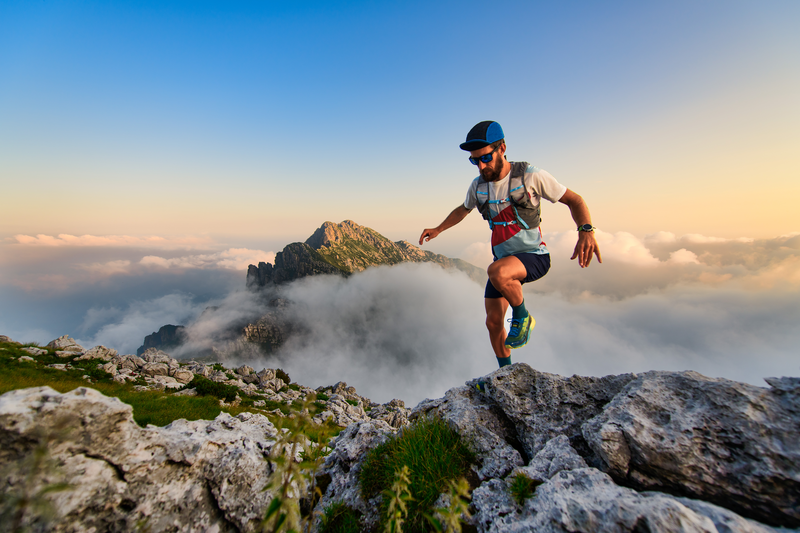
94% of researchers rate our articles as excellent or good
Learn more about the work of our research integrity team to safeguard the quality of each article we publish.
Find out more
CLINICAL TRIAL article
Front. Psychiatry , 24 May 2022
Sec. Neuroimaging
Volume 13 - 2022 | https://doi.org/10.3389/fpsyt.2022.892075
This article is part of the Research Topic Non-invasive Brain Stimulation in Psychiatric Disorders: From Bench to Bedside View all 12 articles
Smoking is currently one of the main public health problems. Smoking cessation is known to be difficult for most smokers because of nicotine dependence. Repetitive transcranial magnetic stimulation (rTMS) over the left dorsolateral prefrontal cortex (DLPFC) has been shown to be effective in the reduction of nicotine craving and cigarette consumption. Here, we evaluated the efficacy of accelerated intermittent theta burst stimulation (aiTBS; four sessions per day for 5 consecutive days) over the left DLPFC in smoking cessation, and we investigated whether the exposure to smoking-related cues compared to neutral cues during transcranial magnetic stimulation (TMS) impacts treatment outcome. A double-blind, randomized, controlled study was conducted in which 89 participants (60 males and 29 females; age 45.62 ± 13.42 years) were randomly divided into three groups: the first group received active aiTBS stimulation while watching neutral videos, the second group received active aiTBS stimulation while watching smoking-related videos and the last group received sham stimulation while watching smoking-related videos. Our results suggest that aiTBS is a tolerable treatment. All treatment groups equally reduced cigarette consumption, nicotine dependence, craving and perceived stress. The effect on nicotine dependence, general craving and perceived stress lasted for at least 1 week after the end of treatment. Active aiTBS over the left DLPFC, combined with smoking related cues, is as effective as active aiTBS combined with neutral cues as well as placebo aiTBS in smoking cessation. These findings extend the results of previous studies indicating that TMS therapy is associated with considerably large placebo effects and that these placebo effects may be further increased when using advanced placebo coil technology.
Clinical Trial Registration: www.clinicaltrials.gov, identifier NCT05271175.
Cigarette smoking is one of the foremost causes of preventable disease and premature death (1–5). According to the World Health Organization (WHO), in 2020, 22.3% of the global population used tobacco (6). Nicotine is a highly addictive chemical compound (7) in tobacco and is released directly in the mesolimbic dopamine pathways where reward processing takes place (8). In 2014, 68% of US adult smokers wanted to quit smoking and in 2017, 55.1% of US adult smokers had made an attempt to quit smoking (9–11). However, only a small percentage of adult smokers (7.4%) actually achieved to quit smoking (11). To support smokers in smoking cessation, behavioral, psychological and pharmacological interventions as well as nicotine replacement therapy are some of the most used interventions (12) with medium to low success rates (12, 13). Recently, there has been growing interest in new, alternative, and effective treatments for smoking cessation.
Transcranial magnetic stimulation (TMS) is a non-invasive brain stimulation therapy (14, 15) that delivers magnetic pulses to a brain region, inducing an electric current that can depolarize neurons and induce action potentials (14). Repetitive (r)TMS protocols have been found to have lasting effects on excitability that can either be (generally) inhibitory (1 Hz) or excitatory (10 Hz) in nature by engaging synaptic plasticity mechanisms, such as long-term potentiation (LTP) and long-term depression (LTD) (16). Theta Burst Stimulation (TBS) is a more recent TMS protocol that delivers a comparable number of pulses in a very short time (17, 18). Two different patterns of TBS were developed: intermittent TBS (iTBS) and continuous TBS (cTBS) which generally increases and decreases cortical excitability, respectively (17).
The dorsolateral prefrontal cortex (DLPFC) is a frontal brain region that plays a crucial role in meso-cortico-limbic and serotonergic systems (19) and is involved in executive functions such as inhibitory control, as well as emotion regulation and decision making; processes modified by substance use and dependence (19–21). Mesolimbic dopamine reward circuits and frontoparietal networks are associated with craving and are activated by addictive drugs (22). Exposure to cigarette-related cues has been associated with activation in the DLPFC (23, 24). Smoking related cues provoke activation of these brain circuits of smokers (24, 25). The combination of rTMS with smoking related cues has been found to be more effective compared to the combination of rTMS alone (26).
Several lines of evidence support the efficacy of high frequency (HF)-rTMS over the left DLPFC in the reduction of nicotine craving and cigarette consumption (21, 24, 27) and cue-induced smoking craving (28). A recently published double blind RCT showed that HF-rTMS (20 Hz) over the left DLPFC for 10 daily sessions is effective in reducing cigarette consumption, craving, dependence as well as in improving anxiety and depressing symptoms (29). According to a recent systematic review, multiple target HF-rTMS may be effective in smoking cessation (21). Accelerated TMS (aTMS), is used increasingly in research and clinical practice and has been shown to be as effective as a standard TMS procedure (30–32). Recently, an accelerated, high-dose, iTBS protocol has shown promising results in patients with treatment resistant depression (33).
A growing body of research highlights the importance of determining the efficacy of TMS in neuropsychiatric disorders using randomized controlled trials (RCT) with placebo-controlled groups. Placebo effects in TMS are a very common phenomenon (34–37) and can have a big influence on the results of a study (38). Several studies indicated that the placebo effect may be a component of the therapeutic response to rTMS in neuropsychiatric disorders like major depressive disorder, and stroke rehabilitation (35, 37).
Considering current knowledge of the efficacy of iTBS in substance use disorders, we investigated in a double-blind randomized control trial efficacy of four iTBS sessions per day during five consecutive days over the left DLPFC in smoking cessation, using the Cool-B65 Active/Placebo (A/P) coil, an advanced coil that is designed to support true “double blinded” clinical trials. Moreover, we wanted to investigate whether the exposure to smoking-related cues during the rTMS treatment, compared to neutral cues impacts cigarette craving. We hypothesized that 20 sessions of accelerated theta burst simulation over the left DLPFC while exposed to smoking-related cues, would reduce cigarette consumption and cigarette cravings, accompanied by reduced stress and motivation to quit smoking to a greater extent than active stimulation combined with neutral cues and sham stimulation with smoking-cues.
One hundred fifty-nine cigarettes smokers, who wanted to quit smoking, were recruited via internet advertisements and printed flyers in the period of April 2019 to December 2020 in Cyprus. Potential participants were screened in a short telephone interview where a total of 104 participants were eligible to participate. Inclusion criteria were the following: (a) aged 18–70, (b) native or fluent Greek speaker. Exclusion criteria were the following: (a) mental objects or implants in the brain, skull or near head (e.g., pacemakers, metal plates), (b) past or current of diagnosis of neurological or psychiatric disorder, (c) use of psychiatric medication, (d) past or current drug or alcohol abuse, other than nicotine, (e) use of IQOS (“I Quit Original Smoking”) or electronic cigarettes (e-cigarettes). A total of 89 participants were included in the final analysis (60 males and 29 females; age 45.62 ± 13.42 years), excluding dropouts (n = 15). The minimum number of participants required was determined by an a priori power analysis where at least a sample size of 100 participants was suggested. [*Measures that suggested this sample size were determined by the mixed model, a small to medium effect size (0.4), at an alpha level of probability of 0.05]. The experiment was carried out in the Cyprus rTMS Center in Larnaca, Cyprus. This study was approved by the Cyprus National Bioethics Committee and written informed consent was obtained from all participants (EEBK/EΠ/2019/08).
A multi-arm parallel group, double-blind, randomized, controlled study was conducted in which participants were randomly divided into three groups: the first group received active iTBS stimulation while watching neutral videos (TMS&N group), the second group received active iTBS stimulation while watching smoking-related videos (TMS&S group) and the last group received sham stimulation while watching smoking-related videos (Sham group). The Latin square design was used for the randomization. Both participants and the investigator who applied the rTMS and administered the self-reported measurements to the participants were blinded to the treatment condition. A second investigator was not blinded to the procedures to be able to set-up the appropriate stimuli. Four iTBS sessions (active or sham) were administrated every day, with 30 min break between them over a 5-day period. Both active iTBS stimulation and sham stimulation were applied over the left DLPFC.
Stimulation was performed using a MagPro X100 (MagVenture, Farum, Denmark) and a figure-of-eight coil (Coil Cool-B65 A/P) for both active and sham stimulation. The Cool-B65 Active/Placebo (A/P) coil is designed to support true “double blinded” clinical trials as it can produce active and placebo stimulation by flipping the coil and can mimic a tapping sensation during placebo condition (39) (see The MagVenture Cool-B65 Active/Placebo (A/P) Coil in Supplementary Material 1 for additional information).
Before the first session, the resting Motor Threshold (rMT) was determined by placing the coil over the left primary motor cortex (40) (see Resting Motor Threshold (rMT) in Supplementary Material 2 for additional information). Stimulation was performed at 100% of rMT. Two experimenters were in the treatment room with the participant. The TMS operator (blinded experimenter) avoided watching the video while it was playing to remain blinded to the procedure and was only looking into the patients' direction. The videos were played by the second researcher.
In both active and sham conditions, an accelerated iTBS (aiTBS) treatment (four sessions with 30 min break between them) was administered daily for a 5-day period over the left DLPFC. Beam_F3 Locator software was used to locate the left DLPFC (41) (see Beam_F3 Locator Software in Supplementary Material 3 for additional information). The stimulation coil was placed at a 45° angle of the midline. iTBS was administrated at 5 Hz and each session included 20 trains with 8 s inter train interval (10 pulses per train at 50 Hz). A total number of 600 pulses was given per session.
Demographic information as well as smoking-habits profile information were collected (Table 1). Participants were asked to report the number of cigarettes usually smoked during a day as well as the type of cigarettes, years of smoking and whether they ever quit smoking and if yes, how many times, to record smoking habits (Table 1).
During the rTMS treatment, participants were instructed to pay attention to videos that were presented on a monitor (Height: 20 cm; Width: 35 cm) placed opposite the treatment chair. Two different forms of videos were used (smoking related videos e.g., a person smoking cigarette in a restaurant and neutral videos e.g., a man cleaning his shoes) in order to elicit craving at the time of stimulation. Each video was presented for approximately 3 min during the stimulation.
Cigarette consumption: (a) Self-reported nicotine consumption: Participants had to daily record the number of cigarettes smoked from the completion of the four sessions until their next treatment visit. Participants were asked not to smoke during the breaks of the four daily rTMS sessions; (b) Carbon monoxide (CO)- evaluated nicotine consumption: CO levels were measured using the piCO Smokerlyzer breath carbon monoxide meter device.
Nicotine dependence: Fagerström test for Nicotine Dependence (FTND) (42) is a short, self-report measure that assesses nicotine dependence. It contains six questions, and the total score is calculated as a sum of these six questions. The total scores of the questionnaire vary from 0 to 10, with lower scores indicating lower dependence on nicotine. This scale has been used previously in Cypriot samples and has been translated into Greek, showing good internal consistency (43, 44).
Craving: (a) Momentary Craving: The Visual Analog Scale (VAS) is a psychometric measurement instrument that measures symptom severity on a continuous scale (45). We used the VAS to assess smoking craving by asking participants to respond to the question “How much do you want to smoke right now?”, on a scale from 0 “no craving” to 100 “most craving ever experienced”; (b) General Craving: Tobacco Craving Questionnaire–Short Form (TCQ-SF) (46) is a self-report measure that assesses tobacco craving in four dimensions: emotionality, craving in anticipation of relief from withdrawal or negative mood; expectancy, craving in anticipation of positive outcomes from smoking; compulsivity, craving in anticipation of an inability to control tobacco use; and purposefulness, craving coupled with intention and planning to smoke. Each factor scale contains three items. TCQ-SF items were rated on a Likert scale of 1 (strongly disagree) to 7 (strongly agree). Total scores vary from 12 to 84, by summing the 12 items and the scores for each factor scale vary from 3 to 21 by summing the three items in each factor scale. A high score indicates high tobacco craving. We translated the TCQ-SF into Greek using the forward and backward-translation procedure (Cronbach's α = 0.90, see Cronbach's alpha in Supplementary Material 4 for additional information).
Perceived Stress: Perceived Stress Scale-4 (PSS-4) (47) is a self-report measure that is used to assess psychological stress. The original PSS comprises 14 items (PSS-14) with two (negative and positive) subscales. We here used the shorter version with four items (PSS-4) that were rated on a Likert scale, ranging from 0 to 4, with those on the positive subscale scored in reverse and the total score was calculated as a sum of these items. The scores vary from 0 to 16, with a higher score indicating higher perceived stress.
Motivation to quit smoking: Participants were asked to estimate how motivated they were to quit smoking from 0 to 100%.
Adverse events: Participants were asked to daily report the adverse events they may have had experienced.
(For the time points of each measurement, see Table 2).
SPSS software version 27.0 was used for the statistical analysis of the data (IBM corporation, Endicott, New York). We calculated the mean score of the 8 VAS scores and 4 CO scores of each day. A one-way ANOVA and Pearson chi-square test were used to test for differences in baseline demographic and smoking-related variables and rMT scores between the three groups. Mixed factorial ANOVAs were conducted to investigate the effect of both the within factor (Time) and the between factor (Group: TMS-N group, TMS-S group, Sham group). The dependent variables used for each model were: cigarette consumption, nicotine dependence, craving and perceived stress. Greenhouse–Geisser and Huynd-Feldt degree of freedom corrections were applied to correct for the non-sphericity the data. Post hoc comparisons using paired-samples t-test were used to evaluate the significance of mean change in cigarette consumption, nicotine dependence, craving and perceived stress at different timepoints. Non-parametric tests were used as the variable Motivation to quit smoking was not normally distributed at all time-point assessments. Non-parametric Wilcoxon signed-rank tests were conducted to evaluate the significance of mean change in Motivation to quit smoking scores at different time points for each Group separately and non-parametric Kruskal–Wallis H tests were conducted to compare the mean scores of motivation to quit of the three Groups at different timepoints. Pearson chi-square test was used to test for differences in adverse events between the active TMS and sham TMS. Finally, a Pearson correlation analysis was applied to correlate a subjective measure (self-reported) with an objective measure (CO) of nicotine consumption. A significance level was set at α = 0.05 for all analyses.
Eight-nine participants completed the entire treatment program (60 males and 29 females; age 45.62 ± 13.42 years; see Enrollment in Supplementary Material for enrollment information and Figure 1 for study recruitment flow diagram). Participant demographics and smoking-related variables are listed in Table 1. Analysis showed that the three groups did not differ significantly in demographic or smoking-related characteristics (all p > 0.05).
A 5 (Time: Baseline, AfterDay1, AfterDay2, After Day3, AfterDay4) × 3 (Group: TMS&N group, TMS&S group, Sham group) mixed factorial ANOVA was conducted for the analysis of the number of cigarettes smoked per day. Mauchly's test indicated that the assumption of sphericity had been violated, χ2(9) = 167.688, p = 0.00, therefore degrees of freedom were corrected using Greenhouse-Geisser of sphericity (ε = 0.470). There was a statistically significant main effect of Time, F(1.879, 142.840) = 166.548, p < 0.0001, ηp2 = 0.687, suggesting a significant decrease in the number of cigarettes smoked per day over time. However, there was no significant effect of Type of Group, F(2, 76) = 0.363, p = 0.697, ηp2 = 0.009 (Figure 2, see Supplementary Table 1 for means and standard deviations). The interaction effect between Time and Group was not statistically significant, F(3.759, 142.840) = 0.414, p = 0.787, ηp2 = 0.011. Post hoc comparisons using paired-samples t-test were used to evaluate the significance of mean change in the number of cigarettes smoked per day at different time points (Table 3). Results indicate that mean scores were statistically significantly lower over time in all the comparisons, except of the pair AfterDay1 vs. AfterDay2, where no statistically significantly changes were found.
Figure 2. Bar graphs showing difference in mean scores of VAS, CO, Cigarettes smoked per day over time. Data are presented as mean ± SEM.
A 6 (Time: Baseline, Day1, Day2, Day3, Day4, Day5) × 3 (Group: TMS&N group, TMS&S group, Sham group) mixed factorial ANOVA was conducted for the analysis of CO scores. Mauchly's test indicated that the assumption of sphericity had been violated, χ2(14) = 340.631, p = 0.00, therefore degrees of freedom were corrected using Greenhouse-Geisser of sphericity (ε = 0.368). The interaction effect between Time and Group was not statistically significant, F(3.678, 154.484) = 1.964, p = 0.109, ηp2 = 0.045. There was a statistically significant main effect of Time, F(1.839, 154.484) = 82.421, p < 0.0001, ηp2 = 0.495, suggesting a significant decrease in CO scores over time. However, there was no significant effect of Group, F(2, 84) = 0.589, p = 0.557, ηp2 = 0.014 (Figure 2, see Supplementary Table 1 for means and standard deviations).
A 3 (Time: Baseline, End of treatment, 1 week follow up) × 3 (Group: TMS&N group, TMS&S group, Sham group) mixed factorial ANOVA was conducted as measured by the FTND. Mauchly's test indicated that the assumption of sphericity had been violated, χ2(2) =11.064, p = 0.004, therefore degrees of freedom were corrected using Huynh-Feldt of sphericity (ε = 0.911). The interaction effect between Time and Group was not statistically significant, F(3.642, 116.549) = 0.095, p = 0.978, ηp2 = 0.003. There was a statistically significant main effect of Time, F(1.821, 116.549) = 119.672, p < 0.0001, ηp2 = 0.652, suggesting a significant decrease in nicotine dependence over time. However, there was no significant effect of Group, F(2, 64) = 1.784, p = 0.176, ηp2 = 0.053 (Figure 3, see Supplementary Table 1 for means and standard deviations). Post-hoc paired sample t-tests were used to evaluate the significance of mean change in FTND scores at different time points (Table 4). Results indicate that mean scores were statistically significantly lower at the End of treatment and at 1 month follow up compared to the baseline, however, no statistically significantly changes were found between the scores at the End of treatment compared to the scores at 1 week follow up.
Figure 3. Bar graphs showing difference in mean scores of FTND, PSS-4, TCQ-SF and Motivation to quit smoking over time. Data are presented as mean ± SEM.
A 6 (Time: Baseline, Day 1, Day 2, Day3, Day 4, Day 5) × 3 (Group: TMS&N group, TMS&S group, Sham group) mixed factorial ANOVA was conducted for the analysis of VAS scores. Mauchly's test indicated that the assumption of sphericity had been violated, χ2(14) = 160.748, p = 0.00, therefore degrees of freedom were corrected using Greenhouse-Geisser of sphericity (ε = 0.539). The interaction effect between Time and Group was not statistically significant, F(5.389, 231.740) = 0.400, p = 0.861, ηp2 = 0.009. There was a statistically significant main effect of Time, F(2.695, 231.740) = 25.667, p < 0.0001, ηp2 = 0.230, suggesting a significant decrease in VAS scores over time. However, there was no significant effect of Group, F(2, 86) = 1.511, p = 0.226, ηp2 = 0.034 (Figure 2, see Supplementary Table 1 for means and standard deviations).
A 3 (Time: Baseline, End of treatment, 1 week follow up) × 3 (Group: TMS&N group, TMS&S group, Sham group) mixed factorial ANOVA was conducted as measured by the TCQ-SF. Mauchly's test indicated that the assumption of sphericity had been violated in both situations, χ2(2) = 11.572, p = 0.003, therefore degrees of freedom were corrected using Huynh-Feldt of sphericity (ε = 0.905). The interaction effect between Time and Group was not statistically significant, F(3.620, 115.845) = 1.320, p = 0.269, ηp2 = 0.040. There was a statistically significant main effect of Time, F(1.810, 115.845) = 32.881, p < 0.0001, ηp2 = 0.339, suggesting a difference in tobacco craving over time. However, there was no significant effect of Group, F(2, 64) = 2.289, p = 0.110, ηp2 = 0.067 (Figure 3, see Supplementary Table 1 for means and standard deviations). Post-hoc paired sample t-tests were used to evaluate the significance of mean change in TCQ-SF scores at different time points (Table 4). Results indicate that mean scores were statistically significantly lower at the End of treatment and at 1 month follow up compared to the baseline, however, no statistically significantly changes were found between the scores at the End of treatment compared to the scores at 1 week follow up.
A 3 (Time: Baseline, End of treatment, 1 week follow up) × 3 (Group: TMS&N group, TMS&S group, Sham group) mixed factorial ANOVA was conducted as measured by PSS-4. The interaction effect between Time and Group was not statistically significant, F(4, 128) = 1.132, p = 0.344, ηp2 = 0.034. There was a statistically significant main effect of Time, F(2, 128) = 9.398, p < 0.0001, ηp2 = 0.128, suggesting a significant decrease in perceived stress over time. However, there was no significant effect of Group, F(2, 64) = 1.415, p = 0.250, ηp2 = 0.042 (Figure 3, see Supplementary Table 1 for means and standard deviations). Post-hoc paired sample t-tests were used to evaluate the significance of mean change in PSS-4 scores at different time points (Table 4). Results indicate that mean scores were statistically significantly lower at the End of treatment and at 1 month follow up compared to the baseline, however, no statistically significantly changes were found between the scores at the End of treatment compared to the scores at 1 week follow up.
Wilcoxon signed-rank tests yielded no statistically significantly changes, expect of the pair End of treatment vs. 1 week follow up of the TMS& N Group (Z = −2.392, p = 0.017) where scores at 1 week follow up (Mean = 72.37, SD = 23.41) were statistically significantly lower compared to the scores at the End of treatment (Mean = 82.41, SD = 20.59). Also, Kruskal–Wallis H tests showed that there were no statistically significant differences in Motivation scores between the different Groups in the baseline, χ2(2) = 0.646, p = 0.724, at the End of treatment, χ2(2) = 0.202, p = 0.904 and at the 1 week follow up, χ2(2) = 0.810, p = 0.667 (Figure 3, see Supplementary Table 1 for means and standard deviations).
Eleven participants (37.93%) of the TMS-N Group, five participants (16.67%) of the TMS&S group and seven participants (23.33%) of the Sham group reported mild adverse events. There were no statistically significant differences between Active and Sham TMS in terms of adverse events as determined by Pearson chi-square test (p = 0.574). The most frequent adverse events were mild headache and sleepiness (Table 5). No severe adverse events such as seizure or mania have been reported in the study.
A Pearson correlation analysis was applied to correlate self-reported and CO-measured nicotine consumption. Results showed a significant positive correlation between the two variables in all timepoints (see Supplementary Table 2).
The current study investigated the efficacy of a rapid accelerated iTBS therapy (four sessions per day for five consecutive days) combined with smoking related cues in smoking cessation. We hypothesized that an active TMS group that is exposed to smoking related videos during stimulation (TMS&S group) shows more improvement with regard to reducing their cigarette consumption and smoking craving compared to the group that receives sham stimulation while watching smoking-related videos (sham group), and to the group receiving active TMS while watching neutral videos (TMS&N group).
In contrast to these expectations, we however found that all conditions, including sham stimulation, were equally effective in reducing cigarette consumption, CO levels, smoking craving and nicotine dependence. Contrary to our expectations and to what is reported in the literature, active TMS combined with smoking related cues was not more effective than active TMS combined with neutral cues, not sham stimulation.
Most interestingly was the fact that our TMS intervention was highly effective in facilitating smoking cessation. Our participants in the active TMS conditions showed 80.7 and 82.59% decrease in cigarette consumption in TMS &N Group and TMS&S group respectively, and 56.38 and 47.59% reduction in nicotine craving in TMS &N Group and TMS&S group respectively. The number of cigarettes smoked per day was statistically significantly lower over time, from the baseline to the End of treatment of the fifth day. These results are consistent with previous TMS trials, which show that rTMS can significantly reduce cigarette consumption and nicotine craving (21, 24, 26). Surprisingly, our advanced placebo coil technology condition specifically designed to support true “double blinded” clinical trials showed to be equally effective in treating smoking cessation. Our participants in Sham group showed 79.1% decrease in cigarette consumption and 59.34% reduction in nicotine craving. A similar reduction in cigarette consumption was found in a recent RCT, where the reduction in the active group was 76.19% (27), although, contrary to our findings, a much smaller reduction in cigarette consumption was found in the sham group (35.29%). Similarly, participants in all conditions showed huge reductions in CO scores (TMS&N group: 62.01%, TMS&S group: 53.42%, Sham group: 61.29%).
We were thus able to show, that, especially when using such an advanced double blind placebo stimulation technology, the placebo effect of TMS in clinical context can be considerably large and even equal to the effect achieved with active TMS stimulation. Placebo effects in TMS are known to be playing a certain role on the clinical results obtained with TMS and have been documented before (35–38). There are several factors that contribute to the enhancement of placebo effect in rTMS studies (38, 48). A systematic review and meta-analysis by Razza et al. (37) evaluated the efficacy of rTMS for depression using data from a sham group of 61 RCTs, concluding that placebo effect sizes in depression trials are rather large (g = 0.8). Previous studies also demonstrated that placebo effects may be a component of the therapeutic response to rTMS (35, 37). The placebo effect was also shown to be larger in more intense TMS protocols [HF rTMS (48)] and especially accelerated protocols (49).
We therefore support that several specific factors not directly associated with rTMS treatment have contributed to the enhanced placebo effect found in the present study. First, our participants were highly motivated to quit smoking. Our data clearly indicate that already at day 1 and 2 during the treatment cycle, a strong effect of both, active and placebo TMS, was revealed. The timeline of these effects indicate that this is likely driven more by the motivation and expectation of our participants rather than by actually induced neuroplastic changes. Second, we used an intensive and state-of-the art TMS design, applying accelerated TMS with multiple sessions per day using theta burst stimulation sequences. It has been shown before that placebo effects scale with the intensity and complexity of the used TMS technology (48, 49). Finally, we used an advanced placebo coil technology capable of creating a true double blind clinical trial and an undistinguishable experience for each participant whether or not to be in a placebo or active stimulation condition. Unlike previous TMS studies, we did not use a simple coil tilting procedure (50), or a standard sham coil (51) to achieve our placebo condition. Instead, we used a novel and advanced placebo coil technology capable of mimicking not only the visual and auditory experience of active TMS, but also the somatosensory skin sensation using a low intensity current stimulator built into the A/P coils and a pair of surface electrodes placed just below the hairline on the scalp of each participant. These factors likely contributed to the fact that we do find our accelerated TMS intervention to be highly effective in reducing cigarette consumption and smoking craving, but not significantly more effective than placebo. The actual effect of our active rTMS had to show statistically to be on top of the highly effective placebo condition, which turned out to be not the case in our trial due to the factors mentioned above.
Additionally, our results demonstrated a statistically significant difference in perceived stress over time. However, due to the absence of a significant effects of the Group and the interaction effect between Time and Group, these results are inconclusive regarding the efficacy of active TMS in reducing perceived stress. Nevertheless, previous findings have shown that left DLPFC is a principal target of noninvasive brain stimulation techniques in regulating stress-related cognitive processes (52). It was reported in the literature that perceived stress may be a barrier to smoking cessation (53), and thus further investigation on the association of perceived stress and smoking cessation during rTMS treatment is required.
The follow up assessment proved that these positive effect in nicotine dependence and perceived stress, as measured by FTND, TCQ-SF and PSS-4, lasts at least 1 week after the End of treatment. The findings of this study have to be seen in light of some limitations. Firstly, we did not measure self-reported cigarette consumption after the fifth day of treatment and during the 1-week follow up. Another potential limitation is the absence of a fourth group receiving sham stimulation while watching neutral videos. Finally, we did not use any formal assessment of blinding efficacy.
Although future RCTs are necessary to validate these conclusions, the present study highlights the importance of placebo effects and the role of specific placebo coil technologies in evaluating the efficacy of TMS in any psychiatric and psychological contexts. This could be used to further improve the administration of TMS based interventions, both for designing better placebo conditions in clinical trials, as well as for utilizing TMS placebo for enhancing coping and other psychological strategies of patients during rTMS treatment (48).
Our findings show that active aiTBS combined with smoking related cues, is as effective as active aiTBS combined with neutral cues as well as placebo aiTBS in smoking cessation. These findings extend the results of previous studies indicating that rTMS therapy is associated with considerably large placebo effects and that these placebo effects may be further increased when using advanced placebo coil technology. These beneficial effects in reducing cigarette consumption and craving for smoking in this and previous studies are likely a combination between the active rTMS effect and the placebo TMS effect. Future RCTs using advanced placebo coil technology are needed to confirm these results. Finally, future studies should emphasize on how to minimize placebo effect on TMS treatment.
The original contributions presented in the study are included in the article/Supplementary Material, further inquiries can be directed to the corresponding author.
The studies involving human participants were reviewed and approved by Cyprus National Bioethics Committee (EEBK/EΠ/2019/08). The patients/participants provided their written informed consent to participate in this study.
GM: conceptualization, methodology, formal analysis, data curation, writing—original draft, and project administration. PM: formal analysis, data curation, and writing—original draft. LP: formal analysis, data curation, and writing—review and editing. AS: conceptualization, methodology, participant recruitment, data collection, formal analysis, data curation, and writing—review and editing. TS: supervision and writing—review and editing. ATS: conceptualization, supervision, and writing—review and editing, and project administration. All authors agree with the contents of the manuscript and were fully involved in the study and preparation of the manuscript and have read the final version of the manuscript and have approved the submission.
The authors declare that the research was conducted in the absence of any commercial or financial relationships that could be construed as a potential conflict of interest.
All claims expressed in this article are solely those of the authors and do not necessarily represent those of their affiliated organizations, or those of the publisher, the editors and the reviewers. Any product that may be evaluated in this article, or claim that may be made by its manufacturer, is not guaranteed or endorsed by the publisher.
We would like to thank all the participants who took part in this study and research assistants Christodoula Gabriel and Elena Papageorgiou for their valuable help with data collection.
The Supplementary Material for this article can be found online at: https://www.frontiersin.org/articles/10.3389/fpsyt.2022.892075/full#supplementary-material
1. World Health Organization. Tobacco (2021). Available online at: https://www.who.int/news-room/fact-sheets/detail/tobacco (accessed January 20, 2022).
2. Teo KK, Ounpuu S, Hawken S, Pandey MR, Valentin V, Hunt D, et al. Tobacco use and risk of myocardial infarction in 52 countries in the INTERHEART study: a case-control study. Lancet. (2006) 368:647–58. doi: 10.1016/S0140-6736(06)69249-0
3. Kondo T, Nakano Y, Adachi S, Murohara T. Effects of tobacco smoking on cardiovascular disease. Circ J. (2019) 83:1980–5. doi: 10.1253/circj.CJ-19-0323
4. Warren GW, Cummings KM. Tobacco and lung cancer: risks, trends, and outcomes in patients with cancer. Am Soc Clin Oncol Educ Book. (2013) 359–64. doi: 10.14694/EdBook_AM.2013.33.359
5. Sasco AJ, Secretan MB, Straif K. Tobacco smoking and cancer: a brief review of recent epidemiological evidence. Lung Cancer. (2004) 45 Suppl 2:S3–9. doi: 10.1016/j.lungcan.2004.07.998
6. World Health Organization. WHO Global Report on Trends in Prevalence of Tobacco Use 2000-2025. 4th ed. Geneva: World Health Organization (2021).
7. Food and Drug Administration. Nicotine Is Why Tobacco Products Are Addictive (2022). Available online at: https://www.fda.gov/tobacco-products/health-effects-tobacco-use/nicotine-why-tobacco-products-are-addictive#2 (accessed January 20, 2022).
8. De Biasi M, Dani JA. Reward, addiction, withdrawal to nicotine. Annu Rev Neurosci. (2011) 34:105–30. doi: 10.1146/annurev-neuro-061010-113734
9. Centers for Disease Control and Prevention. Smoking Cessation: Fast Facts (2021). Available online at: https://www.cdc.gov/tobacco/data_statistics/fact_sheets/cessation/smoking-cessation-fast-facts/index.html (accessed January 20, 2022).
10. Babb S, Malarcher A, Schauer G, Asman K, Jamal A. Quitting smoking among adults - United States, 2000-2015. MMWR Morb Mortal Wkly Rep. (2017) 65:1457–64. doi: 10.15585/mmwr.mm6552a1
11. Creamer MR, Wang TW, Babb S, Cullen KA, Day H, Willis G, et al. Tobacco product use and cessation indicators among adults - United States, 2018. MMWR Morb Mortal Wkly Rep. (2019) 68:1013–9. doi: 10.15585/mmwr.mm6845a2
12. Lancaster T, Stead L, Silagy C, Sowden A. Effectiveness of interventions to help people stop smoking: findings from the Cochrane Library. BMJ. (2000) 321:355–8. doi: 10.1136/bmj.321.7257.355
13. A clinical practice guideline for treating tobacco use and dependence: A US public health service report. The tobacco use and dependence clinical practice guideline panel, staff, and consortium representatives. JAMA. (2000) 283:3244–54. doi: 10.1001/jama.283.24.3244
14. Klomjai W, Katz R, Lackmy-Vallée A. Basic principles of transcranial magnetic stimulation (TMS) and repetitive TMS (rTMS). Ann Phys Rehabil Med. (2015) 58:208–13. doi: 10.1016/j.rehab.2015.05.005
15. Pell GS, Roth Y, Zangen A. Modulation of cortical excitability induced by repetitive transcranial magnetic stimulation: influence of timing and geometrical parameters and underlying mechanisms. Prog Neurobiol. (2011) 93:59–98. doi: 10.1016/j.pneurobio.2010.10.003
16. Gersner R, Kravetz E, Feil J, Pell G, Zangen A. Long-term effects of repetitive transcranial magnetic stimulation on markers for neuroplasticity: differential outcomes in anesthetized and awake animals. J Neurosci. (2011) 31:7521–6. doi: 10.1523/JNEUROSCI.6751-10.2011
17. Chung SW, Hoy KE. Fitzgerald PB. Theta-burst stimulation: a new form of TMS treatment for depression? Depress Anxiety. (2015) 32:182–92. doi: 10.1002/da.22335
18. Blumberger DM, Vila-Rodriguez F, Thorpe KE, Feffer K, Noda Y, Giacobbe P, et al. Effectiveness of theta burst vs. high-frequency repetitive transcranial magnetic stimulation in patients with depression (THREE-D): a randomised non-inferiority trial. Lancet. (2018) 391:1683–92. doi: 10.1016/S0140-6736(18)30295-2
19. Tik M, Hoffmann A, Sladky R, Tomova L, Hummer A, Navarro de Lara L, et al. Towards understanding rTMS mechanism of action: stimulation of the DLPFC causes network-specific increase in functional connectivity. Neuroimage. (2017) 162:289–96. doi: 10.1016/j.neuroimage.2017.09.022
20. Amidfar M, Ko YH, Kim YK. Neuromodulation and cognitive control of emotion. Adv Exp Med Biol. (2019) 1192:545–64. doi: 10.1007/978-981-32-9721-0_27
21. Hauer L, Scarano GI, Brigo F, Golaszewski S, Lochner P, Trinka E, et al. Effects of repetitive transcranial magnetic stimulation on nicotine consumption and craving: a systematic review. Psychiatry Res. (2019) 281:112562. doi: 10.1016/j.psychres.2019.112562
22. Due DL, Huettel SA, Hall WG, Rubin DC. Activation in mesolimbic and visuospatial neural circuits elicited by smoking cues: evidence from functional magnetic resonance imaging. Am J Psychiatry. (2002) 159:954–60. doi: 10.1176/appi.ajp.159.6.954
23. Brody AL, Mandelkern MA, London ED, Childress AR, Lee GS, Bota RG, et al. Brain metabolic changes during cigarette craving. Arch Gen Psychiatry. (2002) 59:1162–72. doi: 10.1001/archpsyc.59.12.1162
24. Amiaz R, Levy D, Vainiger D, Grunhaus L, Zangen A. Repeated high-frequency transcranial magnetic stimulation over the dorsolateral prefrontal cortex reduces cigarette craving and consumption. Addiction. (2009) 104:653–60. doi: 10.1111/j.1360-0443.2008.02448.x
25. Li X, Du L, Sahlem GL, Badran BW, Henderson S, George MS. Repetitive transcranial magnetic stimulation (rTMS) of the dorsolateral prefrontal cortex reduces resting-state insula activity and modulates functional connectivity of the orbitofrontal cortex in cigarette smokers. Drug Alcohol Depend. (2017) 174:98–105. doi: 10.1016/j.drugalcdep.2017.02.002
26. Dinur-Klein L, Dannon P, Hadar A, Rosenberg O, Roth Y, Kotler M, et al. Smoking cessation induced by deep repetitive transcranial magnetic stimulation of the prefrontal and insular cortices: a prospective, randomized controlled trial. Biol Psychiatry. (2014) 76:742–9. doi: 10.1016/j.biopsych.2014.05.020
27. Li X, Hartwell KJ, Henderson S, Badran BW, Brady KT, George MS. Two weeks of image-guided left dorsolateral prefrontal cortex repetitive transcranial magnetic stimulation improves smoking cessation: a double-blind, sham-controlled, randomized clinical trial. Brain Stimul. (2020) 13:1271–9. doi: 10.1016/j.brs.2020.06.007
28. Li X, Hartwell KJ, Owens M, Lematty T, Borckardt JJ, Hanlon CA, et al. Repetitive transcranial magnetic stimulation of the dorsolateral prefrontal cortex reduces nicotine cue craving. Biol Psychiatry. (2013) 73:714–20. doi: 10.1016/j.biopsych.2013.01.003
29. Abdelrahman AA, Noaman M, Fawzy M, Moheb A, Karim AA, Khedr EM, et al. A double-blind randomized clinical trial of high frequency rTMS over the DLPFC on nicotine dependence, anxiety and depression. Sci Rep. (2021) 11:1640. doi: 10.1038/s41598-020-80927-5
30. Baeken C, Vanderhasselt MA, Remue J, Herremans S, Vanderbruggen N, Zeeuws D, et al. Intensive HF-rTMS treatment in refractory medication-resistant unipolar depressed patients. J Affect Disord. (2013) 151:625–31. doi: 10.1016/j.jad.2013.07.008
31. Holtzheimer PE 3rd, McDonald WM, Mufti M, Kelley ME, Quinn S, Corso G, et al. Accelerated repetitive transcranial magnetic stimulation for treatment-resistant depression. Depress Anxiety. (2010) 27:960–3. doi: 10.1002/da.20731
32. Theleritis C, Sakkas P, Paparrigopoulos T, Vitoratou S, Tzavara C, Bonaccorso S, et al. Two versus one high-frequency repetitive transcranial magnetic stimulation session per day for treatment-resistant depression: a randomized sham-controlled trial. J ECT. (2017) 33:190–7. doi: 10.1097/YCT.0000000000000387
33. Cole EJ, Stimpson KH, Bentzley BS, Gulser M, Cherian K, Tischler C, et al. Stanford accelerated intelligent neuromodulation therapy for treatment-resistant depression. Am J Psychiatry. (2020) 177:716–26. doi: 10.1176/appi.ajp.2019.19070720
34. Dollfus S, Lecardeur L, Morello R, Etard O. Placebo response in repetitive transcranial magnetic stimulation trials of treatment of auditory hallucinations in schizophrenia: a meta-analysis. Schizophr Bull. (2016) 42:301–8. doi: 10.1093/schbul/sbv076
35. Jin Y, Pu T, Guo Z, Jiang B, Mu Q. Placebo effect of rTMS on post-stroke motor rehabilitation: a meta-analysis. Acta Neurol Belg. (2021) 121:993–9. doi: 10.1007/s13760-020-01460-w
36. Mansur CG, Myczkowki ML, de Barros Cabral S, Sartorelli Mdo C, Bellini BB, Dias AM, et al. Placebo effect after prefrontal magnetic stimulation in the treatment of resistant obsessive-compulsive disorder: a randomized controlled trial. Int J Neuropsychopharmacol. (2011) 14:1389–97. doi: 10.1017/S1461145711000575
37. Razza LB, Moffa AH, Moreno ML, Carvalho AF, Padberg F, Fregni F, et al. A systematic review and meta-analysis on placebo response to repetitive transcranial magnetic stimulation for depression trials. Prog Neuropsychopharmacol Biol Psychiatry. (2018) 81:105–13. doi: 10.1016/j.pnpbp.2017.10.016
38. Kaptchuk TJ, Goldman P, Stone DA. Stason WB. Do medical devices have enhanced placebo effects? J Clin Epidemiol. (2000) 53:786–92. doi: 10.1016/S0895-4356(00)00206-7
40. Borckardt JJ, Nahas Z, Koola J, George MS. Estimating resting motor thresholds in transcranial magnetic stimulation research and practice: a computer simulation evaluation of best methods. J ECT. (2006) 22:169–75. doi: 10.1097/01.yct.0000235923.52741.72
41. Beam W, Borckardt JJ, Reeves ST, George MS. An efficient and accurate new method for locating the F3 position for prefrontal TMS applications. Brain Stimul. (2009) 2:50–4. doi: 10.1016/j.brs.2008.09.006
42. Heatherton TF, Kozlowski LT, Frecker RC, Fagerström KO. The fagerström test for nicotine dependence: a revision of the Fagerström tolerance questionnaire. Br J Addict. (1991) 86:1119–27. doi: 10.1111/j.1360-0443.1991.tb01879.x
43. Demosthenous S, Zacharia M, Karekla M. Psychometric properties of the Fagerstrom Test for Nicotine Dependence (FTND) in a Greek-Cypriot sample of young adults. In: Poster presented at the ACBS World Conference, Dublin, Ireland. Dublin (2019).
44. Karekla M, Kapsou M, Ioannou VA, Gregoriou I, Christodoulou A, Gkliaou MA. Pilot results from an acceptance and commitment therapy-enhanced smoking cessation intervention for adolescents. Psychol Health. (2010) 25:250.
45. Klimek L, Bergmann KC, Biedermann T, Bousquet J, Hellings P, Jung K, et al. Visual analogue scales (VAS): Measuring instruments for the documentation of symptoms and therapy monitoring in cases of allergic rhinitis in everyday health care: Position Paper of the German Society of Allergology (AeDA) and the German Society of Allergy and Clinical Immunology (DGAKI), ENT Section, in collaboration with the working group on Clinical Immunology, Allergology and Environmental Medicine of the German Society of Otorhinolaryngology, Head and Neck Surgery (DGHNOKHC). Allergo J Int. (2017) 26:16–24. doi: 10.1007/s40629-016-0006-7
46. Heishman SJ, Singleton EG, Pickworth WB. Reliability and validity of a short form of the tobacco craving questionnaire. Nicotine Tob Res. (2008) 10:643–51. doi: 10.1080/14622200801908174
47. Cohen S, Williamson G. Perceived stress in a probability sample of the United States. In: Spacapan S, Oskamp S, editors. The Social Psychology of Health. Newbury Park, CA: Sage (1988), p. 31–67.
48. Granato A, Fantini J, Monti F, Furlanis G, Musho Ilbeh S, Semenic M, et al. Dramatic placebo effect of high frequency repetitive TMS in treatment of chronic migraine and medication overuse headache. J Clin Neurosci. (2019) 60:96–100. doi: 10.1016/j.jocn.2018.09.021
49. Baeken C. Accelerated rTMS: a potential treatment to alleviate refractory depression. Front Psychol. (2018) 9:2017. doi: 10.3389/fpsyg.2018.02017
50. Mennemeier M, Triggs W, Chelette K, Woods A, Kimbrell T, Dornhoffer J. Sham transcranial magnetic stimulation using electrical stimulation of the scalp. Brain Stimul. (2009) 2:168–73. doi: 10.1016/j.brs.2009.02.002
51. Duecker F, Sack AT. Rethinking the role of sham TMS. Front Psychol. (2015) 6:210. doi: 10.3389/fpsyg.2015.00210
52. Era V, Carnevali L, Thayer JF, Candidi M, Ottaviani C. Dissociating cognitive, behavioral and physiological stress-related responses through dorsolateral prefrontal cortex inhibition. Psychoneuroendocrinology. (2021) 124:105070. doi: 10.1016/j.psyneuen.2020.105070
Keywords: smoking cessation, intermittent theta burst stimulation (iTBS), repetitive transcranial magnetic stimulation, provocative smoking cues, placebo effect
Citation: Mikellides G, Michael P, Psalta L, Stefani A, Schuhmann T and Sack AT (2022) Accelerated Intermittent Theta Burst Stimulation in Smoking Cessation: Placebo Effects Equal to Active Stimulation When Using Advanced Placebo Coil Technology. Front. Psychiatry 13:892075. doi: 10.3389/fpsyt.2022.892075
Received: 08 March 2022; Accepted: 04 May 2022;
Published: 24 May 2022.
Edited by:
Alireza Mohammadi, Baqiyatallah University of Medical Sciences, IranReviewed by:
Dominique Januel, EPS Ville Evrard, FranceCopyright © 2022 Mikellides, Michael, Psalta, Stefani, Schuhmann and Sack. This is an open-access article distributed under the terms of the Creative Commons Attribution License (CC BY). The use, distribution or reproduction in other forums is permitted, provided the original author(s) and the copyright owner(s) are credited and that the original publication in this journal is cited, in accordance with accepted academic practice. No use, distribution or reproduction is permitted which does not comply with these terms.
*Correspondence: Georgios Mikellides, Z2VvcmdlLm1pa2VsbGlkZXNAZ21haWwuY29t
Disclaimer: All claims expressed in this article are solely those of the authors and do not necessarily represent those of their affiliated organizations, or those of the publisher, the editors and the reviewers. Any product that may be evaluated in this article or claim that may be made by its manufacturer is not guaranteed or endorsed by the publisher.
Research integrity at Frontiers
Learn more about the work of our research integrity team to safeguard the quality of each article we publish.