- 1Biopharmacy, Department of Pharmaceutical Sciences, University of Basel, Basel, Switzerland
- 2Pharmaceutical Care, Department of Pharmaceutical Sciences, University of Basel, Basel, Switzerland
- 3Institute of Hospital Pharmacy, Solothurner Spitäler AG, Olten, Switzerland
- 4Psychiatric Services Solothurn, Solothurner Spitälerler AG and Faculty of Medicine, University of Basel, Solothurn, Switzerland
We report the case of a 50-year-old male with major depressive disorder (MDD) to illustrate the challenge of finding effective antidepressant pharmacotherapy and the role that the patient’s genetic makeup may play. Recent treatment attempts before clinic admission included venlafaxine and fluoxetine. Venlafaxine was discontinued due to lack of response, and subsequently switched to fluoxetine based on pharmacogenotyping of the P-glycoprotein transporter (P-gp, encoded by ABCB1) by the outpatient psychiatrist. Despite steady state serum levels within the therapeutic range, the patient did not benefit from fluoxetine either, necessitating admission to our clinic. Here a clinical pharmacist-led medication review including additional pharmacogenetic (PGx) analysis resulted in the change of the antidepressant therapy to bupropion. Under the new regimen, established in the in-patient-setting, the patient remitted. However, based on the assessed pharmacokinetics-related gene variants, including CYPs and ABCB1, non-response to fluoxetine could not be conclusively explained. Therefore, we retrospectively selected the serotonin transporter (SERT1, encoded by SLC6A4) for further genetic analysis of pharmacodynamic variability. The patient presented to be a homozygous carrier of the short allele variant in the 5-HTTLPR (S/S) located within the SLC6A4 promoter region, which has been associated with a reduced expression of the SERT1. This case points out the potential relevance of panel PGx testing considering polymorphisms in genes of pharmacokinetic as well as pharmacodynamic relevance.
Introduction
Major depressive disorder (MDD) is a common condition that imposes a high disease burden on the individual patient (1). However, not only the affected patients, but also the healthcare system and society are challenged by the disorder, in particular due to the resulting costs. The majority of the costs are of indirect kind and arise due to unemployment, sick leave and early retirement (2, 3). Therefore, it is important to effectively treat MDD. A relevant pillar in the treatment of MDD is pharmacotherapy. Fortunately, a wide range of marketed antidepressants is available today for clinicians and patients to choose from. Still, treatment of MDD remains challenging as it is known that up to 50% of unipolar depressed patients treated with antidepressants do not respond to their first-line treatment (4, 5). Ineffective antidepressant treatment may prolong the disease state, increasing the burden on the patient, the health care system, and society.
Multiple factors impact the response to antidepressants, including the patient’s genetic makeup. On the one hand, genetic variation can alter the expression and/or activity of enzymes and transporters involved in drug absorption, distribution, metabolism, or excretion (ADME), causing interindividual differences in pharmacokinetics. On the other hand, genetic variation can affect the expression and/or structure of drug targets, potentially interfering with pharmacodynamics. Pharmacokinetic as well as pharmacodynamic alterations may impact both, tolerability and effectiveness of a drug (6).
The role of genetic predisposition in antidepressant response is extensively discussed in basic research as well as in clinical practice (7–9). So far, mainly pharmacokinetics-related genetic markers have found their way into clinical practice. In particular, compelling evidence on the impact of genetic variation of the enzyme cytochrome P450 (CYP) 2D6 and CYP2C19 has led to the publication of guidelines with recommendations for genotype-based selection and dosing of selective serotonin reuptake inhibitors (SSRI) and tricyclic antidepressants (10, 11). Both cytochromes, CYP2D6 and CYP2C19, are highly polymorphic which is reflected by the fact that over 60% of the general European population have a predicted phenotype that deviates from a normal metabolizer (extensive metabolizer, EM) (12). Moreover, the Swiss Society for Anxiety and Depression (SGAD) recommends genotyping of the P-glycoprotein (P-gp, encoded by ABCB1) after antidepressant treatment failure (13). P-gp is an efflux transporter which is also expressed in the blood-brain barrier (BBB), where it has an important gatekeeping role and extrudes various substances including certain antidepressants (14). It is hypothesized that carriers of the respective reference variant (wildtype) have restricted permeability of their BBB to antidepressants that are P-gp-substrates and therefore may only reach a limited concentration in the brain at their site of action (15). This theory is based on a limited number of clinical studies that associated certain ABCB1 polymorphisms to antidepressant treatment response (15–17).
In addition to the afore described pharmacokinetics-related genetic variants, there is also evidence indicating effects of polymorphisms in pharmacodynamic-related genes on antidepressant efficacy and tolerability (18). It still remains controversial whether genetic variants in pharmacodynamically relevant antidepressant targets should be adopted in clinical practice. To date, there are no treatment recommendations based on any pharmacodynamic-related gene variants available for antidepressants. Extensive research is ongoing in this area, in particular studies on polymorphisms in the SLC6A4 gene, encoding for the serotonin transporter (SERT1). The promoter region of the SLC6A4 harbors a highly polymorphic region, named 5-HTTLPR (rs774676466), with a 44 base pair insertion-deletion (INDEL) variation (19). The short variant (S-allele) has a minor allele frequency of about 20% on a global average (20) and has been linked with reduced transcriptional activity and therefore limited expression of the encoded SERT1 (19). The SERT1 facilitates the reuptake of serotonin from the synaptic cleft into the presynapse and is a relevant target of various antidepressants, especially SSRIs (21). Hitherto, multiple studies linked the 5-HTTLPR variation with antidepressant therapy outcome (22, 23). However, it is difficult to apply these findings in practice, as there are currently no guidelines available associating SLC6A4 genotypes with concrete recommendations for antidepressant selection and dosing. Herein we are reporting a case, where the SLC6A4 5-HTTLPR variation was likely causative in the tediously protracted search for an effective antidepressant.
Case presentation
Clinical case and medication history
A 50-year-old male with a long lasting history of recurrent MDD (ICD-10 F33), admitted himself to the medical emergency ward and was referred to our psychiatric crisis intervention unit. There he presented himself with sleeping disorders, rumination, anxiety, a lack of drive and recently increasing suicidal ideation. According to the patient, his current depressive episode started over 2 years ago with the loss of his employment and culminated in an acute deterioration a month prior to admission. At our clinic he was diagnosed with a moderate depressive episode (ICD-10 F33.1), reflected by a score of 19 on the 21-item Hamilton Rating Scale (HAMD-21) (24) and by a score of 26 on the patient-rated Beck Depression Inventory (BDI) (25).
At clinic entry, the patient was under treatment with a combination of low-dose trimipramine (50 mg/d) for sleep promotion, and fluoxetine (40 mg/d) for depression, which was established 3 months earlier by an outpatient psychiatrist (Figure 1). Before starting this treatment, a long-term treatment with venlafaxine was terminated by the outpatient psychiatrist due to ineffectiveness. His decision to switch to fluoxetine was based on two genetic markers of the ABCB1 gene, encoding for P-glycoprotein (Table 1), determined in the laboratory of Viollier AG (Allschwil, Switzerland), as recommended by the SGAD (13).
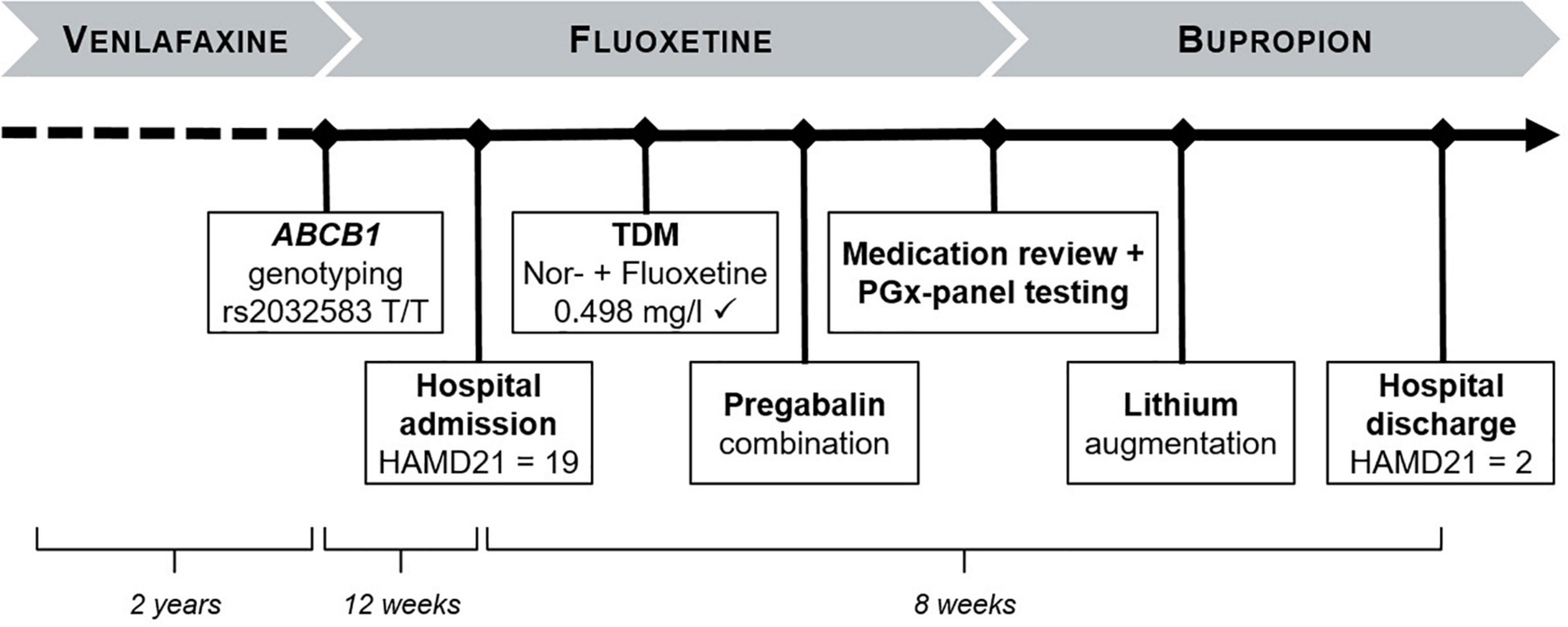
Figure 1. Overview case presentation. TDM, therapeutic drug monitoring; HAMD, Hamilton depression rating scale; PGx, pharmagocenetic.
Despite a daily dose of 40 mg fluoxetine and steady state trough serum levels within the therapeutic range [fluoxetine + norfluoxetine = 0.498 mg/l, ref. 0.120–0.500 mg/l (26)], the patient did not benefit from treatment with fluoxetine. Therapeutic efficacy did not improve in the in-patient-setting and in combination with pregabalin, which was initiated at the clinic due to restlessness and strain. Due to persisting non-response within the first month of hospitalization, a clinical pharmacist-led medication review including additional pharmacogenetic analysis was initiated. This clinical pharmacy service was part of an observational study approved by the local ethics committee (ClinicalTrials.gov identifier: NCT04154553). The patient gave written informed consent for panel pharmacogenotyping and health data retrieval. A buccal swab was collected to apply the commercial pharmacogenotyping service Stratipharm® offered by humatrix AG (Pfungstadt, Germany). In their laboratory, the polymorphisms are determined by applying real-time PCR using the automated Life Technologies QuantStudio 12 k flex (Thermo Fisher, MA, United States) with the respective optimized and commercially available chemistry. The applied commercial PGx panel test includes genetic variants frequently observed in the European population, including alleles discussed in the CPIC guidelines.1 Interpretation of the genotyping results identified the patient as a normal metabolizer (NM, *1 homozygous) for CYP2B6, CYP2C9 and CYP2D6 (Table 1). In addition, the patient’s CYP2C19 phenotype was predicted as intermediate metabolizer (IM, *2 heterozygous) (Table 1). Based on these results and the patient’s history of non-response to venlafaxine, a selective serotonin and norepinephrine reuptake inhibitor (SNRI), and fluoxetine, a selective serotonin reuptake inhibitor (SSRI), a switch to bupropion, a norepinephrine–dopamine reuptake inhibitor, was recommended by the clinical pharmacist. Bupropion is mainly metabolized via CYP2B6 and not a substrate of the P-gp transporter (27, 28). After the patient’s medication was switched from fluoxetine to bupropion, a clinical improvement in drive and mood was observable within 1 week. For further improvement and maintenance treatment, an augmentation with lithium was added. Under this combined treatment regimen (Table 2), the patient remitted and was discharged to out-patient care within 4 weeks of treatment change to bupropion, and after a total of 8 weeks of in-patient care. Remission was quantified at discharge with a HAM-D21 score of 2 and a BDI score of 5, compared to 19, respectively, 26 at clinic admission. When followed up 8 weeks after discharge, the patient was still in remission.
Pharmacogenetic data interpretation and further analysis
Prior to the introduction of bupropion, which eventually proved to be effective, our patient had to endure insufficient antidepressant treatment over the course of more than 2 years. The initial non-response to venlafaxine was attributed to the patients ABCB1 genotype, with no variation for the polymorphisms rs2032583 and rs2235015. Homozygous carriers of the respective wildtype alleles have been associated with a reduced likelihood of depression remission when treated with antidepressants that are P-gp-substrates. Since venlafaxine is a known P-gp-substrate (15, 29), the treating ambulant psychiatrist decided to switch to the SSRI fluoxetine, a non-relevant P-gp-substrate (30, 31). However, despite these considerations, the patient’s depression deteriorated even further under fluoxetine, necessitating in-patient treatment. There, due to the known involvement of polymorph CYPs in the metabolism of venlafaxine and fluoxetine, further panel pharmacogenotyping was initiated. For venlafaxine, there are PGx-based dosing guidelines available, taking the predicted CYP2D6 phenotype into account (32). Fluoxetine is known to be mainly metabolized via the polymorph CYP2D6 and CYP2C9. Although there is no PGx-based dosing guideline available for fluoxetine, genetic variants of CYP2D6 and CYP2C9 have been associated with alterations in its pharmacokinetics (33, 34). However, based on the patient’s genetic analysis (Table 1), both CYP2D6 and CYP2C9 are predicted to have normal activity, suggesting that there are no known drug-gene interactions. This is also reflected in the measured nor-/fluoxetine serum levels, which was within the therapeutic reference range at steady state with a common daily dosage of 40 mg.
The non-response to fluoxetine could not be conclusively explained by the assessed pharmacokinetics-related gene variations, including CYPs and ABCB1. The SERT1 2A (HTR2A), which is part of the commercial panel, was also inconspicuous in relation to fluoxetine (12). Therefore, we retrospectively selected the SERT1 (encoded by SLC6A4) for further genetic analysis of pharmacodynamic variability. We genotyped for the 5-HTTLPR polymorphism applying the protocol described elsewhere (35) and using gDNA isolated from the patient’s whole blood sample using the QIACube® with the QIAamp® DNA Blood Mini Kit (Qiagen, Hilden, Germany). Herein, the patient presented to be a homozygous carrier of the minor short allele variant in the 5-HTTLPR polymorphism (S/S) of the SLC6A4. The 5-HTTLPR S-allele is assumed to cause reduced expression of the SERT1, the target of serotonin reuptake inhibitors including fluoxetine and venlafaxine (19). Several studies associated the 5-HTTLPR major variant, so called L-allele, with an increased likelihood of antidepressant response, especially in Caucasians (22). A recent meta-analysis further specified that the 5-HTTLPR L-allele predicts response specifically to SSRI’s (23). It seems plausible that in the reported case, the present SLC6A4 variant has indeed affected fluoxetine effectiveness. We hypothesize that this is a relevant reason why the patient clearly benefited from a switch to the noradrenaline and dopamine reuptake inhibitor bupropion, which does not target the genetically affected SERT1.
Conclusion and outlook
The patient’s SLC6A4 genotype (S/S) may likely explain why switching to fluoxetine proved ineffective and even led to an acute exacerbation of the depression. The SERT1 is selectively targeted by SSRIs, but its inhibition also contributes to the therapeutic effect of SNRIs and tricyclic antidepressants (36). Consequently, an influence of SLC6A4 genetic variants on the effect of other antidepressants binding the SERT1 seems plausible. However, the number of studies evaluating the effectiveness of non-SSRI antidepressants in context with SLC6A4 polymorphisms is still very limited and recent meta-analyses were unable to detect corresponding effects (23, 37). It may be speculated that a pre-emptive approach in PGx testing of the 5-HTTLPR might have significantly reduced the patient’s burden and even avoided hospitalization. Some commercial pharmacogenetic tests already include SLC6A4 polymorphisms in their panels (38). However, currently there are no recommendations for drug dosing and selection considering polymorphisms in SLC6A4. It also seems noteworthy at this point, that besides effectiveness, SLC6A4 variants have been associated with antidepressant tolerability (39, 40) and even depression susceptibility with SLC6A4 variation as a potential disease modifying factor (41, 42). Further prospective studies are warranted before genotyping of the SERT1 can be recommended as an additional basis for antidepressant selection. Besides SLC6A4, other pharmacodynamically relevant gene variants may gain importance in the near future. Candidate genes under investigation that have been associated with antidepressant efficacy, include genes encoding for the tryptophan hydroxylase (TPH), serotonin receptors (5-HT1A, 5-HT2A, 5-HT6), dopamine receptors (DRD2, DRD4) and others (18). It is conceivable that pharmacokinetic as well as pharmacodynamic gene variants have a combined effect on the efficacy and tolerability of antidepressants. Therefore, a broader polygenetic approach with panel PGx tests is expected to further gain relevance for a personalized medicine approach in selection and dosing of antidepressants.
Data availability statement
The original contributions presented in this study are included in the article/Supplementary Material, further inquiries can be directed to the corresponding author/s.
Ethics statement
The studies involving human participants were reviewed and approved by the Ethikkommission Nordwest- und Zentralschweiz (EKNZ), 4056 Basel, Switzerland. The patients/participants provided their written informed consent to participate in this study. Written informed consent was obtained from the individual(s) for the publication of any potentially identifiable images or data included in this article.
Author contributions
CS, ML, KH, SA, and HM: conceptualization and study design. CS, RM, ML, and HM: investigation and interpretation of genotyping data. TM and MH: psychiatric clinical assessments. CS: writing—original draft preparation and visualization. RM, TM, MH, ML, SA, KH, and HM: writing—additional content, critical review, and editing. HM: supervision. All authors have read and agreed to the published version of the manuscript.
Funding
CS received funding from the “Stiftung zur Förderung des pharmazeutischen Nachwuchses in Basel,” in Basel, Switzerland.
Conflict of interest
The authors declare that the research was conducted in the absence of any commercial or financial relationships that could be construed as a potential conflict of interest.
Publisher’s note
All claims expressed in this article are solely those of the authors and do not necessarily represent those of their affiliated organizations, or those of the publisher, the editors and the reviewers. Any product that may be evaluated in this article, or claim that may be made by its manufacturer, is not guaranteed or endorsed by the publisher.
Acknowledgments
We would like to thank Drs. Med. Rebecca Kungler and Med. Dusica Arsic for their support in patient recruitment.
Supplementary Material
The Supplementary Material for this article can be found online at: https://www.frontiersin.org/articles/10.3389/fpsyt.2022.942268/full#supplementary-material
Footnotes
References
1. Kyu HH, Abate D, Abate KH, Abay SM, Abbafati C, Abbasi N, et al. Global, Regional, and national disability-adjusted life-years (Dalys) for 359 diseases and injuries and healthy life expectancy (Hale) for 195 countries and territories, 1990–2017: a systematic analysis for the global burden of disease study 2017. Lancet. (2018) 392:1859–922. doi: 10.1016/s0140-6736(18)32335-3
2. Sobocki P, Ekman M, Ågren H, Krakau I, Runeson B, Mårtensson B, et al. Resource use and costs associated with patients treated for depression in primary care. Eur J Health Econ. (2007) 8:67–76. doi: 10.1007/s10198-006-0008-3
3. Greenberg PE, Kessler RC, Birnbaum HG, Leong SA, Lowe SW, Berglund PA, et al. The economic burden of depression in the United States: how did it change between 1990 and 2000? J Clin Psychiatry. (2003) 64:1465–75. doi: 10.4088/jcp.v64n1211
4. Keller MB, Lavori PW, Mueller TI, Endicott J, Coryell W, Hirschfeld RM, et al. Time to recovery, chronicity, and levels of psychopathology in major depression: a 5-year prospective follow-up of 431 subjects. Arch Gen Psychiatry. (1992) 49:80916. doi: 10.1001/archpsyc.1992.01820100053010
5. Trivedi MH, Rush AJ, Wisniewski SR, Nierenberg AA, Warden D, Ritz L, et al. Evaluation of outcomes with citalopram for depression using measurement-based care in star*D: implications for clinical practice. Am J Psychiatry. (2006) 163:28–40. doi: 10.1176/appi.ajp.163.1.28
6. Meyer Zu Schwabedissen HE. The role of pharmacogenomics in individualized medicine. In: T Fischer, M Langanke, P Marschall, S Michl editors. Individualized Medicine–Ethical, Economical and Historical Perspectives. (Vol. 7), Cham: Springer (2015). p. 93–112.
7. Stäuble CK, Lampert ML, Mikoteit T, Hatzinger M, Hersberger KE, Meyer Zu Schwabedissen HE. Nonresponse to high-dose bupropion for depression in a patient carrying CYP2B6*6 and CYP2C19*17 variants: a case report. Pharmacogenomics. (2020) 21:1145–50. doi: 10.2217/pgs-2020-0087
8. Stäuble CK, Lampert ML, Mikoteit T, Hatzinger M, Hersberger KE, Meyer Zu Schwabedissen HE. Severe adverse drug reactions to quetiapine in two patients carrying CYP2D6*4 variants: a case report. Int J Mol Sci. (2021) 22:6480. doi: 10.3390/ijms22126480
9. Stäuble CK, Lampert ML, Mikoteit T, Hatzinger M, Hersberger KE, Meyer Zu Schwabedissen HE. Pharmacogenetic-guided antidepressant selection as an opportunity for interprofessional collaboration: a case report. Life. (2021) 11:673. doi: 10.3390/life11070673
10. Hicks J, Sangkuhl K, Swen J, Ellingrod V, Müller D, Shimoda K, et al. Clinical pharmacogenetics implementation consortium guideline (CPIC) for CYP2D6 and CYP2C19 genotypes and dosing of tricyclic antidepressants: 2016 update. Clin Pharmacol Ther. (2017) 102:37–44. doi: 10.1002/cpt.597
11. Hicks J, Bishop JR, Sangkuhl K, Müller D, Ji Y, Leckband S, et al. Clinical pharmacogenetics implementation consortium (CPIC) guideline for CYP2D6 and CYP2C19 genotypes and dosing of selective serotonin reuptake inhibitors. Clin Pharmacol Ther. (2015) 98:127–34. doi: 10.1002/cpt.147
12. Whirl-Carrillo M, McDonagh EM, Hebert JM, Gong L, Sangkuhl K, Thorn CF, et al. Pharmacogenomics knowledge for personalized medicine. Clin Pharmacol Ther. (2012) 92:414–7. doi: 10.1038/clpt.2012.96
13. Holsboer-Trachsler E, Hättenschwiler JA, Beck J, Brand S, Hemmeter UM, Keck ME, et al. Die akutbehandlung depressiver episoden. Swiss Med Forum. (2016) 16:716–24. doi: 10.4414/smf.2016.02704
14. O’Brien FE, Dinan TG, Griffin BT, Cryan JF. Interactions between antidepressants and P-Glycoprotein at the blood-brain barrier: clinical significance of in vitro and in vivo findings. Br J Pharmacol. (2012) 165:289–312. doi: 10.1111/j.1476-5381.2011.01557.x
15. Uhr M, Tontsch A, Namendorf C, Ripke S, Lucae S, Ising M, et al. Polymorphisms in the drug transporter gene abcb1 predict antidepressant treatment response in depression. Neuron. (2008) 57:203–9. doi: 10.1016/j.neuron.2007.11.017
16. Breitenstein B, Scheuer S, Pfister H, Uhr M, Lucae S, Holsboer F, et al. The clinical application of Abcb1 genotyping in antidepressant treatment: a pilot study. CNS Spectrums. (2014) 19:165–75. doi: 10.1017/s1092852913000436
17. Breitenstein B, Scheuer S, Brückl TM, Meyer J, Ising M, Uhr M, et al. Association of Abcb1 gene variants, plasma antidepressant concentration, and treatment response: results from a randomized clinical study. J Psychiatr Res. (2016) 73:86–95. doi: 10.1016/j.jpsychires.2015.11.010
18. Islam F, Gorbovskaya I, Müller DJ. Pharmacogenetic/pharmacogenomic tests for treatment prediction in depression. In: Y-K Kim editor. Major Depressive Disorder. 1305. Singapore: Springer Nature (2021). p. 231–55. doi: 10.1007/978-981-33-6044-0_13
19. Heils A, Teufel A, Petri S, Stöber G, Riederer P, Bengel D, et al. Allelic variation of human serotonin transporter gene expression. J Neurochem. (1996) 66:2621–4. doi: 10.1046/j.1471-4159.1996.66062621.x
20. Phan L, Jin Y, Zhang H, Qiang E, Shekhtman D, Shao D, et al. Alfa: Allele Frequency Aggregator. Bethesda, MD: National Center for Biotechnology Information: U.S. National Library of Medicine (2020).
21. Sangkuhl K, Klein TE, Altman RB. Selective serotonin reuptake inhibitors pathway. Pharmacogenet Genomics. (2009) 19:907–9. doi: 10.1097/fpc.0b013e32833132cb
22. Porcelli S, Fabbri C, Serretti A. Meta-analysis of serotonin transporter gene promoter polymorphism (5-HTTLPR) Association with antidepressant efficacy. Eur Neuropsychopharmacol. (2012) 22:239–58. doi: 10.1016/j.euroneuro.2011.10.003
23. Ren F, Ma Y, Zhu X, Guo R, Wang J, He L. Pharmacogenetic association of Bi- and triallelic polymorphisms of SLC6A4 with antidepressant response in major depressive disorder. J Affect Disord. (2020) 273:254–64. doi: 10.1016/j.jad.2020.04.058
24. Hamilton MA. Rating scale for depression. J Neurol Neurosurg Psychiatry. (1960) 23:56–62. doi: 10.1136/jnnp.23.1.56
25. Beck AT, Beamesderfer A. Assessment of depression: the depression inventory. Mod Probl Pharmacopsychiatry. (1974) 7:151–69. doi: 10.1159/000395074
26. Hiemke C, Bergemann N, Clement H, Conca A, Deckert J, Domschke K, et al. Consensus guidelines for therapeutic drug monitoring in neuropsychopharmacology: update 2017. Pharmacopsychiatry. (2018) 51:9–62. doi: 10.1055/s-0043-116492
27. Jefferson JW, Pradko JF, Muir KT. Bupropion for major depressive disorder: pharmacokinetic and formulation considerations. Clin Ther. (2005) 27:1685–95. doi: 10.1016/j.clinthera.2005.11.011
28. Wang J-S, Zhu H-J, Gibson BB, Markowitz JS, Donovan JL, Devane CL. Sertraline and its metabolite desmethylsertraline, but not bupropion or its three major metabolites, have high affinity for P-Glycoprotein. Biol Pharm Bull. (2008) 31:231–4. doi: 10.1248/bpb.31.231
29. Doran A, Obach RS, Smith BJ, Hosea NA, Becker S, Callegari E, et al. The impact of P-Glycoprotein on the disposition of drugs targeted for indications of the central nervous system: evaluation using the Mdr1a/1b knockout mouse model. Drug Metab Dispos. (2005) 33:165–74. doi: 10.1124/dmd.104.001230
30. Uhr M, Streckler T, Yassouridis A, Holsboer F. Penetration of amitriptyline, but not of fluoxetine, into brain is enhanced in mice with blood-brain barrier deficiency due to Mdr1a P-Glycoprotein gene disruption. Neuropsychopharmacology. (2000) 22:380–7. doi: 10.1016/s0893-133x(99)00095-0
31. O’Brien FE, Clarke G, Dinan TG, Cryan JF, Griffin BT. Human P-Glycoprotein differentially affects antidepressant drug transport: relevance to blood–brain barrier permeability. Int J Neuropsychopharmacol. (2013) 16:2259–72. doi: 10.1017/s1461145713000692
32. KNMP. Dutch Pharmacogenetic Working Group Recommendations. (2022). Available online at: https://www.knmp.nl/dossiers/farmacogenetica (Accessed February 2, 2022)
33. Ring BJ, Eckstein JA, Gillespie JS, Binkley SN, VandenBranden M, Wrighton SA. Identification of the human cytochromes p450 responsible for in vitro formation of R- and S-Norfluoxetine. J Pharmacol Exp Ther. (2001) 297:1044–50.
34. LLerena A, Dorado P, Berecz R, González A, Peñas-LLedó E. Effect of CYP2D6 and CYP2C9 genotypes on fluoxetine and norfluoxetine plasma concentrations during steady-state conditions. Eur J Clin Pharmacol. (2004) 59:869–73. doi: 10.1007/s00228-003-0707-y
35. Vizeli P, Meyer Zu Schwabedissen HE, Liechti ME. Role of serotonin transporter and receptor gene variations in the acute effects of Mdma in healthy subjects. ACS Chem Neurosci. (2019) 10:3120–31. doi: 10.1021/acschemneuro.8b00590
36. Feighner JP. Mechanism of action of antidepressant medications. J Clin Psychiatry. (1999) 60(Suppl. 4):4–11;discussion2–3.
37. Stein K, Maruf AA, Müller DJ, Bishop JR, Bousman CA. Serotonin transporter genetic variation and antidepressant response and tolerability: a systematic review and meta-analysis. J Pers Med. (2021) 11:1334. doi: 10.3390/jpm11121334
38. Bousman CA, Hopwood M. Commercial pharmacogenetic-based decision-support tools in psychiatry. Lancet Psychiatry. (2016) 3:585–90. doi: 10.1016/s2215-0366(16)00017-1
39. Perlis RH, Mischoulon D, Smoller JW, Wan Y-JY, Lamon-Fava S, Lin K-M, et al. Serotonin transporter polymorphisms and adverse effects with fluoxetine treatment. Biol Psychiatry. (2003) 54:879–83. doi: 10.1016/S0006-3223(03)00424-4
40. Murphy GM, Hollander SB, Rodrigues HE, Kremer C, Schatzberg AF. Effects of the serotonin transporter gene promoter polymorphism onmirtazapine and paroxetine efficacy and adverse events in geriatric majordepression. Arch Gen Psychiatry. (2004) 61:1163. doi: 10.1001/archpsyc.61.11.1163
41. Ogilvie AD, Battersby S, Fink G, Harmar AJ, Goodwin GM, Bubb VJ, et al. Polymorphism in serotonin transporter gene associated with susceptibility to major depression. Lancet. (1996) 347:731–3. doi: 10.1016/s0140-6736(96)90079-3
Keywords: pharmacogenetics, depression, pharmaceutical care, SLC6A4, 5-HTT, ABCB1, pharmacodynamics, venlafaxine
Citation: Stäuble CK, Meier R, Lampert ML, Mikoteit T, Hatzinger M, Allemann SS, Hersberger KE and Meyer zu Schwabedissen HE (2022) Case report: Non-response to fluoxetine in a homozygous 5-HTTLPR S-allele carrier of the serotonin transporter gene. Front. Psychiatry 13:942268. doi: 10.3389/fpsyt.2022.942268
Received: 12 May 2022; Accepted: 28 June 2022;
Published: 15 July 2022.
Edited by:
Maria S. Garcia-Gutierrez, Miguel Hernández University of Elche, SpainReviewed by:
Darya Bazovkina, Institute of Cytology and Genetics (RAS), RussiaAarthi Manoharan, Aarupadai Veedu Medical College and Hospital, India
Copyright © 2022 Stäuble, Meier, Lampert, Mikoteit, Hatzinger, Allemann, Hersberger and Meyer zu Schwabedissen. This is an open-access article distributed under the terms of the Creative Commons Attribution License (CC BY). The use, distribution or reproduction in other forums is permitted, provided the original author(s) and the copyright owner(s) are credited and that the original publication in this journal is cited, in accordance with accepted academic practice. No use, distribution or reproduction is permitted which does not comply with these terms.
*Correspondence: Céline K. Stäuble, Y2VsaW5lLnN0YWV1YmxlQHVuaWJhcy5jaA==