- 1Wuhan Mental Health Center, Wuhan, China
- 2Affiliated Wuhan Mental Health Center, Tongji Medical College of Huazhong University of Science and Technology, Wuhan, China
- 3Research Center for Psychological and Health Sciences, China University of Geosciences, Wuhan, China
Premenstrual syndrome (PMS) is a group of psychological, physical, and behavioral symptoms that recur with the menstrual cycle, usually occurring a few days before menstruation and ceasing with the onset of menstruation. Premenstrual dysphoric disorder (PMDD) is a severe form of PMS that has been included in a subcategory of depression in the Diagnostic and Statistical Manual of Mental Disorders (DSM-V) according to the latest diagnostic criteria. Patients usually present with mild to moderate emotional and physical symptoms that affect their routine work, social activities, and family lives. The pathogenesis of PMDD remains unclear, and some researchers believe that it is related to fluctuations in ovarian hormone levels. However, the details of the interrelationships and regulating effects between ovarian hormones, symptoms, and the brain need to be more comprehensively determined. Recent studies have revealed some novel findings on PMS and PMDD based on brain morphology, function, and metabolism. Additionally, multiple studies have suggested that PMS and PMDD are closely related to brain structural and functional variations in certain core temporal lobe regions, such as the amygdala and hippocampus. We summarized neuroimaging studies of PMS and PMDD related to the temporal lobe by retrospectively reviewing relevant literature over the past decade. This review contributes to further clarifying the significant role of the temporal lobe in PMS and PMDD and understanding the neurochemical links between hormones, symptoms, and the brain.
Introduction
Estrogen and progesterone have far-reaching effects on females throughout their lifespan (1). Many female psychiatric disorders are related to reproductive events, such as pubertal depression, premenstrual dysphoric disorder (PMDD), and postpartum depression (2, 3). Approximately 85% of females have experienced mild premenstrual symptoms at least once (4), and 57% of women of childbearing age experienced mild “anger/irritability” or “tearfulness/mood swings” during the premenstrual period (5). About 30–40% of women suffer from premenstrual syndrome (PMS) (6, 7), and 3–8% of females suffer from PMDD (8). PMS incidence is approximately 21.1% in China, and the incidence of PMDD is 2.1% (9). The symptoms range across mental, physical, and behavioral dimensions (10), and difficulty in regulating emotion is the clinical manifestation (4, 6, 11, 12). Remarkably, PMDD is a relatively stable and disabling disease that usually impacts patients' occupational activities, interpersonal relationships, social life, and family life (6, 13) and is responsible for 14.5 million disability-adjusted life years in the United States (8). As a disorder with familial risk, it displays 44 to 56% heritability (14, 15), and the global incidence and associated suicide rate have been continuously increasing (16, 17). In 2013, the American Psychiatric Association classified PMDD as a subcategory of depressive disorders in the Diagnostic and Statistical Manual of Mental Disorders (DSM-V) (4, 18). Currently, diagnosis mainly depends on self-rating scales such as the Daily Record of Severity of Problems. Nevertheless, this diagnostic model is also prone to misdiagnosis of patients (11). Therapeutic modalities include pharmacological, non-pharmacological, and surgical approaches. Usually, selective serotonin reuptake inhibitors are the first-line treatment for PMDD, and ovariectomy is the recommended plan following failure of conservative treatments (6, 10).
In the past three decades, the application of modern science and technology to investigate PMS and PMDD has lagged significantly behind that of other diseases. Today, while neuroimaging research is starting to be applied to these disorders, the neuropathological mechanisms are unclear (11). Even so, mounting evidence has confirmed that certain temporal lobe regions, such as the amygdala and hippocampus, are the most vulnerable regions in PMS and PMDD. This indicates that the temporal lobes have a prominent role in the pathogenesis of PMS and PMDD (19). Furthermore, relevant findings are being continuously updated. Hence, it is indispensable to conduct retrospective analyses and collate results from the most up-to-date literature. Aiming to provide the latest reference for a more comprehensive and in-depth understanding of PMS and PMDD, the present mini-review summarized relevant studies from recent years. These findings are summarized in Table 1.
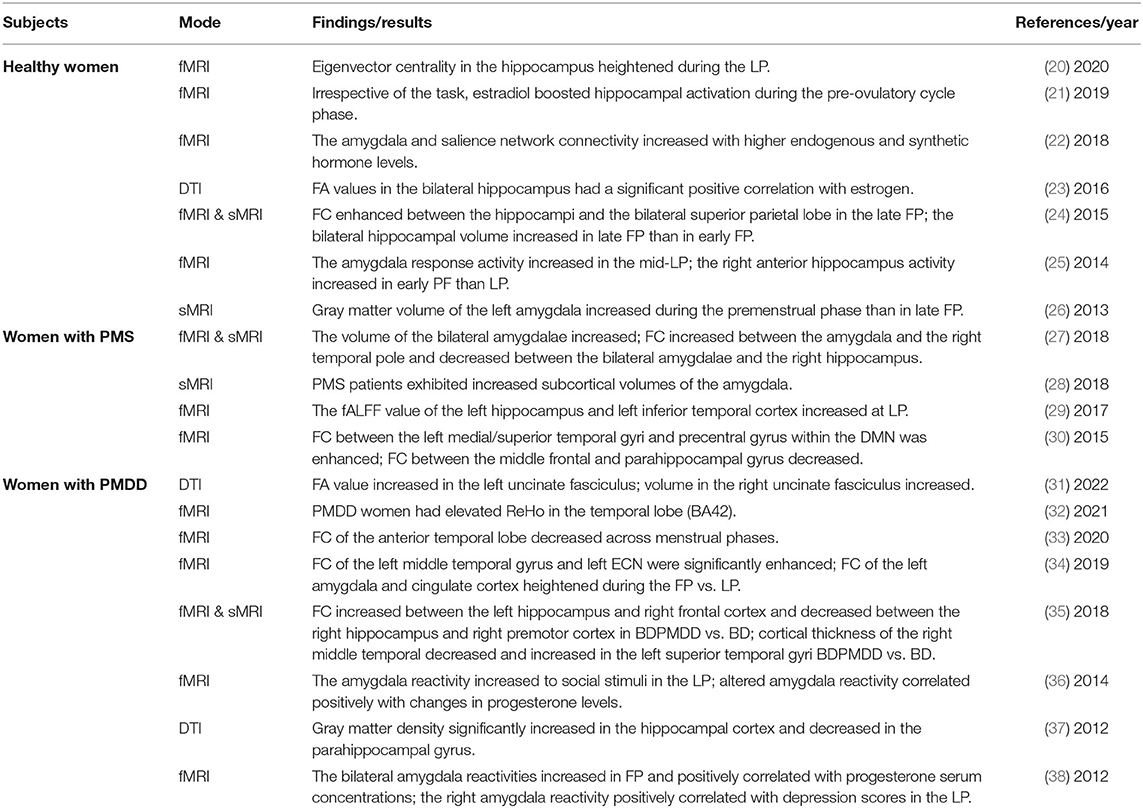
Table 1. Summary of findings of the neuroimaging studies in the menstrual cycle, PMS, or PMDD associated with the temporal lobe.
Article types
Mini-Review.
Manuscript Formatting
Critical Regions of the Temporal Lobe in PMS and PMDD
Amygdala
It is well-known that the medial temporal lobe (MTL) includes the amygdala, hippocampal region, and entorhinal cortex and is responsible for memory and emotion processing (39). Extensive research has shown that the amygdala exhibits variation in structural and functional tendencies during the menstrual cycle in healthy women (22, 26, 40, 41). In other words, ovarian hormones can trigger the remodeling of the amygdala. For example, structural magnetic resonance imaging (sMRI) analyses demonstrated an increase in gray matter volume of the amygdala from the late luteal phase (LP) to the late follicular phase (FP) in healthy subjects (26). In addition, menstrual cycle-related variations in gray matter volume in the amygdala were associated with the severity of negative affect (19). Furthermore, functional magnetic resonance imaging (fMRI) research showed that progesterone selectively increased amygdala reactivity and regulated the functional coupling of the amygdala with some distant brain regions (22, 40, 41). These alterations may involve regulatory feedback mechanisms related to the menstrual cycle. In contrast, morphological measurements in PMS patients, compared with healthy individuals, indicated significantly enlarged subcortical volumes in the bilateral amygdala (27, 28). Moreover, several studies have verified that enhanced functional activity of the amygdala correlated with emotion dysregulation such as degree of anxiety and depression in LP (12, 38). In terms of functional connectivity (FC), FC between the right amygdala and left anterior cingulate cortex and between the right precentral gyrus and left medial prefrontal cortex exhibited positive correlations with the severity of premenstrual symptoms in PMS females (27). In PMDD women, FC between the left amygdala and cingulate cortex and between the right amygdala and the middle frontal gyrus was markedly strengthened in the FP compared with the LP (34).
The amygdala is located deep in the MTL and plays an essential role in the limbic system and central emotional circuitry (27). Its function involves memory, arousal, alertness, and attention, especially in emotion processing and pain (42–44). Previous findings have shown that anxiety (45), depression (46), and acute and chronic pain (44, 47) could induce abnormalities in the amygdala. Furthermore, the structural and functional alterations of the amygdala in PMS and PMDD are closely associated with emotional regulation (33). Sufficient research evidence suggests that fluctuations in sex hormones, especially progesterone, may be a pivotal factor in brain structural remodeling (2, 38). Given that emotional instability is a core symptom of PMS and PMDD and the amygdala plays a vital role in emotional processing, it is not difficult to explain why neuroimaging abnormalities of the amygdala are so prevalent in patients. The critical question is, how exactly do sex hormones affect the brain? Currently, researchers generally agree that damage to the amygdala leads to aberrations in top-down inhibition processes in the limbic system, which is the core pathological mechanism of PMDD (12, 19, 38, 48). Heightened amygdala responsiveness to social (36) and negative emotional (49) stimuli in PMDD patients confirms the breakdown of another aspect of this inhibitory regulation. Concurrent research on hormones, symptoms, and function surprisingly found that concentrations of sex hormones significantly influence the severity of symptoms and brain activity (36, 38, 48). These findings collectively support the notion that this disruption in top-down inhibition may be the key link in the pathogenesis of PMDD. Although this is the most mainstream academic view on PMDD, there is still some controversy. For instance, Pessoa proposed that the key to emotion regulation is not the limbic system but interactions spanning the entire neuroaxis of a network model of the emotional brain. This network mainly constitutes a functional integration system and emphasizes that abnormal interactions among multiple brain regions lead to PMDD rather than giving the amygdala dominant status in the limbic system (43).
Hippocampus and Parahippocampal Gyrus
More recent evidence has shown that the hippocampus is a rich-club hub and the most sensitive region to menstrual cycle changes (50). In healthy females, the hippocampus displayed more intense reactivity during cognitive and affective processes during FP (21, 24), and the responses of the hippocampus were strengthened in the late FP and mid-LP compared with the early FP and late LP during an affective task (25, 51). At the structural level, gray matter volume in the bilateral hippocampi increased in the late FP compared with the early FP (24), and an enlarged volume from LP to FP was positively related to improvements in verbal memory performance (52). Moreover, serum concentrations of estradiol positively correlated with the volume size and fractional anisotropy (FA) values in the hippocampus (23). Overall, it can clearly be seen that the level of estradiol is positively correlated with functional activity (21, 51), volume (24), and white matter integrity (23) of the hippocampus. In contrast, patients with PMDD presented more significantly increases in gray matter density in the hippocampus and decreases in gray matter density in the parahippocampal gyrus than healthy women (37). During the LP, functional activities of the left hippocampus and left inferior temporal cortex were clearly enhanced in PMS (29). Additionally, FC between the right parahippocampal gyrus and middle frontal gyrus in PMS females was weakened (30). It is worth noting that patients with bipolar disorder comorbid with PMDD (BDPMDD) also exhibited diverse abnormalities in FC, such as an enhancement between the left hippocampus and left inferior temporal cortex and a decrease between the right hippocampus and right premotor cortex (35). To date, numerous human and animal studies have confirmed that estrogen has the potential to modify the structure, metabolism, and function of the hippocampus.
How do sex hormones act on the hippocampus? These actions are mainly due to the neurotrophic influence of estradiol (53) and the synaptic remodeling and energy homeostasis effects of progesterone (54). Both estrogen-dependent synaptic remodeling (50) and progesterone-dependent enhancements in synaptic density (54) have been observed in the hippocampus. Indeed, ovarian gonadal hormones can pass through the blood-brain barrier and subsequently affect mood and behavioral patterns by regulating neural activity (2). Notably, sex hormones can profoundly affect the hypothalamus-pituitary-adrenal (HPA) axis and neurotransmitters such as serotonin, dopaminergic, and γ-aminobutyric acid (GABA) (55). These substances are all involved in affective and cognitive processes and in the pathogenesis of depressive disorders (55–57). A rat model of premenstrual depression found that GABA and allopregnanolone expression levels decreased in the hippocampus and that depressive-like symptoms disappeared after ovariectomy and relapsed after hormone priming therapy (58). These findings are in alignment with the theory that neuronal activity in patients with depressive symptoms is being influenced by GABA-mediated inhibition.
Another key question is why the amygdala and hippocampal region are the most susceptible regions in PMS and PMDD. In brief, the amygdala and hippocampus are potential targets of sex hormone effects (50). There is an extensive distribution of estradiol and progesterone receptors in the limbic system, and these receptors are the most densely concentrated in the neurons and glial cells of the amygdala and hippocampus (50, 59). Therefore, ovarian hormones and neurotransmitters and their receptors are crucial factors in inducing structural and functional abnormalities in the amygdala and hippocampal region and subsequently triggering symptoms of PMS and PMDD. As the most vulnerable nodes, the amygdala and hippocampus are expected to be specific neuroimaging targets to assist in diagnosing and treating PMS and PMDD. For instance, it may be that women who do not respond to conservative therapy and refuse hysterectomy and oophorectomy may respond to transcranial magnetic stimulation of these regions, which may alleviate or eradicate symptoms.
Other Regions of the Temporal Lobe
In addition to the MTL, other temporal lobe regions are also relevant in PMS and PMDD. Fractional amplitude of low-frequency fluctuation (fALFF) values in the left inferior temporal cortex and left hippocampus were higher in PMS females than in healthy individuals (29). Regional homogeneity (ReHo) in the left inferior temporal cortex was more intense during the LP in PMS females than in healthy women (60). With exposure to emotional stimuli, functional activity in the temporal lobe in PMDD patients was activated to a greater degree than in controls (32). Notably, increased connectivity between the left medial temporal gyrus (MTG) and precentral gyrus negatively correlated with the severity of depression in PMS (30). Furthermore, PMDD patients exhibited hyperconnectivity between the middle temporal gyrus and right amygdala in the FP compared with level of connectivity in the LP (34). Nonetheless, another study observed different manifestations: reduced cortical connectivity across the whole brain, especially within the temporal lobe, and mediation analysis further indicated that 57% of those connections were related to emotional and behavioral symptoms in PMDD (33). The above findings unanimously indicated that the abnormal connectome of the temporal gyrus might mediate deficits in emotion regulation in PMS and PMDD.
Additionally, other evidence suggests that the fusiform gyrus also changes with the menstrual cycle in healthy women. An fMRI study reported abnormal functional activity in the amygdala, fusiform gyrus, inferior temporal gyrus, and middle temporal gyrus (61). Progesterone has been shown to trigger decreases in neural activity in the amygdala and fusiform gyrus, whereas it increases the hippocampal response (62). Moreover, in an emotional recognition task, hypoconnectivity between the fusiform gyrus and amygdala was directly linked to progesterone in healthy subjects (41). However, the existing studies on PMS and PMDD rarely include the fusiform gyrus, and thus, specific neuroimaging characterizations are lacking.
Temporal Lobe-Related Neural Circuitry in PMS and PMDD
Regions with different properties and locations in the brain form neural circuits through various forms of complex connections. When a particular node is abnormal, other brain regions within the circuit are likely to be disrupted, thereby affecting the overall function of the circuit. In fact, the amygdala and hippocampus occupy core positions in several pivotal neural circuits, such as the hippocampus-amygdala, hippocampus-entorhinal cortex, hippocampus-prefrontal circuit, amygdala-prefrontal cortex, and amygdala-thalamus circuits (63).
Of note, significant variations in several circuits assessed with neuroimaging have also been found in PMS and PMDD. Taking the hippocampus-amygdala circuit as an example, functional activity in this circuit basically coincides with fluctuating levels of ovarian gonadal hormones across menstrual cycle phases in healthy females (61). Namely, the hippocampus-amygdala circuit was positively associated with estradiol levels. As previously described, progesterone and its derivatives regulate emotion and memory processing by modulating the activity of the amygdala, which in turn affects the hippocampus and fusiform gyrus, which are also related to this neural circuit (62). PMS females exhibited weakened FC between the amygdala and right hippocampus and increased FC between the amygdala and right temporal lobe compared with healthy women (27). The above statement is affiliated with the functioning of the hippocampus-amygdala circuit. Particularly, in addition to the amygdala-hippocampus circuit, previous results in PMS and PMDD have focused on the amygdala-prefrontal cortex circuit. Under physiological conditions, the prefrontal lobe maintains bottom-up inhibitory effects on the amygdala and keeps the amygdala in a highly inhibited state, which is vital for maintaining normal emotional behavior. When exposed to estrogen and progestin, inhibitory effects of the prefrontal cortex on the amygdala are weakened, leading to overactivation of the amygdala, which can lead to premenstrual symptoms (12, 19, 38, 48). It is noteworthy that a brain structure study found that the central circuitry of emotion, which includes the amygdala, lateral prefrontal cortex, and orbitofrontal cortex, participated in the pathogenesis of PMS (28). By its nature, abnormal activation of the endocrine system in women and its effects on cognitive and affective circuits may underlie the pathogenesis of PMS and PMDD (33). Admittedly, existing research on neural circuits of PMS and PMDD is insufficient, and a more meticulous analysis of the relevant neural circuits is urgently needed.
Temporal Lobe-Related Brain Networks in PMS and PMDD
Besides neural circuits, brain regions also constitute neural networks—the execution and maintenance of normal function in bodies rely on homeostasis within and coordination between these networks (64, 65). Previous neuroimaging studies have investigated features of several brain networks in healthy women during the menstrual cycle, but few have focused on PMS and PMDD (33). Earlier findings demonstrated that estrogen and progesterone could mediate sensitivity of mood, cognition, and impairments in brain networks (20). Recently, a refined study gathered MRI data from a woman for 30 consecutive days and repeated it a year later: the recombination and rhythm of the human brain network involved in the menstrual cycle were discovered, and it was confirmed that estradiol plays a crucial role in the brain network (66). Regarding the default mode network (DMN), multiple FC abnormalities, including connections with the parahippocampal gyrus, medial superior temporal gyrus, and MTG, were found in women with PMS (30). In executive control networks (ECN), the connectivity between the left ECN and left middle temporal gyrus was significantly enhanced and closely correlated with emotion regulation in patients with PMDD (34). This finding indicated that the intrinsic network dynamics in the left ECN differ between PMDD patients and healthy subjects. Similarly, a network dynamics study found that fluctuations in ovarian hormone levels during the menstrual cycle affect core brain regions, which may profoundly impact the dynamics of the whole brain network (67). More importantly, a comprehensive topological rearrangement took place in brain networks in healthy women under the influence of sex hormones (31). Topological changes were also present in PMS. Comparing topological parameters in patients from mid-FP to late-LP and healthy subjects demonstrated reduced segregation and enhanced integration among functional brain networks (33). In particular, the specific network for emotional regulation consisting of the amygdala, hippocampus, thalamus, and ventral striatum was closely related to the evaluation and expression of emotion (68). This discovery provided further clues that revealed interactive effects between emotions, brain networks, and sex hormone fluctuations. Moreover, structural research has verified that the fiber tracts responsible for connecting the temporal lobe showed significant microstructural changes, and the FA value in the superior longitudinal fasciculus positively correlated with the severity of premenstrual symptoms in those with PMDD (69). Conversely, unlike other psychiatric disorders, PMDD patients had higher structural integrity of the white matter. This opposite performance could be explained by the neuroprotection of sex hormones positively shaping brain structure in hormone-dependent diseases (69).
Overall, insight into the susceptibility and plasticity of network dynamics and topology is necessary to comprehensively explore the neuropathological mechanisms underlying PMS and PMDD (50, 66). However, existing studies of brain network dynamics seriously lack convincing evidence, especially regarding the structural aspects. On the bright side, existing findings evaluating brain networks have mainly converged on temporal lobe-related regions such as the amygdala and hippocampus, which coincided with research on independent brain regions. All these mechanisms are illustrated in Figure 1.
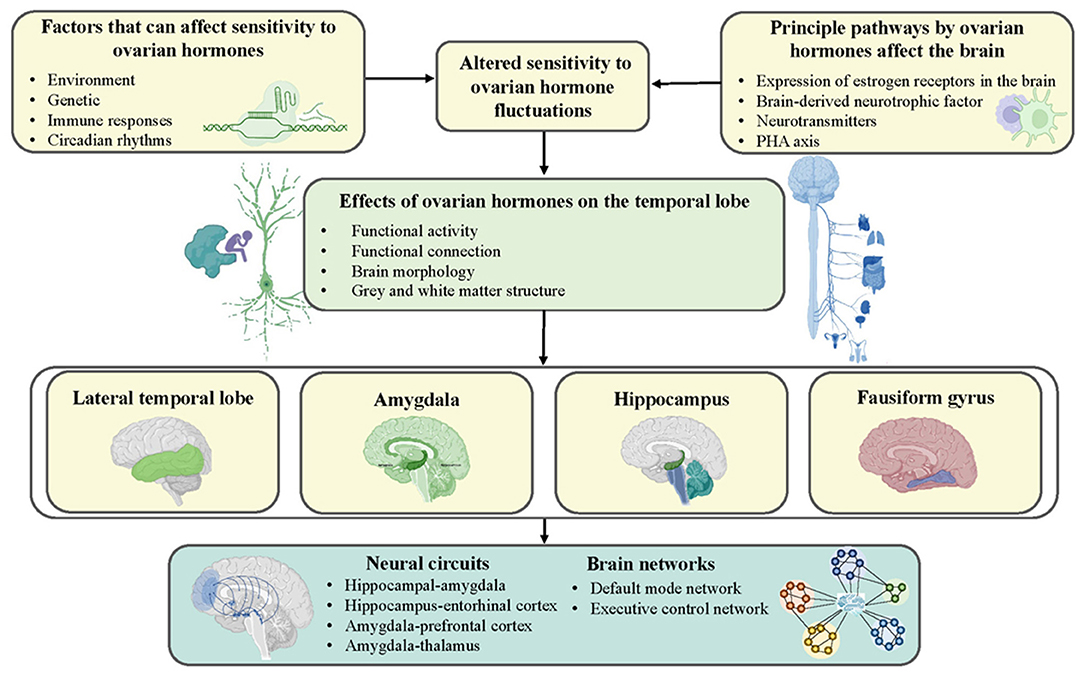
Figure 1. Schematic representation of possible mechanisms by which ovarian hormones contribute to the development of PMS and PMDD. Genetics, environment, immune response, and circadian rhythms increase women's sensitivity to ovarian hormones and shape the temporal lobe by affecting neurotransmitters and hormonal systems.
Conclusion
In general, harmonious covariations between the metabolism, structure, and function of the brain and ovarian gonadal hormone levels during the menstrual cycle exist in healthy females. In contrast, the diverse array of inappropriate manifestations and remodeling of metabolism, structure, and function have occurred in multiple regions in PMS and PMDD women. However, there was a high level of heterogeneity in the current results due to differences in MRI scanning equipment and sequence parameters, analysis methods, and the menstrual phase in which women were scanned. Nevertheless, the existing neuroimaging findings showed high consistency regarding the involvement of temporal lobe regions, such as the amygdala and hippocampus, which confirms the prominent role of the temporal lobe in PMS and PMDD. Furthermore, genetics and environmental factors, abnormal immune responses, and changes in circadian rhythms trigger increased sensitivity to sex hormones, which in turn affect other neurotransmitters and hormonal systems (e.g., brain-derived neurotrophic factor), thereby influencing the temporal lobe and further affecting mental health and behavior (70–72). Therefore, in near future, it is essential to perform research at multiple time points using multimodal neuroimaging techniques, and more attention is warranted on biomarkers, specific neural circuits, structural-functional coupling, vulnerability and controllability of network dynamics, and comorbidity with other psychiatric diseases. Similarly, specific distinctions of menstrual cycle stages and more detailed analyses of brain subregions are needed. If these types of studies can be performed, they will provide a valid foundation of evidence to guide the individualized identification, classification, and treatment of PMS and PMDD.
Author Contributions
All authors contributed to the article and approved the submitted version.
Funding
This work was supported by the Health Commission of Wuhan scientific research project (WX19D62 to LL).
Conflict of Interest
The authors declare that the research was conducted in the absence of any commercial or financial relationships that could be construed as a potential conflict of interest.
Publisher's Note
All claims expressed in this article are solely those of the authors and do not necessarily represent those of their affiliated organizations, or those of the publisher, the editors and the reviewers. Any product that may be evaluated in this article, or claim that may be made by its manufacturer, is not guaranteed or endorsed by the publisher.
Acknowledgments
The authors thank all females affected by PMS or PMDD who make our research meaningful.
References
1. Bakker J. The sexual differentiation of the human brain: role of sex hormones vs. sex chromosomes. Curr Top Behav Neurosci. (2019) 43:45–67. doi: 10.1007/7854_2018_70
2. Stickel S, Wagels L, Wudarczyk O, Jaffee S, Habel U, Schneider F, et al. Neural correlates of depression in women across the reproductive lifespan—an fmri review. J Affect Disord. (2019) 246:556–70. doi: 10.1016/j.jad.2018.12.133
3. Slyepchenko A, Minuzzi L, Frey BN. Comorbid premenstrual dysphoric disorder and bipolar disorder: a review. Front Psychiatry. (2021) 12:719241. doi: 10.3389/fpsyt.2021.719241
4. Diagnostic and Statistical Manual of Mental Disorders. 5th Ed. Arlington, VA: American Psychiatric Association (2013).
5. Tschudin S, Bertea PC, Zemp E. Prevalence and predictors of premenstrual syndrome and premenstrual dysphoric disorder in a population-based sample. Arch Womens Ment Health. (2010) 13:485–94. doi: 10.1007/s00737-010-0165-3
6. Ryu A, Kim TH. Premenstrual syndrome: a mini review. Maturitas. (2015) 82:436–40. doi: 10.1016/j.maturitas.2015.08.010
7. Tolossa FW, Bekele ML. Prevalence, impacts and medical managements of premenstrual syndrome among female students: cross-sectional study in college of health sciences, Mekelle university, Mekelle, Northern Ethiopia. BMC Womens Health. (2014) 14:52. doi: 10.1186/1472-6874-14-52
8. Halbreich U, Borenstein J, Pearlstein T, Kahn LS. The prevalence, impairment, impact, and burden of Premenstrual dysphoric disorder (Pms/Pmdd). Psychoneuroendocrinology. (2003) 28:1–23. doi: 10.1016/S0306-4530(03)00098-2
9. Qiao M, Zhang H, Liu H, Luo S, Wang T, Zhang J, et al. Prevalence of premenstrual syndrome and premenstrual dysphoric disorder in a population-based sample in China. Eur J Obstet Gynecol Reprod Biol. (2012) 162:83–6. doi: 10.1016/j.ejogrb.2012.01.017
10. Lanza di Scalea T, Pearlstein T. Premenstrual dysphoric disorder. Med Clin North Am. (2019) 103:613–28. doi: 10.1016/j.mcna.2019.02.007
11. Epperson CN, Steiner M, Hartlage SA, Eriksson E, Schmidt PJ, Jones I, et al. Premenstrual dysphoric disorder: evidence for a new category for Dsm-5. Am J Psychiatry. (2012) 169:465–75. doi: 10.1176/appi.ajp.2012.11081302
12. Petersen N, Ghahremani DG, Rapkin AJ, Berman SM, Liang L, London ED. Brain activation during emotion regulation in women with premenstrual dysphoric disorder. Psychol Med. (2018) 48:1795–802. doi: 10.1017/S0033291717003270
13. Hylan TR, Sundell K, Judge R. The impact of premenstrual symptomatology on functioning and treatment-seeking behavior: experience from the United States, United Kingdom, and France. J Women's Health Gend-Based Med. (1999) 8:1043–52. doi: 10.1089/jwh.1.1999.8.1043
14. Kendler KS, Karkowski LM, Corey LA, Neale MC. Longitudinal population-based twin study of retrospectively reported premenstrual symptoms and lifetime major depression. Am J Psychiatry. (1998) 155:1234–40. doi: 10.1176/ajp.155.9.1234
15. Treloar SA, Heath AC, Martin NG. Genetic and environmental influences on premenstrual symptoms in an Australian twin sample. Psychol Med. (2002) 32:25–38. doi: 10.1017/S0033291701004901
16. Gao M, Gao D, Sun H, Cheng X, An L, Qiao M. Trends in research related to premenstrual syndrome and premenstrual dysphoric disorder from 1945 to 2018: a bibliometric analysis. Front Public Health. (2021) 9:596128. doi: 10.3389/fpubh.2021.596128
17. Wittchen HU, Becker E, Lieb R, Krause P. Prevalence, incidence and stability of premenstrual dysphoric disorder in the community. Psychol Med. (2002) 32:119–32. doi: 10.1017/S0033291701004925
18. Francesmonneris A, Pincus H, First M. Diagnostic and Statistical Manual of Mental Disorders: Dsm-V. American Psychiatric Association (2013).
19. Dubol M, Epperson CN, Sacher J, Pletzer B, Derntl B, Lanzenberger R, et al. Neuroimaging the menstrual cycle: a multimodal systematic review. Front Neuroendocrinol. (2021) 60:100878. doi: 10.1016/j.yfrne.2020.100878
20. Toffoletto S, Lanzenberger R, Gingnell M, Sundström-Poromaa I, Comasco E. Emotional and cognitive functional imaging of estrogen and progesterone effects in the female human brain: a systematic review. Psychoneuroendocrinology. (2014) 50:28–52. doi: 10.1016/j.psyneuen.2014.07.025
21. Pletzer B, Harris TA, Scheuringer A, Hidalgo-Lopez E. The cycling brain: menstrual cycle related fluctuations in hippocampal and fronto-striatal activation and connectivity during cognitive tasks. Neuropsychopharmacology. (2019) 44:1867–75. doi: 10.1038/s41386-019-0435-3
22. Engman J, Sundström Poromaa I, Moby L, Wikström J, Fredrikson M, Gingnell M. Hormonal cycle and contraceptive effects on amygdala and salience resting-state networks in women with previous affective side effects on the pill. Neuropsychopharmacology. (2018) 43:555–63. doi: 10.1038/npp.2017.157
23. Barth C, Steele CJ, Mueller K, Rekkas VP, Arélin K, Pampel A, et al. In-Vivo dynamics of the human hippocampus across the menstrual cycle. Sci Rep. (2016) 6:32833. doi: 10.1038/srep32833
24. Lisofsky N, Mårtensson J, Eckert A, Lindenberger U, Gallinat J, Kühn S. Hippocampal volume and functional connectivity changes during the female menstrual cycle. Neuroimage. (2015) 118:154–62. doi: 10.1016/j.neuroimage.2015.06.012
25. Bayer J, Schultz H, Gamer M, Sommer T. Menstrual-cycle dependent fluctuations in ovarian hormones affect emotional memory. Neurobiol Learn Mem. (2014) 110:55–63. doi: 10.1016/j.nlm.2014.01.017
26. Ossewaarde L, van Wingen GA, Rijpkema M, Bäckström T, Hermans EJ, Fernández G. Menstrual cycle-related changes in amygdala morphology are associated with changes in stress sensitivity. Hum Brain Mapp. (2013) 34:1187–93. doi: 10.1002/hbm.21502
27. Deng D, Pang Y, Duan G, Liu H, Liao H, Liu P, et al. Larger volume and different functional connectivity of the amygdala in women with premenstrual syndrome. Eur Radiol. (2018) 28:1900–8. doi: 10.1007/s00330-017-5206-0
28. Liu P, Wei Y, Fan Y, Liao H, Wang G, Li R, et al. Cortical and subcortical changes in patients with premenstrual syndrome. J Affect Disord. (2018) 235:191–7. doi: 10.1016/j.jad.2018.04.046
29. Liao H, Duan G, Liu P, Liu Y, Pang Y, Liu H, et al. Altered fractional amplitude of low frequency fluctuation in premenstrual syndrome: a resting state fmri study. J Affect Disord. (2017) 218:41–8. doi: 10.1016/j.jad.2017.04.045
30. Liu Q, Li R, Zhou R, Li J, Gu Q. Abnormal resting-state connectivity at functional mri in women with premenstrual syndrome. PLoS ONE. (2015) 10:e0136029. doi: 10.1371/journal.pone.0136029
31. Arélin K, Mueller K, Barth C, Rekkas PV, Kratzsch J, Burmann I, et al. Progesterone mediates brain functional connectivity changes during the menstrual cycle-a pilot resting state mri study. Front Neurosci. (2015) 9:44. doi: 10.3389/fnins.2015.00044
32. Gao M, Qiao M, An L, Wang G, Wang J, Song C, et al. Brain reactivity to emotional stimuli in women with premenstrual dysphoric disorder and related personality characteristics. Aging. (2021) 13:19529–41. doi: 10.18632/aging.203363
33. Dan R, Reuveni I, Canetti L, Weinstock M, Segman R, Goelman G, et al. Trait-related changes in brain network topology in premenstrual dysphoric disorder. Horm Behav. (2020) 124:104782. doi: 10.1016/j.yhbeh.2020.104782
34. Petersen N, Ghahremani DG, Rapkin AJ, Berman SM, Wijker N, Liang L, et al. Resting-state functional connectivity in women with Pmdd. Transl Psychiatry. (2019) 9:339. doi: 10.1038/s41398-019-0670-8
35. Syan SK, Minuzzi L, Smith M, Costescu D, Allega OR, Hall GBC, et al. Brain structure and function in women with comorbid bipolar and premenstrual dysphoric disorder. Front Psychiatry. (2017) 8:301. doi: 10.3389/fpsyt.2017.00301
36. Gingnell M, Ahlstedt V, Bannbers E, Wikström J, Sundström-Poromaa I, Fredrikson M. Social stimulation and corticolimbic reactivity in premenstrual dysphoric disorder: a preliminary study. Biol Mood Anxiety Disord. (2014) 4:3. doi: 10.1186/2045-5380-4-3
37. Jeong HG, Ham BJ, Yeo HB, Jung IK, Joe SH. Gray matter abnormalities in patients with premenstrual dysphoric disorder: an optimized voxel-based morphometry. J Affect Disord. (2012) 140:260–7. doi: 10.1016/j.jad.2012.02.010
38. Gingnell M, Morell A, Bannbers E, Wikström J, Sundström Poromaa I. Menstrual cycle effects on amygdala reactivity to emotional stimulation in premenstrual dysphoric disorder. Horm Behav. (2012) 62:400–6. doi: 10.1016/j.yhbeh.2012.07.005
39. Squire LR, Stark CE, Clark RE. The medial temporal lobe. Annu Rev Neurosci. (2004) 27:279–306. doi: 10.1146/annurev.neuro.27.070203.144130
40. Dan R, Canetti L, Keadan T, Segman R, Weinstock M, Bonne O, et al. Sex differences during emotion processing are dependent on the menstrual cycle phase. Psychoneuroendocrinology. (2019) 100:85–95. doi: 10.1016/j.psyneuen.2018.09.032
41. van Wingen GA, van Broekhoven F, Verkes RJ, Petersson KM, Bäckström T, Buitelaar JK, et al. Progesterone selectively increases amygdala reactivity in women. Mol Psychiatry. (2008) 13:325–33. doi: 10.1038/sj.mp.4002030
42. Tye KM, Prakash R, Kim SY, Fenno LE, Grosenick L, Zarabi H, et al. Amygdala circuitry mediating reversible and bidirectional control of anxiety. Nature. (2011) 471:358–62. doi: 10.1038/nature09820
43. Pessoa L. A network model of the emotional brain. Trends Cogn Sci. (2017) 21:357–71. doi: 10.1016/j.tics.2017.03.002
44. Simons LE, Moulton EA, Linnman C, Carpino E, Becerra L, Borsook D. The human amygdala and pain: evidence from neuroimaging. Hum Brain Mapp. (2014) 35:527–38. doi: 10.1002/hbm.22199
45. Etkin A, Wager TD. Functional neuroimaging of anxiety: a meta-analysis of emotional processing in ptsd, social anxiety disorder, and specific phobia. Am J Psychiatry. (2007) 164:1476–88. doi: 10.1176/appi.ajp.2007.07030504
46. Murray EA, Wise SP, Drevets WC. Localization of dysfunction in major depressive disorder: prefrontal cortex and amygdala. Biol Psychiatry. (2011) 69:e43–54. doi: 10.1016/j.biopsych.2010.09.041
47. Bornhövd K, Quante M, Glauche V, Bromm B, Weiller C, Büchel C. Painful stimuli evoke different stimulus-response functions in the amygdala, prefrontal, insula and somatosensory cortex: a single-trial fmri study. Brain. (2002) 125:1326–36. doi: 10.1093/brain/awf137
48. Comasco E, Sundström-Poromaa I. Neuroimaging the menstrual cycle and premenstrual dysphoric disorder. Curr Psychiatry Rep. (2015) 17:77. doi: 10.1007/s11920-015-0619-4
49. Protopopescu X, Tuescher O, Pan H, Epstein J, Root J, Chang L, et al. Toward a functional neuroanatomy of premenstrual dysphoric disorder. J Affect Disord. (2008) 108:87–94. doi: 10.1016/j.jad.2007.09.015
50. McEwen BS, Milner TA. Understanding the broad influence of sex hormones and sex differences in the brain. J Neurosci Res. (2017) 95:24–39. doi: 10.1002/jnr.23809
51. Albert K, Pruessner J, Newhouse P. Estradiol levels modulate brain activity and negative responses to psychosocial stress across the menstrual cycle. Psychoneuroendocrinology. (2015) 59:14–24. doi: 10.1016/j.psyneuen.2015.04.022
52. Protopopescu X, Butler T, Pan H, Root J, Altemus M, Polanecsky M, et al. Hippocampal structural changes across the menstrual cycle. Hippocampus. (2008) 18:985–8. doi: 10.1002/hipo.20468
53. Eberling JL, Wu C, Tong-Turnbeaugh R, Jagust WJ. Estrogen- and tamoxifen-associated effects on brain structure and function. Neuroimage. (2004) 21:364–71. doi: 10.1016/j.neuroimage.2003.08.037
54. Acharya KD, Nettles SA, Sellers KJ, Im DD, Harling M, Pattanayak C, et al. The progestin receptor interactome in the female mouse hypothalamus: interactions with synaptic proteins are isoform specific and ligand dependent. eNeuro. (2017) 4: ENEURO.0272–17.2017. doi: 10.1523/ENEURO.0272-17.2017
55. Zsido RG, Villringer A, Sacher J. Using positron emission tomography to investigate hormone-mediated neurochemical changes across the female lifespan: implications for depression. Int Rev Psychiatry. (2017) 29:580–96. doi: 10.1080/09540261.2017.1397607
56. Almey A, Milner TA, Brake WG. Estrogen receptors in the central nervous system and their implication for dopamine-dependent cognition in females. Horm Behav. (2015) 74:125–38. doi: 10.1016/j.yhbeh.2015.06.010
57. Borrow AP, Cameron NM. Estrogenic mediation of serotonergic and neurotrophic systems: implications for female mood disorders. Prog Neuropsychopharmacol Biol Psychiatry. (2014) 54:13–25. doi: 10.1016/j.pnpbp.2014.05.009
58. Wei S, Geng X, Li Z, Xu K, Hu M, Wu H, et al. A forced swim-based rat model of premenstrual depression: effects of hormonal changes and drug intervention. Aging. (2020) 12:24357–70. doi: 10.18632/aging.202249
59. Brinton RD, Thompson RF, Foy MR, Baudry M, Wang J, Finch CE, et al. Progesterone receptors: form and function in brain. Front Neuroendocrinol. (2008) 29:313–39. doi: 10.1016/j.yfrne.2008.02.001
60. Liao H, Pang Y, Liu P, Liu H, Duan G, Liu Y, et al. Abnormal spontaneous brain activity in women with premenstrual syndrome revealed by regional homogeneity. Front Hum Neurosci. (2017) 11:62. doi: 10.3389/fnhum.2017.00062
61. Dreher JC, Schmidt PJ, Kohn P, Furman D, Rubinow D, Berman KF. Menstrual cycle phase modulates reward-related neural function in women. Proc Natl Acad Sci U S A. (2007) 104:2465–70. doi: 10.1073/pnas.0605569104
62. van Wingen G, van Broekhoven F, Verkes RJ, Petersson KM, Bäckström T, Buitelaar J, et al. How progesterone impairs memory for biologically salient stimuli in healthy young women. J Neurosci. (2007) 27:11416–23. doi: 10.1523/JNEUROSCI.1715-07.2007
63. Cossart R, Khazipov R. How development sculpts hippocampal circuits and function. Physiol Rev. (2022) 102:343–78. doi: 10.1152/physrev.00044.2020
64. Gao Y, Wang M, Yu R, Li Y, Yang Y, Cui X, et al. Abnormal default mode network homogeneity in treatment-naive patients with first-episode depression. Front Psychiatry. (2018) 9:697. doi: 10.3389/fpsyt.2018.00697
65. Gao Y, Wang X, Xiong Z, Ren H, Liu R, Wei Y, et al. Abnormal fractional amplitude of low-frequency fluctuation as a potential imaging biomarker for first-episode major depressive disorder: a resting-state fmri study and support vector machine analysis. Front Neurol. (2021) 12:751400. doi: 10.3389/fneur.2021.751400
66. Pritschet L, Santander T, Taylor CM, Layher E, Yu S, Miller MB, et al. Functional reorganization of brain networks across the human menstrual cycle. Neuroimage. (2020) 220:117091. doi: 10.1016/j.neuroimage.2020.117091
67. Hidalgo-Lopez E, Mueller K, Harris T, Aichhorn M, Sacher J, Pletzer B. Human menstrual cycle variation in subcortical functional brain connectivity: a multimodal analysis approach. Brain Struct Funct. (2020) 225:591–605. doi: 10.1007/s00429-019-02019-z
68. Davey CG, Whittle S, Harrison BJ, Simmons JG, Byrne ML, Schwartz OS, et al. Functional brain-imaging correlates of negative affectivity and the onset of first-episode depression. Psychol Med. (2015) 45:1001–9. doi: 10.1017/S0033291714002001
69. Gu X, Dubol M, Stiernman L, Wikström J, Hahn A, Lanzenberger R, et al. White matter microstructure and volume correlates of premenstrual dysphoric disorder. J Psychiatry Neurosci. (2022) 47:E67–76. doi: 10.1503/jpn.210143
70. Comasco E, Hahn A, Ganger S, Gingnell M, Bannbers E, Oreland L, et al. Emotional fronto-cingulate cortex activation and brain derived neurotrophic factor polymorphism in premenstrual dysphoric disorder. Hum Brain Mapp. (2014) 35:4450–8. doi: 10.1002/hbm.22486
71. Hantsoo L, Epperson CN. Premenstrual dysphoric disorder: epidemiology and treatment. Curr Psychiatry Rep. (2015) 17:87. doi: 10.1007/s11920-015-0628-3
Keywords: premenstrual syndrome, premenstrual dysphoric disorder, temporal lobe, magnetic resonance imaging, multimodal neuroimaging
Citation: Long JY, Wang YJ, Liu LZ and Zhang J (2022) The Prominent Role of the Temporal Lobe in Premenstrual Syndrome and Premenstrual Dysphoric Disorder: Evidence From Multimodal Neuroimaging. Front. Psychiatry 13:954211. doi: 10.3389/fpsyt.2022.954211
Received: 27 May 2022; Accepted: 08 June 2022;
Published: 28 June 2022.
Edited by:
Qinji Su, Guangxi Medical University, ChinaReviewed by:
Hongxian Shen, Central South University, ChinaXuyi Wang, Central South University, China
Copyright © 2022 Long, Wang, Liu and Zhang. This is an open-access article distributed under the terms of the Creative Commons Attribution License (CC BY). The use, distribution or reproduction in other forums is permitted, provided the original author(s) and the copyright owner(s) are credited and that the original publication in this journal is cited, in accordance with accepted academic practice. No use, distribution or reproduction is permitted which does not comply with these terms.
*Correspondence: Lianzhong Liu, 184923047@qq.com; Juan Zhang, 1365716662@qq.com
†These authors have contributed equally to this work and share first authorship