- 1School of Education, Xinyang College, Xinyang, China
- 2Department of Radiology, Xijing Hospital, Fourth Military Medical University, Xi’an, China
- 3Department of Mental Health, Xi’an Medical University, Xi’an, China
- 4Department of Psychiatry, Second Affiliated Hospital, Army Medical University, Chongqing, China
- 5Department of Psychiatry, Xijing Hospital, Fourth Military Medical University, Xi’an, China
- 6Department of Clinical Psychology, Fourth Military Medical University, Xi’an, China
- 7Department of Military Medical Psychology, Fourth Military Medical University, Xi’an, China
Background: Auditory verbal hallucinations (AVH) are a core symptom of schizophrenia. Low-frequency (e.g., 1 Hz) repetitive transcranial magnetic stimulation (rTMS) targeting language processing regions (e.g., left TPJ) has been evident as a potential treatment for AVH. However, the underlying neural mechanisms of the rTMS treatment effect remain unclear. The present study aimed to investigate the effects of 1 Hz rTMS on functional connectivity (FC) of the temporoparietal junction area (TPJ) seed with the whole brain in schizophrenia patients with AVH.
Methods: Using a single-blind placebo-controlled randomized clinical trial, 55 patients with AVH were randomly divided into active treatment group (n = 30) or placebo group (n = 25). The active treatment group receive 15-day 1 Hz rTMS stimulation to the left TPJ, whereas the placebo group received sham rTMS stimulation to the same site. Resting-state fMRI scans and clinical measures were acquired for all patients before and after treatment. The seed-based (left TPJ) static and DFC was used to assess the connectivity characteristics during rTMS treatment in patients with AVH.
Results: Overall, symptom improvement following 1 Hz rTMS treatment was found in the active treatment group, whereas no change occurred in the placebo group. Moreover, decreased static FC (SFC) of the left TPJ with the right temporal lobes, as well as increased SFC with the prefrontal cortex and subcortical structure were observed in active rTMS group. Increased dynamic FC (DFC) of the left TPJ with frontoparietal areas was also found in the active rTMS group. However, seed-based SFC and DFC were reduced to a great extent in the placebo group. In addition, these changed FC (SFC) strengths in the active rTMS group were associated with reduced severity of clinical outcomes (e.g., positive symptoms).
Conclusion: The application of 1 Hz rTMS over the left TPJ may affect connectivity characteristics of the targeted region and contribute to clinical improvement, which shed light on the therapeutic effect of rTMS on schizophrenia with AVH.
1. Introduction
Auditory verbal hallucinations (AVH) are the most characteristic symptom of schizophrenia (1) and are defined as the conviction of hearing and perceiving “sound” without corresponding external stimulus input (2). Despite years of research, the neural mechanism of AVH continues to be unclear. Recently, neuroimaging studies have demonstrated the underlying functional and structural abnormalities associated with AVH in distributed brain regions, including Broca’s area, insula, hippocampal, and subcortical regions (3–6), which involved in speech processing, attention, and memory. The most consistent report is that the speech-processing regions of the superior temporal cortex are abnormal in terms of functional (7, 8) and anatomical (9, 10) alterations in schizophrenia with AVH.
Repetitive transcranial magnetic stimulation (rTMS) applies a repetitive pulsed magnetic field to the scalp, inducing an electric field in a discrete region of the brain (11), and thus alters the neuronal activity underneath the stimulate target (12). rTMS in low-frequency mode is thought to result in cortical inhibition (13). Low-frequency rTMS has been evident as a treatment for AVH. In the first study, Hoffman and colleagues indicated a reduction in AVH after 1-Hz rTMS targeting the superior temporal gyrus in schizophrenia patients compared to sham stimulation (14). Subsequently, numerous studies have replicated the preliminary finding (15–19). Meta-analysis studies have demonstrated a medium to a large effect size of low-frequency rTMS on AVH (20–22), although negative findings were reported (23, 24). The evidence supports the potential of low-frequency rTMS for the reduction of AVH. However, the underlying neural mechanisms of symptom improvement following rTMS treatment need further clarification.
Schizophrenia has been considered a dysconnectivity syndrome (25). And abnormal functional connectivity (FC) patterns between the distributed brain areas have been found in schizophrenia patients with AVH (26–28) and have been associated with the clinical severity of AVH (29). Interestingly, several studies have reported the effects of low-frequency rTMS on brain activity and connectivity in schizophrenia with AVH. In a small sample study, increased task-related activity in brain areas that involved in speech processing was observed in patients with AVH after 1 Hz rTMS treatment (30). Low-frequency rTMS mode also reduced brain metabolism in the left superior temporary gyrus and interconnected region, as well as enhanced metabolism in the contralateral cortex and the frontal lobes in schizophrenia patients with AVH (31).
Using the left temporoparietal junction area (TPJ) as seed, Vercammen and colleagues have found an increased FC between the seed and right insula in patients receiving 1 Hz rTMS applied to the left TPJ, while the sham stimulation group showed a decreased FC between the seed and left anterior cingulate (32). Recently, our study has demonstrated that low-frequency rTMS treatment in schizophrenia patients with AVH can induce the global and local topological properties changes of the whole brain functional network and were associated with the reduction of AVH (33). The results suggest that the application of low-frequency rTMS targeting the left TPJ may affect neural activity and connectivity in the targeted site and associated remote regions.
The purpose of present study was to investigate the underlying mechanism of 1 Hz rTMS treatment on schizophrenia with AVH using seed-based FC analysis. Using the left TPJ as seed, on the one hand, we replicated the results from the previous study (32) by using the static functional connectivity (SFC) analysis; on the other hand, provided Supplementary information by using seed-based dynamic functional connective (DFC) approach. We speculated that 1 Hz rTMS would induce beneficial static or dynamic FC (DFC) changes of left TPJ seed in patients with AVH after treatment and could be related to the improvement of clinical symptoms.
2. Material and methods
2.1. Participants
Fifty-eight patients were recruited in the study. Randomization of patients was performed outside the study group by simple random number generated from computer. Patients were allocated to one of the treatment conditions: rTMS stimulation to the left TPJ region (n = 32) or sham stimulation to the same site (n = 26). Supplementary Figure 1 shows the CONSORT flowchart with study enrollment, visits, and attrition. The diagnosis was confirmed by experienced psychiatrists according to the criteria of the Structured Clinical Interview for Diagnosis and Statistical Manual of Mental Disorder (DSM-V). The inclusion criteria for the patients met the follows: (1) AVH daily occurred with no less than two antipsychotic medications, and (2) at least five episodes of AVH per day during the past month. All patients received a stable dose of antipsychotic medications throughout the study period. All groups were matched on age, gender, education, and duration of illness. Exclusion criteria for all patients included the follows: (1) previous or current neurological disease, (2) history of head injury, (3) alcohol or drug abuse, and (4) contraindications to MRI scans.
Informed consent was obtained from all participants. The investigation was carried out by the Declaration of Helsinki and approved by the Medical Ethnic Committee of Xijing Hospital. This study was registered in China Clinical Trials (registration number: ChiCTR2100041876).1
2.2. Clinical measurements
The psychotic symptoms were evaluated by the positive and negative symptom scale (PANSS) (34), and the severity of AVH was assessed by the auditory hallucination rating scale (AHRS) (15). All clinical measures were executed by experienced psychiatrists at pretreatment and posttreatment for all patients.
2.3. rTMS procedure
Unlike our previous studies using case-control paradigm (33, 35–37), this study had a single-blind parallel design, only the rTMS administrator rather than researchers or raters knew the conditions to which patients were assigned. Stimulation was performed by a Magstim Rapid System (YIRUDE, Wuhan, China), using a 70 mm figure-of-eight coil. The stimulation site was determined based on the 10–20 international electrode location system (T3/P3). Active rTMS stimulation was carried out at 110% of the individual resting motor threshold (MT). The placebo condition was stimulated with a Magstim sham coil at the same location, which produced a similar sound stimulus, but no magnetic field through the skull. Each patient received a total of 15 rTMS sessions, each lasting 15 min, for a total of 9,000 pluses, over the course of 15 consecutive days.
2.4. Neuroimaging data acquisition and processing
Images were acquired on a 3.0-Tesla scanner (GE Medical Systems, Milwaukee, WI) with a standard 8-channel phased-array head coil. The images were scanned twice in patients (baseline and after treatment). Participants were instructed to stay awake and kept their eyes closed during the scanning duration. Functional images were collected using a gradient-echo-planar imaging sequence with the following parameters: repetitive time (RT) = 2,000 ms, echo time (ET) = 40 ms, matrix = 64 × 64, field of view (FOV) = 260 × 260 mm2, flip angle = 90°, 45 slices, with 3.5 mm slice thickness and no gap. A total of 210 whole brain volumes were acquired. An anatomical image was also obtained using an MP-RAGE sequence with the following parameters: TR = 8.1 ms, TE = 3.2 ms, matrix size = 256 × 256, flip angle = 12°, FOV = 240 × 240 mm2, 176 slices, and with 1.0 mm thickness (no gap).
Data were processed in Matlab 2018b platform (MathWorks, Natick, MA) using the DPABI toolbox.2 For each subject, the first 10 functional images were discarded and were then corrected for differences in slice timing and head motion. The corrected images were coregistered to the T1-weighted anatomical image. The coregistered anatomical images were segmented into gray matter, white matter, and cerebrospinal fluid. The following images were normalized into standardized Montreal Neurological Institute (MNI) space by the DARTEL algorithm and smoothed with a 6 mm Gaussian kernel. Subsequently, linear detrending and temporal filtering (0.01–0.1 Hz) were executed. Finally, nuisance covariates, including head motion, white matter signal, cerebrospinal fluid signal, and global mean signal, were regressed out.
2.5. Static functional connectivity analysis
Based on the previous study (32), the left TPJ seed was defined as a 10 mm radius sphere surrounding a central voxel (Figure 1). Resting-State fMRI Data Analysis (REST) toolkit (38) was used to calculate the seed-based resting-state SFC. The Pearson correlation coefficients between the time courses of the seed and the time series of each voxel of the whole brain were calculated. Correlation coefficients were transformed into Z-map with Fisher’s transformations to improve the normality of the data.
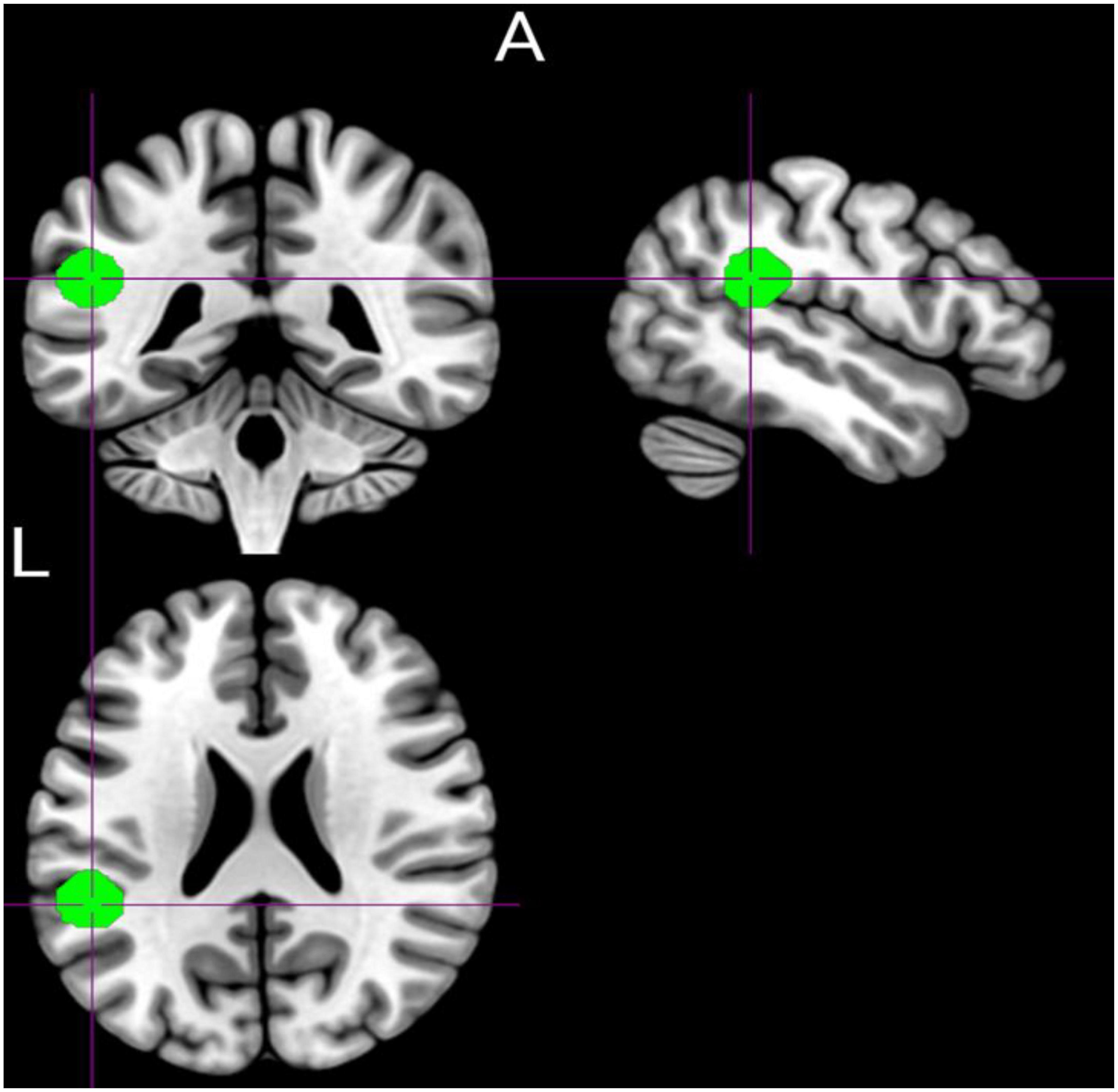
Figure 1. Graphical representation of the prior defined region of interest (left temporoparietal junction area) was defined using the Marsbar toolbox (MNI: −50.7, −41.4, 22.7).
2.6. Dynamic functional connectivity analysis
The DFC was computed by using a sliding window approach through the DynamicBC toolbox (39). DFC calculates the correlation of time series according to a certain length of the sliding window. Based on the previous literature (40, 41), the length of the sliding window was set a 50 TRs, and the window overlap was set at 90%, resulting 31 windows for each participant. The temporal correlation coefficient between the time course of seed and time series all brain voxels within each sliding window was calculated and represented the DFC changes occurring entire scan course. Unlike the classic DFC studies that applied the k-means clustering algorithm to measure the frequency and structure of reoccurring FC patterns based on windowed covariance matrices between the predefined regions of interest (ROIs) or independent components (ICs) (42, 43), the variance of each voxel across all windows on seed-based DFC denoted the temporal variability in the strength of connectivity. Higher DFC variability indicated greater fluctuations of FC strength over time. Referring to the previous study (40, 44), the Z-valued FC variance maps were used in statistical analyses. To verity the results of DFC, the window size was changed with 40 TRs (overlap 90%) reanalyzed DFC for all participants.
2.7. Statistical analysis
Independent-sample t-test and chi-square test were used to test the clinical characteristic differences between the active treatment group and placebo group according to the nature of the data. In addition, a paired-sample t-test was used to test the changes in symptoms and FC between the patients before and after treatment. All results were controlled with age, sex, and head motion as covariates and were reported applied the Gaussian random field (GRF) correction with a voxel-level threshold of p < 0.05 and a cluster-level threshold of p < 0.05, the minus cluster size was set 30.
2.8. Correlation analysis
To investigate the associations between altered FC values and clinical responses, the mean values of altered SFC and DFC were extracted and then correlated with the scores of clinical responses with Pearson correlation analysis. A two-tailed p-level of 0.05 was considered as the criterion of statistical significance and corrected for multiple comparisons with the false discovery rate correction (FDR) method.
3. Results
3.1. Patient characteristics
Due to excessive head movement (head motion parameters are greater than 3.0 mm and 3.0 degrees), data from three patients had to be excluded, leaving 30 patients in the active treatment group and 25 patients in the placebo group. There were no statistical significances between the two group in demographical variables, including age (t = 0.644, p = 0.478), sex (χ2 = 0.045, p = 0.832), and education (t = 0.458, p = 0.756), and clinical measures, including illness duration (t = 0.525, p = 0.602), medication dosage (t = 0.218, p = 0.828), positive symptom (t = 0.983, p = 0.330), negative symptom (t = 0.178, p = 0.860), general symptom (t = 0.537, p = 0.593), and AVH (t = 0.689, p = 0.494). Details are displayed in Table 1.
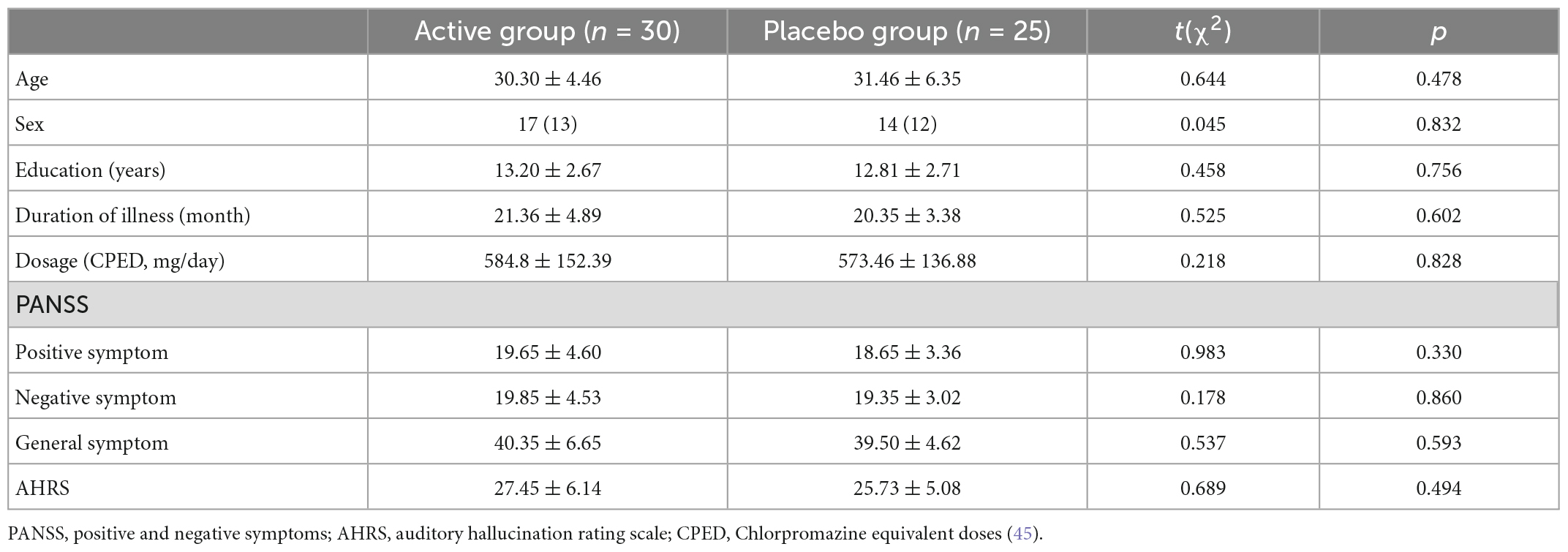
Table 1. Demographic and clinical characteristics of the two patient groups receiving either rTMS or placebo treatment.
3.2. Treatment effect of rTMS
Changes in clinical symptoms over time were assessed separately for two groups. Pretreatment and posttreatment measures showed significant decreases in positive symptom (t = 6.197, p = 0.000), general symptom (t = 2.661, p = 0.011), and AVH (t = 6.542, p = 0.000) scores in the active treatment group (Figure 2A). But the clinical measures showed no change at all in the placebo group over time (all p > 0.05) (Figure 2B).
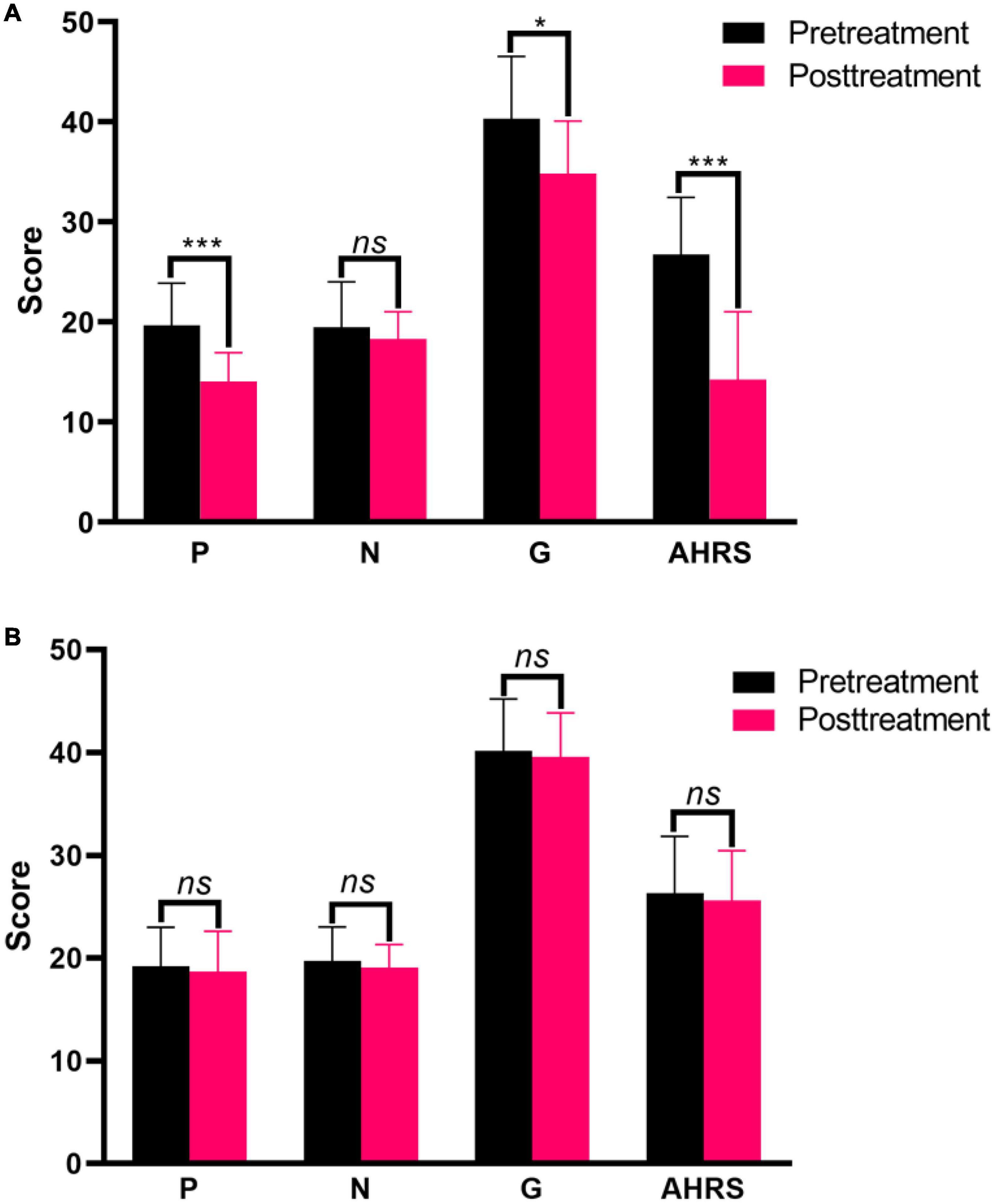
Figure 2. Clinical symptom responses between before and after treatment in the active treatment group (A) and placebo group (B). *p < 0.05, ***p < 0.001. ns, non-significance.
3.3. Static functional connectivity analysis of left TPJ seed
For SFC analysis, the active treatment group showed significant FC changes in the left TPJ seed over the course of the treatment (Table 2 and Figure 3). Specifically, the patients in the active treatment group had increased FC with the right superior frontal gyrus, right supplementary motor, and bilateral putamen, as well as decreased FC with the right inferior temporal gyrus and right middle temporal gyrus (Figure 3A).
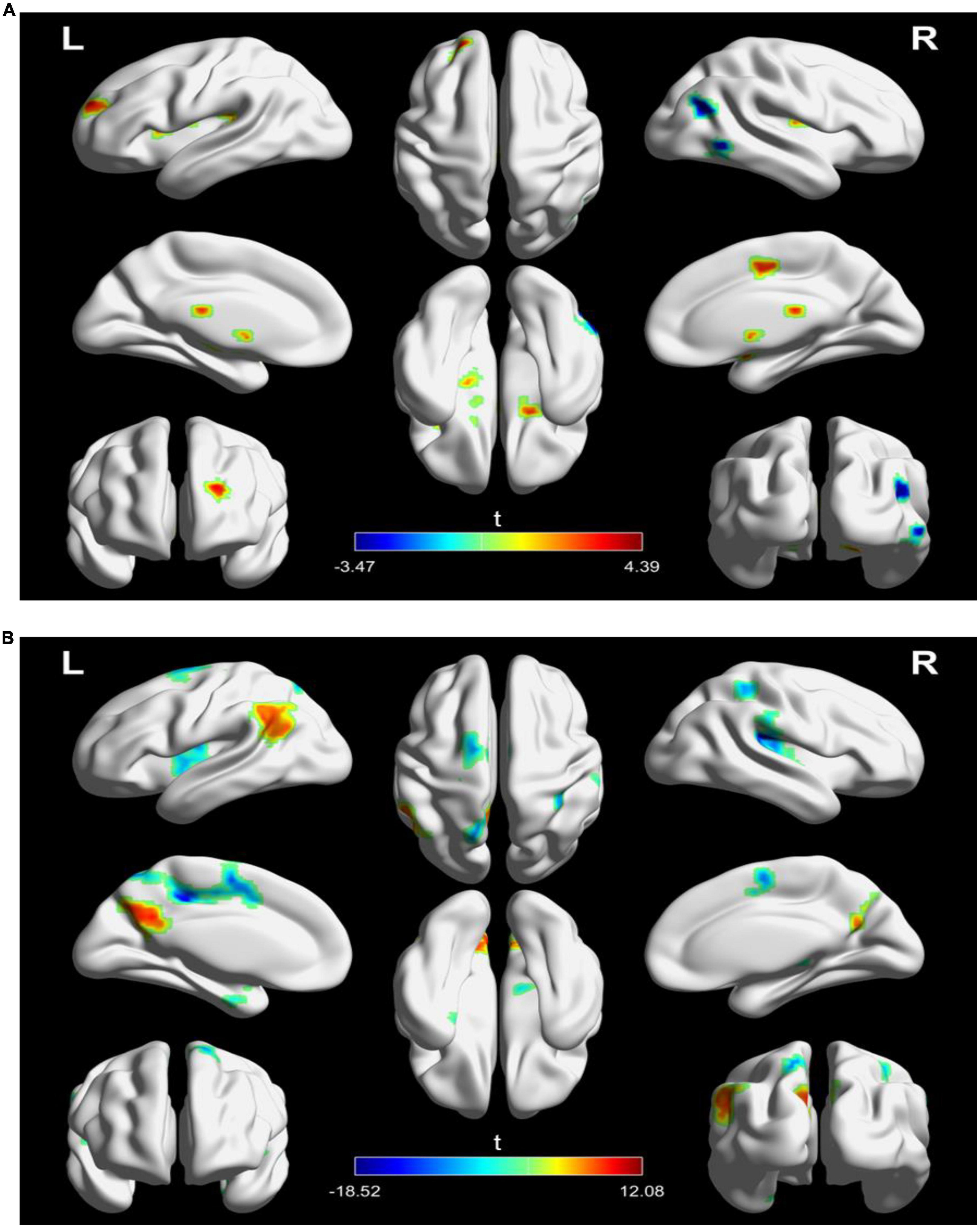
Figure 3. Significantly changes in static functional connectivity (SFC) of the left TPJ seed between before and after treatment in the two groups. (A) Significantly changes in SFC of the left TPJ seed in the active treatment group (posttreatment vs. pretreatment). (B) Significantly changes in SFC of the left TPJ seed in the placebo group (posttreatment vs. pretreatment). The warm color represents higher connectivity, and the cool color represents lower connectivity (GRF correction, p < 0.05, size > 30). TPJ, left temporoparietal junction; GRF, Gaussian random field.
The placebo group on the other hand showed increased FC of the left TPJ with the left parietal cortex (e.g., angular and precuneus), as well as decreased FC of the left TPJ with left middle cingulate gyrus and bilateral insula (Figure 3A).
3.4. Dynamics functional connectivity analysis of left TPJ seed
For DFC analysis, there was a significantly increased DFC between the seed and left inferior frontal gyrus and right inferior parietal lobule in the active treatment group after treatment (Table 3 and Figure 4A). But decreased DFC of the seed with the right inferior occipital gyrus, right anterior cingulate gyrus, and right superior parietal lobule was observed in the placebo group (Table 3 and Figure 4B).
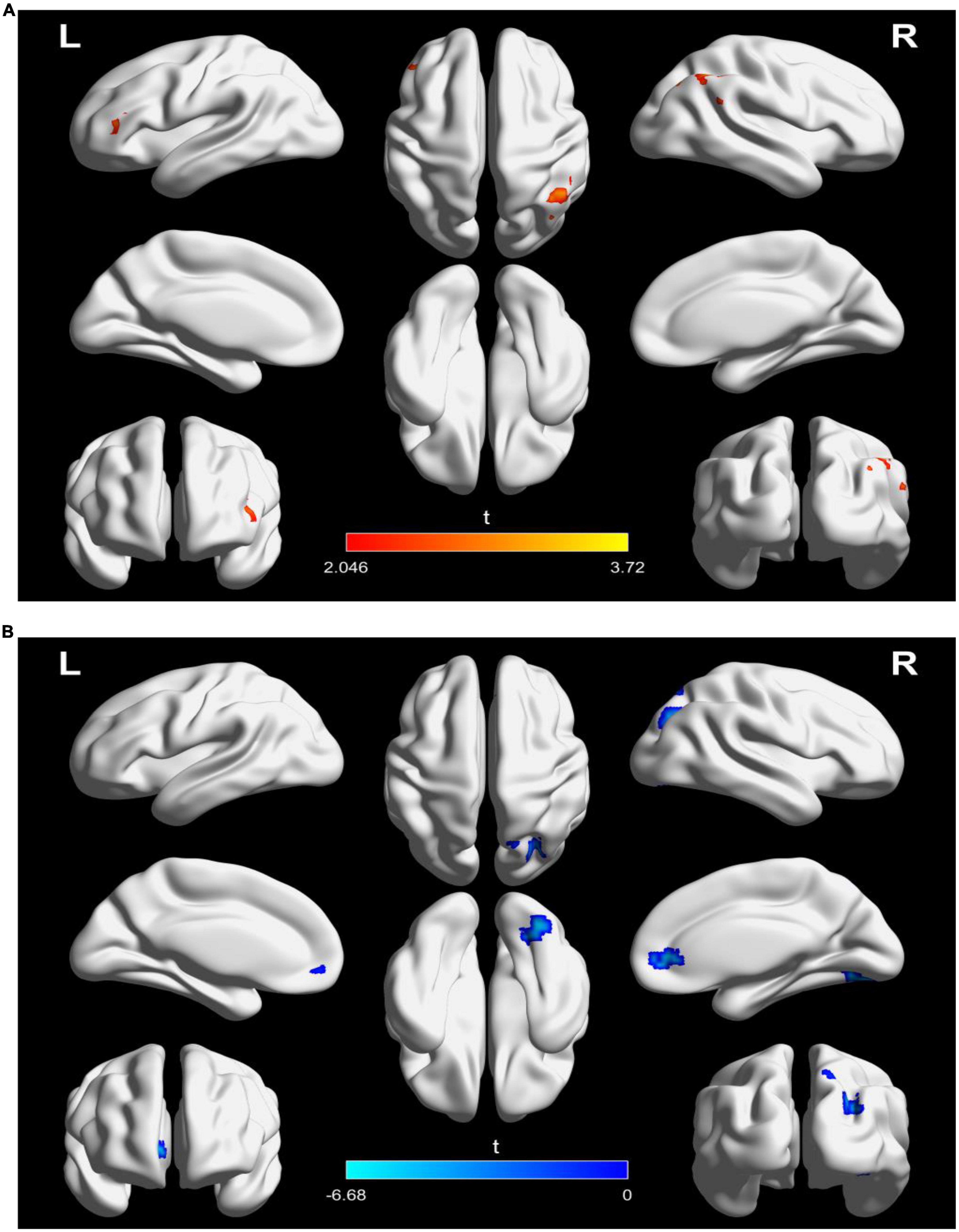
Figure 4. Significantly changes in dynamic functional connectivity (DFC) of the left TPJ seed between before and after treatment in the two groups. (A) Significantly changes in DFC of the left TPJ seed in the active treatment group (posttreatment vs. pretreatment). (B) Significantly changes in DFC of the left TPJ seed in the placebo group (posttreatment vs. pretreatment). The warm color represents higher connectivity, and the cool color represents lower connectivity (GRF correction, p < 0.05, size > 30). TPJ, left temporoparietal junction; GRF, Gaussian random field.
We performed a reproducibility analysis to verify that current findings reflected actual DFC changes in patients instead of difference of experimental parameters. For windows of 40 TRs with 90% overlap, we identified consistent alterations of DFC among the active treatment group and placebo group. The details are displayed in Supplementary Figure 2.
3.5. Correlation analysis results
The correlation analysis showed that the SFC different values between posttreatment and pretreatment in the right supplementary motor cortex (r = −0.4000, p = 0.029) and right putamen (r = −0.384, p = 0.036) were significantly negatively with the positive symptom score changes of PANSS in the active treatment group, respectively (Figure 5). In addition, the SFC different value between after and before treatment in the right inferior temporal gyrus was marginally significantly correlated with the AVH score change in the active treatment group (r = −0.349, p = 0.059) (Figure 5). However, the correlations between the other static or DFC different values and clinical responses did not reach the statistically significant level (all p > 0.05).
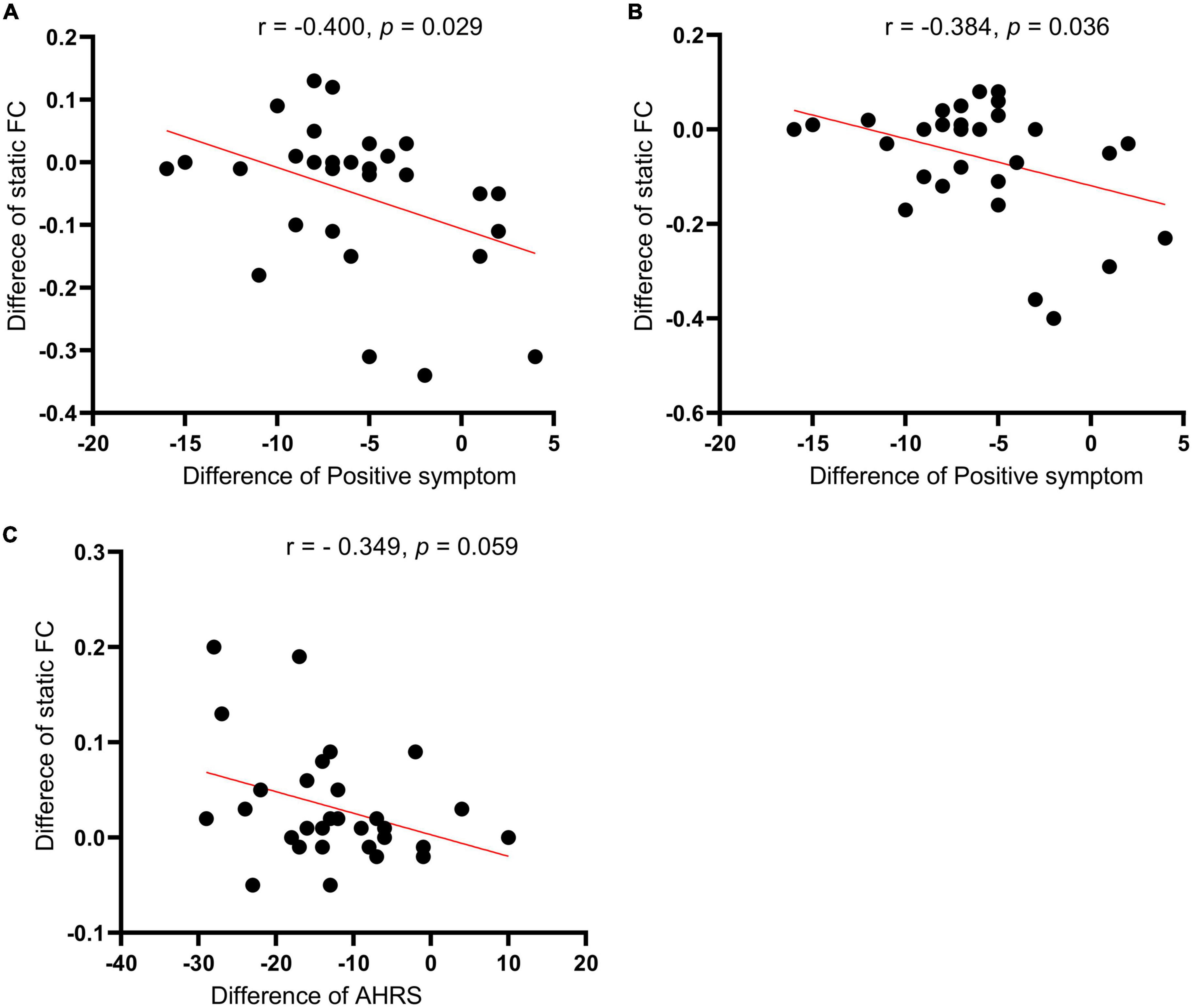
Figure 5. Correlations of the seed-based (left TPJ) static functional connectivity (SFC) difference values (posttreatment vs. pretreatment) in the left supplementary motor cortex (A) and right putamen (B) with the positive symptom score changes of PNASS in active treatment group. (C) Correlations of the seed-based SFC difference values (posttreatment vs. pretreatment) in the right inferior temporal gyrus with AVH score changes in active treatment group. TPJ, left temporoparietal junction; PANSS, positive and negative symptom scale; AHRS, auditory hallucination rating scale.
4. Discussion
This study investigated the effects of l Hz rTMS on static and DFC in schizophrenia patients with AVH using the stimulation target (left TPJ) as seed. The results showed that active rTMS treatment could reduce the severity of clinical symptoms, whereas the placebo group appeared no symptom changes over the course of treatment. The results regarding alterations in clinical symptoms were fully consistent with the previous report (32). The results also agree with the meta-analysis studies (19, 20, 46) and confirmed the effectiveness of low-frequency rTMS treatment on AVH in schizophrenia with our previous studies (33, 35–37). Moreover, rTMS treatment induced beneficial connectivity changes of the seed in the active treatment group, including increased static FC (SFC) with prefrontal regions (e.g., right superior frontal gyrus and right supplementary motor cortex) and decreased SFC with the temporal lobe (e.g., right inferior temporal gyrus and middle temporal gyrus). In addition, increased DFC in the prefrontal (e.g., inferior frontal gyrus) and parietal (e.g., inferior parietal lobule) cortices was also observed in the active treatment group. But the placebo group showed a large proportion of reduced SFC or DFC of the seed after sham stimulation. In addition, these changed FC properties in the active treatment group were associated with a reduction in the severity of clinical symptoms (e.g., positive symptoms). These results were partially consistent with the previous study (32). Clinical characteristics and sample size of patients may be the main reasons for the differences in results. Our findings provided Supplementary information and suggested that induced broader FC changes relevant to the TPJ target site in schizophrenia patients could be the underlying neural mechanism of low-frequency rTMS treatment on AVH.
AVH may arise from a mismatch of inner speech and its attribution (47). The mismatch can be considered as a deficit in inner speech production or its perception and understanding (48). Functional imaging studies have explored the neural correlates of AVH and have suggested that experiencing AVH is primarily involved the language-related network associated with the Broca area, the anterior insula, the inferior frontal gyrus, the middle temporal gyrus, the superior temporal gyrus (4, 49). Although structural and functional neuroimaging evidence has suggested that schizophrenia is associated with reduced left-hemisphere language lateralization (50–52), the right hemisphere is apparently involved in the meditation of left hemisphere language function (53–55), since the disturbance in the interaction of hemispheres appears in schizophrenia (53). Previous studies have suggested that the inferior temporal gyrus and middle temporal gyrus are involved in language and semantic processing. Thus, the right inferior temporal gyrus and right middle temporal gyrus as left hemisphere homologs may play crucial roles in the pathophysiology of schizophrenia. AVH was associated with elevated metabolic activity and cortical involvement in the two regions (4, 56), which supports the hypothesis that AVH arises from the misinterpretation of inner speech and the aberrant activation of the langue-related areas.
There is evidence that schizophrenia is linked to dysfunction in distributed neural circuits (57, 58). rTMS has the potential to produce a distal effect that induces connection changes in remote brain regions beyond the stimulation site (59, 60) through long-term potentiation and long-term depression-like mechanisms (61). Studies have demonstrated that decreased metabolism in the auditor cortex, Broca area, and cingulate gyrus after TMS treatment relative to sham (62), which implies that the clinical effect of rTMS may come from a normalization of the hyperactivity of language regions involved in the emergence of AVH. In line with these findings, we found that low-frequency rTMS decreased the SFC of seed with the right temporal lobes (e.g., right inferior temporal gyrus and right middle temporal gyrus) in the active group and may suggest that the low-frequency TMS could have inhibitory effect on hyperconnectivity between the seed and language related areas, since rTMS in a low-frequency mode produce a reduction in cortical excitability of underlying brain tissue (63, 64). The previous studies suggest that rTMS might induce local changes at the stimulate site and adjacent remote areas (32, 65). Left TPJ as a critical stimulation target implicated in AVH generation and perception (3, 48). Then, decreased connectivity of the stimulate site with contralateral temporal lobes may decrease the likelihood of inner speech intrusions.
In contrast, increased SFC of the left TPJ seed with the brain areas, including the right superior frontal gyrus, right supplementary motor cortex, and bilateral putamen was observed in the active treatment group after rTMS stimulation. The low-frequency rTMS-induced strong connectivity with these areas might be explained by the release of transcallosal inhibition (66). For instance, enhanced activation is detected in the right hemisphere after left hemisphere injury (67). As we know, the superior frontal gyrus is located at the superior part of the prefrontal cortex and has been reported to be involved in a wide variety of cognitive and attention tasks (68, 69), which is a critical node of the cognitive control network (70). This cortex is associated with decreased cognitive control (71, 72) and decreased connectivity with other brain regions in schizophrenia patients (73, 74). The supplementary motor cortex participates in the preparation and execution of movements (75, 76), while the activation of this region is reduced during a motor task in schizophrenia patients (77) and may reflect the motor dysfunction in schizophrenia (78, 79), since motor symptom is one of clinical presentation of schizophrenia and frequently occur throughout the course of this disease (80, 81). In addition, the putamen is a subcortical structure that forms the basal ganglia and is involved in the regulation of perception and motor controls (82, 83). Degree centrality is decreased in the putamen in patients with AVH (84) and reflects abnormal connectivity of the putamen with the whole-brain network. Thus, the results mentioned above were agreed with the accumulating evidence (29, 85) and suggested that AVH are not solely associated with local brain dysfunction, but with abnormal neural networks, including cognitive control, motor, and perception processing systems.
However, low-frequency rTMS treatment over the left TPJ appeared to enhance the involvement of these dysfunctional brain regions and help restore their normal functions. Specifically, the putamen is rich in dopaminergic neurons, and abnormal dopaminergic transmission in the putamen is related to positive symptoms such as hallucinations (86). Therefore, increased connectivity with the putamen after active rTMS treatment in patients could normalize the dopaminergic activity of basal ganglia and contribute to the reduction of positive symptoms. Moreover, the supplementary motor cortex is involved in the imaging of speech in addition to movements (87). During an auditory verbal imagery task (imaging a sentence being spoken by another person), which requires generating and monitoring inner speech, schizophrenia patients with AVH had reduced activation in the supplementary motor area (88). The authors clarify that a predisposition to verbal hallucinations may be related to an inability to activate areas involved in monitoring inner language. In addition, the inferior temporal gyrus subserves language and sematic processing (89). Patients with AVH had increased local spontaneous neural activity in the inferior temporal gyrus (90). Thus, functional abnormalities in the cortices may cause a mismatch between perceived and predicted outcomes of intrinsic speech activity and associate with the experience of AVH. We found that rTMS treatment significantly (or marginally) induced the alternated connectivity of the TPJ with the right inferior temporal gyrus and supplementary motor cortex, and then could result in a restoration of the cortical functions and a reduction in the severity of clinical symptoms.
The human brain works in a dynamic approach to integrate, coordinate, and respond to external and internal stimuli across multiple time scales (43). Thus, DFC can capture the wealth of information contained within the time-varying features in interregional functional interactions and reveal additional alternations in schizophrenia that could not be discovered by SFC (91, 92). We found that increased connectivity of the seed with the left inferior frontal gyrus and right inferior parietal lobule were detected only by the DFC approach in the active treatment group after rTMS stimulation. The left inferior frontal gyrus is involved in langue processing. Underactivation of the left inferior frontal gyrus has been reported in patients with schizophrenia during various langue tasks, including verbal learning and speech comprehension (93), and semantic encoding (94). The inferior parietal lobule is known to be a central hub of multisensory integration (95). In schizophrenia, neuroimaging studies have found that the inferior parietal has reduced cortical thickness (96), functional activation (97), and hemispheric asymmetry (98). Deficits in this area can cause perception dysfunction in schizophrenia (99, 100). The enhanced synchronization of the seed with these two regions as detected by DFC in the active treatment group might be beneficial for the recovery of speech and perceptional functions. These findings provided a comprehensive understanding of the treatment effect of rTMS treatment in schizophrenia.
Despite wed did not observe any clinical improvements after placebo treatment, some alternations based on the SFC and DFC analyses were presented in the placebo group, similar to the previous study (32). The decreased connectivity of the seed with the most brain regions, including the cingulate cortex, insula, superior parietal lobule, and inferior occipital lobe, was observed, which may represent the continuous deterioration of clinical symptoms over time, since hypoconnectity is dominant in schizophrenia (101, 102) and AVH (103, 104), and these regions critically represent the pathophysiology of schizophrenia (105–107) as well as AVH (49, 108–110). On the contrast, the placebo group also had increased connectivity of the seed with certain parietal areas (e.g., left angular and left precuneus) by SFC analysis. Abnormal functional (e.g., hypoactivation) (111, 112) and structural (e.g., reduced gray matter volume) (113, 114) alternations in the left angular and left precuneus have been reported in schizophrenia and appeared to be correlated with the severity of AVH (115, 116). Thus, the increased synchronization in these brain regions underlies core deficits of schizophrenia and may imply a neural compensation to overcome the primary functional defects in patients with AVH. Nevertheless, the results suggested that future studies may need to adequately seek the placebo effects and real rTMS effects by directly comparing placebo-responders and rTMS responder following rTMS treatment.
5. Conclusion
In summary, we observed that clinical improvement in the active treatment group coincided with FC alternations of the TPJ seed over the course of rTMS treatment. Low-frequency rTMS treatment of the left TPJ area could modulate neural circuits implicated in AVH, which contribute to the clinical improvement in schizophrenia.
Data availability statement
The original contributions presented in this study are included in the article/Supplementary material, further inquiries can be directed to the corresponding authors.
Ethics statement
The studies involving human participants were reviewed and approved by the Medical Ethics Committee of the Xijing Hospital. The patients/participants provided their written informed consent to participate in this study.
Author contributions
YX, MG, and ZW designed and organized the research. YX, YH, ZM, and ZW collected the imaging and clinical data. YX and PF analyzed the data. YX, MG, and YH wrote and revised the manuscript. PF and HW supervised the project and provided fund support. All authors contributed to the article and approved the submitted version.
Funding
This study was funded by the Natural Science Foundation of China (Nos. 81971255 and 81571651), Postdoctoral Science Foundation (No. 2019M653963), and the Military Medical Science and Technology Youth Training Program of China (No. 20QNPY049).
Conflict of interest
The authors declare that the research was conducted in the absence of any commercial or financial relationships that could be construed as a potential conflict of interest.
Publisher’s note
All claims expressed in this article are solely those of the authors and do not necessarily represent those of their affiliated organizations, or those of the publisher, the editors and the reviewers. Any product that may be evaluated in this article, or claim that may be made by its manufacturer, is not guaranteed or endorsed by the publisher.
Supplementary material
The Supplementary Material for this article can be found online at: https://www.frontiersin.org/articles/10.3389/fpsyt.2023.1071769/full#supplementary-material
Supplementary Figure 1 | The flowchart of study.
Supplementary Figure 2 | Significantly changes in dynamic functional connectivity (DFC) (window of 40 TRs with 90% overlap) of the left TPJ seed between before and after treatment in the two groups. (A) Significantly changes in DFC of the left TPJ seed in the active treatment group (posttreatment vs. pretreatment). (B) Significantly changes in DFC of the left TPJ seed in the placebo group (posttreatment vs. pretreatment). The warm color represents higher connectivity, and the cool color represents lower connectivity (GRF correction, p < 0.05, size > 30). TPJ, left temporoparietal junction; GRF, Gaussian random field.
Footnotes
References
1. Andreasen N, Flaum M. Schizophrenia: the characteristic symptoms. Schizophr Bull. (1991) 17:27–49. doi: 10.1093/schbul/17.1.27
2. Hugdahl K, Sommer I. Auditory Verbal Hallucinations in Schizophrenia from a Levels of Explanation Perspective. Schizophr Bull. (2018) 44:234–41. doi: 10.1093/schbul/sbx142
3. Allen P, Larøi F, McGuire P, Aleman A. The hallucinating brain: a review of structural and functional neuroimaging studies of hallucinations. Neurosci Biobehav Rev. (2008) 32:175–91. doi: 10.1016/j.neubiorev.2007.07.012
4. Jardri R, Pouchet A, Pins D, Thomas P. Cortical activations during auditory verbal hallucinations in schizophrenia: a coordinate-based meta-analysis. Am J Psychiatry. (2011) 168:73–81. doi: 10.1176/appi.ajp.2010.09101522
5. Sommer I, Diederen K, Blom J, Willems A, Kushan L, Slotema K, et al. Auditory verbal hallucinations predominantly activate the right inferior frontal area. Brain. (2008) 131:3169–77. doi: 10.1093/brain/awn251
6. Zmigrod L, Garrison J, Carr J, Simons J. The neural mechanisms of hallucinations: a quantitative meta-analysis of neuroimaging studies. Neurosci Biobehav Rev. (2016) 69:113–23. doi: 10.1016/j.neubiorev.2016.05.037
7. van de Ven V, Formisano E, Röder C, Prvulovic D, Bittner R, Dietz M, et al. The spatiotemporal pattern of auditory cortical responses during verbal hallucinations. Neuroimage. (2005) 27:644–55. doi: 10.1016/j.neuroimage.2005.04.041
8. Dierks T, Linden D, Jandl M, Formisano E, Goebel R, Lanfermann H, et al. Activation of Heschl’s gyrus during auditory hallucinations. Neuron. (1999) 22:615–21. doi: 10.1016/S0896-6273(00)80715-1
9. Modinos G, Costafreda S, van Tol M, McGuire P, Aleman A, Allen P. Neuroanatomy of auditory verbal hallucinations in schizophrenia: a quantitative meta-analysis of voxel-based morphometry studies. Cortex. (2013) 49:1046–55. doi: 10.1016/j.cortex.2012.01.009
10. Sun J, Maller J, Guo L, Fitzgerald P. Superior temporal gyrus volume change in schizophrenia: a review on region of interest volumetric studies. Brain Res Rev. (2009) 61:14–32. doi: 10.1016/j.brainresrev.2009.03.004
11. Barker A. An introduction to the basic principles of magnetic nerve stimulation. J Clin Neurophysiol. (1991) 8:26–37. doi: 10.1097/00004691-199101000-00005
12. Rossi S, Hallett M, Rossini P, Pascual-Leone A. Safety, ethical considerations, and application guidelines for the use of transcranial magnetic stimulation in clinical practice and research. Clin Neurophysiol. (2009) 120:2008–39. doi: 10.1016/j.clinph.2009.08.016
13. Chen R, Classen J, Gerloff C, Celnik P, Wassermann E, Hallett M, et al. Depression of motor cortex excitability by low-frequency transcranial magnetic stimulation. Neurology. (1997) 48:1398–403. doi: 10.1212/WNL.48.5.1398
14. Hoffman R, Boutros N, Berman R, Roessler E, Belger A, Krystal J, et al. Transcranial magnetic stimulation of left temporoparietal cortex in three patients reporting hallucinated “voices”. Biol Psychiatry. (1999) 46:130–2. doi: 10.1016/S0006-3223(98)00358-8
15. Hoffman R, Hawkins K, Gueorguieva R, Boutros N, Rachid F, Carroll K, et al. Transcranial magnetic stimulation of left temporoparietal cortex and medication-resistant auditory hallucinations. Arch Gen Psychiatry. (2003) 60:49–56. doi: 10.1001/archpsyc.60.1.49
16. Hoffman R, Gueorguieva R, Hawkins K, Varanko M, Boutros N, Wu Y. Temporoparietal transcranial magnetic stimulation for auditory hallucinations: safety, efficacy and moderators in a fifty patient sample. Biol Psychiatry. (2005) 58:97–104. doi: 10.1016/j.biopsych.2005.03.041
17. Poulet E, Brunelin J, Bediou B, Bation R, Forgeard L, Dalery J, et al. Slow transcranial magnetic stimulation can rapidly reduce resistant auditory hallucinations in schizophrenia. Biol Psychiatry. (2005) 57:188–91. doi: 10.1016/j.biopsych.2004.10.007
18. Montagne-Larmurier A, Etard O, Razafimandimby A, Morello R, Dollfus S. Two-day treatment of auditory hallucinations by high frequency rTMS guided by cerebral imaging: a 6 month follow-up pilot study. Schizophr Res. (2009) 113:77–83.
19. Slotema C, Blom J, van Lutterveld R, Hoek H, Sommer I. Review of the efficacy of transcranial magnetic stimulation for auditory verbal hallucinations. Biol Psychiatry. (2014) 76:101–10. doi: 10.1016/j.biopsych.2013.09.038
20. Tranulis C, Sepehry A, Galinowski A, Stip E. Should we treat auditory hallucinations with repetitive transcranial magnetic stimulation? A meta analysis. Can J Psychiatry. (2008) 53:577–86. doi: 10.1177/070674370805300904
21. Slotema C, Blom J, Hoek H, Sommer I, Sommer I. Should we expand the toolbox of psychiatric treatment methods to include repetitive transcranial magnetic stimulation (rTMS)? A meta-analysis of the efficacy of rTMS in psychiatric disorders. J Clin Psychiatry. (2010) 71:873–84. doi: 10.4088/jcp.08m04872gre
22. Aleman A, Sommer I, Sommer I, Kahn R. Efficacy of slow repetitive transcranial magnetic stimulation in the treatment of resistant auditory hallucinations in schizophrenia: a meta-analysis. J Clin Psychiatry. (2007) 68:416–21. doi: 10.4088/jcp.v68n0310
23. Slotema C, Blom J, de Weijer A, Diederen K, Goekoop R, Looijestijn J, et al. Can low-frequency repetitive transcranial magnetic stimulation really relieve medication-resistant auditory verbal hallucinations? Negative results from a large randomized controlled trial. Biol Psychiatry. (2011) 69:450–6. doi: 10.1016/j.biopsych.2010.09.051
24. Loo C, Sainsbury K, Mitchell P, Hadzi-Pavlovic D, Sachdev PS. A sham-controlled trial of left and right temporal rTMS for the treatment of auditory hallucinations. Psychol Med. (2010) 40:541–6. doi: 10.1017/S0033291709990900
25. Stephan K, Baldeweg T, Friston K. Synaptic plasticity and dysconnection in schizophrenia. Biol Psychiatry. (2006) 59:929–39. doi: 10.1016/j.biopsych.2005.10.005
26. Vercammen A, Knegtering H, den Boer J, Liemburg E, Aleman A. Auditory hallucinations in schizophrenia are associated with reduced functional connectivity of the temporo-parietal area. Biol Psychiatry. (2010) 67:912–8. doi: 10.1016/j.biopsych.2009.11.017
27. Chang X, Collin G, Xi Y, Cui L, Scholtens L, Sommer I, et al. Resting-state functional connectivity in medication-naïve schizophrenia patients with and without auditory verbal hallucinations: a preliminary report. Schizophr Res. (2017) 188:75–81. doi: 10.1016/j.schres.2017.01.024
28. Alderson-Day B, McCarthy-Jones S, Fernyhough C. Hearing voices in the resting brain: a review of intrinsic functional connectivity research on auditory verbal hallucinations. Neurosci Biobehav Rev. (2015) 55:78–87.
29. Wolf N, Sambataro F, Vasic N, Frasch K, Schmid M, Schönfeldt-Lecuona C, et al. Dysconnectivity of multiple resting-state networks in patients with schizophrenia who have persistent auditory verbal hallucinations. J Psychiatry Neurosci. (2011) 36:366–74. doi: 10.1503/jpn.110008
30. Fitzgerald P, Sritharan A, Benitez J, Daskalakis Z, Jackson G, Kulkarni J, et al. A preliminary fMRI study of the effects on cortical activation of the treatment of refractory auditory hallucinations with rTMS. Psychiatry Res. (2007) 155:83–8. doi: 10.1016/j.pscychresns.2006.12.011
31. Horacek J, Brunovsky M, Novak T, Skrdlantova L, Klirova M, Bubenikova-Valesova V, et al. Effect of low-frequency rTMS on electromagnetic tomography (LORETA) and regional brain metabolism (PET) in schizophrenia patients with auditory hallucinations. Neuropsychobiology. (2007) 55:132–42. doi: 10.1159/000106055
32. Vercammen A, Knegtering H, Liemburg E, den Boer J, Aleman A. Functional connectivity of the temporo-parietal region in schizophrenia: effects of rTMS treatment of auditory hallucinations. J Psychiatr Res. (2010) 44:725–31. doi: 10.1016/j.jpsychires.2009.12.011
33. Xie Y, He Y, Guan M, Wang Z, Zhou G, Ma Z, et al. Low-frequency rTMS treatment alters the topographical organization of functional brain networks in schizophrenia patients with auditory verbal hallucination. Psychiatry Res. (2022) 309:114393. doi: 10.1016/j.psychres.2022.114393
34. Kay S, Fiszbein A, Opler L. The positive and negative syndrome scale (PANSS) for schizophrenia. Schizophr Bull. (1987) 13:261–76. doi: 10.1093/schbul/13.2.261
35. Xie Y, Guan M, Wang Z, Mazhujing Z, Wang H, Fang P, et al. rTMS induces brain functional and structural alternations in schizophrenia patient with auditory verbal hallucination. Front Neurosci. (2021) 15:722894. doi: 10.3389/fnins.2021.722894
36. Xie Y, He Y, Guan M, Zhou G, Wang Z, Ma Z, et al. Impact of low-frequency rTMS on functional connectivity of the dentate nucleus subdomains in schizophrenia patients with auditory verbal hallucination. J Psychiatr Res. (2022) 149:87–96. doi: 10.1016/j.jpsychires.2022.02.030
37. Xie Y, Cai Y, Guan M, Wang Z, Ma Z, Fang P, et al. The alternations of nucleus accumbent in schizophrenia patients with auditory verbal hallucinations during low-frequency rTMS treatment. Front Psychiatry. (2022) 13:971105. doi: 10.3389/fpsyt.2022.971105
38. Song X, Dong Z, Long X, Li S, Zuo X, Zhu C, et al. Rest: a toolkit for resting-state functional magnetic resonance imaging data processing. PLoS One. (2011) 6:e25031. doi: 10.1371/journal.pone.0025031
39. Liao W, Wu G, Xu Q, Ji G, Zhang Z, Zang Y, et al. Dynamicbc: a matlab toolbox for dynamic brain connectome analysis. Brain Connect. (2014) 4:780–90. doi: 10.1089/brain.2014.0253
40. Zhou B, Chen Y, Zheng R, Jiang Y, Li S, Wei Y, et al. Alterations of static and dynamic functional connectivity of the nucleus accumbens in patients with major depressive disorder. Front Psychiatry. (2022) 13:877417. doi: 10.3389/fpsyt.2022.877417
41. Yao Y, He H, Duan M, Li S, Li C, Chen X, et al. The effects of music intervention on pallidum-DMN circuit of schizophrenia. Biomed Res Int. (2020) 2020:4107065. doi: 10.1155/2020/4107065
42. Allen E, Damaraju E, Plis S, Erhardt E, Eichele T, Calhoun V. Tracking whole-brain connectivity dynamics in the resting state. Cereb Cortex. (2014) 24:663–76. doi: 10.1093/cercor/bhs352
43. Hutchison R, Womelsdorf T, Allen E, Bandettini P, Calhoun V, Corbetta M, et al. Dynamic functional connectivity: promise, issues, and interpretations. Neuroimage. (2013) 80:360–78. doi: 10.1016/j.neuroimage.2013.05.079
44. Han X, Wu X, Wang Y, Sun Y, Ding W, Cao M, et al. Alterations of resting-state static and dynamic functional connectivity of the dorsolateral prefrontal cortex in subjects with internet gaming disorder. Front Hum Neurosci. (2018) 12:41. doi: 10.3389/fnhum.2018.00041
45. Woods S. Chlorpromazine equivalent doses for the newer atypical antipsychotics. J Clin Psychiatry. (2003) 64:663–7. doi: 10.4088/jcp.v64n0607
46. Slotema C, Aleman A, Daskalakis Z, Sommer I. Meta-analysis of repetitive transcranial magnetic stimulation in the treatment of auditory verbal hallucinations: update and effects after one month. Schizophr Res. (2012) 142:40–5. doi: 10.1016/j.schres.2012.08.025
47. Upthegrove R, Broome M, Caldwell K, Ives J, Oyebode F, Wood S. Understanding auditory verbal hallucinations: a systematic review of current evidence. Acta Psychiatr Scand. (2016) 133:352–67. doi: 10.1111/acps.12531
48. Waters F, Allen P, Aleman A, Fernyhough C, Woodward T, Badcock J, et al. Auditory hallucinations in schizophrenia and nonschizophrenia populations: a review and integrated model of cognitive mechanisms. Schizophr Bull. (2012) 38:683–93. doi: 10.1093/schbul/sbs045
49. Kühn S, Gallinat J. Quantitative meta-analysis on state and trait aspects of auditory verbal hallucinations in schizophrenia. Schizophr Bull. (2012) 38:779–86. doi: 10.1093/schbul/sbq152
50. Sommer I, Aleman A, Ramsey N, Bouma A, Kahn R. Handedness, language lateralisation and anatomical asymmetry in schizophrenia: meta-analysis. Br J Psychiatry. (2001) 178:344–51. doi: 10.1192/bjp.178.4.344
51. Chance S, Casanova M, Switala A, Crow T. Auditory cortex asymmetry, altered minicolumn spacing and absence of ageing effects in schizophrenia. Brain. (2008) 131:3178–92. doi: 10.1093/brain/awn211
52. Bleich-Cohen M, Hendler T, Kotler M, Strous R. Reduced language lateralization in first-episode schizophrenia: an fMRI index of functional asymmetry. Psychiatry Res. (2009) 171:82–93. doi: 10.1016/j.pscychresns.2008.03.002
53. Kircher T, Liddle P, Brammer M, Williams S, Murray R, McGuire P. Reversed lateralization of temporal activation during speech production in thought disordered patients with schizophrenia. Psychol Med. (2002) 32:439–49. doi: 10.1017/S0033291702005287
54. Weiss E, Hofer A, Golaszewski S, Siedentopf C, Felber S, Fleischhacker W. Language lateralization in unmedicated patients during an acute episode of schizophrenia: a functional MRI study. Psychiatry Res. (2006) 146:185–90. doi: 10.1016/j.pscychresns.2005.11.003
55. Mitchell R, Crow T. Right hemisphere language functions and schizophrenia: the forgotten hemisphere?. Brain. (2005) 128:963–78. doi: 10.1093/brain/awh466
56. Bentaleb L, Beauregard M, Liddle P, Stip E. Cerebral activity associated with auditory verbal hallucinations: a functional magnetic resonance imaging case study. J Psychiatry Neurosci. (2002) 27:110–5.
57. Stephan K, Friston K, Frith C. Dysconnection in schizophrenia: from abnormal synaptic plasticity to failures of self-monitoring. Schizophr Bull. (2009) 35:509–27. doi: 10.1093/schbul/sbn176
58. Zhou Y, Liang M, Tian L, Wang K, Hao Y, Liu H, et al. Functional disintegration in paranoid schizophrenia using resting-state fMRI. Schizophr Res. (2007) 97:194–205. doi: 10.1016/j.schres.2007.05.029
59. Barrett J, Della-Maggiore V, Chouinard P, Paus T. Mechanisms of action underlying the effect of repetitive transcranial magnetic stimulation on mood: behavioral and brain imaging studies. Neuropsychopharmacology. (2004) 29:1172–89. doi: 10.1038/sj.npp.1300411
60. Paus T, Jech R, Thompson C, Comeau R, Peters T, Evans A. Transcranial magnetic stimulation during positron emission tomography: a new method for studying connectivity of the human cerebral cortex. J Neurosci. (1997) 17:3178–84. doi: 10.1523/JNEUROSCI.17-09-03178.1997
61. Wang H, Wang X, Scheich H. LTD and LTP induced by transcranial magnetic stimulation in auditory cortex. Neuroreport. (1996) 7:521–5. doi: 10.1097/00001756-199601310-00035
62. Kindler J, Homan P, Jann K, Federspiel A, Flury R, Hauf M, et al. Reduced neuronal activity in language-related regions after transcranial magnetic stimulation therapy for auditory verbal hallucinations. Biol Psychiatry. (2013) 73:518–24.
63. Fitzgerald P, Fountain S, Daskalakis ZJA. Comprehensive review of the effects of rTMS on motor cortical excitability and inhibition. Clin Neurophysiol. (2006) 117:2584–96. doi: 10.1016/j.clinph.2006.06.712
64. Di Lazzaro V, Oliviero A, Mazzone P, Pilato F, Saturno E, Dileone M, et al. Short-term reduction of intracortical inhibition in the human motor cortex induced by repetitive transcranial magnetic stimulation. Exp Brain Res. (2002) 147:108–13. doi: 10.1007/s00221-002-1223-5
65. Bais L, Liemburg E, Vercammen A, Bruggeman R, Knegtering H, Aleman A. Effects of low frequency rTMS treatment on brain networks for inner speech in patients with schizophrenia and auditory verbal hallucinations. Prog Neuropsychopharmacol Biol Psychiatry. (2017) 78:105–13. doi: 10.1016/j.pnpbp.2017.04.017
66. Chiarello C, Maxfield L. Varieties of interhemispheric inhibition, or how to keep a good hemisphere down. Brain Cogn. (1996) 30:81–108. doi: 10.1006/brcg.1996.0006
67. Hartwigsen G, Saur D, Price C, Ulmer S, Baumgaertner A, Siebner H. Perturbation of the left inferior frontal gyrus triggers adaptive plasticity in the right homologous area during speech production. Proc Natl Acad Sci USA. (2013) 110:16402–7. doi: 10.1073/pnas.1310190110
68. Corbetta M, Shulman G. Control of goal-directed and stimulus-driven attention in the brain. Nat Rev Neurosci. (2002) 3:201–15. doi: 10.1038/nrn755
69. Hu S, Ide J, Zhang S, Li C. The right superior frontal gyrus and individual variation in proactive control of impulsive response. J Neurosci. (2016) 36:12688–96. doi: 10.1523/JNEUROSCI.1175-16.2016
70. Li W, Qin W, Liu H, Fan L, Wang J, Jiang T, et al. Subregions of the human superior frontal gyrus and their connections. Neuroimage. (2013) 78:46–58. doi: 10.1016/j.neuroimage.2013.04.011
71. Tully L, Lincoln S, Liyanage-Don N, Hooker C. Impaired cognitive control mediates the relationship between cortical thickness of the superior frontal gyrus and role functioning in schizophrenia. Schizophr Res. (2014) 152:358–64. doi: 10.1016/j.schres.2013.12.005
72. Yasuda Y, Okada N, Nemoto K, Fukunaga M, Yamamori H, Ohi K, et al. Brain Morphological and functional features in cognitive subgroups of schizophrenia. Psychiatry Clin Neurosci. (2020) 74:191–203. doi: 10.1111/pcn.12963
73. Salgado-Pineda P, Caclin A, Baeza I, Junqué C, Bernardo M, Blin O, et al. Schizophrenia and frontal cortex: where does it fail?. Schizophr Res. (2007) 91:73–81. doi: 10.1016/j.schres.2006.12.028
74. Wu X, Zeng L, Shen H, Yuan L, Qin J, Zhang P. Functional network connectivity alterations in schizophrenia and depression. Psychiatry Res. (2017) 263:113–20. doi: 10.1016/j.pscychresns.2017.03.012
75. Shibasaki H, Sadato N, Lyshkow H, Yonekura Y, Honda M, Nagamine T, et al. Both primary motor cortex and supplementary motor area play an important role in complex finger movement. Brain. (1993) 116:1387–98. doi: 10.1093/brain/116.6.1387
76. Tanji J. The supplementary motor area in the cerebral cortex. Neurosci Res. (1994) 19:251–68. doi: 10.1016/0168-0102(94)90038-8
77. Rogowska J, Gruber S, Yurgelun-Todd D. Functional magnetic resonance imaging in schizophrenia: cortical response to motor stimulation. Psychiatry Res. (2004) 130:227–43. doi: 10.1016/j.pscychresns.2003.12.004
78. Schröder J, Wenz F, Schad L, Baudendistel K, Knopp M. Sensorimotor cortex and supplementary motor area changes in schizophrenia: a study with functional magnetic resonance imaging. Br J Psychiatry. (1995) 167:197–201. doi: 10.1192/bjp.167.2.197
79. Walther S, Strik W. Motor symptoms and schizophrenia. Neuropsychobiology. (2012) 66:77–92. doi: 10.1159/000339456
80. Whitty P, Owoeye O, Waddington J. Neurological signs and involuntary movements in schizophrenia: intrinsic to and informative on systems pathobiology. Schizophr Bull. (2009) 35:415–24. doi: 10.1093/schbul/sbn126
81. Pappa S, Dazzan P. Spontaneous movement disorders in antipsychotic-naive patients with first-episode psychoses: a systematic review. Psychol Med. (2009) 39:1065–76. doi: 10.1017/S0033291708004716
82. Starr C, Sawaki L, Wittenberg G, Burdette J, Oshiro Y, Quevedo A, et al. The contribution of the putamen to sensory aspects of pain: insights from structural connectivity and brain lesions. Brain. (2011) 134:1987–2004. doi: 10.1093/brain/awr117
83. Haber S. Corticostriatal circuitry. Dialogues Clin Neurosci. (2016) 18:7–21. doi: 10.31887/DCNS.2016.18.1/shaber
84. Chen C, Wang H, Wu S, Huang H, Zou J, Chen J, et al. Abnormal degree centrality of bilateral putamen and left superior frontal gyrus in schizophrenia with auditory hallucinations: a resting-state functional magnetic resonance imaging study. Chin Med J. (2015) 128:3178–84. doi: 10.4103/0366-6999.170269
85. Diederen K, Neggers S, de Weijer A, van Lutterveld R, Daalman K, Eickhoff S, et al. Aberrant resting-state connectivity in non-psychotic individuals with auditory hallucinations. Psychol Med. (2013) 43:1685–96. doi: 10.1017/S0033291712002541
86. Toda M, Abi-Dargham A. Dopamine hypothesis of schizophrenia: making sense of it all. Curr Psychiatry Rep. (2007) 9:329–36. doi: 10.1007/s11920-007-0041-7
87. McGuire P, Silbersweig D, Murray R, David A, Frackowiak R, Frith C. Functional anatomy of inner speech and auditory verbal imagery. Psychol Med. (1996) 26:29–38. doi: 10.1017/S0033291700033699
88. McGuire P, David A, Murray R, Frackowiak R, Frith C, Wright I, et al. Abnormal monitoring of inner speech: a physiological basis for auditory hallucinations. Lancet. (1995) 346:596–600. doi: 10.1016/S0140-6736(95)91435-8
89. Onitsuka T, Shenton M, Salisbury D, Dickey C, Kasai K, Toner S, et al. Middle and inferior temporal gyrus gray matter volume abnormalities in chronic schizophrenia: an MRI study. Am J Psychiatry. (2004) 161:1603–11. doi: 10.1176/appi.ajp.161.9.1603
90. Zhuo C, Zhu J, Wang C, Wang L, Li J, Qin W, et al. Increased local spontaneous neural activity in the left precuneus specific to auditory verbal hallucinations of schizophrenia. Chin Med J. (2016) 129:809–13. doi: 10.4103/0366-6999.178974
91. Damaraju E, Allen E, Belger A, Ford J, McEwen S, Mathalon D, et al. Dynamic functional connectivity analysis reveals transient states of dysconnectivity in schizophrenia. Neuroimage Clin. (2014) 5:298–308. doi: 10.1016/j.nicl.2014.07.003
92. Rabany L, Brocke S, Calhoun V, Pittman B, Corbera S, Wexler B, et al. Dynamic functional connectivity in schizophrenia and autism spectrum disorder: convergence, divergence and classification. Neuroimage Clin. (2019) 24:101966. doi: 10.1016/j.nicl.2019.101966
93. Dollfus S, Razafimandimby A, Delamillieure P, Brazo P, Joliot M, Mazoyer B, et al. Atypical hemispheric specialization for language in right-handed schizophrenia patients. Biol Psychiatry. (2005) 57:1020–8. doi: 10.1016/j.biopsych.2005.01.009
94. Jeong B, Wible C, Hashimoto R, Kubicki M. Functional and anatomical connectivity abnormalities in left inferior frontal gyrus in schizophrenia. Hum Brain Mapp. (2009) 30:4138–51. doi: 10.1002/hbm.20835
95. Ruby P, Decety J. Effect of subjective perspective taking during simulation of action: a pet investigation of agency. Nat Neurosci. (2001) 4:546–50. doi: 10.1038/87510
96. Venkatasubramanian G, Jayakumar P, Keshavan M, Gangadhar B. Schneiderian first rank symptoms and inferior parietal lobule cortical thickness in antipsychotic-naïve schizophrenia. Prog Neuropsychopharmacol Biol Psychiatry. (2011) 35:40–6. doi: 10.1016/j.pnpbp.2010.07.023
97. Cleghorn J, Garnett E, Nahmias C, Firnau G, Brown G, Kaplan R, et al. Increased frontal and reduced parietal glucose metabolism in acute untreated schizophrenia. Psychiatry Res. (1989) 28:119–33. doi: 10.1016/0165-1781(89)90040-1
98. Smiley J, Konnova K, Bleiwas C. Cortical thickness, neuron density and size in the inferior parietal lobe in schizophrenia. Schizophr Res. (2012) 136:43–50.
99. Torrey E. Schizophrenia and the inferior parietal lobule. Schizophr Res. (2007) 97:215–25. doi: 10.1016/j.schres.2007.08.023
100. Cheng C, Hsiao F, Hsieh Y, Wang P. Dysfunction of inferior parietal lobule during sensory gating in patients with amnestic mild cognitive impairment. Front Aging Neurosci. (2020) 12:39. doi: 10.3389/fnagi.2020.00039
101. Li S, Hu N, Zhang W, Tao B, Dai J, Gong Y, et al. Dysconnectivity of multiple brain networks in schizophrenia: a meta-analysis of resting-state functional connectivity. Front Psychiatry. (2019) 10:482. doi: 10.3389/fpsyt.2019.00482
102. Dong D, Wang Y, Chang X, Luo C, Yao D. Dysfunction of large-scale brain networks in schizophrenia: a meta-analysis of resting-state functional connectivity. Schizophr Bull. (2018) 44:168–81. doi: 10.1093/schbul/sbx034
103. Zhang L, Li B, Wang H, Li L, Liao Q, Liu Y, et al. Decreased middle temporal gyrus connectivity in the language network in schizophrenia patients with auditory verbal hallucinations. Neurosci Lett. (2017) 653:177–82. doi: 10.1016/j.neulet.2017.05.042
104. Zhao Z, Li X, Feng G, Shen Z, Li S, Xu Y, et al. Altered effective connectivity in the default network of the brains of first-episode, drug-naive schizophrenia patients with auditory verbal hallucinations. Front Hum Neurosci. (2018) 12:456. doi: 10.3389/fnhum.2018.00456
105. Fornito A, Yücel M, Dean B, Wood S, Pantelis C. Anatomical abnormalities of the anterior cingulate cortex in schizophrenia: bridging the gap between neuroimaging and neuropathology. Schizophr Bull. (2009) 35:973–93. doi: 10.1093/schbul/sbn025
106. Fornito A, Yücel M, Wood S, Adamson C, Velakoulis D, Saling M, et al. Surface-based morphometry of the anterior cingulate cortex in first episode schizophrenia. Hum Brain Mapp. (2008) 29:478–89. doi: 10.1002/hbm.20412
107. Xu Y, Zhuo C, Qin W, Zhu J, Yu C. Altered spontaneous brain activity in schizophrenia: a meta-analysis and a large-sample study. Biomed Res Int. (2015) 2015:204628. doi: 10.1155/2015/204628
108. Barber L, Reniers R, Upthegrove R. A review of functional and structural neuroimaging studies to investigate the inner speech model of auditory verbal hallucinations in schizophrenia. Transl Psychiatry. (2021) 11:582. doi: 10.1038/s41398-021-01670-7
109. Zhang Z, Shi J, Yuan Y, Hao G, Yao Z, Chen N. Relationship of auditory verbal hallucinations with cerebral asymmetry in patients with schizophrenia: an event-related fMRI study. J Psychiatr Res. (2008) 42:477–86. doi: 10.1016/j.jpsychires.2007.04.003
110. Stephane M, Hagen M, Lee J, Uecker J, Pardo P, Kuskowski M, et al. About the mechanisms of auditory verbal hallucinations: a positron emission tomographic study. J Psychiatry Neurosci. (2006) 31:396–405.
111. Salgado-Pineda P, Junqué C, Vendrell P, Baeza I, Bargalló N, Falcón C, et al. Decreased cerebral activation during CPT performance: structural and functional deficits in schizophrenic patients. Neuroimage. (2004) 21:840–7. doi: 10.1016/j.neuroimage.2003.10.027
112. Kumari V, Aasen I, Taylor P, Ffytche D, Das M, Barkataki I. Neural dysfunction and violence in schizophrenia: an fMRI investigation. Schizophr Res. (2006) 84:144–64. doi: 10.1016/j.schres.2006.02.017
113. Nierenberg J, Salisbury D, Levitt J, David E, McCarley R, Shenton M. Reduced left angular gyrus volume in first-episode schizophrenia. Am J Psychiatry. (2005) 162:1539–41. doi: 10.1176/appi.ajp.162.8.1539
114. Fusar-Poli P, Borgwardt S, Crescini A, Deste G, Kempton M, Lawrie S, et al. Neuroanatomy of vulnerability to psychosis: a voxel-based meta-analysis. Neurosci Biobehav Rev. (2011) 35:1175–85. doi: 10.1016/j.neubiorev.2010.12.005
115. Nenadic I, Smesny S, Schlösser R, Sauer H, Gaser C. Auditory hallucinations and brain structure in schizophrenia: voxel-based morphometric study. Br J Psychiatry. (2010) 196:412–3. doi: 10.1192/bjp.bp.109.070441
Keywords: auditory verbal hallucinations, schizophrenia, fMRI, functional connectivity, rTMS, left temporoparietal junction region
Citation: Xie Y, Guan M, He Y, Wang Z, Ma Z, Fang P and Wang H (2023) The Static and dynamic functional connectivity characteristics of the left temporoparietal junction region in schizophrenia patients with auditory verbal hallucinations during low-frequency rTMS treatment. Front. Psychiatry 14:1071769. doi: 10.3389/fpsyt.2023.1071769
Received: 16 October 2022; Accepted: 09 January 2023;
Published: 25 January 2023.
Edited by:
Di Zhao, Shanghai Jiao Tong University, ChinaReviewed by:
Zhaowen Liu, Massachusetts General Hospital and Harvard Medical School, United StatesYue Ding, Shanghai Mental Health Center, China
Copyright © 2023 Xie, Guan, He, Wang, Ma, Fang and Wang. This is an open-access article distributed under the terms of the Creative Commons Attribution License (CC BY). The use, distribution or reproduction in other forums is permitted, provided the original author(s) and the copyright owner(s) are credited and that the original publication in this journal is cited, in accordance with accepted academic practice. No use, distribution or reproduction is permitted which does not comply with these terms.
*Correspondence: Yuanjun Xie, eGlleXVhbmpAZ21haWwuY29t; Peng Fang,
ZmFuZ3BlbmdAZm1tdS5lZHUuY24=; Huaning Wang,
MTM2MDkxNjEzNDFAMTYzLmNvbQ==