- 1Yangtze University Health Science Center, Jingzhou, China
- 2Mental Health Institute of Yangtze University, Jingzhou, China
- 3Jingzhou Mental Health Center, Jingzhou, China
Omega-3 polyunsaturated fatty acids (PUFAs) can play important roles in maintaining mental health and resistance to stress, and omega-3 PUFAs supplementation can display beneficial effects on both the prevention and treatment of depressive disorders. Although the underlying mechanisms are still unclear, accumulated evidence indicates that omega-3 PUFAs can exhibit pleiotropic effects on the neural structure and function. Thus, they play fundamental roles in brain activities involved in the mood regulation. Since depressive symptoms have been assumed to be of central origin, this review aims to summarize the recently published studies to identify the potential neurobiological mechanisms underlying the anti-depressant effects of omega-3 PUFAs. These include that of (1) anti-neuroinflammatory; (2) hypothalamus-pituitary-adrenal (HPA) axis; (3) anti-oxidative stress; (4) anti-neurodegeneration; (5) neuroplasticity and synaptic plasticity; and (6) modulation of neurotransmitter systems. Despite many lines of evidence have hinted that these mechanisms may co-exist and work in concert to produce anti-depressive effects, the potentially multiple sites of action of omega-3 PUFAs need to be fully established. We also discussed the limitations of current studies and suggest future directions for preclinical and translational research in this field.
Introduction
Depression is a mental disorder characterized by the sadness, loss of interest in activities, and decreased energy. It is often accompanied by the cognitive impairment and different physical symptoms. In severe cases, it may lead to suicidal tendencies (1). Depression is a high-incidence mental illness that has affected more than 264 million people of all ages worldwide by 2021 (2). Subclinical depression has a higher incidence in the general population. The rate of subclinical depression can reach up to 17% in the primary care and community setting (3, 4). Moreover, the rate for the high school students is 22.9%, while that for the college students have reached 36.56%. Most currently available antidepressants can target monoamine neurotransmitter function. However, current pharmacological treatments of depression suffer from major problems, such as a low rate of response, slow onset of therapeutic effects, loss of efficacy over time, and serious side effects. Therefore, development of novel strategies both for the prevention and treatment of depression has become increasingly important in today's medical field.
Omega-3 polyunsaturated fatty acids (PUFAs) are currently an attractive candidate for the prevention and treatment of depressive symptoms (5, 6). Being an essential nutrient, humans cannot synthesize omega-3 PUFAs de novo, therefore, these fatty acids must be obtained through diet or supplementation. Fatty acids are the most abundant organic compounds in the brain, making up 60% of the dry weight, among which 20% of these fatty acids are PUFAs. The two most abundant PUFAs in the brain are omega-3 docosahexaenoic acid (DHA, C22:6 ω-3) and omega-6 PUFAs arachidonic acid (AA, C20:4 ω-6) (7). Brain function is heavily dependent on adequate omega-3 PUFAs levels. Omega-3 PUFAs, mainly DHA and eicosapentaenoic acid (EPA) which have strong anti-inflammatory and inflammation-resolving effects, also antagonizing the pro-inflammatory effects of omega-6 PUFAs which are the precursors of pro-inflammatory mediators. The balance between omega-6 PUFAs and omega-3 PUFAs is essential for homeostasis and the proper functioning of the central nervous system (CNS) to promote mental health and prevent neurological diseases (7, 8). Since omega-3 PUFAs and omega-6 PUFAs compete for incorporation into cell membranes, a balanced intake of these different type of PUFAs is essential (8). In modern society, human diets are unbalanced between omega-3 PUFAs and omega-6 PUFAs that may restrict the supply of omega-3 PUFAs to the tissues leading to a mild or severe omega-3 PUFAs deficiency in both developed and developing countries worldwide (9–12).
As an integral component of cell membranes, omega-3 PUFAs can increase membrane fluidity and permeability. Omega-3 PUFAs are largely esterified to the phospholipid in the cell membrane. Once omega-3 PUFAs are released from the membrane following neurotransmitter receptor-mediated activation of specific phospholipase A2 (PLA2) enzymes, they can act as secondary messengers and regulate signal transduction, either directly or indirectly by their bioactive derivatives (13, 14). Omega-3 PUFAs and their derivatives regulate various processes within the CNS, such as neuroinflammation, neurotransmission, synaptic plasticity, neurogenesis, neurodegeneration, and thereby mood and behavior. Omega-3 PUFAs deficiency are associated with many neurological disorders, including Alzheimer's disease, major depression and anxiety disorder (7, 14). There is a substantial body of evidence that provides general support for the beneficial effects of omega-3 PUFAs supplementation on brain structure and function in healthy human subjects (15).
There is a considerable amount of literature regarding the mechanism of action of omega-3 PUFAs to improve physical health and brain functioning [see, e.g., (8, 14, 16–18)], and many of them have been further considered as possible mechanisms for omega-3 PUFAs to improve depressive symptoms. Omega-3 PUFAs supplementation in depressed subjects has many structural and functional benefits for the brain, including promoting neurogenesis and neural repairment, preventing neuroinflammation and neurodegeneration, improving mood, cognition and memory etc., thus, exerting a wide range of ameliorating effects on depression (19–21). They are nutritious and safe, and when used in combination with other antidepressants, they can accelerate and increase efficacy significantly (5).
However, the antidepressant mechanisms of omega-3 PUFAs acting on the CNS are still not fully understood. Nowadays, there is limited data on human brain with respect to the antidepressant effect of omega-3 PUFAs. This paper, for the first time, makes a systematic review about the research progress in this field over the last decade, starting with the cellular and molecular basis of omega-3 PUFAs. This will provide information reference for the future research and clinical practice.
The cellular and molecular basis of omega-3 PUFAs
As shown in Figure 1, omega-3 PUFAs have a wide range of effects at the molecular and cellular levels, which may produce profound influences on mental health. We now summarize the main effects as follows:
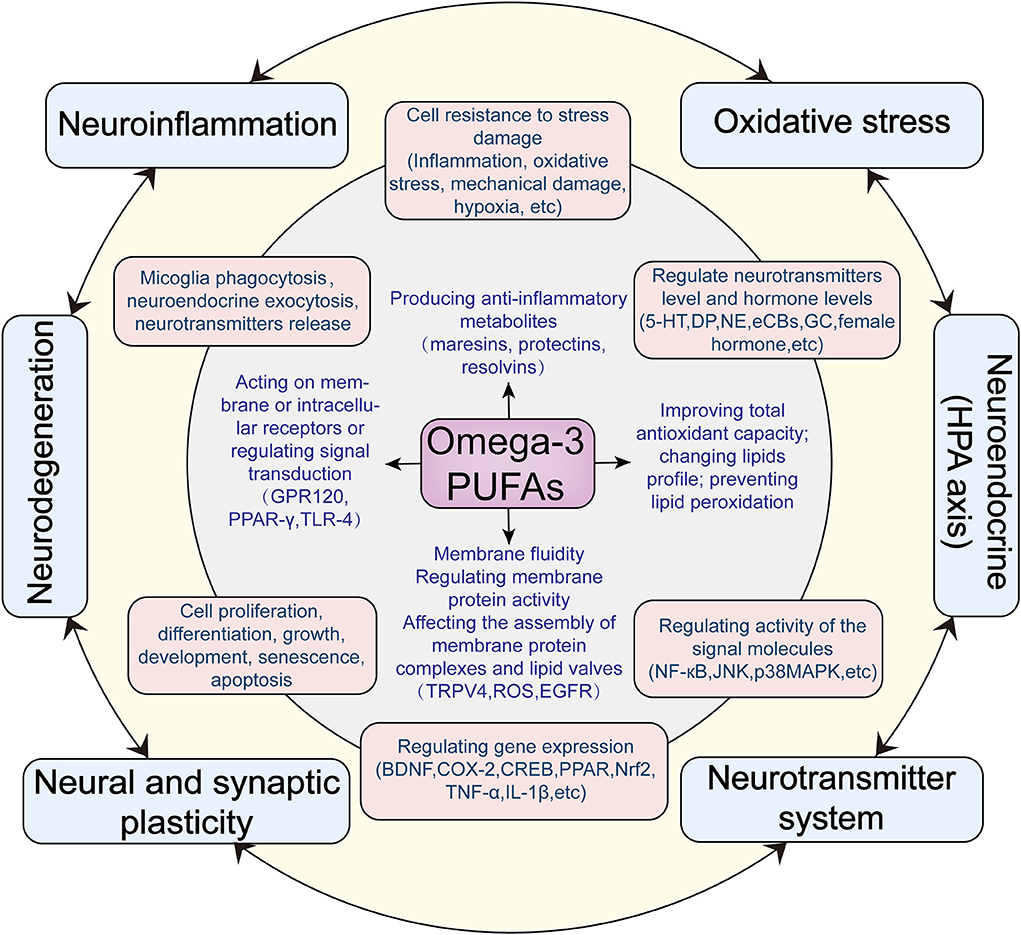
Figure 1. Hypothesized antidepressant mechanisms of omega-3 PUFAs acting on the central nervous system.
Increasing cell membrane fluidity and lipid bilayer elasticity, thereby influencing the structure of lipid microdomains, the interaction and function of proteins (including receptors, channel proteins, enzymes) in the membrane
Through optimizing the membrane fluidity, omega-3 PUFAs can improve the binding of neurotransmitter and their receptors and ion channel function in the membrane (22, 23). It has been reported that DHA can facilitate gamma-aminobutyric acid (GABA) systems binding and increase the rate of its receptor desensitization by modulating the elasticity of the lipid bilayer (24–26).
Stimulating cell membrane expansion and promoting membrane fusion
It has been found that by activating the plasma membrane protein syntaxin 3, omega-3 PUFAs can stimulate cell membrane expansion at the nerve growth cones, thereby promoting neurite outgrowth (27). As an enriched component in brain membrane phospholipids, DHA plays important roles in neurite outgrowth and neurotransmitter releases, the latter is a process involving membrane fusion and soluble N-ethylmaleimide-sensitive fusion factor attachment protein receptors (SNARE) complex binding or disassembly (23, 28).
Regulatory role on signal transduction
Omega-3 PUFAs can act as agonist ligands for G protein-coupled receptor 40 (GPR40) and G protein-coupled receptor 120 (GPR120), peroxisome proliferator-activated receptors (PPARs) and retinoid X receptor α (RXRA). In addition, omega-3 PUFAs can also downregulate nuclear factor kappa-B (NF-κB) through their inhibitory effects on toll-like receptor 4 (TLR4) or binding to peroxisome proliferator-activated receptor-γ (PPARγ) (29–31). Omega-3 PUFAs may exert neurological benefits through activating corresponding receptors such as GPR120, GPR40 as well as PPARs (23). Using RXRA conditional knockout mice, unesterfied DHA has been found to promote spinogenesis, synapse formation and transmission in vivo in a RXRA-dependent manner (32).
Regulating gene expression and epigenetic modifications
As previously reported, numerous brain genes expression was found to be regulated by omega-3 PUFAs supplementation (33). In addition, omega-3 PUFAs supplementation can modulate DNA methylation and histone modifications (34, 35). For example, high-mobility group box 1 (HMGB1), a nuclear regulator of gene expression, acts as an endogenous danger signal to activate inflammatory responses (36). Omega-3 PUFAs can prevent traumatic brain injury-induced inflammatory response through deacetylation of the HMGB1/NF-κB pathway.
Antagonizing inflammation and modulating immune response
Omega-3 PUFAs and their derivatives are multifunctional regulators of inflammation (37). Omega-3 PUFAs can competitively inhibit AA metabolic enzymes by competing with AA in vivo, thereby inhibiting AA mediated production of inflammatory substances. Omega-3 PUFAs plays important roles as the precursor of specialized pro-resolving mediators (SPMs), such as maresins, protectins, resolvins, and lipoxins, all of which are involved in the process of inflammation resolution (38). Omega-3 PUFAs can regulate nuclear transcription factors in the nucleus to inhibit the expression of inflammatory factors. By binding to the specific receptor such as GPR120, omega-3 PUFAs can downregulate the proinflammatory signal pathways, such as NF-κB and c-Jun N-terminal kinases (JNK)-related pathways (39, 40). Omega-3 PUFAs can also inhibit TLR4 and tumor necrosis factor receptor (TNFR), thereby inhibiting the expression of pro-inflammatory factors (32). As to cellular immune response, omega-3 PUFAs can also increase the expression of the macrophage or microglia M2 phenotype, thereby promoting the resolution of inflammation (41, 42).
Affecting mitochondrial function and reactive oxygen species homeostasis
Through changing mitochondrial membrane phospholipid composition and membrane viscosity, omega-3 PUFAs have various effects on mitochondrial function (i.e., membrane potential, respiration, individual complex activities, and ROS production). Supplementation with omega-3 PUFAs increases EPA or DHA while decreases omega-6 PUFAs in mitochondrial membrane, and can increase cardiolipin, a critical phospholipid for optimal mitochondrial function. Some literature reports that omega-3 PUFAs increases the antioxidant potential through increasing the activity of glutathione-related antioxidant enzyme and superoxide dismutase activity (43). As to the effect of omega-3 PUFAs supplementation on ROS production within the mitochondria, studies have shown inconsistent results possibly due to different experimental conditions (43–45).
Affecting cell proliferation, cell viability, cell repair or apoptosis
Many studies have reported that dietary omega-3 PUFAs improves neural viability, promotes the proliferation of neurocytes, benefits brain cell survival and repair, and inhibits apoptosis through neurotrophic, anti-apoptotic, and anti-inflammatory signaling (46–48). In addition, DHA can increase neurogenesis through influencing cell-fate decision of adult neural stem cells and survival of the newly born cells (49).
Effects of omega-3 PUFAs on depression
A number of epidemiological studies have shown that appropriate omega-3 PUFAs intake or higher serum omega-3 PUFAs are associated with lower risk of depression (50–52). Similarly, the depressed people exhibited lower levels of omega-3 PUFAs in blood samples than the health controls (53, 54). Furthermore, many human studies have also pointed out that the intake ratio of omega-3 PUFAs: omega-6 PUFAs is inversely associated with the risk of depressive symptoms (55, 56). Selective dietary deprivation of omega-3 PUFAs over several generations or post-weaning has consistently been shown to increase the expression of depression/anxiety-like behavior without affecting general locomotor activity in rodents. Some researchers have further suggested that omega-3 PUFAs index (which refers to the sum of EPA and DHA in red blood cells) may be used as potential treatment response marker for youthful depressed patients receiving omega-3 PUFAs (57). The expert consensus panel has reached up to consensus on using omega-3 PUFAs in the prevention and treatment of MDD subgroups such as pregnant women, children, and older adults (5).
Low levels of omega-3 PUFAs, particularly EPA, are found to be associated with depressive/anxious mood, low cognitive function, sleep disturbance, aggression and impulsive behaviors (58–61). Omega-3 PUFAs supplementation can improve many aspects of depressed patients including emotion regulation skills, cognitive function, sleep, and so on (58, 62–64). In addition, it has been reported that lower omega-3 PUFAs intake or serum omega-3 PUFAs levels are associated with greater risk of suicide attempt and MDD (65).
Several cross-sectional studies fail to find significant associations between omega-3 PUFAs and depressive symptoms (66, 67). This may mean that not all subtypes of depression are responsive to omega-3 PUFAs treatment. MDD is well recognized as a multifactorial disease which can be caused by biological, psychological or social factors. A simple lack of omega-3 PUFAs does not necessarily trigger depression, or sufficient omega-3 PUFAs can certainly avoid depression. The effects of omega-3 PUFAs on depression can be confounded by the etiology, physical constitution of patients, characteristic of fish oil (including purity, ratio of EPA to DHA), dose and duration of fish oil intake and so on.
Possible anti-depressant mechanisms of action of omega-3 PUFAs
Anti-neuroinflammatory effects of omega-3 PUFAs
Multiple lines of evidence have shown that there is a strong correlation between inflammation and depression. This evidence includes: (1) Depression is often accompanied by increased neuroinflammation (68). In the CNS, increased pro-inflammatory cytokines, which may come from the periphery or be produced from cells within the CNS, and activation of microglia as the resident immune cells of the brain are observed in MDD by imaging and post-mortem studies (69–73). (2) The central administration of exogenous inflammatory irritants can induce depressive symptoms (74, 75). (3) Chronic psychological or physiological stress results in neuroinflammation, which plays an important role in the occurrence of depression (76–79). (4) Neuroinflammation has close relationships with deficiency of monoamine neurotransmitter, dysfunction of brain neurotransmitters, hyperactivation of HPA axis, oxidative stress, neurodegeneration, cognitive dissonance and so on (80).
Omega-3 PUFAs and their derivates are effective in reducing neuroinflammation and has the therapeutic potential in treating neuroinflammation-related brain or mental diseases, such as Alzheimer's disease and substance abuse (81–83). In addition, it has been found that during development, deficiency of omega-3 PUFAs in diet dysregulates offspring's microglial homeostasis and increases microglial-driven inflammatory response, resulting in excessive synaptic pruning and subsequent behavioral abnormalities in mice (84).
It has been suggested that inflammation can be used as a predictive biomarker for response to omega-3 PUFAs in MDD (85). Both chronic inflammation and omega-3 PUFAs deficiency have often been found to be associated with MDD (86, 87). A number of studies have pointed out that omega-3 PUFAs (particular EPA) can probably exert some of their clinical effects via anti-inflammatory mechanisms of action, and patients with high inflammation levels have better improvement in depressive symptoms in response to omega-3 PUFAs supplementation (17, 88). Indirect evidences have pointed out that anti-neuroinflammatory mechanisms may be implicated in the antidepressant effects of omega-3 PUFAs (89). In vitro cell culture studies have shown that omega-3 PUFAs prevents the inflammatory response of microglia, which may be implicated in its antidepressant effects (90, 91). Moreover, supplementation of omega-3 PUFAs significantly reduced doxycycline (DOX)-induced neuroinflammation and effectively protected DOX-induced depressive behaviors (92).
Role of omega-3 PUFAs in the modulation of functions of HPA axis
Hyperactivity of HPA axis, with resulting high cortisol levels is commonly found in depressed patients. Disfunction of glucocorticoid receptors (GRs) which impair the HPA axis negative feedback is one of the main causes of HPA axis hyperactivity. The brain GR, especially expressed in the hypothalamic paraventricular nucleus, hippocampus and prefrontal cortex, are generally assumed to subserve the bulk of glucocorticoid feedback regulation of the HPA axis (93).
Preclinical and clinical data has reported that low plasma omega-3 PUFAs levels have correlation with higher corticotrophin-releasing factor (CRF) (94) and higher plasma cortisol (95–97), while supplementation with omega-3 PUFAs can reduce CRF expression and corticosterone secretion (98, 99). Healthy men treated with 3 weeks of fish oil intake show a decreased cortisol response to acute mental stress (100). As to high chronically stressed men subjects, omega-3 PUFAs phosphatidylserine supplementation is also found to improve the function of HPA axis (101). In a rat model of depression, corticosterone hypersecretion induced by chronic restraint stress is dampened by omega-3 PUFAs supplementation (102). On the aspects of the CNS, evidence shows that nutritional omega-3 deficiency dampens the GR signaling pathway in the PFC of mice, which is associated with dendritic arborization in PFC as well as emotional deficits (103). It has also been shown that omega-3 PUFA supplement ameliorates the decreased expression of GR in the hippocampus of parous rats induced by the omega-3 deficient diet, which may promote the hyperactivity of the HPA axis and postpartum depression (104).
The possible mechanism of omega-3 PUFAs in regulating HPA activity may be related to the fact that omega-3 PUFAs can significantly down-regulate the expression of inflammatory factor while increasing the negative feedback sensitivity of HPA axis. Indeed, inflammatory cytokines and their related signaling pathways have been well-known to inhibit GR function, further leading to attenuated negative-feedback inhibition of the HPA axis (105, 106). The anti-inflammatory properties of omega-3 PUFAs can thereby help to reduce the irritation of inflammatory stress on the secretion of CRF and thus inhibit HPA hyperactivity (107, 108). In rat cortical cultures, DHA treatment inhibits corticosterone-induced downregulation of GR expression on βIII- tubulin-positive neurons, which may contribute to the beneficial effect of DHA on ameliorating stress-induced neuronal damage (109). Up-regulation of GR expression by omega-3 PUFAs may also be related to down-regulation the expression of miRNA218 which is a post-transcriptional regulator of GR gene expression. Studies in female rats have reported that omega-3 PUFAs supplementation improve the maternal-pup separation-induced postpartum depression and post-menopausal depression, possibly involving the effects on HPA axis activities associated with reduced miRNA-218 expression and increased GR expression in the hippocampus (107, 110).
Anti-central oxidative stress effects of omega-3 PUFAs
Depression is often accompanied by excessive oxidative stress in the brain, which may lead to neurotoxicity and neuronal degenerative processes including decrease in neuroplasticity, neurogenesis, and an increase in apoptosis (111, 112). Many reasons including abundant O2.-/H2O2 by-products of mitochondrial respiration resulting from the brains extraordinary ATP demand, action potential dependent Ca2+ signaling-induced oxidative stress, glutamate (Glu)-induced excitotoxicity, extremely high content of unsaturated fatty acids and modest endogenous antioxidant defenses, make the brain especially vulnerable to oxidative stress (113–115). A number of the preclinical and clinical studies have reported increased oxidative biomarkers but lowered levels of antioxidants in the neurobiology of depression (116–118).
Evidence suggests that omega-3 PUFAs supplementation could attenuate oxidative stress in the brain (119–122), which might provide beneficial effects in depression prevention and treatment (51, 123). Postnatal omega-3 PUFAs supplementation can significantly enhance glutathione levels and reduce lipid peroxidation in the dentate gyrus and the cerebellum of prenatal ethanol exposure animals (124). A systematic review and meta-analysis of clinical trials have indicated that omega-3 PUFAs supplementation can enhance antioxidant defense through increasing serum total antioxidant capacity, glutathione peroxidase (GPx) activity, while reducing malondialdehyde levels (125). Oral administration of omega-3 prevents protein carbonylation and lipid peroxidation, and decreases the activity of myeloperoxidase, while improves the activities of superoxide dismutase and catalase in the brain of rats subjected to stress events (126). Using proton magnetic resonance spectroscopy, researchers found that the supplementation of 12-wk omega-3 decreases in vivo thalamus glutathione concentration in patients “at risk” for major depression (127). Several mechanisms can be suggested for the effect of omega-3 PUFAs on oxidative stress, including: (1) Increasing superoxide dismutase activity, elevating resistance to ROS damages and decreasing lipid peroxidation; (2) Inhibiting cyclooxygenase-2 (COX-2) enzyme activity. COX-2 metabolizes AA to inflammatory and oxidant prostaglandins which may promote lipid peroxidation; (3) Increasing the expression of nuclear factor-erythroid 2-related factor 2 (Nrf2) which is a transcriptional regulator that can effectively mediate antioxidant response by stimulating expression of the various antioxidant and anti-inflammatory genes (125, 128). In rat primary astrocytes, omega-3 PUFAs treatment can reduce ROS generation and enhance the antioxidant defense through Nrf2 activation under basal and oxidative stress conditions, suggesting that enrichment of astrocytes with omega-3 PUFAs may help to protect neurons in harmful conditions (129). Transcriptomic analyses of human hippocampal progenitor cell show that both EPA and DHA treatment regulates immune response pathways and Nrf2-mediated antioxidant pathways, which may be the molecular mechanisms underlying the preventive effect of omega-3 PUFAs on cortisol-induced decrease in neurogenesis and increase in apoptosis (130).
It has been found that only among participants with increased oxidative stress biomarkers, the omega-3 PUFAs index is negatively correlated with depressive symptoms, suggesting that oxidative stress status may be taken as a potential predictor of response to omega-3 PUFAs treatment of depression (131). Moreover, evidence indicates that omega-3 PUFAs may be more effective in improving the depressive symptoms of coronary heart disease patients with higher levels of oxidative stress marker (132). Omega-3 PUFAs can also prevent the brain's oxidative damage by decreasing the levels of protein carbonylation, lipid peroxidation, and the concentrations of nitrite/nitrate, and reducing myeloperoxidase activity, while increasing superoxide dismutase and catalase activities, which may contribute to the inhibitory effects of omega-3 PUFAs on the depressive-like behavior of the rats subjected to early or late life stress (126).
Anti-neurodegenerative effects of omega-3 PUFAs
Neurodegenerative disorders have been closely related to depression (133). The physiological factors underlying various neurodegenerative changes include: increased inflammation level, enhanced oxidative stress damage, decreased secretion of the brain-derived neurotrophic factor (BDNF) and excessive glucocorticoid level associated with the chronic stress. The evidence of the correlation between neurodegeneration and depression can be mainly derived from the following aspects: (1) Hippocampal and the pre-frontal cortex (PFC) volume has been observed to be consistently reduced in the depressed patients (134–136); (2) Decreased levels of BDNF, dendritic atrophy, decreased neurogenesis, and increased neuronal death in patients with depression (137, 138); (3) Depression and other neurodegenerative diseases can exhibit a high co-morbidity (139). Previous epidemiological studies have shown that the co-morbidity rate of depression and Alzheimer's disease was about 40% (140), and the co-morbidity rate of Parkinson's disease was about 30% (141).
Omega-3 PUFAs play important roles in preventing neurodegeneration by inhibiting neuroinflammation, promoting synaptic plasticity and neurogenesis, facilitating nervous system repair, and protecting against the reduction of gray matter volume and the decline of white matter integrity (19, 142, 143). Studies have found that omega-3 PUFAs can promote the proliferation and migration of nerve cells, and inhibit apoptosis (48, 144). According to a ten-year follow-up study, higher levels of plasma EPA and DHA are associated with a slower decline in medial temporal lobe volume and a lower risk of dementia in older adults (145). Higher blood EPA level is found to have correlations with lower gray matter atrophy in the right amygdala, while higher atrophy of the right amygdala is correlated with more severe depressive symptoms (146).
Omega-3 PUFAs can prevent neurodegeneration through modulating a variety of pathways, including anti-apoptotic (147, 148), anti-oxidative (149–151), and anti-inflammatory pathways (152). Omega-3 PUFAs supplementation have been also found to increase the synthesis of neutrophic factor BDNF (153–155). BDNF plays important role in protecting against neurodegeneration and promoting neuronal plasticity, thereby having potential in depression treatment (156, 157). In addition, changes in the activities of telomerase and mammalian target of rapamycin (mTOR) may also be involved in the anti-neurodegeneration actions of omega-3 PUFAs (158, 159). Clinical trials have also confirmed that omega-3 PUFAs can effectively improve neurodegenerative diseases (such as Alzheimer's and Parkinson's diseases) with an associated improvement of the co-morbid depressive symptoms (160).
Pro-neuroplastic and pro-synaptic plasticity effects of omega-3 PUFAs
Depression is deeply connected with irregular neural plasticity processes which are often found in the prefrontal cortex, hippocampus, amygdala and other limbic systems (161). Impaired neural plasticity can be primarily reflected in decreased neurogenesis, reduced dendritic spine density, decreased synapse number and strength, reduced synaptic remodeling, dendritic atrophy, as well as reward circuit dysregulation (162–166). At present, it has been found that the vast majority of antidepressant treatments, including physical therapy (such as electroconvulsive therapy, transcranial direct current stimulation, and transcranial alternating current stimulation) and drug therapy (such as fluoxetine, ketamine, and TJZL184), can primarily exert their antidepressant effects by regulating neural plasticity (167–170).
Omega-3 PUFAs have been shown to promote neuroplasticity in multiple ways. DHA supplementation could effectively promote neurogenesis by increasing the proliferation of neural stem/progenitor cells (NSPCs) as well as the number of NSPCs differentiating into the neurons and promoting the survival of newly born neurons (49). Moreover, increasing the brain levels of omega-3 PUFAs can increase the synthesis of new dendritic spines and synapses (171). The underlying mechanisms for the pro-neurogenesis effect of omega-3 PUFAs may be related to activation of proliferation-related pathways involving signaling molecular including GPR40, p38 MAPK, cAMP-response element binding protein (CREB), and BDNF (172–174). By using transgenic fat-1 mice rich in endogenous omega-3 PUFAs, researchers have found that substantial increase in brain DHA can significantly promote hippocampal neurogenesis and increase the genesis of dendritic spines of CA1 pyramidal neurons (172).
Omega-3 PUFAs supplementation could also regulate synaptic formation, synaptic transmission and affect synaptic plasticity (32, 171, 175). Omega-3 PUFAs may play an important role in regulating the expression of several important neural and glial proteins such as E-cadherin, early growth response 1, postsynaptic density protein 95, and signaling factors that have been implicated in synaptic plasticity [such as N-methyl-D-aspartic acid (NMDA) receptor and Fyn] (176). It has been reported that omega-3 PUFAs deficiency can reduce long-term potentiation (LTP), the concentrations of glutamate receptor subunits, and synaptic vesicle proteins at the hippocampal glutamatergic synapses (177).
In addition, DHA and EPA can be converted into endocannabinoids (eCBs) docosahexaenoyl ethanolamide (DHEA) and eicosapentaenoyl ethanolamide (EPEA) which exerts physiological effects through activating eCB receptors. DHEA and EPEA have been reported to exhibit various immunomodulatory and anti-inflammatory activities and effects on food intake and mood (20, 178). The eCBs can induce short-term changes and long-term synaptic plasticity in the whole nervous system, because eCBs synthesized in the postsynaptic neurons can function reversely to regulate the presynaptic input (179). Maternal omega-3 PUFAs deficiency can induce the impairment of eCBs gating of LTP in hippocampus of weaned pups (180). It has been also found that life-long omega-3 PUFAs deficiency can lead to the specific inhibition of eCBs-mediated long-term synaptic depression in the prelimbic prefrontal cortex and the accumbens of adult mice. This effect is accompanied by decreased CB1 receptor function which is associated with impaired emotional behavior (181).
Neurotransmitter system modulatory effects of omega-3 PUFAs
The neurotransmitter and neurotransmitter receptor hypothesis of depression propose that various disorders in multiple neurotransmitter systems are involved in etiopathology of depression. Emerging literature has also shown that depression might be associated with the various molecular abnormalities and functional deficiency in brain transmitter systems including that in 5-hydroxytryptamine (5-HT), dopamine (DA), norepinephrine (NE), Glu and GABA (157). Accumulating evidences indicate that the neurotransmitter transmission predominantly depends on adequate level of omega-3 PUFAs or optimal omega-6 PUFAs: omega-3 PUFA ratio in the brain (23). Being rich in membranes of the synaptic terminals, DHA has been considered to be important for the function of neurochemical transmission. Low availability of omega-3 PUFAs can influence the synthesis, synaptic release, uptake of multiple neurotransmitters including 5-HT, DA, NE, Glu and GABA.
Accumulating evidences indicate that the normal functional activities of neurotransmitter systems depend on adequate levels of omega-3 PUFAs in the brain (23, 182). Omega-3 PUFAs have wide effects on the synthesis, synaptic release, uptake of multiple neurotransmitters including 5-HT, DA, NE, Glu and GABA (23, 183, 184). It has been pointed out that improving the transmission of 5-HT and DA; reducing 5-HT2 receptor and increasing D2 receptor in the frontal cortex may be the possible mechanisms underlying the beneficial effects of omega-3 PUFAs on depression (23). It is reported that fish-oil supplementation produces an antidepressant-like effect in LPS-induced depression model and this effect is related to decreased expression of indoleamine-2,3-Dioxygenase and elevated 5-HT levels in the hippocampus (185). Omega-3 PUFAs can also influence the expression levels of multiple neurotransmitter receptors. It has been shown that DHA supplementation can prevent the increase of binding density of 5-TH receptors (5-HT1A and 5-HT2A), CB1 and GABA-A receptors induced by the high saturated fat diet, which have been related to the cognitive function of the brain (186).
In addition, previous studies have demonstrated that omega-3 PUFAs deficiency can aggravate the age-associated decrease in glutamatergic synaptic efficacy in the hippocampal CA1 (187). It can further affect the glutamatergic synapse development and anxiety-like behavior in male adult rats (188). It has also been found to decrease the subunits of NMDA receptors NR2A, NR2B in rodents (188–190). Similarly, omega-3 PUFAs deficiency also leads to decreased concentrations of Glu receptor subunits (GluA1, GluA2 and NR2B) and other synaptic vesicle proteins in the hippocampal synaptosomes of mice (177).
Preclinical and clinical findings have suggested that eCBs/CB1R signaling can contribute to depression risk and omega-3 PUFAs can exert the anti-depressant effects through altering the PUFA-derived eCBs levels in the whole brain (181, 191–193). It has been reported that omega-3 PUFAs supplementation can increase plasma DHEA and EPEA levels and increased EPEA levels are positively related to the clinical remission rate of MDD patients (180).
The correlations of the six mechanisms of omega-3 PUFAs action
These six aspects of omega-3 PUFAs action may not play independent parts, but just like different aspects of the same thing they probably work in an interconnected system. The simplified model for the assumed interconnection of the six mechanisms are shown in Figure 1. Neuroinflammation and oxidative stress often flame each other and have been considered as the major causes of neurodegeneration followed by MDD (194). Chronic stress-induced hyperactivation of the HPA-axis and neuroinflammation can create a vicious cycle, lead to dysfunction of neurotransmitters system, impair neuroplasticity and promote neurodegeneration (195–199). It has been indicated that HPA axis hyperactivation causes inflammation response in MDD. Immunological communication between the CNS and the body periphery triggers neuroinflammation, which further induces the failure of glucocorticoid negative feedback within the brain. In addition, inflammatory factors activate the kynurenine pathway resulting in the reduction of serotonin biosynthesis and the increased production of neurotoxic metabolites, and eventually neurodegeneration (198). There are lots of papers which have reviewed the correlations between neuroinflammation and neural or synaptic plasticity, neuroinflammation and neurotransmitter systems, neurotransmitter systems and synaptic plasticity, which can be referred to the following references (200–203). We also summarized the experimental designs and results of animal studies reported in the references in this paper. As shown in Table 1.
According to research results, omega-3 PUFAs deficiency often leads to dysfunction of multiple neurobiological systems, such as neuroinflammation, inactivated GR signaling pathway and HPA axis hyperactivity, deteriorated serotoninergic, noradrenalinergic and dopaminergic neurotransmission, impaired neurogenesis, neurodegeneration and so on (96, 103, 208). It has been found that chronic dietary omega-3 PUFAs deficiency led to a significant reduction in 5-HT and NA content, increased production of kynurenine, along with HPA axis hyperactivity, higher proinflammatory cytokine production associated with higher expression of TLR2 and TLR4 and increased expression of oligomeric Aβ in hippocampus of female rats (209).
Both clinical and pre-clinical evidences have shown that the alterations of many different aspects of the CNS are involved in the anti-depressant effect of omega-3 PUFAs supplementation (63, 88, 89, 130, 184, 205, 206, 208, 210–213). Therefore, the six antidepressant mechanisms of omege-3 PUFAs that we summarized probably act synergistically but not separately. However, there is currently no clear conclusion on how these mechanisms are linked or how they interact, and whether there are causal links. All these questions require further studies to be answered.
Based on existing evidence, we summarized a variety of central mechanisms of omega-3 PUFAs anti-depressant actions, from molecular mechanisms to cellular mechanisms to neurobiological mechanisms. At the molecular level, omega-3 PUFAs directly changes lipid profiles, regulating membrane fluidity and membrane-associated cellular processes such as the assembly of membrane protein complexes and lipid valves; neurotransmitters and neuroendocrine exocytosis as well as microglia phagocytosis. Through metabolism and acting on the plasma membrane or intracellular receptors, omega-3 PUFAs can improve total antioxidant capacity; prevent lipid peroxidation: produce anti-inflammatory metabolites and regulate inflammatory signal pathways. At the cellular level, omega-3 PUFAs indirectly affect a wide range of cellular activities, such as the cell resistance to stress damage; various signaling transduction pathways; the neurotransmitter systems; the whole-genome expression profile; cell proliferation, differentiation, growth, development, senescence, apoptosis and so on. Based on its molecular and cellular mechanisms at the neurobiological level, omega-3 PUFAs exert a widespread and far-reaching influence on the mood regulating function of the central nervous system. Omega-3 PUFAs can improve neuroinflammation; dysfunction of neuroendocrine (HPA axis); oxidative stress; neurodegeneration; neuroplasticity; neurotransmitters system and so on. All these effects may play roles in the prevention and improvement of depression. Abbreviations: TRPV4, Transient receptor potential vanilloid 4; ROS, Reactive oxygen species; EGFR, Epidermal growth factor receptor; GPR120,G-protein coupled receptor 120; PPARγ, Peroxisome proliferator-activated receptor-gamma; TLR4, Toll-like receptor 4; COX-2, Cyclooxygenase-2; BDNF, Brain-derived neurotrophic factor; CREB, cAMP-response element binding protein; PPAR, peroxisome proliferator-activated receptor; Nrf2, nuclear factor-erythroid 2-related factor 2; 5-HT, 5-hydroxytryptamine; NE, Norepinephrine; CB, Cannabinoids; GC, Glucocorticoid; NF-κB, nuclear factor kappa-B; JNK, c-Jun N-terminal kinases; p38MAPK, p38 mitogen-activated protein kinase.
Future directions
The pathological mechanisms of depression have been found to be closely associated with multiple aspects of neural functions. Currently, omega-3 PUFAs are a kind of molecules of diverse biological activities, capable of producing multiple antidepressant effects in the CNS. The six possible mechanisms summarized in this review are probably interconnected in complex manner and can function synergistically to produce the anti-depressant effects. However, there is a critical need for well-designed systematic researches to identify the eventual unitary anti-depression mechanism from various different actions of omega-3 PUFAs.
Although there have been numerous studies published that have demonstrated the regulation of various physiological functions by omega-3 PUFAs in depressive disorders, there are still insufficient reports related to the causal mechanisms of omega-3 PUFAs anti-depressant action. Omega-3 PUFAs can have diverse regulatory effects on neurogenesis, synaptic plasticity, oxidative stress, neuroendocrine, and neurotransmitter transduction, but the specific molecular regulatory pathways remain largely unclear. In addition, it is also unclear to how these PUFAs affect depressive symptoms from the molecular to the behavioral level. To answer this question may depend on the thorough understanding about the role of omega-3 PUFAs in human life as well as the pathophysiological nature of depression.
It is still unclear which ones are direct or indirect mechanisms; which ones are the primary effects and which ones are the secondary mechanisms of the anti-depressant effect of omega-3 PUFAs. Clarifying these issues which will help establish the central pharmacological action and pharmacodynamics of omega-3 PUFAs.
In order to facilitate translation to application, future research may need to elucidate the anti-depressant mechanism of omega-3 PUFAs action by using quantitative systems pharmacology and to identify the clinical biomarkers and the antidepressant-response biomarkers in target subgroups of depressed patients.
Moreover, omega-3 PUFAs may play different roles in different depressed patients with different constitutions. Further studies should be conducted to explore the potential different mechanisms of the action of omega-3 PUFAs on depression in children, adolescents, postpartum women, and eldly adults or depressed patients with concomitant physical diseases such as cardiovascular disorders. This will help the personalized application of omega-3 PUFAs in different subgroups of MDD.
In addition, according to ISNPR's 2019 practice guideline for the assisted treatment of depression with omega-3 PUFAs, omega-3 PUFAs are found to be more effective as an adjuvant treatment than monotherapy for MDD treatment. So, what are the specific mechanisms by which omega-3 PUFAs can accelerate or enhance effects of other antidepressants? This requires further studies to explore the exact mechanisms underlying greater efficacy of clinical antidepressants in combination with omega-3 PUFAs. This may be the good news for clinical use of antidepressants that act quickly but are often associated with side effects, or work too slowly, or do not work significantly.
Conclusions
This article provides a review of the neurobiological mechanisms underlying the antidepressant effects of omega-3 PUFAs. Based on the accumulated evidence from recent publications, we identified six potential mechanisms, including: (1) anti-neuroinflammatory; (2) anti-oxidative stress; (3) modulation of HPA axis; (4) anti-neurodegeneration; (5) neuroplasticity and synaptic plasticity; and (6) modulation of neurotransmitter systems. All these antidepressant mechanisms may be based on the molecular action and cellular effects of omega-3 PUFAs, however, how these processes work remains largely unknown. Although neurobiological mechanisms are probably interconnected and interdependent, the multiple sites of action of omega-3 PUFAs are still needed to be clarified. This review contributes to a better understanding the potential mechanisms of benefit of omega-3 PUFA and may provide useful references for the development of new strategies for the treatment of depressive disorder with omega-3 PUFAs.
Author contributions
J-TZ was responsible for the study conception and design and the writing and revising of the manuscript. LZ performed the collection, the writing of the manuscript, and provided the technique support. BL made revisions and polishing to the paper and provided the funding support. J-YX and Y-QC made material support and grammar checking. All authors contributed to and have approved the final manuscript.
Funding
This study was supported by Scientific Research Project of Education Department of Hubei Province (B2021048).
Acknowledgments
The authors thank Prof. Wen-Juan Lin, Institute of Psychology, Chinese Academy of Sciences, Beijing, China for writing consultant and Prof. Mao-Sheng Ran, Department of Social Work and Social Administration, The University of Hong Kong, Hong Kong SAR for writing revising.
Conflict of interest
The authors declare that the research was conducted in the absence of any commercial or financial relationships that could be construed as a potential conflict of interest.
Publisher's note
All claims expressed in this article are solely those of the authors and do not necessarily represent those of their affiliated organizations, or those of the publisher, the editors and the reviewers. Any product that may be evaluated in this article, or claim that may be made by its manufacturer, is not guaranteed or endorsed by the publisher.
References
1. Kessler RC, Barber C, Birnbaum HG, Frank RG, Greenberg PE, Rose RM, et al. Depression in the workplace: effects on short-term disability. Health Aff (Millwood). (2017) 18:163. doi: 10.1377/hlthaff.18.5.163
2. WHO. Depression. World Health Organization. (2021). Available online at: https://www.who.int/news-room/fact-sheets/detail/depression (accessed September 13, 2021).
3. Chen X, Zhang X, Zhu X, Wang G. Efficacy of an internet-based intervention for subclinical depression (Moodbox) in China: study protocol for a randomized controlled Trial. Front Psychiatry. (2021) 11:585920. doi: 10.3389/fpsyt.2020.585920
4. Wang X, Lin H, Jiang X, Ma M, Shi D, Fan C, et al. Effect of Electroacupuncture and counseling on sub-threshold depression: a study protocol for a multicenter randomized controlled trial. Front Psychiatry. (2020) 11:346. doi: 10.3389/fpsyt.2020.00346
5. Guu TW, Mischoulon D, Sarris J, Hibbeln J, McNamara RK, Hamazaki K, et al. International society for nutritional psychiatry research practice guidelines for omega-3 fatty acids in the treatment of major depressive disorder. Psychother Psychosom. (2019) 88:263–73. doi: 10.1159/000502652
6. Mocking RJT, Harmsen I, Assies J, Koeter MWJ, Ruhé HG, Schene AH. Meta-analysis and meta-regression of omega-3 polyunsaturated fatty acid supplementation for major depressive disorder. Transl Psychiatry. (2016) 6:e756. doi: 10.1038/tp.2016.29
7. Lange KW. Omega-3 fatty acids and mental health. Global Health J. (2020) 4:18–30. doi: 10.1016/j.glohj.2020.01.004
8. Wysoczański T, Sokoła-Wysoczańska E, Pekala J, Lochyński S, Czyz K, Bodkowski R, et al. Omega-3 fatty acids and their role in central nervous system - a review. Curr Med Chem. (2016) 23:816–31. doi: 10.2174/0929867323666160122114439
9. Coltell O, Sorlí JV, Asensio EM, Barragán R, González JI, Giménez-Alba IM, et al. Genome-wide association study for serum omega-3 and omega-6 polyunsaturated fatty acids: exploratory analysis of the sex-specific effects and dietary modulation in mediterranean subjects with metabolic syndrome. Nutrients. (2020) 12:310. doi: 10.3390/nu12020310
10. Stark KD, Van Elswyk ME, Higgins MR, Weatherford CA, Salem N. Global survey of the omega-3 fatty acids, docosahexaenoic acid and eicosapentaenoic acid in the blood stream of healthy adults. Prog Lipid Res. (2016) 63:132–52. doi: 10.1016/j.plipres.2016.05.001
11. O' Donovan F, Carney S, Kennedy J, Hayes H, Pender N, Boland F, et al. Associations and effects of omega-3 polyunsaturated fatty acids on cognitive function and mood in healthy adults: a protocol for a systematic review of observational and interventional studies. BMJ Open. (2019) 9:e027167. doi: 10.1136/bmjopen-2018-027167
12. Micha R, Khatibzadeh S, Shi P, Fahimi S, Lim S, Andrews KG, et al. Global, regional, and national consumption levels of dietary fats and oils in 1990 and 2010: a systematic analysis including 266 country-specific nutrition surveys. BMJ. (2014) 348:g2272. doi: 10.1136/bmj.g2272
13. Figlewicz DP, Witkamp RF. Fatty acids as cell signals in ingestive behaviors. Physiol Behav. (2020) 223:112985. doi: 10.1016/j.physbeh.2020.112985
14. Bazinet RP, Layé S. Polyunsaturated fatty acids and their metabolites in brain function and disease. Nat Rev Neurosci. (2014) 15:771–85. doi: 10.1038/nrn3820
15. McNamara RK, Asch RH, Lindquist DM, Krikorian R. Role of polyunsaturated fatty acids in human brain structure and function across the lifespan: an update on neuroimaging findings. Prostaglandins Leukot Essent Fatty Acids. (2018) 136:23–34. doi: 10.1016/j.plefa.2017.05.001
16. Janssen CIF, Kiliaan AJ. Long-Chain polyunsaturated fatty acids (Lcpufa) from genesis to senescence: the influence of Lcpufa on neural development, aging, and neurodegeneration. Prog Lipid Res. (2014) 53:1–17. doi: 10.1016/j.plipres.2013.10.002
17. McNamara RK, Almeida DM. Omega-3 polyunsaturated fatty acid deficiency and progressive neuropathology in psychiatric disorders: a review of translational evidence and candidate mechanisms. Harv Rev Psychiatry. (2019) 27:94. doi: 10.1097/HRP.0000000000000199
18. Shahidi F, Ambigaipalan P. Omega-3 polyunsaturated fatty acids and their health benefits. Annu Rev Food Sci Technol. (2018) 9:345–81. doi: 10.1146/annurev-food-111317-095850
19. Cutuli D. Functional and structural benefits induced by omega-3 polyunsaturated fatty acids during aging. Curr Neuropharmacol. (2017) 15:534–42. doi: 10.2174/1570159X14666160614091311
20. Kalkman HO, Hersberger M, Walitza S, Berger GE. Disentangling the molecular mechanisms of the antidepressant activity of omega-3 polyunsaturated fatty acid: a comprehensive review of the literature. Int J Mol Sci. (2021) 22:4393. doi: 10.3390/ijms22094393
21. Grosso G, Pajak A, Marventano S, Castellano S, Galvano F, Bucolo C, et al. Role of omega-3 fatty acids in the treatment of depressive disorders: a comprehensive meta-analysis of randomized clinical trials. PLoS ONE. (2014) 9:e96905. doi: 10.1371/journal.pone.0096905
22. Bruno MJ, Koeppe RE, Andersen OS. Docosahexaenoic acid alters bilayer elastic properties. Proc Natl Acad Sci U S A. (2007) 104:9638–43. doi: 10.1073/pnas.0701015104
23. DiNicolantonio JJ, O'Keefe JH. The importance of marine omega-3s for brain development and the prevention and treatment of behavior, mood, and other brain disorders. Nutrients. (2020) 12:2333. doi: 10.3390/nu12082333
24. Søgaard R, Werge TM, Bertelsen C, Lundbye C, Madsen KL, Nielsen CH, et al. Gaba(a) receptor function is regulated by lipid bilayer elasticity. Biochemistry. (2006) 45:13118–29. doi: 10.1021/bi060734+
25. Guixà-González R, Javanainen M, Gómez-Soler M, Cordobilla B, Domingo JC, Sanz F, et al. Membrane omega-3 fatty acids modulate the oligomerisation kinetics of adenosine A2a and dopamine D2 receptors. Sci Rep. (2016) 6:19839. doi: 10.1038/srep19839
26. Yehuda S, Rabinovitz S, Carasso RL, Mostofsky DI. The role of polyunsaturated fatty acids in restoring the aging neuronal membrane. Neurobiol Aging. (2002) 23:843–53. doi: 10.1016/S0197-4580(02)00074-X
27. Darios F, Davletov B. Omega-3 and omega-6 fatty acids stimulate cell membrane expansion by acting on syntaxin 3. Nature. (2006) 440:813–7. doi: 10.1038/nature04598
28. Pongrac JL, Slack PJ, Innis SM. Dietary polyunsaturated fat that is low in (N-3) and high in (N-6) fatty acids alters the snare protein complex and nitrosylation in rat hippocampus. J Nutr. (2007) 137:1852–6. doi: 10.1093/jn/137.8.1852
29. Jalili M, Hekmatdoost A. Dietary Ω-3 fatty acids and their influence on inflammation via toll-like receptor pathways. Nutrition. (2021) 85:111070. doi: 10.1016/j.nut.2020.111070
30. Hilgendorf KI, Johnson CT, Mezger A, Rice SL, Norris AM, Demeter J, et al. Omega-3 fatty acids activate ciliary Ffar4 to control adipogenesis. Cell. (2019) 179:1289–305. doi: 10.1016/j.cell.2019.11.005
31. Wang Y, Xiang Y, Xin VW, Wang XW, Peng XC, Liu XQ, et al. Dendritic cell biology and its role in tumor immunotherapy. J Hematol Oncol. (2020) 13:107. doi: 10.1186/s13045-020-00939-6
32. Cao H, Li MY, Li G, Li SJ, Wen B, Lu Y, et al. Retinoid X receptor A regulates dha-dependent spinogenesis and functional synapse formation in vivo. Cell Rep. (2020) 31:107649. doi: 10.1016/j.celrep.2020.107649
33. Sopian NFA, Ajat M, Shafie NI, Noor MHM, Ebrahimi M, Rajion MA, et al. Does short-term dietary omega-3 fatty acid supplementation influence brain hippocampus gene expression of zinc transporter-3? Int J Mol Sci. (2015) 16:15800–10. doi: 10.3390/ijms160715800
34. Chen X, Pan Z, Fang Z, Lin W, Wu S, Yang F, et al. Omega-3 polyunsaturated fatty acid attenuates traumatic brain injury-induced neuronal apoptosis by inducing autophagy through the upregulation of sirt1-mediated deacetylation of beclin-1. J Neuroinflammation. (2018) 15:1–15. doi: 10.1186/s12974-018-1345-8
35. Lee HS, Barraza-Villarreal A, Hernandez-Vargas H, Sly PD, Biessy C, Ramakrishnan U, et al. Modulation of DNA methylation states and infant immune system by dietary supplementation with Ω-3 Pufa during pregnancy in an intervention study. Am J Clin Nutr. (2013) 98:480–7. doi: 10.3945/ajcn.112.052241
36. Wang Y, Zhong J, Zhang X, Liu Z, Yang Y, Gong Q, et al. The role of Hmgb1 in the pathogenesis of type 2 diabetes. J Diabetes Res. (2016) 2016:2543268. doi: 10.1155/2016/2543268
37. Larrieu T, Layé S. Food for mood: relevance of nutritional omega-3 fatty acids for depression and anxiety. Front Physiol. (2018) 9:1047. doi: 10.3389/fphys.2018.01047
38. Serhan CN, Chiang N, Dalli J. New pro-resolving N-3 mediators bridge resolution of infectious inflammation to tissue regeneration. Mol Aspects Med. (2018) 64:1–17. doi: 10.1016/j.mam.2017.08.002
39. Huang Q, Wang T, Wang HY. Ginsenoside Rb2 enhances the anti-inflammatory effect of Ω-3 fatty acid in Lps-Stimulated Raw2647 macrophages by upregulating Gpr120 expression. Acta Pharmacol Sin. (2017) 38:192–200. doi: 10.1038/aps.2016.135
40. Im D-S. Functions of omega-3 fatty acids and Ffa4 (Gpr120) in macrophages. Eur J Pharmacol. (2016) 785:36–43. doi: 10.1016/j.ejphar.2015.03.094
41. Liu B, Zhang Y, Yang Z, Liu M, Zhang C, Zhao Y, et al. Ω-3 Dpa protected neurons from neuroinflammation by balancing microglia M1/M2 polarizations through inhibiting Nf-Kb/Mapk P38 signaling and activating neuron-Bdnf-Pi3k/Akt pathways. Mar Drugs. (2021) 19:587. doi: 10.3390/md19110587
42. Gutiérrez S, Svahn SL, Johansson ME. Effects of omega-3 fatty acids on immune cells. Int J Mol Sci. (2019) 20:5028. doi: 10.3390/ijms20205028
43. Sullivan EM, Pennington ER, Green WD, Beck MA, Brown DA, Shaikh SR. Mechanisms by which dietary fatty acids regulate mitochondrial structure-function in health and disease. Adv Nutr. (2018) 9:247–62. doi: 10.1093/advances/nmy007
44. Stanley WC, Khairallah RJ, Dabkowski ER. Update on lipids and mitochondrial function: impact of dietary N-3 polyunsaturated fatty acids. Curr Opin Clin Nutr Metab Care. (2012) 15:122–6. doi: 10.1097/MCO.0b013e32834fdaf7
45. Zhang Y, Jiang L, Hu W, Zheng Q, Xiang W. Mitochondrial dysfunction during in vitro hepatocyte steatosis is reversed by omega-3 fatty acid-induced up-regulation of Mitofusin 2. Metabolism. (2011) 60:767–75. doi: 10.1016/j.metabol.2010.07.026
46. Cardoso C, Afonso C, Bandarra NM. Dietary Dha and health: cognitive function ageing. Nutr Res Rev. (2016) 29:281–94. doi: 10.1017/S0954422416000184
47. Gómez-Soler M, Cordobilla B, Morató X, Fernández-Dueñas V, Domingo JC, Ciruela F. Triglyceride form of docosahexaenoic acid mediates neuroprotection in experimental parkinsonism. Front Neurosci. (2018) 12:604. doi: 10.3389/fnins.2018.00604
48. Shi JP, Fu W, Liu J. −3 Pufa attenuates Lps-induced neuro-injury of neonatal rats through the Pi3k/Akt pathway. Neuroscience. (2019) 414:112–27. doi: 10.1016/j.neuroscience.2019.06.027
49. Lo Van A, Hachem M, Lagarde M, Bernoud-Hubac N. Omega-3 docosahexaenoic acid is a mediator of fate-decision of adult neural stem cells. Int J Mol Sci. (2019) 20:4240. doi: 10.3390/ijms20174240
50. Grosso G, Micek A, Marventano S, Castellano S, Mistretta A, Pajak A, et al. Dietary N-3 Pufa, fish consumption and depression: a systematic review and meta-analysis of observational studies. J Affect Disord. (2016) 205:269–81. doi: 10.1016/j.jad.2016.08.011
51. Sánchez-Villegas A, Álvarez-Pérez J, Toledo E, Salas-Salvadó J, Ortega-Azorín C, Zomeño MD, et al. Seafood consumption, omega-3 fatty acids intake, and life-time prevalence of depression in the predimed-plus trial. Nutrients. (2018) 10:2000. doi: 10.3390/nu10122000
52. Murphy RA, Devarshi PP, Ekimura S, Marshall K, Mitmesser SH. Serum long chain omega-3 fatty acids and depression among adults in the united states: an analysis of Nhanes 2011–2012. J Affect Disorders. (2021) 4:100089. doi: 10.1016/j.jadr.2021.100089
53. Trebatická J, Hradečná Z, Surovcová A, Katrenčíková B, Gushina I, Waczulíková I, et al. Omega-3 fatty-acids modulate symptoms of depressive disorder, serum levels of omega-3 fatty acids and omega-6/omega-3 ratio in children. A randomized, double-blind and controlled trial. Psychiatry Res. (2020) 287:112911. doi: 10.1016/j.psychres.2020.112911
54. Thesing CS, Bot M, Milaneschi Y, Giltay EJ, Penninx BW. Bidirectional longitudinal associations of omega-3 polyunsaturated fatty acid plasma levels with depressive disorders. J Psychiatr Res. (2020) 124:1–8. doi: 10.1016/j.jpsychires.2020.02.011
55. Zhang R, Sun J, Li Y, Zhang D. Associations of N-3, N-6 fatty acids intakes and N-6: N-3 ratio with the risk of depressive symptoms: Nhanes 2009–2016. Nutrients. (2020) 12:240. doi: 10.3390/nu12010240
56. Lin PY, Chang CH, Chong MFF, Chen H, Su KP. Polyunsaturated fatty acids in perinatal depression: a systematic review and meta-analysis. Biol Psychiatry. (2017) 82:560–9. doi: 10.1016/j.biopsych.2017.02.1182
57. Chang JPC, Su KP. Nutritional neuroscience as mainstream of psychiatry: the evidence- based treatment guidelines for using omega-3 fatty acids as a new treatment for psychiatric disorders in children and adolescents. Clin Psychopharmacol Neurosci. (2020) 18:469–83. doi: 10.9758/cpn.2020.18.4.469
58. Jahangard L, Sadeghi A, Ahmadpanah M, Holsboer-Trachsler E, Sadeghi Bahmani D, Haghighi M, et al. Influence of adjuvant omega-3-polyunsaturated fatty acids on depression, sleep, and emotion regulation among outpatients with major depressive disorders - results from a double-blind, randomized and placebo-controlled clinical trial. J Psychiatr Res. (2018) 107:48–56. doi: 10.1016/j.jpsychires.2018.09.016
59. Beier AM, Lauritzen L, Galfalvy HC, Cooper TB, Oquendo MA, Grunebaum MF, et al. Low plasma eicosapentaenoic acid levels are associated with elevated trait aggression and impulsivity in major depressive disorder with a history of comorbid substance use disorder. J Psychiatr Res. (2014) 57:133–40. doi: 10.1016/j.jpsychires.2014.06.012
60. Chiu CC, Frangou S, Chang CJ, Chiu WC, Liu HC, Sun IW, et al. Associations between N-3 Pufa concentrations and cognitive function after recovery from late-life depression. Am J Clin Nutr. (2012) 95:420–7. doi: 10.3945/ajcn.111.015784
61. Emery S, Häberling I, Berger G, Baumgartner N, Strumberger M, Albermann M, et al. Verbal memory performance in depressed children and adolescents: associations with Epa but Not Dha and depression severity. Nutrients. (2020) 12:3630. doi: 10.3390/nu12123630
62. Mazereeuw G, Herrmann N, Oh PI, Ma DWL, Wang CT, Kiss A, et al. Omega-3 fatty acids, depressive symptoms, and cognitive performance in patients with coronary artery disease: analyses from a randomized, double-blind, placebo-controlled trial. J Clin Psychopharmacol. (2016) 36:436–44. doi: 10.1097/JCP.0000000000000565
63. Gu M, Li X, Yan L, Zhang Y, Yang L, Li S, et al. Endogenous Ω-3 fatty acids in fat-1 mice attenuated depression-like behaviors, spatial memory impairment and relevant changes induced by olfactory bulbectomy. Prostaglandins Leukot Essent Fatty Acids. (2021) 171:102313. doi: 10.1016/j.plefa.2021.102313
64. Samieri C, Féart C, Proust-Lima C, Peuchant E, Dartigues JF, Amieva H, et al. Ω-3 Fatty acids and cognitive decline: modulation by Apoeε4 allele and depression. Neurobiol Aging. (2011) 32:2317.e13–e22. doi: 10.1016/j.neurobiolaging.2010.03.020
65. Du J, Zhu M, Bao H, Li B, Dong Y, Xiao C, et al. The role of nutrients in protecting mitochondrial function and neurotransmitter signaling: implications for the treatment of depression, Ptsd, and suicidal behaviors. Crit Rev Food Sci Nutr. (2016) 56:2560–78. doi: 10.1080/10408398.2013.876960
66. Lange KW. Lack of evidence for efficacy of omega-3 fatty acids in depression. Acta Psychiatr Scand. (2021) 144:415–6. doi: 10.1111/acps.13357
67. Reigada L, Buchanan E, Hazeltine D, Shakil H, Polokowski A. A pilot randomized controlled trial testing supplements of omega-3 fatty acids, probiotics, combination or placebo on symptoms of depression, anxiety and stress. J Affect Disord. (2021) 5:100141. doi: 10.1016/j.jadr.2021.100141
68. Tang M, Liu T, Jiang P, Dang R. The interaction between autophagy and neuroinflammation in major depressive disorder: from pathophysiology to therapeutic implications. Pharmacol Res. (2021) 168:105586. doi: 10.1016/j.phrs.2021.105586
69. Xiao K, Luo Y, Liang X, Tang J, Wang J, Xiao Q, et al. Beneficial effects of running exercise on hippocampal microglia and neuroinflammation in chronic unpredictable stress-induced depression model rats. Transl Psychiatry. (2021) 11:1–12. doi: 10.1038/s41398-021-01571-9
70. Guo X, Rao Y, Mao R, Cui L, Fang Y. Common cellular and molecular mechanisms and interactions between microglial activation and aberrant neuroplasticity in depression. Neuropharmacology. (2020) 181:108336. doi: 10.1016/j.neuropharm.2020.108336
71. Ma K, Zhang H, Baloch Z. Pathogenetic and therapeutic applications of tumor necrosis factor-A (Tnf-A) in major depressive disorder: a systematic review. Int J Mol Sci. (2016) 17:733. doi: 10.3390/ijms17050733
72. Enache D, Pariante C, Mondelli V. Markers of central inflammation in major depressive disorder: a systematic review and meta-analysis of studies examining cerebrospinal fluid, positron emission tomography and post-mortem brain tissue. Brain Behav Immun. (2019) 81:24–40. doi: 10.1016/j.bbi.2019.06.015
73. Drevets WC, Wittenberg GM, Bullmore ET, Manji HK. Immune targets for therapeutic development in depression: towards precision medicine. Nat Rev Drug Discov. (2022) 21:224–44. doi: 10.1038/s41573-021-00368-1
74. Li Z, Li Z, Lv X, Li Z, Xiong L, Hu X, et al. Intracerebroventricular administration of interferon-alpha induced depressive-like behaviors and neurotransmitter changes in rhesus monkeys. Front Neurosci. (2020) 14:1171. doi: 10.3389/fnins.2020.585604
75. Zhang J, Lin W, Tang M, Zhao Y, Zhang K, Wang X, et al. Inhibition of Jnk ameliorates depressive-like behaviors and reduces the activation of pro-inflammatory cytokines and the phosphorylation of glucocorticoid receptors at serine 246 induced by neuroinflammation. Psychoneuroendocrinology. (2020) 113:104580. doi: 10.1016/j.psyneuen.2019.104580
76. Silberstein S, Liberman AC, dos Santos Claro PA, Ugo MB, Deussing JM, Arzt E. Stress-related brain neuroinflammation impact in depression: role of the corticotropin-releasing hormone system and P2x7 receptor. Neuroimmunomodulation. (2021) 28:52–60. doi: 10.1159/000515130
77. Ito N, Sasaki K, Takemoto H, Kobayashi Y, Isoda H, Odaguchi H. Emotional impairments and neuroinflammation are induced in male mice invulnerable to repeated social defeat stress. Neuroscience. (2020) 443:148–63. doi: 10.1016/j.neuroscience.2020.07.023
78. Dionisie V, Filip G, Manea M, Movileanu R, Moisa E, Manea M, et al. Neutrophil-to-lymphocyte ratio, a novel inflammatory marker, as a predictor of bipolar type in depressed patients: a quest for biological markers. J Clin Med. (2021) 10:1924. doi: 10.3390/jcm10091924
79. Zainal N, Newman M. Increased inflammation predicts nine-year change in major depressive disorder diagnostic status. J Abnorm Psychol. (2021) 130:829. doi: 10.1037/abn0000716
80. Felger JC, Lotrich FE. Inflammatory cytokines in depression: neurobiological mechanisms and therapeutic implications. Neuroscience. (2013) 246:199–229. doi: 10.1016/j.neuroscience.2013.04.060
81. Peng S, Peng Z, Qin M, Huang L, Zhao B, Wei L, et al. Targeting neuroinflammation: the therapeutic potential of Ω-3 Pufas in substance abuse. Nutrition. (2021) 83:111058. doi: 10.1016/j.nut.2020.111058
82. Joffre C, Dinel AL, Chataigner M, Pallet V, Layé S. N-3 polyunsaturated fatty acids and their derivates reduce neuroinflammation during aging. Nutrients. (2020) 12:647. doi: 10.3390/nu12030647
83. Yan L, Xie Y, Satyanarayanan SK, Zeng H, Liu Q, Huang M, et al. Omega-3 Polyunsaturated fatty acids promote brain-to-blood clearance of B-amyloid in a mouse model with Alzheimer's Disease. Brain Behav Immun. (2020) 85:35–45. doi: 10.1016/j.bbi.2019.05.033
84. Madore C, Leyrolle Q, Morel L, Rossitto M, Greenhalgh AD, Delpech JC, et al. Essential omega-3 fatty acids tune microglial phagocytosis of synaptic elements in the mouse developing brain. Nat Commun. (2020) 11:6133. doi: 10.1038/s41467-020-19861-z
85. Rapaport MH, Nierenberg AA, Schettler PJ, Kinkead B, Cardoos A, Walker R, et al. Inflammation as a predictive biomarker for response to omega-3 fatty acids in major depressive disorder: a proof-of-concept study. Mol Psychiatry. (2016) 21:71–9. doi: 10.1038/mp.2015.22
86. Chang JPC, Lin CY, Lin PY, Shih YH, Chiu TH, Ho M, et al. Polyunsaturated fatty acids and inflammatory markers in major depressive episodes during pregnancy. Prog Neuro-Psychopharmacol Biol Psychiatry. (2018) 80(Pt C):273–8. doi: 10.1016/j.pnpbp.2017.05.008
87. Kiecolt-Glaser JK, Belury MA, Porter K, Beversdorf DQ, Lemeshow S, Glaser R. Depressive symptoms, omega-6:omega-3 fatty acids, and inflammation in older adults. Psychosom Med. (2007) 69:217–24. doi: 10.1097/PSY.0b013e3180313a45
88. Grosso G, Galvano F, Marventano S, Malaguarnera M, Bucolo C, Drago F, et al. Omega-3 fatty acids and depression: scientific evidence and biological mechanisms. Oxid Med Cell Longev. (2014) 2014:313570. doi: 10.1155/2014/313570
89. Levant B. N-3 (Omega-3) polyunsaturated fatty acids in the pathophysiology and treatment of depression: pre-clinical evidence. CNS Neurol Disord Drug Targets. (2013) 12:450–9. doi: 10.2174/1871527311312040003
90. Lu DY, Tsao YY, Leung YM, Su KP. Docosahexaenoic acid suppresses neuroinflammatory responses and induces heme oxygenase-1 expression in Bv-2 microglia: implications of antidepressant effects for Ω-3 fatty acids. Neuropsychopharmacology. (2010) 35:2238–48. doi: 10.1038/npp.2010.98
91. Inoue T, Tanaka M, Masuda S, Ohue-Kitano R, Yamakage H, Muranaka K, et al. Omega-3 polyunsaturated fatty acids suppress the inflammatory responses of lipopolysaccharide-stimulated mouse microglia by activating Sirt1 pathways. Biochim Biophys Acta, Mol Cell Biol Lipids. (2017) 1862:552–60. doi: 10.1016/j.bbalip.2017.02.010
92. Wu Y, Dang R, Tang M, Cai H, Li H, Liao D, et al. Long chain omega-3 polyunsaturated fatty acid supplementation alleviates doxorubicin-induced depressive-like behaviors and neurotoxicity in rats: involvement of oxidative stress and neuroinflammation. Nutrients. (2016) 8:243. doi: 10.3390/nu8040243
93. Herman JP, McKlveen JM, Ghosal S, Kopp B, Wulsin A, Makinson R, et al. Regulation of the hypothalamic-pituitary-adrenocortical stress response. Compr Physiol. (2016) 6:603–21. doi: 10.1002/cphy.c150015
94. Hibbeln JR, Bissette G, Umhau JC, George DT. Omega-3 status and cerebrospinal fluid corticotrophin releasing hormone in perpetrators of domestic violence. Biol Psychiatry. (2004) 56:895–7. doi: 10.1016/j.biopsych.2004.08.021
95. Song C, Zhang XY, Manku M. Increased phospholipase A2 activity and inflammatory response but decreased nerve growth factor expression in the olfactory bulbectomized rat model of depression: effects of chronic ethyl-eicosapentaenoate treatment. J Neurosci. (2009) 29:14–22. doi: 10.1523/JNEUROSCI.3569-08.2009
96. Thesing CS, Bot M, Milaneschi Y, Giltay EJ, Penninx BWJH. Omega-3 polyunsaturated fatty acid levels and dysregulations in biological stress systems. Psychoneuroendocrinology. (2018) 97:206–15. doi: 10.1016/j.psyneuen.2018.07.002
97. Levant B, Ozias MK, Davis PF, Winter M, Russell KL, Carlson SE, et al. Decreased brain docosahexaenoic acid content produces neurobiological effects associated with depression: interactions with reproductive status in female rats. Psychoneuroendocrinology. (2008) 33:1279–92. doi: 10.1016/j.psyneuen.2008.06.012
98. Morgese MG, Tucci P, Mhillaj E, Bove M, Schiavone S, Trabace L, et al. Lifelong nutritional omega-3 deficiency evokes depressive-like state through soluble beta amyloid. Mol Neurobiol. (2017) 54:2079–89. doi: 10.1007/s12035-016-9809-2
99. Mocking RJT, Ruhé HG, Assies J, Lok A, Koeter MWJ, Visser I, et al. Relationship between the Hypothalamic-Pituitary-Adrenal-Axis and fatty acid metabolism in recurrent depression. Psychoneuroendocrinology. (2013) 38:1607–17. doi: 10.1016/j.psyneuen.2013.01.013
100. Delarue J, Matzinger O, Binnert C, Schneiter P, Chioléro R, Tappy L. Fish oil prevents the adrenal activation elicited by mental stress in healthy men. Diabetes Metab. (2003) 29:289–95. doi: 10.1016/S1262-3636(07)70039-3
101. Hellhammer J, Hero T, Franz N, Contreras C, Schubert M. Omega-3 fatty acids administered in phosphatidylserine improved certain aspects of high chronic stress in men. Nutr Res. (2012) 32:241–50. doi: 10.1016/j.nutres.2012.03.003
102. Ferraz AC, Delattre AM, Almendra RG, Sonagli M, Borges C, Araujo P, et al. Chronic Ω-3 fatty acids supplementation promotes beneficial effects on anxiety, cognitive and depressive-like behaviors in rats subjected to a restraint stress protocol. Behav Brain Res. (2011) 219:116–22. doi: 10.1016/j.bbr.2010.12.028
103. Larrieu T, Hilal ML, De Smedt-Peyrusse V, Sans N, Layé S. Nutritional omega-3 deficiency alters glucocorticoid receptor-signaling pathway and neuronal morphology in regionally distinct brain structures associated with emotional deficits. Neural Plast. (2016) 2016:8574830. doi: 10.1155/2016/8574830
104. Tang M, Liu Y, Wang L, Li H, Cai H, Zhang M, et al. An Ω-3 fatty acid-deficient diet during gestation induces depressive-like behavior in rats: the role of the Hypothalamo-Pituitary-Adrenal (Hpa) system. Food Funct. (2018) 9:3481–8. doi: 10.1039/C7FO01714F
105. Pace TWW, Hu F, Miller AH. Cytokine-effects on glucocorticoid receptor function: relevance to glucocorticoid resistance and the pathophysiology and treatment of major depression. Brain Behav Immun. (2007) 21:9–19. doi: 10.1016/j.bbi.2006.08.009
106. Escoll P, Ranz I, Muñoz-Antón N, van-den-Rym A, Alvarez-Mon M, Martínez-Alonso C, et al. Sustained Interleukin-1β exposure modulates multiple steps in glucocorticoid receptor signaling, promoting split-resistance to the transactivation of prominent anti-inflammatory genes by glucocorticoids. Mediators Inflamm. (2015) 2015:347965. doi: 10.1155/2015/347965
107. Choi JE, Kim EY, Park Y. N-3 Pufa improved pup separation-induced postpartum depression via serotonergic pathway regulated by mirna. J Nutr Biochem. (2020) 84:108417. doi: 10.1016/j.jnutbio.2020.108417
108. Madison AA, Belury MA, Andridge R, Renna ME, Shrout MR, Malarkey WB, et al. Omega-3 supplementation and stress reactivity of cellular aging biomarkers: an ancillary substudy of a randomized, controlled trial in midlife adults. Mol Psychiatry. (2021) 26:3034–42. doi: 10.1038/s41380-021-01077-2
109. Pusceddu MM, Nolan YM, Green HF, Robertson RC, Stanton C, Kelly P, et al. The Omega-3 polyunsaturated fatty acid docosahexaenoic acid (Dha) reverses corticosterone-induced changes in cortical neurons. Int J Neuropsychopharmacol. (2016) 19:pyv130. doi: 10.1093/ijnp/pyv130
110. Choi JE, Borkowski K, Newman JW, Park Y. N-3 Pufa improved post-menopausal depression induced by maternal separation and chronic mild stress through serotonergic pathway in rats-effect associated with lipid mediators. J Nutr Biochem. (2021) 91:108599. doi: 10.1016/j.jnutbio.2021.108599
111. Bhatt S, Nagappa AN, Patil CR. Role of oxidative stress in depression. Drug Discov Today. (2020) 25:1270–6. doi: 10.1016/j.drudis.2020.05.001
112. de Queiroz Oliveira T, de Sousa CNS, Vasconcelos GS, de Sousa LC, de Oliveira AA, Patrocínio CFV, et al. Brain antioxidant effect of mirtazapine and reversal of sedation by its combination with alpha-lipoic acid in a model of depression induced by corticosterone. J Affect Disord. (2017) 219:49–57. doi: 10.1016/j.jad.2017.05.022
113. Cobley JN, Fiorello ML, Bailey DM. 13 reasons why the brain is susceptible to oxidative stress. Redox Biol. (2018) 15:490–503. doi: 10.1016/j.redox.2018.01.008
114. Lopresti AL. Mitochondrial dysfunction and oxidative stress: relevance to the pathophysiology and treatment of depression. Neurobiol Depress. (2019) 159−68. doi: 10.1016/B978-0-12-813333-0.00015-9
115. Głombik K, Budziszewska B, Basta-Kaim A. Mitochondrial-targeting therapeutic strategies in the treatment of depression. Mitochondrion. (2021) 58:169–78. doi: 10.1016/j.mito.2021.03.006
116. Liu T, Zhong S, Liao X, Chen J, He T, Lai S, et al. A meta-analysis of oxidative stress markers in depression. PLoS ONE. (2015) 10:e0138904. doi: 10.1371/journal.pone.0138904
117. Duffy SL, Lagopoulos J, Cockayne N, Hermens DF, Hickie IB, Naismith SL. Oxidative stress and depressive symptoms in older adults: a magnetic resonance spectroscopy study. J Affect Disord. (2015) 180:29–35. doi: 10.1016/j.jad.2015.03.007
118. Bouvier E, Brouillard F, Molet J, Claverie D, Cabungcal JH, Cresto N, et al. Nrf2-Dependent persistent oxidative stress results in stress-induced vulnerability to depression. Mol Psychiatry. (2017) 22:1701–13. doi: 10.1038/mp.2016.144
119. Farooqui AA, Farooqui T. Prevention of Oxidative Stress by Omega-3 Fatty Acids in the Brain. Omega-3 fatty acids. Cham: Springer (2016). p. 239–49.
120. Serini S, Calviello G. Reduction of oxidative/nitrosative stress in brain and its involvement in the neuroprotective effect of N-3 Pufa in Alzheimer's Disease. Curr Alzheimer Res. (2016) 13:123–34. doi: 10.2174/1567205012666150921101147
121. Tofighi N, Asle-Rousta M, Rahnema M, Amini R. Protective effect of alpha-linoleic acid on Aβ-induced oxidative stress, neuroinflammation, and memory impairment by alteration of A7 Nachr and Nmdar gene expression in the hippocampus of rats. Neurotoxicology. (2021) 85:245–53. doi: 10.1016/j.neuro.2021.06.002
122. Singh PK, Singh MK, Yadav RS, Nath R, Mehrotra A, Rawat A, et al. Omega-3 fatty acid attenuates oxidative stress in cerebral cortex, cerebellum, and hippocampus tissue and improves neurobehavioral activity in chronic lead-induced neurotoxicity. Nutr Neurosci. (2019) 22:83–97. doi: 10.1080/1028415X.2017.1354542
123. Katrenčíková B, Vaváková M, Paduchová Z, Nagyová Z, Garaiova I, Muchová J, et al. Oxidative stress markers and antioxidant enzymes in children and adolescents with depressive disorder and impact of omega-3 fatty acids in randomised clinical trial. Antioxidants. (2021) 10:1256. doi: 10.3390/antiox10081256
124. Patten AR, Brocardo PS, Christie BR. Omega-3 supplementation can restore glutathione levels and prevent oxidative damage caused by prenatal ethanol exposure. J Nutr Biochem. (2013) 24:760–9. doi: 10.1016/j.jnutbio.2012.04.003
125. Heshmati J, Morvaridzadeh M, Maroufizadeh S, Akbari A, Yavari M, Amirinejad A, et al. Omega-3 fatty acids supplementation and oxidative stress parameters: a systematic review and meta-analysis of clinical trials. Pharmacol Res. (2019) 149:104462. doi: 10.1016/j.phrs.2019.104462
126. Réus GZ, Maciel AL, Abelaira HM, de Moura AB, de Souza TG, Dos Santos TR, et al. Ω-3 and folic acid act against depressive-like behavior and oxidative damage in the brain of rats subjected to early- or late-life stress. Nutrition. (2018) 53:120–33. doi: 10.1016/j.nut.2018.03.006
127. Duffy SL, Lagopoulos J, Cockayne N, Lewis SJG, Hickie IB, Hermens DF, et al. The Effect of 12-Wk Ω-3 fatty acid supplementation on in vivo thalamus glutathione concentration in patients “at Risk” for major depression. Nutrition. (2015) 31:1247–54. doi: 10.1016/j.nut.2015.04.019
128. Golpour P, Nourbakhsh M, Mazaherioun M, Janani L, Nourbakhsh M, Yaghmaei P. Improvement of Nrf2 gene expression and antioxidant status in patients with type 2 diabetes mellitus after supplementation with omega-3 polyunsaturated fatty acids: a double-blind randomised placebo-controlled clinical trial. Diabetes Res Clin Pract. (2020) 162:108120. doi: 10.1016/j.diabres.2020.108120
129. Zgórzyńska E, Dziedzic B, Gorzkiewicz A, Stulczewski D, Bielawska K, Su KP, et al. Omega-3 polyunsaturated fatty acids improve the antioxidative defense in rat astrocytes via an Nrf2-dependent mechanism. Pharmacol Rep. (2017) 69:935–42. doi: 10.1016/j.pharep.2017.04.009
130. Borsini A, Stangl D, Jeffries AR, Pariante CM, Thuret S. The role of omega-3 fatty acids in preventing glucocorticoid-induced reduction in human hippocampal neurogenesis and increase in apoptosis. Transl Psychiatry. (2020) 10:1–12. doi: 10.1038/s41398-020-00908-0
131. Bigornia SJ, Harris WS, Falcon LM, Ordovas JM, Lai CQ, Tucker KL. The omega-3 index is inversely associated with depressive symptoms among individuals with elevated oxidative stress biomarkers. J Nutr. (2016) 146:758–66. doi: 10.3945/jn.115.222562
132. Mazereeuw G, Herrmann N, Andreazza AC, Scola G, Ma DW, Oh PI, et al. Oxidative stress predicts depressive symptom changes with omega-3 fatty acid treatment in coronary artery disease patients. Brain Behav Immun. (2017) 60:136–41. doi: 10.1016/j.bbi.2016.10.005
133. Zhang J, Lin W. The Jnk signaling pathway as a potential new target for depression. Chin Sci Bull. (2018) 63:1998–2009. doi: 10.1360/N972018-00157
134. Chan SW, Harmer CJ, Norbury R, O'Sullivan U, Goodwin GM, Portella MJ. Hippocampal volume in vulnerability and resilience to depression. J Affect Disord. (2016) 189:199–202. doi: 10.1016/j.jad.2015.09.021
135. Han K, Ham B, Kim Y. Development of neuroimaging-based biomarkers in major depression. Adv Exp Med Biol. (2021) 1305:85–99. doi: 10.1007/978-981-33-6044-0_6
136. Sacuiu S, Insel PS, Mueller S, Tosun D, Mattsson N, Jack CR Jr, et al. Chronic depressive symptomatology in mild cognitive impairment is associated with frontal atrophy rate which hastens conversion to Alzheimer Dementia. Am J Geriatr Psychiatry. (2016) 24:126–35. doi: 10.1016/j.jagp.2015.03.006
137. Liu W, Ge T, Leng Y, Pan Z, Fan J, Yang W, et al. The role of neural plasticity in depression: from hippocampus to prefrontal cortex. Neural Plast. (2017) 2017:6871089. doi: 10.1155/2017/6871089
138. Eyre H, Baune BT. Neuroplastic changes in depression: a role for the immune system. Psychoneuroendocrinology. (2012) 37:1397–416. doi: 10.1016/j.psyneuen.2012.03.019
139. Baquero M, Martín N. Depressive symptoms in neurodegenerative diseases. World J Clin Cases. (2015) 3:682–93. doi: 10.12998/wjcc.v3.i8.682
140. Zhao QF, Tan L, Wang HF, Teng J, Yu JT. The prevalence of neuropsychiatric symptoms in Alzheimer's disease: systematic review and meta-analysis. J Affect Disord. (2016) 206:8. doi: 10.1016/j.jad.2016.04.054
141. Khedr EM, Abdelrahman AA, Elserogy Y, Zaki AF, Gamea A. Depression and anxiety among patients with Parkinson's Disease: frequency, risk factors, and impact on quality of life. Egypt J Neurol Psych. (2020) 56:1–9. doi: 10.1186/s41983-020-00253-5
142. Dyall SC. Long-chain omega-3 fatty acids and the brain: a review of the independent and shared effects of Epa, Dpa and Dha. Front Aging Neurosci. (2015) 7:52. doi: 10.3389/fnagi.2015.00052
143. Tomaszewski N, He X, Solomon V, Lee M, Mack WJ, Quinn JF, et al. Effect of Apoe genotype on plasma docosahexaenoic acid (Dha), eicosapentaenoic acid, arachidonic acid, and hippocampal volume in the Alzheimer's Disease cooperative study-sponsored Dha clinical trial. J Alzheimers Dis. (2020) 74:975–90. doi: 10.3233/JAD-191017
144. Cutuli D, Landolfo E, Nobili A, De Bartolo P, Sacchetti S, Chirico D, et al. Behavioral, neuromorphological, and neurobiochemical effects induced by omega-3 fatty acids following basal forebrain cholinergic depletion in aged mice. Alzheimers Res Ther. (2020) 12:1–21. doi: 10.1186/s13195-020-00705-3
145. Thomas A, Baillet M, Proust-Lima C, Féart C, Foubert-Samier A, Helmer C, et al. Blood polyunsaturated omega-3 fatty acids, brain atrophy, cognitive decline, and dementia risk. Alzheimers Dement. (2021) 17:407–16. doi: 10.1002/alz.12195
146. Samieri C, Maillard P, Crivello F, Proust-Lima C, Peuchant E, Helmer C, et al. Plasma long-chain omega-3 fatty acids and atrophy of the medial temporal lobe. Neurology. (2012) 79:642–50. doi: 10.1212/WNL.0b013e318264e394
147. Boneva NB, Kikuchi M, Minabe Y, Yamashima T. Neuroprotective and ameliorative actions of polyunsaturated fatty acids against neuronal diseases: implication of fatty acid-binding proteins (Fabp) and G protein-coupled receptor 40 (Gpr40) in adult neurogenesis. J Pharmacol Sci. (2011) 116:163–72. doi: 10.1254/jphs.10R34FM
148. Shashikumar S, Pradeep H, Chinnu S, Rajini P, Rajanikant G. Alpha-linolenic acid suppresses dopaminergic neurodegeneration induced by 6-Ohda in C. Elegans Physiol Behav. (2015) 151:563–9. doi: 10.1016/j.physbeh.2015.08.025
149. Wu FJ, Xue Y, Liu XF, Xue CH, Wang JF, Du L, et al. The protective effect of eicosapentaenoic acid-enriched phospholipids from sea cucumber cucumaria frondosa on oxidative stress in Pc12 cells and Samp8 mice. Neurochem Int. (2014) 64:9–17. doi: 10.1016/j.neuint.2013.10.015
150. Wang D, Zhang L, Wen M, Du L, Gao X, Xue C, et al. Enhanced neuroprotective effect of Dha and Epa-enriched phospholipids against 1-Methyl-4-Phenyl-1, 2, 3, 6-tetrahydropyridine (Mptp) induced oxidative stress in mice brain. J Funct Foods. (2016) 25:385–96. doi: 10.1016/j.jff.2016.06.014
151. Tatsumi Y, Kato A, Sango K, Himeno T, Kondo M, Kato Y, et al. Omega-3 Polyunsaturated fatty acids exert anti-oxidant effects through the nuclear factor (Erythroid-Derived 2)-related factor 2 pathway in immortalized mouse schwann cells. J Diabetes Investig. (2019) 10:602–12. doi: 10.1111/jdi.12931
152. Chitre NM, Moniri NH, Murnane KS. Omega-3 fatty acids as druggable therapeutics for neurodegenerative disorders. CNS Neurol Disord Drug Targets. (2019) 18:735–49. doi: 10.2174/1871527318666191114093749
153. Abdel-Maksoud SM, Hassanein SI, Gohar NA, Attia SMM, Gad MZ. Investigation of Brain-Derived Neurotrophic Factor (Bdnf) gene expression in hypothalamus of obese rats: modulation by omega-3 fatty acids. Nutr Neurosci. (2017) 20:443–8. doi: 10.1080/1028415X.2016.1180859
154. Sugasini D, Yalagala PCR, Subbaiah PV. Plasma Bdnf is a more reliable biomarker than erythrocyte omega-3 index for the omega-3 fatty acid enrichment of brain. Sci Rep. (2020) 10:10809. doi: 10.1038/s41598-020-67868-9
155. Yu JZ, Wang J, Sheridan SD, Perlis RH, Rasenick MM. N-3 Polyunsaturated fatty acids promote astrocyte differentiation and neurotrophin production independent of camp in patient-derived neural stem cells. Mol Psychiatry. (2020) 26:4605–15. doi: 10.1038/s41380-020-0786-5
156. Colucci-D'Amato L, Speranza L, Volpicelli F. Neurotrophic factor bdnf, physiological functions and therapeutic potential in depression, neurodegeneration and brain cancer. Int J Mol Sci. (2020) 21:7777. doi: 10.3390/ijms21207777
157. Deacon G, Kettle C, Hayes D, Dennis C, Tucci J. Omega 3 polyunsaturated fatty acids and the treatment of depression. Crit Rev Food Sci Nutr. (2017) 57:212–23. doi: 10.1080/10408398.2013.876959
158. Shirooie S, Nabavi SF, Dehpour AR, Belwal T, Habtemariam S, Argüelles S, et al. Targeting Mtors by omega-3 fatty acids: a possible novel therapeutic strategy for neurodegeneration? Pharmacol Res. (2018) 135:37–48. doi: 10.1016/j.phrs.2018.07.004
159. Boccardi V, Tinarelli C, Mecocci P. Effect of Mediterranean Diet on Healthy Brain Aging: Involvement of Telomerase. Role of the Mediterranean Diet in the Brain and Neurodegenerative Diseases. Academic Press (2018). p. 89–101.
160. Freund-Levi Y, Eriksdotter-Jönhagen M, Cederholm T, Basun H, Faxén-Irving G, Garlind A, et al. Omega-3 fatty acid treatment in 174 patients with mild to moderate alzheimer disease: omegad study: a randomized double-blind trial. Arch Neurol. (2006) 63:1402–8. doi: 10.1001/archneur.63.10.1402
161. Yang T, Nie Z, Shu H, Kuang Y, Chen X, Cheng J, et al. The role of Bdnf on neural plasticity in depression. Front Cell Neurosci. (2020) 14:82. doi: 10.3389/fncel.2020.00082
162. Aleksandrova LR, Phillips AG. Neuroplasticity as a convergent mechanism of ketamine and classical psychedelics. Trends Pharmacol Sci. (2021) 42:929–42. doi: 10.1016/j.tips.2021.08.003
163. Aboul-Fotouh S. Behavioral effects of nicotinic antagonist mecamylamine in a rat model of depression: prefrontal cortex level of bdnf protein and monoaminergic neurotransmitters. Psychopharmacology (Berl). (2015) 232:1095–105. doi: 10.1007/s00213-014-3745-5
164. Burgdorf J, Colechio EM, Stanton P, Panksepp J. Positive emotional learning induces resilience to depression: a role for Nmda receptor-mediated synaptic plasticity. Curr Neuropharmacol. (2017) 15:3–10. doi: 10.2174/1570159X14666160422110344
165. Wohleb ES, Terwilliger R, Duman CH, Duman RS. Stress-induced neuronal colony stimulating factor 1 provokes microglia-mediated neuronal remodeling and depressive-like behavior. Biol Psychiatry. (2018) 83:38–49. doi: 10.1016/j.biopsych.2017.05.026
166. Belujon P, Grace AA. Restoring mood balance in depression: ketamine reverses deficit in dopamine-dependent synaptic plasticity. Biol Psychiatry. (2014) 76:927–36. doi: 10.1016/j.biopsych.2014.04.014
167. Micheli L, Ceccarelli M, D'Andrea G, Tirone F. Depression and adult neurogenesis: positive effects of the antidepressant fluoxetine and of physical exercise. Brain Res Bull. (2018) 143:181–93. doi: 10.1016/j.brainresbull.2018.09.002
168. Gellén B, Völgyi K, Györffy BA, Darula Z, Hunyadi-Gulyás É, Baracskay P, et al. Proteomic investigation of the prefrontal cortex in the rat clomipramine model of depression. J Proteomics. (2017) 153:53–64. doi: 10.1016/j.jprot.2016.06.027
169. Guo F, Zhang Q, Zhang B, Fu Z, Wu B, Huang C, et al. Burst-firing patterns in the prefrontal cortex underlying the neuronal mechanisms of depression probed by antidepressants. Eur J Neurosci. (2014) 40:3538–47. doi: 10.1111/ejn.12725
170. Li Z, Ruan M, Chen J, Fang Y. Major depressive disorder: advances in neuroscience research and translational applications. Neurosci Bull. (2021) 37:863–80. doi: 10.1007/s12264-021-00638-3
171. Wurtman RJ. Synapse formation in the brain can be enhanced by co-administering three specific nutrients. Eur J Pharmacol. (2017) 817:20–1. doi: 10.1016/j.ejphar.2017.09.038
172. Yamashima T. ‘Pufa–Gpr40–Creb Signaling'hypothesis for the adult primate neurogenesis. Prog Lipid Res. (2012) 51:221–31. doi: 10.1016/j.plipres.2012.02.001
173. Akerele OA, Cheema SK. Maternal diet high in omega-3 fatty acids upregulate genes involved in neurotrophin signalling in fetal brain during pregnancy in C57bl/6 mice. Neurochem Int. (2020) 138:104778. doi: 10.1016/j.neuint.2020.104778
174. Zhao WN, Hylton NK, Wang J, Chindavong PS, Alural B, Kurtser I, et al. Activation of Wnt and Creb signaling pathways in human neuronal cells in response to the omega-3 fatty acid docosahexaenoic acid (Dha). Mol Cell Neurosci. (2019) 99:103386. doi: 10.1016/j.mcn.2019.06.006
175. Sidhu VK, Huang BX, Desai A, Kevala K, Kim HY. Role of Dha in aging-related changes in mouse brain synaptic plasma membrane proteome. Neurobiol Aging. (2016) 41:73–85. doi: 10.1016/j.neurobiolaging.2016.02.007
176. Crupi R, Marino A, Cuzzocrea S. N-3 fatty acids: role in neurogenesis and neuroplasticity. Curr Med Chem. (2013) 20:2953–63. doi: 10.2174/09298673113209990140
177. Aryal S, Hussain S, Drevon CA, Nagelhus E, Hvalby Ø, Jensen V, et al. Omega-3 fatty acids regulate plasticity in distinct hippocampal glutamatergic synapses. Eur J Neurosci. (2019) 49:40–50. doi: 10.1111/ejn.14224
178. McDougle DR, Watson JE, Abdeen AA, Adili R, Caputo MP, Krapf JE, et al. Anti-inflammatory Ω-3 endocannabinoid epoxides. Proc Natl Acad Sci U S A. (2017) 114:E6034–E43.
179. Winters BL, Vaughan CW. Mechanisms of endocannabinoid control of synaptic plasticity. Neuropharmacology. (2021) 197:108736. doi: 10.1016/j.neuropharm.2021.108736
180. Thomazeau A, Bosch-Bouju C, Manzoni O, Layé S. Nutritional N-3 Pufa deficiency abolishes endocannabinoid gating of hippocampal long-term potentiation. Cerebral Cortex. (2017) 27:2571–9. doi: 10.1093/cercor/bhw052
181. Lafourcade M, Larrieu T, Mato S, Duffaud A, Sepers M, Matias I, et al. Nutritional Omega-3 deficiency abolishes endocannabinoid-mediated neuronal functions. Nat Neurosci. (2011) 14:345–50. doi: 10.1038/nn.2736
182. Chalon S. Omega-3 fatty acids and monoamine neurotransmission. Prostaglandins Leukot Essent Fatty Acids. (2006) 75:259–69. doi: 10.1016/j.plefa.2006.07.005
183. Yang R, Zhang MQ, Xue Y, Yang R, Tang MM. Dietary of N-3 polyunsaturated fatty acids influence neurotransmitter systems of rats exposed to unpredictable chronic mild stress. Behav Brain Res. (2019) 376:112172. doi: 10.1016/j.bbr.2019.112172
184. Hsu MC, Tung CY, Chen HE. Omega-3 polyunsaturated fatty acid supplementation in prevention and treatment of maternal depression: putative mechanism and recommendation. J Affect Disord. (2018) 238:47–61. doi: 10.1016/j.jad.2018.05.018
185. Carabelli B, Delattre AM, Waltrick APF, Araújo G, Suchecki D, Machado RB, et al. Fish-oil supplementation decreases indoleamine-2,3-dioxygenase expression and increases hippocampal serotonin levels in the Lps depression model. Behav Brain Res. (2020) 390:112675. doi: 10.1016/j.bbr.2020.112675
186. Yu Y, Wu Y, Patch C, Wu Z, Szabo A, Li D, et al. Dha prevents altered 5-Ht1a, 5-Ht2a, Cb1 and Gabaa receptor binding densities in the brain of male rats fed a high-saturated-fat diet. J Nutr Biochem. (2013) 24:1349–58. doi: 10.1016/j.jnutbio.2012.11.002
187. Latour A, Grintal B, Champeil-Potokar G, Hennebelle M, Lavialle M, Dutar P, et al. Omega-3 fatty acids deficiency aggravates glutamatergic synapse and astroglial aging in the rat hippocampal Ca1. Aging Cell. (2013) 12:76–84. doi: 10.1111/acel.12026
188. Moreira JD, Knorr L, Ganzella M, Thomazi AP, de Souza CG, de Souza DG, et al. Omega-3 fatty acids deprivation affects ontogeny of glutamatergic synapses in rats: relevance for behavior alterations. Neurochem Int. (2010) 56:753–9. doi: 10.1016/j.neuint.2010.02.010
189. Frajerman A, Scoriels L, Kebir O, Chaumette B. Shared biological pathways between antipsychotics and omega-3 fatty acids: a key feature for schizophrenia preventive treatment? Int J Mol Sci. (2021) 22:6881. doi: 10.3390/ijms22136881
190. Calon F, Lim GP, Morihara T, Yang F, Ubeda O, Salem N, et al. Dietary N-3 polyunsaturated fatty acid depletion activates caspases and decreases Nmda receptors in the brain of a transgenic mouse model of Alzheimer's Disease. Eur J Neurosci. (2005) 22:617–26. doi: 10.1111/j.1460-9568.2005.04253.x
191. Ramsden CE, Zamora D, Makriyannis A, Wood JT, Mann JD, Faurot KR, et al. Diet-induced changes in N-3- and N-6-derived endocannabinoids and reductions in headache pain and psychological distress. J Pain. (2015) 16:707–16. doi: 10.1016/j.jpain.2015.04.007
192. Fitzgerald JM, Chesney SA, Lee TS, Brasel K, Larson CL, Hillard CJ, et al. Circulating endocannabinoids and prospective risk for depression in trauma-injury survivors. Neurobiol Stress. (2021) 14:100304. doi: 10.1016/j.ynstr.2021.100304
193. Balvers MG, Verhoeckx KC, Bijlsma S, Rubingh CM, Meijerink J, Wortelboer HM, et al. Fish oil and inflammatory status alter the N-3 to N-6 balance of the endocannabinoid and oxylipin metabolomes in mouse plasma and tissues. Metabolomics. (2012) 8:1130–47. doi: 10.1007/s11306-012-0421-9
194. Bakunina N, Pariante CM, Zunszain PA. Immune mechanisms linked to depression via oxidative stress and neuroprogression. Immunology. (2015) 144:365–73. doi: 10.1111/imm.12443
195. Kim YK, Na KS, Myint AM, Leonard BE. The role of pro-inflammatory cytokines in neuroinflammation, neurogenesis and the neuroendocrine system in major depression. Prog Neuro-Psychopharmacol Biol Psychiatry. (2016) 64:277–84. doi: 10.1016/j.pnpbp.2015.06.008
196. Ahmad MH, Rizvi MA, Fatima M, Mondal AC. Pathophysiological implications of neuroinflammation mediated Hpa axis dysregulation in the prognosis of cancer and depression. Mol Cell Endocrinol. (2021) 520:111093. doi: 10.1016/j.mce.2020.111093
197. Duman RS, Sanacora G, Krystal JH. Altered connectivity in depression: Gaba and glutamate neurotransmitter deficits and reversal by novel treatments. Neuron. (2019) 102:75–90. doi: 10.1016/j.neuron.2019.03.013
198. Nikkheslat N. Targeting inflammation in depression: ketamine as an anti-inflammatory antidepressant in psychiatric emergency. Brain Behav Immun Health. (2021) 18:100383. doi: 10.1016/j.bbih.2021.100383
199. Hu W, Zhang Y, Wu W, Yin Y, Huang D, Wang Y, et al. Chronic glucocorticoids exposure enhances neurodegeneration in the frontal cortex and hippocampus via Nlrp-1 inflammasome activation in male mice. Brain Behav Immun. (2016) 52:58–70. doi: 10.1016/j.bbi.2015.09.019
200. Golia MT, Poggini S, Alboni S, Garofalo S, Ciano Albanese N, Viglione A, et al. Interplay between inflammation and neural plasticity: both immune activation and suppression impair Ltp and Bdnf expression. Brain Behav Immun. (2019) 81:484–94. doi: 10.1016/j.bbi.2019.07.003
201. Miller AH, Raison CL. The role of inflammation in depression: from evolutionary imperative to modern treatment target. Nat Rev Immunol. (2016) 16:22–34. doi: 10.1038/nri.2015.5
202. Duman RS, Aghajanian GK, Sanacora G, Krystal JH. Synaptic plasticity and depression: new insights from stress and rapid-acting antidepressants. Nat Med. (2016) 22:238–49. doi: 10.1038/nm.4050
203. Vose LR, Stanton PK. Synaptic plasticity, metaplasticity and depression. Curr Neuropharmacol. (2017) 15:71–86. doi: 10.2174/1570159X14666160202121111
204. Labrousse VF, Nadjar A, Joffre C, Costes L, Aubert A, Grégoire S, et al. Short-term long chain omega3 diet protects from neuroinflammatory processes and memory impairment in aged mice. PLoS ONE. (2012) 7:e36861. doi: 10.1371/journal.pone.0036861
205. Larrieu T, Hilal ML, Hilal LM, Fourrier C, De Smedt-Peyrusse V, Sans N, et al. Nutritional omega-3 modulates neuronal morphology in the prefrontal cortex along with depression-related behaviour through corticosterone secretion. Transl Psychiatry. (2014) 4:e437. doi: 10.1038/tp.2014.77
206. Cigliano L, Spagnuolo MS, Boscaino F, Ferrandino I, Monaco A, Capriello T, et al. Dietary supplementation with fish oil or conjugated linoleic acid relieves depression markers in mice by modulation of the Nrf2 pathway. Mol Nutr Food Res. (2019) 63:1900243. doi: 10.1002/mnfr.201900243
207. Peng Z, Zhang C, Yan L, Zhang Y, Yang Z, Wang J, et al. Epa is more effective than Dha to improve depression-like behavior, glia cell dysfunction and hippcampal apoptosis signaling in a chronic stress-induced rat model of depression. Int J Mol Sci. (2020) 21:1769. doi: 10.3390/ijms21051769
208. Song C, Shieh CH, Wu YS, Kalueff A, Gaikwad S, Su KP. The role of omega-3 polyunsaturated fatty acids eicosapentaenoic and docosahexaenoic acids in the treatment of major depression and Alzheimer's Disease: acting separately or synergistically? Prog Lipid Res. (2016) 62:41–54. doi: 10.1016/j.plipres.2015.12.003
209. Morgese MG, Schiavone S, Maffione AB, Tucci P, Trabace L. Depressive-like phenotype evoked by lifelong nutritional omega-3 deficiency in female rats: crosstalk among kynurenine, toll-like receptors and amyloid beta oligomers. Brain Behav Immun. (2020) 87:444–54. doi: 10.1016/j.bbi.2020.01.015
210. Grant R, Guest J. Role of omega-3 pufas in neurobiological health. Adv Neurobiol. (2016) 12:247–74. doi: 10.1007/978-3-319-28383-8_13
211. Schachter HM, Kourad K, Merali Z, Lumb A, Tran K, Miguelez M. Effects of omega-3 fatty acids on mental health. Evid Rep Technol Assess. (2005) 1–11.
212. Borsini A, Nicolaou A, Camacho-Muñoz D, Kendall AC, Di Benedetto MG, Giacobbe J, et al. Omega-3 polyunsaturated fatty acids protect against inflammation through production of Lox and Cyp450 lipid mediators: relevance for major depression and for human hippocampal neurogenesis. Mol Psychiatry. (2021) 26:6773–88. doi: 10.1038/s41380-021-01160-8
Keywords: omega-3 PUFAs, depression, neurotransmitter systems, neuroplasticity, synaptic plasticity, neuroinflammation, neurodegeneration, HPA axis
Citation: Zhou L, Xiong J-Y, Chai Y-Q, Huang L, Tang Z-Y, Zhang X-F, Liu B and Zhang J-T (2022) Possible antidepressant mechanisms of omega-3 polyunsaturated fatty acids acting on the central nervous system. Front. Psychiatry 13:933704. doi: 10.3389/fpsyt.2022.933704
Received: 01 May 2022; Accepted: 15 August 2022;
Published: 31 August 2022.
Edited by:
Dominic D'Agostino, University of South Florida, United StatesReviewed by:
Cristiano Capurso, University of Foggia, ItalyZezhi Li, Guangzhou Medical University, China
Copyright © 2022 Zhou, Xiong, Chai, Huang, Tang, Zhang, Liu and Zhang. This is an open-access article distributed under the terms of the Creative Commons Attribution License (CC BY). The use, distribution or reproduction in other forums is permitted, provided the original author(s) and the copyright owner(s) are credited and that the original publication in this journal is cited, in accordance with accepted academic practice. No use, distribution or reproduction is permitted which does not comply with these terms.
*Correspondence: Jun-Tao Zhang, zhangjuntao@yangzteu.edu.cn; Bo Liu, Drliubo2011@163.com