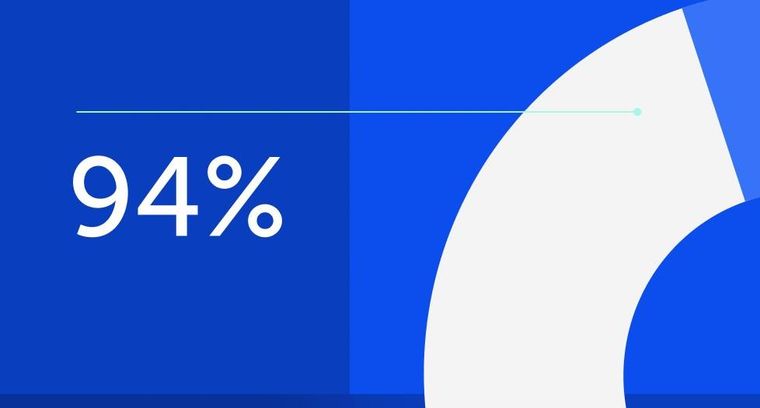
94% of researchers rate our articles as excellent or good
Learn more about the work of our research integrity team to safeguard the quality of each article we publish.
Find out more
MINI REVIEW article
Front. Psychiatry, 26 May 2022
Sec. Child and Adolescent Psychiatry
Volume 13 - 2022 | https://doi.org/10.3389/fpsyt.2022.908895
This article is part of the Research TopicEtiology and Treatment for Children and Adolescents with Autism Spectrum DisorderView all 18 articles
Vitamin D (VD) deficiency during pregnancy and early brain development is an important environmental risk factor for autism spectrum disorder (ASD). Its specific mechanism of action is still unclear. However, one study on the correlation between metabolomics and VD levels in children with ASD has found that the whole-blood arginine (Arg) levels of children with ASD are significantly negatively correlated with serum VD levels, suggesting that the effect of VD on ASD may be related to the signaling pathway involving Arg. Arg is a precursor of nitric oxide (NO), and changes in its levels most directly affect NO levels and signal transduction pathways. NO, a biologically active free radical, is both a neurotransmitter and a neuromodulator in the central nervous system and is related to the pathogeneses of various neurological diseases. The NO signaling pathway is not only affected by VD levels but also closely related to ASD through a series of mechanisms, such as neurotransmitter imbalance, immune disorders, and oxidative stress. Therefore, the effect of VD on ASD may be achieved via regulation of the NO signaling pathway. The current review discusses the relationship among VD, NO, and ASD as suggested by a large body of evidence in the literature in an effort to provide clues for researchers on the pathogenesis of ASD and the mechanism of VD's impact on ASD.
Autism spectrum disorder (ASD) refers to a group of neurodevelopmental disorders that start in early childhood. These disorders, whose main symptoms are social communication impairment and repetitive stereotyped behaviors and interests, have a high prevalence and high disability rate. ASD is one of the fastest-growing severe diseases in the world (1). In 2016, the World Health Organization estimated that 1 child among every 160 has ASD worldwide. In 2018, the US Centers for Disease Control and Prevention reported that the prevalence of ASD in 8-year-old children was as high as 1/59 (2); in 2020, the prevalence reached 1/54 and still maintained a large male-to-female ratio (4.3:1) (3). The onset of the disease occurs during childhood, and the disease often persists throughout life. Its etiology and pathogenesis are still unclear, so it remains one of the most challenging global health problems. It is generally believed that ASD is the result of the combined effects of genetic factors and environmental factors (4, 5).
Nutrition is an environmental factor that interacts with genes from the beginning of the embryonic period and has major impacts on organisms. Vitamin D (VD) is an important nutrient associated with ASD. In 2008, Cannell's epidemiological survey found that the levels of VD in the American population decreased year over year, while the prevalence of ASD increased year over year during the same period; in addition, the prevalence was high in areas with heavy air pollution, cities, and high-altitude areas where VD absorption is reduced. This evidence, combined with evidence of the effects of VD on brain development, led to the hypothesis that VD deficiency may be the etiological factor leading to ASD (6). Since then, research on the correlation between VD and ASD has received extensive attention in academic circles. Subsequent studies have shown that maternal VD deficiency during pregnancy and early brain development is an important environmental risk factor for the development of ASD. However, its specific mechanism of action remains unclear. In a study on the correlation between metabolomics and VD levels in children with ASD, our research team found that the levels of whole-blood arginine (Arg) in children with ASD are significantly negatively correlated with the levels of serum VD, suggesting that the effect of VD on ASD may be related to signaling pathways involving Arg (7). Arg is the precursor of nitric oxide (NO), which most directly affects the NO signal transduction pathway. NO, a biologically active free radical, is both a neurotransmitter and a neuromodulator in the central nervous system and is related to the pathogeneses of various neurological diseases (8). According to both domestic and international studies, the NO signaling pathway is not only affected by VD levels but also closely related to ASD through a series of mechanisms, such as neurotransmitter imbalance, immune disorders, and oxidative stress. Therefore, the effect of VD on ASD may be achieved via regulation of the NO signaling pathway. The current review discusses the relationship among VD, NO and ASD as suggested by a large body of evidence in the literature in an effort to provide clues for researchers on the pathogenesis of ASD and the mechanism of VD's impact on ASD.
VD is a fat-soluble vitamin and is the only chemical substance in our body that is obtained from sunlight. It is also known as the “sunshine vitamin.” VD is obtained in very small amounts from a normal diet, but it is primarily synthesized endogenously in the skin after irradiation by ultraviolet light. After being synthesized, VD binds to plasma VD-binding protein and is transported to the liver, where it is catalyzed by 25-hydroxylase to generate 25(OH)D; then, 25(OH)D is further hydroxylated by 1α-hydroxylase in the kidneys to generate the biologically active compound 1,25(OH)2D. 1, 25(OH) 2D can also activate a variety of target genes through the VD receptor (VDR) to affect the activities of most organs in the body, including the brain. 25(OH)D is the main form of VD in the blood circulation and is used as an indicator of the nutritional VD status.
It was previously believed that the main role of VD was to regulate calcium and phosphorus metabolism and affect bone growth and development. In recent years, however, studies have shown that changes in VD levels can increase the risks of breast cancer, diabetes, dyslipidemia, cardiovascular disease, etc. (9–11). With the discovery that large amounts of 1α hydroxylase (the key enzyme in 25(OH)D synthesis) and VDR are widely present in the brain, it has been gradually recognized that VD plays important roles in brain activity (12). For example, VD plays a very important role in brain development (13, 14). VD deficiency in early life can lead to abnormal brain development and can impair learning, memory, cognition and other functions (15, 16). VD deficiency is also closely related to depression, seasonal psychosis, schizophrenia, Alzheimer's disease and other diseases (17–21). These findings suggest that this “sunshine vitamin” is a valuable sun-derived resource for humans and that its deficiency at different stages of human development and under different genetic backgrounds may have significant impacts on human health.
In 2010, Meguid et al. reported that the serum 25(OH)D levels of Egyptian children with ASD were lower than those of normal control children (22). Subsequently, decreased VD levels in children and adolescents with ASD have been reported around the world (23–28) and found to be correlated with the clinical symptoms of ASD (29–34). Maternal vitamin D levels during pregnancy were inversely associated with the risk of autism in children (35–37). Lower first trimester maternal serum levels of 25(OH)D were associated with an increased risk of developing autism in offspring (38). In a study of 4,229 mother-child pairs, Vinkhuyzen et al. found that scores of autism-like behavior (measured by the Social Responsiveness Scale) in 6-year-olds were significantly greater among children whose mothers had VD deficiency in the second and third trimesters (39). Multiple meta-analyses on the association between ASD and VD levels have shown that 25(OH)D levels in children with ASD are significantly lower than those in healthy controls (40), and insufficient VD levels during pregnancy are a risk factor for ASD in offspring (41).
Jia et al. administered VD to children with ASD and found that high-dose supplementation with VD could improve the core symptoms of the disorder (32, 42–44). In an international open-label prospective study, mothers who had a child with ASD were given 5,000 IU of daily VD supplementation when they became pregnant again, and the mothers continued to give the newborns 1,000 IU of daily VD supplementation after delivery until the age of 3 years. The prevalence (5%) was significantly lower than the reported comorbidity rate of ASD siblings (20%) (45). In contrast, Aizza and Kerley et al. found that VD was ineffective in improving the clinical symptoms of ASD (46), but the therapeutic dose of VD used in those two studies (2,000 IU/day) was much lower than that of Jia et al. (150,000 IU, muscle, once/month for 3 consecutive months), and the serum 25(OH)D levels of the children with ASD at the end of the study were <40 ng/ml. Another study showed that under the condition of supplementing the same dose of VD (2,000 IU/day), the level of VD in children with ASD was significantly lower than that in children with asthma (47). These findings have been suggested that children with ASD require higher doses of vitamin D supplementation and that increasing the levels of 25(OH)D in the serum of children with ASD to above 40 ng/ml can exert a therapeutic effect (48). The latest randomized controlled trials and meta-analyses of VD in the treatment of ASD have also shown that VD can improve the core symptoms of ASD (49, 50).
Valproic acid (VPA), a deacetylase inhibitor, is an anticonvulsant and mood-stabilizing drug. Prenatal exposure to VPA can induce ASD behavior in male offspring, which is one of the methods for constructing ASD animal models (51). Studies have shown that the serum 25(OH)D levels of VPA-induced ASD animal models are significantly lower than those of normal controls. Compared with controls, pups of VD-deficient rats (VDD rats) fed a diet deprived of VD exhibit altered ultrasonic vocalizations and stereotyped repetitive behaviors, as well as impaired social interactions during adolescence (52). Early VD supplementation in infant rats with VPA-induced ASD significantly improves related developmental and behavioral issues (53).
In conclusion, VD deficiency is closely related to the pathogenesis of ASD, and supplementation with high-dose VD can improve the core symptoms of ASD. However, the mechanism of interaction between VD and ASD is still unclear.
NO is a highly active intracellular and intercellular messenger molecule that is widely distributed in various tissues, especially nervous tissue. NO has physiological functions such as immune regulation, neurotransmission, blood pressure regulation and platelet aggregation inhibition, and changes in its concentrations are closely related to physiological functions (54). In the central nervous system, NO is both a neurotransmitter and a neuromodulator. At low concentrations, NO plays physiological roles in the functions of nerve cells and vascular cells, while at high concentrations, NO can be toxic and lead to cell death, which is related to the pathogeneses of various neurological diseases, including stroke and neurodegenerative diseases (55).
NO is generated from Arg in a reaction catalyzed by NO synthase (NOS). There are two types of NOS: native and inducible. Native NOS is inherent in cells and is divided into neuronal NOS (nNOS) and endothelial NOS (eNOS). Under normal physiological conditions, NO is produced in appropriate amounts through the action of native NOS, thereby exhibiting important physiological functions. nNOS is the major subtype of NOS in the central nervous system. The N-methyl-D-aspartate receptor (NMDAR) at the postsynaptic membrane is activated by glutamate (Glu), which in turn causes Ca2+ influx, activates nNOS, catalyzes Arg reactions, and generates citrulline and NO. NO acts on adjacent neurons through diffusion, thereby inducing long-term potentiation (LTP) related to learning and memory and exerting its biological activity (56). eNOS enables endothelial cells to continuously release NO so that vascular smooth muscle is in a state of relaxation, which plays an important role in the regulation of cerebral blood flow. iNOS is mainly distributed in glial cells (including microglia and astrocytes) in the brain. Unlike native NOS (nNOS or eNOS), inducible NOS (iNOS) does not exert biological activity under normal physiological conditions; rather, it is generated and activated only under pathological conditions (such as inflammation, cancer, trauma, etc.) to further generate large amounts of NO.
There are three main pathways downstream of NO. First, NO can activate soluble guanylate cyclase to generate cGMP, which leads to the transcriptional activation of different genes through the cGMP-PKG-CREB pathway. Second, NO can directly or indirectly lead to S-nitrosation (SNO) of many proteins and receptors, thereby changing the activity of receptors, the interactions between proteins and the localization of proteins, etc., resulting in changes in related signaling pathways (57–60). Dysregulation of NO and SNO signaling is involved in the progression of many neurodevelopmental, neurobehavioral and neurodegenerative diseases. In addition, elevated NO levels increase nitrosative stress, nitrite formation, and tyrosine nitration of proteins and may ultimately induce cell death. NO reacts with superoxide anion radicals (O2−) to generate peroxynitrite, which ultimately damages DNA, fats and proteins during oxidative stress (61, 62) (Figure 1).
Figure 1. Schematic diagram of NO function in the brain. NO can relax vascular smooth muscle, thereby regulating cerebral blood flow. Arg is catalyzed by different NOS to generate NO under physiological and pathological conditions. NO activates soluble guanylate cyclase to produce cGMP which interacts with many intracellular proteins such as PKG. PKG leads to CREB phosphorylation which leads into transcriptional activation of different genes. NO, directly and indirectly, leads to S-nitrosylation (SNO) of many proteins and receptors. SNO of proteins can alter the receptor activity, protein-protein interaction and protein localization leading to alteration in signaling. Increased level of NO increases proxynitrite formation, tyrosine nitration of proteins, which ultimately may lead into cell death.
Although the pathogenesis of ASD is unclear, neurotransmitters, oxidative stress, and immune and nutritional factors are believed to play important roles in the pathogenesis of ASD. These factors are closely related to the NO signaling pathway. Multiple studies have shown that the levels of NO signaling pathway members are altered in children with ASD. These findings suggest that NO may play an important role in the pathogenesis of ASD.
Both clinical studies and meta-analyses have found that serum and red blood cells (RBC) NO or nitrite a (metabolite of NO) levels in children with ASD are higher than those in normal children (63–68). Abnormal transport of L-Arg, the raw material of NO, is also related to ASD. Metabolomics studies have found that children with ASD have increased plasma Arg levels and decreased renal Arg clearance (69). Arg is transferred into neurons via cationic amino acid transporter 3 (CAT-3), and genetic testing of children with ASD has identified missense mutations in the CAT-3 gene (70).
ASD patients have abnormal levels of neurotransmitters, and abnormal Glu metabolism is considered to be one of the causes of ASD (71). Glu can activate NMDARs, thereby activating nNOS and resulting in NO production. Changes in Glu levels can lead to changes in NO levels in the nervous system. Immune disturbance mechanisms are also recognized to be involved in the pathogenesis of ASD. Lipopolysaccharide (LPS), a complex of lipids and polysaccharides, is the main component of the cell walls of gram-negative bacteria. LPS injection in pregnant rats can cause ASD symptoms in offspring and is thus currently a commonly used method to induce an immune ASD model in rats (72). However, LPS can induce the expression of iNOS in glial cells (73), thereby causing the production of large amounts of NO. In addition, there is an imbalance between oxidation and antioxidant capacity in children with ASD, and oxidative stress can increase the production of NO by neurons, which has a synergistic effect with the neuronal damage caused by oxygen free radicals (74). Furthermore, the ratio of males to females in ASD is ~4–5:1; this sex difference in the incidence of ASD has long been a popular research topic. Notably, NO is closely related to the hypothalamic-gonadal axis, and the activity of nNOS in neurons is also strictly regulated by estrogen (75). Therefore, NO is closely related not only to ASD but also to the intersection of the abovementioned pathogeneses of ASD and is very likely to be a direct target for the treatment of ASD induced by various causes.
1,25(OH)2D is closely related to NO production, and 1, 25(OH)2D and NO can modulate each other's concentrations. VD is a steroid hormone that is mainly derived from the conversion of 7-dehydrocholesterol in the skin under the action of ultraviolet rays. After two-step hydroxylation, the active product 1,25(OH)2D is formed, which binds to VDR. VDR forms a heterodimer with retinoic acid receptor (RXR), which specifically recognizes the VD response element (VDRE) and regulates the transcription of target genes. The VD signaling pathway can regulate NO and NOS levels. In a study on VDR-knockout mice, the loss of VDR resulted in decreased levels of native NOS and increased expression of arginase 2, which catalyzes the hydrolysis of Arg. Arginase 2 further competed with NOS for Arg to hydrolyze it into ornithine (Orn) and urea (UR), resulting in reduced Arg bioavailability and reduced NO levels (76). In vitro studies have found that 1,25(OH)2D can inhibit LPS-stimulated changes in iNOS mRNA levels in macrophages and significantly reduce NO production (77). 1,25(OH)2D also regulates NO production and iNOS expression in macrophages, endothelial cells, osteoblasts, microglia, macrophages, and astrocytes (78, 79). Population studies have revealed significant negative correlations between serum NO and VD levels in normal adolescents (80). In addition, NO can inversely regulate 1,25(OH)2D levels. CYP27B1 is a 1α hydroxylase gene whose expression is bidirectionally regulated by NO levels. The transcription of CYP27B1 can be upregulated at low concentrations of NO but inhibited by high concentrations of NO, thereby further affecting the levels of 1,25(OH)2D (81). Therefore, VD levels are closely related to NO levels, and the two influence each other.
VD deficiency during pregnancy and early brain development is an important environmental risk factor for ASD, and high-dose VD can improve the core symptoms of ASD. The serum VD levels in children with ASD are significantly negatively correlated with the levels of the raw material for NO synthesis, Arg. NO, one of the most important signaling molecules in the brain, is involved in the occurrence of various brain-related diseases and is an important pathological molecule in ASD, Its role in ASD is mediated by a series of mechanisms, such as neurotransmitter imbalance, immune disorders, and oxidative stress. VD can regulate the levels of molecules in the NO signaling pathway. This review hypothesizes a potential mechanism in which abnormal levels of NO during the critical period of brain development caused by various factors (including abnormalities in neurotransmitters, immune disorders, etc.) are important factors in the pathogenesis of ASD, and VD affects the pathogenesis and severity of ASD by regulating NO levels. If this hypothesis is verified, monitoring NO levels during pregnancy and early brain development may become an important strategy for ASD risk prediction, and NO levels may guide the timing and dosing of VD supplementation. Researches on this pathway could contribute to detect more objective criteria for the diagnosis of ASD. The combination of VD and NO- related issues might indicate possible subtype of ASD. Eventually this may lead to more effective prevention and treatment of ASD.
BW, LX, and HD contributed to conception of the review and wrote sections of the manuscript. BW, HL, and XY participated in the literature search. BW wrote the first draft of the manuscript. All authors contributed to manuscript revision, read, and approved the submitted version.
The authors declare that the research was conducted in the absence of any commercial or financial relationships that could be construed as a potential conflict of interest.
All claims expressed in this article are solely those of the authors and do not necessarily represent those of their affiliated organizations, or those of the publisher, the editors and the reviewers. Any product that may be evaluated in this article, or claim that may be made by its manufacturer, is not guaranteed or endorsed by the publisher.
1. Lyall K, Croen L, Daniels J, Fallin MD, Ladd-Acosta C, Lee BK, et al. The changing epidemiology of autism spectrum disorders. Annu Rev Public Health. (2017) 38:81–102. doi: 10.1146/annurev-publhealth-031816-044318
2. Baio J, Wiggins L, Christensen DL, Maenner MJ, Daniels J, Warren Z, et al. Prevalence of autism spectrum disorder among children aged 8 years - autism and developmental disabilities monitoring network, 11 sites, United States, 2014. MMWR Surveill Summ. (2018) 67:1–23. doi: 10.15585/mmwr.ss6706a1
3. Maenner MJ, Shaw KA, Baio J, Washington A, Patrick M, DiRienzo M, et al. Prevalence of autism spectrum disorder among children aged 8 years - autism and developmental disabilities monitoring network, 11 sites, United States, 2016. MMWR Surveill Summ. (2020) 69:1–12. doi: 10.15585/mmwr.ss6904a1
4. Shen L, Zhang K, Feng C, Chen Y, Li S, Iqbal J, et al. iTRAQ-based proteomic analysis reveals protein profile in plasma from children with autism. Proteomics Clin Appl. (2018) 12:e1700085. doi: 10.1002/prca.201700085
5. Tordjman S, Somogyi E, Coulon N, Kermarrec S, Cohen D, Bronsard G, et al. Gene × Environment interactions in autism spectrum disorders: role of epigenetic mechanisms. Front Psychiatry. (2014) 5:53. doi: 10.3389/fpsyt.2014.00053
7. Wang B, Dong H, Feng J, Li H, Zhang Y, Jia F, et al. Retrospective analysis of the correlation between serum vitamin D levels and blood amino acids levels in children with autism: exploration of possible mechanisms of the effect of vitamin D on autism. Res Autism Spectr Disord. (2021) 80:101707. doi: 10.1016/j.rasd.2020.101707
8. Förstermann U, Sessa WC. Nitric oxide synthases: regulation and function. Eur Heart J. (2012) 33:829–37:837a−837d. doi: 10.1093/eurheartj/ehr304
9. de la Guía-Galipienso F, Martínez-Ferran M, Vallecillo N, Lavie CJ, Sanchis-Gomar F, Pareja-Galeano H. Vitamin D and cardiovascular health. Clin Nutr. (2021) 40:2946–57. doi: 10.1016/j.clnu.2020.12.025
10. de La Puente-Yagüe M, Cuadrado-Cenzual MA, Ciudad-Cabañas MJ, Hernández-Cabria M, Collado-Yurrita L. Vitamin D: and its role in breast cancer. Kaohsiung J Med Sci. (2018) 34:423–7. doi: 10.1016/j.kjms.2018.03.004
11. Mitri J Pittas AG. Vitamin D and diabetes. Endocrinol Metab Clin North Am. (2014) 43:205–32. doi: 10.1016/j.ecl.2013.09.010
12. Bivona G, Gambino CM, Iacolino G, Ciaccio M. Vitamin D and the nervous system. Neurol Res. (2019) 41:827–35. doi: 10.1080/01616412.2019.1622872
13. Eyles D, Burne T, McGrath J. Vitamin D in fetal brain development. Semin Cell Dev Biol. (2011) 22:629–36. doi: 10.1016/j.semcdb.2011.05.004
14. Harms LR, Burne TH, Eyles DW, McGrath JJ. Vitamin D and the brain. Best Pract Res Clin Endocrinol Metab. (2011) 25:657–9. doi: 10.1016/j.beem.2011.05.009
15. Mokry LE, Ross S, Morris JA, Manousaki D, Forgetta V, Richards JB. Genetically decreased vitamin D and risk of Alzheimer disease. Neurology. (2016) 87:2567–74. doi: 10.1212/WNL.0000000000003430
16. Banerjee A, Khemka VK, Ganguly A, Roy D, Ganguly U, Chakrabarti S. Vitamin D and Alzheimer's disease: neurocognition to therapeutics. Int J Alzheimers Dis. (2015) 2015:192747. doi: 10.1155/2015/192747
17. Parker GB, Brotchie H, Graham RK. Vitamin D and depression. J Affect Disord. (2017) 208:56–61. doi: 10.1016/j.jad.2016.08.082
18. Cui X, McGrath JJ, Burne THJ, Eyles DW. Vitamin D and schizophrenia: 20 years on. Mol Psychiatry. (2021) 26:2708–20. doi: 10.1038/s41380-021-01025-0
19. Stumpf WE, Privette TH. Light, vitamin D and psychiatry. role of 1,25 dihydroxyvitamin D3 (soltriol) in etiology and therapy of seasonal affective disorder and other mental processes. Psychopharmacology. (1989) 97:285–94. doi: 10.1007/BF00439440
20. Jayedi A, Rashidy-Pour A, Shab-Bidar S. Vitamin D status and risk of dementia and Alzheimer's disease: a meta-analysis of dose-response (†). Nutr Neurosci. (2019) 22:750–9. doi: 10.1080/1028415X.2018.1436639
21. Bivona G, Lo Sasso B, Gambino CM, Giglio RV, Scazzone C, Agnello L, et al. The role of Vitamin D as a biomarker in Alzheimer's disease. Brain Sci. (2021) 11:334. doi: 10.3390/brainsci11030334
22. Meguid NA, Hashish AF, Anwar M, Sidhom G. Reduced serum levels of 25-hydroxy and 1,25-dihydroxy vitamin D in Egyptian children with autism. J Altern Complement Med. (2010) 16:641–5. doi: 10.1089/acm.2009.0349
23. Du L, Shan L, Wang B, Feng JY, Xu ZD, Jia FY. Serum levels of 25-hydroxyvitamin D in children with autism spectrum disorders. Zhongguo Dang Dai Er Ke Za Zhi. (2015) 17:68–71. doi: 10.7499/j.issn.1008-8830.2015.01.015
24. Sengenç E, Kiykim E, Saltik S. Vitamin D levels in children and adolescents with autism. J Int Med Res. (2020) 48:300060520934638. doi: 10.1177/0300060520934638
25. Alzghoul L, Al-Eitan LN, Aladawi M, Odeh M, Abu Hantash O. The association between serum vitamin D3 levels and autism among jordanian boys. J Autism Dev Disord. (2020) 50:3149–54. doi: 10.1007/s10803-019-04017-w
26. Arastoo AA, Khojastehkia H, Rahimi Z, Khafaie MA, Hosseini SA, Mansouri MT, et al. Evaluation of serum 25-Hydroxy vitamin D levels in children with autism spectrum disorder. Ital J Pediatr. (2018) 44:150. doi: 10.1186/s13052-018-0587-5
27. Bener A, Khattab AO, Al-Dabbagh MM. Is high prevalence of vitamin D deficiency evidence for autism disorder?: In a highly endogamous population. J Pediatr Neurosci. (2014) 9:227–33. doi: 10.4103/1817-1745.147574
28. Petruzzelli MG, Marzulli L, Margari F, De Giacomo A, Gabellone A, Giannico OV, et al. Vitamin D deficiency in autism spectrum disorder: a cross-sectional study. Dis Markers. (2020) 2020:9292560. doi: 10.1155/2020/9292560
29. Dong HY, Wang B, Li HH, Shan L, Jia FY. Correlation between serum 25-hydroxyvitamin D level and core symptoms of autism spectrum disorder in children. Zhonghua Er Ke Za Zhi. (2017) 55:916–19. doi: 10.3760/cma.j.issn.0578-1310.2017.12.010
30. Desoky T, Hassan MH, Fayed HM, Sakhr HM. Biochemical assessments of thyroid profile, serum 25-hydroxycholecalciferol and cluster of differentiation 5 expression levels among children with autism. Neuropsychiatr Dis Treat. (2017) 13:2397–403. doi: 10.2147/NDT.S146152
31. El-Ansary A, Cannell JJ, Bjørklund G, Bhat RS, Al Dbass AM, Alfawaz HA, et al. In the search for reliable biomarkers for the early diagnosis of autism spectrum disorder: the role of vitamin D. Metab Brain Dis. (2018) 33:917–31. doi: 10.1007/s11011-018-0199-1
32. Saad K, Abdel-Rahman AA, Elserogy YM, Al-Atram AA, Cannell JJ, Bjørklund G, et al. Vitamin D status in autism spectrum disorders and the efficacy of vitamin D supplementation in autistic children. Nutr Neurosci. (2016) 19:346–51. doi: 10.1179/1476830515Y.0000000019
33. Mostafa GA, Al-Ayadhi LY. Reduced serum concentrations of 25-hydroxy vitamin D in children with autism: relation to autoimmunity. J Neuroinflammation. (2012) 9:201. doi: 10.1186/1742-2094-9-201
34. Zhang Y Wu T. Relationship between serum folic acid and 25(OH)D levels and intelligence and core symptoms in children with autism. Wei Sheng Yan Jiu. (2021) 50:957–61. doi: 10.19813/j.cnki.weishengyanjiu.2021.06.014
35. Pérez-López FR, Pilz S, Chedraui P. Vitamin D supplementation during pregnancy: an overview. Curr Opin Obstet Gynecol. (2020) 32:316–21. doi: 10.1097/GCO.0000000000000641
36. Windham GC, Pearl M, Poon V, Berger K, Soriano JW, Eyles D, et al. Maternal vitamin D levels during pregnancy in Association With Autism Spectrum Disorders (ASD) or Intellectual Disability (ID) in offspring; exploring non-linear patterns and demographic sub-groups. Autism Res. (2020) 13:2216–29. doi: 10.1002/aur.2424
37. Sourander A, Upadhyaya S, Surcel HM, Hinkka-Yli-Salomäki S, Cheslack-Postava K, Silwal S, et al. Maternal vitamin D levels during pregnancy and offspring autism spectrum disorder. Biol Psychiatry. (2021) 90:790–7. doi: 10.1016/j.biopsych.2021.07.012
38. Chen J, Xin K, Wei J, Zhang K, Xiao H. Lower maternal serum 25(OH) D in first trimester associated with higher autism risk in Chinese offspring. J Psychosom Res. (2016) 89:98–101. doi: 10.1016/j.jpsychores.2016.08.013
39. García-Serna AM, Morales E. Neurodevelopmental effects of prenatal vitamin D in humans: systematic review and meta-analysis. Mol Psychiatry. (2020) 25:2468–81. doi: 10.1038/s41380-019-0357-9
40. Wang T, Shan L, Du L, Feng J, Xu Z, Staal WG, et al. Serum concentration of 25-hydroxyvitamin D in autism spectrum disorder: a systematic review and meta-analysis. Eur Child Adolesc Psychiatry. (2016) 25:341–50. doi: 10.1007/s00787-015-0786-1
41. Wang Z, Ding R, Wang J. The association between vitamin D status and Autism Spectrum Disorder (ASD): a systematic review and meta-analysis. Nutrients. (2020) 13:86. doi: 10.3390/nu13010086
42. Feng J, Shan L, Du L, Wang B, Li H, Wang W, et al. Clinical improvement following vitamin D3 supplementation in Autism Spectrum Disorder. Nutr Neurosci. (2017) 20:284–90. doi: 10.1080/1028415X.2015.1123847
43. Jia F, Wang B, Shan L, Xu Z, Staal WG, Du L. Core symptoms of autism improved after vitamin D supplementation. Pediatrics. (2015) 135:e196–8. doi: 10.1542/peds.2014-2121
44. Moradi H, Sohrabi M, Taheri H, Khodashenas E, Movahedi A. Comparison of the effects of perceptual-motor exercises, vitamin D supplementation and the combination of these interventions on decreasing stereotypical behavior in children with autism disorder. Int J Dev Disabil. (2018) 66:122–32. doi: 10.1080/20473869.2018.1502068
45. Stubbs G, Henley K, Green J. Autism: Will vitamin D supplementation during pregnancy and early childhood reduce the recurrence rate of autism in newborn siblings? Med Hypotheses. (2016) 88:74–8. doi: 10.1016/j.mehy.2016.01.015
46. Kerley CP, Power C, Gallagher L, Coghlan D. Lack of effect of vitamin D(3) supplementation in autism: a 20-week, placebo-controlled RCT. Arch Dis Child. (2017) 102:1030–6. doi: 10.1136/archdischild-2017-312783
47. Kerley CP, Elnazir B, Greally P, Coghlan D. Blunted serum 25(OH)D response to vitamin D(3) supplementation in children with autism. Nutr Neurosci. (2020) 23:537–42. doi: 10.1080/1028415X.2018.1529342
48. Jia F, Shan L, Wang B, Li H, Feng J, Xu Z, et al. Fluctuations in clinical symptoms with changes in serum 25(OH) vitamin D levels in autistic children: three cases report. Nutr Neurosci. (2019) 22:863–6. doi: 10.1080/1028415X.2018.1458421
49. Javadfar Z, Abdollahzad H, Moludi J, Rezaeian S, Amirian H, Foroughi AA, et al. Effects of vitamin D supplementation on core symptoms, serum serotonin, and interleukin-6 in children with autism spectrum disorders: a randomized clinical trial. Nutrition. (2020) 79–80:110986. doi: 10.1016/j.nut.2020.110986
50. Song L, Luo X, Jiang Q, Chen Z, Zhou L, Wang D, et al. Vitamin D supplementation is beneficial for children with autism spectrum disorder: a meta-analysis. Clin Psychopharmacol Neurosci. (2020) 18:203–13. doi: 10.9758/cpn.2020.18.2.203
51. Schneider T, Przewłocki R. Behavioral alterations in rats prenatally exposed to valproic acid: animal model of autism. Neuropsychopharmacology. (2005) 30:80–9. doi: 10.1038/sj.npp.1300518
52. Ali A, Vasileva S, Langguth M, Alexander S, Cui X, Whitehouse A, et al. Developmental vitamin D deficiency produces behavioral phenotypes of relevance to autism in an animal model. Nutrients. (2019) 11:1187. doi: 10.3390/nu11051187
53. Du L, Zhao G, Duan Z, Li F. Behavioral improvements in a valproic acid rat model of autism following vitamin D supplementation. Psychiatry Res. (2017) 253:28–32. doi: 10.1016/j.psychres.2017.03.003
54. Lowenstein CJ, Dinerman JL, Snyder SH. Nitric oxide: a physiologic messenger. Ann Intern Med. (1994) 120:227–37. doi: 10.7326/0003-4819-120-3-199402010-00009
55. Nakamura T, Prikhodko OA, Pirie E, Nagar S, Akhtar MW, Oh CK, et al. Aberrant protein S-nitrosylation contributes to the pathophysiology of neurodegenerative diseases. Neurobiol Dis. (2015) 84:99–108. doi: 10.1016/j.nbd.2015.03.017
56. Huang EP. Synaptic plasticity: a role for nitric oxide in LTP. Curr Biol. (1997) 7:R141–3. doi: 10.1016/S0960-9822(97)70073-3
57. Jaffrey SR, Erdjument-Bromage H, Ferris CD, Tempst P, Snyder SH. Protein S-nitrosylation: a physiological signal for neuronal nitric oxide. Nat Cell Biol. (2001) 3:193–7. doi: 10.1038/35055104
58. Smith BC, Marletta MA. Mechanisms of S-nitrosothiol formation and selectivity in nitric oxide signaling. Curr Opin Chem Biol. (2012) 16:498–506. doi: 10.1016/j.cbpa.2012.10.016
59. Stamler JS, Lamas S, Fang FC. Nitrosylation. the prototypic redox-based signaling mechanism. Cell. (2001) 106:675–83. doi: 10.1016/S0092-8674(01)00495-0
60. Stamler JS, Simon DI, Osborne JA, Mullins ME, Jaraki O, Michel T, et al. S-nitrosylation of proteins with nitric oxide: synthesis and characterization of biologically active compounds. Proc Natl Acad Sci USA. (1992) 89:444–8. doi: 10.1073/pnas.89.1.444
61. Deckel AW. Nitric oxide and nitric oxide synthase in Huntington's disease. J Neurosci Res. (2001) 64:99–107. doi: 10.1002/jnr.1057
62. Tripathi MK, Kartawy M, Amal H. The role of nitric oxide in brain disorders: autism spectrum disorder and other psychiatric, neurological, and neurodegenerative disorders. Redox Biol. (2020) 34:101567. doi: 10.1016/j.redox.2020.101567
63. Tostes MH, Teixeira HC, Gattaz WF, Brandão MA, Raposo NR. Altered neurotrophin, neuropeptide, cytokines and nitric oxide levels in autism. Pharmacopsychiatry. (2012) 45:241–3. doi: 10.1055/s-0032-1301914
64. Chen L, Shi XJ, Liu H, Mao X, Gui LN, Wang H, et al. Oxidative stress marker aberrations in children with autism spectrum disorder: a systematic review and meta-analysis of 87 studies (N = 9109). Transl Psychiatry. (2021) 11:15. doi: 10.1038/s41398-020-01135-3
65. Sögüt S, Zoroglu SS, Ozyurt H, Yilmaz HR, Ozugurlu F, Sivasli E, et al. Changes in nitric oxide levels and antioxidant enzyme activities may have a role in the pathophysiological mechanisms involved in autism. Clin Chim Acta. (2003) 331:111–7. doi: 10.1016/S0009-8981(03)00119-0
66. Sweeten TL, Posey DJ, Shankar S, McDougle CJ. High nitric oxide production in autistic disorder: a possible role for interferon-gamma. Biol Psychiatry. (2004) 55:434–7. doi: 10.1016/j.biopsych.2003.09.001
67. Cohen BI. Ammonia (NH3), nitric oxide (NO) and nitrous oxide (N2O)–the connection with infantile autism. Autism. (2006) 10:221–3. doi: 10.1177/1362361306062027
68. Zoroglu SS, Yürekli M, Meram I, Sögüt S, Tutkun H, Yetkin O, et al. Pathophysiological role of nitric oxide and adrenomedullin in autism. Cell Biochem Funct. (2003) 21:55–60. doi: 10.1002/cbf.989
69. Anwar A, Abruzzo PM, Pasha S, Rajpoot K, Bolotta A, Ghezzo A, et al. Advanced glycation endproducts, dityrosine and arginine transporter dysfunction in autism - a source of biomarkers for clinical diagnosis. Mol Autism. (2018) 9:3. doi: 10.1186/s13229-017-0183-3
70. Nava C, Rupp J, Boissel JP, Mignot C, Rastetter A, Amiet C, et al. Hypomorphic variants of cationic amino acid transporter 3 in males with autism spectrum disorders. Amino Acids. (2015) 47:2647–58. doi: 10.1007/s00726-015-2057-3
71. Fatemi SH. The hyperglutamatergic hypothesis of autism. Prog Neuropsychopharmacol Biol Psychiatry. (2008) 32:911, author reply 912–3. doi: 10.1016/j.pnpbp.2007.11.005
72. Solmaz V, Erdogan MA, Alnak A, Meral A, Erbaş O. Erythropoietin shows gender dependent positive effects on social deficits, learning/memory impairments, neuronal loss and neuroinflammation in the lipopolysaccharide induced rat model of autism. Neuropeptides. (2020) 83:102073. doi: 10.1016/j.npep.2020.102073
73. Lin L, Zhang Q, Fan H, Zhao H, Yang Y. Myocardin-related transcription factor A mediates LPS-Induced iNOS transactivation. Inflammation. (2020) 43:1351–61. doi: 10.1007/s10753-020-01213-0
74. Guan P, Ai XM, Yu RT, Gao LD. Study of the mechanism of cultured neuron injury mediated by nitric oxide during hypoxia and oxidative stress. Fa Yi Xue Za Zhi. (2001) 17:79–81:85. doi: 10.3969/j.issn.1004-5619.2001.02.005
75. Bellefontaine N, Hanchate NK, Parkash J, Campagne C, de Seranno S, Clasadonte J, et al. Nitric oxide as key mediator of neuron-to-neuron and endothelia-to-glia communication involved in the neuroendocrine control of reproduction. Neuroendocrinology. (2011) 93:74–89. doi: 10.1159/000324147
76. Andrukhova O, Slavic S, Zeitz U, Riesen SC, Heppelmann MS, Ambrisko TD, et al. Vitamin D is a regulator of endothelial nitric oxide synthase and arterial stiffness in mice. Mol Endocrinol. (2014) 28:53–64. doi: 10.1210/me.2013-1252
77. Chang JM, Kuo MC, Kuo HT, Hwang SJ, Tsai JC, Chen HC, et al. 1-alpha,25-Dihydroxyvitamin D3 regulates inducible nitric oxide synthase messenger RNA expression and nitric oxide release in macrophage-like RAW 264.7 cells. J Lab Clin Med. (2004) 143:14–22. doi: 10.1016/j.lab.2003.08.002
78. Willems HM, van den Heuvel EG, Carmeliet G, Schaafsma A, Klein-Nulend J, Bakker AD. VDR dependent and independent effects of 1,25-dihydroxyvitamin D3 on nitric oxide production by osteoblasts. Steroids. (2012) 77:126–31. doi: 10.1016/j.steroids.2011.10.015
79. Molinari C, Uberti F, Grossini E, Vacca G, Carda S, Invernizzi M, et al. 1α,25-dihydroxycholecalciferol induces nitric oxide production in cultured endothelial cells. Cell Physiol Biochem. (2011) 27:661–8. doi: 10.1159/000330075
80. Al-Daghri NM, Bukhari I, Yakout SM, Sabico S, Khattak MNK, Aziz I, et al. Associations of serum nitric oxide with Vitamin D and other metabolic factors in apparently healthy adolescents. Biomed Res Int. (2018) 2018:1489132. doi: 10.1155/2018/1489132
Keywords: autism spectrum disorder, nitric oxide, vitamin D, nitric oxide synthase, arginine
Citation: Wang B, Dong H, Li H, Yue X and Xie L (2022) A Probable Way Vitamin D Affects Autism Spectrum Disorder: The Nitric Oxide Signaling Pathway. Front. Psychiatry 13:908895. doi: 10.3389/fpsyt.2022.908895
Received: 31 March 2022; Accepted: 25 April 2022;
Published: 26 May 2022.
Edited by:
Wouter G. Staal, Radboud University Nijmegen Medical Centre, NetherlandsReviewed by:
Marwa Meguid, Ain Shams University, EgyptCopyright © 2022 Wang, Dong, Li, Yue and Xie. This is an open-access article distributed under the terms of the Creative Commons Attribution License (CC BY). The use, distribution or reproduction in other forums is permitted, provided the original author(s) and the copyright owner(s) are credited and that the original publication in this journal is cited, in accordance with accepted academic practice. No use, distribution or reproduction is permitted which does not comply with these terms.
*Correspondence: Lin Xie, eGllbGluQGpsdS5lZHUuY24=
Disclaimer: All claims expressed in this article are solely those of the authors and do not necessarily represent those of their affiliated organizations, or those of the publisher, the editors and the reviewers. Any product that may be evaluated in this article or claim that may be made by its manufacturer is not guaranteed or endorsed by the publisher.
Research integrity at Frontiers
Learn more about the work of our research integrity team to safeguard the quality of each article we publish.