquid and aerosols can pose many health risks to users (Figure 1). Further, while there is significant overlap in gene expression changes after electronic cigarette or tobacco cigarette use, there are several genes that are distinctly altered by electronic cigarette vapor and therefore may pose distinct health risks (82). Likely attributed to the lack of federal regulation, unlabeled components have been identified in electronic cigarette liquids with nuclear magnetic resonance spectroscopy analysis (83). Significant differences in labeled nicotine and actual nicotine content have also been found in commercially available electronic cigarette liquids, ranging from 35% less nicotine than what was labeled, up to 30% more nicotine than labeled (84). Alarmingly, nicotine has also been detected in electronic cigarette cartridges labeled as “nicotine-free” (84–86). These discrepancies between labeled and measured nicotine content in electronic cigarette liquids pose a significant risk to never smokers, who report a greater desire to use nicotine-free electronic cigarettes in comparison to nicotine-containing electronic cigarettes (87). Indeed, a study in Norway found that adolescents were three times more likely to vape nicotine-free electronic cigarettes as compared to nicotine-containing electronic cigarettes (88). While about half of nicotine-free electronic cigarette users from this cohort were able to quit the following year, it is important to note that from 2017 to 2019, about 15% of users each year transitioned from nicotine-free to nicotine-containing electronic cigarettes (88). Considering that nicotine has been detected in electronic cigarette liquids labeled as nicotine-free, these users may have unknowingly consumed nicotine, thereby priming their subsequent transition to nicotine-containing electronic cigarettes.
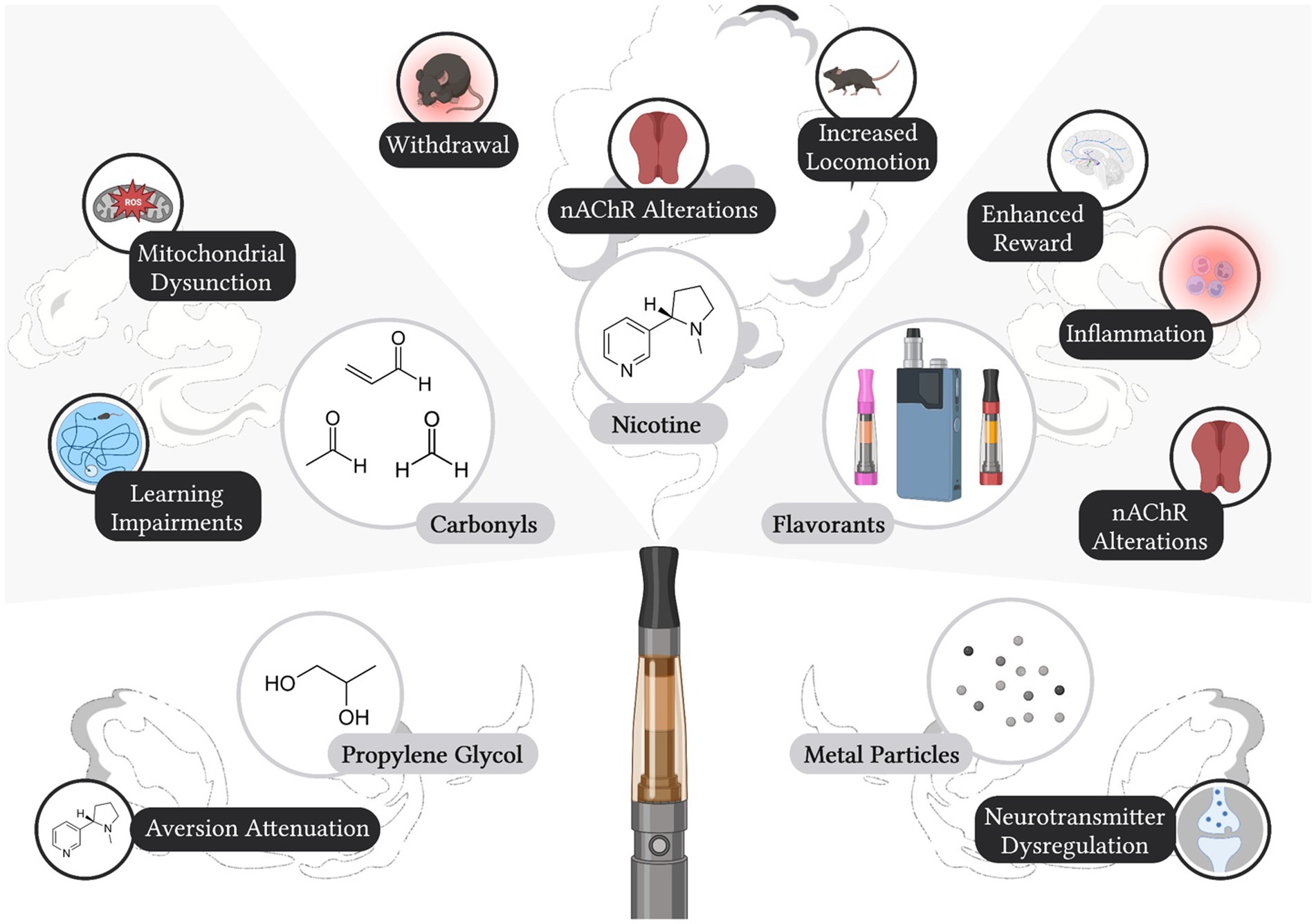
Figure 1. Labeled and Unlabeled Constituents in Electronic Cigarettes and Identified Effects on Neurobiology and Behavior. Electronic cigarette liquids and/or aerosols have been shown to contain nicotine, propylene glycol, carbonyls, flavorants, and metal particulates, all of which can induce effects on biological processes and/or behavior. Created with Biorender.com.
Nicotine
Nicotine is the main psychoactive constituent present in both tobacco cigarettes and electronic cigarette liquid. Nicotine acts on ionotropic nicotinic acetylcholine receptors (nAChRs), where ligand binding results in channel pore opening and cation influx across the membrane (89). nAChRs are pentameric, assembling with various combinations of α and β nAChR subunits for a wide variety of nAChR subtypes with distinct pharmacokinetics, expression patterns, and actions on the cholinergic system (90, 91). Acting on nAChRs, nicotine exerts its reinforcing properties through the mesolimbic dopaminergic pathway (92). Systemic nicotine administration results in dopamine release in the nucleus accumbens (93), an effect shown to be mediated by β2* nAChRs in the ventral tegmental area (92, 94). Subsequent studies have demonstrated that the α4α6β2* nAChRs are necessary for nicotine self-administration in rodents (94). Moreover, nicotine also exerts aversive properties, particularly though the medial habenula to interpeduncular nucleus pathway (95). The medial habenula is enriched in α5*, α3*, β4* nAChRs, which have been shown to mediate nicotine aversion, drug-taking behavior, and/or withdrawal (96–101).
Nicotine vape solutions
Propylene glycol and vegetable glycerin are additives commonly used as humectants (e.g., to control moisture levels in food and beauty products) and are the main liquids used to dissolve nicotine into solution to generate electronic cigarette aerosol for inhalation. Electronic cigarette solutions are marked commercially as “nicotine juice,” “e-juice,” “nicotine liquid,” or “nicotine solution.” Although the Food and Drug Administration recognizes propylene glycol and vegetable glycerin as “generally safe” for oral consumption, they have not yet been approved for inhalation (102). With electronic cigarette development and use significantly increasing within the last decade (4), the potential long-term effects of inhaled propylene glycol, vegetable glycerin, or both together on the brain and body have yet to be fully elucidated. Although propylene glycol and vegetable glycerin are both used in the electronic cigarette solutions, studies have focused on propylene glycol or the combined effects of vegetable glycerin and propylene glycol, rather than investigating vegetable glycerin alone.
Propylene glycol has been shown to interact with other compounds present in electronic cigarettes to induce synergistic effects. For example, HEK-293T cells exposed to both propylene glycol and vanilla electronic cigarette flavorant exhibited increased calcium signaling, which was attributed to activation of the aldehyde-sensitive receptor TRPA1 (103). Specifically, at higher concentrations, vanilla flavorant and propylene glycol together induced more robust calcium influx than either alone (103). TRPA1 receptors are expressed in brain microvasculature and play a functional role to maintain blood–brain-barrier integrity (104). Since acute exposure to non-flavored electronic cigarette aerosol was also shown to activate TRPA1 receptors in endothelial cells, this suggests that combined effects of propylene glycol and vanilla chemical flavorant may potentiate the adverse effect of each other or may exert greater side effects in vivo (105). Moreover, propylene glycol has also been shown to interact with nicotine to affect brain reward thresholds in male rats, as assessed with ICSS (78). While propylene glycol alone did not alter ICSS thresholds, co-administration of propylene glycol and nicotine decreased the aversion-associated increase in ICSS thresholds, which was induced by the high dose of nicotine alone (78). These findings indicate that at doses found in commercial electronic cigarette liquids, propylene glycol mitigates nicotine’s aversive properties and thus may promote higher levels of nicotine consumption (78), thereby increasing the product’s addiction liability. Maternal electronic cigarette exposure has also been shown to affect offspring. Surprisingly, prenatal exposure to vegetable glycerin with propylene glycol vapor was sufficient to induce deficits in long-term novel object memory (106), thus highlighting the importance of understanding the impact of electronic cigarette exposure in utero.
Carbonyls
When heated together, propylene glycol and vegetable glycerin decompose to generate carbonyls in the aerosol, which most notably include acrolein, acetaldehyde, and formaldehyde (107–109). Many studies have found detectable levels of carbonyls in electronic cigarette aerosol, but variable levels have been reported across these studies, likely due to differences in the vape solution’s pH, heating temperature, propylene glycol/vegetable glycerin ratios, and individual differences in user vaping behavior (109–112). Importantly, in humans, these carbonyls can be detected in the airway following electronic cigarette use (113), and their respective metabolites can be further detected in the urine (108, 114, 115). While it is important to acknowledge that electronic cigarettes emit significantly fewer carbonyls than tobacco cigarettes (116), limited studies have investigated the level of carbonyl emission from electronic cigarettes, nor have they investigated the implications with long-term exposure.
Both acetaldehyde and acrolein are considered neurotoxins due to their effects on oxidative stress, which has been proposed to underlie neurodegenerative diseases including Alzheimer’s and Parkinson’s (117–119). Acetaldehyde and acrolein have been detected in both nicotine-free and nicotine-containing electronic cigarette aerosol, but interestingly, they are often not detected in the liquids themselves (86, 109, 120). These findings indicate that the process of heating the chemicals in the electronic cigarette liquid causes the formation of the aerosolized carbonyls. Metabolites of acetaldehyde and acrolein can also be detected in the urine following electronic cigarette vapor exposure (108, 115). Interestingly, both acetaldehyde and acrolein similarly impair cellular respiration. Acetaldehyde was shown to alter cellular respiration in cultured microvascular endothelial hBMVEC cells, which mainly comprise the blood–brain-barrier, thereby suggesting negative effects on blood–brain-barrier integrity (121).Further, in cultured primary cortical neurons, acetaldehyde impaired mitochondrial respiration via NOX-mediated activation (122), and in brain mitochondria in vitro, acrolein inhibited mitochondrial respiration via complex I (123). Similar effects have also been observed in vivo, as 3 days of electronic cigarette vapor exposure resulted in NOX2-mediated changes in mitochondrial respiration in the frontal cortex of male mice (124). In this study, acrolein was proposed to induce the NOX2-mediated impairments in cellular respiration (124). Interestingly, a study in humans revealed that current electronic cigarette use, but not past electronic cigarette use, was significantly correlated with mitochondrial DNA damage and dysfunction (82), which supports the translational relevance of these pre-clinical findings. Together, these findings indicate the acute nature of electronic cigarette exposure in mitigating negative outcomes.
In addition to acetaldehyde and acrolein, formaldehyde is also a product of propylene glycol and vegetable glycerin degradation (107–109). Formaldehyde is a well-documented carcinogen associated with adverse health consequences following acute exposure at higher doses, in addition to chronic lower levels of exposure (125). Formaldehyde levels in electronic cigarette aerosol have been documented to range from 0.07–0.15 parts per million (ppm), which is below the 5 ppm threshold for acute toxicity (126). Nevertheless, evidence demonstrates that chronic exposure to even low levels of formaldehyde can induce significant behavioral and molecular changes. For example, 7 days of gaseous exposure to a low dose of formaldehyde impaired spatial learning in the Morris water maze in male mice (127, 128). Furthermore, a chronically administered low dose of formaldehyde altered monoamine levels, including norepinephrine, epinephrine, dopamine, and serotonin, in the brain of male mice (127). Specifically, following 7 days of formaldehyde exposure at levels much lower than those emitted from electronic cigarettes, 0.0005 ppm formaldehyde exposure decreased norepinephrine and epinephrine levels, whereas 0.003 ppm decreased all of the monoamine levels (127). Moreover, 12 weeks of exposure of a low dose of aerosolized formaldehyde induced an upregulation in the number of corticotropin releasing hormone immunoreactive neurons in the paraventricular nucleus of female mice (129). Interestingly, an increased number of corticotropin releasing hormone neurons in the paraventricular nucleus has been observed in individuals diagnosed with major depressive disorder, as compared to healthy controls (130), suggesting clinical relevance of the findings from mice. Taken together, these studies provide evidence that long-term formaldehyde exposure at levels similar to electronic cigarette emission can lead to significant changes in brain mechanisms underlying cognitive function, potentially including depression.
Metals
Electronic cigarettes have been considered ‘safer’ than combustible tobacco cigarettes, such as with lower carbonyl levels. However, a main counterindication of this ‘safe’ assessment is the presence of inhaled metal particulates in electronic cigarette aerosols, which are at levels greater than that found in tobacco cigarette smoke (131, 132). Metals are likely leached into electronic cigarette liquids and vape during aerosol production, when electronic cigarette liquids come into contact with the metal heating coil (133). Like carbonyls, these metals can also be detected in the urine, saliva, exhaled breath, and blood of electronic cigarette users (131, 133). Electronic cigarette-emitted metal particulates have been shown to readily cross the blood–brain-barrier, as evidenced by metal accumulation in the mouse brain (134). Moreover, following electronic cigarette exposure, arsenic, chromium, copper, iron, manganese, nickel, lead, selenium, strontium, and zinc were found to accumulate across different regions of the brain, with the greatest accumulation observed in the anterior frontal cortex and striatum (134). Specifically, in the anterior frontal cortex, copper and strontium were enriched, whereas arsenic, chromium, copper, iron, lead, and selenium were enriched in the striatum (134). Like other types of electronic cigarette emissions, the type and relative proportion of metals that might accumulate in the brain are expected to vary among commercially available brands due to differences in the vape liquid constituents and electronic cigarette device characteristics (135). Of note, metal inhalation has been shown to induce behavioral changes in male rats, including a decrease in locomotor activity following chromium inhalation (136). Both arsenic and lead are well-known neurotoxins, with the greatest negative effects occurring during earlier neurodevelopmental stages. Maternal exposure to either arsenic or lead during gestation or developmental exposure during adolescence has been shown to result in an overall increase in monoamine signaling in the brain, which persisted into adulthood in rats (137). Unexpectedly, co-exposure of both arsenic and lead led to an opposing effect with decreased monoamine signaling (137). These data highlight the potential differential effects of each constituent and unknown effects with multiple metals present in the aerosols. This illustrates the need to expand our understanding of the effects of metals in electronic cigarettes for various stages of neurodevelopment. It is also important to note that metal components are often found in higher concentrations in less expensive products (24, 138–140), which would presumably be purchased at higher levels by those of lower socioeconomic status or adolescent users with limited income. Given this, future research should be directed at investigating whether increased health disparities will become more evident with long-term product use by marginalized communities of lower socioeconomic status.
Electronic cigarette flavorants
As the electronic cigarette market constantly evolves, more palatable flavorants consistently emerge to attract a broader audience of consumers. In 2014, a study identified over 7,000 commercially available electronic cigarette flavors (141), which does not take into account the many variations of chemicals used to create a singular flavor (e.g., fruit or candy). Mint and fruit electronic cigarette flavors are preferred by individuals across ages (young adult to adult) and smoking status (never, current, or former electronic cigarette/tobacco cigarette user) (142–144). Thus, an enhanced understanding of how the chemicals used to generate the variety of electronic cigarette flavorants impact the reinforcing properties of nicotine, drug use patterns, and health outcomes will be essential to ascertain. However, this has been difficult for the scientific community given the numerous chemical combinations used to generate the flavors and limited time/resources as commercialized flavors constantly evolve from company to company and across time.
Mentholated products
One of the most consistent findings for the impact of flavorants on nicotine product use is the effect of menthol. More positive attitudes have been reported toward mint/menthol flavored electronic cigarettes compared to tobacco flavored electronic cigarettes, which was evidenced by an increased reported satisfaction and increased likelihood to repeat use (145). In those who smoke mentholated tobacco cigarettes, decreased smoking cessation rates are found (146), indicating that mentholated products have increased addiction liability. Unfortunately, due to focused marketing by companies, individuals that are younger and/or from marginalized ethnic backgrounds disproportionately use menthol-containing products (146–149), which has led to notable health disparities within our society. Findings in humans have been supported by rodent models that demonstrate menthol enhances the rewarding and reinforcing properties of nicotine. For instance, administration of menthol-flavored electronic cigarette extract reduced a conditioned taste aversion, as compared to nicotine alone, in a two-bottle test with male and female adolescent rats (150). Following menthol administration, male rats self-administered more intravenous nicotine infusions and increased their motivation to obtain nicotine (151), and male mice self-administered electronic cigarette vapor containing menthol and nicotine at greater levels than nicotine vapor alone (61). Together, these findings provide strong evidence that the addition of menthol to electronic cigarette products leads to greater dependence and addiction liability.
Green apple flavored products
Green apple is a characterizing fruit flavor commonly found in electronic cigarette liquids, which is acquired by the addition of the chemical farnesol (61, 152). In adolescents, the green apple flavorant was found to increase vaping behavior compared to both menthol-flavored and unflavored electronic cigarettes (152). These findings in humans may be due to a positive association of green apple based on the individual’s history (e.g., positive conditioning with fruit candy consumption as a child) and/or due to biological effects of the chemical on the reward-related neurocircuitry of the brain. Henderson and colleagues have led a series of important studies that reveal the biological effects of farnesol. They found that green apple-flavored nicotine vapor is self-administered at a higher level than unflavored nicotine vapor in adult male mice (61), supporting the enhanced reinforcing properties of farnesol with nicotine. Further, green apple flavorant alone can induce a conditioned place preference, in addition to enhancing nicotine’s rewarding effects, in both male and female adult mice (153, 154), thereby demonstrating rewarding properties on its own. At the cellular level, farnesol can affect the kinetics of the nicotinic acetylcholine receptor, which is the receptor on which nicotine binds to induce its reinforcing and rewarding effects (4, 153). Specifically, 24 h of farnesol pretreatment induced a shorter desensitization period for the nicotinic receptors containing the α4 and β2 subunits (153), which would allow for more permissible receptor re-activation in the presence of nicotine. Chronic farnesol administration also increased the firing rate of nicotinic acetylcholine receptor-expressing neurons in the ventral tegmental area (153). Thus, the addition of the green apple flavorant induces neurobiological changes in the brain’s reward-related circuitry, which enhances nicotine’s rewarding properties to reinforce continued product use.
Impact on inflammatory processes
Nicotine has been shown to induce both inflammatory and anti-inflammatory effects based on a number of factors, which may include dose, duration of treatment, route of administration, and underlying mechanisms (67, 155, 156). In general, nicotine has been characterized as exerting mainly anti-inflammatory effects throughout the brain and body (157). Like tobacco cigarette smoke, flavored electronic cigarette vapor has also been shown to induce pro-inflammatory markers in the brain, potentially due to nicotine, other constituents present in the vapor, or the interaction of nicotine and the constituents (158, 159). After 14 days, grape flavored electronic cigarette exposure increased in TNF-α in the cerebral cortex of male mice (158). A common electronic cigarette brand, JUUL, provides the vape liquid in an encapsulated pod, and vapor emitted from the JUUL pod has been shown to induce several pro-inflammatory responses in nucleus accumbens sub-regions in female mice following long term exposure (159). In the nucleus accumbens shell, aerosol from both mint/menthol and mango flavored JUULs increased the expression of TNF-α, IL-1β, and IL-6 following 1 and 3 months of exposure (159). In contrast, inflammatory markers in the nucleus accumbens core increased in a time-dependent manner; TNF-α expression was increased following both 1 and 3 months of JUUL exposure, but IL-1β was increased only following 1 month of JUUL exposure (159). Consistent with nicotine’s anti-inflammatory effects, chronic nicotine administration via an osmotic minipump did not increase either TNF-α or IL-1β in the nucleus accumbens in male mice (160), thereby supporting the notion that the constituents in the JUUL pods, or the interaction of nicotine with the constituents, led to the changes in inflammatory markers. Thus, these findings highlight the need to understand the potential impact of different chemical constituents on signaling in the brain.
In summary, based on the findings reviewed above, it is evident that all components of electronic cigarette emissions, including those labeled and unlabeled, can possess the potential to alter reward-related processing and behavior. However, it is important to acknowledge that the relative amounts of acrolein, acetaldehyde, formaldehyde, and metals released from electronic cigarette aerosol vary and may be lower than that examined in these reviewed studies. It is equally important to acknowledge that drug use also affects individuals on a longitudinal scale, and as such, chronic exposure to different constituents present in electronic cigarette aerosol may influence health outcomes, which will not be revealed until after many years of product use. Moreover, individual constituents may combine to induce synergistic effects that are different than each constituent alone, as evidenced by propylene glycol exposure and metal particulate exposure. Given that electronic cigarette use has been associated with cognitive effects, such as depression (18), and common biological pathways metabolize nicotine and psychiatric medications, it is also important to consider the intersection of electronic cigarette constituents and metabolizing enzymes.
Alterations to CYP450 enzyme function and drug interactions
Cytochrome P450 (CYP450) enzymes play a critical role in drug metabolism, especially for therapeutic compounds used to treat symptoms associated with depression and other psychiatric disorders. Tobacco cigarette smoking has been shown to affect the expression of different enzymes within the CYP family, thus increasing the risk for CYP-mediated drug interactions. Most notably, polycyclic hydrocarbons in tobacco cigarette smoke induce expression of CYP1A2, which is essential for the breakdown of the antidepressant fluvoxamine (161); thus, given the increased metabolism, one would expect a reduced effect of fluvoxamine in a chronic tobacco smoker. However, the current clinical implications of electronic cigarette use and prescription drug interactions are largely unknown. In this section, we will review current scientific data derived from pre-clinical studies.
In vitro studies have demonstrated that electronic cigarette liquid can alter CYP450 enzyme activity in various cell culture conditions (162–164) (Table 1). Administration of vape liquid has been shown to upregulate the expression of CYP2A6, CYP2U1, CYP2E1, and CYP2S1 mRNA (162), and exposure to condensed electronic cigarette aerosol induces CYP1A1 and CYP1B1 activity (164). In contrast, solution from nicotine-free vape liquids (strawberry poptart and apple watermelon flavors) was shown to inhibit the activity of CYP2A6 (163).Together, these findings demonstrate both CYP isoform-specific and direction-specific effects following exposure to different electronic cigarette solutions. Of note, CYP2A6 is the isoform that metabolizes nicotine (73, 174), indicating a potential for altered drug use patterns based on the constituents in the vape liquid.
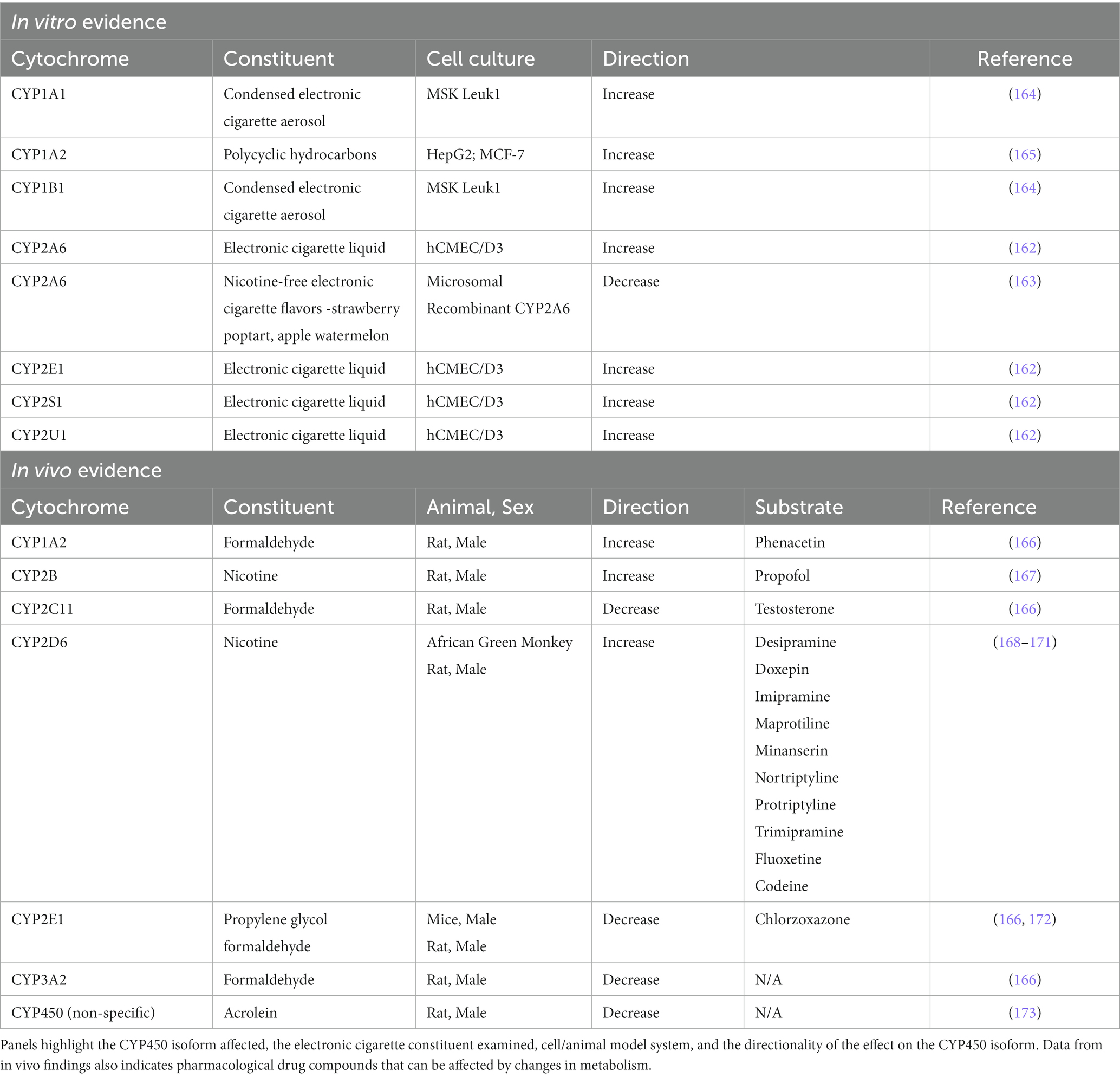
Table 1. CYP450 alterations in vitro or in vivo following exposure to constituents in electronic cigarettes.
In vivo studies have provided further insight into the potential clinical implications associated with altered CYP450 metabolism. In an important study by Khokhar and Tyndale, the authors found that 7 days of nicotine treatment increased CYP2B expression in the brain, but not liver, and surprisingly, this change in metabolism was sufficient to potentiate the sleep-inducing effects of the general anesthetic propofol in male rats (167). In addition to nicotine, carbonyls may also influence metabolism. For instance, acrolein has been found to inhibit CYP450 enzymes (173), and formaldehyde specifically reduces the levels of CYP2C11, CYP2E1, and CYP3A2, but increases CYP1A2, in male rats (166). Interestingly, the effects of formaldehyde on enzymatic activity led to decreased testosterone (steroid hormone) and chlorzoxazone (muscle relaxant) clearance, but increased phenacetin (analgesic) clearance (166). Propylene glycol has further been shown to inhibit CYP2E1, leading to decreased chlorzoxazone clearance by greater than 80% in male mice (172). It is interesting to note that CYP2E1 is a minor metabolizer of some antidepressants, including the selective serotonin reuptake inhibitor, fluoxetine, and monoamine oxidase inhibitor, moclobemide (168).
The CYP2D enzyme family is a well-documented major metabolizer of antidepressants, including desipramine, doxepin, imipramine, maprotiline, mianserin, nortriptyline, protriptyline, and trimipramine (168). Interestingly, in the frontal cortex, hippocampus, striatum, and cerebellum, chronic nicotine administration was shown to upregulate CYP2D6 mRNA in male rats (169) and CYP2D mRNA and protein in African green monkeys (170). This nicotine-mediated CYP2D induction has been shown to be sufficient to alter codeine metabolism in the brain but not in the plasma of male rats (171). Furthermore, these alterations were enough to increase codeine’s analgesic effect during the tail flick test (171). This suggests that long-term electronic cigarette use may alter the metabolism of these antidepressants, thereby affecting therapeutic efficacy and side effects. Of further note, genetic polymorphisms have been associated with drug bioavailability. For instance, altered blood plasma bioavailability of the antidepressant fluoxetine is found in individuals expressing CYP2D6 polymorphisms (175). Specifically, different allelic variations in CYP2D6 influence enzyme activity leading to either ultra-metabolizers or poor-metabolizers of CYP2D6 substrates (175, 176). Theoretically, a higher level of metabolism would be expected to terminate the drug action sooner, thereby limiting the effectiveness of the drug. In contrast, a decreased metabolism would be expected to allow for prolonged drug effectiveness and/or to increase the likelihood of off-target adverse effects with increased side-effects due to accumulation of the drug compound. Indeed, individuals with genetic polymorphisms in CYP2D6 were more likely to have participated in more antidepressant medication trials and also have been shown to switch among antidepressants more often (176, 177). These findings could either suggest that CYP2D6 polymorphism leads to: (1) an increase in depression severity/incidence or (2) an insufficient therapeutic response and/or excessive side effects. Given that studies have failed to find an association between CYP2D6 polymorphism and incidence of depression (178), the most likely conclusion is the latter, in which individuals have an increased need to try different therapeutics in search of a positive therapeutic response (176, 177). In sum, individual constituents found in electronic cigarette liquid and aerosol can significantly impact CYP450 enzyme activity (Table 1), which represents an important consideration when evaluating therapeutic effectiveness in the clinic for patients suffering from depression.
Conclusion
The recent development and use of electronic cigarettes, as well as a general lack of regulatory oversight, has led many individuals to be exposed to chemicals that have unknown long-term effects on the brain. While a clear positive correlation between electronic cigarette use and depression has been established (18, 47), the causality and mitigating factors affecting this relationship are largely undetermined. Therefore, as we go forward, it will be necessary to more precisely investigate the acute and long-term effects of all constituents found in the aerosols and the impact of these factors at various stages of neurodevelopment. This includes both labeled ingredients, such as propylene glycol, vegetable glycerin, nicotine, and various chemical flavorants (menthol, green apple, mango, and others), as well as unlabeled ingredients present in the aerosols of the devices. These can include carbonyls (acetaldehyde, acrolein, formaldehyde) and heavy metals (arsenic, chromium, copper, iron, manganese, nickel, lead, selenium, strontium, zinc). It will also be important to consider the effects of these constituents on the CYP450 enzyme family and related implications for therapeutic efficacy of psychiatric medications. Due to the large variation in the quantities of each constituent across products and an ever-evolving product marketplace, it will continue to be challenging to fully understand the clinical relevance of electronic cigarette use on the individual’s health. However, given our current understanding and the potential adverse implications for public health, regulatory agencies should take a more proactive role in overseeing the production and commercialization of electronic cigarette products.
Author contributions
MB, AM, and CDF contributed to the manuscript drafts, and all authors approved the final version. MB created the figure.
Funding
This work was supported by grants from the Tobacco-Related Disease Research Program (TRDRP) (T31IR1767 and T32IR4866 to CDF and T32DT5202 to MB).
Conflict of interest
The authors declare that the research was conducted in the absence of any commercial or financial relationships that could be construed as a potential conflict of interest.
Publisher’s note
All claims expressed in this article are solely those of the authors and do not necessarily represent those of their affiliated organizations, or those of the publisher, the editors and the reviewers. Any product that may be evaluated in this article, or claim that may be made by its manufacturer, is not guaranteed or endorsed by the publisher.
References
1. Tiku, N. (2015). Startup Behind the Lambo of Vaporizers Just Launched an Intelligent E-Cigarette The Verge: Vox Media. [Accessed October 27, 2022].
2. World Health Organization (2011). Report on the Global Tobacco Epidemic, 2011: Warning About the Dangers of Tobacco. Geneva.
4. Le Foll, B, Piper, ME, Fowler, CD, Tonstad, S, Bierut, L, Lu, L, et al. Tobacco and nicotine use. Nat Rev Dis Primers. (2022) 8:19. doi: 10.1038/s41572-022-00346-w
5. Prevention CfDCa (2022). More Than 3 Million Youth Reported Using a Tobacco Product in 2022. https://www.cdc.gov/media/releases/2022/p1110-youth-tobaco.html
6. Weaver, SR, Majeed, BA, Pechacek, TF, Nyman, AL, Gregory, KR, and Eriksen, MP. Use of electronic nicotine delivery systems and other tobacco products among USA adults, 2014: results from a National Survey. Int J Public Health. (2015). doi: 10.1007/s00038-015-0761-0
7. DeVito, EE, and Krishnan-Sarin, S. E-cigarettes: impact of E-liquid components and device characteristics on nicotine exposure. Curr Neuropharmacol. (2018) 16:438–59. doi: 10.2174/1570159X15666171016164430
8. Allen, JG, Flanigan, SS, LeBlanc, M, Vallarino, J, MacNaughton, P, Stewart, JH, et al. Flavoring chemicals in E-cigarettes: diacetyl, 2,3-Pentanedione, and acetoin in a sample of 51 products, including fruit-, candy-, and cocktail-Flavored E-cigarettes. Environ Health Perspect. (2016) 124:733–9. doi: 10.1289/ehp.1510185
9. George, J, Hussain, M, Vadiveloo, T, Ireland, S, Hopkinson, P, Struthers, AD, et al. Cardiovascular effects of switching from tobacco cigarettes to electronic cigarettes. J Am Coll Cardiol. (2019) 74:3112–20. doi: 10.1016/j.jacc.2019.09.067
10. Cibella, F, Campagna, D, Caponnetto, P, Maria, CM, Russo, C, Cockcroft, DW, et al. Lung function and respiratory symptoms in a randomized smoking cessation trial of electronic cigarettes. Clin Sci. (2016) 130:1929–37. doi: 10.1042/CS20160268
11. Hajek, P, Phillips-Waller, A, Przulj, D, Pesola, F, Myers Smith, K, Bisal, N, et al. A randomized trial of E-cigarettes versus nicotine-replacement therapy. N Engl J Med. (2019) 380:629–37. doi: 10.1056/NEJMoa1808779
12. Caponnetto, P, DiPiazza, J, Kim, J, Maglia, M, and Polosa, R. A single-arm, open-label, pilot, and feasibility study of a high nicotine strength E-cigarette intervention for smoking cessation or reduction for people with schizophrenia Spectrum disorders who smoke cigarettes. Nicot Tobacco Res. (2021) 23:1113–22. doi: 10.1093/ntr/ntab005
13. Flacco, ME, Fiore, M, Acuti Martellucci, C, Ferrante, M, Gualano, MR, Liguori, G, et al. Tobacco vs. electronic cigarettes: absence of harm reduction after six years of follow-up. Eur Rev Med Pharmacol Sci. (2020) 24:3923–34. doi: 10.26355/eurrev_202004_20859
14. Hefner, K, Valentine, G, and Sofuoglu, M. Electronic cigarettes and mental illness: reviewing the evidence for help and harm among those with psychiatric and substance use disorders. Am J Addict. (2017) 26:306–15. doi: 10.1111/ajad.12504
15. Hammond, D, Reid, JL, Burkhalter, R, O'Connor, RJ, Goniewicz, ML, Wackowski, OA, et al. Trends in E-cigarette brands, devices and the nicotine profile of products used by youth in England, Canada and the USA: 2017-2019. Tob Control. (2021). doi: 10.1136/tobaccocontrol-2020-056371
16. Pham, T, Williams, JVA, Bhattarai, A, Dores, AK, Isherwood, LJ, and Patten, SB. Electronic cigarette use and mental health: a Canadian population-based study. J Affect Disord. (2020) 260:646–52. doi: 10.1016/j.jad.2019.09.026
17. Clendennen, SL, Chen, B, Sumbe, A, and Harrell, MB. Patterns in mental health symptomatology and cigarette, E-cigarette, and marijuana use among Texas youth and Young adults amid the coronavirus disease 2019 pandemic. Nicot Tobacco Res. (2023) 25:266–73. doi: 10.1093/ntr/ntac205
18. Lechner, WV, Janssen, T, Kahler, CW, Audrain-Mcgovern, J, and Leventhal, AM. Bi-directional associations of electronic and combustible cigarette use onset patterns with depressive symptoms in adolescents. Prev Med. (2017) 96:73–8. doi: 10.1016/j.ypmed.2016.12.034
19. Aleyan, S, Cole, A, Qian, W, and Leatherdale, ST. Risky business: a longitudinal study examining cigarette smoking initiation among susceptible and non-susceptible E-cigarette users in Canada. BMJ Open. (2018) 8:e021080. doi: 10.1136/bmjopen-2017-021080
20. Primack, BA, Shensa, A, Sidani, JE, Hoffman, BL, Soneji, S, Sargent, JD, et al. Initiation of traditional cigarette smoking after electronic cigarette use among tobacco-Naïve us Young adults. Am J Med. (2018) 131:e1-.e9:443. doi: 10.1016/j.amjmed.2017.11.005
21. Kowitt, SD, Osman, A, Ranney, LM, Heck, C, and Goldstein, AO. E-cigarette use among adolescents not susceptible to using cigarettes. Prev Chronic Dis. (2018) 15:E18. doi: 10.5888/pcd15.170368
22. Adzrago, D, Shi, Y, and Fujimoto, K. Association between perceived health risks of E-cigarettes and actual E-cigarette use, based on cigarette smoking status and sexual and gender minority status among U.S. adults. J Public Health. (2022). doi: 10.1007/s10389-021-01674-z
23. Vu, T-HT, Groom, A, Hart, JL, Tran, H, Landry, RL, Ma, JZ, et al. Socioeconomic and demographic status and perceived health risks of E-cigarette product contents among youth: results from a National Survey. Health Promot Pract. (2020) 21:148S–56S. doi: 10.1177/1524839919882700
24. Gaiha, SM, Rao, P, and Halpern-Felsher, B. Sociodemographic factors associated with adolescents’ and Young adults’ susceptibility, use, and intended future use of different E-cigarette devices. Int J Environ Res Public Health. (2022) 19:1941. doi: 10.3390/ijerph19041941
25. Jiang, N, Xu, S, Li, L, El-Shahawy, O, Freudenberg, N, Shearston, JA, et al. The mediating effect of E-cigarette harm perception in the relationship between E-cigarette advertising exposure and E-cigarette use. Int J Environ Res Public Health. (2022) 19:6215. doi: 10.3390/ijerph19106215
26. Sun, T, Lim, CCW, Chung, J, Cheng, B, Davidson, L, Tisdale, C, et al. Vaping on Tiktok: a systematic thematic analysis. Tob Control. (2021). doi: 10.1136/tobaccocontrol-2021-056619
27. Hung, M, Spencer, A, Goh, C, Hon, ES, Cheever, VJ, Licari, FW, et al. The Association of Adolescent E-cigarette harm perception to advertising exposure and marketing type. Arch Public Health. (2022) 80:114. doi: 10.1186/s13690-022-00867-6
28. Elton-Marshall, T, Driezen, P, Fong, GT, Cummings, KM, Persoskie, A, Wackowski, O, et al. Adult perceptions of the relative harm of tobacco products and subsequent tobacco product use: longitudinal findings from waves 1 and 2 of the population assessment of tobacco and health (path) study. Addict Behav. (2020) 106:106337. doi: 10.1016/j.addbeh.2020.106337
29. State System E-Cigarette Fact Sheet (2022). Prevention CfDCa, editor.: Centers for Disease Control and Prevention.
31. Bandiera, FC, Loukas, A, Wilkinson, AV, and Perry, CL. Associations between tobacco and nicotine product use and depressive symptoms among college students in Texas. Addict Behav. (2016) 63:19–22. doi: 10.1016/j.addbeh.2016.06.024
32. King, JL, Reboussin, D, Cornacchione Ross, J, Wiseman, KD, Wagoner, KG, and Sutfin, EL. Polytobacco use among a nationally representative sample of adolescent and Young adult E-cigarette users. J Adolesc Health. (2018) 63:407–12. doi: 10.1016/j.jadohealth.2018.04.010
33. Lee, Y, and Lee, K-S. Association of Depression and Suicidality with electronic and conventional cigarette use in south Korean adolescents. Subst Use Misuse. (2019) 54:934–43. doi: 10.1080/10826084.2018.1552301
34. Kim, JS, and Kim, K. Electronic cigarette use and suicidal Behaviors among adolescents. J Public Health. (2021) 43:274–80. doi: 10.1093/pubmed/fdz086
35. Leventhal, AM, Strong, DR, Sussman, S, Kirkpatrick, MG, Unger, JB, Barrington-Trimis, JL, et al. Psychiatric comorbidity in adolescent electronic and conventional cigarette use. J Psychiatr Res. (2016) 73:71–8. doi: 10.1016/j.jpsychires.2015.11.008
36. Hefner, K, Rosenheck, R, Merrel, J, Coffman, M, Valentine, G, and Sofuoglu, M. E-cigarette use in veterans seeking mental health and/or substance use services. J Dual Diagn. (2016) 12:109–17. doi: 10.1080/15504263.2016.1172895
37. Farrell, KR, Karey, E, Xu, S, Gibbon, G, Gordon, T, and Weitzman, M. E-cigarette use, systemic inflammation, and depression. Int J Environ Res Public Health. (2021) 18:10402. doi: 10.3390/ijerph181910402
38. Masaki, K, Taketa, RM, Nakama, MK, Kawamoto, CT, and Pokhrel, P. Relationships between depressive symptoms, anxiety, impulsivity and cigarette and E-cigarette use among Young adults. Hawaii J Health Soc Welf. (2022) 81:51–7.
39. Dunbar, MS, Tucker, JS, Ewing, BA, Pedersen, ER, Miles, JNV, Shih, RA, et al. Frequency of E-cigarette use, health status, and risk and protective health Behaviors in adolescents. J Addict Med. (2017) 11:55–62. doi: 10.1097/ADM.0000000000000272
40. Wiernik, E, Airagnes, G, Lequy, E, Gomajee, R, Melchior, M, Le Faou, AL, et al. Electronic cigarette use is associated with depressive symptoms among smokers and former smokers: cross-sectional and longitudinal findings from the Constances cohort. Addict Behav. (2019) 90:85–91. doi: 10.1016/j.addbeh.2018.10.021
41. Becker, TD, Arnold, MK, Ro, V, Martin, L, and Rice, TR. Systematic review of electronic cigarette use (vaping) and mental health comorbidity among adolescents and Young adults. Nicot Tobacco Res. (2021) 23:415–25. doi: 10.1093/ntr/ntaa171
42. Fluharty, M, Taylor, AE, Grabski, M, and Munafo, MR. The Association of Cigarette Smoking with depression and anxiety: a systematic review. Nicot Tobacco Res. (2017) 19:3–13. doi: 10.1093/ntr/ntw140
43. Cummins, SE, Zhu, SH, Tedeschi, GJ, Gamst, AC, and Myers, MG. Use of E-cigarettes by individuals with mental health conditions. Tob Control. (2014) 23:48–53. doi: 10.1136/tobaccocontrol-2013-051511
44. Riehm, KE, Young, AS, Feder, KA, Krawczyk, N, Tormohlen, KN, Pacek, LR, et al. Mental health problems and initiation of E-cigarette and combustible cigarette use. Pediatrics. (2019) 144:e20182935. doi: 10.1542/peds.2018-2935
45. Stanislawska Kubiak, M, Wojciak, RW, Trzeszczynska, N, Czajeczny, D, Samborski, W, and Mojs, E. Who is happier: smoker or non-smoker? Smoking in medical students from the perspective of positive psychology. Eur Rev Med Pharmacol Sci. (2019) 23:2174–81. doi: 10.26355/eurrev_201903_17263
46. Stickley, A, Koyanagi, A, Roberts, B, Leinsalu, M, Goryakin, Y, and McKee, M. Smoking status, nicotine dependence and happiness in nine countries of the former Soviet Union. Tob Control. (2015) 24:190–7. doi: 10.1136/tobaccocontrol-2014-052092
47. Bandiera, FC, Loukas, A, Li, X, Wilkinson, AV, and Perry, CL. Depressive symptoms predict current E-cigarette use among college students in Texas. Nicotine Tob Res. (2017) 19:1102–6. doi: 10.1093/ntr/ntx014
48. Watkins, SS, Koob, GF, and Markou, A. Neural mechanisms underlying nicotine addiction: acute positive reinforcement and withdrawal. Nicotine Tob Res. (2000) 2:19–37. doi: 10.1080/14622200050011277
49. Prochaska, JJ, Das, S, and Young-Wolff, KC. Smoking, mental illness, and public health. Annu Rev Public Health. (2017) 38:165–85. doi: 10.1146/annurev-publhealth-031816-044618
50. Audrain-McGovern, J, Rodriguez, D, Pianin, S, and Alexander, E. Initial E-cigarette Flavoring and nicotine exposure and E-cigarette uptake among adolescents. Drug Alcohol Depend. (2019) 202:149–55. doi: 10.1016/j.drugalcdep.2019.04.037
51. APA . Diagnostic and statistical manual of mental disorders (Dsm–5). 5th Edition ed. Arlington, VA: American Psychological Association (2013).
52. Adzrago, D, Sulley, S, Mamudu, L, Ormiston, CK, and Williams, F. The influence of Covid-19 pandemic on the frequent use of E-cigarettes and its association with substance use and mental health symptoms. Behav Sci. (2022) 12:453. doi: 10.3390/bs12110453
53. Azagba, S, Ebling, T, Adekeye, OT, and Shan, L. Mental health condition indicators and E-cigarette use among sexual minority youth. J Affect Disord. (2022) 319:1–7. doi: 10.1016/j.jad.2022.09.032
54. Donaldson, CD, Stupplebeen, DA, Fecho, CL, Ta, T, Zhang, X, and Williams, RJ. Nicotine vaping for relaxation and coping: race/ethnicity differences and social connectedness mechanisms. Addict Behav. (2022) 132:107365. doi: 10.1016/j.addbeh.2022.107365
55. Adzrago, D, Fujimoto, K, Harrell, MB, Jones, A, and Wilkerson, JM. Association between E-cigarette use Behaviors and perceived harmfulness of E-cigarettes and anxiety/depression symptoms among black/African American adults. Prev Med Rep. (2023) 31:102080. doi: 10.1016/j.pmedr.2022.102080
56. Manning, K, Mayorga, NA, Garey, L, Kauffman, BY, Buckner, JD, and Zvolensky, MJ. The role of anxiety sensitivity and fatigue severity in predicting E-cigarette dependence, barriers to cessation, and cravings among Young adults. Subst Use Misuse. (2021) 56:2059–65. doi: 10.1080/10826084.2021.1967990
57. Nguyen, HV, and Mital, S. Effects of E-cigarette use on mental health among youths: quasi-experimental evidence from Canada. Addiction. (2022) 117:2673–82. doi: 10.1111/add.15943
58. McLaughlin, I, Dani, JA, and De Biasi, M. Nicotine withdrawal. Curr Top Behav Neurosci. (2015) 24:99–123. doi: 10.1007/978-3-319-13482-6_4
59. Pergadia, ML, Newcomer, JW, and Gilbert, DG. Depression and nicotine withdrawal associations with combustible and electronic cigarette use. Int J Environ Res Public Health. (2020) 17:9334. doi: 10.3390/ijerph17249334
60. Frie, JA, Underhill, J, Zhao, B, de Guglielmo, G, Tyndale, RF, and Khokhar, JY. Openvape: an open-source E-cigarette vapor exposure device for rodents. eNeuro. (2020) 7:ENEURO.0279-20.2020. doi: 10.1523/ENEURO.0279-20.2020
61. Cooper, SY, Akers, AT, and Henderson, BJ. Flavors enhance nicotine vapor self-administration in male mice. Nicot Tobacco Res. (2021) 23:566–72. doi: 10.1093/ntr/ntaa165
62. Smith, LC, Kallupi, M, Tieu, L, Shankar, K, Jaquish, A, Barr, J, et al. Validation of a nicotine vapor self-administration model in rats with relevance to electronic cigarette use. Neuropsychopharmacology. (2020) 45:1909–19. doi: 10.1038/s41386-020-0734-8
63. Henderson, BJ, and Cooper, SY. Nicotine formulations impact reinforcement-related Behaviors in a mouse model of vapor self-administration. Drug Alcohol Depend. (2021) 224:108732. doi: 10.1016/j.drugalcdep.2021.108732
64. Palmatier, MI, Smith, AL, Odineal, EM, Williams, EA, Sheppard, AB, and Bradley, CA. Nicotine self-administration with tobacco flavor additives in male rats. Nicot Tobacco Res. (2020) 22:224–31. doi: 10.1093/ntr/ntz053
65. Lallai, V, Chen, YC, Roybal, MM, Kotha, ER, Fowler, JP, Staben, A, et al. Nicotine E-cigarette vapor inhalation and self-Administration in a Rodent Model: sex- and nicotine delivery-specific effects on metabolism and behavior. Addict Biol. (2021) 26:e13024. doi: 10.1111/adb.13024
66. DeNoble, VJ, and Mele, PC. Intravenous nicotine self-Administration in Rats: effects of Mecamylamine, Hexamethonium and naloxone. Psychopharmacology. (2006) 184:266–72. doi: 10.1007/s00213-005-0054-z
67. Lallai, V, Manca, L, and Fowler, CD. E-cigarette vape and lung Ace2 expression: implications for coronavirus vulnerability. Environ Toxicol Pharmacol. (2021) 86:103656. doi: 10.1016/j.etap.2021.103656
68. Corrigall, WA . Nicotine self-Administration in Animals as a dependence model. Nicotine Tob Res. (1999) 1:11–20. doi: 10.1080/14622299050011121
69. Fowler, CD, and Kenny, PJ. Intravenous nicotine self-administration and Cue-induced reinstatement in mice: effects of nicotine dose, rate of drug infusion and prior instrumental training. Neuropharmacology. (2011) 61:687–98. doi: 10.1016/j.neuropharm.2011.05.012
70. Lallai, V, Chen, YC, Roybal, MM, Kotha, ER, Fowler, JP, Staben, A, et al. Nicotine E-Cigarette Vapor Inhalation and self-Administration in a Rodent Model: sex- and nicotine delivery-specific effects on metabolism and behavior. Addict Biol. (2021):e13024. doi: 10.1111/adb.13024
71. Shao, XM, Lopez, B, Nathan, D, Wilson, J, Bankole, E, Tumoyan, H, et al. A mouse model for chronic intermittent electronic cigarette exposure exhibits nicotine pharmacokinetics resembling human vapers. J Neurosci Methods. (2019) 326:108376. doi: 10.1016/j.jneumeth.2019.108376
72. Flouris, AD, Chorti, MS, Poulianiti, KP, Jamurtas, AZ, Kostikas, K, Tzatzarakis, MN, et al. Acute impact of active and passive electronic cigarette smoking on serum cotinine and lung function. Inhal Toxicol. (2013) 25:91–101. doi: 10.3109/08958378.2012.758197
73. Chen, YC, Fowler, JP, Wang, J, Watson, CJW, Sherafat, Y, Staben, A, et al. The novel Cyp2a6 inhibitor, Dlci-1, decreases nicotine self-Administration in Mice. J Pharmacol Exp Ther. (2020) 372:21–9. doi: 10.1124/jpet.119.260653
74. Kanamori, K, Ahmad, SM, Shin, CS, Hamid, A, and Lutfy, K. Identification of 5-Hydroxycotinine in the plasma of nicotine-treated mice: implications for cotinine metabolism and disposition in vivo. Drug Metab Dispos. (2022) 50:1454–63. doi: 10.1124/dmd.122.001059
75. Birnie, MT, Levis, SC, Mahler, SV, and Baram, TZ. Developmental trajectories of anhedonia in preclinical models current topics in Behavioral. Neurosciences. (2022) 58:23–41. doi: 10.1007/7854_2021_299
76. Walf, AA, and Frye, CA. The use of the elevated plus maze as an assay of anxiety-related behavior in rodents. Nat Protoc. (2007) 2:322–8. doi: 10.1038/nprot.2007.44
77. Fowler, CD, Tuesta, L, and Kenny, PJ. Role of Alpha5* nicotinic acetylcholine receptors in the effects of acute and chronic nicotine treatment on brain reward function in mice. Psychopharmacology. (2013) 229:503–13. doi: 10.1007/s00213-013-3235-1
78. Harris, AC, Muelken, P, Haave, Z, Swain, Y, Smethells, JR, and LeSage, MG. Propylene glycol, a major electronic cigarette constituent, attenuates the adverse effects of high-dose nicotine as measured by intracranial self-stimulation in rats. Drug Alcohol Depend. (2018) 193:162–8. doi: 10.1016/j.drugalcdep.2018.08.037
79. Ponzoni, L, Braida, D, Carboni, L, Moretti, M, Viani, P, Clementi, F, et al. Persistent cognitive and affective alterations at late withdrawal stages after long-term intermittent exposure to tobacco smoke or electronic cigarette vapour: behavioural changes and their neurochemical correlates. Pharmacol Res. (2020) 158:104941. doi: 10.1016/j.phrs.2020.104941
80. Carboni, L, Ponzoni, L, Braida, D, Sala, M, Gotti, C, and Zoli, M. Altered Mrna levels of stress-related peptides in mouse Hippocampus and caudate-putamen in withdrawal after long-term intermittent exposure to tobacco smoke or electronic cigarette vapour. Int J Mol Sci. (2021) 22:599. doi: 10.3390/ijms22020599
81. Garrett, PI, Honeycutt, SC, Marston, C, Allen, N, Barraza, AG, Dewey, M, et al. Nicotine-free vapor inhalation produces Behavioral disruptions and anxiety-like Behaviors in mice: effects of puff duration, session length, sex, and flavor. Pharmacol Biochem Behav. (2021) 206:173207. doi: 10.1016/j.pbb.2021.173207
82. Tommasi, S, Pabustan, N, Li, M, Chen, Y, Siegmund, KD, and Besaratinia, A. A novel role for vaping in mitochondrial gene dysregulation and inflammation fundamental to disease development. Sci Rep. (2021) 11:22773. doi: 10.1038/s41598-021-01965-1
83. Hahn, J, Monakhova, YB, Hengen, J, Kohl-Himmelseher, M, Schussler, J, Hahn, H, et al. Electronic cigarettes: overview of chemical composition and exposure estimation. Tob Induc Dis. (2014) 12:23. doi: 10.1186/s12971-014-0023-6
84. Raymond, BH, Collette-Merrill, K, Harrison, RG, Jarvis, S, and Rasmussen, RJ. The nicotine content of a sample of E-cigarette liquid manufactured in the United States. J Addict Med. (2018) 12:127–31. doi: 10.1097/ADM.0000000000000376
85. Goniewicz, ML, Hajek, P, and McRobbie, H. Nicotine content of electronic cigarettes, its release in vapour and its consistency across batches: regulatory implications. Addiction. (2014) 109:500–7. doi: 10.1111/add.12410
86. Naeem, MNM, Zain, SMSM, Ng, C, and Noh, MFM. Chemical constituents in E-cigarette liquids and aerosols. J Environ Prot. (2020) 11:664–81. doi: 10.4236/jep.2020.119040
87. Leventhal, AM, Goldenson, NI, Barrington-Trimis, JL, Pang, RD, and Kirkpatrick, MG. Effects of non-tobacco Flavors and nicotine on E-cigarette product appeal among Young adult never, former, and current smokers. Drug Alcohol Depend. (2019) 203:99–106. doi: 10.1016/j.drugalcdep.2019.05.020
88. Tokle, R, Brunborg, GS, and Vedoy, TF. Adolescents' use of nicotine-free and nicotine E-cigarettes: a longitudinal study of vaping transitions and vaper characteristics. Nicotine Tob Res. (2022) 24:400–7. doi: 10.1093/ntr/ntab192
89. Gotti, C, and Clementi, F. Neuronal nicotinic receptors: from structure to pathology. Prog Neurobiol. (2004) 74:363–96. doi: 10.1016/j.pneurobio.2004.09.006
90. Wills, L, Ables, JL, Braunscheidel, KM, Caligiuri, SPB, Elayouby, KS, Fillinger, C, et al. Neurobiological mechanisms of nicotine reward and aversion. Pharmacol Rev. (2022) 74:271–310. doi: 10.1124/pharmrev.121.000299
91. Gipson, CD, and Fowler, CD. Nicotinic receptors underlying nicotine dependence: evidence from transgenic mouse models. Curr Top Behav Neurosci. (2020) 45:101–21. doi: 10.1007/7854_2020_134
92. Picciotto, MR, Zoli, M, Rimondini, R, Lena, C, Marubio, LM, Pich, EM, et al. Acetylcholine receptors containing the Beta2 subunit are involved in the reinforcing properties of nicotine. Nature. (1998) 391:173–7. doi: 10.1038/34413
93. Fu, Y, Matta, SG, Gao, W, Brower, VG, and Sharp, BM. Systemic nicotine stimulates dopamine release in nucleus Accumbens: Re-evaluation of the role of N-methyl-D-aspartate receptors in the ventral tegmental area. J Pharmacol Exp Ther. (2000) 294:458–65.
94. Pons, S, Fattore, L, Cossu, G, Tolu, S, Porcu, E, McIntosh, JM, et al. Crucial role of Alpha4 and Alpha6 nicotinic acetylcholine receptor subunits from ventral tegmental area in systemic nicotine self-administration. J Neurosci. (2008) 28:12318–27. doi: 10.1523/JNEUROSCI.3918-08.2008
95. Fowler, CD, and Kenny, PJ. Nicotine aversion: neurobiological mechanisms and relevance to tobacco dependence vulnerability. Neuropharmacology. (2014) 76 Pt B:533-44). doi: 10.1016/j.neuropharm.2013.09.008
96. Fowler, CD, Lu, Q, Johnson, PM, Marks, MJ, and Kenny, PJ. Habenular Alpha5 nicotinic receptor subunit signalling controls nicotine intake. Nature. (2011) 471:597–601. doi: 10.1038/nature09797
97. Elayouby, KS, Ishikawa, M, Dukes, AJ, Smith, ACW, Lu, Q, Fowler, CD, et al. Alpha3* nicotinic acetylcholine receptors in the Habenula-interpeduncular nucleus circuit regulate nicotine intake. J Neurosci. (2021) 41:1779–87. doi: 10.1523/JNEUROSCI.0127-19.2020
98. Frahm, S, Slimak, MA, Ferrarese, L, Santos-Torres, J, Antolin-Fontes, B, Auer, S, et al. Aversion to nicotine is regulated by the balanced activity of Beta4 and Alpha5 nicotinic receptor subunits in the medial Habenula. Neuron. (2011) 70:522–35. doi: 10.1016/j.neuron.2011.04.013
99. Salas, R, Cook, KD, Bassetto, L, and De Biasi, M. The Alpha3 and Beta4 nicotinic acetylcholine receptor subunits are necessary for nicotine-induced seizures and Hypolocomotion in mice. Neuropharmacology. (2004) 47:401–7. doi: 10.1016/j.neuropharm.2004.05.002
100. Salas, R, Pieri, F, and De Biasi, M. Decreased signs of nicotine withdrawal in mice null for the Beta4 nicotinic acetylcholine receptor subunit. J Neurosci. (2004) 24:10035–9. doi: 10.1523/JNEUROSCI.1939-04.2004
101. Salas, R, Sturm, R, Boulter, J, and De Biasi, M. Nicotinic receptors in the Habenulo-interpeduncular system are necessary for nicotine withdrawal in mice. J Neurosci. (2009) 29:3014–8. doi: 10.1523/JNEUROSCI.4934-08.2009
103. Erythropel, HC, Jabba, SV, Dewinter, TM, Mendizabal, M, Anastas, PT, Jordt, SE, et al. Formation of Flavorant–propylene glycol adducts with novel toxicological properties in chemically unstable E-cigarette liquids. Nicotine Tob Res. (2019) 21:1248–58. doi: 10.1093/ntr/nty192
104. Chung, S, Baumlin, N, Dennis, JS, Moore, R, Salathe, SF, Whitney, PL, et al. Electronic cigarette vapor with nicotine causes airway Mucociliary dysfunction preferentially via Trpa1 receptors. Am J Respir Crit Care Med. (2019) 200:1134–45. doi: 10.1164/rccm.201811-2087OC
105. Yang, E, and Kaddoumi, A. Role of endothelial Trpa1 expression in blood-brain barrier dysfunction. Alzheimer's Dement. (2021):17(S2). doi: 10.1002/alz.058550
106. Church, JS, Chace-Donahue, F, Blum, JL, Ratner, JR, Zelikoff, JT, and Schwartzer, JJ. Neuroinflammatory and Behavioral outcomes measured in adult offspring of mice exposed prenatally to E-cigarette aerosols. Environ Health Perspect. (2020) 128:047006. doi: 10.1289/EHP6067
107. Jaegers, NR, Hu, W, Weber, TJ, and Hu, JZ. Low-temperature (≪ 200 °C) degradation of electronic nicotine delivery system liquids generates toxic aldehydes. Sci Rep. (2021) 11:7800. doi: 10.1038/s41598-021-87044-x
108. Conklin, DJ, Ogunwale, MA, Chen, Y, Theis, WS, Nantz, MH, Fu, X-A, et al. Electronic cigarette-generated aldehydes: the contribution of E-liquid components to their formation and the use of urinary aldehyde metabolites as biomarkers of exposure. Aerosol Sci Technol. (2018) 52:1219–32. doi: 10.1080/02786826.2018.1500013
109. Kosmider, L, Cox, S, Zaciera, M, Kurek, J, Goniewicz, ML, McRobbie, H, et al. Daily exposure to formaldehyde and acetaldehyde and potential health risk associated with use of high and low nicotine E-liquid concentrations. Sci Rep. (2020) 10:6546. doi: 10.1038/s41598-020-63292-1
110. Farsalinos, KE, and Gillman, G. Carbonyl emissions in E-cigarette aerosol: a systematic review and methodological considerations. Front Physiol. (2017) 8:1119. doi: 10.3389/fphys.2017.01119
111. Etter, J-F, and Bugey, A. E-cigarette liquids: Constancy of content across batches and accuracy of Labeling. Addict Behav. (2017) 73:137–43. doi: 10.1016/j.addbeh.2017.05.012
112. Kośmider, L, Kimber, CF, Kurek, J, Corcoran, O, and Dawkins, LE. Compensatory puffing with lower nicotine concentration E-liquids increases carbonyl exposure in E-cigarette aerosols. Nicot Tobacco Res. (2018) 20:998–1003. doi: 10.1093/ntr/ntx162
113. Samburova, V, Bhattarai, C, Strickland, M, Darrow, L, Angermann, J, Son, Y, et al. Aldehydes in exhaled breath during E-cigarette vaping: pilot study results. Toxics. (2018) 6:46. doi: 10.3390/toxics6030046
114. Lorkiewicz, P, Keith, R, Lynch, J, Jin, L, Theis, W, Krivokhizhina, T, et al. Electronic cigarette solvents, Juul E-liquids, and biomarkers of exposure: in vivo evidence for Acrolein and Glycidol in E-cig-derived aerosols. Chem Res Toxicol. (2022) 35:283–92. doi: 10.1021/acs.chemrestox.1c00328
115. McRobbie, H, Phillips, A, Goniewicz, ML, Smith, KM, Knight-West, O, Przulj, D, et al. Effects of switching to electronic cigarettes with and without concurrent smoking on exposure to nicotine, carbon monoxide, and Acrolein. Cancer Prev Res. (2015) 8:873–8. doi: 10.1158/1940-6207.CAPR-15-0058
116. Bekki, K, Uchiyama, S, Ohta, K, Inaba, Y, Nakagome, H, and Kunugita, N. Carbonyl compounds generated from electronic cigarettes. Int J Environ Res Public Health. (2014) 11:11192–200. doi: 10.3390/ijerph111111192
117. Williams, TI, Lynn, BC, Markesbery, WR, and Lovell, MA. Increased levels of 4-Hydroxynonenal and Acrolein, neurotoxic markers of lipid peroxidation, in the brain in mild cognitive impairment and early Alzheimer's disease. Neurobiol Aging. (2006) 27:1094–9. doi: 10.1016/j.neurobiolaging.2005.06.004
118. Ambaw, A, Zheng, L, Tambe, MA, Strathearn, KE, Acosta, G, Hubers, SA, et al. Acrolein-mediated neuronal cell death and alpha-Synuclein aggregation: implications for Parkinson's disease. Mol Cell Neurosci. (2018) 88:70–82. doi: 10.1016/j.mcn.2018.01.006
119. Heldt, NA, Reichenbach, N, McGary, HM, and Persidsky, Y. Effects of electronic nicotine delivery systems and cigarettes on systemic circulation and blood-brain barrier. Am J Pathol. (2021) 191:243–55. doi: 10.1016/j.ajpath.2020.11.007
120. Beauval, N, Antherieu, S, Soyez, M, Gengler, N, Grova, N, Howsam, M, et al. Chemical evaluation of electronic cigarettes: multicomponent analysis of liquid refills and their corresponding aerosols. J Anal Toxicol. (2017) 41:670–8. doi: 10.1093/jat/bkx054
121. Mekala, N, Gheewala, N, Rom, S, Sriram, U, and Persidsky, Y. Blocking of P2x7r reduces mitochondrial stress induced by alcohol and electronic cigarette exposure in brain microvascular endothelial cells. Antioxidants. (2022) 11:1328. doi: 10.3390/antiox11071328
122. Haorah, J, Ramirez, SH, Floreani, N, Gorantla, S, Morsey, B, and Persidsky, Y. Mechanism of alcohol-induced oxidative stress and neuronal injury. Free Radic Biol Med. (2008) 45:1542–50. doi: 10.1016/j.freeradbiomed.2008.08.030
123. Picklo, MJ, and Montine, TJ. Acrolein inhibits respiration in isolated brain mitochondria. Biochim Biophys Acta Mol basis Dis. (2001) 1535:145–52. doi: 10.1016/S0925-4439(00)00093-4
124. Kuntic, M, Oelze, M, Steven, S, Kroller-Schon, S, Stamm, P, Kalinovic, S, et al. Short-term E-cigarette vapour exposure causes vascular oxidative stress and dysfunction: evidence for a close connection to brain damage and a key role of the phagocytic Nadph oxidase (Nox-2). Eur Heart J. (2020) 41:2472–83. doi: 10.1093/eurheartj/ehz772
126. Lestari, KS, Humairo, MV, and Agustina, U. Formaldehyde vapor concentration in electronic cigarettes and health complaints of electronic cigarettes smokers in Indonesia. J Environ Public Health. (2018) 2018:1–6. doi: 10.1155/2018/9013430
127. Li, F, Yujie, Q, Gong, S, Zhang, H, and Ding, S. Learning and memory impairment of mice caused by gaseous formaldehyde. Environ Res. (2020) 184:109318. doi: 10.1016/j.envres.2020.109318
128. Cheng, J, Zhang, L, Tang, Y, and Li, Z. The toxicity of continuous long-term low-dose formaldehyde inhalation in mice. Immunopharmacol Immunotoxicol. (2016) 38:495–501. doi: 10.1080/08923973.2016.1248844
129. Sari, DK, Kuwahara, S, Tsukamoto, Y, Hori, H, Kunugita, N, Arashidani, K, et al. Effect of prolonged exposure to low concentrations of formaldehyde on the Corticotropin releasing hormone neurons in the hypothalamus and adrenocorticotropic hormone cells in the pituitary gland in female mice. Brain Res. (2004) 1013:107–16. doi: 10.1016/j.brainres.2004.03.070
130. Raadsheer, FC, Hoogendijk, WJG, Stam, FC, Tilders, FJH, and Swaab, DF. Increased numbers of Corticotropin-releasing hormone expressing neurons in the hypothalamic paraventricular nucleus of depressed patients. Neuroendocrinology. (1994) 60:436–44. doi: 10.1159/000126778
131. Olmedo, P, Rodrigo, L, Grau-Pérez, M, Hilpert, M, Navas-Acién, A, Téllez-Plaza, M, et al. Metal exposure and biomarker levels among E-cigarette users in Spain. Environ Res. (2021) 202:111667. doi: 10.1016/j.envres.2021.111667
132. Williams, M, Villarreal, A, Bozhilov, K, Lin, S, and Talbot, P. Metal and silicate particles including nanoparticles are present in electronic cigarette cartomizer fluid and aerosol. PLoS One. (2013) 8:e57987. doi: 10.1371/journal.pone.0057987
133. Zhao, D, Aravindakshan, A, Hilpert, M, Olmedo, P, Rule, AM, Navas-Acien, A, et al. Metal/metalloid levels in electronic cigarette liquids, aerosols, and human biosamples: a systematic review. Environ Health Perspect. (2020) 128:036001. doi: 10.1289/EHP5686
134. Re, DB, Hilpert, M, Saglimbeni, B, Strait, M, Ilievski, V, Coady, M, et al. Exposure to E-cigarette aerosol over two months induces accumulation of neurotoxic metals and alteration of essential metals in mouse brain. Environ Res. (2021) 202:111557. doi: 10.1016/j.envres.2021.111557
135. Hess, CA, Olmedo, P, Navas-Acien, A, Goessler, W, Cohen, JE, and Rule, AM. E-cigarettes as a source of toxic and potentially carcinogenic metals. Environ Res. (2017) 152:221–5. doi: 10.1016/j.envres.2016.09.026
136. Salama, A, Hegazy, R, and Hassan, A. Intranasal chromium induces acute brain and lung injuries in rats: assessment of different potential hazardous effects of environmental and occupational exposure to chromium and introduction of a novel pharmacological and toxicological animal model. PLoS One. (2016) 11:e0168688. doi: 10.1371/journal.pone.0168688
137. Saritha, S, Davuljigari, CB, Kumar, KP, and Reddy, GR. Effects of combined arsenic and Lead exposure on the brain monoaminergic system and Behavioral functions in rats: reversal effect of Miadmsa. Toxicol Ind Health. (2019) 35:89–108. doi: 10.1177/0748233718814990
138. Omaiye, EE, Williams, M, Bozhilov, KN, and Talbot, P. Design features and elemental/metal analysis of the atomizers in pod-style electronic cigarettes. PLoS One. (2021) 16:e0248127. doi: 10.1371/journal.pone.0248127
139. Brown, CJ, and Cheng, JM. Electronic cigarettes: product characterisation and design considerations. Tob Control. (2014) 23:4–10. doi: 10.1136/tobaccocontrol-2013-051476
140. Pinto, MI, Thissen, J, Hermes, N, Cunningham, A, Digard, H, and Murphy, J. Chemical characterisation of the vapour emitted by an E-cigarette using a ceramic Wick-based technology. Sci Rep. (2022) 12:16497. doi: 10.1038/s41598-022-19761-w
141. Zhu, S-H, Sun, JY, Bonnevie, E, Cummins, SE, Gamst, A, Yin, L, et al. Four hundred and sixty brands of E-cigarettes and counting: implications for product regulation. Tob Control. (2014) 23:iii3–9. doi: 10.1136/tobaccocontrol-2014-051670
142. Romijnders, K, Boesveldt, G, and Vries, T. E-liquid flavor preferences and individual factors related to vaping: a survey among Dutch never-users, smokers, dual users, and exclusive vapers. Int J Environ Res Public Health. (2019) 16:4661. doi: 10.3390/ijerph16234661
143. Berg, CJ . Preferred Flavors and reasons for E-cigarette use and discontinued use among never, current, and former smokers. Int J Public Health. (2016) 61:225–36. doi: 10.1007/s00038-015-0764-x
144. Chen, JC, Green, K, Fryer, C, and Borzekowski, D. Perceptions about E-cigarette Flavors: a qualitative investigation of Young adult cigarette smokers who use E-cigarettes. Addict Res Theory. (2019) 27:420–8. doi: 10.1080/16066359.2018.1540693
145. Li, W, Vargas-Rivera, M, Eissenberg, TE, Shihadeh, A, Talih, S, and Maziak, W. Effect of menthol/mint-Flavored pods on Young Juul E-cigarette Users' subjective experience, puffing behavior, and nicotine exposure: a pilot study. Drug Alcohol Depend. (2022) 237:109516. doi: 10.1016/j.drugalcdep.2022.109516
146. Foulds, J, Hooper, MW, Pletcher, MJ, and Okuyemi, KS. Do smokers of menthol cigarettes find it harder to quit smoking? Nicotine Tob Res. (2010) 12:S102–9. doi: 10.1093/ntr/ntq166
147. Wickham, RJ . The biological impact of menthol on tobacco dependence. Nicot Tobacco Res. (2020) 22:1676–84. doi: 10.1093/ntr/ntz239
148. Lallai, V, and Fowler, CD. More than just Chillin': interactive effects of menthol and nicotine in drug reward. Neuropsychopharmacology. (2017) 42:2283–4. doi: 10.1038/npp.2017.107
149. Okuyemi, KS, Faseru, B, Sanderson Cox, L, Bronars, CA, and Ahluwalia, JS. Relationship between menthol cigarettes and smoking cessation among African American light smokers. Addiction. (2007) 102:1979–86. doi: 10.1111/j.1360-0443.2007.02010.x
150. Harris, AC, Muelken, P, Swain, Y, Palumbo, M, Jain, V, Goniewicz, ML, et al. Non-nicotine constituents in E-cigarette aerosol extract attenuate Nicotine's aversive effects in adolescent rats. Drug Alcohol Depend. (2019) 203:51–60. doi: 10.1016/j.drugalcdep.2019.05.023
151. Biswas, L, Harrison, E, Gong, Y, Avusula, R, Lee, J, Zhang, M, et al. Enhancing effect of menthol on nicotine self-Administration in Rats. Psychopharmacology. (2016) 233:3417–27. doi: 10.1007/s00213-016-4391-x
152. Jackson, A, Green, B, Erythropel, HC, Kong, G, Cavallo, DA, Eid, T, et al. Influence of menthol and Green apple E-liquids containing different nicotine concentrations among youth E-cigarette users. Exp Clin Psychopharmacol. (2021) 29:355–65. doi: 10.1037/pha0000368
153. Avelar, AJ, Akers, AT, Baumgard, ZJ, Cooper, SY, Casinelli, GP, and Henderson, BJ. Why Flavored vape products may be attractive: Green apple tobacco flavor elicits reward-related behavior, upregulates Nachrs on Vta dopamine neurons, and alters midbrain dopamine and Gaba neuron function. Neuropharmacology. (2019) 158:107729. doi: 10.1016/j.neuropharm.2019.107729
154. Cooper, SY, Akers, AT, and Henderson, BJ. Green apple E-cigarette Flavorant Farnesene triggers reward-related behavior by promoting high-sensitivity Nachrs in the ventral tegmental area. eNeuro. (2020) 7:ENEURO.0172-20.2020. doi: 10.1523/ENEURO.0172-20.2020
155. Zhang, W, Lin, H, Zou, M, Yuan, Q, Huang, Z, Pan, X, et al. Nicotine in inflammatory diseases: anti-inflammatory and pro-inflammatory effects. Front Immunol. (2022) 13:826889. doi: 10.3389/fimmu.2022.826889
156. Filippini, P, Cesario, A, Fini, M, Locatelli, F, and Rutella, S. The Yin and Yang of non-neuronal Alpha7-nicotinic receptors in inflammation and autoimmunity. Curr Drug Targets. (2012) 13:644–55. doi: 10.2174/138945012800399008
157. Piao, W-H, Campagnolo, D, Dayao, C, Lukas, RJ, Wu, J, and Shi, F-D. Nicotine and inflammatory neurological disorders. Acta Pharmacol Sin. (2009) 30:715–22. doi: 10.1038/aps.2009.67
158. Prasedya, ES, Ambana, Y, Martyasari, NWR, Aprizal, YM, and Nurrijawati, S. Short-term E-cigarette toxicity effects on brain cognitive memory functions and inflammatory responses in mice. Toxicol Res. (2020) 36:267–73. doi: 10.1007/s43188-019-00031-3
159. Moshensky, A, Brand, CS, Alhaddad, H, Shin, J, Masso-Silva, JA, Advani, I, et al. Effects of mango and mint pod-based E-cigarette aerosol inhalation on inflammatory states of the brain, lung, heart, and Colon in mice. elife. (2022) 11:e67621. doi: 10.7554/eLife.67621
160. Adeluyi, A, Guerin, L, Fisher, ML, Galloway, A, Cole, RD, Chan, SSL, et al. Microglia morphology and Proinflammatory Signaling in the nucleus Accumbens during nicotine withdrawal. Sci Adv. (2019) 5:eaax7031. doi: 10.1126/sciadv.aax7031
161. Zevin, S, and Benowitz, NL. Drug interactions with tobacco smoking. Clin Pharmacokinet. (1999) 36:425–38. doi: 10.2165/00003088-199936060-00004
162. Zuikova, E, Ioannides, C, Bailey, A, and Marczylo, T. Effects of nicotine and E-cigarette fluids on cytochromes P450 in Hcmec/D3 blood-brain barrier cell line. Tobacco Prevention Cessation. (2018) 4:90508. doi: 10.18332/tpc/90508
163. Winters, BR, Kochar, TK, Clapp, PW, Jaspers, I, and Madden, MC. Impact of E-cigarette liquid Flavoring agents on activity of microsomal recombinant Cyp2a6, the primary nicotine-metabolizing enzyme. Chem Res Toxicol. (2020) 33:1689–97. doi: 10.1021/acs.chemrestox.9b00514
164. Sun, Y-W, Kosinska, W, and Guttenplan, JB. E-cigarette aerosol condensate enhances metabolism of benzo(a)pyrene to genotoxic products, and induces Cyp1a1 and Cyp1b1, likely by activation of the aryl hydrocarbon receptor. Int J Environ Res Public Health. (2019) 16:2468. doi: 10.3390/ijerph16142468
165. Quattrochi, LC, and Tukey, RH. The human cytochrome Cyp1a2 gene contains regulatory elements responsive to 3-Methylcholanthrene. Mol Pharmacol. (1989) 36:66–71.
166. Xu, M, Tang, H, Rong, Q, Zhang, Y, Li, Y, Zhao, L, et al. The effects of formaldehyde on cytochrome P450 isoform activity in rats. Biomed Res Int. (2017) 2017:1–7. doi: 10.1155/2017/6525474
167. Khokhar, JY, and Tyndale, RF. Drug metabolism within the brain changes drug response: selective manipulation of brain Cyp2b alters Propofol effects. Neuropsychopharmacology. (2011) 36:692–700. doi: 10.1038/npp.2010.202
168. Cacabelos, R, and Torrellas, C. Pharmacogenomics of antidepressants. J Psychiatry Depression Anxiety. (2015) 3077–3088. doi: 10.24966/PDA-0150/100001
169. Yue, J, Miksys, S, Hoffmann, E, and Tyndale, RF. Chronic nicotine treatment induces rat Cyp2d in the brain but not in the liver: an investigation of induction and time course. J Psychiatry Neurosci. (2008) 33:54–63.
170. Miller, RT, Miksys, S, Hoffmann, E, and Tyndale, RF. Ethanol self-administration and nicotine treatment increase brain levels of Cyp2d in African Green monkeys. Br J Pharmacol. (2014) 171:3077–88. doi: 10.1111/bph.12652
171. McMillan, DM, and Tyndale, RF. Nicotine increases codeine analgesia through the induction of brain Cyp2d and central activation of codeine to morphine. Neuropsychopharmacology. (2015) 40:1804–12. doi: 10.1038/npp.2015.32
172. Thomsen, MS, Loft, S, Roberts, DW, and Poulsen, HE. Cytochrome P4502e1 inhibition by propylene glycol prevents acetaminophen (paracetamol) hepatotoxicity in mice without cytochrome P4501a2 inhibition. Pharmacol Toxicol. (1995) 76:395–9. doi: 10.1111/j.1600-0773.1995.tb00168.x
173. Cooper, KO, Witz, G, and Witmer, C. The effects of alpha, Beta-unsaturated aldehydes on hepatic thiols and thiol-containing enzymes. Fundam Appl Toxicol. (1992) 19:343–9. doi: 10.1016/0272-0590(92)90172-E
174. Tanner, JA, and Tyndale, RF. Variation in Cyp2a6 activity and personalized medicine. J Pers Med. (2017) 7:18. doi: 10.3390/jpm7040018
175. Dorado, P, Berecz, R, GonzLez, AP, PeAs-Lled, EM, and Llerena, AN. Effect of Cyp2d6 and Cyp2c9 genotypes on fluoxetine and Norfluoxetine plasma concentrations during steady-state conditions. Eur J Clin Pharmacol. (2004) 59:869–73. doi: 10.1007/s00228-003-0707-y
176. Brandl, EJ, Tiwari, AK, Zhou, X, Deluce, J, Kennedy, JL, Müller, DJ, et al. Influence of Cyp2d6 and Cyp2c19 gene variants on antidepressant response in obsessive-compulsive disorder. Pharm J. (2014) 14:176–81. doi: 10.1038/tpj.2013.12
177. Bijl, MJ, Visser, LE, Hofman, A, Vulto, AG, van Gelder, T, Stricker, BH, et al. Influence of the Cyp2d6*4 polymorphism on dose, switching and discontinuation of antidepressants. Br J Clin Pharmacol. (2008) 65:558–64. doi: 10.1111/j.1365-2125.2007.03052.x
Keywords: nicotine, anxiety, depression, e-cigarette, vape, constituents, CYP450
Citation: Bautista M, Mogul AS and Fowler CD (2023) Beyond the label: current evidence and future directions for the interrelationship between electronic cigarettes and mental health. Front. Psychiatry. 14:1134079. doi: 10.3389/fpsyt.2023.1134079
Edited by:
Kristine C-Y McGrath, University of Technology Sydney, AustraliaReviewed by:
Kabirullah Lutfy, Western University of Health Sciences, United StatesHyma Gogineni, Western University of Health Sciences, United States
Copyright © 2023 Bautista, Mogul and Fowler. This is an open-access article distributed under the terms of the Creative Commons Attribution License (CC BY). The use, distribution or reproduction in other forums is permitted, provided the original author(s) and the copyright owner(s) are credited and that the original publication in this journal is cited, in accordance with accepted academic practice. No use, distribution or reproduction is permitted which does not comply with these terms.
*Correspondence: Christie D. Fowler, Y2Rmb3dsZXJAdWNpLmVkdQ==