- 1Laboratory of Repair Enzymes, Institute of Chemical Biology and Fundamental Medicine, Novosibirsk, Russia
- 2Department of Natural Sciences, Novosibirsk State University, Novosibirsk, Russia
- 3Laboratory of Molecular Genetics and Biochemistry, Mental Health Research Institute, Tomsk National Research Medical Center of the Russian Academy of Sciences, Tomsk, Russia
The immune system is generally known to be the primary defense mechanism against pathogens. Any pathological conditions are reflected in anomalies in the immune system parameters. Increasing evidence suggests the involvement of immune dysregulation and neuroinflammation in the pathogenesis of schizophrenia. In this systematic review, we summarized the available evidence of abnormalities in the immune system in schizophrenia. We analyzed impairments in all immune system components and assessed the level of bias in the available evidence. It has been shown that schizophrenia is associated with abnormalities in all immune system components: from innate to adaptive immunity and from humoral to cellular immunity. Abnormalities in the immune organs have also been observed in schizophrenia. Evidence of increased C-reactive protein, dysregulation of cytokines and chemokines, elevated levels of neutrophils and autoantibodies, and microbiota dysregulation in schizophrenia have the lowest risk of bias. Peripheral immune abnormalities contribute to neuroinflammation, which is associated with cognitive and neuroanatomical alterations and contributes to the pathogenesis of schizophrenia. However, signs of severe inflammation are observed in only about 1/3 of patients with schizophrenia. Immunological parameters may help identify subgroups of individuals with signs of inflammation who well respond to anti-inflammatory therapy. Our integrative approach also identified gaps in knowledge about immune abnormalities in schizophrenia, and new horizons for the research are proposed.
Introduction
All body systems are interconnected in varying degrees in health and pathology. The immune system is no exception. They are activated when interacting with an infectious agent or spontaneously affecting everything, including the central nervous system. In some cases, this can lead to pathological changes in human mental status. Schizophrenia, as an example of mental illness, is no exception. Ideas that inflammation and immunity are involved in the pathogenesis of schizophrenia have been around for decades, but they do not lose their relevance to this day (1). Back in 1930, Dameshek W. found quantitative changes in white blood cells in patients with schizophrenia (2). In 1937, Lehmann-Facius proposed the idea of a humoral autoimmune factor in the pathogenesis of schizophrenia (3). In 1976, E. Fuller Torrey and Michael R. Peterson proposed a viral hypothesis for schizophrenia, describing, among other things, the possible influence of concomitant immune perturbations (4). Over time, hypotheses about a direct immunocompetent culprit for the onset of schizophrenia have multiplied: general inflammation hypothesis (5), macrophage-T-cell hypothesis (6–8), autoantibody hypothesis (9), microglia hypothesis (10). In this systematic review, we summarized and systematized the available data on changes in various parts of the immune system in schizophrenia. The main question of this systematic review was the following: what changes and in what components of the immune system are found in schizophrenia and how are they associated with the disease?
Brief Overview of the Immune System
The immune system is generally known to be the primary defense mechanism against pathogens and has two arms: the innate (nonspecific) immune system and the adaptive (acquired) immune system (11). The mechanisms of innate immunity inactivate a significant part of pathogens. The adaptive immune response is activated when innate mechanisms are ineffective. Besides, innate immunity plays a vital role in response to tissue damage (aseptic inflammation), resulting in removing apoptotic and necrotic cells and tissue repair. The effector functions of these two arms provide molecular and cellular components (Figure 1). The nonspecific innate immune system recognizes pathogens through pattern recognition receptors (PRRs) (12). Extracellular soluble PRRs (mannose-binding lectin (MBL), lipopolysaccharide-binding protein (LBP), C-reactive protein (CRP), etc.) bind microbial products in liquid media and ensure their opsonization and phagocytosis. Membrane-bound and cytoplasmic PRRs recognize the pathogen-associated molecular patterns (PAMPs) or damage-associated molecular patterns (DAMPs) and initiate an immune response. Membrane-bound PRRs are mainly represented by Toll-like receptors (TLRs). Cytoplasmic PRRs include nucleotide oligomerization domain (NOD)-like receptors (NLRs), C-type lectin receptors (CLRs), retinoic acid-inducible gene-I (RIG-I)-like receptors (RLRs), etc. The complement system is involved in the opsonization of microorganisms and initiating inflammation. Natural antibodies bind exogenous and self-components and participate in their clearance. PAMPs or DAMPs sensing by PRRs engage cellular components in an immune response. The cellular components of innate immunity include phagocytes, dendritic cells, NK cells, mast cells, and B-1 cells. T-cells and B-cells are components of the adaptive immune system. B-cells, particularly plasma cells, produce antibodies, while T-cells provide recognition and destruction of cells carrying foreign antigens and regulate the immune response. Cytokines and chemokines orchestrate the effector functions of all components. However, this is a simplified representation. The division into cellular and molecular components is provisional, and many components are involved in both adaptive and innate reactions.
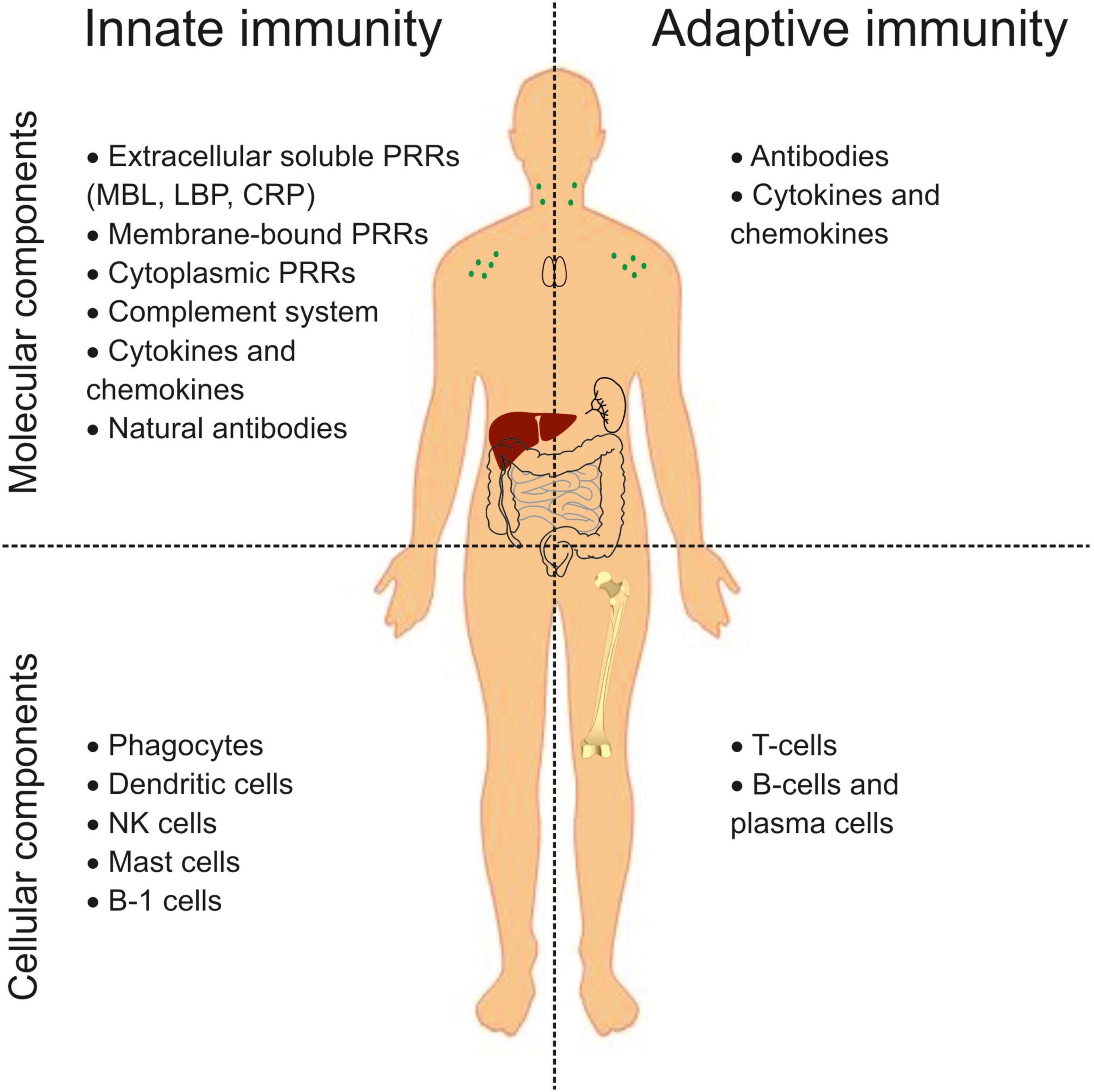
Figure 1. An overview of the innate and adaptive immune system components. PRRs, pattern recognition receptors; MBL, mannose binding lectin; LBP, lipopolysaccharide binding protein; CRP, C-reactive protein, NK, natural killer.
Search Methods and Evidence Quality Assessment
We followed the standards by Preferred Reporting Items for Systematic Reviews and Meta-Analyses (PRISMA 2020) (13, 14). We performed an electronic search using PubMed, Google Scholar, Scopus, and Web of Science to extract the necessary information from database inception to January 2022. The following basic search terms were used (both alone and in combinations): “schizophrenia,” “schizoaffective,” “psychosis,” ‘psychotic,” “meta-analysis,” “systematic,” “review.” The following additional terms were used in combination with the basic search terms: “immune system,” “inflammation,” “PRR,” “CRP,” “sCD14,” “LBP,” “MBL,” “TLR,” “NLR,” “complement,” “cytokine,” “chemokine,” “antibodies,” “antibody,” “IgG,” “IgA,” “IgM,” “natural antibodies,” “autoantibodies,” “Toxoplasma gondii,” “NMDAR,” “GAD65,” “gluten,” “cell,” “natural killer,” “NK,” “dendritic cell,” “neutrophil,” “eosinophil,” “basophil,” “monocyte,” “macrophage,” “microglia,” “lymphocyte,” “B cell,” “CD20,” “T cell,” “CD3,” “CD4,” “bone marrow,” “thymus,” “lymphatic,” “lymphatic nodes,” “spleen,” “liver,” “gut,” “intestine,” “axis,” “microbiota.” PRISMA 2020 flowchart diagram is presented in Figure 2 (15). One researcher reviewed titles and abstracts of the first 50 results of each query and then selected the reports. Automation tools to remove records were not used. Then two independent researchers (EAE and MMM) assessed the relevance of all articles retrieved. The final reference list was compiled based on the relevance of the topics covered in this review after the consensus of the researchers. Meta-analyses and systematic reviews were primarily included in the full text of the review. In their absence, case–control and/or cohort studies and/or case report were included. The main findings of this systematic review summarized in tables are based on 101 studies, including 33 meta-analyses, 5 systematic reviews and 63 other studies. However, the final bibliography contains more studies to broaden the context of the review.
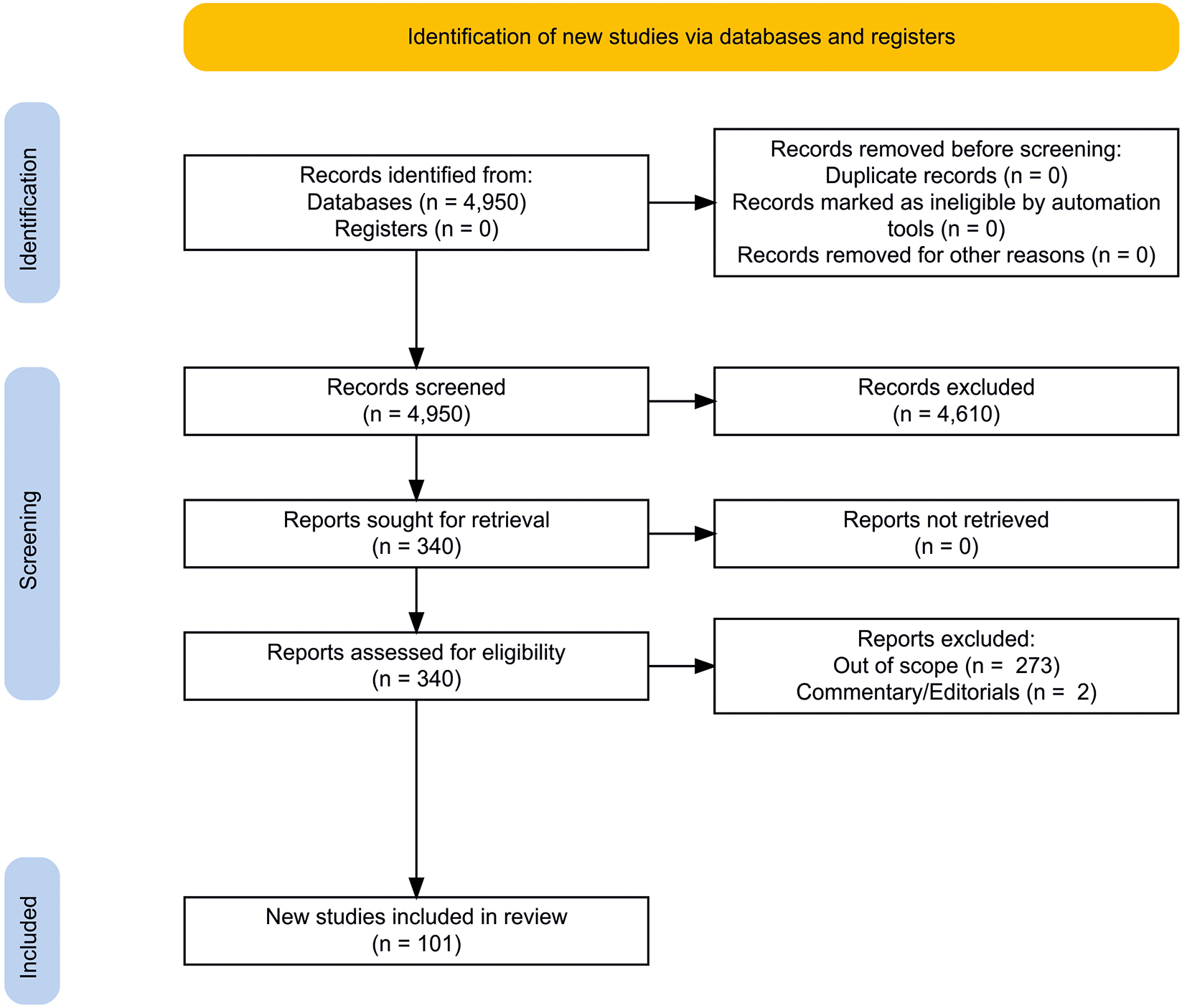
Figure 2. PRISMA 2020 flowchart diagram. Created using an online tool: https://estech.shinyapps.io/prisma_flowdiagram/ (15).
The quality of the evidence found was assessed based on the risk of bias (the higher the risk of bias, the lower evidence quality). The following criteria were used to assess the risk of bias (Table 1), similarly, to Tanaka et al. (16) with some modifications. The risk of bias was assessed by two independent researchers (EAE and MMM). The final assessment of the risk of bias was approved after the consensus of the two researchers.
Alterations in the Innate Immune System in Schizophrenia
Molecular Components of the Innate Immune System
Activation of the innate inflammatory response is mediated by a complex network of interactions between numerous molecular and cellular components of the immune system. Dysregulation of this complex network contributes to chronic inflammation. Available data on abnormalities in the molecular components of the innate immune system associated with schizophrenia are presented in Table 2.
Pattern-Recognition Receptors
C-reactive protein is an acute-phase protein and one of the first discovered extracellular soluble PRRs. Several meta-analyses have confirmed that blood CRP levels are elevated in schizophrenia. In one of the first meta-analyses, Miller B. et al. showed that CRP levels were significantly elevated in patients with schizophrenia compared to healthy individuals (17). The prevalence of elevated CRP levels in patients with schizophrenia was 28%. Another study showed that CRP levels were moderately elevated in schizophrenia and that antipsychotic medication had no effect on CRP levels (18). Another meta-analysis also confirmed these observations (19). More recently, Osimo E.F. et al. reported that patients with high (>3 mg/L) CRP levels had a greater risk of psychosis compared to patients with low (≤3 mg/L) baseline CRP levels (pooled odds ratio was 1.5) (20). However, this association decreased after excluding participants with suspected infection (CRP > 10 mg/L). Besides, CRP levels have been associated with a risk of increased positive symptoms, cognitive impairment, microbiota disturbance, and metabolic syndrome in subjects with schizophrenia (21). Evidence for elevated CRP levels in schizophrenia has a low risk of bias (Table 2).
Other extracellular soluble PRRs have been less studied in schizophrenia. Soluble CD14 (sCD14) levels but not LBP levels were elevated in schizophrenic patients compared with healthy controls (22). The level of MBL did not differ between patients with schizophrenia and healthy controls (23). These data may indicate increased intestinal permeability in schizophrenia (see below).
Studies of membrane-bound PRRs are rare and controversial. There is evidence that TLR1, TLR2, TLR4, TLR6, and TLR9 expression levels in peripheral blood mononuclear cells (PBMCs) are reduced in schizophrenia, TLR3 and TLR7 levels are elevated, and TLR5 and TLR8 levels do not differ from controls (24). In another study, TLR5 and TLR8 expression levels in PBMCs decreased in drug-naïve first-episode psychosis individuals before and after treatment compared to controls (25). A lower functional activity of TLRs after agonist activation has also been shown. TLR3 but not TLR4 expression levels were higher in PBMCs of drug-naïve patients (26). The therapy did not affect the expression level of these receptors. In another study, increased expression of TLR4 and TLR5 in PBMCs was found in drug-naive patients (27). TLR4 expression returned to normal after treatment. Generally, further studies of changes in TLRs expression on various immune cells and their functional activity in schizophrenia are needed.
Studies of cytoplasmic PRRs are also rare in schizophrenia. A recent report showed that increased expression of NLRP3 and NLRC4, together with high baseline levels of circulating components of IL-18, was observed in the blood of patients with schizophrenia and bipolar disorder compared with healthy controls (28). Also, a correlation between the levels of cholesterol types in the blood and the expression of inflammasome system elements in these diseases has been shown. There is also evidence that NLRP3 and NLRC4 in microglia and astrocytes mediate sterile inflammasome activation and neuroinflammation (29). However, more research is needed. Data on the expression level of cytoplasmic PRRs and the activity of inflammasomes may expand our understanding of the activation of inflammatory pathways in schizophrenia.
Complement System
The complement system is involved in initiating the inflammatory response. A recent systematic review showed that the activity of the classical complement pathway did not differ between schizophrenia and controls (30). The meta-analysis results in this study showed that serum levels of complement components C3 and C4 did not differ between schizophrenic patients and controls. This evidence has a moderate risk of bias (Table 2). However, there is evidence of increased levels of C4 in the cerebrospinal fluid in patients compared with healthy individuals after adjusting for sex and age (31). Complement component C4 is known to mediate synaptic pruning (32). Therefore, the study of anomalies in C4 and other complement system components may be of translational importance.
Cytokines and Chemokines
Cytokines secreted by activated immune cells orchestrate the effector functions of the immune response. Although cytokines regulate not only innate but also adaptive responses, we have placed them in this section.
Changes in cytokine levels in schizophrenia are probably the most studied but controversial area of research in biological psychiatry. Numerous systematic reviews and meta-analyses have been published on serum cytokines in schizophrenia (33–39). Despite some controversy, all of these meta-analyses suggest cytokine dysregulation in schizophrenia and a predominance of pro-inflammatory cytokines in the serum of patients. Levels of interleukin (IL)-6, IL-1β, IL-12, tumor necrosis factor (TNF)-α, and transforming growth factor (TGF)-β were elevated in schizophrenia, while levels of IL-2, IL- 4, and IL-17 did not change (38). Cytokine levels differ in first-episode psychosis patients, acutely relapsed inpatients, and chronic patients. Also, cytokine levels are associated with the severity of clinical symptoms. Besides, antipsychotic treatment significantly affects cytokine levels (40–42). The levels of cytokines in the cerebrospinal fluid of patients are also altered. In particular, the levels of IL-1β, IL-6, and IL-8 are significantly elevated in the cerebrospinal fluid of patients compared to healthy controls (43, 44). Interestingly, IL-8 is a chemotactic cytokine or chemokine CXCL8 and promotes neutrophil and monocyte chemotaxis. IL-8 increased not only in the cerebrospinal fluid (43, 44), but also in the serum of first-episode psychosis patients and multiple-episode schizophrenia individuals, as confirmed by meta-analyses (45, 46). Among other chemokines, monocyte chemoattractant protein 1 (MCP-1 or CCL2), macrophage inflammatory protein-1β (MIP-1β or CCL4), and eotaxin-1 (CCL11) were also elevated in serum of patients (46). The serum levels of MIP-1α (CCL3), fractalkine (CXCL1) and interferon gamma-induced protein 10 (IP-10 or CXCL10) did not differ between patients and controls (46). Importantly, MCP-1 (CCL2) serum levels were higher in first-episode psychosis and multiple-episode schizophrenia, while MIP-1β (CCL4) and eotaxin-1 (CCL11) levels were higher only in multiple-episode schizophrenia (46). Thus, chemokine levels are also altered in schizophrenia (47).
Altogether, the available evidence for changes in the levels of cytokines and chemokines in schizophrenia has a low risk of bias (Table 2), so they can be considered reliable.
Natural Antibodies
Natural antibodies are germline-encoded immunoglobulins found in individuals without prior antigenic exposure (48). They are also characterized as oligospecific low-affinity immunoglobulins that recognize phylogenetically conserved epitopes. Natural antibodies bind exogenous (mainly bacterial) and self-components (apoptotic cell fragments, oxidative neo-epitopes, and other autoantigens, including DAMPs). Natural antibodies perform homeostatic functions and mitigate autoimmune and inflammatory responses.
Natural antibodies to intestinal bacteria have been found in patients with schizophrenia. In particular, according to a meta-analysis, IgA, IgM, IgG to gram-negative bacteria, LPS, and anti-Saccharomyces cerevisiae antibodies are present in patients with schizophrenia (49). This evidence has a moderate risk of bias (Table 2). The formation of such antibodies may be associated with gut dysbiosis and increased intestinal permeability. Data from this meta-analysis of increased blood levels of alpha-1-antitrypsin, lipopolysaccharide-binding protein (LBP), soluble CD14 (sCD14) also suggest an increase in intestinal permeability in schizophrenia.
Natural IgM and IgA to cytokines/chemokines, tryptophan catabolites (TRYCATs), NO-cysteinyl, malondialdehyde, and other oxidative specific epitopes were found in patients with schizophrenia (50). Changes in the level of these specific natural antibodies are associated with some clinical phenotypes of schizophrenia. For instance, patients with deficit schizophrenia exhibit increased IgA responses directed to quinolinic acid, picolinic acid, and xanthurenic acid and relatively lowered IgA levels to anthranilic acid and kynurenic acid when compared to patients with nondeficit schizophrenia (51). Increased production of IgA to TRYCATs was significantly associated with the severity of anxiety and depression symptoms in schizophrenia (52). Besides, deficit schizophrenia is accompanied by a significant decrease in IgM to TRYCATs (53). A decrease in natural IgM to malondialdehyde is associated with schizophrenia with severe psychomotor retardation (54). The authors of these studies indicate that IgM and IgA to TRYCATs are regulatory, so a reduced IgM response to TRYCATs may indicate downregulation of the TRYCATs pathway in schizophrenia (50).
The pool of natural antibodies includes antibodies with catalytic properties (55). Such antibodies bind and catalytically modify the antigen. Catalytic antibodies are only a minor part of natural antibodies but significantly enhance the immune system’s functionality (55). Catalytic antibodies hydrolyzing DNA (56), RNA and miRNA (57, 58), histones (59), and myelin basic protein (MBP) (60, 61) have been described in schizophrenia. Notably, these antigens are DAMPs. Therefore, on the one hand, their hydrolysis and removal can mitigate the immune response to these antigens. On the other hand, an increase in the level of catalytic antibodies is accompanied by immune dysfunction. Besides, some catalytic antibodies may be pathogenic. Indeed, MBP-hydrolyzing antibodies of autistic spectrum disorder patients have been shown to reduce long-term potentiation in the rat hippocampus (62). Consequently, further research into the role of natural catalytic antibodies in schizophrenia is needed.
Cellular Components of the Innate Immune System
Leukocytes play a central role in the functioning of innate immunity. Leukocytes include natural killer cells, dendritic cells, neutrophils, macrophages, eosinophils, basophils, and monocytes. Summarized data on changes in the cellular components of the innate immune system are presented in Table 3. These changes will be discussed in more detail below.
Natural Killers
Natural killers are cells of the innate immune system with solid cytolytic activity. These cells protect host against microbial agents and tumors (63). In the context of mental diseases and schizophrenia NK cells are rarely studied. One 2013 meta-analysis on lymphocytes in schizophrenia describes NK cells. The authors of the study demonstrate both a relative and absolute increase in the number of NK cells in patients with schizophrenia compared with healthy donors (64). This evidence has a moderate risk of bias (Table 2). However, later studies show contradictory results. For example, works published later than the meta-analysis (2018 and 2021, respectively) indicate that the level of NK cells may be lower than that of healthy donors (65) or not change at all (66). Tarantino et al. (66) also describe an increase in HLA-DR expression on activated CD56+ cells in patients with schizophrenia. The expression of this receptor positively correlates with the expression of activated NK cell group 2 isoform C (NKG2C) receptor (overexpression of this receptor is usually characteristic of viral diseases, especially cytomegalovirus infection). In turn, the NK cell-activating receptor also known as Nkp46 receptor or CD335 (one of the main inducers of the cytotoxic properties of NK cells) significantly decreased in patients with schizophrenia. It correlated negatively with the expression of HLA-DR.
Dendritic Cells
Dendritic cells are highly specialized antigen-presenting cells responsible for initiating the primary immune response (67). Studies describing dendritic cells in schizophrenia are practically not found. Only one case-control study of 2009 (68) demonstrates a significant relative decrease in dendritic cells compared to healthy donors.
Neutrophils
Neutrophils are among the most studied cells of the immune system in schizophrenia. The neutrophil-to-lymphocyte ratio is a good marker of immune system dysregulation in various diseases and is used as a prognostic criterion (69). The second major area of work is investigating the production of reactive oxygen species by neutrophils in schizophrenia. Three new meta-analyses (2019, 2020, and 2020, respectively) demonstrate an increase in the level of neutrophils in the blood of patients with schizophrenia and an increase in the neutrophil-to-lymphocyte ratio (70–72). Several studies describe an increase in neutrophil production of reactive oxygen species in schizophrenic patients (73–75). The evidence presented has a low risk of bias (Table 3). All of the above studies point to a possible active role of neutrophils in the pathogenesis of schizophrenia. Pinkhas S. et al. (73) states that bactericidal activity in patients with schizophrenia has not changed. Sometimes not quite usual correlations between neutrophils and manifestations of the disease are investigated. Fang S-H et Al. found that the higher the neutrophil-to-lymphocyte ratio in patients, the lower their quality and quantity of sleep (76).
Eosinophils and Basophils
Eosinophil activity is mainly associated with allergic reactions, fungal infections, and parasitic infestations (77). In this regard, studies of eosinophils in schizophrenia are pretty rare. A 2020 meta-analysis states that in all the studies reviewed, the level of eosinophils in the blood of patients with schizophrenia did not differ from the level in healthy donors (72). One case-control study, published the same year, describes a significant increase in the concentration of eosinophils in the blood of unmedicated schizophrenia patients (78). Jakobsen M. I. et Al. in 2018 published a comparison of the concentration of various immune cells in the blood of male and female patients with schizophrenia (79). The only statistically significantly different cells in the two groups were eosinophils. Women had significantly fewer of them than men. In 2008, a study was published stating that in patients with schizophrenia, the concentration of CCL11/Eotaxin, one of the proteins of eosinophil chemotaxis, significantly increases in the blood (80). In 2013, Fernandez-Egea E. et Al. found an increase in the same protein in the blood of young, healthy volunteers who use cannabis. Since there is a pattern between cannabis use and the incidence of schizophrenia, CCL11/Eotaxin may somehow be involved in the pathogenesis of schizophrenia (81). As for eosinophils, the main activity of basophils is associated with allergic reactions and multicellular parasites (82). Studies of basophils in schizophrenia are practically non-existent. One 2020 meta-analysis states that basophil counts are not significantly different from healthy donors (72). Overall, the evidence presented for no difference in eosinophils and basophils in schizophrenia has a moderate risk of bias (Table 3).
Monocytes
Monocytes are one of the key players in the inflammation process. It was previously believed that monocytes are a temporary link before their transformation into tissue phagocytes (83). Even though the transformation of monocytes into macrophage and dendritic subpopulations is still one of the essential functions of monocytes, it has now been established that these cells also have their immune activities in tissues. So, they can play the role of short-lived tissue effector cells (84). Three new meta-analyses (2019, 2020, and 2020, respectively) demonstrate an increase in the level of monocytes in the blood of patients with schizophrenia and an increase in the neutrophil-to-lymphocyte ratio (70–72). This evidence has a low risk of bias (Table 3). In addition to leaving the tissues and forming an increased pool of tissue phagocytes, monocytes in the blood of patients with schizophrenia also have their damaging activities. Kowalski J. et al. in 2001 described the increased production of pro-inflammatory cytokines such as IL-1β and TNF-α by monocytes (85). Beumer W. et al. (86) also show the increased content of circulating monocyte-associated pro-inflammatory cytokines in patients with schizophrenia.
Macrophages
An increased concentration of macrophages in patients with schizophrenia is found in the cerebrospinal fluid (87). In perivascular spaces (88, 89), and subependymal zone (90), CD163+ cells are found in significantly higher amounts in patients with schizophrenia than in healthy donors. Studies describe excessive production of macrophage-associated pro-inflammatory cytokines (86). Expression of the CD68 receptor on macrophages of schizophrenic patients does not change compared to healthy controls (91, 92).
Impairments in Adaptive Immunity in Schizophrenia
Changes in the Cellular Components of Adaptive Immunity
The main cellular components of adaptive immunity are T and B cells. Changes in their level are summarized in Table 4 and discussed in more detail below.
Lymphocytes (Total)
A 2020 meta-analysis (72) describes unchanged total lymphocyte counts in patients with schizophrenia compared with healthy donors. This evidence has a moderate risk of bias (Table 4). Studies not included in the meta-analysis demonstrate elevated levels of lymphocytes (93), and vice versa, more common lymphopenia in patients with schizophrenia (94).
B Cells
B cells are attracting the attention of researchers due to their ability to produce autoantibodies that may play a negative role in the pathogenesis of schizophrenia. Ezeoke A. et Al. in their systematic review found 20 different autoantibodies whose titers were significantly higher in patients with schizophrenia than in healthy donors (95). A 2019 systematic review (96) analyzed studies on B cells’ level in the blood of patients with schizophrenia. Sixteen of the twenty-two studies found no change in the blood concentration of B cells in patients with schizophrenia (two studies included only the group of patients on therapy), two studies found a decrease in B cells (in both studies, patients were medicated), and four – increase in concentration (two studies included only a group of patients were medicated). Increased CD20+ cell density has been found in the hippocampus of patients with schizophrenia (97). B cells may contribute to the pathogenesis of schizophrenia not so much by changing their number but by changing the composition of synthesized autoantibodies. For more information on autoantibodies in schizophrenia, see section “Changes in the Molecular Components of Adaptive Immunity: Antibodies and Autoimmunity in Schizophrenia.”
T Cells
The idea of T cell pathogenesis of schizophrenia first appeared in 1980 (6). These ideas turned into theory in 1992–1995 (7, 8). The ability of T cells to induce neuroinflammation and neurodegeneration maintains an interest in these cells in the context of the pathogenesis of schizophrenia (98). A 2013 meta-analysis (64) describes an increase in the absolute level of CD3+ and CD4+ cells and an increase in the CD4/CD8 ratio in the blood of patients with schizophrenia relative to healthy donors. This evidence has a moderate risk of bias (Table 4). An increase in T cell concentration has also been shown for CSF (99) and the hippocampus (97) of schizophrenic patients. Muller et al. in 1998 showed a significant increase in CD8+γ/δ+ lymphocytes (100). Mazzrello et al. in 2004 demonstrated a significant decrease in the percentage of CD8+ cells (101). Two 2007 case-control studies showed a decrease in T cell proliferative response (102, 103). In 2014 Ding et al. (104) demonstrated higher proportions of Th17 cells relative to healthy donors.
Changes in the Molecular Components of Adaptive Immunity: Antibodies and Autoimmunity in Schizophrenia
Antibodies are the main effectors of the second line of defense of the immune system. Alterations in the level or reactivity of antibodies are associated with various pathologies. The available evidence for altered antibody levels or reactivity to microbial or self-antigens in schizophrenia is summarized in Table 5.
Antibodies to Microbial Antigens
Parasitic, bacterial, and viral infections are associated with the risk of developing schizophrenia. In a population-based register study of Benros M. E. et al., it was shown that hospitalization due to any infection increased the risk of schizophrenia by 60%, and in the presence of an autoimmune and infectious disease, the risk increased by 125% (105). Prenatal or childhood infections also increase the risk of schizophrenia (106, 107).
The most marked association of schizophrenia is shown with parasitic infection caused by Toxoplasma gondii. The meta-analysis by Torrey E. F. et al. showed that patients with schizophrenia had an increased prevalence of antibodies (primarily IgG class) against Toxoplasma gondii (108). According to another meta-analysis, 7.6% of individuals with acute psychosis had IgM antibodies to Toxoplasma gondii, and the risk of detecting such antibodies was 1.7-fold higher compared to healthy controls (109). The association was stronger in chronic schizophrenia than in first-episode psychosis. An association with a geographic region is also revealed. In a meta-analysis by Sutterland A. L. et al., an association with Toxoplasma gondii infection has been confirmed for schizophrenia as well as bipolar disorder, obsessive-compulsive disorder, and addiction, but not for major depression (110). Thus, evidence for an association of schizophrenia with Toxoplasma gondii infection has a low risk of bias (Table 5). A statistically significant association of schizophrenia (including the presence of antibodies to relevant pathogens) has been found with infections other than Toxoplasma gondii, including Chlamydophila pneumoniae, Chlamydophila psittaci, Human Endogenous Retrovirus W, Human Herpesvirus 2, and Borna Disease Virus (111). Deficit schizophrenia was associated with seropositivity for antibodies to cytomegalovirus (112). But this evidence was moderately biased (Table 5). Nevertheless, association does not imply causation, so studies on the role of antibodies to microbial antigens in the etiopathogenesis of schizophrenia are needed.
Autoantibodies
There is no convincing evidence that schizophrenia is an autoimmune disease. However, some of the observations found in psychosis are consistent with modified Witebsky’s postulates of autoimmune pathogenicity, suggesting that illness in some subgroups of patients has an autoimmune component (113). Some studies indicate that schizophrenia is associated with an increased prevalence of autoimmune diseases and the presence of autoantibodies. Schizophrenia has been associated with several autoimmune diseases, including autoimmune hepatitis, multiple sclerosis, rheumatoid arthritis, systemic lupus erythematosus, autoimmune thyroiditis, Graves’ disease, type 1 diabetes, Guillain-Barré syndrome, and psoriasis (114). These diseases significantly increase the risk of developing schizophrenia and vice versa. Conversely, the risk of subsequent autoimmune diseases is significantly increased in schizophrenia, especially with concomitant infectious pathology (115).
Infections, antigenic mimicry, and other unknown causes contribute to the breakdown of Infections, antigenic mimicry, and other unknown causes contribute to auto-tolerance breakdown, the activation of autoimmune mechanisms, and the generation of autoantibodies in schizophrenia. Environmental toxic effects, metabolic disorders, oxidative stress can also cause changes in the antigenic properties of self-molecules in schizophrenia (116). This can lead to the recognition of autoantigens by the immune system and the generation of autoantibodies. Ezeoke A. et al. showed in a systematic review that an increase in the prevalence of 20 different autoantibodies was observed in patients with schizophrenia compared with controls (95). In particular, antinuclear antibodies, anti-cardiolipin IgG and IgM, anti-double-stranded DNA (dsDNA), and anti-single-stranded DNA (ssDNA), anti-gliadin, anti-histone, and other autoantibodies have been found. Nevertheless, marked study heterogeneity and inconsistency have been observed.
Patients with various forms of psychosis showed the presence of antibodies to multiple neuroantigens, including ionotropic glutamate receptors (N-Methyl-D-Aspartate Receptor (NMDAR) and α-amino-3-hydroxy-5-methyl-4-isoxazolepropionic acid receptor (AMPAR)), dopamine D2 receptors, metabotropic GABA receptors, voltage-gated potassium channels, M1 and M2 cholinergic receptors (117–119). One early meta-analysis showed that 7.98% of patients with schizophrenia were anti-NMDAR antibody positive. Of these, 1.46% had antibodies of the IgG subclass (120). Another meta-analysis showed that elevated titers of anti-NMDAR antibodies in patients with schizophrenia were detected three times more often than in healthy people (121). Antibodies of various classes (IgG, IgM, and IgA) against NR1, NR1/NR2B, and NR2A/NR2B subunits were found in patients. Also, NR2A/NR2B antibody levels were significantly higher in patients with first-episode psychosis than in healthy controls (121). Evidence-based umbrella review also confirms increased NMDAR seropositivity in schizophrenia (122). Thus, the evidence for increased anti-NMDAR seropositivity in schizophrenia has a low risk of bias (Table 5).
In general, results on anti-NMDAR antibodies in schizophrenia varied significantly. Some studies failed to detect anti-NMDAR antibodies in any patient with psychosis (123), while others reported high seropositivity in both patients with psychosis (9,4%) and healthy controls (8,5%) (124). More recently, Cullen A.E. et al. reported that this heterogeneity in results was significantly associated with assay type, illness stage and study quality (125). The use of a live cell-based assay resulted in higher estimates of anti-NMDAR antibody prevalence. The prevalence of anti-NMDAR seropositivity was more elevated in exclusively first-episode psychosis samples than in mixed or multi-episode samples. Therefore, it is necessary to standardize assay procedures and consider clinical factors to obtain more accurate estimates of anti-NMDAR antibody prevalence in schizophrenia.
Anti-NMDAR antibodies are biomarkers for autoimmune encephalitis. Detection of anti-NMDAR antibodies in subgroups of patients without neurological symptoms made it possible to identify a new nosological entity, autoimmune psychosis (126). There is evidence that anti-NMDAR antibodies can induce internalization and reduce the density of surface NMDARs expressed in hippocampal neurons (127). Further research is needed to identify the role of anti-NMDAR antibodies in provoking psychotic symptoms.
Other anti-neuronal antibodies have been found in schizophrenia. Meta-analysis showed that a prevalence of autoantibodies to glutamic acid decarboxylase (GAD65) was 4.6% in schizophrenia compared to 2.7% in healthy controls (128). The prevalence of antibodies to the voltage-gated potassium channel (VGKC) complex was 1.5% in psychoses compared to 0.7% in healthy controls (128). This evidence has a moderate risk of bias (Table 5). Other circulating IgG autoantibodies have also been detected in schizophrenia (129). It is also shown that the treatment affects the level of antibodies. The generation of autoantibodies may be associated with an impaired blood-brain barrier in schizophrenia. This is indicated by the results of a recent meta-analysis (130). An increased level of total protein in the cerebrospinal fluid (CSF) and elevated CSF/serum albumin ratio was revealed in schizophrenia.
Some evidence suggests an association between gluten sensitivity and schizophrenia (Table 5). A meta-analysis showed that biomarkers of gluten sensitivity (anti-gluten, IgG and IgA to gliadin, anti-transglutaminase 2 IgA, and anti-wheat antibodies) were elevated in various forms of psychosis compared with healthy donors (131). This is consistent with the results of other work that examined the reactivity of antibodies toward gluten proteins in schizophrenia (132). It was shown that the reactivity of antibodies to gluten in patients with schizophrenia differed from that in people with celiac disease. In particular, gluten-sensitive schizophrenic patients did not show elevated levels of anti-transglutaminase 2 IgA. Thus, the immune response to gluten in schizophrenia and celiac disease is different.
Generally, antibodies with different reactivity have been found in schizophrenia, but their pathological functions remain elucidated.
Changes in the Immune System Organs Associated With Schizophrenia
The previously described changes in molecular and cellular immunity may reflect the pathology of the immune system organs in schizophrenia. However, studies at the level of immune system organs are limited. Available data are summarized in Table 6.
The bone marrow is the site of hematopoietic stem cells that generate all immune cells. The first mention of bone marrow anomalies in schizophrenia was published in 1964 (133). But since then, there have been few studies of bone marrow in schizophrenia, which are represented mainly by clinical cases. There is an intriguing report of the induction of severe chronic psychosis after allogeneic stem cell transplantation from a donor with schizophrenia (134). In another case, persistent remission of psychotic symptoms in treatment-resistant schizophrenia after bone marrow transplantation from a healthy donor is reported (135). There is also evidence that severe megaloblastic anemia may be accompanied by psychotic symptoms (136). These data may indicate the involvement of bone marrow anomalies in the pathogenesis of schizophrenia, but further research is required to identify specific mechanisms and pathways. However, some changes may be associated with antipsychotic therapy. Some antipsychotics (olanzapine, clozapine, phenothiazines, etc.) are known to cause agranulocytosis, neutropenia, monocytopenia, and thrombopenia (137–139).
The thymus is the second most crucial primary organ of the immune system in which T cell lymphopoiesis occurs. The T cell abnormalities outlined in Section “T Cells” may be associated with thymus dysfunction. Kinney et al. proposed the hypothesis of abnormal immune system development and thymus dysfunction in schizophrenia (140, 141). This hypothesis suggests that schizophrenia is associated with pre- or perinatal exposure to adverse factors that cause latent immune vulnerability. The manifestation of this vulnerability is related to changes in immune function and thymus involution after puberty. Individuals become more susceptible to infections that contribute to schizophrenia (140, 141). However, there are no studies of the thymus in schizophrenia, and this hypothesis requires experimental confirmation. Patients with 22q11.2 deletion syndrome can help reveal the immunological basis of thymus dysfunction and psychosis. With a variety of clinical manifestations, including congenital heart disease, velopharyngeal insufficiency, and recurrent infections, such patients have thymic hypoplasia and suffer from psychotic symptoms (142, 143). Moreover, about 30% of patients with this syndrome develop schizophrenia, with significantly increased risk (about 12 to 80 folds) than the general population (144). There is evidence of an increased percentage of Th17 in adults with psychotic symptoms compared with non-psychotic adults with 22q11.2 deletion syndrome (145). These data highlight the importance of Th17 cells in developing psychiatric symptoms.
The glymphatic system and meningeal lymphatic vessels are involved in removing waste products from the central nervous system. The meningeal lymphatic vessels drain waste products from the cerebrospinal and interstitial fluid to the deep cervical lymph nodes. Impairment of meningeal lymphatic function induced cognitive impairment in mice (146). The cognitive and social deficits observed in schizophrenia may also be associated with lymphatic dysfunction. There is evidence of a change in the functional activity of the lymphoid tissue of the inguinal lymph nodes in patients with schizophrenia (147). In particular, a higher amount of blast forms of the lymphocytes and activated lymphocytes and a high pynocytose activity of the reticular cells and macrophages was found in schizophrenia. But this study turned out to be the only one directly related to schizophrenia, so further research is needed.
Like the lymph nodes, the spleen is a secondary organ of the immune system. Studies of the spleen and its function in schizophrenia are also rare. Decreased expression of the colony-stimulating factor 1 receptor (CSF1R) was found in the spleen but not in the cerebellum and parietal cortex in schizophrenia compared with controls (148). CSF1R interacts with the transcription factor PU.1 (SPI1) and regulates microglia and macrophages’ proliferation, differentiation, and survival. The SPI1 levels in the cerebellum and spleen were significantly higher in the schizophrenia group than in the control group (148). There is also evidence that clozapine affects the expression of activity-dependent neuroprotective protein 2 (ADNP2) in the spleen of mice, which may indicate treatment-induced autophagy dysfunction. These data may indicate the existence of the brain-spleen axis, whose dysregulation may contribute to schizophrenia.
The liver is also involved in immunity as it synthesizes acute phase proteins and contains various subpopulations of lymphocytes. There is a hypothesis about liver dysfunction and schizophrenia (149). Patients with schizophrenia exhibited a significant prevalence of chronic liver diseases than those in the general population (150, 151). The meta-analysis also found an approximately 3-fold increase in the risk of hepatitis B and C in patients with schizophrenia (152). The prevalence of hepatitis B and C in schizophrenia was 6 and 7%, respectively. But at the same time, according to a meta-analysis, patients with schizophrenia have a lower risk of liver cancer (153). Liver dysfunction in schizophrenia may be associated with changes in gene expression. For example, BDNF expression levels from the liver in schizophrenic patients were significantly higher than in healthy controls (154). Interestingly, there were no differences in BDNF expression in the spleen. A negative correlation was observed between mature BDNF in the parietal cortex and mature BDNF in the liver. Apolipoprotein A1 levels were reduced in the liver as well as in the brain, red blood cells, cerebrospinal fluid, and serum of schizophrenic patients (155). In another study, 14 proteins were shown to be significantly altered in liver samples from schizophrenia patients compared to healthy controls, with some of these proteins being related to oxidative stress (156). Typical and atypical antipsychotics are also known to alter gene expression in the liver of patients (157). Therefore, dysregulation of the brain-liver axis may be associated with schizophrenia.
Liver dysfunction could potentially be related to the gut microbiome (158). According to the “gut-liver” axis concept, a shift in gut microbiota composition can result in dysfunction of the gut mucosal barrier (“leaky gut” syndrome). This, in turn, can lead to increased translocation of bacteria to the liver via the portal vein, activation of the immune response, and migration of immune cells to the liver, which likely plays a crucial role in liver dysfunction (159). Interestingly, air pollution and long-term NO2 exposure are associated with disruption of the gut microbiome and liver dysfunction in schizophrenia (160).
Increased intestinal permeability (“leaky gut” syndrome) leading to the inappropriate access of antigens to the gut-associated lymphoid tissue plays an essential role in peripheral and neuroinflammation (161). Increased leakage of the tight epithelial junctions of the intestine in schizophrenia may be associated with changes in zonulin (pre-haptoglobulin 2) concentrations. Zonulin is a protein that increases small intestine permeability and contributes to innate intestinal immunity. Remarkably, increased serum zonulin levels have been observed in schizophrenia patients (162, 163). Also, significant associations of gene polymorphisms of haptoglobulin (a product of zonulin processing) are found in schizophrenia (164).
As an essential constituent of the mucosal immune system, gut-associated lymphoid tissue may also be associated with the development of schizophrenia. Usually, immune cells in gut-associated lymphoid tissue can be activated in response to both specific antigens and microbial signals, including lipopolysaccharide, flagellin, or DNA. The microbiota also influences immune cells through metabolic products such as tryptophan metabolites and short-chain fatty acids. For example, short-chain fatty acids activate and maintain Treg cells through G-protein-coupled receptors (165). Several studies indicate that activation of brain autoreactive T cells occurs in the gut-associated lymphoid tissue (166). On the one hand, resting circulating autoreactive T cells receive positive stimulatory signals from particular microbial subsets of the gut microbiota. On the other hand, a deficiency in inhibitory signals from luminal bacteria impairs local Treg induction and function, promoting autoreactive T cells’ activation (166). Significant changes in the microbiota composition (including mycobiota and phageome) were found in the oropharynx and intestines of patients with schizophrenia compared to non-psychiatric controls (167–171). Moreover, microbiota dysregulation or dysbiosis has been associated with behavioral anomalies in rodent studies (172). Notably, antipsychotic treatment may contribute to gut microbiome alteration (173). Evidence for microbiota dysregulation in schizophrenia has a low risk of bias (Table 6). Thus, gut microbiota dysregulation in schizophrenia may impair the gastrointestinal barrier, immune homeostasis, brain development, and neurotransmission, which is associated with chronic low-grade inflammation and the formation of autoreactive T cells (161, 166, 174). Therefore, the microbiota-gut-brain axis dysregulation should be considered as an essential factor in the pathogenesis of schizophrenia [for more information, see (175–177)].
Altogether, some changes in the organs of the immune system are characteristic of schizophrenia. But studies of the immune system at the organ level are rare and more research is needed.
Immune-Associated Alterations in the Brain
Activation of peripheral immunity affects the central nervous system and contributes to neuroinflammation in schizophrenia (178). Conversely, neuroinflammation, such as that caused by neuroinfection, induces a systemic pro-inflammatory state (179). Overall, the role of peripheral inflammation in the pathophysiology of schizophrenia is well supported by multiple findings of elevated inflammatory markers in the brains of patients (Table 7). The results of postmortem studies indicate an increase in the expression of many pro-inflammatory genes, including Serpin family A member 3 (SERPINA3) and Interferon-induced transmembrane protein (IFITM) in the brains of schizophrenic patients (180, 181). A recent meta-analysis also showed an increase in pro-inflammatory gene expression at the transcript (TNFα, IL1β, IL6, and IL8) and protein (TNFα and IL1β) levels in the brains of schizophrenic patients compared to healthy individuals (182). These changes may be associated with increased activity of nuclear factor kappa B (NF-κB), which regulates the expression of pro-inflammatory factors (183, 184). Higher NF-κB activity associated with increased expression of NF-κB signaling pathway components found in the prefrontal cortex of patients with schizophrenia (183). These data has a low risk of bias (Table 7). Besides, changes in density and signs of microglia activation were found in the brains of patients. According to a 2015 systematic review, four studies state that microglial cell density increases, while three studies indicate cell density does not change (185). PET studies of microglial activation in patients with schizophrenia have found increased cell activation in the early stages of the disease at the most severe symptomatology (185). A 2019 meta-analysis describes an increase in 18-kDa translocator protein (TPSO) in patients with schizophrenia compared to healthy controls (186). Although there is evidence that central low-grade inflammation in schizophrenia is not always accompanied by increased TSPO expression or ligand binding (187). Also, several studies describe an increase in HLA-DR expression on microglial cells in patients with schizophrenia (91, 188). In some studies, no differences in HLA-DR expression are found (189, 190). Thus, the evidence for microglial activation in schizophrenia has moderate risk of bias (Table 7). Another line of evidence indicates immune cells infiltration of brain tissue (88, 191, 192). Perivascular macrophages (CD163+) have been identified in the brain parenchyma of patients in about 40% of cases of schizophrenia with “severe inflammation” (88). This is associated with increased levels of soluble intercellular adhesion molecule-1 (sICAM1) in serum, mRNA expression of ICAM1 and VE-cadherin in the brain, and enrichment of ICAM1 in the brain endothelium (88). Besides, an increased density of CD3+ T-lymphocytes and CD20+ B-lymphocytes was found in some brain areas in about 1/3 of patients with schizophrenia (191, 192). All these data indicate neurovascular endothelial dysfunction and hyperpermeability of the blood-brain barrier in schizophrenia (193), which can be caused, among other things, by peripheral immune abnormalities.
It should be especially emphasized that signs of neuroinflammation are observed only in certain subgroups of patients. For example, an increase in the expression of pro-inflammatory genes is observed in only about 40% of patients (180). Infiltration of brain tissue by immune cells was also observed in 1/3 of patients (191, 192). Signs of peripheral inflammation and elevated cytokine levels are found in only a subset of schizophrenia patients who are linked to poorer response to antipsychotic treatment (178, 194). Combining data on the levels of peripheral inflammatory markers and post-mortem brain markers using cluster analysis methods, it was found that approximately 30 to 50% of patients with schizophrenia can be classified to a subtype with increased levels of inflammation (195–197). Interestingly, inflammatory subtypes are observed in both antipsychotic naïve first-episode schizophrenia patients (198) and chronic psychosis spectrum patients (197). Moreover, the proportion of patients with a severe inflammatory phenotype is approximately the same for first-episode and chronic patients and is about 36% (197, 198). Thus, subgroups with high inflammation are distinguished among patients.
Importantly, peripheral and neuroinflammation is associated with cognitive and neuroanatomical alterations. Patients with chronic psychosis in the high inflammatory subtype had greater cortical thickness and subcortical volumes than individuals in the low inflammatory subtype (197). Individuals with first-episode schizophrenia in the high inflammatory subtype had greater thickness in the right parahippocampus, bank superior sulcus, and caudal anterior cingulate compared to patients in the low inflammatory subtype (198). Neuroinflammation is also associated with brain white matter pathology, as the density of orbitofrontal white matter neurons was increased in patients with high levels of inflammation (199). Also, plasma CRP levels were inversely correlated with the thickness of the prefrontal cortex of patients (200). Moreover, patients with elevated peripheral inflammatory markers suffer from more severe cognitive impairments than patients without active inflammation (201, 202). For example, patients with elevated serum CRP showed significantly worse working memory than controls (200). Additionally, chronic patients in the high inflammatory subtype had impairments in inhibitory behavioral control and visuospatial working memory (197). Peripheral inflammation measured by highly sensitive CRP levels is also associated with changes in brain perfusion and with psychotic symptom scores (203).
Together, these data indicate that peripheral immune alterations influence the central nervous system and contribute to the pathogenesis of schizophrenia. These findings may also be associated with endothelial dysfunction and hyperpermeability of the blood-brain barrier (193).
Overview of the Immune System Abnormalities in Schizophrenia
We have summarized the previously described abnormalities of the immune system in schizophrenia in Figure 3. Changes in the molecular components of innate immunity are associated with activation of the inflammatory response (Section “Molecular Components of the Innate Immune System”). This is associated with increased extracellular soluble PRRs and abnormal expression of membrane-bound and cytoplasmic PRRs. Activation of the innate immune response is reflected in an increase in pro-inflammatory cytokines in serum and cerebrospinal fluid. Changes in the molecular components of adaptive immunity (Section “Changes in the Molecular Components of Adaptive Immunity: Antibodies and Autoimmunity in Schizophrenia”) are associated with increased levels of antibodies to T. gondii and other pathogens and an increased prevalence of anti-NMDAR and anti-gluten antibodies. Dysbiosis and increased intestinal permeability are associated with natural antibodies to gram-negative bacteria and LPS.
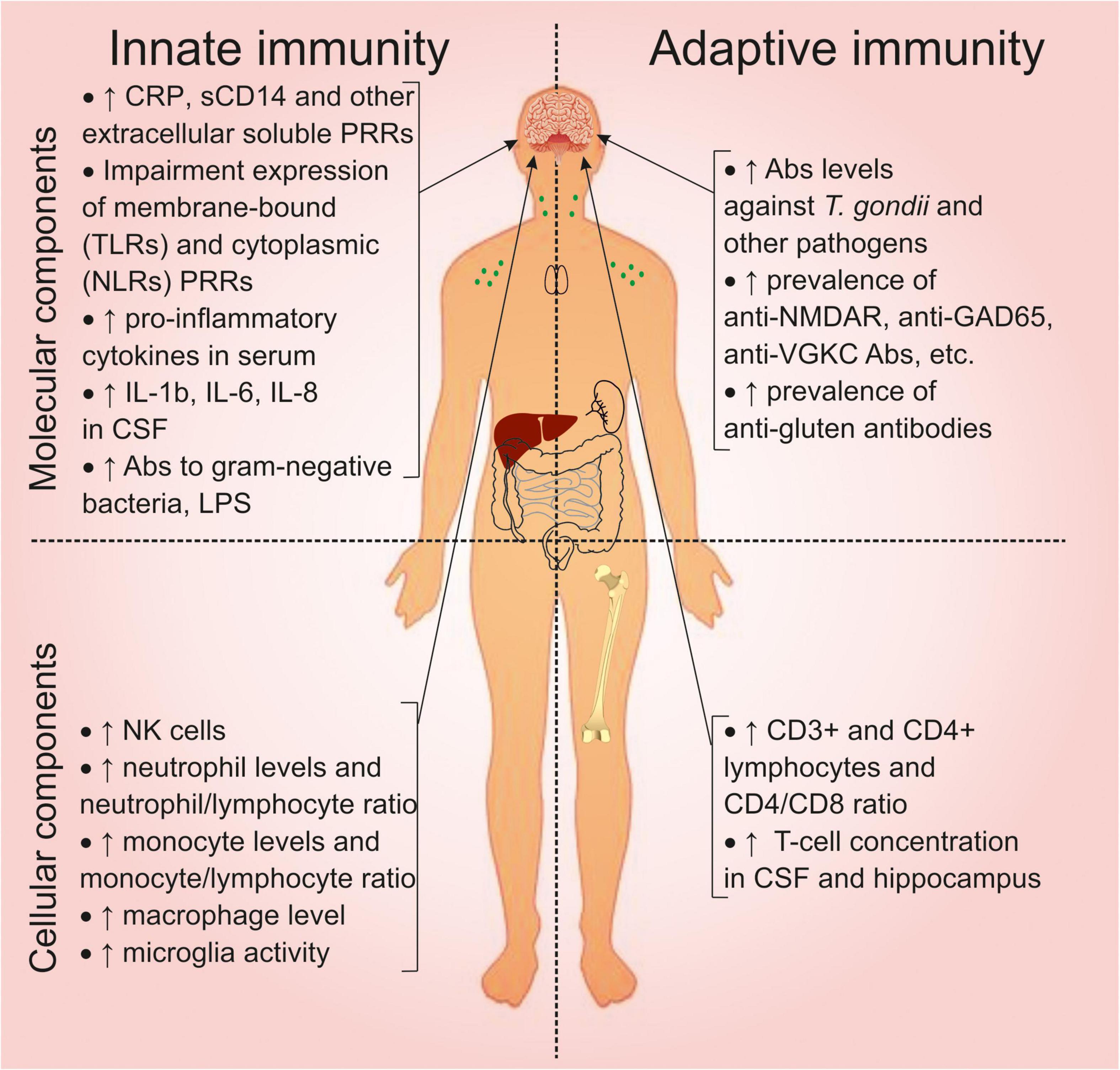
Figure 3. Overview of the immune system abnormalities associated with schizophrenia. CRP, C-reactive protein; CD, cluster of differentiation; PRRs, pattern recognition receptors; TLRs, toll-like receptors; NLRs, (NOD)-like receptors; IL, interleukin; CSF, cerebrospinal fluid; LPS, lipopolysaccharide; NK, natural killer; NMDAR, N-methyl-D-aspartate receptor; GAD, glutamic acid decarboxylase; VGKC, voltage-gated potassium channel.
Abnormalities in the cellular component of innate immunity (Sections “Cellular Components of the Innate Immune System” and “Changes in the Cellular Components of Adaptive Immunity”) are associated with increased levels of NK cells, neutrophils, monocytes, macrophages, and microglia activation. The cellular components of adaptive immunity are characterized by increased CD3+ and CD4+ lymphocytes and T cells in the cerebrospinal fluid. Although the quantitative changes in cells are within the reference values.
All these changes indicate activation of the peripheral immune system. This in turn promotes neuroinflammation, which leads to cognitive and neuroanatomical alterations (Section “Immune-Associated Alterations in the Brain”). However, only a certain subtype (30 to 50% of all patients) exhibits inflammatory phenotype.
Thus, schizophrenia is accompanied by abnormalities in all immune system components.
Usefulness of Immunological Data in Psychiatric Clinical Practice: Stratification of Patients
Schizophrenia is a heterogeneous disease in clinical manifestations and laboratory tests, including immune parameters. The current clinical diagnosis of schizophrenia does not consider the disease heterogeneity and does not allow individualized treatment prescriptions based on neurobiology. As stated above (Section “Immune-Associated Alterations in the Brain”), only some subsets of patients show signs of peripheral and neuroinflammation and respond poorly to antipsychotic treatment (194, 204). Therefore, immunological data may help stratify patients and identify subgroups with distinct phenotypes. However, the usefulness of a single immune-inflammatory marker for patient stratification may be low (205), so multiple parameters should be used. Several attempts have been made to stratify patients with psychosis and identify different endophenotypes based on clinical features (206). However, other researchers suggest that all clinical and biological data should be considered.
There are studies using inflammation-profiling approaches to stratify patients by level of inflammation (204, 207–210). For example, a recent study showed that using data on mRNA transcript levels of 56 immune genes using machine learning algorithms can distinguish between people with first-episode psychosis, chronic psychosis, and controls with >80% accuracy (210). The OPTiMiSE cohort study showed that uncontrolled clustering allowed the identification of clinical subtypes of first-episode psychosis patients (207). For one of the subtypes, lower levels of IL-15, higher levels of the chemokine ligand CXC (CXCL) 12, and prior exposure to cytomegalovirus were shown to be associated with a lack of remission.
Maes M. and colleagues proposed a hypothesis about the Immune-inflammatory response system (IRS) and the Compensatory Immune-Regulatory Reflex System (CIRS) in schizophrenia (50). An increase in the levels of various acute-phase proteins and cytokines/chemokines is associated with the activation of the IRS in schizophrenia. In contrast, CIRS aims to reduce inflammatory and immune responses and includes IL-1 receptor antagonist (sIL-1RA), sIL-2R and tumor necrosis factor-α receptors, Th-2 and Treg producing IL-4 and IL-10 and other components with immunoregulatory and anti-inflammatory effects. Clinical endophenotypes of schizophrenia differ in IRS and CIRS parameters. Therefore, immunological data on changes in IRS and CIRS parameters may better differentiate specific endophenotypes of schizophrenia. Moreover, Maes M. and colleagues propose to combine clinical and molecular immunological data, i.e., use all sets of traits to divide patients into subgroups with different phenotypes. They are supposed to use the nomothetic network approach (211).
Thus, immune-inflammatory data may help identify specific patient subgroups and disease biotypes (212). The use of clustering algorithms and machine learning methods will improve the accuracy of patient stratification (213). This stratification of patients with schizophrenia using inflammatory biosignatures and machine learning may also help identify subgroups of individuals who well respond to anti-inflammatory therapy, especially among antipsychotic-resistant patients.
Unresolved Issues and Promising Future Research Directions for the Immune System in Schizophrenia
This systematic review identified gaps in knowledge about immune abnormalities in schizophrenia. In schizophrenia, soluble PRRs (CRP, sCD14, etc.) have been well studied. However, studies on membrane-bound and cytoplasmic PRRs are rare and controversial. Therefore, it is necessary to focus on changes in the expression level and functional activity of PRRs in schizophrenia. Unraveling these mechanisms will help develop new therapeutic strategies to influence inflammatory responses in schizophrenia. The study of the function of complement system proteins in the brain may also have translation perspectives. The functional role of the detected autoantibodies in schizophrenia is not fully understood. Further research is needed to elucidate the contribution of anti-NMDAR antibodies to the pathogenesis of schizophrenia. Complex changes in cytokine networks may reflect the heterogeneity of schizophrenia. The use of machine learning methods will help unravel this complex interaction in schizophrenia. The cellular components of the immune system are also impaired in schizophrenia, but the reasons for this remain elusive. The bone marrow is the source of all immune cells, so the study of hematopoietic stem cells can shed light on the causes of altered immune cell function. Studies of other immune organs can also help elucidate the state of the immune system in schizophrenia.
Limitations
There are some limitations in the present systematic review. Firstly, it cannot be unequivocally stated that all published works on the topic of the review have been studied by the authors. Some relevant works could escape the attention of the authors. Secondly, meta-analyses and systematic reviews were frequently used in this systematic review, which may lead to the accumulation of Type I errors (214). Thirdly, the bias rating scale was developed by the authors of this work based on the scale from the study by Tanaka et al. (16); this scale may itself be biased.
Conclusion
Schizophrenia is associated with abnormalities in all immune system components: from innate to adaptive immunity and from humoral to cellular immunity. Changes in the immune system indicate the activation of inflammatory responses in about 1/3 of patients with schizophrenia. Peripheral immune abnormalities contribute to neuroinflammation, which is associated with cognitive and neuroanatomical alterations and contributes to the pathogenesis of schizophrenia. Immunological parameters may help identify subgroups of individuals who well respond to anti-inflammatory therapy.
Data Availability Statement
The original contributions presented in the study are included in the article/supplementary material, further inquiries can be directed to the corresponding author/s.
Author Contributions
EE conceptualized the review. EE and MM searched the literature, critically analyzed, and wrote the draft manuscript. VB and SI interpreted and discussed the results. All authors contributed to the article and approved the final manuscript.
Funding
This work was supported by the Russian Science Foundation under grant 21-75-00102 to EE (Sections “Molecular Components of the Innate Immune System,” “Changes in the Immune System Organs Associated With Schizophrenia,” “Immune-Associated Alterations in the Brain,” and “Overview of the Immune System Abnormalities in Schizophrenia”) and 20-15-00162 to VB (Sections “Cellular Components of the Innate Immune System,” “Changes in the Cellular Components of Adaptive Immunity,” and “Changes in the Molecular Components of Adaptive Immunity: Antibodies and Autoimmunity in Schizophrenia”).
Conflict of Interest
The authors declare that the research was conducted in the absence of any commercial or financial relationships that could be construed as a potential conflict of interest.
Publisher’s Note
All claims expressed in this article are solely those of the authors and do not necessarily represent those of their affiliated organizations, or those of the publisher, the editors and the reviewers. Any product that may be evaluated in this article, or claim that may be made by its manufacturer, is not guaranteed or endorsed by the publisher.
References
1. Khandaker GM, Cousins L, Deakin J, Lennox BR, Yolken R, Jones PB. Inflammation and immunity in schizophrenia: implications for pathophysiology and treatment. Lancet Psychiatry. (2015) 2:258–70. doi: 10.1016/S2215-0366(14)00122-9
2. Dameshek W. The white blood cells in dementia praecox and dementia paralytica. Arch Neurol Psychiatry. (1930) 24:855.
3. Lehmann-Facius H. Liquoruntersuchungen bei destruktiven Erkrankungen des Nervensystems besonders bei Schizophrenien. Neurol Psychiatry. (1937) 157:109–15. doi: 10.1007/bf02870716
4. Torrey EF, Peterson MR. The viral hypothesis of schizophrenia. Schizophr Bull. (1976) 2:136. doi: 10.1093/schbul/2.1.136
5. Feigenson KA, Kusnecov AW, Silverstein SM. Inflammation and the two-hit hypothesis of schizophrenia. Neurosci Biobehav Rev. (2014) 38:72–93. doi: 10.1016/j.neubiorev.2013.11.006
6. Yyland H, Ness A, Lunde H. Lymphocyte subpopulations in peripheral blood from schizophrenic patients. Acta Psychiatr Scand. (1980) 61:313–8. doi: 10.1111/j.1600-0447.1980.tb00584.x
7. Smith RS. A comprehensive macrophage-T-lymphocyte theory of schizophrenia. Med Hypotheses. (1992) 39:248–57. doi: 10.1016/0306-9877(92)90117-u
8. Smith RS, Maes M. The macrophage-T-lymphocyte theory of schizophrenia: additional evidence. Med Hypotheses. (1995) 45:135–41. doi: 10.1016/0306-9877(95)90062-4
9. Knight JG, Knight A, Menkes DB, Mullen PE. Autoantibodies against brain septal region antigens specific to unmedicated schizophrenia? Biol Psychiatry. (1990) 28:467–74. doi: 10.1016/0006-3223(90)90480-p
10. Monji A, Kato T, Kanba S. Cytokines and schizophrenia: microglia hypothesis of schizophrenia. Psychiatry Clin Neurosci. (2009) 63:257–65. doi: 10.1111/j.1440-1819.2009.01945.x
11. Kellie S, Al-Mansour Z. Overview of the immune system. In: Skwarczynski M, Toth I editors. Micro and Nanotechnology in Vaccine Development. Norwich: William Andrew Publishing (2017). p. 63–81.
12. Li D, Wu M. Pattern recognition receptors in health and diseases. Signal Transduct Target Ther. (2021) 6:291. doi: 10.1038/s41392-021-00687-0
13. Page MJ, McKenzie JE, Bossuyt PM, Boutron I, Hoffmann TC, Mulrow CD, et al. The PRISMA 2020 statement: an updated guideline for reporting systematic reviews. BMJ. (2021) 372:n71.
14. Page MJ, Moher D, Bossuyt PM, Boutron I, Hoffmann TC, Mulrow CD, et al. PRISMA 2020 explanation and elaboration: updated guidance and exemplars for reporting systematic reviews. BMJ. (2021) 372:n16. doi: 10.1136/bmj.n160
15. Haddaway NR, Pritchard CC, McGuinness LA. PRISMA2020: R Package and ShinyApp for Producing PRISMA 2020 Compliant Flow Diagrams. Zenodo (2021). doi: 10.5281/zenodo.5082518
16. Tanaka M, Vécsei L. Monitoring the redox status in multiple sclerosis. Biomedicines. (2020) 8:406. doi: 10.3390/biomedicines8100406
17. Miller BJ, Culpepper N, Rapaport MH. C-reactive protein levels in schizophrenia: a review and meta-analysis. Clin Schizophr Relat Psychoses. (2014) 7:223–30. doi: 10.3371/CSRP.MICU.020813
18. Fernandes BS, Steiner J, Bernstein HG, Dodd S, Pasco JA, Dean OM, et al. C-reactive protein is increased in schizophrenia but is not altered by antipsychotics: meta-analysis and implications. Mol Psychiatry. (2016) 21:554–64. doi: 10.1038/mp.2015.87
19. Wang Z, Li P, Chi D, Wu T, Mei Z, Cui G. Association between C-reactive protein and risk of schizophrenia: an updated meta-analysis. Oncotarget. (2017) 8:75445. doi: 10.18632/oncotarget.17995
20. Osimo EF, Baxter L, Stochl J, Perry BI, Metcalf SA, Kunutsor SK, et al. Longitudinal association between CRP levels and risk of psychosis: a meta-analysis of population-based cohort studies. NPJ Schizophr. (2021) 7:31. doi: 10.1038/s41537-021-00161-4
21. Fond G, Lançon C, Auquier P, Boyer L. C-reactive protein as a peripheral biomarker in schizophrenia. An updated systematic review. Front Psychiatry. (2018) 9:392. doi: 10.3389/fpsyt.2018.00392
22. Severance EG, Gressitt KL, Stallings CR, Origoni AE, Khushalani S, Leweke FM, et al. Discordant patterns of bacterial translocation markers and implications for innate immune imbalances in schizophrenia. Schizophr Res. (2013) 148:130–7. doi: 10.1016/j.schres.2013.05.018
23. Mayilyan KR, Arnold JN, Presanis JS, Soghoyan AF, Sim RB. Increased complement classical and mannan-binding lectin pathway activities in schizophrenia. Neurosci Lett. (2006) 404:336–41. doi: 10.1016/j.neulet.2006.06.051
24. Kozłowska E, Agier J, Wysokiński A, Łucka A, Sobierajska K, Brzezińska-Błaszczyk E. The expression of toll-like receptors in peripheral blood mononuclear cells is altered in schizophrenia. Psychiatry Res. (2019) 272:540–50. doi: 10.1016/j.psychres.2018.12.138
25. Juncal-Ruiz M, Riesco-Davila L, Vazquez-Bourgon J, Ortiz-Garcia de la Foz V, Son MV, Ayesa-Arriola R, et al. Expression and functionality study of 9 toll-like receptors in 33 drug-naive non-affective first-episode psychosis individuals: a 3-month study. Int J Mol Sci. (2020) 21:6106. doi: 10.3390/ijms21176106
26. Balaji R, Subbanna M, Shivakumar V, Abdul F, Venkatasubramanian G, Debnath M. The pattern of expression of toll-like receptor (TLR)-3 and-4 genes in drug-naïve and antipsychotic treated patients diagnosed with schizophrenia. Psychiatry Res. (2020) 285:112727. doi: 10.1016/j.psychres.2019.112727
27. Kéri S, Szabó C, Kelemen O. Antipsychotics influence Toll-like receptor (TLR) expression and its relationship with cognitive functions in schizophrenia. Brain Behav Immun. (2017) 62:256–64. doi: 10.1016/j.bbi.2016.12.011
28. Szabo A, O‘Connell KS, Ueland T, Sheikh MA, Agartz I, Andreou D, et al. Increased circulating IL-18 levels in severe mental disorders indicate systemic inflammasome activation. Brain Behav Immun. (2022) 99:299–306. doi: 10.1016/j.bbi.2021.10.017
29. Freeman L, Guo H, David CN, Brickey WJ, Jha S, Ting JPY. NLR members NLRC4 and NLRP3 mediate sterile inflammasome activation in microglia and astrocytes. J Exp Med. (2017) 214:1351–70. doi: 10.1084/jem.20150237
30. Mongan D, Sabherwal S, Susai SR, Föcking M, Cannon M, Cotter DR. Peripheral complement proteins in schizophrenia: a systematic review and meta-analysis of serological studies. Schizophr Res. (2020) 222:58–72. doi: 10.1016/j.schres.2020.05.036
31. Gallego JA, Blanco EA, Morell C, Lencz T, Malhotra AK. Complement component C4 levels in the cerebrospinal fluid and plasma of patients with schizophrenia. Neuropsychopharmacology. (2021) 46:1140–4. doi: 10.1038/s41386-020-00867-6
32. Sekar A, Bialas AR, De Rivera H, Davis A, Hammond TR, Kamitaki N, et al. Schizophrenia risk from complex variation of complement component 4. Nature. (2016) 530:177–83. doi: 10.1038/nature16549
33. Potvin S, Stip E, Sepehry AA, Gendron A, Bah R, Kouassi E. Inflammatory cytokine alterations in schizophrenia: a systematic quantitative review. Biol Psychiatry. (2008) 63:801–8. doi: 10.1016/j.biopsych.2007.09.024
34. Miller BJ, Buckley P, Seabolt W, Mellor A, Kirkpatrick B. Meta-analysis of cytokine alterations in schizophrenia: clinical status and antipsychotic effects. Biol Psychiatry. (2011) 70:663–71. doi: 10.1016/j.biopsych.2011.04.013
35. Goldsmith DR, Rapaport MH, Miller BJ. A meta-analysis of blood cytokine network alterations in psychiatric patients: comparisons between schizophrenia, bipolar disorder and depression. Mol Psychiatry. (2016) 21:1696–709. doi: 10.1038/mp.2016.3
36. Upthegrove R, Manzanares-Teson N, Barnes NM. Cytokine function in medication-naive first episode psychosis: a systematic review and meta-analysis. Schizophr Res. (2014) 155:101–8. doi: 10.1016/j.schres.2014.03.005
37. Zhou X, Tian B, Han HB. Serum interleukin-6 in schizophrenia: a system review and meta-analysis. Cytokine. (2021) 141:155441. doi: 10.1016/j.cyto.2021.155441
38. Momtazmanesh S, Zare-Shahabadi A, Rezaei N. Cytokine alterations in schizophrenia: an updated review. Front Psychiatry. (2019) 10:892. doi: 10.3389/fpsyt.2019.00892
39. Rodrigues-Amorim D, Rivera-Baltanas T, Spuch C, Caruncho HJ, Gonzalez-Fernandez A, Olivares JM, et al. Cytokines dysregulation in schizophrenia: a systematic review of psychoneuroimmune relationship. Schizophr Res. (2018) 197:19–33. doi: 10.1016/j.schres.2017.11.023
40. Capuzzi E, Bartoli F, Crocamo C, Clerici M, Carrà G. Acute variations of cytokine levels after antipsychotic treatment in drug-naïve subjects with a first-episode psychosis: a meta-analysis. Neurosci Biobehav Rev. (2017) 77:122–8. doi: 10.1016/j.neubiorev.2017.03.003
41. Tourjman V, Kouassi É, Koué MÈ, Rocchetti M, Fortin-Fournier S, Fusar-Poli P, et al. Antipsychotics’ effects on blood levels of cytokines in schizophrenia: a meta-analysis. Schizophr Res. (2013) 151:43–7. doi: 10.1016/j.schres.2013.10.011
42. Romeo B, Brunet-Lecomte M, Martelli C, Benyamina A. Kinetics of cytokine levels during antipsychotic treatment in schizophrenia: a meta-analysis. Int J Neuropsychopharmacol. (2018) 21:828–36. doi: 10.1093/ijnp/pyy062
43. Gallego JA, Blanco EA, Husain-Krautter S, Fagen EM, Moreno-Merino P, del Ojo-Jiménez JA, et al. Cytokines in cerebrospinal fluid of patients with schizophrenia spectrum disorders: new data and an updated meta-analysis. Schizophr Res. (2018) 202:64–71. doi: 10.1016/j.schres.2018.07.019
44. Wang AK, Miller BJ. Meta-analysis of cerebrospinal fluid cytokine and tryptophan catabolite alterations in psychiatric patients: comparisons between schizophrenia, bipolar disorder, and depression. Schizophr Bull. (2018) 44:75–83. doi: 10.1093/schbul/sbx035
45. Çakici N, Sutterland AL, Penninx BW, Dalm VA, de Haan L, van Beveren NJ. Altered peripheral blood compounds in drug-naïve first-episode patients with either schizophrenia or major depressive disorder: a meta-analysis. Brain Behav Immun. (2020) 88:547–58.
46. Frydecka D, Krzystek-Korpacka M, Lubeiro A, Stramecki F, Stańczykiewicz B, Beszłej JA, et al. Profiling inflammatory signatures of schizophrenia: a cross-sectional and meta-analysis study. Brain Behav Immun. (2018) 71:28–36. doi: 10.1016/j.bbi.2018.05.002
47. Stuart MJ, Baune BT. Chemokines and chemokine receptors in mood disorders, schizophrenia, and cognitive impairment: a systematic review of biomarker studies. Neurosci Biobehav Rev. (2014) 42:93–115. doi: 10.1016/j.neubiorev.2014.02.001
48. Reyneveld GI, Savelkoul HF, Parmentier HK. Current understanding of natural antibodies and exploring the possibilities of modulation using veterinary models. A review. Front Immunol. (2020) 11:2139. doi: 10.3389/fimmu.2020.02139
49. Safadi JM, Quinton AM, Lennox BR, Burnet PW, Minichino A. Gut dysbiosis in severe mental illness and chronic fatigue: a novel trans-diagnostic construct? A systematic review and meta-analysis. Mol Psychiatry. (2021) 27:141–53. doi: 10.1038/s41380-021-01032-1
50. Roomruangwong C, Noto C, Kanchanatawan B, Anderson G, Kubera M, Carvalho AF, et al. The role of aberrations in the immune-inflammatory response system (IRS) and the compensatory immune-regulatory reflex system (CIRS) in different phenotypes of schizophrenia: the IRS-CIRS theory of schizophrenia. Mol Neurobiol. (2020) 57:778–97. doi: 10.1007/s12035-019-01737-z
51. Kanchanatawan B, Sirivichayakul S, Ruxrungtham K, Carvalho AF, Geffard M, Ormstad H, et al. Deficit, but not nondeficit, schizophrenia is characterized by mucosa-associated activation of the tryptophan catabolite (TRYCAT) pathway with highly specific increases in IgA responses directed to picolinic, xanthurenic, and quinolinic acid. Mol Neurobiol. (2018) 55:1524–36. doi: 10.1007/s12035-017-0417-6
52. Kanchanatawan B, Thika S, Sirivichayakul S, Carvalho AF, Geffard M, Maes M. In schizophrenia, depression, anxiety, and physiosomatic symptoms are strongly related to psychotic symptoms and excitation, impairments in episodic memory, and increased production of neurotoxic tryptophan catabolites: a multivariate and machine learning study. Neurotox Res. (2018) 33:641–55. doi: 10.1007/s12640-018-9868-4
53. Kanchanatawan B, Sirivichayakul S, Ruxrungtham K, Carvalho AF, Geffard M, Anderson G, et al. Deficit schizophrenia is characterized by defects in IgM-mediated responses to tryptophan catabolites (TRYCATs): a paradigm shift towards defects in natural self-regulatory immune responses coupled with mucosa-derived TRYCAT pathway activation. Mol Neurobiol. (2018) 55:2214–26. doi: 10.1007/s12035-017-0465-y
54. Maes M, Sirivichayakul S, Kanchanatawan B, Carvalho AF. In schizophrenia, psychomotor retardation is associated with executive and memory impairments, negative and psychotic symptoms, neurotoxic immune products and lower natural IgM to malondialdehyde. World J Biol Psychiatry. (2020) 21:383–401. doi: 10.1080/15622975.2019.1701203
55. Ermakov EA, Nevinsky GA, Buneva VN. Immunoglobulins with non-canonical functions in inflammatory and autoimmune disease states. Int J Mol Sci. (2020) 21:5392. doi: 10.3390/ijms21155392
56. Ermakov EA, Smirnova LP, Parkhomenko TA, Dmitrenok PS, Krotenko NM, Fattakhov NS, et al. DNA-hydrolysing activity of IgG antibodies from the sera of patients with schizophrenia. Open Biol. (2015) 5:150064. doi: 10.1098/rsob.150064
57. Ermakov EA, Ivanova SA, Buneva VN, Nevinsky GA. Hydrolysis by catalytic IgGs of microRNA specific for patients with schizophrenia. IUBMB Life. (2018) 70:153–64. doi: 10.1002/iub.1712
58. Ermakov EA, Ivanova SA, Buneva VN, Nevinsky GA. Blood-derived RNA-and microRNA-hydrolyzing IgG antibodies in schizophrenia patients. Biochemistry. (2018) 83:507–26. doi: 10.1134/S0006297918050048
59. Ermakov EA, Parshukova DA, Nevinsky GA, Buneva VN. Natural catalytic iggs hydrolyzing histones in schizophrenia: are they the link between humoral immunity and inflammation? Int J Mol Sci. (2020) 21:7238. doi: 10.3390/ijms21197238
60. Parshukova D, Smirnova LP, Ermakov EA, Bokhan NA, Semke AV, Ivanova SA, et al. Autoimmunity and immune system dysregulation in schizophrenia: IgGs from sera of patients hydrolyze myelin basic protein. J Mol Recognit. (2019) 32:e2759. doi: 10.1002/jmr.2759
61. Parshukova DA, Smirnova LP, Kornetova EG, Semke AV, Buneva VN, Ivanova SA. IgG-Dependent hydrolysis of myelin basic protein of patients with different courses of schizophrenia. J Immunol Res. (2020) 2020:8986521. doi: 10.1155/2020/8986521
62. Gonzalez-Gronow M, Cuchacovich M, Francos R, Cuchacovich S, Blanco A, Sandoval R, et al. Catalytic autoantibodies against myelin basic protein (MBP) isolated from serum of autistic children impair in vitro models of synaptic plasticity in rat hippocampus. J Neuroimmunol. (2015) 287:1–8. doi: 10.1016/j.jneuroim.2015.07.006
63. Morvan MG, Lanier LL. NK cells and cancer: you can teach innate cells new tricks. Nat Rev Cancer. (2016) 16:7–19. doi: 10.1038/nrc.2015.5
64. Miller BJ, Gassama B, Sebastian D, Buckley P, Mellor A. Meta-analysis of lymphocytes in schizophrenia: clinical status and antipsychotic effects. Biol Psychiatry. (2013) 73:993–9. doi: 10.1016/j.biopsych.2012.09.007
65. Karpiński P, Samochowiec J, Frydecka D, Sa̧siadek MM, Misiak B. Further evidence for depletion of peripheral blood natural killer cells in patients with schizophrenia: a computational deconvolution study. Schizophr Res. (2018) 201:243–8. doi: 10.1016/j.schres.2018.04.026
66. Tarantino N, Leboyer M, Bouleau A, Hamdani N, Richard JR, Boukouaci W, et al. Natural killer cells in first-episode psychosis: an innate immune signature? Mol Psychiatry. (2021) 26:5297–306. doi: 10.1038/s41380-020-01008-7
67. Banchereau J, Briere F, Caux C, Davoust J, Lebecque S, Liu YJ, et al. Immunobiology of dendritic cells. Annu Rev Immunol. (2000) 18:767–811.
68. Fernandez-Egea E, Vértes PE, Flint SM, Turner L, Mustafa S, Hatton A, et al. Peripheral immune cell populations associated with cognitive deficits and negative symptoms of treatment-resistant schizophrenia. PLoS One. (2016) 11:e0155631. doi: 10.1371/journal.pone.0155631
69. Forget P, Khalifa C, Defour JP, Latinne D, Van Pel MC, De Kock M. What is the normal value of the neutrophil-to-lymphocyte ratio? BMC Res Notes. (2017) 10:12. doi: 10.1186/s13104-016-2335-5
70. Karageorgiou V, Milas GP, Michopoulos I. Neutrophil-to-lymphocyte ratio in schizophrenia: a systematic review and meta-analysis. Schizophr Res. (2019) 206:4–12. doi: 10.1016/j.schres.2018.12.017
71. Mazza MG, Lucchi S, Rossetti A, Clerici M. Neutrophil-lymphocyte ratio, monocyte-lymphocyte ratio and platelet-lymphocyte ratio in non-affective psychosis: a meta-analysis and systematic review. World J Biol Psychiatry. (2020) 21:326–38. doi: 10.1080/15622975.2019.1583371
72. Jackson AJ, Miller BJ. Meta-analysis of total and differential white blood cell counts in schizophrenia. Acta Psychiatr Scand. (2020) 142:18–26. doi: 10.1111/acps.13140
73. Sirota P, Gavrieli R, Wolach B. Overproduction of neutrophil radical oxygen species correlates with negative symptoms in schizophrenic patients: parallel studies on neutrophil chemotaxis, superoxide production and bactericidal activity. Psychiatry Res. (2003) 121:123–32. doi: 10.1016/s0165-1781(03)00222-1
74. Melamed Y, Sirota P, Dicker DR, Fishman P. Superoxide anion production by neutrophils derived from peripheral blood of schizophrenic patients. Psychiatry Res. (1998) 77:29–34. doi: 10.1016/s0165-1781(97)00124-8
75. Kaminska T, Szuster-Ciesielska A, Wysocka A, Marmurowska-Michalowska H, Dubas-Slemp H, Kandefer-Szerszen M. Serum cytokine level and production of reactive oxygen species (ROS) by blood neutrophils from a schizophrenic patient with hypersensitivity to neuroleptics. Med Sci Monit. (2003) 9:CS71–5.
76. Fang SH, Suzuki K, Lim CL, Chung MS, Ku PW, Chen LJ. Associations between sleep quality and inflammatory markers in patients with schizophrenia. Psychiatry Res. (2016) 246:154–60. doi: 10.1016/j.psychres.2016.09.032
77. Lacy P, Rosenberg HF, Walsh GM. Eosinophil overview: structure, biological properties, and key functions. Eosinophils. (2014) 1178:1–12. doi: 10.1007/978-1-4939-1016-8_1
78. Steiner J, Frodl T, Schiltz K, Dobrowolny H, Jacobs R, Fernandes BS, et al. Innate immune cells and C-reactive protein in acute first-episode psychosis and schizophrenia: relationship to psychopathology and treatment. Schizophr Bull. (2020) 46:363–73. doi: 10.1093/schbul/sbz068
79. Hällgren R, Venge P, Wistedt B. Elevated serum levels of lactoferrin and eosinophil cationic protein in schizophrenic patients. Br J Psychiatry. (1982) 140:55–60. doi: 10.1192/bjp.140.1.55
80. Teixeira AL, Reis HJ, Nicolato R, Brito-Melo G, Correa H, Teixeira MM, et al. Increased serum levels of CCL11/eotaxin in schizophrenia. Prog Neuro Psychopharmacol Biol Psychiatry. (2008) 32:710–4. doi: 10.1016/j.pnpbp.2007.11.019
81. Fernandez-Egea E, Scoriels L, Theegala S, Giro M, Ozanne SE, Burling K, et al. Cannabis use is associated with increased CCL11 plasma levels in young healthy volunteers. Prog Neuro Psychopharmacol Biol Psychiatry. (2013) 46:25–8. doi: 10.1016/j.pnpbp.2013.06.011
82. Hawkins WW, Evans MK. White blood cells and lymphoid tissue in vitamin B6 insufficiency. Am J Physiol. (1952) 170:160–7. doi: 10.1152/ajplegacy.1952.170.1.160
83. Ginhoux F, Jung S. Monocytes and macrophages: developmental pathways and tissue homeostasis. Nat Rev Immunol. (2014) 14:392–404. doi: 10.1038/nri3671
84. Jakubzick C, Gautier EL, Gibbings SL, Sojka DK, Schlitzer A, Johnson TE, et al. Minimal differentiation of classical monocytes as they survey steady-state tissues and transport antigen to lymph nodes. Immunity. (2013) 39:599–610. doi: 10.1016/j.immuni.2013.08.007
85. Kowalski J, Blada P, Kucia K, Madej A, Herman ZS. Neuroleptics normalize increased release of interleukin-1β and tumor necrosis factor-α from monocytes in schizophrenia. Schizophr Res. (2001) 50:169–75. doi: 10.1016/s0920-9964(00)00156-0
86. Beumer W, Gibney SM, Drexhage RC, Pont-Lezica L, Doorduin J, Klein HC, et al. The immune theory of psychiatric diseases: a key role for activated microglia and circulating monocytes. J Leukoc Biol. (2012) 92:959–75. doi: 10.1189/jlb.0212100
87. Nikkilä HV, Müller K, Ahokas A, Miettinen K, Rimón R, Andersson LC. Accumulation of macrophages in the CSF of schizophrenic patients during acute psychotic episodes. Am J Psychiatry. (1999) 156:1725–9. doi: 10.1176/ajp.156.11.1725
88. Cai HQ, Catts VS, Webster MJ, Galletly C, Liu D, O’Donnell M, et al. Increased macrophages and changed brain endothelial cell gene expression in the frontal cortex of people with schizophrenia displaying inflammation. Mol Psychiatry. (2020) 25:761–75. doi: 10.1038/s41380-018-0235-x
89. Purves-Tyson TD, Robinson K, Brown AM, Boerrigter D, Cai HQ, Weissleder C, et al. Increased macrophages and C1qA, C3, C4 transcripts in the midbrain of people with schizophrenia. Front Immunol. (2020) 11:2002. doi: 10.3389/fimmu.2020.02002
90. Weissleder C, North HF, Bitar M, Fullerton JM, Sager R, Barry G, et al. Reduced adult neurogenesis is associated with increased macrophages in the subependymal zone in schizophrenia. Mol Psychiatry. (2021) 26:6880–95. doi: 10.1038/s41380-021-01149-3
91. Bayer TA, Buslei R, Havas L, Falkai P. Evidence for activation of microglia in patients with psychiatric illnesses. Neurosci Lett. (1999) 271:126–8. doi: 10.1016/s0304-3940(99)00545-5
92. Falke E, Han LY, Arnold SE. Absence of neurodegeneration in the thalamus and caudate of elderly patients with schizophrenia. Psychiatry Res. (2000) 93:103–10. doi: 10.1016/s0165-1781(00)00104-9
93. Özdin S, Böke Ö. Neutrophil/lymphocyte, platelet/lymphocyte and monocyte/lymphocyte ratios in different stages of schizophrenia. Psychiatry Res. (2019) 271:131–5. doi: 10.1016/j.psychres.2018.11.043
94. Kronfol Z, Turner R, Nasrallah H, Winokur G. Leukocyte regulation in depression and schizophrenia. Psychiatry Res. (1984) 13:13–8. doi: 10.1016/0165-1781(84)90114-8
95. Ezeoke A, Mellor A, Buckley P, Miller B. A systematic, quantitative review of blood autoantibodies in schizophrenia. Schizophr Res. (2013) 150:245–51. doi: 10.1016/j.schres.2013.07.029
96. van Mierlo HC, Broen JC, Kahn RS, de Witte LD. B-cells and schizophrenia: a promising link or a finding lost in translation? Brain Behav Immun. (2019) 81:52–62. doi: 10.1016/j.bbi.2019.06.043
97. Busse S, Busse M, Schiltz K, Bielau H, Gos T, Brisch R, et al. Different distribution patterns of lymphocytes and microglia in the hippocampus of patients with residual versus paranoid schizophrenia: further evidence for disease course-related immune alterations? Brain Behav Immun. (2012) 26:1273–9. doi: 10.1016/j.bbi.2012.08.005
98. Debnath M. Adaptive immunity in schizophrenia: functional implications of T cells in the etiology, course and treatment. J Neuroimmune Pharmacol. (2015) 10:610–9. doi: 10.1007/s11481-015-9626-9
99. Nikkila H, Müller K, Ahokas A, Miettinen K, Andersson LC, Rimón R. Abnormal distributions of T-lymphocyte subsets in the cerebrospinal fluid of patients with acute schizophrenia. Schizophr Res. (1995) 14:215–21. doi: 10.1016/0920-9964(94)00039-b
100. Müller N, Schlesinger BC, Hadjamu M, Riedel M, Schwarz M, Ackenheil M, et al. Increased frequency of CD8 positive gamma/delta T-lymphocytes (CD8+ γ/δ+) in unmedicated schizophrenic patients: relation to impairment of the blood-brain barrier and HLA-DPA* 02011. Schizophr Res. (1998) 32:69–71. doi: 10.1016/s0920-9964(98)00036-x
101. Mazzarello V, Cecchini A, Fenu G, Rassu M, Dessy LA, Lorettu L, et al. Lymphocytes in schizophrenic patients under therapy: serological, morphological and cell subset findings. Ital J Anat Embryol. (2004) 109:177–88.
102. Matloubi H, Vodjgani M, Nasehi AA, Niknam MH, Kazemnejad A, Salehi E, et al. Decreased T cell response to mitogen and increased anti-cytoplasmic antibody in drug-free schizophrenic patients. Iran J Immunol. (2007) 4:32–7.
103. Craddock RM, Lockstone HE, Rider DA, Wayland MT, Harris LJ, McKenna PJ, et al. Altered T-cell function in schizophrenia: a cellular model to investigate molecular disease mechanisms. PLoS One. (2007) 2:e692. doi: 10.1371/journal.pone.0000692
104. Ding M, Song X, Zhao J, Gao J, Li X, Yang G, et al. Activation of Th17 cells in drug naïve, first episode schizophrenia. Prog Neuro Psychopharmacol Biol Psychiatry. (2014) 51:78–82. doi: 10.1016/j.pnpbp.2014.01.001
105. Benros ME, Nielsen PR, Nordentoft M, Eaton WW, Dalton SO, Mortensen PB. Autoimmune diseases and severe infections as risk factors for schizophrenia: a 30-year population-based register study. Am J Psychiatry. (2011) 168:1303–10. doi: 10.1176/appi.ajp.2011.11030516
106. Davies C, Segre G, Estradé A, Radua J, De Micheli A, Provenzani U, et al. Prenatal and perinatal risk and protective factors for psychosis: a systematic review and meta-analysis. Lancet Psychiatry. (2020) 7:399–410. doi: 10.1016/S2215-0366(20)30057-2
107. Jiang HY, Zhang X, Pan LY, Ma YC. Childhood infection and subsequent risk of psychotic disorders in adults: a systematic review and meta-analysis. Asian J Psychiatry. (2020) 54:102275. doi: 10.1016/j.ajp.2020.102275
108. Torrey EF, Bartko JJ, Lun ZR, Yolken RH. Antibodies to Toxoplasma gondii in patients with schizophrenia: a meta-analysis. Schizophr Bull. (2007) 33:729–36. doi: 10.1093/schbul/sbl050
109. Monroe JM, Buckley PF, Miller BJ. Meta-analysis of anti-Toxoplasma gondii IgM antibodies in acute psychosis. Schizophr Bull. (2015) 41:989–98. doi: 10.1093/schbul/sbu159
110. Sutterland AL, Fond G, Kuin A, Koeter MWJ, Lutter R, Van Gool T, et al. Beyond the association. Toxoplasma gondii in schizophrenia, bipolar disorder, and addiction: systematic review and meta-analysis. Acta Psychiatr Scand. (2015) 132:161–79. doi: 10.1111/acps.12423
111. Arias I, Sorlozano A, Villegas E, de Dios Luna J, McKenney K, Cervilla J, et al. Infectious agents associated with schizophrenia: a meta-analysis. Schizophr Res. (2012) 136:128–36. doi: 10.1016/j.schres.2011.10.026
112. Dickerson F, Kirkpatrick B, Boronow J, Stallings C, Origoni A, Yolken R. Deficit schizophrenia: association with serum antibodies to cytomegalovirus. Schizophr Bull. (2006) 32:396–400. doi: 10.1093/schbul/sbi054
113. Al-Diwani AA, Pollak TA, Irani SR, Lennox BR. Psychosis: an autoimmune disease? Immunology. (2017) 152:388–401. doi: 10.1111/imm.12795
114. Benros ME, Eaton WW, Mortensen PB. The epidemiologic evidence linking autoimmune diseases and psychosis. Biol Psychiatry. (2014) 75:300–6. doi: 10.1016/j.biopsych.2013.09.023
115. Benros ME, Pedersen MG, Rasmussen H, Eaton WW, Nordentoft M, Mortensen PB. A nationwide study on the risk of autoimmune diseases in individuals with a personal or a family history of schizophrenia and related psychosis. Am J Psychiatry. (2014) 171:218–26. doi: 10.1176/appi.ajp.2013.13010086
116. Ermakov EA, Dmitrieva EM, Parshukova DA, Kazantseva DV, Vasilieva AR, Smirnova LP. Oxidative stress-related mechanisms in schizophrenia pathogenesis and new treatment perspectives. Oxid Med Cell Longev. (2021) 2021:8881770. doi: 10.1155/2021/8881770
117. Deakin J, Lennox BR, Zandi MS. Antibodies to the N-methyl-D-aspartate receptor and other synaptic proteins in psychosis. Biol Psychiatry. (2014) 75:284–91. doi: 10.1016/j.biopsych.2013.07.018
118. Pathmanandavel K, Starling J, Merheb V, Ramanathan S, Sinmaz N, Dale RC, et al. Antibodies to surface dopamine-2 receptor and N-methyl-D-aspartate receptor in the first episode of acute psychosis in children. Biol Psychiatry. (2015) 77:537–47. doi: 10.1016/j.biopsych.2014.07.014
119. Borda T, Gomez R, Berría M I, Sterin-Borda L. Antibodies against astrocyte M1 and M2 muscarinic cholinoceptor from schizophrenic patients’ sera. Glia. (2004) 45:144–54. doi: 10.1002/glia.10312
120. Pollak TA, McCormack R, Peakman M, Nicholson TR, David AS. Prevalence of anti-N-methyl-d-aspartate (n.d.) receptor antibodies in patients with schizophrenia and related psychoses: a systematic review and meta-analysis. Psychol Med. (2014) 44:2475–87. doi: 10.1017/s003329171300295x
121. Pearlman DM, Najjar S. Meta-analysis of the association between N-methyl-d-aspartate receptor antibodies and schizophrenia, schizoaffective disorder, bipolar disorder, and major depressive disorder. Schizophr Res. (2014) 157:249–58. doi: 10.1016/j.schres.2014.05.001
122. Carvalho AF, Solmi M, Sanches M, Machado MO, Stubbs B, Ajnakina O, et al. Evidence-based umbrella review of 162 peripheral biomarkers for major mental disorders. Transl Psychiatry. (2020) 10:152. doi: 10.1038/s41398-020-0835-5
123. de Witte LD, Hoffmann C, van Mierlo HC, Titulaer MJ, Kahn RS, Martinez-Martinez P. Absence of N-methyl-D-aspartate receptor IgG autoantibodies in schizophrenia: the importance of cross-validation studies. JAMA Psychiatry. (2015) 72:731–3. doi: 10.1001/jamapsychiatry.2015.0526
124. Dahm L, Ott C, Steiner J, Stepniak B, Teegen B, Saschenbrecker S, et al. Seroprevalence of autoantibodies against brain antigens in health and disease. Ann Neurol. (2014) 76:82–94. doi: 10.1002/ana.24189
125. Cullen AE, Palmer-Cooper EC, Hardwick M, Vaggers S, Crowley H, Pollak TA, et al. Influence of methodological and patient factors on serum NMDAR IgG antibody detection in psychotic disorders: a meta-analysis of cross-sectional and case-control studies. Lancet Psychiatry. (2021) 8:109–20. doi: 10.1016/S2215-0366(20)30432-6
126. Pollak TA, Lennox BR, Müller S, Benros ME, Prüss H, van Elst LT, et al. Autoimmune psychosis: an international consensus on an approach to the diagnosis and management of psychosis of suspected autoimmune origin. Lancet Psychiatry. (2020) 7:93–108. doi: 10.1016/S2215-0366(19)30290-1
127. Wenke NK, Kreye J, Andrzejak E, van Casteren A, Leubner J, Murgueitio MS, et al. N-methyl-D-aspartate receptor dysfunction by unmutated human antibodies against the NR1 subunit. Ann Neurol. (2019) 85:771–6. doi: 10.1002/ana.25460
128. Grain R, Lally J, Stubbs B, Malik S, LeMince A, Nicholson TR, et al. Autoantibodies against voltage-gated potassium channel and glutamic acid decarboxylase in psychosis: a systematic review, meta-analysis, and case series. Psychiatry Clin Neurosci. (2017) 71:678–89. doi: 10.1111/pcn.12543
129. Whelan R, St Clair D, Mustard CJ, Hallford P, Wei J. Study of novel autoantibodies in schizophrenia. Schizophr Bull. (2018) 44:1341–9. doi: 10.1093/schbul/sbx175
130. Orlovska-Waast S, Köhler-Forsberg O, Brix SW, Nordentoft M, Kondziella D, Krogh J, et al. Cerebrospinal fluid markers of inflammation and infections in schizophrenia and affective disorders: a systematic review and meta-analysis. Mol Psychiatry. (2019) 24:869–87. doi: 10.1038/s41380-018-0220-4
131. Lachance LR, McKenzie K. Biomarkers of gluten sensitivity in patients with non-affective psychosis: a meta-analysis. Schizophr Res. (2014) 152:521–7. doi: 10.1016/j.schres.2013.12.001
132. Samaroo D, Dickerson F, Kasarda DD, Green PH, Briani C, Yolken RH, et al. Novel immune response to gluten in individuals with schizophrenia. Schizophr Res. (2010) 118:248–55. doi: 10.1016/j.schres.2009.08.009
133. Hirata-Hibi MOTOE, Fessel WJ. The bone marrow in schizophrenia. Arch Gen Psychiatry. (1964) 10:414–9. doi: 10.1001/archpsyc.1964.01720220092014
134. Sommer IE, Van Bekkum DW, Klein H, Yolken R, De Witte L, Talamo G. Severe chronic psychosis after allogeneic SCT from a schizophrenic sibling. Bone Marrow Transplant. (2015) 50:153–4. doi: 10.1038/bmt.2014.221
135. Miyaoka T, Wake R, Hashioka S, Hayashida M, Oh-Nishi A, Azis IA, et al. Remission of psychosis in treatment-resistant schizophrenia following bone marrow transplantation: a case report. Front Psychiatry. (2017) 8:174. doi: 10.3389/fpsyt.2017.00174
136. Bachmeyer C, Bourguiba R, Gkalea V, Papageorgiou L. Vegan diet as a neglected cause of severe megaloblastic anemia and psychosis. Am J Med. (2019) 132:e850–1. doi: 10.1016/j.amjmed.2019.06.025
137. Stip E, Langlois R, Thuot C, Mancini-Marïe A. Fatal agranulocytosis: the use of olanzapine in a patient with schizophrenia and myelodysplasia. Prog Neuro Psychopharmacol Biol Psychiatry. (2007) 31:297–300. doi: 10.1016/j.pnpbp.2006.08.005
138. Rudolf J, Grond M, Neveling M, Heiss WD. Clozapine-induced agranulocytosis and thrombopenia in a patient with dopaminergic psychosis. J Neural Transm. (1997) 104:1305–11. doi: 10.1007/BF01294731
139. Patel R, Lima A, Burke C, Hoffman M. Monocytopenia in clozapine-induced agranulocytosis: insights into pathophysiology and treatment. BMJ Case Rep. (2019) 12:bcr–2018–226016. doi: 10.1136/bcr-2018-226016
140. Kinney DK, Hintz K, Shearer EM, Barch DH, Riffin C, Whitley K, et al. A unifying hypothesis of schizophrenia: abnormal immune system development may help explain roles of prenatal hazards, post-pubertal onset, stress, genes, climate, infections, and brain dysfunction. Med Hypotheses. (2010) 74:555–63. doi: 10.1016/j.mehy.2009.09.040
141. Anders S, Kinney DK. Abnormal immune system development and function in schizophrenia helps reconcile diverse findings and suggests new treatment and prevention strategies. Brain Res. (2015) 1617:93–112. doi: 10.1016/j.brainres.2015.02.043
142. Ryan AK, Goodship JA, Wilson DI, Philip N, Levy A, Seidel H, et al. Spectrum of clinical features associated with interstitial chromosome 22q11 deletions: a European collaborative study. J Med Genet. (1997) 34:798–804. doi: 10.1136/jmg.34.10.798
143. Lima K, Abrahamsen TG, Foelling I, Natvig S, Ryder LP, Olaussen RW. Low thymic output in the 22q11.2 deletion syndrome measured by CCR9+ CD45RA+ T cell counts and T cell receptor rearrangement excision circles. Clin Exp Immunol. (2010) 161:98–107. doi: 10.1111/j.1365-2249.2010.04152.x
144. Karayiorgou M, Simon TJ, Gogos JA. 22q11.2 microdeletions: linking DNA structural variation to brain dysfunction and schizophrenia. Nat Rev Neurosci. (2010) 11:402–16. doi: 10.1038/nrn2841
145. Vergaelen E, Schiweck C, Van Steeland K, Counotte J, Veling W, Swillen A, et al. A pilot study on immuno-psychiatry in the 22q11.2 deletion syndrome: a role for Th17 cells in psychosis? Brain Behav Immun. (2018) 70:88–95. doi: 10.1016/j.bbi.2018.03.022
146. Da Mesquita S, Louveau A, Vaccari A, Smirnov I, Cornelison RC, Kingsmore KM, et al. Functional aspects of meningeal lymphatics in ageing and Alzheimer’s disease. Nature. (2018) 560:185–91. doi: 10.1038/s41586-018-0368-8
147. Averkin VS, Bonartsev PD, IuI S. Ultrastructure of schizophrenic patients’ lymph nodes. Zh Nevropato Psikhiatr Im S S Korsakova. (1976) 76:1056–61.
148. Zhang J, Chang L, Pu Y, Hashimoto K. Abnormal expression of colony-stimulating factor 1 receptor (CSF1R) and transcription factor PU. 1 (SPI1) in the spleen from patients with major psychiatric disorders: a role of brain–spleen axis. J Affect Disord. (2020) 272:110–5. doi: 10.1016/j.jad.2020.03.128
149. Krøll J. Schizophrenia and liver dysfunction. Med Hypotheses. (2001) 56:634–6. doi: 10.1054/mehy.2000.1254
150. Fuller BE, Rodriguez VL, Linke A, Sikirica M, Dirani R, Hauser P. Prevalence of liver disease in veterans with bipolar disorder or schizophrenia. Gen Hosp Psychiatry. (2011) 33:232–7. doi: 10.1016/j.genhosppsych.2011.03.006
151. Hsu JH, Chien IC, Lin CH, Chou YJ, Chou P. Increased risk of chronic liver disease in patients with schizophrenia: a population-based cohort study. Psychosomatics. (2014) 55:163–71. doi: 10.1016/j.psym.2013.06.001
152. Lluch E, Miller BJ. Rates of hepatitis B and C in patients with schizophrenia: a meta-analysis. Gen Hosp Psychiatry. (2019) 61:41–6. doi: 10.1016/j.genhosppsych.2019.10.007
153. Xu D, Chen G, Kong L, Zhang W, Hu L, Chen C, et al. Lower risk of liver cancer in patients with schizophrenia: a systematic review and meta-analysis of cohort studies. Oncotarget. (2017) 8:102328. doi: 10.18632/oncotarget.21679
154. Yang B, Ren Q, Zhang JC, Chen QX, Hashimoto K. Altered expression of BDNF, BDNF pro-peptide and their precursor proBDNF in brain and liver tissues from psychiatric disorders: rethinking the brain–liver axis. Transl Psychiatry. (2017) 7:e1128–1128. doi: 10.1038/tp.2017.95
155. Huang JT, Wang L, Prabakaran S, Wengenroth M, Lockstone HE, Koethe D, et al. Independent protein-profiling studies show a decrease in apolipoprotein A1 levels in schizophrenia CSF, brain, and peripheral tissues. Mol Psychiatry. (2008) 13:1118–28. doi: 10.1038/sj.mp.4002108
156. Prabakaran S, Wengenroth M, Lockstone HE, Lilley K, Leweke FM, Bahn S. 2-D DIGE analysis of liver and red blood cells provides further evidence for oxidative stress in schizophrenia. J Proteome Res. (2007) 6:141–9. doi: 10.1021/pr060308a
157. Choi KH, Higgs BW, Weis S, Song J, Llenos IC, Dulay JR, et al. Effects of typical and atypical antipsychotic drugs on gene expression profiles in the liver of schizophrenia subjects. BMC Psychiatry. (2009) 9:57. doi: 10.1186/1471-244X-9-57
158. Konturek PC, Harsch IA, Konturek K, Schink M, Konturek T, Neurath MF, et al. Gut–liver axis: how do gut bacteria influence the liver? Med Sci. (2018) 6:79. doi: 10.3390/medsci6030079
159. Yang X, Di Lu JZ, Lin Z, Yang M, Xu X. The gut-liver axis in immune remodeling: new insight into liver diseases. Int J Biol Sci. (2020) 16:2357. doi: 10.7150/ijbs.46405
160. Yi W, Ji Y, Gao H, Pan R, Wei Q, Cheng J, et al. Does the gut microbiome partially mediate the impact of air pollutants exposure on liver function? Evidence based on schizophrenia patients. Environ Pollut. (2021) 291:118135. doi: 10.1016/j.envpol.2021.118135
161. Yuan X, Kang Y, Zhuo C, Huang XF, Song X. The gut microbiota promotes the pathogenesis of schizophrenia via multiple pathways. Biochem Biophys Res Commun. (2019) 512:373–80. doi: 10.1016/j.bbrc.2019.02.152
162. Usta A, Kılıç F, Demirdaş A, Işık Ü, Doğuç DK, Bozkurt M. Serum zonulin and claudin-5 levels in patients with schizophrenia. Eur Arch Psychiatry Clin Neurosci. (2021) 271:767–73. doi: 10.1007/s00406-020-01152-9
163. Barber GS, Sturgeon C, Fasano A, Cascella NG, Eaton WW, McMahon RP, et al. Elevated zonulin, a measure of tight-junction permeability, may be implicated in schizophrenia. Schizophr Res. (2019) 211:111–2. doi: 10.1016/j.schres.2019.07.006
164. Wan C, La Y, Zhu H, Yang Y, Jiang L, Chen Y, et al. Abnormal changes of plasma acute phase proteins in schizophrenia and the relation between schizophrenia and haptoglobin (Hp) gene. Amino Acids. (2007) 32:101–8. doi: 10.1007/s00726-005-0292-8
165. Furusawa Y, Obata Y, Fukuda S, Endo TA, Nakato G, Takahashi D, et al. Commensal microbe-derived butyrate induces the differentiation of colonic regulatory T cells. Nature. (2013) 504:446–50. doi: 10.1038/nature12721
166. Wekerle H. Brain autoimmunity and intestinal microbiota: 100 trillion game changers. Trends Immunol. (2017) 38:483–97. doi: 10.1016/j.it.2017.03.008
167. Nguyen TT, Kosciolek T, Eyler LT, Knight R, Jeste DV. Overview and systematic review of studies of microbiome in schizophrenia and bipolar disorder. J Psychiatr Res. (2018) 99:50–61. doi: 10.1016/j.jpsychires.2018.01.013
168. Schwarz E, Maukonen J, Hyytiäinen T, Kieseppä T, Orešiè M, Sabunciyan S, et al. Analysis of microbiota in first episode psychosis identifies preliminary associations with symptom severity and treatment response. Schizophr Res. (2018) 192:398–403. doi: 10.1016/j.schres.2017.04.017
169. Yolken RH, Severance EG, Sabunciyan S, Gressitt KL, Chen O, Stallings C, et al. Metagenomic sequencing indicates that the oropharyngeal phageome of individuals with schizophrenia differs from that of controls. Schizophr Bull. (2015) 41:1153–61. doi: 10.1093/schbul/sbu197
170. Zhang X, Pan LY, Zhang Z, Zhou YY, Jiang HY, Ruan B. Analysis of gut mycobiota in first-episode, drug-naïve Chinese patients with schizophrenia: a pilot study. Behav Brain Res. (2020) 379:112374. doi: 10.1016/j.bbr.2019.112374
171. Thirion F, Speyer H, Hansen TH, Nielsen T, Fan Y, Le Chatelier E, et al. Alteration of gut microbiome in patients with schizophrenia indicates links between bacterial tyrosine biosynthesis and cognitive dysfunction. Biol Psychiatry Glob Open Sci. (2022). doi: 10.1016/j.bpsgos.2022.01.009 [Epub ahead of print].
172. Klein-Petersen AW, Köhler-Forsberg O, Benros ME. Infections, antibiotic treatment, and the Microbiome in relation to schizophrenia. Schizophr Res. (2021) 234:71–7. doi: 10.1016/j.schres.2019.11.033
173. Seeman MV. The gut microbiome and antipsychotic treatment response. Behav Brain Res. (2021) 396:112886. doi: 10.1016/j.bbr.2020.112886
174. Zheng P, Zeng B, Liu M, Chen J, Pan J, Han Y, et al. The gut microbiome from patients with schizophrenia modulates the glutamate-glutamine-GABA cycle and schizophrenia-relevant behaviors in mice. Sci Adv. (2019) 5:eaau8317.
175. Nemani K, Ghomi RH, McCormick B, Fan X. Schizophrenia and the gut–brain axis. Prog Neuro Psychopharmacol Biol Psychiatry. (2015) 56:155–60.
176. Rogers GB, Keating DJ, Young RL, Wong ML, Licinio J, Wesselingh S. From gut dysbiosis to altered brain function and mental illness: mechanisms and pathways. Mol Psychiatry. (2016) 21:738–48. doi: 10.1038/mp.2016.50
177. Kelly JR, Minuto C, Cryan JF, Clarke G, Dinan TG. The role of the gut microbiome in the development of schizophrenia. Schizophr Res. (2021) 234:4–23. doi: 10.1016/j.schres.2020.02.010
178. Millett CE, Burdick KE, Kubicki MR. The effects of peripheral inflammation on the brain—a neuroimaging perspective. Harv Rev Psychiatry. (2022) 30:54–8. doi: 10.1097/HRP.0000000000000323
179. Bechter K. Updating the mild encephalitis hypothesis of schizophrenia. Prog Neuro Psychopharmacol Biol Psychiatry. (2013) 42:71–91. doi: 10.1016/j.pnpbp.2012.06.019
180. Fillman SG, Cloonan N, Catts VS, Miller LC, Wong J, McCrossin T, et al. Increased inflammatory markers identified in the dorsolateral prefrontal cortex of individuals with schizophrenia. Mol Psychiatry. (2013) 18:206–14. doi: 10.1038/mp.2012.110
181. Trépanier MO, Hopperton KE, Mizrahi R, Mechawar N, Bazinet RP. Postmortem evidence of cerebral inflammation in schizophrenia: a systematic review. Mol Psychiatry. (2016) 21:1009–26. doi: 10.1038/mp.2016.90
182. Van Kesteren CFMG, Gremmels H, De Witte LD, Hol EM, Van Gool AR, Falkai PG, et al. Immune involvement in the pathogenesis of schizophrenia: a meta-analysis on postmortem brain studies. Transl Psychiatry. (2017) 7:e1075–1075. doi: 10.1038/tp.2017.4
183. Volk DW, Moroco AE, Roman KM, Edelson JR, Lewis DA. The role of the nuclear factor-κB transcriptional complex in cortical immune activation in schizophrenia. Biol Psychiatry. (2019) 85:25–34. doi: 10.1016/j.biopsych.2018.06.015
184. Murphy CE, Walker AK, O’Donnell M, Galletly C, Lloyd AR, Liu D, et al. Peripheral NF-κB dysregulation in people with schizophrenia drives inflammation: putative anti-inflammatory functions of NF-κB kinases. Transl Psychiatry. (2022) 12:21. doi: 10.1038/s41398-021-01764-2
185. Laskaris LE, Di Biase MA, Everall I, Chana G, Christopoulos A, Skafidas E, et al. Microglial activation and progressive brain changes in schizophrenia. Br J Pharmacol. (2016) 173:666–80. doi: 10.1111/bph.13364
186. Marques TR, Ashok AH, Pillinger T, Veronese M, Turkheimer FE, Dazzan P, et al. Neuroinflammation in schizophrenia: meta-analysis of in vivo microglial imaging studies. Psychol Med. (2019) 49:2186–96. doi: 10.1017/S0033291718003057
187. Notter T, Coughlin JM, Gschwind T, Weber-Stadlbauer U, Wang Y, Kassiou M, et al. Translational evaluation of translocator protein as a marker of neuroinflammation in schizophrenia. Mol Psychiatry. (2018) 23:323–34. doi: 10.1038/mp.2016.248
188. Radewicz K, Garey LJ, Gentleman SM, Reynolds R. Increase in HLA-DR immunoreactive microglia in frontal and temporal cortex of chronic schizophrenics. J Neuropathol Exp Neurol. (2000) 59:137–50. doi: 10.1093/jnen/59.2.137
189. Steiner J, Bielau H, Brisch R, Danos P, Ullrich O, Mawrin C, et al. Immunological aspects in the neurobiology of suicide: elevated microglial density in schizophrenia and depression is associated with suicide. J Psychiatr Res. (2008) 42:151–7. doi: 10.1016/j.jpsychires.2006.10.013
190. Steiner J, Mawrin C, Ziegeler A, Bielau H, Ullrich O, Bernstein HG, et al. Distribution of HLA-DR-positive microglia in schizophrenia reflects impaired cerebral lateralization. Acta Neuropathol. (2006) 112:305–16. doi: 10.1007/s00401-006-0090-8
191. Schlaaff K, Dobrowolny H, Frodl T, Mawrin C, Gos T, Steiner J, et al. Increased densities of T and B lymphocytes indicate neuroinflammation in subgroups of schizophrenia and mood disorder patients. Brain Behav Immun. (2020) 88:497–506. doi: 10.1016/j.bbi.2020.04.021
192. Bogerts B, Winopal D, Schwarz S, Schlaaff K, Dobrowolny H, Mawrin C, et al. Evidence of neuroinflammation in subgroups of schizophrenia and mood disorder patients: a semiquantitative postmortem study of CD3 and CD20 immunoreactive lymphocytes in several brain regions. Neurol Psychiatry Brain Res. (2017) 23:2–9. doi: 10.1016/j.npbr.2016.11.001
193. Najjar S, Pahlajani S, De Sanctis V, Stern JN, Najjar A, Chong D. Neurovascular unit dysfunction and blood–brain barrier hyperpermeability contribute to schizophrenia neurobiology: a theoretical integration of clinical and experimental evidence. Front Psychiatry. (2017) 8:83. doi: 10.3389/fpsyt.2017.00083
194. Mondelli V, Ciufolini S, Belvederi Murri M, Bonaccorso S, Di Forti M, Giordano A, et al. Cortisol and inflammatory biomarkers predict poor treatment response in first episode psychosis. Schizophr Bull. (2015) 41:1162–70. doi: 10.1093/schbul/sbv028
195. Fillman SG, Sinclair D, Fung SJ, Webster MJ, Shannon Weickert C. Markers of inflammation and stress distinguish subsets of individuals with schizophrenia and bipolar disorder. Transl Psychiatry. (2014) 4:e365–365. doi: 10.1038/tp.2014.8
196. Boerrigter D, Weickert TW, Lenroot R, O’Donnell M, Galletly C, Liu D, et al. Using blood cytokine measures to define high inflammatory biotype of schizophrenia and schizoaffective disorder. J Neuroinflammation. (2017) 14:188. doi: 10.1186/s12974-017-0962-y
197. Lizano P, Lutz O, Xu Y, Rubin LH, Paskowitz L, Lee AM, et al. Multivariate relationships between peripheral inflammatory marker subtypes and cognitive and brain structural measures in psychosis. Mol Psychiatry. (2021) 26:3430–43. doi: 10.1038/s41380-020-00914-0
198. Hoang D, Xu Y, Lutz O, Bannai D, Zeng V, Bishop JR, et al. Inflammatory subtypes in antipsychotic-Naïve first-episode schizophrenia are associated with altered brain morphology and topological organization. Brain Behav Immun. (2022) 100:297–308. doi: 10.1016/j.bbi.2021.11.019
199. Najjar S, Pearlman DM. Neuroinflammation and white matter pathology in schizophrenia: systematic review. Schizophr Res. (2015) 161:102–12. doi: 10.1016/j.schres.2014.04.041
200. Jacomb I, Stanton C, Vasudevan R, Powell H, O’Donnell M, Lenroot R, et al. C-reactive protein: higher during acute psychotic episodes and related to cortical thickness in schizophrenia and healthy controls. Front Immunol. (2018) 9:2230. doi: 10.3389/fimmu.2018.02230
201. Fillman SG, Weickert TW, Lenroot RK, Catts SV, Bruggemann JM, Catts VS, et al. Elevated peripheral cytokines characterize a subgroup of people with schizophrenia displaying poor verbal fluency and reduced Broca’s area volume. Mol Psychiatry. (2016) 21:1090–8. doi: 10.1038/mp.2015.90
202. Dickerson F, Stallings C, Origoni A, Boronow J, Yolken R. C-reactive protein is associated with the severity of cognitive impairment but not of psychiatric symptoms in individuals with schizophrenia. Schizophr Res. (2007) 93:261–5. doi: 10.1016/j.schres.2007.03.022
203. Fond G, Garosi A, Faugere M, Campion JY, Lancon C, Boyer L, et al. Peripheral inflammation is associated with brain SPECT perfusion changes in schizophrenia. Eur J Nucl Med Mol Imaging. (2021) 49:905–12. doi: 10.1007/s00259-021-05529-3
204. Bishop JR, Zhang L, Lizano P. Inflammation subtypes and translating inflammation-related genetic findings in schizophrenia and related psychoses: a perspective on pathways for treatment stratification and novel therapies. Harv Rev Psychiatry. (2022) 30:59. doi: 10.1097/HRP.0000000000000321
205. Misiak B, Bartoli F, Carrà G, Stańczykiewicz B, Gładka A, Frydecka D, et al. Immune-inflammatory markers and psychosis risk: a systematic review and meta-analysis. Psychoneuroendocrinology. (2021) 127:105200. doi: 10.1016/j.psyneuen.2021.105200
206. Oomen PP, Begemann MJ, Brand BA, de Haan L, Veling W, Koops S, et al. Longitudinal clinical and functional outcome in distinct cognitive subgroups of first-episode psychosis: a cluster analysis. Psychol Med. (2021). [Online ahead of print]. doi: 10.1017/S0033291721004153
207. Martinuzzi E, Barbosa S, Daoudlarian D, Bel Haj Ali W, Gilet C, Fillatre L, et al. Stratification and prediction of remission in first-episode psychosis patients: the OPTiMiSE cohort study. Transl Psychiatry. (2019) 9:20.
208. Jeffries CD, Perkins DO, Fournier M, Do KQ, Cuenod M, Khadimallah I, et al. Networks of blood proteins in the neuroimmunology of schizophrenia. Transl Psychiatry. (2018) 8:112. doi: 10.1038/s41398-018-0158-y
209. Perkins DO, Jeffries CD, Addington J, Bearden CE, Cadenhead KS, Cannon TD, et al. Towards a psychosis risk blood diagnostic for persons experiencing high-risk symptoms: preliminary results from the NAPLS project. Schizophr Bull. (2015) 41:419–28. doi: 10.1093/schbul/sbu099
210. Enrico P, Delvecchio G, Turtulici N, Pigoni A, Villa FM, Perlini C, et al. Classification of psychoses based on immunological features: a machine learning study in a large cohort of first-episode and chronic patients. Schizophr Bull. (2021) 47:1141–55. doi: 10.1093/schbul/sbaa190
211. Maes M, Anderson G. False dogmas in schizophrenia research: toward the reification of pathway phenotypes and pathway classes. Front Psychiatry. (2021) 12:663985. doi: 10.3389/fpsyt.2021.663985
212. Ioannou M, Foiselle M, Mallet J, Stam EL, Godin O, Dubertret C, et al. Towards precision medicine: what are the stratification hypotheses to identify homogeneous inflammatory subgroups. Eur Neuropsychopharmacol. (2021) 45:108–21. doi: 10.1016/j.euroneuro.2020.11.001
213. Mothi SS, Sudarshan M, Tandon N, Tamminga C, Pearlson G, Sweeney J, et al. Machine learning improved classification of psychoses using clinical and biological stratification: update from the bipolar-schizophrenia network for intermediate phenotypes (B-SNIP). Schizophr Res. (2019) 214:60–9. doi: 10.1016/j.schres.2018.04.037
Keywords: schizophrenia, immune system, inflammation, cytokines, antibodies, T cell, B cell
Citation: Ermakov EA, Melamud MM, Buneva VN and Ivanova SA (2022) Immune System Abnormalities in Schizophrenia: An Integrative View and Translational Perspectives. Front. Psychiatry 13:880568. doi: 10.3389/fpsyt.2022.880568
Received: 21 February 2022; Accepted: 30 March 2022;
Published: 25 April 2022.
Edited by:
Milica Milovan Borovcanin, University of Kragujevac, SerbiaReviewed by:
Bernhard Bogerts, Otto von Guericke University Magdeburg, GermanyYasin Hasan Balcioglu, Bakirkoy Prof Mazhar Osman Training and Research Hospital for Psychiatry, Neurology, and Neurosurgery, Turkey
Copyright © 2022 Ermakov, Melamud, Buneva and Ivanova. This is an open-access article distributed under the terms of the Creative Commons Attribution License (CC BY). The use, distribution or reproduction in other forums is permitted, provided the original author(s) and the copyright owner(s) are credited and that the original publication in this journal is cited, in accordance with accepted academic practice. No use, distribution or reproduction is permitted which does not comply with these terms.
*Correspondence: Evgeny A. Ermakov, ZXZnZW55X2VybWFrb3ZAbWFpbC5ydQ==