- 1Department of Radiology, Renmin Hospital of Wuhan University, Wuhan, China
- 2Department of Radiology, Ren Ji Hospital, School of Medicine, Shanghai Jiao Tong University, Shanghai, China
- 3State Key Laboratory of Magnetic Resonance and Atomic and Molecular Physics, National Center for Magnetic Resonance in Wuhan, Wuhan Institute of Physics and Mathematics, Innovation Academy for Precision Measurement Science and Technology, Chinese Academy of Sciences, Wuhan, China
- 4University of Chinese Academy of Sciences, Beijing, China
Biological sex may play a large role in cigarette use and cessation outcomes and neuroimaging studies have demonstrated that cigarette smoking is associated with sex-related differences in brain structure and function. However, less is known about sex-specific alterations in spontaneous brain activity in cigarette smokers. In this study, we investigated the sex-related effects of cigarette smoking on local spontaneous brain activity using regional homogeneity (ReHo) based on resting-state fMRI. Fifty-six smokers (24 females) and sixty-three (25 females) healthy non-smoking controls were recruited. Whole-brain voxelwise 2-way analysis of covariance of ReHo was performed to detect brain regions with sex-dependent alterations on the spontaneous brain activity. Compared to non-smokers, smokers exhibited significant ReHo differences in several brain regions, including the right medial orbitofrontal cortex extended to the ventral striatum/amygdala/parahippocampus, left precuneus, and bilateral cerebellum crus. Smoking and sex interaction analysis revealed that male smokers showed significantly lower ReHo in the right ventral striatum, left cerebellum crus1, and left fusiform gyrus compared to male non-smokers, whereas there are no significant differences between female smokers and non-smokers. Furthermore, the ReHo within the left cerebellum crus1 was negatively correlated with craving scores in male smokers but not in female smokers. Such sex-dependent differences in spontaneous brain activity lays a foundation for further understanding the neural pathophysiology of sex-specific effects of nicotine addiction and promoting more effective health management of quitting smoking.
Introduction
Tobacco smoking, the leading cause of preventable death worldwide, is associated with many serious health problems including cardiovascular disease and lung cancer (1). Despite the growing number of evidence-based treatments for smoking addiction, the efficacy remains moderate, with high relapse rate (2). Biological sex may play a large role in patterns of nicotine use and cessation. outcomes For instance, higher smoking rates, greater reinforcement of nicotine and better nicotine replacement therapies has been reported among men than women (3). Women are inclined to smoke for stress relief and mood regulation, and difficult to maintain abstinence (4). Therefore, sex differences may be crucial to target the neural pathophysiology of nicotine addiction and develop more effective relapse prevention therapies (5).
Neuroimaging studies showed that cigarette smoking is associated with sex-specific alterations in brain structure and function. Compared to sex-matched non-smokers, male rather than female nicotine-dependent subjects had a larger volume in the left putamen and a smaller volume in the left caudate, whereas female but not male smokers showed a smaller volume in the right amygdala (6). Exposure to smoking cues, direct comparisons between male and female smokers revealed that male smokers showed greater reactivity in reward-related brain regions (7) and greater coupling between the interhemispheric regions within the executive control network (8) than female smokers. In contrast, female smokers had greater connectivity within the limbic and default mode network than male smokers (9, 10). Earlier work of our team analyzed middle-aged chronic heavy smokers and found that the regional homogeneity (ReHo) of the right paracentral lobule of male smokers was significantly different from that of female smokers (11). Another study of our team reported that young male but not young female smokers had decreased resting-state functional connectivity (rsFC) between the right amygdala and right orbitofrontal cortex (OFC) (12). RsFC of the amygdala-OFC circuity was negatively correlated with the craving score in male but not female smokers (12). Despite increasing knowledge of the sex-specific effects of tobacco use on brain structure and connectivity between spatially distinct brain regions, it remains unclear whether cigarette smoking has differential influence on the local spontaneous brain activity between men and women, particularly young adults.
Resting-state fMRI (rs-fMRI) provides a non-invasive method of assessing changes in resting-state brain activity and functional connectivity across the whole-brain (13). ReHo measures the synchronization of intra-regional spontaneous low-frequency BOLD signal fluctuations by calculating Kendall's coefficient of concordance (14), which have high test-retest reliability (15). The ReHo metric has been widely used to investigate abnormal local spontaneous brain activity in psychiatric and neurologic conditions (16). Recent literature review demonstrated differences in adolescent vulnerability to neurotoxicity of drugs of abuse from adult (17). Brain imaging studies showed humans might achieve full adulthood after the ages of 25 and 30 (18, 19). Hence, it is important to study the abnormal changes of brain function in smokers at younger age group. In view of the gender influence of smoking on neural structure and functional networks, we assume that young male and female smokers would show constant change patterns in the reward-related areas [as in our previous article (11)], and display different change patterns in local synchronization of the brain.
To test our hypothesis, we employed ReHo method to depict spontaneous brain activity and then performed a voxel-wise analysis to examine the intrinsic brain activity differences between smokers and non-smokers, and to further explore the sex interaction on smoking dependency. Such sex-dependent differences in spontaneous brain activity lays a foundation for further understanding the neural pathophysiology nicotine addiction and promoting more effective health management of quitting smoking.
Materials and Methods
Subjects
Sixty cigarette smokers (25 females) and 67 healthy nonsmoking controls (28 females) aged 18–29 years participated in this study. The Mini International Neuropsychiatric Interview was used to exclude participants who had substance use disorder other than nicotine dependence, current Axis 1 DSM V psychiatric diagnoses, or significant medical conditions. Exclusion criteria for both smokers and non-smokers were a history of head trauma or injury causing loss of consciousness lasting >3 min or associated with skull fracture or intracranial bleeding, or had irremovable magnetically active objects on or within their body. Smokers were defined as those who smoked at least 10 cigarettes/day on any given day during the last year. The severity of nicotine addiction was determined from the Fagerström Test for Nicotine Dependence (FTND) (20), and the measurement of “craving” for cigarettes was evaluated using a brief questionnaire of smoking urges (21). All smokers had no period of smoking abstinence longer than 3 months in the past year. It was about 1 h since last cigarette before smokers were scanned. Non-smokers had not smoked more than five cigarettes in their lifetimes. All participants were administered a set of questionnaires at the beginning of the study, namely, the Self-rating Anxiety Scale (SAS), Self-rating Depression Scale (SDS) and Barratt Impulsiveness Scale (BIS) version 11. The dataset of subjects were described in our previous article (12), but the analyses of this study are different from that in previous reports; here we focus on exploring sex-specific effects of cigarette smoking on spontaneous brain activity.
The current study adhered to the Declaration of Helsinki and was approved by the Research Ethics Committee of Renji Hospital, School of Medicine of Shanghai Jiaotong University. All participants were informed of the measurements and the experimental procedures of the study before their MRI examinations. Each participant provided written informed consent.
Data Acquisition
Images were obtained using a 3.0-T GE Signa HDx (Milwaukee, WI, USA) scanner with a standard 8-channel head coil. Restraining foam pads were used to reduce head motion, and earplugs were used to reduce scanner noise. Before MRI scanning, all subjects were instructed to relax with their eyes closed while refraining from falling asleep and without engaging in any directed, systematic thought. The physiological state of the smokers when they were in the scanner as spontaneous, rather than abstinence or satiety, that was, none of the smokers felt the acute urge to smoke or experience any withdrawal symptoms confirmed by a self-report completed by each participant immediately after the scan.
Rs-fMRI data were acquired using a gradient-echo echo-planar imaging sequence (TR = 2,000 ms, TE = 24 ms, flip angle = 90°, matrix = 64 × 64, FOV = 230 × 230 mm2, thickness = 4 mm with no gap, 34 slices). For each participant, the rs-fMRI scan lasted 7 min and 20 s and a total of 220 volumes were acquired. The axial T1-weighted and T2-weighted images were also performed to confirm the absence of structural lesions. All images were evaluated by two experienced neuroradiologists and no participants were excluded on this basis.
Image Preprocessing
Rs-fMRI data were preprocessed using the Data Processing Assistant for Resting-State fMRI (http://rfmri.org/DPARSF). After removing the first 10 volumes for each subject, the remaining 210 volumes were corrected for slice timing and realigned to the first volume for head-motion correction. The Friston 24-parameter model (i.e., six head motion parameters, six head motion parameters one time point before, and 12 corresponding squared items) was performed to regress out the head motion effects. The scrubbing strategy was further carried out to correct the head motion effects. Time points with framewise displacement larger than 0.2 mm were identified and removed along with 1 back and 2 forward neighbors (22). Subjects with <90 time points after scrubbing were not used because too few remaining time points may lead to unreliable results (23). As a result, four smokers and four non-smokers were discarded, and a total of 56 smokers (24 females) and 63 non-smokers (25 females) were ultimately used in the ReHo analysis. The detailed demographic and clinical data of the subjects used in this study are provided in Table 1. Smokers and non-smokers were not statistically different in terms of time points removed (28.08 ± 32.07 for smokers, 36.25 ± 32.66 for non-smokers, p = 0.17). Afterward, the functional images were normalized to the standard Montreal Neurological Institute (MNI) space and resampled to a 3-mm isotropic voxel. Then, linear and quadratic trends as well as white matter and corticospinal fluid signals were removed. Finally, temporal bandpass filtering (0.01–0.1 Hz) was performed to reduce the effects of high-frequency physiological noise.
ReHo Analysis
Based on the temporally bandpass-filtered data, the ReHo value for each voxel was defined as the KCC of the time series of a given voxel and those of its 26 nearest neighbors. For standardization purposes, each individual ReHo map was divided by its mean ReHo within a brain mask without non-brain tissue (14). Finally, the ReHo maps were smoothed by 6 mm full width at half maximum Gaussian kernel.
Statistical Analysis
A 2-way analysis of variance (ANOVA) was used to identify differences among groups in age, education level, SAS, SDS and BIS scores. A voxelwise 2-way analysis of covariance (ANCOVA) with age and education level as covariates was performed to evaluate the main effects of group (smokers vs. non-smokers) and sex (male vs. female) as well as the interaction between group and sex. A false discovery rate (FDR) corrected p-value of 0.05 was used for multiple comparisons. When the group effects occurred, we further explore the specific smoking effects (i.e., smokers > non-smokers or smokers < non-smokers) on ReHo. We extracted the averaged ReHo from significant clusters showing group effects, and then performed the 1-way ANCOVA controlling for age and education level to determine the specific smoking effects on ReHo. When the group-by-sex interaction effects occurred, the sex-specific effects of smoking on ReHo were investigated. The mean ReHo values from clusters showing group-by-sex interaction effects were extracted, and then the 1-way ANCOVAs controlling for age and education level were conducted to compare male smokers vs. male non-smokers and female smokers vs. female non-smokers separately.
For smokers, Pearson correlation analysis was performed to investigate whether there were correlations between the altered ReHo and the smoking-related variables (i.e., duration of smoking, age at first smoking, FTND and craving scores). A p-value of < 0.05 was considered significant.
Results
Demographic and Clinical Measures of Participants
Table 1 lists the demographic and clinical measures of the subjects used in this study. No significant difference was found in gender among the groups. For age, no main effect of group (p = 0.77) or group by sex interaction (p = 0.83) were found, while there was a significant main effect of sex (p = 0.002). For years of education, significant main effect of group (p < 0.001) and group by sex interaction (p < 0.001) were revealed, while there was no main effect of sex (p = 0.35). Specifically, male smokers had higher education level than female smokers (p = 0.006), while male non-smokers had lower education level than female non-smokers (p = 0.005).
There were no significant differences in duration of smoking (p = 0.09), FTND (p = 0.42) or craving scores (p = 0.88) between male and female smokers, while male smokers had an earlier age at first smoking (p < 0.001) than female smokers. Smokers as a whole had higher scores than non-smokers on SAS (p < 0.001), SDS (p < 0.001) and BIS (p = 0.003), which is consistent with prior studies showing that smoking has often been associated with increased depression, anxiety and impulsivity (24, 25). Women had higher scores on SAS (p = 0.07), SDS (p = 0.03) and BIS (p = 0.003) than men. Although the SAS, SDS and BIS scores of smokers were higher than those of non-smokers, they did not meet the criteria for comorbid mood or attention disorders.
ReHo Changes in Smokers
Statistically significant main effects of smoking were observed for ReHo (voxel-level p < 0.05 FDR corrected; Figure 1). Further 1-way ANCOVA with age and education as covariates demonstrated that compared to non-smokers, smokers had significant greater ReHo in the left precuneus and right cerebellum posterior lobe (crus1/2) while lower ReHo in the right medial OFC extending to the ventral striatum/amygdala/parahippocampal gyrus, right lateral OFC, and left cerebellum anterior lobe (crus1) extending to the inferior temporal gyrus/fusiform gyrus (Table 2).
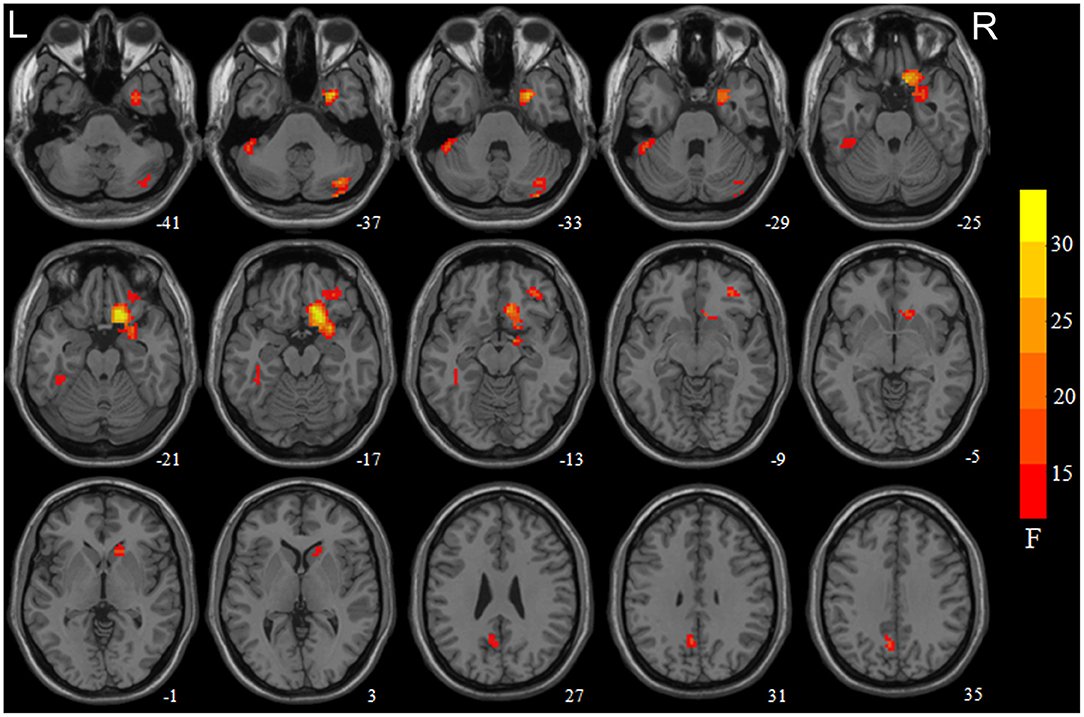
Figure 1. Brain areas with significant main effect of smoking on regional homogeneity (ReHo) revealed by the 2-way analysis of covariance with age and education levels as covariates. Regions in red-yellow are brain areas where ReHo was significantly altered in smokers relative non-smokers. The areas include the right medial orbitofrontal cortex (OFC) extend to the ventral striatum/amygdala/parahippocampa gyrus, right cerebellum crus1/2, left precuneus, left cerebellum crus1 extended to the inferior temporal gyrus/fusiform gyrus, and right lateral OFC. The results were multiple compared at voxel-level p < 0.05 (FDR corrected). The left side of the image corresponds to the left hemisphere of the brain.
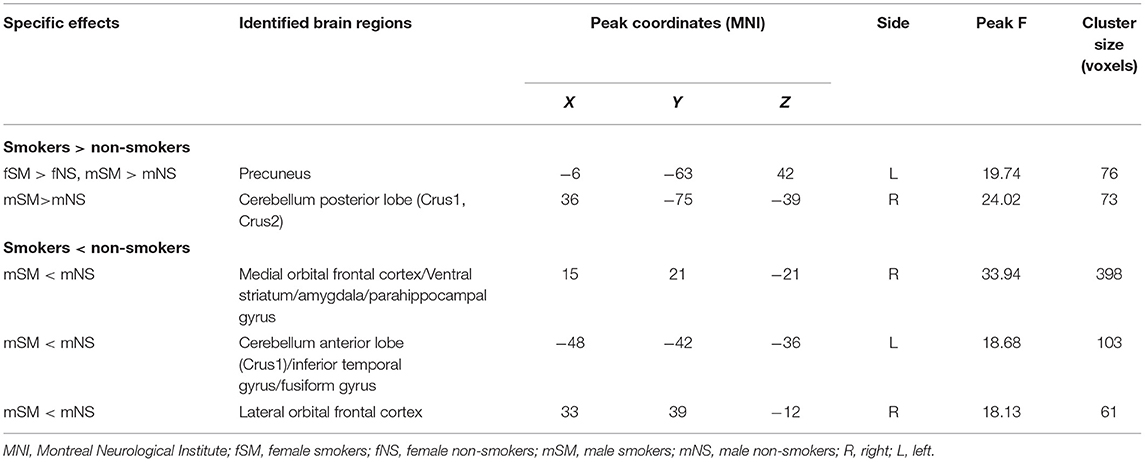
Table 2. Brain regions showing significant main effect of smoking on regional homogeneity (ReHo) (voxel-level p < 0.05 corrected for false discovery rate).
At a second stage, we analyzed the simple effects of smoking in the females and males in a separate analysis within the brain regions showing main effects of smoking. The male smokers were compared with male non-smokers and female smokers with female non-smokers. Independent 1-way ANCOVA controlling for age and education revealed that the main effects of smoking mostly came from male smokers except that female smokers had significantly higher ReHo as compared to female non-smokers in the left precuneus (for details, see Table 2).
A significant smoking and sex interaction effect on ReHo was identified in the left fusiform gyrus, left cerebellum crus1 extending to the inferior temporal gyrus, and right ventral striatum/putamen (voxel-level p < 0.05 FDR corrected, Table 3; Figure 2A). Post-hoc analyses revealed that male smokers had lower ReHo than male non-smokers in these regions, but no differences were observed between female smokers and non-smokers (Figure 2B). The ReHo in these regions showing interaction effects between smoking and sex did not significantly differ between male and female non-smokers, whereas male smokers demonstrated less ReHo than female smokers in these regions.
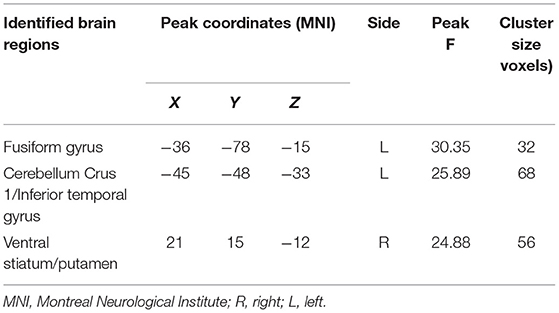
Table 3. Brain regions showing significant smoking-by-sex interaction on regional homogeneity (ReHo) (voxel-level p < 0.05 corrected for false discovery rate).
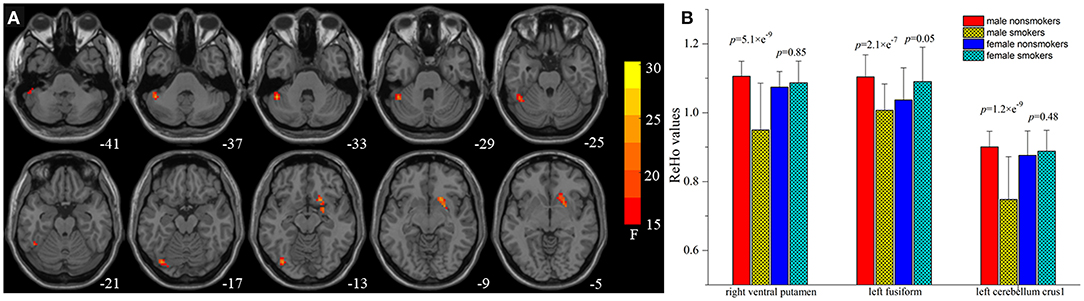
Figure 2. Brain areas with interaction between smoking and sex on regional homogeneity (ReHo) by the 2-way analysis of covariance (ANCOVA) with age and education levels as covariates. (A) Brain regions showing smoking-sex interaction on ReHo include the left fusiform gyrus, left cerebellum crus1 extended to the inferior temporal gyrus, and right ventral striatum [voxel-level p < 0.05 (FDR corrected]. (B) Post-hoc 1-way ANCOVA analyses revealed that male smokers had lower ReHo than male non-smokers in the right ventral striatum (p = 5.1 × e−9), left fusiform gyrus (p = 2.1 × e−7), left cerebellum crus1 extended to the inferior temporal gyrus (p = 1.2 × e−9), whereas female smokers showed no but no differences on ReHo values in these regions.
We also found that the lower ReHo in the left cerebellum crus1 exhibited higher craving scores in male smokers (r = −0.402; p = 0.022), whereas there was no significant correlation between the ReHo and the craving scores in female smokers (r = 0.110; p = 0.608) (Figure 3). The ReHo in the left cerebellum crus1 were more strongly correlated with craving in male smokers than in female smokers (p = 0.03).
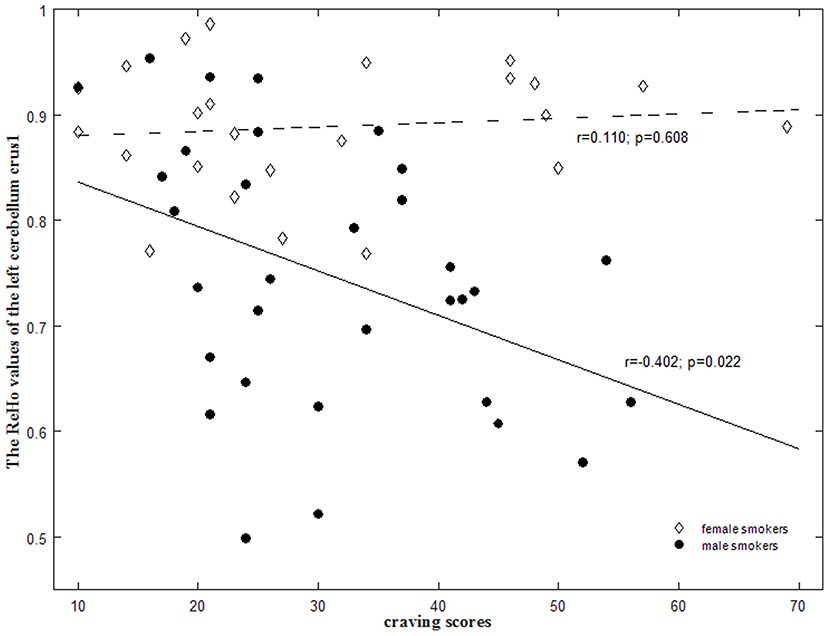
Figure 3. Sex-specific correlations between the regional homogeneity (ReHo) values and smoking-related measures in smokers. There were significant negative correlation of the mean ReHo values in the left cerebellum crus1 with the craving scores in male smokers (r = −0.402; p = 0.022) but not in female smokers (r = 0.110; p = 0.608). These correlations between ReHo values and craving scores were significantly different between male and female smokers (p = 0.03).
Discussion
In this study, we investigated sex-specific differences in local spontaneous brain activity between smokers and healthy non-smoking subjects using rs-fMRI and ReHo analysis. Our findings demonstrated that both male and female smokers showed higher ReHo in the left precuneus compared to sex-matched non-smokers while only male smokers had greater ReHo value in the cerebellum crus 1/2. However, male smokers had lower ReHo in the right medial OFC extending to the ventral staiatum/amygdala/parahippocampal gyrus, right lateral OFC, left cerebellum crus 1 extending to the inferior temporal gyrus, and left fusiform gyrus. Moreover, the ReHo in the left cerebellum crus1 was negatively correlated with craving scores in male smokers but not in female smokers. Taken together, these findings suggest that cigarette smoking may have differential effects on the spontaneous brain activity between men and women, especially in regions that are associated with reward and motivation functions, which are known to be affected by nicotine dependence.
One of the main results of this study using smoking by sex interaction, was found to reduce ReHo in several regions, such as the right ventral striatum/putamen, left fusiform gyrus, and left cerebellum crus 1 extending to the inferior temporal gyrus (Table 3; Figure 2). The ventral striatum is functionally implicated in reward anticipation, reward-related behaviors, and reward outcome of individuals with substance use disorders (26) and it is a reinforcement site (27). Men who smoke for the reinforcing effects of nicotine have significant responses in the ventral striatum. Using the same dataset and functional connectivity analysis, Lin et al. found that smoking has differential effects on the amygdala-OFC circuits between men and women, which is characterized by greater functional connectivity in the male circuits than that in women (12). Therefore, the change of ventral striatum activity may indicate that the reward learning defects of nicotine-addicted male individuals will eventually lead to the impairment of decision-making ability.
There is increasing evidence that cerebellum is involved in substance use disorders (28). Cerebellum was considered as a critical component of the addiction circuit, which is related to coordination of the smoking movement, motivation driving, and inhibition control (28). Male smokers, but not female smokers, have great cerebellar β2-nicotinic acetylcholine receptors (nAChRs) availability (29) and low putamen D2/D3 receptor availability (30), which may affect the up- and down-regulation. A PET studies showed that cigarette smoking increased density of nicotine binding and increased CBF in the cerebellum, which was correlated with plasma nicotine levels (31). Unlike the consensus that the GMV of cerebellum crus 1 in nicotine dependents is less than that in the controls (32–35), the activation of cerebellum varied with the expected degree of drug during exposure to drug-related cues rather than neutral cues (28, 36). In this study, we found decreased ReHo in the cerebellum crus 1/2 in male smokers (Table 3; Figure 2), while our previous studies on middle-aged heavy smokers reported that the ReHo of cerebellum increased (11). Lower ReHo in the left cerebellum crus 1 was correlated with the higher craving scores (r = −0.402; p = 0.022) in male smokers. This may be explained by the significant differences in smoking duration (SM 5.78 ± 2.84 years, SW 4.50 ± 2.50 years vs. 25.42 ± 9.10 years), and the complex mechanisms of chronic smoking, such as early dysfunction and late compensation Interestingly, female smokers did not show the same pattern of brain activity as male smokers. This finding may be partially explained by circulations in the menstrual cycle phase that modulates the reward-related regions in females (37, 38). An arterial spin labeling study examined the neural activity in response to video clip of smoking clues in females, differences emerged in the medial OFC in the follicular phase of the cycle (when progesterone levels are low), but no significant differences were observed in the luteal phase (39). Together, one may speculate that altered activity in the ventral striatum and cerebellum may indicate the selective effects of smoking on dopamine receptors and nicotinic receptors in men and women. The effect might be hampered in male smokers because of their greater reliance on nicotine. Female smokers may be exempted from smoking addiction because of their menstrual cycle.
Our team has been focusing on structural and functional MRI to study the neural mechanism underlying substance addictions. For example, Wu et al. (11) revealed that ReHo was significantly reduced in default-mode, frontoparietal attention and inhibition control networks, and regions related to motor planning increased in among the middle-aged chronic smokers (11). Lin et al. (40) and Cai et al. (35) reported the decreased nodal efficiency in default-mode and cerebellum-striatum networks, respectively, among the middle-aged chronic smokers. Qiu et al. comparing the differences of whole-brain ALFF between young adult smokers and controls, it was found that the ALFF of smoking group in the right MPFC/ventral striatum and left temporal gyrus was significantly higher than that of healthy group (41). Similarly, compared to non-smokers, our study observed that significant spontaneous brain activity changes in the right medial OFC extending to the ventral striatum, amygdala and parahippocampal gyrus of young adult smokers (Table 2; Figure 1), which is consistent with our previous results.
It is well-accepted that addiction produce the long-lasting functional and structural plasticity alterations in the corticostriatal-limbic circuitry (42). The medial OFC, ventral striatum, and amygdala, are core components of the corticostriatal-limbic circuitry related to addiction. The medial OFC is involved in integrating endogenous and exogenous information to make decision, playing an important role in the development and maintenance of addictive behaviors (43). The parahippocampal gyrus and amygdala are brain areas associated with emotional and memory processing (44), which may mediate the relief from negative emotions by smoking (45). The mesocorticolimbic dopamine systems including the amygdala, nucleus accumbens, and prefrontal cortex, are thought to be involved in burst release of dopamine and production of pleasure feelings (46). The finding of dysfunction within the prefrontal-striatal circuits is analogous to previous task-related fMRI studies showing greater activity in the reward regions in response to SCs relative to non-SCs (47–49). Similarly, rs-fMRI studies showed disrupted activity in the medial frontal cortex and precuneus/posterior cingulate cortex (11, 50, 51). Moreover, structural MRI studies exhibited that smokers had lower gray matter volumes or densities in the medial frontal cortex, precuneus, parahippocampal gyrus and temporal lobe (52–55) and reduced cortical thickness in the medial OFC (56). Taken together, our study backed up the idea that the imbalance between cognitive and reward functions in the brain may lead to smoking addiction.
Several limitations in this study must be considered before interpreting the findings. First, the number of participants in each group is relatively small, which may bias the sex-specific effects of cigarette smoking on spontaneous brain activity. Second, age or education level was not well-matched among groups. Although these factors were used as covariates in the statistical analysis, potential effects from these factors cannot be absolutely ruled out. Future studies with matched larger samples should be needed to verify the results. Third, this study is staged, longitudinal study is needed to draw conclusions about whether sex differences are a cause or a consequence of nicotine addiction. Therefore, our findings should be considered as preliminary and further longitudinal studies with larger sample size should be investigated to formulate final conclusions.
In summary, the harm of cigarette smoking is recessive, and to a certain extent it is lagging. It is crucial to investigate the different neural mechanisms underlying male and female in smoking behaviors and nicotine dependence. Our findings indicate that cigarette smoking has differential effects on reward-related activity between men and women. Our study may provide a methodological framework for education of quitting smoke, intervention treatment, and health management.
Data Availability Statement
The raw data supporting the conclusions of this article will be made available by the authors, without undue reservation.
Ethics Statement
The studies involving human participants were reviewed and approved by the Research Ethics Committee of Renji Hospital, School of Medicine of Shanghai Jiaotong University. The patients/participants provided their written informed consent to participate in this study.
Author Contributions
FL and HL designed and conceptualized the study. XH, YW, WD, YS, and YZ collected the clinical and MRI data. YK organized the data. ZW and FL analyzed the MRI data and wrote the manuscript. All authors contributed to the article and approved the submitted version.
Funding
This work was supported by the National Natural Science Foundation of China (Grant Nos. 81571757, 82171885) and the Frontier Scientific Significant Breakthrough Project of CAS (Grant No. QYZDB-SSW-SLH046), the Shanghai Science and Technology Committee Project (Natural Science Funding; grant number. 20ZR1433200), and the Explorer Project in Shanghai (Grant No. 21TS1400700).
Conflict of Interest
The authors declare that the research was conducted in the absence of any commercial or financial relationships that could be construed as a potential conflict of interest.
Publisher's Note
All claims expressed in this article are solely those of the authors and do not necessarily represent those of their affiliated organizations, or those of the publisher, the editors and the reviewers. Any product that may be evaluated in this article, or claim that may be made by its manufacturer, is not guaranteed or endorsed by the publisher.
References
1. Britton J. Death, disease, and tobacco. Lancet. (2017) 389:1861–2. doi: 10.1016/S0140-6736(17)30867-X
2. Rigotti NA, Kruse GR, Livingstone-Banks J, Hartmann-Boyce J. Treatment of tobacco smoking: a review. JAMA. (2022) 327:566–77. doi: 10.1001/jama.2022.0395
3. McKee SA, Smith PH, Kaufman M, Mazure CM, Weinberger AH. Sex differences in varenicline efficacy for smoking cessation: a meta-analysis. Nicotine Tob Res. (2016) 18:1002–11. doi: 10.1093/ntr/ntv207
4. Smith PH, Bessette AJ, Weinberger AH, Sheffer CE, McKee SA. Sex/gender differences in smoking cessation: a review. Prev Med. (2016) 92:135–40. doi: 10.1016/j.ypmed.2016.07.013
5. Becker JB, Hu M. Sex differences in drug abuse. Front Neuroendocrin. (2008) 29:36–47. doi: 10.1016/j.yfrne.2007.07.003
6. Franklin TR, Wetherill RR, Jagannathan K, Johnson B, Mumma J, Hager N, et al. The effects of chronic cigarette smoking on gray matter volume: influence of sex. PLos ONE. (2014) 9:e104102. doi: 10.1371/journal.pone.0104102
7. Dumais KM, Franklin TR, Jagannathan K, Hager N, Gawrysiak M, Betts J, et al. Multi-site exploration of sex differences in brain reactivity to smoking cues: consensus across sites and methodologies. Drug Alcohol Depen. (2017) 178:469–76. doi: 10.1016/j.drugalcdep.2017.05.044
8. McCarthy JM, Dumais KM, Zegel M, Pizzagalli DA, Olson DP, Moran LV, et al. Sex differences in tobacco smokers: executive control network and frontostriatal connectivity. Drug Alcohol Depen. (2019) 195:59–65. doi: 10.1016/j.drugalcdep.2018.11.023
9. Beltz AM, Berenbaum SA, Wilson SJ. Sex differences in resting state brain function of cigarette smokers and links to nicotine dependence. Exp Clin Psychopharm. (2015) 23:247–54. doi: 10.1037/pha0000033
10. Wetherill RR, Jagannathan K, Shin J, Franklin TR. Sex differences in resting state neural networks of nicotine-dependent cigarette smokers. Addict Behav. (2014) 39:789–92. doi: 10.1016/j.addbeh.2014.01.006
11. Wu GY, Yang SQ, Zhu L, Lin FC. Altered spontaneous brain activity in heavy smokers revealed by regional homogeneity. Psychopharmacology. (2015) 232:2481–9. doi: 10.1007/s00213-015-3881-6
12. Lin F, Han X, Wang Y, Ding W, Sun Y, Zhou Y, et al. Sex-specific effects of cigarette smoking on caudate and amygdala volume and resting-state functional connectivity. Brain Imaging Behav. (2021) 15:1–13. doi: 10.1007/s11682-019-00227-z
13. Gusnard DA, Raichle ME. Searching for a baseline: functional imaging and the resting human brain. Nat Rev Neurosci. (2001) 2:685–94. doi: 10.1038/35094500
14. Zang YF, Jiang TZ, Lu YL, He Y, Tian LX. Regional homogeneity approach to fMRI data analysis. Neuroimage. (2004) 22:394–400. doi: 10.1016/j.neuroimage.2003.12.030
15. Zuo XN, Xu T, Jiang LL, Yang Z, Cao XY, He Y, et al. Toward reliable characterization of functional homogeneity in the human brain: preprocessing, scan duration, imaging resolution and computational space. Neuroimage. (2013) 65:374–86. doi: 10.1016/j.neuroimage.2012.10.017
16. Jiang LL, Zuo XN. Regional homogeneity: a multimodal, multiscale neuroimaging marker of the human connectome. Neuroscientist. (2016) 22:486–505. doi: 10.1177/1073858415595004
17. Kwan LY, Eaton DL, Andersen SL, Dow-Edwards D, Levin ED, Talpos J, et al. This is your teen brain on drugs: in search of biological factors unique to dependence toxicity in adolescence. Neurotoxicol Teratol. (2020) 81:106916. doi: 10.1016/j.ntt.2020.106916
18. Dosenbach NU, Nardos B, Cohen AL, Fair DA, Power JD, Church JA, et al. Prediction of individual brain maturity using fMRI. Science. (2010) 329:1358–61. doi: 10.1126/science.1194144
19. Lynch KM, Cabeen RP, Toga AW, Clark KA. Magnitude and timing of major white matter tract maturation from infancy through adolescence with Noddi. Neuroimage. (2020) 212:116672. doi: 10.1016/j.neuroimage.2020.116672
20. Heatherton TF, Kozlowski LT, Frecker RC, Fagerstrom KO. The fagerstrom test for nicotine dependence - a revision of the fagerstrom tolerance questionnaire. Br J Addict. (1991) 86:1119–27. doi: 10.1111/j.1360-0443.1991.tb01879.x
21. Cox LS, Tiffany ST, Christen AG. Evaluation of the brief questionnaire of smoking urges (qsu-brief) in laboratory and clinical settings. Nicotine Tob Res. (2001) 3:7–16. doi: 10.1080/14622200020032051
22. Power JD, Barnes KA, Snyder AZ, Schlaggar BL, Petersen SE. Steps toward optimizing motion artifact removal in functional connectivity MRI; a reply to carp. Neuroimage. (2013) 76:439–41. doi: 10.1016/j.neuroimage.2012.03.017
23. Yan CG, Cheung B, Kelly C, Colcombe S, Craddock RC, Di Martino A, et al. A comprehensive assessment of regional variation in the impact of head micromovements on functional connectomics. Neuroimage. (2013) 76:183–201. doi: 10.1016/j.neuroimage.2013.03.004
24. Mitchell SH. Measuring impulsivity and modeling its association with cigarette smoking. Behav Cogn Neurosci Rev. (2004) 3:261–75. doi: 10.1177/1534582305276838
25. Covey LS, Glassman AH, Stetner F. Depression and depressive symptoms in smoking cessation. Compr Psychiatry. (1990) 31:350–4. doi: 10.1016/0010-440X(90)90042-Q
26. Luijten M, Schellekens AF, Kuhn S, Machielse MW, Sescousse G. Disruption of reward processing in addiction : an image-based meta-analysis of functional magnetic resonance imaging studies. JAMA Psychiatry. (2017) 74:387–98. doi: 10.1001/jamapsychiatry.2016.3084
27. Cosgrove KP, Wang S, Kim SJ, McGovern E, Nabulsi N, Gao H, et al. Sex differences in the brain's dopamine signature of cigarette smoking. J Neurosci. (2014) 34:16851–5. doi: 10.1523/JNEUROSCI.3661-14.2014
28. Moulton EA, Elman I, Becerra LR, Goldstein RZ, Borsook D. The cerebellum and addiction: insights gained from neuroimaging research. Addict Biol. (2014) 19:317–31. doi: 10.1111/adb.12101
29. Cosgrove KP, Esterlis I, McKee SA, Bois F, Seibyl JP, Mazure CM, et al. Sex differences in availability of beta(2)*-nicotinic acetylcholine receptors in recently abstinent tobacco smokers. Arch Gen Psychiat. (2012) 69:418–27. doi: 10.1001/archgenpsychiatry.2011.1465
30. Brown AK, Mandelkern MA, Farahi J, Robertson C, Ghahremani DG, Sumerel B, et al. Sex differences in striatal dopamine D2/D3 receptor availability in smokers and non-smokers. Int J Neuropsychopharmacol. (2012) 15:989–94. doi: 10.1017/S1461145711001957
31. Domino EF, Ni L, Xu Y, Koeppe RA, Guthrie S, Zubieta JK. Regional cerebral blood flow and plasma nicotine after smoking tobacco cigarettes. Prog Neuropsychopharmacol Biol Psychiatry. (2004) 28:319–27. doi: 10.1016/j.pnpbp.2003.10.011
32. Kuhn S, Romanowski A, Schilling C, Mobascher A, Warbrick T, Winterer G, et al. Brain grey matter deficits in smokers: focus on the cerebellum. Brain Struct Funct. (2012) 217:517–22. doi: 10.1007/s00429-011-0346-5
33. Peng P, Li M, Liu H, Tian YR, Chu SL, Van Halm-Lutterodt N, et al. Brain structure alterations in respect to tobacco consumption and nicotine dependence: a comparative voxel-based morphometry study. Front Neuroanat. (2018) 12:43. doi: 10.3389/fnana.2018.00043
34. Shen Z, Huang P, Wang C, Qian W, Yang Y, Zhang M. Cerebellar gray matter reductions associate with decreased functional connectivity in nicotine-dependent individuals. Nicotine Tob Res. (2017) 20:440–47. doi: 10.1093/ntr/ntx168
35. Cai Z, Wang P, Liu B, Zou Y, Wu S, Tian J, et al. To explore the mechanism of tobacco addiction using structural and functional MRI: a preliminary study of the role of the cerebellum-striatum circuit. Brain Imag Behav. (2021). doi: 10.1007/s11682-021-00546-0. [Epub ahead of print].
36. Volkow ND, Wang GJ, Ma YM, Fowler JS, Zhu W, Maynard L, et al. Expectation enhances the regional brain metabolic and the reinforcing effects of stimulants in cocaine abusers. J Neurosci. (2003) 23:11461–8. doi: 10.1523/JNEUROSCI.23-36-11461.2003
37. Lynch WJ, Sofuoglu M. Role of progesterone in nicotine addiction: evidence from initiation to relapse. Exp Clin Psychopharm. (2010) 18:451–61. doi: 10.1037/a0021265
38. Dreher JC, Schmidt PJ, Kohn P, Furman D, Rubinow D, Berman KF. Menstrual cycle phase modulates reward-related neural function in women. Proc Natl Acad Sci USA. (2007) 104:2465–70. doi: 10.1073/pnas.0605569104
39. Franklin TR, Jagannathan K, Wetherill RR, Johnson B, Kelly S, Langguth J, et al. Influence of menstrual cycle phase on neural and craving responses to appetitive smoking cues in naturally cycling females. Nicotine Tob Res. (2015) 17:390–7. doi: 10.1093/ntr/ntu183
40. Lin FC, Wu GY, Zhu L, Lei H. Altered brain functional networks in heavy smokers. Addict Biol. (2015) 20:809–19. doi: 10.1111/adb.12155
41. Qiu X, Han X, Wang Y, Ding W, Sun Y, Zhou Y, et al. Interaction between smoking and internet gaming disorder on spontaneous brain activity. Front Psychiatry. (2020) 11:586114. doi: 10.3389/fpsyt.2020.586114
42. Hyman SE, Malenka RC, Nestler EJ. Neural mechanisms of addiction: the role of reward-related learning and memory. Annu Rev Neurosci. (2006) 29:565–98. doi: 10.1146/annurev.neuro.29.051605.113009
43. Volkow ND, Fowler JS, Wang GJ. The addicted human brain: insights from imaging studies. J Clin Invest. (2003) 111:1444–51. doi: 10.1172/JCI18533
44. Koob GF, Volkow ND. Neurocircuitry of addiction. Neuropsychopharmacol. (2010) 35:217–38. doi: 10.1038/npp.2009.110
45. Clancy N, Zwar N, Richmond R. Depression, smoking and smoking cessation: a qualitative study. Fam Pract. (2013) 30:587–92. doi: 10.1093/fampra/cmt032
46. Stamatakis AM, Sparta DR, Jennings JH, McElligott ZA, Decot H, Stuber GD. Amygdala and bed nucleus of the stria terminalis circuitry: implications for addiction-related behaviors. Neuropharmacology. (2014) 76:320–8. doi: 10.1016/j.neuropharm.2013.05.046
47. Franklin TR, Lohoff FW, Wang Z, Sciortino N, Harper D, Li Y, et al. Dat genotype modulates brain and behavioral responses elicited by cigarette cues. Neuropsychopharmacol. (2009) 34:717–28. doi: 10.1038/npp.2008.124
48. Franklin TR, Wang Z, Wang J, Sciortino N, Harper D, Li Y, et al. Limbic activation to cigarette smoking cues independent of nicotine withdrawal: a perfusion fMRI study. Neuropsychopharmacol. (2007) 32:2301–9. doi: 10.1038/sj.npp.1301371
49. Wetherill RR, Young KA, Jagannathan K, Shin J, O'Brien CP, Childress AR, et al. The impact of sex on brain responses to smoking cues: a perfusion fMRI study. Biol Sex Differ. (2013) 4:9. doi: 10.1186/2042-6410-4-9
50. Tang J, Liao Y, Deng Q, Liu T, Chen X, Wang X, et al. Altered spontaneous activity in young chronic cigarette smokers revealed by regional homogeneity. Behav Brain Funct. (2012) 8:44. doi: 10.1186/1744-9081-8-44
51. Yu R, Zhao L, Tian J, Qin W, Wang W, Yuan K, et al. Regional homogeneity changes in heavy male smokers: a resting-state functional magnetic resonance imaging study. Addiction Biology. (2013) 18:729–31. doi: 10.1111/j.1369-1600.2011.00359.x
52. Liao YH, Tang JS, Liu TQ, Chen XG, Hao W. Differences between smokers and non-smokers in regional gray matter volumes: a voxel-based morphometry study. Addict Biol. (2012) 17:977–80. doi: 10.1111/j.1369-1600.2010.00250.x
53. Gallinat J, Meisenzahl E, Jacobsen LK, Kalus P, Bierbrauer J, Kienast T, et al. Smoking and structural brain deficits: a volumetric Mr investigation. Eur J Neurosci. (2006) 24:1744–50. doi: 10.1111/j.1460-9568.2006.05050.x
54. Brody AL, Mandelkern MA, Jarvik ME, Lee GS, Smith EC, Huang JC, et al. Differences between smokers and nonsmokers in regional gray matter volumes and densities. Biol Psychiatry. (2004) 55:77–84. doi: 10.1016/S0006-3223(03)00610-3
55. Sutherland MT, Riedel MC, Flannery JS, Yanes JA, Fox PT, Stein EA, et al. Chronic cigarette smoking is linked with structural alterations in brain regions showing acute nicotinic drug-induced functional modulations. Behav Brain Funct. (2016) 12:16. doi: 10.1186/s12993-016-0100-5
Keywords: sex, regional homogeneity, resting-state fMRI, craving, smokers
Citation: Wen Z, Han X, Wang Y, Ding W, Sun Y, Kang Y, Zhou Y, Lei H and Lin F (2022) Sex-Dependent Alterations of Regional Homogeneity in Cigarette Smokers. Front. Psychiatry 13:874893. doi: 10.3389/fpsyt.2022.874893
Received: 13 February 2022; Accepted: 28 March 2022;
Published: 25 April 2022.
Edited by:
Yanhui Liao, Zhejiang University School of Medicine, ChinaReviewed by:
Lixia Tian, Beijing Jiaotong University, ChinaKai Yuan, Xidian University, China
Chun Xue Wu, Capital Medical University, China
Copyright © 2022 Wen, Han, Wang, Ding, Sun, Kang, Zhou, Lei and Lin. This is an open-access article distributed under the terms of the Creative Commons Attribution License (CC BY). The use, distribution or reproduction in other forums is permitted, provided the original author(s) and the copyright owner(s) are credited and that the original publication in this journal is cited, in accordance with accepted academic practice. No use, distribution or reproduction is permitted which does not comply with these terms.
*Correspondence: Fuchun Lin, ZmNsaW4mI3gwMDA0MDt3aXBtLmFjLmNu
†These authors have contributed equally to this work and share first authorship