- 1Department of Medical Imaging, Tianyou Hospital of Wuhan University of Science and Technology, Wuhan, China
- 2Key Laboratory of Occupational Hazards and Identification, Wuhan University of Science and Technology, Wuhan, China
- 3Department of Neurosurgery, Tianyou Hospital of Wuhan University of Science and Technology, Wuhan, China
- 4Department of Medical Imaging, Renmin Hospital of Wuhan University, Wuhan, China
While abnormal neuroimaging features have been reported in patients suffering from right temporal lobe epilepsy (rTLE), the value of altered degree centrality (DC) as a diagnostic biomarker for rTLE has yet to be established. As such, the present study was designed to examine DC abnormalities in rTLE patients in order to gauge the diagnostic utility of these neuroimaging features. In total, 68 patients with rTLE and 73 healthy controls (HCs) participated in this study. Imaging data were analyzed using DC and receiver operating characteristic (ROC) methods. Ultimately, rTLE patients were found to exhibit reduced right caudate DC and increased left middle temporal gyrus, superior parietal gyrus, superior frontal gyrus, right precuneus, frontal gyrus Inferior gyrus, middle-superior frontal gyrus, and inferior parietal gyrus DC relative to HC. ROC analyses indicated that DC values in the right caudate nucleus could be used to differentiate between rTLE patients and HCs with a high degree of sensitivity and specificity. Together, these results thus suggest that rTLE is associated with abnormal DC values in the right caudate nucleus, underscoring the relevance of further studies of the underlying pathophysiology of this debilitating condition.
Introduction
Temporal lobe epilepsy (TLE) is a chronic neurological disease that can arise in response to a range of factors (1). Repeated abnormal epileptic discharges in affected patients can contribute to neuronal degeneration and necrotic death, in turn contributing to impaired cognition and altered brain tissue structural characteristics (2, 3). These structural changes in the brain of TLE patients can also disrupt normal memory and learning activity (4). TLE accounts for over 40% of all epilepsy cases and is the most common form thereof (5). However, at present, the diagnosis of epilepsy is largely dependent on a combination of medical history and EEG results. While EEG can accurately diagnose epilepsy in some cases, the successful recording of epileptic discharges generally only occurs in half of affected patients, and some healthy individuals may also exhibit false-positive EEG readings (6). As seizures occur without warning and are transient in nature, this can further complicate diagnostic efforts. Recent advances in neuroimaging technologies have led to a growing focus on the use of computer-aided imaging as a means of automating efforts to diagnose patients and localize lesions (7–9). Multimodal neuroimaging efforts can also clarify changes in the brains of TLE patients, providing new insight into the pathogenesis of this disease while aiding in efforts to predict and diagnose affected individuals (9).
Several different non-invasive, high-resolution neuroimaging modalities have been leveraged to aid in the diagnosis of specific neurological conditions. To date, several different functional magnetic resonance imaging (fMRI) studies have reported detecting a distinct blood oxygen level-dependent (BOLD) signal in brain regions where seizure foci are located (10, 11). These fMRI techniques enable the effective localization of epileptic lesions with a combination of high temporal and spatial resolution (12). Reduced regional signal in the right posterior cingulate cortex and precuneus (PCu) regions, for example, has been identified as a biomarker characteristic of patients with epilepsy (13, 14). Moreover, altered amplitude of low-frequency fluctuation (ALFF) values in particular regions of the brain can reliably differentiate between epilepsy patients and healthy controls (HCs) with high levels of sensitivity and specificity (15, 16). Structural MRI studies have revealed that several brain regions in patients with epilepsy also exhibit structural abnormalities detectable through neuroimaging (17). However, no simple and accurate neuroimaging biomarker capable of guiding the early detection of TLE has yet been established.
Degree centrality (DC) is a whole-brain connectivity index that describes the global features of a given node through the use of graph theory models to assess the functional connectivity between that node and nodes throughout the brain (18). DC-based analytical approaches have recently been used to successfully evaluate patients diagnosed with schizophrenia, depression, and mild cognitive impairment (19–21). Moreover, the combination of DC and machine learning algorithms can predict responses to treatment in children with epilepsy undergoing antiepileptic medication treatment (22). Increases in voxel-wise DC values in specific brain regions correspond to an increased degree of global connectivity, whereas reductions in voxel-wise DC values are indicative of a reduction in the degree of global connectivity. Studies of voxel-based DC have been used to analyze altered brain functionality in patients with epilepsy at the whole-brain level, with one recent report having revealed the existence of abnormal DC findings in the medial superior frontal gyrus (MSFG), left dorsolateral superior frontal gyrus, right inferior parietal lobule, and the left postcentral region of adults with epilepsy (9). Therefore, it is necessary to combine DC and operating characteristic (ROC) methods when exploring imaging biomarkers of right temporal lobe epilepsy (rTLE).
The present study was developed with the goal of assessing whether rTLE patients exhibit abnormal DC activity, with the hypothesis that these abnormal DC values could be utilized as neuroimaging biomarkers to reliably distinguish between rTLE patients and HC individuals.
Materials and methods
Participants
In total, this study enrolled 68 patients with rTLE diagnosed as per the criteria established by the International Anti-Epilepsy League that were recruited from Tianyou Hospital Affiliated to Wuhan University of Science and Technology. In addition, 73 age- and sex-matched HC individuals were recruited from among patients at Tianyou hospital undergoing routine physical examinations. To be eligible for study inclusion, rTLE patients had to meet the following criteria: (1) patients exhibited typical TLE symptoms suggestive of the presence of epileptic foci in the right temporal lobe; (2) patients exhibited interictal EEG results consistent with the potential presence of right temporal lobe lesions; and (3) patients exhibited MRI results indicative of right hippocampal atrophy or sclerosis. Patients were excluded if they were left-handed or had any history of traumatic brain injury, psychiatric disease, or other neurological diseases. All participants provided written informed consent. The medical ethics committee of Tianyou Hospital Affiliated to Wuhan University of Science and Technology approved this study, which was consistent with the Declaration of Helsinki.
Magnetic resonance imaging acquisition and processing
A 3.0T Philips MRI instrument was used to collect all resting-state fMRI data at Tianyou Hospital. Participants were directed to remain awake with their eyes closed during imaging. All echoplanar imaging sequences were acquired with the following settings: repetition time/echo time (TR/TE) 2000/30 ms, 31 slices, 220 × 220 matrix, 90° flip angle, 24 cm field of view, 5 mm thick layers without gaps. The processing of all fMRI data was performed using the Data Processing Assistant for rs-fMRI, which functions with SPM12 implemented in MATLAB. The first 10 images for each participant were excluded from the analysis to allow for patient adaptation and to mitigate the potential effects of initial MRI signal instability. Participants were excluded if they exhibited maximum displacement >2 mm in the x-, y-, or z-axis directions or >2° of maximum angular rotation after correction for head movement and slice timing. The corrected imaging data were spatially normalized to the MNI space and resampled at 3 mm × 3 mm × 3 mm, after which they were subjected to temporal bandpass filtering (0.01–0.08 Hz) and linear detrending. Certain spurious covariates were removed from these images, including signals from ventricular seed-related regions and white matter central regions, as well as 24 head motion parameters derived from rigid body correction. Global signals were preserved when processing resting-state functional connectivity data, as in prior reports (23).
Degree centrality calculation
Degree centrality measurements were made using the REST-DC toolkit from the REST package1. Pearson correlation coefficients were used to compute DC values, with Pearson correlation coefficients corresponding to the relationship between all voxel pairs in a time series being used to establish a graph for each study participant. In this graph, individual brain voxels are regarded as nodes, while significant correlations between nodes are regarded as edges. The n*n Pearson correlation coefficient matrix between each pair of voxels was then utilized to establish subjective whole-brain functional connectivity. To enhance the normalization of these data, they were transformed into a Z-score matrix by applying Fisher’s R-to-Z transformation, with the sum of Z values between selected voxels and all other voxels being used to generate weighted voxel DC values. To eliminate any potential false connectivity, a Pearson correlation coefficient threshold of r > 0.25 was established by thresholding each correlation at P < 0.01.
Statistical analysis
Data were analyzed using SPSS 22.0 (SPSS Inc., IL, United States). Demographic differences were compared between groups using two-sample t-tests and chi-square tests.
Correlation analysis
Degree centrality values were extracted from brain regions that exhibited abnormalities between these two groups, after which Pearson correlation coefficients were used to detect the relationships between these abnormal DC values and clinical variables of interest.
Results
Demographics and clinical features
Participant demographic and clinical characteristics are shown in Table 1. There were no significant differences in age, sex, or education level between rTLE patients and HCs (P > 0.05).
Degree centrality differences
Significant differences in DC values in different regions of the brain were next identified by comparing data from rTLE patients and HCs. Relative to HC individuals, rTLE patients exhibited significantly reduced DC values in the right caudate gyrus, as well as significantly increased DC values in the left middle temporal gyrus (MTG), superior parietal gyrus (SPG), superior frontal gyrus (SFG) and right PCu, inferior frontal gyrus (IFG), MSFG, and inferior parietal gyrus (IPG; Figure 1 and Table 2). There were no significant correlations between any of the identified DC values and rTLE patient disease duration.
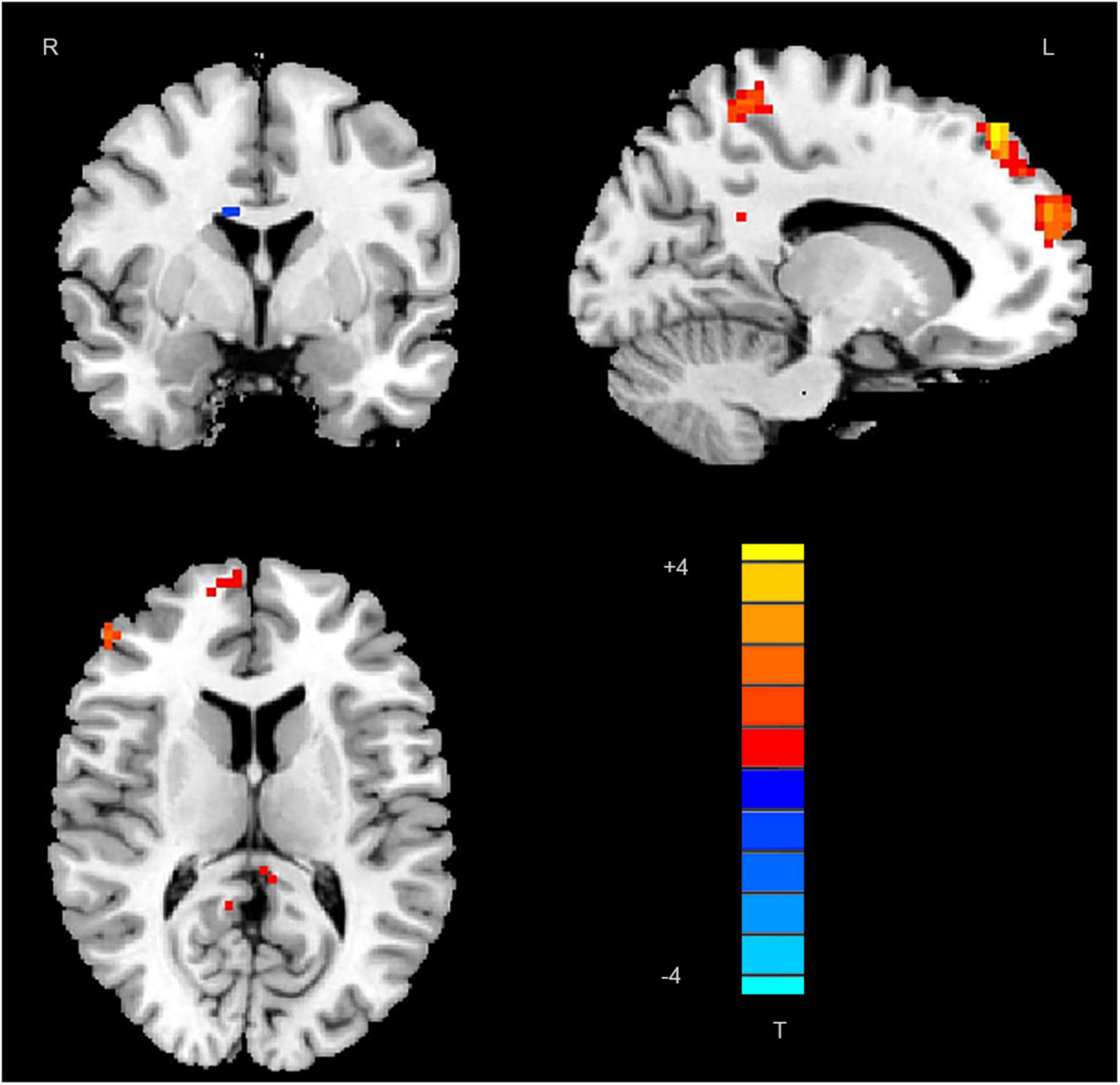
Figure 1. Decreased DC values in the right caudate and increased DC values in the left middle temporal gyrus, superior parietal gyrus, superior frontal gyrus, right precuneus, frontal gyrus Inferior gyrus, middle-superior frontal gyrus, and inferior parietal gyrus in patients. DC, degree centrality; MTG, middle temporal gyrus; SPG, superior parietal gyrus; SFG, superior frontal gyrus; PCu, precuneus; IFG, frontal gyrus Inferior gyrus; MSFG, middle-superior frontal gyrus; and IPG, inferior parietal gyrus.
Receiver operating characteristic results
In total, rTLE patients exhibited abnormal DC values in 8 regions of the brain (right caudate, left MTG, SFG, SPG, right PCu, IFG, MSFG, and IPG). Subsequent ROC analyses indicated that abnormal DC values in the right caudate nucleus exhibited the highest AUC value (0.8898; Figure 2).
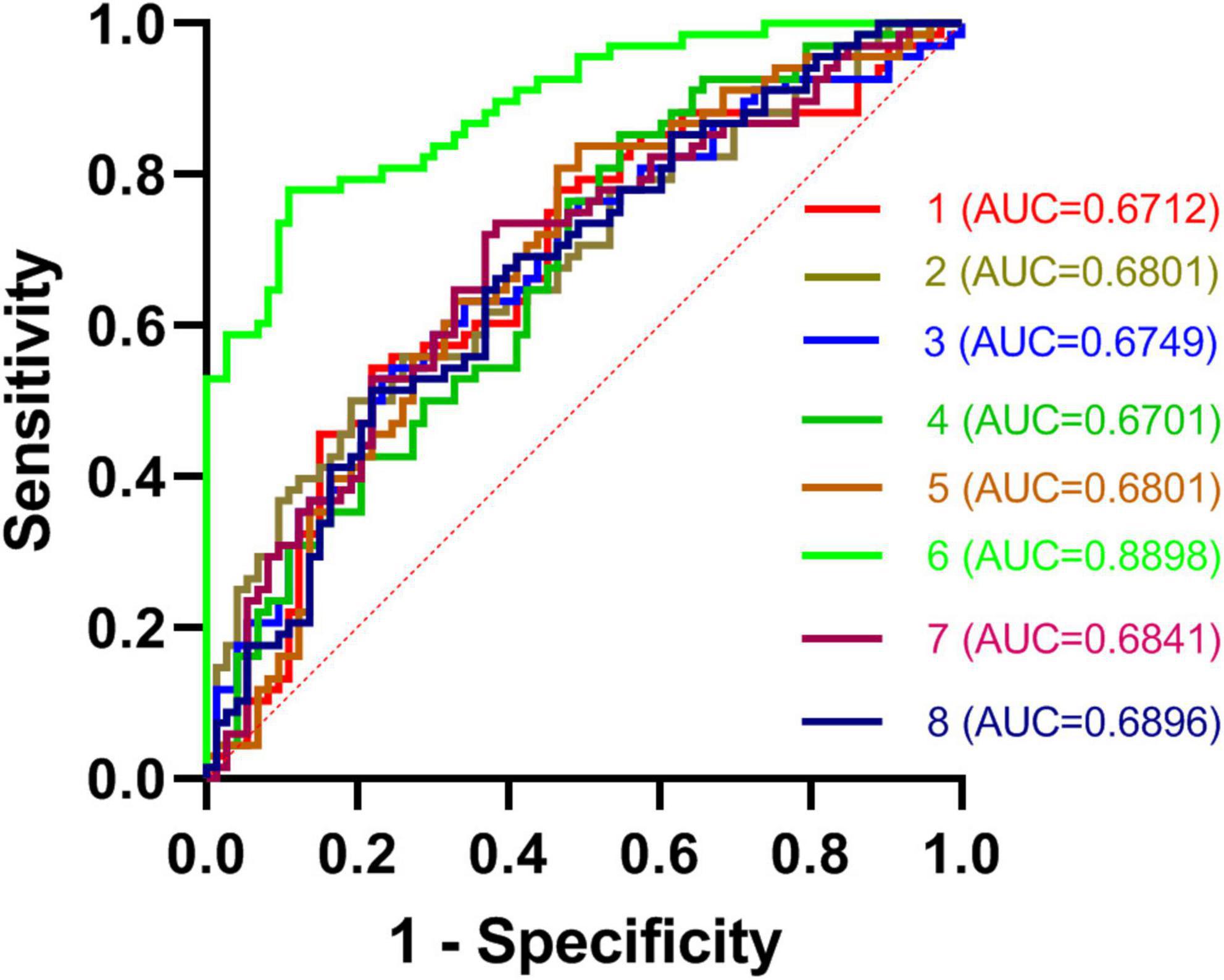
Figure 2. ROC curves for the differentiation between TLE patients and HCs based on DC values in abnormal brain regions. TLE, temporal lobe epilepsy; ROC, receiver operating characteristic; HCs, healthy controls; and DC, degree centrality.
Discussion
Here, DC values were used to differentiate between rTLE patients and HCs. Overall, patients diagnosed with rTLE were found to exhibit reduced DC values in the right caudate with corresponding increases in DC values in the left MTG, SPG, SFG, and right PCu, IFG, MSFG, and IPG relative to HCs. The abnormal DC values in the right caudate nucleus were able to effectively discriminate between these two participant groups with an AUC value of 0.8898.
Receiver operating characteristic analyses are commonly used for the diagnosis of neuropsychiatric disorders such as schizophrenia or major depression (24–26). To be reliable, a diagnostic indicator must exhibit an ROC value of 0.6 or higher (27). The AUC value derived from the ROC analysis of the right caudate nucleus was higher than that for any other tested regions exhibiting DC abnormalities between rTLE patients and controls. The caudate nucleus is an important mediator of cognition, and certain neurological disorders have been shown to differentially impact the dorsal and ventral caudate nuclei (28). Both functional activity and connectivity can be used to subdivide the caudate into these ventral and dorsal regions, with the dorsal caudate nucleus being closely associated with the dorsolateral prefrontal cortex and shaping executive function and working memory (29, 30). In contrast, the ventral caudate nucleus is more closely linked to the limbic system and to pain processing and other emotional functions. Many studies have evaluated the caudate nucleus in patients (31, 32). Here, right caudate nucleus DC values were significantly reduced in rTLE patients relative to HCs, with the connection strength between this region and other regions of the brain thus being reduced, potentially explaining the observed declines in learning, attention, and memory that these patients experience. However, these reduced DC values were not correlated with patient symptom severity or disease duration, suggesting that they are independent of disease severity in rTLE patients. Notably, this DC reduction in the right caudate nucleus was able to readily differentiate between rTLE patients and HCs, suggesting that this change is characteristic of this form of epilepsy.
The frontal lobe is a key mediator of cognitive control, with abnormalities in the structure and function of the frontal lobe being reported in prior studies of patients with TLE (33, 34). Patients exhibiting cognitive impairments including decreased language fluency and processing speed have been reported relative to HCs, as has a case of patients with notably poor cognitive function. Here, abnormal DC values in the MFG were observed in rTLE patients, potentially partially accounting for the cognitive deficits that TLE patients experience.
Many structural and functional studies of TLE patients focus on the temporal lobe, including the amygdala, HIC, parahippocampal gyrus, and entorhinal cortex (1). The MTG is an important component of semantic memory and language processing-related activity (35, 36). Recently, one study found that TLE was associated with alterations in the intrinsic connectivity of both temporal regions (37). The temporal lobe is also closely related to social cognition and emotional processing (4). Moreover, the MTG is a key node in a broad network of frontal, parietal, occipital, and subcortical structures, with abnormal MTG activation thus potentially being an important pathophysiological component of rTLE-related brain damage (38). Abnormal MTG activity may also impact temporal lobe function given its role as a regulator of language processing and semantic memory. Observed increases in DC in the MTG in rTLE patients may thus be linked to cognitive deficits, although memory testing was not employed in the present study.
The parietal lobe is closely related to key cognitive and sensory functions including memory, attention, motor learning, spatial perception, and visuomotor integration (39, 40). Anatomical and functional characteristics are used to subdivide the parietal lobe into five regions, two of which are related to motor processes and visual orientation, whereas the remaining three are associated with reasoning, working memory, visual perception, attention, and spatial cognition (41). The SPL region has been specifically linked to interaction and locomotion in macaques. Adult TLE patients exhibt increases in SPL density detected through structural MRI and fMRI studies (42). The SPL and the associated intraparietal groove are important in the context of multi-object tracking as analyzed based on changes in BOLD signal, with both of these regions being related to sustained attention and object indexing (43). The SPL additionally interacts with the prefrontal cortex to coordinate stimulus-oriented processes and executive function, and correlates with inhibition in the left inferior parietal cortex (44). Neuroimaging analyses have demonstrated a relationship between decreased visual acuity and the bilateral SPL (43). The contralateral posterior parietal cortex, ipsilateral inferior frontal gyrus, and ipsilateral frontal areas also exhibit more robust anatomical connections to the right posterior SPL rather than the left SPL. The results of this study suggest that increases in DC values in the SPL may be linked to the pathogenesis of rTLE.
The PCu is the master node of the default mode network, and is involved in the processing of visuospatial information, metaphors, mental imagery, and episodic memory (45). Structural MRI studies have revealed that significantly altered spontaneous brain activity in the bilateral PCu is linked to the pathophysiology of epilepsy and depression (46). Functional MRI analyses have further highlighted decreased activation clusters in the precuneus and supramarginal gyrus of TLE patients as compared to HCs, with positive correlations between conceptual creativity and the gray matter volume of the PCu (47). Enhanced PCu cortical surface area is also linked to morphological variations in the adult midsagittal brain. Recent work suggests that the PCu may also coordinate correlations between vivid memories and egocentric perspectives, with an increase in PCu volume being related to an increased tendency to recall egocentric episodic autobiographical memories (48). Reduced right PCu ReHo has been reported to be related to higher levels of verbal innovation capacity linked to improved flexibility, ingenuity, and fluency (49). These characteristics are common among TLE patients. One meta-analysis found PCu to be activated in response to familiarity (50). The PCu can also promote consciousness networks that exhibit selective hypometabolism in epilepsy patients (51). Here, enhanced PCu DC values in rTLE patients may thus represent a compensatory mechanism associated with the pathogenesis of this disease.
There are multiple limitations to this study. For one, patients were treated with antiepileptic drugs, which may have influenced the brain networks in these patients. However, it was not possible to exclude antiepileptic drug use from a patient care perspective. Second, this study had a limited sample size. Third, neuropsychological scale assessments for all patients were not available owing to patient reluctance to undergo these analyses.
In summary, these data suggest that rTLE patients exhibit abnormal DC values in several regions of the brain, with altered DC in the right caudate offering potential value as neuroimaging biomarker of rTLE.
Data availability statement
The original contributions presented in the study are included in the article/supplementary material, further inquiries can be directed to the corresponding authors.
Ethics statement
The studies involving human participants were reviewed and approved by the Medical Ethics Committee of the Tianyou Hospital Affiliated to Wuhan University of Science and Technology. The patients/participants provided their written informed consent to participate in this study.
Author contributions
RG: writing the manuscript. YZ, HJ, and JJ: study conceptualization, organization, and analysis outcome verification. JJ and HW: data collection and analysis. HW, HR, and SJ: manuscript preparation. RG, HR, and SJ: collect pictures and process them. All authors contributed to the article and approved the submitted version.
Funding
The investigation was supported by the grant from the Natural Science Joint Foundation of Hubei province (Nos. WJ2019H232 and WJ2019H233) and Health of Hubei Province Scientific Resarch Project (2020CFB512).
Acknowledgments
The authors thank all individuals who served as study subjects and MJEditor (www.mjeditor.com) for its linguistic assistance during the preparation of this manuscript.
Conflict of interest
The authors declare that the research was conducted in the absence of any commercial or financial relationships that could be construed as a potential conflict of interest.
Publisher’s note
All claims expressed in this article are solely those of the authors and do not necessarily represent those of their affiliated organizations, or those of the publisher, the editors and the reviewers. Any product that may be evaluated in this article, or claim that may be made by its manufacturer, is not guaranteed or endorsed by the publisher.
Footnotes
References
1. Vinti V, Dell’Isola GB, Tascini G, Mencaroni E, Di Cara G, Striano P, et al. Temporal lobe epilepsy and psychiatric comorbidity. Front Neurol. (2021) 12:775781. doi: 10.3389/fneur.2021.775781
2. Falconer MA, Serafetinides EA, Corsellis JN. Etiology and pathogenesis of temporal lobe epilepsy. Arch Neurol. (1964) 10:233–48. doi: 10.1001/archneur.1964.00460150003001
3. Shah P, Bassett DS, Wisse LE, Detre JA, Stein JM, Yushkevich PA, et al. Structural and functional asymmetry of medial temporal subregions in unilateral temporal lobe epilepsy: a 7T MRI study. Hum Brain Mapp. (2019) 40:2390–8. doi: 10.1002/hbm.24530
4. Smolensky IV, Zubareva OE, Kalemenev SV, Lavrentyeva VV, Dyomina AV, Karepanov AA, et al. Impairments in cognitive functions and emotional and social behaviors in a rat lithium-pilocarpine model of temporal lobe epilepsy. Behav Brain Res. (2019) 372:112044. doi: 10.1016/j.bbr.2019.112044
5. Galovic M, van Dooren VQ, Postma TS, Vos SB, Caciagli L, Borzì G, et al. Progressive cortical thinning in patients with focal epilepsy. JAMA Neurol. (2019) 76:1230–9. doi: 10.1001/jamaneurol.2019.1708
6. Amin U, Benbadis SR. The role of EEG in the erroneous diagnosis of epilepsy. J Clin Neurophysiol. (2019) 36:294–7. doi: 10.1097/WNP.0000000000000572
7. Gao Y, Zheng J, Li Y, Guo D, Wang M, Cui X, et al. Abnormal default-mode network homogeneity in patients with temporal lobe epilepsy. Medicine. (2018) 97:e11239. doi: 10.1097/MD.0000000000011239
8. Gao Y, Zheng J, Li Y, Guo D, Wang M, Cui X, et al. Decreased functional connectivity and structural deficit in alertness network with right-sided temporal lobe epilepsy. Medicine. (2018) 97:e0134. doi: 10.1097/MD.0000000000010134
9. Gao Y, Xiong Z, Wang X, Ren H, Liu R, Bai B, et al. Abnormal degree centrality as a potential imaging biomarker for right temporal lobe epilepsy: a resting-state functional magnetic resonance imaging study and support vector machine analysis. Neuroscience. (2022) 487:198–206. doi: 10.1016/j.neuroscience.2022.02.004
10. Sarica C, Yamamoto K, Loh A, Elias GJ, Boutet A, Madhavan R, et al. Blood oxygen level-dependent (BOLD) response patterns with thalamic deep brain stimulation in patients with medically refractory epilepsy. Epilepsy Behav. (2021) 122:108153. doi: 10.1016/j.yebeh.2021.108153
11. Qin Y, Jiang S, Zhang Q, Dong L, Jia X, He H, et al. BOLD-fMRI activity informed by network variation of scalp EEG in juvenile myoclonic epilepsy. NeuroImage Clin. (2019) 22:101759. doi: 10.1016/j.nicl.2019.101759
12. Tang Y, Choi JY, Alexopoulos A, Murakami H, Daifu-Kobayashi M, Zhou Q, et al. Individual localization value of resting-state fMRI in epilepsy presurgical evaluation: a combined study with stereo-EEG. Clin Neurophysiol. (2021) 132:3197–206. doi: 10.1016/j.clinph.2021.07.028
13. Gonen OM, Moffat BA, Desmond PM, Lui E, Kwan P, O’Brien TJ. Seven-tesla quantitative magnetic resonance spectroscopy of glutamate, γ-aminobutyric acid, and glutathione in the posterior cingulate cortex/precuneus in patients with epilepsy. Epilepsia. (2020) 61:2785–94. doi: 10.1111/epi.16731
14. Zhou S, Xiong P, Ren H, Tan W, Yan Y, Gao Y. Aberrant dorsal attention network homogeneity in patients with right temporal lobe epilepsy. Epilepsy Behav. (2020) 111:107278. doi: 10.1016/j.yebeh.2020.107278
15. Zhu J, Xu C, Zhang X, Qiao L, Wang X, Zhang X, et al. Altered amplitude of low-frequency fluctuations and regional homogeneity in drug-resistant epilepsy patients with vagal nerve stimulators under different current intensity. CNS Neurosci Ther. (2021) 27:320–9. doi: 10.1111/cns.13449
16. Wang J, Li Y, Wang Y, Huang W. Multimodal data and machine learning for detecting specific biomarkers in pediatric epilepsy patients with generalized tonic-clonic seizures. Front Neurol. (2018) 9:1038. doi: 10.3389/fneur.2018.01038
17. Reddy SD, Younus I, Sridhar V, Reddy DS. Neuroimaging biomarkers of experimental epileptogenesis and refractory epilepsy. Int J Mol Sci. (2019) 20:220. doi: 10.3390/ijms20010220
18. Grubb J, Lopez D, Mohan B, Matta J. Network centrality for the identification of biomarkers in respondent-driven sampling datasets. PLoS One. (2021) 16:e0256601. doi: 10.1371/journal.pone.0256601
19. Chen C, Wang H-L, Wu S-H, Huang H, Zou J-L, Chen J, et al. Abnormal degree centrality of bilateral putamen and left superior frontal gyrus in schizophrenia with auditory hallucinations: a resting-state functional magnetic resonance imaging study. Chine Med J. (2015) 128:3178–84. doi: 10.4103/0366-6999.170269
20. Li S, Chen Y, Duan G, Pang Y, Liu H, Wei Y, et al. Altered Brain Network Degree Centrality in Major Depressive Disorder via Electro-Acupuncture Stimulation at Baihui (GV20). Nanning: Reaearch Square. (2021). doi: 10.21203/rs.3.rs-525264/v1
21. Li H, Jia X, Li Y, Jia X, Yang Q. Aberrant amplitude of low-frequency fluctuation and degree centrality within the default mode network in patients with vascular mild cognitive impairment. Brain Sci. (2021) 11:1534. doi: 10.3390/brainsci11111534
22. Wang X, Hu T, Yang Q, Jiao D, Yan Y, Liu L. Graph-theory based degree centrality combined with machine learning algorithms can predict response to treatment with antiepileptic medications in children with epilepsy. J Clin Neurosci. (2021) 91:276–82. doi: 10.1016/j.jocn.2021.07.016
23. Hahamy A, Calhoun V, Pearlson G, Harel M, Stern N, Attar F, et al. Save the global: global signal connectivity as a tool for studying clinical populations with functional magnetic resonance imaging. Brain Connect. (2014) 4:395–403. doi: 10.1089/brain.2014.0244
24. Wanderley Espinola C, Gomes JC, Mônica Silva Pereira J, dos Santos WP. Detection of major depressive disorder, bipolar disorder, schizophrenia and generalized anxiety disorder using vocal acoustic analysis and machine learning: an exploratory study. Res Biomed Eng. (2022):1–17. doi: 10.1007/s42600-022-00222-2
25. Shor O, Glik A, Yaniv-Rosenfeld A, Valevski A, Weizman A, Khrennikov A, et al. EEG p-adic quantum potential accurately identifies depression, schizophrenia and cognitive decline. PLoS One. (2021) 16:e0255529. doi: 10.1371/journal.pone.0255529
26. Lin C-H, Su H, Hung C-C, Lane H-Y, Shiea J. Characterization of potential protein biomarkers for major depressive disorder using matrix-assisted laser desorption ionization/time-of-flight mass spectrometry. Molecules. (2021) 26:4457. doi: 10.3390/molecules26154457
27. Obuchowski NA, Bullen JA. Receiver operating characteristic (ROC) curves: review of methods with applications in diagnostic medicine. Phys Med Biol. (2018) 63:07TR01. doi: 10.1088/1361-6560/aab4b1
28. Doi T, Fan Y, Gold JI, Ding L. The caudate nucleus contributes causally to decisions that balance reward and uncertain visual information. eLife. (2020) 9:e56694. doi: 10.7554/eLife.56694
29. Bick SK, Patel SR, Katnani HA, Peled N, Widge A, Cash SS, et al. Caudate stimulation enhances learning. Brain. (2019) 142:2930–7. doi: 10.1093/brain/awz254
30. Driscoll ME, Bollu PC, Tadi P. Neuroanatomy, nucleus caudate. StatPearls [Internet], ed. M. E. Driscoll (Treasure Island, FL: StatPearls Publishing) (2021).
31. Jiang Y, Nie L. ML of Striatum rs-fMRI Features Predicts TLE Diagnosis. Nanning: Reaearch Square. (2021). doi: 10.21203/rs.3.rs-638717/v1
32. Du T, Chen Y, Shi L, Liu D, Liu Y, Yuan T, et al. Deep brain stimulation of the anterior nuclei of the thalamus relieves basal ganglia dysfunction in monkeys with temporal lobe epilepsy. CNS Neurosci Ther. (2021) 27:341–51. doi: 10.1111/cns.13462
33. Faglioni P. The frontal lobe. In: L Pizzamiglio, G Denes editors. Handbook of Clinical and Experimental Neuropsychology. London: Psychology Press (2020). p. 525–70.
34. Bonet CNR, Hwang G, Hermann B, Struck AF, Cook CJ, Nair VA, et al. Neuroticism in temporal lobe epilepsy is associated with altered limbic-frontal lobe resting-state functional connectivity. Epilepsy Behav. (2020) 110:107172. doi: 10.1016/j.yebeh.2020.107172
35. Reyes A, Kaestner E, Ferguson L, Jones JE, Seidenberg M, Barr WB, et al. Cognitive phenotypes in temporal lobe epilepsy utilizing data-and clinically driven approaches: moving toward a new taxonomy. Epilepsia. (2020) 61:1211–20. doi: 10.1111/epi.16528
36. Buck S, Bastos F, Baldeweg T, Vargha-Khadem F. A functional MRI paradigm suitable for language and memory mapping in pediatric temporal lobe epilepsy. Front Neurol. (2020) 10:1384. doi: 10.3389/fneur.2019.01384
37. Yaranagula SD, Asranna A, Nagappa M, Nayak CS, Pratyusha P, Mundlamuri RC, et al. Sleep profile and polysomnography in patients with drug-resistant temporal lobe epilepsy (TLE) due to hippocampal sclerosis (HS) and the effect of epilepsy surgery on sleep-a prospective cohort study. Sleep Med. (2021) 80:176–83. doi: 10.1016/j.sleep.2020.12.016
38. Jain P, Tomlinson G, Snead C, Sander B, Widjaja E. Systematic review and network meta-analysis of resective surgery for mesial temporal lobe epilepsy. J Neurol Neurosurg Psychiatry. (2018) 89:1138–44. doi: 10.1136/jnnp-2017-317783
39. Buxbaum LJ, Randerath J. Limb apraxia and the left parietal lobe. Handb Clin Neurol. (2018) 151:349–63. doi: 10.1016/B978-0-444-63622-5.00017-6
40. Coslett HB, Schwartz MF. The parietal lobe and language. Handb Clin Neurol. (2018) 151:365–75. doi: 10.1016/B978-0-444-63622-5.00018-8
41. Rizzolatti G, Rozzi S. The mirror mechanism in the parietal lobe. Handb Clin Neurol. (2018) 151:555–73. doi: 10.1016/B978-0-444-63622-5.00028-0
42. Husbani MAR. The default mode network in patient with temporal lobe epilepsy (TLE) : a resting state fMRI study. Asian J Med Biomed. (2020) 4:1–8.
43. Shuhada J, Husbani M, Hamid A. The default mode network in patient with temporal lobe epilepsy (TLE) : a resting state fMRI study. Asian J Med Biomed. (2020) 4:23–30.
44. Howell ST, Grushina A, Holzner F, Brugger J. Thermal scanning probe lithography—a review. Microsyst Nanoeng. (2020) 6:1–24. doi: 10.1038/s41378-019-0124-8
45. Cavanna AE, Trimble MR. The precuneus: a review of its functional anatomy and behavioural correlates. Brain. (2006) 129:564–83. doi: 10.1093/brain/awl004
46. Zhu X, He Z, Luo C, Qiu X, He S, Peng A, et al. Altered spontaneous brain activity in MRI-negative refractory temporal lobe epilepsy patients with major depressive disorder: a resting-state fMRI study. J Neurol Sci. (2018) 386:29–35. doi: 10.1016/j.jns.2018.01.010
47. Shuhada J, Husbani M, Hamid A, Muhammad A, editors. Evaluation of default mode network in temporal lobe epilepsy patients and healthy subjects: a preliminary result. Proceedings of the IOP Conference Series: Materials Science and Engineering. Bristol: IOP Publishing (2020).
48. Oyegbile TO, VanMeter JW, Motamedi GK, Bell WL, Gaillard WD, Hermann BP. Default mode network deactivation in pediatric temporal lobe epilepsy: relationship to a working memory task and executive function tests. Epilepsy Behav. (2019) 94:124–30. doi: 10.1016/j.yebeh.2019.02.031
49. Liu W, Yue Q, Wu X, Gong Q, Zhou D. Abnormal blood oxygen level-dependent fluctuations and remote connectivity in sleep-related hypermotor epilepsy. Acta Neurol Scand. (2021) 143:514–20. doi: 10.1111/ane.13379
50. Dharan AL, Bowden SC, Lai A, Peterson AD, Cheung MW-L, Woldman W, et al. Resting-state functional connectivity in the idiopathic generalized epilepsies: a systematic review and meta-analysis of EEG and MEG studies. Epilepsy Behav. (2021) 124:108336. doi: 10.1016/j.yebeh.2021.108336
Keywords: temporal lobe epilepsy, degree centrality, magnetic resonance imaging, receiver operating characteristic, caudate
Citation: Guo R, Zhao Y, Jin H, Jian J, Wang H, Jin S and Ren H (2022) Abnormal hubs in global network as neuroimaging biomarker in right temporal lobe epilepsy at rest. Front. Psychiatry 13:981728. doi: 10.3389/fpsyt.2022.981728
Received: 29 June 2022; Accepted: 08 July 2022;
Published: 27 July 2022.
Edited by:
Qinji Su, Guangxi Medical University, ChinaReviewed by:
Xiaobing Jiang, Huazhong University of Science and Technology, ChinaYunhua Zhang, Hubei Provincial Hospital of Traditional Chinese Medicine, China
Copyright © 2022 Guo, Zhao, Jin, Jian, Wang, Jin and Ren. This is an open-access article distributed under the terms of the Creative Commons Attribution License (CC BY). The use, distribution or reproduction in other forums is permitted, provided the original author(s) and the copyright owner(s) are credited and that the original publication in this journal is cited, in accordance with accepted academic practice. No use, distribution or reproduction is permitted which does not comply with these terms.
*Correspondence: Haibo Wang, aGFpX2JvX3dhbmdAZm94bWFpbC5jb20=; Shengxi Jin, anN4MjQ1NTg1Mjk2NkBxcS5jb20=; Hongwei Ren, MTQyMTQ5NDlAcXEuY29t