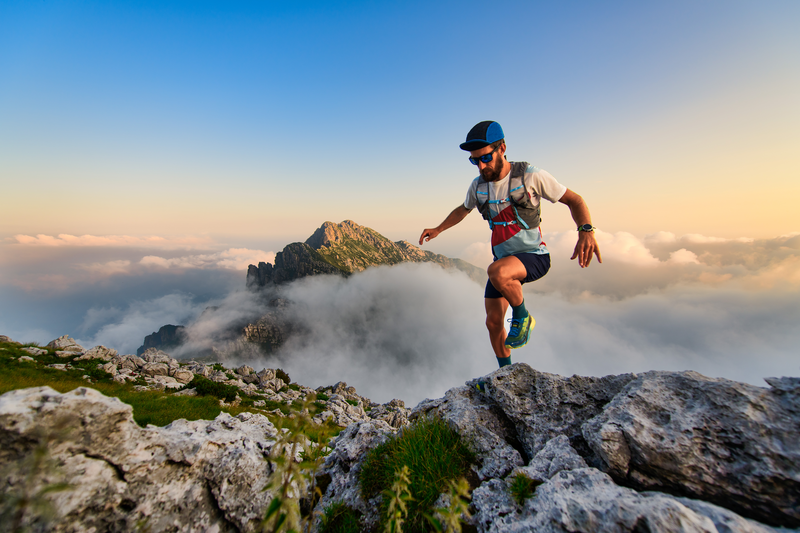
94% of researchers rate our articles as excellent or good
Learn more about the work of our research integrity team to safeguard the quality of each article we publish.
Find out more
REVIEW article
Front. Psychiatry , 24 November 2022
Sec. Neuroimaging
Volume 13 - 2022 | https://doi.org/10.3389/fpsyt.2022.994372
This article is part of the Research Topic Rising Stars in Neuroimaging and Stimulation 2022 View all 5 articles
Schizophrenia and related psychoses are complex neuropsychiatric diseases representing dysconnectivity across multiple scales, through the micro (cellular), meso (brain network), manifest (behavioral), and social (interpersonal) levels. In vivo human neuroimaging, particularly at ultra-high field (UHF), offers unprecedented opportunity to examine multiscale dysconnectivity in psychosis. In this review, we provide an overview of the literature to date on UHF in psychosis, focusing on microscale findings from magnetic resonance spectroscopy (MRS), mesoscale studies on structural and functional magnetic resonance imaging (fMRI), and multiscale studies assessing multiple neuroimaging modalities and relating UHF findings to behavior. We highlight key insights and considerations from multiscale and longitudinal studies and provide recommendations for future research on UHF neuroimaging in psychosis.
Schizophrenia, coined term for “split mind,” is a complex neuropsychiatric illness characterized by a range of positive, negative, and cognitive symptoms (1). Positive symptoms include hallucinations (false perceptions) and delusions (false beliefs) that are often characteristic of psychotic disorders. Negative symptoms refer to a decrease in typical behaviors, such as reduced motivation, pleasure, speech and expressed affect. Finally, cognitive symptoms manifest as impaired performance across a range of neuropsychological domains, including language, memory, and attention. These symptoms are evident in psychosis, an umbrella term that groups individuals diagnosed with schizophrenia and related psychotic disorders (e.g., schizoaffective disorder, schizophreniform disorder, bipolar disorder with psychotic features, etc.).
Psychotic disorders can be understood as disorders of connectivity across multiple scales, ranging from micro (cellular level) to meso (brain network level) to manifest (behavioral level) to social (interpersonal level) scales (Figure 1). At the microscale, psychosis is associated with dysfunctional glutamatergic and dopaminergic transmission across synapses as well as altered astrocyte signaling (2, 3). At the meso-scale, psychosis is characterized by both structural and functional dysconnectivity of brain networks (4–6). Behaviorally, individuals with psychosis manifest a wide range of symptoms that point toward dysfunction in relational processing (e.g., relational memory, social cognition, linguistic processing), affecting interpersonal relationships at the social scale (7–9). Thus, psychosis does not arise from abnormality in any single point source but likely results from a sum of aberrant interactions between networks of features within and across scales.
Figure 1. Micro-scale dysconnectivity observed at synapses and circuits; meso-scale dysconnectivity across brain networks; manifest-scale dysconnectivity between how words are put together, actions are put together, and memories are put together; social-scale carries dysconnectivity at various interpersonal relations hips.
Beyond identifying dysconnectivity at multiple scales, a complete picture of psychosis is only possible through understanding the way in which these scales interact and influence one another. For example, micro-level dysfunction (e.g., aberrant concentrations of neural metabolites and neurotransmission), may present itself in meso-level dysfunction (e.g., brain dysconnectivity), which manifests as abnormal speech and behavioral patterns that affect the interpersonal relationships of individuals living with schizophrenia (10). Multiscale studies incorporating measures from the micro to social scale are, therefore, of critical importance to connect social and behavioral findings with cellular and network level disruptions in psychosis. In vivo human neuroimaging can provide insight into multiscale dysconnectivity at the microscale (e.g., neurotransmitter dysfunction through magnetic resonance spectroscopy; MRS; and positron emission tomography; PET) and mesoscale (e.g., structural and functional dysconnectivity through magnetic resonance imaging; MRI), while simultaneously allowing for acquisition of data at the manifest (e.g., behavioral, clinical, cognitive) and social (e.g., interpersonal) scales.
Ultra-high field (UHF) neuroimaging (e.g., 7T MRI/MRS) refers to magnetic resonance imaging using a magnetic field strength (b0) of greater than or equal to 7.0 Tesla. UHF offers many benefits relative to lower fields strengths (e.g., 3T) that can facilitate multiscale dysconnectivity research in psychiatric populations (11, 12). Quantitative improvements of UHF include greater signal-to-noise (SNR) and contrast-to-noise ratios and higher spatial resolution. This leads to qualitative advantages over lower field strengths, such as the ability to visualize smaller structures and layer-level structural and functional dysconnectivity. UHF-MRS also presents qualitative and quantitative improvements over standard MRS, as we discuss below, such as better differentiation and identification of previously undetectable metabolites at the microscale. Increased sensitivity and resolution translate to greater power to detect effects in smaller samples, as well as at the individual level, giving impetus to precision psychiatry (13, 14). In this review, we provide an overview of the literature to date on UHF in psychosis, highlighting key insights and challenges of this work, and provide recommendations for future research. We emphasize multiscale UHF studies in psychosis throughout, particularly those studies incorporating measures across the micro, meso, manifest, and social scales.
A specific advantage offered by UHF-MRS systems is the ability to resolve the neurometabolic spectrum along with high SNR. The SNR gain from 3T to 7T is especially pronounced for glutamate, glutamine, and gamma aminobutyric acid (GABA). Glutamine and glutamate are molecules in a dynamic equilibrium, reflecting the integrity of distinct cellular compartments (neurons and astrocytes) in the brain, but separating their spectra is challenging at lower field strengths. The reduced error rates in quantifying these molecules at UHF translates to a reduced need for large sample sizes to observe a given effect. This is especially helpful in the study of schizophrenia where neurobiological heterogeneity is a prime suspect for poor therapeutic progress.
UHF proton MRS (1HMRS) has overcome several methodological limitations evident at lower field strengths, such as separating highly coupled spins, improving SNR of metabolites with low in vivo concentrations, increased spectral dispersion, lower uncertainty in spectral quantification such as smaller Cramer lower bounds (CRLB) and coefficients of variance (CV). For example, glutathione is typically isolated as a secondary metabolite to GABA at lower field strengths using a single shot with spectral editing which can lead to inconsistent results (15), whereas at 7T, glutathione can be more reliably detected and separated from other metabolites. Specialized pulse sequences, a series of gradients and radiofrequency (RF) pulses, required to isolate highly coupled metabolites at lower field strengths are not necessary with UHF-1HMRS as it is better able to resolve structurally similar metabolites such as glutamate and glutamine. Furthermore, UHF-1HMRS resolves the glutamate signals that contaminate GABA and glutathione peaks at clinically feasible scan times for many brain regions, producing improved spectral outputs for GABA and glutathione when assessing the pathology of psychiatric illnesses (16).
A major working hypothesis regarding synapse-level dysconnectivity in psychosis is centered around three neurotransmitters: dopamine, glutamate and GABA. Of these, the relatively higher concentration and wider distribution of glutamate and GABA compared to dopamine (17) makes them suitable candidates for quantification using 1HMRS. Currently there are 17 published articles reporting metabolites in patients who are at a pre-psychotic (clinical high risk) stage, experiencing first episode psychosis (FEP), or with established schizophrenia using UHF-1HMRS (Table 1).
Table 1. Ultra-high field magnetic resonance spectroscopy/magnetic resonance spectroscopic imaging findings.
Most of these studies report on GABA and glutamate. Wijtenburg et al. (18) assessed glutamine, glutamate, GABA, and lactate differences among patients with schizophrenia, first-degree relatives, and healthy controls in five brain regions and reported glutamate differences in the anterior cingulate cortex (ACC); GABA, glutamine, and glutamate differences in the centrum semiovale; and no metabolite differences in the dorsolateral prefrontal cortex, hippocampus, or thalamus. Thakkar et al. (19) similarly studied patients, relatives and unrelated healthy controls, reporting a reduction in GABA and glutamate among patients. A reduction in GABA was also reported by Wang et al. (20) across several brain regions (ACC, orbitofrontal cortex, and thalamus) and by Marsman et al. (21) in the prefrontal cortex. This reduction in a network of metabolites is significantly correlated with severity of cognitive deficits among patients living with first episode schizophrenia. Reid et al. (22) found a correlation between GABA levels and cognitive function among patients with schizophrenia. GABA reduction may precede symptom onset, becoming apparent in the thalamus even at the clinical high-risk state (23).
While the ACC has been the most preferred site of voxel placement for 7T MRS studies in psychosis to date (24), a more recent study using a lipid suppression coil focused on deeper gray matter structures (2D magnetic resonance spectroscopic imaging, MRSI), reporting a generalized glutamate reduction in medicated patients that related to their psychomotor speed (25). Using a more spatially resolved 2D approach for glutamate quantification based on chemical exchange saturation (GluCEST), Roalf et al. (26) noted an overall decline in glutamate levels in patients with early stage psychosis. The advantage of GluCEST is the ability to quantify regional brain glutamate distribution across multiple cross-sectional whole brain slices in a reasonably short time period (27), thus promising more extensive studies of glutamate physiology in the near future.
Besides glutamate, glutamine and GABA, several studies have investigated glutathione—a critical marker of healthy neuro-glial interaction (20, 28–31) (see Table 1). In general, there is a higher degree of variability in cortical glutathione concentration among patients (32), with a subset showing higher glutathione levels and distinct patterns of clinical symptoms during first episode of psychosis (33), with better outcomes over time (28), especially in relation to their functional trajectory (34). In contrast, those with pronounced reduction in glutathione may have higher residual symptom burden (35). Limongi et al. (36) proposed that higher glutathione levels could reverse the glutamate-mediated dysconnectivity based on resting-state functional MRI-based causal modeling in combination with 7T MRS. In line with this, youth who are at a high risk for psychosis have higher glutathione in association with superior social functioning (37, 38).
UHF-1HMRS has greatly facilitated the detection of multiple metabolites in a single spectrum and the study of multiscale associations between metabolites and clinical measures. While many studies agree with prior findings at 3T; making direct comparisons across different studies is challenging due to differences in pulse sequences used to isolate and suppress signal, different quantification, fitting, tissue correction, water suppression techniques, and different preference of reference metabolites. Although these differences are small within a single study, there is a growing area of 1HMRS development that accounts for these differences (39). This could aid in the process to develop a uniform protocol to assess the quality of reported metrics for future studies to enable targeted treatment based on potential biomarkers.
Although macromolecule contamination does not appear to vary among patients and healthy controls, it may still affect the estimations of glutamate, GABA, and glutamine which could affect the interpretation of the pathophysiology of metabolites. The MRS pulse sequence and field strength used to detect and isolate metabolite(s) of interest could help eliminate and/or better estimate the effects of macromolecule contaminations. At lower field strengths, especially with the use of spectral editing, studies report GABA as GABA+ to indicate the macromolecule contamination. Using higher field strengths, high order shimming and local power optimization is crucial to minimize field-dependent inhomogeneities and to ensure accurate RF pulse frequency profiles. Inaccurate RF pulse profiles are common among pulses with larger flip angles such as 180° and to achieve the exact 180° flip can be difficult resulting in inaccurate isolation of metabolites. Furthermore, with increasing field strengths, transverse (T2) relaxation times and J-modulation effects decrease at a faster rate relative to lower field strengths. Hence, the quality of results is dependent on MRS pulse sequence use and effective echo times to ensure better detection. In the case of using a long echo time to ensure detection of GABA, 7T poses higher specific absorption rate, also known as tissue heating, when using a continuous pulse along with signal decay with shorter T2 relaxations. This poses a problem when detecting internal references such as creatine and water. Sequences have become robust to target signal loss via shorter echo times, however, the quality of data using CRLB or CV have reported higher than the accepted 20% threshold.
To date, MRS has been the most studied modality with UHF neuroimaging in psychosis, and many of these studies have investigated multiscale associations at two or more levels, from the microscale to the social scale. Though important differences are detectable at smaller sample sizes, future studies with larger samples can ensure representativeness and allow for investigation of potential mediating/moderating effects of sociodemographic (e.g., age, sex, gender) and clinical (e.g., psychosis stage, comorbidities) variables. Conducting longitudinal research across multiple scales will be essential to understand the therapeutic utility of MRS markers in the long term.
UHF also confers both quantitative and qualitative advantages to lower field strengths in terms of brain structure. One of the most promising applications of ultra-high field structural magnetic resonance imaging (UHF-sMRI) is the potential to non-invasively image the laminar structure of the brain in vivo, which is not possible at lower field strengths. In addition, UHF-sMRI can also more clearly visualize smaller structures and subregions. A prime example relevant to psychiatric applications is the hippocampus, its subfields, and adjacent white matter output regions, which are strongly implicated in schizophrenia (40, 41). Quantitatively, UHF-sMRI can also provide a better delineation of the gray-white matter boundary, which improves tissue segmentation and accuracy of cortical metrics, such as cortical thickness and surface area. Finally, higher image resolution can mitigate partial volume effects due to smaller voxel sizes. Together, these advantages improve precision and sensitivity of structural MRI at UHF, allowing for more detailed mesoscale and multiscale investigations.
To date, 11 studies have assessed brain structure using UHF-sMRI in schizophrenia-spectrum disorders, including schizophrenia, schizoaffective disorder, first-episode psychosis, and childhood-onset schizophrenia (Table 2). Iwabuchi et al. (42) compared machine learning classification of gray matter and white matter intensity images acquired at 3T and 7T, showing improved accuracy, sensitivity, and specificity of patient classification at 7T. Reduced hippocampus and hippocampal subfield volumes are a robust finding at lower field strengths in schizophrenia-spectrum disorders (43, 44). These findings have been replicated at 7T in first-episode psychosis (41) and childhood-onset schizophrenia (45), with the latter reporting multiscale associations with cognitive impairment. In an innovative study highlighting the qualitative improvements of UHF-sMRI, Kirov et al. (46) visualized the granule cell layer of the hippocampal dentate gyrus at 232 × 232 × 1,500 μm in 16 schizophrenia patients and 15 healthy controls. They observed that lower neuroradiologist-rated intensity was related to diagnosis, but not to symptom severity or disease duration.
Other UHF-sMRI findings highlight multiscale associations between psychotic symptoms and structural alterations in schizophrenia, particularly within the cingulum bundle and cingulate cortex. Palaniyappan et al. (47) reported associations between negative formal thought disorder (i.e., impoverished thought) and morphometric alterations in gray matter intensity in cingulate cortex as well as insula, striatum, precuneus, and frontotemporal regions. These authors later observed that decreased magnetization transfer ratio, a proxy for reduced myelin content, in the left cingulum bundle was associated with Schneiderian delusion severity (48). UHF diffusion-weighted MRI has also implicated the cingulum bundle in psychotic symptoms. Pan et al. (33) found associations between high conceptual disorganization and decreased fractional anisotropy in the cingulum as well as decreased fiber volumes extending from this seed region. More recently, Liang et al. (49) recovered a consistent subtype of patients with schizophrenia with marked and generalized cortical thinning; this subtype was noticeable even when a smaller sample was studied using 7T compared to 3T MRI, and was associated with higher 7T-MRS derived glutamate levels. In another novel application, Kim et al. (50) used a hybrid 7T MRI-PET to report prefrontal serotonin transporter distribution in relation to insight in schizophrenia.
UHF-sMRI studies in psychosis have transcended simple replication of findings observed at lower field strengths. Researchers now have the opportunity to visualize small brain structures at unprecedented resolutions in vivo, as exemplified by imaging of the hippocampus dentate granule cell layer (46). UHF-sMRI also yields better classification of patients and controls relative to lower field strengths, which will bolster efforts toward translational neuroscience and precision psychiatry.
Alongside these advantages, there are several considerations in structural UHF neuroimaging. Of particular relevance to psychiatry, UHF-sMRI is highly sensitive to motion artifacts, which are often more pronounced in psychiatric populations (51). Moreover, high-field strengths lead to increased susceptibility artifacts, especially within deep brain regions commonly the focus of psychiatric studies (e.g., hippocampus). Potential artifacts can counteract the benefits of UHF neuroimaging and should be minimized during both data acquisition (e.g., prospective motion correction) and processing (e.g., retrospective motion correction, gradient correction). Though UHF improvements in SNR allow for smaller samples to detect effects, most UHF-sMRI studies to date include less than 30 patients, which brings into question the representativeness of these findings. Future work in larger and more diverse samples (e.g., age, sex, gender, language, ethnicity, psychosis stage) are warranted. Larger samples would also allow for further investigation into potential confounding or moderating variables, including sociodemographics and clinical comorbidities. Incorporating these considerations into future work examining dysconnectivity and multiscale associations at the micro and social scales will build toward a broader picture of psychosis.
UHF functional MRI (UHF-fMRI) enjoys the same advantages as UHF-sMRI in terms of increased spatial resolution, leading to smaller voxel sizes and, thus, reduced partial volume errors. This improves blood-oxygen-level-dependent signal estimates from smaller brain regions, particularly in subcortical structures, and allows for more precise estimates of brain connectivity. Moreover, UHF confers increased temporal resolution as well as shortened acquisition times in some cases, which allows for greater sensitivity and benefits participants with less time in the scanner, which is particularly relevant for those with psychiatric disorders, such as schizophrenia. Novel techniques, including direct imaging of neuronal activity (DIANA-fMRI) recently demonstrated in rodents (52), offer unprecedented potential to combine spatial and temporal resolution to make precise neurophysiological inferences using UHF-fMRI.
We identified 11 UHF-fMRI studies, including 8 resting-state and 3 task-based studies using either a Stroop or passive listening task (Table 3). The majority of these (n = 9) employed first-episode psychosis or first-episode schizophrenia samples. Resting-state findings included both increased and decreased connectivity patterns in psychosis relative to healthy controls (53, 54), consistent with findings at lower field strengths (5). During a passive listening task, Doucet et al. (55) reported auditory cortex hyperactivity and altered tonotopic organization in schizophrenia patients with a history of hallucinations. Multiscale studies have linked functional dysconnectivity to disease duration (54), positive symptom severity (56, 57), the Stroop effect (10, 58, 59), and glutamate/glutathione dysfunction through MRS (10, 36, 59). For example, Limongi et al. (10) identified associations between altered glutamatergic neurotransmission (microscale) and functional dysconnectivity (mesoscale) in FEP, which was associated with Stroop performance (manifest-scale) and social withdrawal (social-scale). More recently, Alonso-Sanchez et al. (60) demonstrated that a computational linguistic measure of similarity among adjacent words (word-to-word semantic vector relationship in spoken text generated outside the scanner) is increased in first-episode schizophrenia, and this abnormality is related to higher intrinsic inhibitory tone within the Broca’s area and semantic hub, parameterized using a spectral dynamic causal model of resting-state 7T fMRI acquisition.
UHF-fMRI in psychosis has primarily leveraged quantitative improvements over lower field strengths, including greater signal to noise ratio and reduced partial volume effects relative to lower field strengths. This leads to greater sensitivity (14) in modest samples (Ns = 14–30 in Table 3) and in smaller brain regions while maintaining effect sizes comparable to those at lower field strengths (54). To date, UHF-fMRI studies have supported previous findings of both resting-state and task-based functional dysconnectivity in psychosis and linked these alterations to dysfunction over multiple scales, from the microscale to the social scale.
Though UHF-fMRI brings with it several advantages in terms of spatiotemporal resolution that benefit studies involving small brain regions and connectivity, these have not yet been widely implemented in schizophrenia. Dedicated UHF-fMRI acquisition protocols may help bring out both the quantitative and qualitative advantages of UHF in fMRI research. At the same time, as with UHF-sMRI, head motion is a major consideration for UHF-fMRI studies, as its effects on signal integrity are exacerbated at higher magnetic field strengths, which can be particularly problematic in populations with more excessive motion concerns (e.g., psychosis; (51)]. Several technological advances in MRI acquisition protocols (61, 62) and processing techniques (63) are promising in terms of mitigating these issues, and should be incorporated into future work investigating broader features at the manifest-scale (e.g., cognition) and probing longitudinal trajectories. In addition, few task-based UHF-fMRI studies have been conducted in psychosis, though task-fMRI provides a great opportunity to examine multiscale associations at all levels and particularly between the meso and manifest scales. As with most UHF studies in psychosis to date, future work with larger and more diverse samples across psychosis stages will allow for greater representativeness and examination of broader questions related to sensitivity and specificity of findings.
Psychiatric symptoms fluctuate over time as do their underlying neurobiological markers, which underscores the importance of longitudinal multiscale investigations. Though the relative novelty and limited accessibility of UHF scanners have hindered acquisition of longitudinal data, three longitudinal studies have been published to date. These studies primarily focused on metabolite variations between first-episode psychosis patients and healthy controls using UHF-MRS.
Jeon et al. (64) examined astroglial integrity using MRS indexed by myo-inositol concentration in the ACC in first-episode psychosis patients before and after the first 6 months of treatment as well as healthy controls. Astrocytes serve many roles such as maintaining reductive-oxidative processes, synaptic integrity, and myelination along with removing extracellular glutamate from the synaptic cleft space. However, it is challenging to perform in vivo imaging of astrocytes, hence the use of myo-inositol as a biomarker to assess astroglial activity. At baseline, FEP patients showed reduced myo-inositol concentration in the ACC relative to controls that normalized after treatment, indicating dynamic astroglial processing in FEP and supporting myo-inositol as a biomarker of psychosis.
Continuing redox imbalances, glutathione and glutamate have been observed to improve early intervention outcomes among patients that are treatment resistant to dopamine-blocking antipsychotics. Dempster et al. (28) assessed baseline glutathione and glutamate levels in the dorsal ACC, reporting no significant differences between patients and controls. However, higher glutathione levels were associated with faster treatment response, suggesting that treatments targeting glutathione levels may improve outcomes of early intervention in first episode psychosis. Jeon et al. (2) investigated the relationship between abnormal glutamatergic neurotransmission and illness trajectory in schizophrenia, but observed no significant progressive change of glutamate levels in the dorsal ACC over a 6-month period.
Increasing availability of UHF scanners along with pulse sequence and coil developments will facilitate longitudinal studies assessing structural, functional, and chemical metrics in the pathophysiology of schizophrenia across various illness stages. Collaborative efforts to harmonize and acquire 7T data across the emerging global centers will be critical to account for relatively small sample sizes reported in the current literature.
This review highlighted key findings, insights, challenges, and future directions of studies to-date of psychosis using UHF neuroimaging. Due to its increased SNR, resolution, and sensitivity, UHF neuroimaging shows promise in uncovering novel neurobiological mechanisms underlying psychiatric disorders and disentangling findings derived from lower field strengths. With the rapid increase in popularity and interest in applying UHF in psychiatric and neurocognitive disorders, there is an expectation for the sophisticated modality to provide new or confirm existing findings at lower field strengths with concrete biomarkers for psychosis. Replication of robust findings at 3T, such as hippocampal volume reductions in schizophrenia and associations with symptoms, demonstrate that potential artifacts at UHF can be addressed. UHF studies that judiciously combine multiple scales of measurements from live subjects are providing greater insight into the cascading effects of dysconnectivity from the synapse-to-the-streets, clarifying multiple manifestations of psychosis. Expanding UHF multiscale studies to combine structural and functional imaging with neuro-metabolite studies will allow researchers to target as-of-yet undetectable metabolites and gather evidence of metabolite imbalances at the brain network level. Incorporating these studies with behavioral and experiential measures at the manifest and social scales will facilitate translational research and broader clinical applications, including precision diagnostics and treatment. UHF research in psychiatry is still in its infancy and most studies are affected by small sample sizes and limited acquisitions, yet its sensitivity in demonstrating neurochemical, structural, and functional changes in psychosis despite these limitations highlights its clinical potential.
KL led preparing the review and drafted the initial version along with KK who wrote a part of the first draft. LP conceived, directed the review, and revised the manuscript. All authors approved the final draft and agreed to be accountable for the content of the work.
LP acknowledges research support from the Tanna Schulich Chair of Neuroscience and Mental Health (Schulich School of Medicine, Western University: 2019-2022); Monique H. Bourgeois Chair in Developmental Disorders and Graham Boeckh Foundation (Douglas Research Centre, McGill University) and salary award from the Fonds de Recherche du Quebec-Sante (FRQS). KL was supported by a Mitacs Accelerate fellowship in partnership with Otsuka Canada. KK was supported by an Ontario Graduate Scholarship. The funders were not involved in the study design, data collection, analysis, interpretation of data, the writing of this article or the decision to submit it for publication.
We thank Joe Gati and Rob Bartha of the Centre for Functional and Metabolic Mapping (CFMM), Robarts Research Institute, Western University for their advice on technical aspects of this chapter. We are also grateful to Jean Theberge for his critical inputs.
LP reports personal fees from Janssen Canada, Otsuka Canada, SPMM Course Limited, UK, Canadian Psychiatric Association; book royalties from Oxford University Press; investigator-initiated educational grants from Janssen Canada, Sunovion and Otsuka Canada in the last 5 years outside the submitted work. KL reports speaker’s honoraria from Otsuka Canada outside this work.
The remaining author declares that the research was conducted in the absence of any commercial or financial relationships that could be construed as a potential conflict of interest.
All claims expressed in this article are solely those of the authors and do not necessarily represent those of their affiliated organizations, or those of the publisher, the editors and the reviewers. Any product that may be evaluated in this article, or claim that may be made by its manufacturer, is not guaranteed or endorsed by the publisher.
1. Kahn RS, Sommer IE, Murray RM, Meyer-Lindenberg A, Weinberger DR, Cannon TD, et al. Schizophrenia. Nat Rev Dis Primers. (2015) 1:15067. doi: 10.1038/nrdp.2015.67
2. Jeon P, Limongi R, Ford SD, Mackinley M, Dempster K, Théberge J, et al. Progressive changes in glutamate concentration in early stages of schizophrenia: a longitudinal 7-tesla Mrs study. Schizophr Bull Open. (2021) 2:sgaa072. doi: 10.1093/schizbullopen/sgaa072
3. McCutcheon RA, Krystal JH, Howes OD. Dopamine and glutamate in schizophrenia: biology, symptoms and treatment. World Psychiatry. (2020) 19:15–33. doi: 10.1002/wps.20693
4. Guo S, He N, Liu Z, Linli Z, Tao H, Palaniyappan L. Brain-wide functional dysconnectivity in schizophrenia: parsing diathesis, resilience, and the effects of clinical expression. Can J Psychiatry. (2020) 65:21–9. doi: 10.1177/0706743719890174
5. Pettersson-Yeo W, Allen P, Benetti S, McGuire P, Mechelli A. Dysconnectivity in schizophrenia: where are we now? Neurosci Biobehav Rev. (2011) 35:1110–24. doi: 10.1016/j.neubiorev.2010.11.004
6. van den Heuvel MP, Fornito A. Brain networks in schizophrenia. Neuropsychol Rev. (2014) 24:32–48. doi: 10.1007/s11065-014-9248-7
7. Lepage M, Hawco C, Bodnar M. Relational memory as a possible neurocognitive marker of schizophrenia. JAMA Psychiatry. (2015) 72:946–7.
8. Raucher-Chéné D, Thibaudeau E, Sauvé G, Lavigne KM, Lepage M. Understanding others as a mediator between verbal memory and negative symptoms in schizophrenia-spectrum disorder. J Psychiatr Res. (2021) 143:429–35. doi: 10.1016/j.jpsychires.2021.10.007
9. Palaniyappan L. Dissecting the neurobiology of linguistic disorganisation and impoverishment in schizophrenia. Semin Cell Dev Biol. (2021) 129:47–60. doi: 10.1016/j.semcdb.2021.08.015
10. Limongi R, Jeon P, Mackinley M, Das T, Dempster K, Théberge J, et al. Glutamate and dysconnection in the salience network: neurochemical, effective connectivity, and computational evidence in schizophrenia. Biol Psychiatry. (2020) 88:273–81. doi: 10.1016/j.biopsych.2020.01.021
11. Palaniyappan L, Kanagasabai K, Lavigne KM. Psychiatric applications as Uhf. In: Markenroth Bloch K, Guye M, Poser BA editors. Ultra-High Field Neuro Mri. Amsterdam: Elsevier (in press).
12. Cattarinussi G, Delvecchio G, Maggioni E, Bressi C, Brambilla P. Ultra-high field imaging in major depressive disorder: a review of structural and functional studies. J Affect Disord. (2021) 290:65–73. doi: 10.1016/j.jad.2021.04.056
13. Cai Y, Hofstetter S, van der Zwaag W, Zuiderbaan W, Dumoulin SO. Individualized cognitive neuroscience needs 7t: comparing numerosity maps at 3t and 7t Mri. NeuroImage. (2021) 237:118184. doi: 10.1016/j.neuroimage.2021.118184
14. Neuner I, Veselinović T, Ramkiran S, Rajkumar R, Schnellbaecher GJ, Shah NJ. 7t Ultra-high-field neuroimaging for mental health: an emerging tool for precision psychiatry? Trans Psychiatry. (2022) 12:36. doi: 10.1038/s41398-022-01787-3
15. Choi IY, Andronesi OC, Barker P, Bogner W, Edden RA, Kaiser LG, et al. Spectral editing in 1h magnetic resonance spectroscopy: experts’ consensus recommendations. NMR Biomed. (2021) 34:e4411. doi: 10.1002/nbm.4411
16. Younis S, Hougaard A, Christensen CE, Vestergaard MB, Petersen ET, Boer VO, et al. Feasibility of glutamate and gaba detection in pons and thalamus at 3t and 7t by proton magnetic resonance spectroscopy. Front Neurosci. (2020) 14:559314. doi: 10.3389/fnins.2020.559314
17. Post MR, Sulzer D. The chemical tools for imaging dopamine release. Cell Chem Biol. (2021) 28:748–64. doi: 10.1016/j.chembiol.2021.04.005
18. Wijtenburg SA, Wang M, Korenic SA, Chen S, Barker PB, Rowland LM. Metabolite alterations in adults with schizophrenia, first degree relatives, and healthy controls: a multi-region 7t Mrs study. Front Psychiatry. (2021) 12:584. doi: 10.3389/fpsyt.2021.656459
19. Thakkar KN, Rösler L, Wijnen JP, Boer VO, Klomp DW, Cahn W, et al. 7t Proton magnetic resonance spectroscopy of gamma-aminobutyric acid, glutamate, and glutamine reveals altered concentrations in patients with schizophrenia and healthy siblings. Biol Psychiatry. (2017) 81:525–35. doi: 10.1016/j.biopsych.2016.04.007
20. Wang AM, Pradhan S, Coughlin JM, Trivedi A, DuBois SL, Crawford JL, et al. Assessing brain metabolism with 7-T proton magnetic resonance spectroscopy in patients with first-episode psychosis. JAMA Psychiatry. (2019) 76:314–23. doi: 10.1001/jamapsychiatry.2018.3637
21. Marsman A, Mandl RC, Klomp DW, Bohlken MM, Boer VO, Andreychenko A, et al. Gaba and glutamate in schizophrenia: a 7 T 1h-Mrs study. NeuroImage Clin. (2014) 6:398–407.
22. Reid MA, Salibi N, White DM, Gawne TJ, Denney TS, Lahti AC. 7t Proton magnetic resonance spectroscopy of the anterior cingulate cortex in first-episode schizophrenia. Schizophr Bull. (2019) 45:180–9. doi: 10.1093/schbul/sbx190
23. Quiñones GM, Mayeli A, Yushmanov VE, Hetherington HP, Ferrarelli F. Reduced gaba/glutamate in the thalamus of individuals at clinical high risk for psychosis. Neuropsychopharmacology. (2021) 46:1133–9. doi: 10.1038/s41386-020-00920-4
24. Sydnor VJ, Roalf DR. A meta-analysis of ultra-high field glutamate, glutamine, gaba and glutathione 1hmrs in psychosis: implications for studies of psychosis risk. Schizophr Res. (2020) 226:61–9. doi: 10.1016/j.schres.2020.06.028
25. Broeders TAA, Bhogal AA, Morsinkhof LM, Schoonheim MM, Röder CH, Edens M, et al. Glutamate levels across deep brain structures in patients with a psychotic disorder and its relation to cognitive functioning. J Psychopharmacol. (2022) 36:489–97. doi: 10.1177/02698811221077199
26. Roalf DR, Nanga RPR, Rupert PE, Hariharan H, Quarmley M, Calkins ME, et al. Glutamate imaging (Glucest) reveals lower brain glucest contrast in patients on the psychosis spectrum. Mol Psychiatry. (2017) 22:1298–305. doi: 10.1038/mp.2016.258
27. Cember ATJ, Nanga RPR, Reddy R. Glutamate-weighted cest (Glucest) imaging for mapping neurometabolism: an update on the state of the art and emerging findings from in vivo applications. NMR Biomed. (2022) e4780. doi: 10.1002/nbm.4780
28. Dempster K, Jeon P, MacKinley M, Williamson P, Théberge J, Palaniyappan L. Early treatment response in first episode psychosis: a 7-T magnetic resonance spectroscopic study of glutathione and glutamate. Mol Psychiatry. (2020) 25:1640–50. doi: 10.1038/s41380-020-0704-x
29. Godlewska BR, Minichino A, Emir U, Angelescu I, Lennox B, Micunovic M, et al. Brain glutamate concentration in men with early psychosis: a magnetic resonance spectroscopy case–control study at 7 T. Trans Psychiatry. (2021) 11:1–7. doi: 10.1038/s41398-021-01477-6
30. Yang K, Longo L, Narita Z, Cascella N, Nucifora FC, Coughlin JM, et al. A multimodal study of a first episode psychosis cohort: potential markers of antipsychotic treatment resistance. Mol Psychiatry. (2022) 27:1184–91. doi: 10.1038/s41380-021-01331-7
31. Papa M, De Luca C, Petta F, Alberghina L, Cirillo G. Astrocyte–neuron interplay in maladaptive plasticity. Neurosci Biobehav Rev. (2014) 42:35–54. doi: 10.1016/j.neubiorev.2014.01.010
32. Palaniyappan L, Sabesan P, Li X, Luo Q. Schizophrenia increases variability of the central antioxidant system: a meta-analysis of variance from Mrs studies of glutathione. Front Psychiatry. (2021) 12:796466. doi: 10.3389/fpsyt.2021.796466
33. Pan Y, Dempster K, Jeon P, Théberge J, Khan AR, Palaniyappan L. Acute conceptual disorganization in untreated first-episode psychosis: a combined magnetic resonance spectroscopy and diffusion imaging study of the cingulum. J Psychiatry Neurosci. (2021) 46:E337–46. doi: 10.1503/jpn.200167
34. MacKinley M, Ford SD, Jeon P, Théberge J, Palaniyappan L. Central oxidative stress and early vocational outcomes in first episode psychosis: a 7-tesla magnetic resonance spectroscopy study of glutathione. Schizophr Bull. (2022) 48:921–30. doi: 10.1093/schbul/sbac012
35. Kumar J, Liddle EB, Fernandes CC, Palaniyappan L, Hall EL, Robson SE, et al. Glutathione and glutamate in schizophrenia: a 7t Mrs study. Mol Psychiatry. (2020) 25:873–82.
36. Limongi R, Jeon P, Théberge J, Palaniyappan L. Counteracting effects of glutathione on the glutamate-driven excitation/inhibition imbalance in first-episode schizophrenia: a 7t Mrs and dynamic causal modeling study. Antioxidants. (2021) 10:75. doi: 10.3390/antiox10010075
37. Jeon P, Limongi R, Ford SD, Branco C, Mackinley M, Gupta M, et al. Glutathione as a molecular marker of functional impairment in patients with at-risk mental state: 7-tesla 1h-Mrs study. Brain Sci. (2021) 11:941. doi: 10.3390/brainsci11070941
38. Rowland L, Pradhan S, Korenic S, Wijtenburg S, Hong L, Edden R, et al. Elevated brain lactate in schizophrenia: a 7 T magnetic resonance spectroscopy study. Trans Psychiatry. (2016) 6:e967. doi: 10.1038/tp.2016.239
39. Bell TK, Godfrey KJ, Ware AL, Yeates KO, Harris AD. Harmonization of multi-site mrs data with combat. Neuroimage. (2022) 257:119330. doi: 10.1016/j.neuroimage.2022.119330
40. Lieberman JA, Girgis RR, Brucato G, Moore H, Provenzano F, Kegeles L, et al. Hippocampal dysfunction in the pathophysiology of schizophrenia: a selective review and hypothesis for early detection and intervention. Mol Psychiatry. (2018) 23:1764–72. doi: 10.1038/mp.2017.249
41. Park MTM, Jeon P, Khan AR, Dempster K, Chakravarty MM, Lerch JP, et al. Hippocampal neuroanatomy in first episode psychosis: a putative role for glutamate and serotonin receptors. Prog Neuropsychopharmacol Biol Psychiatry. (2021) 110:110297. doi: 10.1016/j.pnpbp.2021.110297
42. Iwabuchi S, Liddle PF, Palaniyappan L. Clinical utility of machine-learning approaches in schizophrenia: improving diagnostic confidence for translational neuroimaging. Front Psychiatry. (2013) 4:95. doi: 10.3389/fpsyt.2013.00095
43. van Erp TG, Hibar DP, Rasmussen JM, Glahn DC, Pearlson GD, Andreassen OA, et al. Subcortical brain volume abnormalities in 2028 individuals with schizophrenia and 2540 healthy controls via the enigma consortium. Mol Psychiatry. (2016) 21:547.
44. Haukvik UK, Tamnes CK, Söderman E, Agartz I. Neuroimaging hippocampal subfields in schizophrenia and bipolar disorder: a systematic review and meta-analysis. J Psychiatr Res. (2018) 104:217–26. doi: 10.1016/j.jpsychires.2018.08.012
45. Zhou D, Liu S, Zhou X, Berman R, Broadnax D, Gochman P, et al. 7 T Mri reveals hippocampal structural abnormalities associated with memory intrusions in childhood-onset schizophrenia. Schizophr Res. (2018) 202:431–2. doi: 10.1016/j.schres.2018.07.023
46. Kirov II, Hardy CJ, Matsuda K, Messinger J, Cankurtaran CZ, Warren M, et al. In vivo 7 tesla imaging of the dentate granule cell layer in schizophrenia. Schizophr Res. (2013) 147:362–7. doi: 10.1016/j.schres.2013.04.020
47. Palaniyappan L, Mahmood J, Balain V, Mougin O, Gowland PA, Liddle PF. Structural correlates of formal thought disorder in schizophrenia: an ultra-high field multivariate morphometry study. Schizophr Res. (2015) 168:305–12. doi: 10.1016/j.schres.2015.07.022
48. Palaniyappan L, Al-Radaideh A, Mougin O, Das T, Gowland P, Liddle PF. Aberrant myelination of the cingulum and schneiderian delusions in schizophrenia: a 7t magnetization transfer study. Psychol Med. (2019) 49:1890–6. doi: 10.1017/S0033291718002647
49. Liang L, Heinrichs RW, Liddle PF, Jeon P, Théberge J, Palaniyappan L. Cortical impoverishment in a stable subgroup of schizophrenia: validation across various stages of psychosis. Schizophr Res. (2022). doi: 10.1016/j.schres.2022.05.013
50. Kim JH, Son YD, Kim HK, Kim JH. Association between lack of insight and prefrontal serotonin transporter availability in antipsychotic-free patients with schizophrenia: a high-resolution pet study with [(11)C]Dasb. Neuropsychiatr Dis Treat. (2021) 17:3195–203. doi: 10.2147/NDT.S336126
51. Makowski C, Lepage M, Evans AC. Head motion: the dirty little secret of neuroimaging in psychiatry. J Psychiatry Neurosci. (2019) 44:62–8. doi: 10.1503/jpn.180022
52. Toi PT, Jang HJ, Min K, Kim S-P, Lee S-K, Lee J, et al. In vivo direct imaging of neuronal activity at high temporospatial resolution. Science. (2022) 378:160–8. doi: 10.1126/science.abh4340
53. Dey A, Dempster K, MacKinley M, Jeon P, Das T, Khan A, et al. Conceptual disorganization and redistribution of resting-state cortical hubs in untreated first-episode psychosis: a 7t study. NPJ Schizophr. (2021) 7:1–9. doi: 10.1038/s41537-020-00130-3
54. Hua J, Blair NI, Paez A, Choe A, Barber AD, Brandt A, et al. Altered functional connectivity between sub-regions in the thalamus and cortex in schizophrenia patients measured by resting state bold Fmri at 7t. Schizophr Res. (2019) 206:370–7. doi: 10.1016/j.schres.2018.10.016
55. Doucet GE, Luber MJ, Balchandani P, Sommer IE, Frangou S. Abnormal auditory tonotopy in patients with schizophrenia. NPJ Schizophr. (2019) 5:16. doi: 10.1038/s41537-019-0084-x
56. Limongi R, Mackinley M, Dempster K, Khan AR, Gati JS, Palaniyappan L. Frontal–striatal connectivity and positive symptoms of schizophrenia: implications for the mechanistic basis of prefrontal Rtms. Eur Arch Psychiatry Clin Neurosci. (2021) 271:3–15. doi: 10.1007/s00406-020-01163-6
57. Lottman KK, Gawne TJ, Kraguljac NV, Killen JF, Reid MA, Lahti AC. Examining resting-state functional connectivity in first-episode schizophrenia with 7t Fmri and meg. NeuroImage Clin. (2019) 24:101959. doi: 10.1016/j.nicl.2019.101959
58. Gawne TJ, Overbeek GJ, Killen JF, Reid MA, Kraguljac NV, Denney TS, et al. A multimodal magnetoencephalography 7 T Fmri and 7 T proton Mr spectroscopy study in first episode psychosis. npj Schizophr. (2020) 6:1–9. doi: 10.1038/s41537-020-00113-4
59. Overbeek G, Gawne TJ, Reid MA, Salibi N, Kraguljac NV, White DM, et al. Relationship between cortical excitation and inhibition and task-induced activation and deactivation: a combined magnetic resonance spectroscopy and functional magnetic resonance imaging study at 7t in first-episode psychosis. Biol Psychiatry Cogn Neurosci Neuroimaging. (2019) 4:121–30. doi: 10.1016/j.bpsc.2018.10.002
60. Alonso-Sánchez MF, Limongi R, Gati J, Palaniyappan L. Language network self-inhibition and semantic similarity in first-episode schizophrenia: a computational-linguistic and effective connectivity approach. Schizophr Res. (2022). doi: 10.1016/j.schres.2022.04.007
61. Gras V, Poser BA, Wu X, Tomi-Tricot R, Boulant N. Optimizing bold sensitivity in the 7t human connectome project resting-state Fmri protocol using plug-and-play parallel transmission. NeuroImage. (2019) 195:1–10. doi: 10.1016/j.neuroimage.2019.03.040
62. Vu AT, Jamison K, Glasser MF, Smith SM, Coalson T, Moeller S, et al. Tradeoffs in pushing the spatial resolution of Fmri for the 7t human connectome project. Neuroimage. (2017) 154:23–32. doi: 10.1016/j.neuroimage.2016.11.049
63. Huang P, Carlin JD, Henson RN, Correia MM. Improved motion correction of submillimetre 7t Fmri time series with boundary-based registration (Bbr). NeuroImage. (2020) 210:116542. doi: 10.1016/j.neuroimage.2020.116542
64. Jeon P, Mackinley M, Théberge J, Palaniyappan L. The trajectory of putative astroglial dysfunction in first episode schizophrenia: a longitudinal 7-tesla mrs study. Sci Rep. (2021) 11:22333. doi: 10.1038/s41598-021-01773-7
65. Brandt AS, Unschuld PG, Pradhan S, Lim IA, Churchill G, Harris AD, et al. Age-related changes in anterior cingulate cortex glutamate in schizophrenia: a H mrs study at 7 tesla. Schizophr Res. (2016) 172:101–5. doi: 10.1016/j.schres.2016.02.017
66. Posporelis S, Coughlin JM, Marsman A, Pradhan S, Tanaka T, Wang H, et al. Decoupling of brain temperature and glutamate in recent onset of schizophrenia: a 7t proton magnetic resonance spectroscopy study. Biol Psychiatry Cogn Neurosci Neuroimaging. (2018) 3:248–54. doi: 10.1016/j.bpsc.2017.04.003
67. Hua J, Brandt AS, Lee S, Blair NI, Wu Y, Lui S, et al. Abnormal grey matter arteriolar cerebral blood volume in schizophrenia measured with 3d inflow-based vascular-space-occupancy Mri at 7t. Schizophr Bull. (2017) 43:620–32. doi: 10.1093/schbul/sbw109
68. Lottman KK, White DM, Kraguljac NV, Reid MA, Calhoun VD, Catao F, et al. Four-way multimodal fusion of 7 T imaging data using an Mcca+Jica model in first-episode schizophrenia. Hum Brain Mapp. (2018) 39:1475–88. doi: 10.1002/hbm.23906
69. Palaniyappan L, Al-Radaideh A, Mougin O, Gowland P, Liddle PF. Combined white matter imaging suggests myelination defects in visual processing regions in schizophrenia. Neuropsychopharmacology. (2013) 38:1808–15. doi: 10.1038/npp.2013.80
Keywords: schizophrenia, psychosis, ultra-high field neuroimaging, 7 tesla, magnetic resonance spectroscopy, magnetic resonance imaging, multiscale
Citation: Lavigne KM, Kanagasabai K and Palaniyappan L (2022) Ultra-high field neuroimaging in psychosis: A narrative review. Front. Psychiatry 13:994372. doi: 10.3389/fpsyt.2022.994372
Received: 14 July 2022; Accepted: 08 November 2022;
Published: 24 November 2022.
Edited by:
Stefan Borgwardt, University of Lübeck, GermanyReviewed by:
Tsutomu Takahashi, University of Toyama, JapanCopyright © 2022 Lavigne, Kanagasabai and Palaniyappan. This is an open-access article distributed under the terms of the Creative Commons Attribution License (CC BY). The use, distribution or reproduction in other forums is permitted, provided the original author(s) and the copyright owner(s) are credited and that the original publication in this journal is cited, in accordance with accepted academic practice. No use, distribution or reproduction is permitted which does not comply with these terms.
*Correspondence: Lena Palaniyappan, bGVuYS5wYWxhbml5YXBwYW5AbWNnaWxsLmNh
Disclaimer: All claims expressed in this article are solely those of the authors and do not necessarily represent those of their affiliated organizations, or those of the publisher, the editors and the reviewers. Any product that may be evaluated in this article or claim that may be made by its manufacturer is not guaranteed or endorsed by the publisher.
Research integrity at Frontiers
Learn more about the work of our research integrity team to safeguard the quality of each article we publish.