- Department of Neurology (Sleep Disorders), The Affiliated Chaohu Hospital of Anhui Medical University, Hefei, China
Objectives: To examine serum concentrations of aquaporin-4 (AQP4), connexin-30 (CX30), connexin-43 (CX43), and their correlations with cognitive function in the patients with chronic insomnia disorder (CID).
Methods: We enrolled 76 subjects with CID and 32 healthy controls (HCs). Serum levels of AQP4, CX30, and CX43 were measured by enzyme-linked immunosorbent assays. Sleep quality was assessed with the Pittsburgh Sleep Quality Index (PSQI) and polysomnography, and mood was evaluated with 17-item Hamilton Depression Rating Scale and 14-item Hamilton Anxiety Rating Scale. Cognitive function was evaluated by the Chinese-Beijing Version of Montreal Cognitive Assessment (MoCA-C) and Nine Box Maze Test.
Results: The serum levels of AQP4, CX43, and CX30 were significantly reduced in the CID group compared to the HCs. Partial correlation analysis showed that the biomarkers showed no significant correlations with PSQI score, AHI, ODI and TS90, but AQP4, CX43, and CX30 were positively associated with the percentage and total time of slow wave sleep in the CID group. Serum concentrations of AQP4 and CX30 were positively associated with MoCA-C score in the CID group, and AQP4 level negatively correlated with spatial working memory errors.
Conclusions: Subjects with CID patients have decreased serum levels of AQP4, CX30, and CX43 indicating astrocyte dysfunction, which could be related to poor objective sleep quality and/or cognition dysfunction.
Introduction
Insomnia is characterized by difficulty in initiating and/or maintaining sleep with unintentional early morning awakenings along with significant impairment of daytime functioning (1). Chronic insomnia disorder (CID) is a heavy burden to individuals and society as it is associated with physical and mental diseases (2–4). Despite recent progress in the treatment of chronic insomnia, many patients still struggle with this condition (5). One reason is that the mechanism underlying CID remains unclear.
Whether CID is a result of subtle brain injury remains a topic of intense debate. A correlation between brain injury and insomnia is supported by imaging findings showing that CID patients have some degree of brain damage (6, 7). Magnetic resonance imaging revealed atrophy in frontal and subcortical areas and changes in the white matter tracts connecting these areas in patients with CID compared to healthy controls (HCs) (8). Another group proposed that insomnia is related to brain functional connectivity abnormalities (9). Unfortunately, neuroimaging study reproducibility has been increasingly questioned due to low test-retest reliability, and identifying additional biomarkers is of particular interest to researchers and clinicians (10). Serological testing is a promising option because of the simplicity and convenience. In addition, past studies have mainly shown a relationship between insomnia and specific brain regions (11). Few researchers have focused on changes in more minute structures, such as at the cellular level.
Although astrocytes outnumber neurons, it is thought that astrocytes only perform a nutritional and supportive function in the brain. In 1895, Cajal proposed that astrocytes regulate sleep by extending their processes into synapses during sleep and retracting these processes during wakefulness (12). However, it was not until 2009 that the first evidence show that the sleep/wake cycle was regulated by these glial cells (13). Since then, the importance of astrocytes in sleep regulation has become increasingly apparent. It is widely known that brain damage is associated with cognitive impairment (14). While cognition is traditionally attributed to neuronal activity, it is becoming increasingly clear that astrocytes also contribute to cognitive function and sleep regulation (15). Thus, it is necessary to conduct more studies to explore correlations between astrocyte function and the states of cognition and sleep.
We previously showed that changes in levels of neurofilament heavy and light chains, neuron-specific enolase, and S100 calcium binding protein B (S100B), which reflect structural damage in neurons and astrocytes, were correlated with insomnia severity and/or cognitive impairment (16). More importantly, even after long-term effective treatment, the serum S100B levels did not significantly restore to normal concentration in the patients with CID (16). Thus, the relief/remission of their illness may not be accompanied by normalization of changed protein levels (damage of astrocytes structure may be a trait) (16). Subjects with CID also exhibit significantly lower levels of glial cell line-derived neurotrophic factor (GDNF) and brain-derived neurotrophic factor (BDNF) than healthy people (17). These findings strongly implicate astrocyte dysfunction in CID, but more evidence is needed to support this hypothesis (17). Additional studies are needed to investigate other astrocyte-related markers to better understand the mechanism of CID.
Previous studies typically focused astrocytes themselves rather than their networks. However, one of the unique characteristics of astrocytes is the high level of connexins including connexin-30 and -43 (CX30 and CX43) (18). Connexins form gap-junction channels that are responsible for direct intercellular communication and allow small molecules and ions to pass freely between cells (19). Recently, increased Cx43 levels were found during recovery sleep in adult male Wistar rats that previously experienced sleep deprivation (20). Cx30 and Cx43 mRNA show time-of-day-dependent expression in the suprachiasmatic nucleus of the hypothalamus, which is believed to play an important role in sleep-wake regulation (21). These results suggested that astrocyte connexins contribute to sleep regulation. Another major function of sleep is to clear out metabolic waste products from the brain through the glymphatic system (22). Aquaporin-4 (AQP4) is mainly expressed on astrocytic end-feet, which play a significant role in waste clearance (23). Previous findings revealed that AQP4 deletion impaired glymphatic transport and short-term memory after chronic sleep deprivation in mice (24). However, there is no research describing a link between AQP4 and CID or investigating whether serum AQP4 levels are associated with cognitive function in subjects with CID.
AQP4 and CXs have been investigated in neurological diseases such as Parkinson's disease, hypoxic brain edema, and traumatic brain injury (25–28). For example, Ramiro et al. found that serum AQP4 levels correlated with stroke severity and predicted early neurological function improvement in stroke patients (29). Swelling of astrocytic end-foot processes is accompanied by decreased cell membrane expression of AQP4 and CXs (30, 31). Protein level alterations in the brain may be in part reflected in the blood, but the precise source of serum AQP4 and CXs has not been clarified (32). One possible explanation is the presence of brain-derived vesicles containing AQP4 and CXs. When astrocyte function decreases, fewer brain-derived vesicles containing AQP4 and CXs are released, leading to lower serum concentrations.
The aim of this study was to investigate the possibility of astrocyte dysfunction in CID, we measured serum levels of AQP4, CX43, and CX30 in subjects with CID and HCs. We also explored whether altered serum levels of these astrocyte-related markers correlated with poor sleep quality and/or cognitive impairment, and if they could be used to distinguish between the CID and HC groups.
Materials and Methods
Subjects
A total of 108 people, including 76 subjects with CID and 32 HCs, were involved in the study. The flowchart of the study participants is presented in Figure 1. All subjects were recruited from the Clinic of Sleep Disorders in the Affiliated Chaohu Hospital of Anhui Medical University. The patients (30 males and 46 females between 18 and 60 years old) were diagnosed with CID following the criteria in the third edition of the International Classification of Sleep Disorders (33). Furthermore, they should meet the following criteria: (1) age is 18–60 years old; (2) normal ability to complete the assessment scales and the memory test without problem in hearing and vision; (3) not experiencing any somatic disease (including immunologic, endocrine, cardiovascular, neurologic, liver, or kidney disease or organic brain disease) or any other psychiatric disorders; (4) not taking sedatives, antidepressants, antipsychotics, or any other drugs within 4 weeks; (5) non-pregnant or non-lactating females.
Thirty-two HCs were chosen as the control group using the following criteria: (a) no complaints or history of insomnia or mood disorders, (b) total score <7 on the Pittsburgh Sleep Quality Index (PSQI), (c) total score <7 on the Hamilton Depression Rating Scale-17 (HAMD), (d) a total score <7 on the Hamilton Anxiety Scale-14 (HAMA), and (e) Montreal Cognitive Assessment, Chinese-Beijing Version (MoCA-C) score >26 (34–37). Control subjects were recruited from the physical examination centers at the same hospitals. They received a comprehensive medical involving a medical history review, electrocardiogram, blood testing (hematology and serology), and physical examination. The control subjects were also matched to cases by age, gender, geographic region, and ethnicity. As pre-established criteria, any somatic disease (including immunologic, endocrine, cardiovascular, neurologic, liver, or kidney disease or organic brain disease) or any other psychiatric disorders were excluded.
Baseline Data Collection
Information on demographic characteristics, medical history, and family history were collected using a questionnaire developed by our group.
Assessment of Mood States
Depression and anxiety severity were separately assessed with the HAMD and HAMA, respectively (35, 36). The maximum score on the HAMD is 52, and the highest score on the HAMA is 56. A higher total score indicates greater depression or anxiety symptom severity. In China, the HAMD score cut-offs are as follows: ≤ 7, no depression; ≥8, mild depression; ≥18, moderate depression; and ≥24, severe depression. HAMA ≥7 indicates that the patient may have anxiety, and HAMA <7 indicates that the patient has no anxiety.
Subjective and Objective Sleep Evaluations
The PSQI is designed to measure subjective quality of sleep over the past month. It measures seven components of sleep: latency, quality, duration, disturbances, efficiency, the use of sleep medications, and daytime dysfunction. A higher total score indicates worse sleep quality (34).
All participants underwent one night of polysomnography (PSG) to acclimatize to the novel environment followed by 2 nights of PSG to collect study data. The recording assessed sleep continuity and structure. The former was measured as total sleep time (TST), sleep efficiency (SE), and sleep onset latency (SOL); the latter was evaluated for the following parameters: percentages of sleep stage 1 (N1%) and slow wave sleep (SWS, N3%), time awake after sleep onset, and time (REM) and percentage (REM%) of rapid eye movement sleep, apnea hypopnea index (AHI), oxygen desaturation index (ODI), SpO2 time <90% (TS90). Sleep parameters were staged according to the 2012 American Academy of Sleep Medicine Manual for the Scoring of Sleep and Associated Events recommendations (37).
Evaluation of Cognitive Function
Cognitive function was evaluated by experienced clinicians using MoCA-C and a modified version of the Nine Box Maze Test (NBMT). The MoCA-C scale is used for a screening of general cognitive abilities. The maximum score for the MoCA-C is thirty, however, the score <26 indicates the cognitive impairment in China (38). A modified version of the Nine Box Maze Test (NBMT) was used to evaluate memory, including spatial/object working memories (SWM/OWM), spatial/object reference memories (SRM/ORM), and object recognition memory (ORcM) (39). The testing procedures are described in detail elsewhere (40). The numbers of errors were counted to examine SWM, OWM, SRM, ORM, and ORcM performance.
Serum Sample Collection
Serum from blood samples was obtained the morning following PSG and immediately stored at −80°C until analysis. Serum concentrations of AQP4, CX43, and CX30 were measured by quantitative sandwich enzyme-linked immunosorbent assays according to the manufacturer's instructions (Wuhan colorfulGene biological technology, Wuhan, China).
Statistical Analysis
All data were analyzed using SPSS® 20.0 for Windows (IBM Corp., Armonk, NY, USA). Normally distributed data are expressed as mean ± standard deviation (SD). Mann-Whitney U-tests were performed to analyze non-parametric data expressed as the 25th, 50th, and 75th percentiles (P25, P50, and P75, respectively). Partial correlation analyses were used to analyze correlations between serum biomarkers and sleep parameters and PSQI (adjusted for sex, age, education, HAMD, and HAMA) and between serum biomarkers and general cognitive function and multi-dimensional memory (adjusted for sex, age, education, HAMD, HAMA, and PSQI). The diagnostic accuracies of biomarkers were evaluated by constructing receiver operating characteristic (ROC) curves and calculating the areas under the curve (AUCs). Differences were considered significant at P < 0.05 for all analyses.
Results
General Information and Sleep Parameters
There were no significant differences in sex, age, or education between the two groups (Ps > 0.05, Table 1). Compared to the HC group, patients with CID had significantly higher HAMD and HAMA scores (Ps < 0.001, Table 1). Meanwhile, the patients with CID had significantly longer SOL and N1, and significantly lower SE, TST, and N3 than the HC (Ps < 0.001, Table 2). There were no significant differences in AHI, ODI, and TS90 between the two groups (Ps > 0.05, Table 2). These results indicated that the patients who were recruited into the study met our inclusion criteria. Sleep parameters are detailed in Table 2.
Cognitive Function and Serum Biomarkers in CID
Patients with CID had significantly lower MoCA-C scores (P < 0.001) and more SWM (P < 0.001) and ORcM (P < 0.01) errors than HC (Table 3). CID patients had significantly lower serum levels of AQP4, CX43, and CX30 (Ps < 0.001) compared to the HC group (Figure 2).
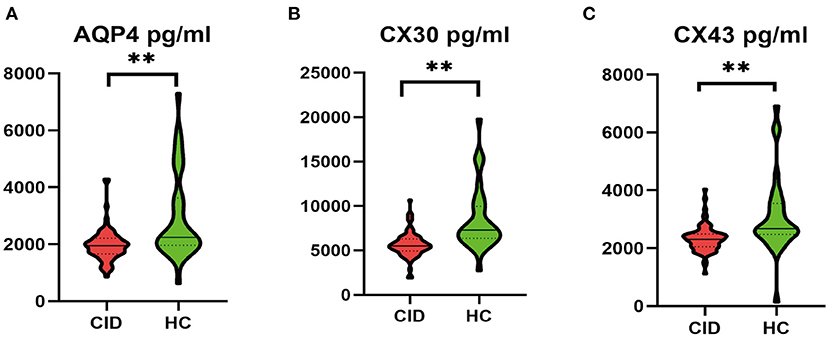
Figure 2. Comparison of serum protein levels between CID patients and HCs. **P < 0.01. AQP4, aquaporin-4; CID, chronic insomnia disorder; CX30, connexin 30; CX43, connexin 43; HC, healthy control.
Correlations Between Biomarkers, Sleep Parameters, and Cognitive Performance in CID
Partial correlations (adjusted for age, sex, education HAMD, and HAMA) between serum biomarkers and objective sleep parameters are listed in Table 4. The serum concentrations of AQP4, CX43, and CX30 were positively correlated with N3 (r = 0.250, P < 0.05; r = 0.323, P < 0.01; r = 0.334, P < 0.01). The same results were also observed in N3% (r = 0.233, P < 0.05; r = 0.302, P < 0.05; r = 0.335, P < 0.01). There were no significant correlations between serum astrocyte-related marker levels and PSQI scores, AHI, ODI, and TS90 (Ps >0.05). Partial correlation analysis (adjusted for age, sex, PSQI, HAMD, HAMA, and education) indicated that serum AQP4 level was positively correlated with MoCA-C score (r = 0.357, P < 0.01) and negatively correlated with SWM errors (r = −0.435, P < 0.01; Table 5). CX30 level was negatively correlated with MoCA-C score (r = 0.2.71, P < 0.01; Table 5).
Discussion
CID Is Associated With Astrocyte Dysfunction
In present study, we found that serum AQP4, CX43, and CX30 levels were significantly lower in the CID group compared to healthy individuals. These results indicate a certain extent of astrocytes dysfunction in patients with CID.
Our group has spent several years exploring the correlation between insomnia and astrocytes (16, 17). We found altered serum levels of astrocyte-related biomarkers in CID patients, with increases in S100B and glial fibrillary acidic protein and decreases in BDNF and GDNF, which led us to hypothesize a role of astrocyte dysfunction in CID (14). Interestingly, serum S100B levels did not normalize after effective therapy, indicating that astrocyte dysfunction may be an inherent trait in subjects who develop CID (13). However, this evidence is still preliminary (16). To obtain more results to support this hypothesis, we evaluated another group of astrocyte-related markers: AQP4, CX43, and CX30.
AQP4 and CXs are mainly expressed in astrocytes in the brain. We are aware of only one study describing the levels of AQP4 in the blood in neurological disorders, suggesting the lower the serum AQP4 levels, the higher the number of cerebral microbleed evaluated by MRI in the patients with Intracerebral Hemorrhage (32). This indicated an association between serum AQP4 levels and brain structure damage to some extent. Moreover, astrocytes dysfunction is found in most of brain pathologies, and are associated with changes in CX expression and functions. The basal swelling of astrocytic end-foot processes is accompanied by decreased expression of CX at the membrane (31). Obstructive sleep apnea (OSA) commonly co-occurred with insomnia and related to cognitive problems (41, 42). In addition, a recent study showed an increase in astrocytic AQP4 levels in mice, following exposure to chronic intermittent hypoxia (one of the main features of OSA) (43). In this study, none of the patients had OSA based on the respiratory PSG parameters. Thus, we have reason to believe that patients with CID have some astrocytes dysfunction according to the current study.
Astrocyte-Related Markers Were Associated With Sleep Homeostasis
Astrocytes are able to detect and respond to neuronal activity by increasing local calcium (Ca2+) transients. These astrocytes communicate through CXs and release gliotransmitters to modulate neuronal activity at multiple locations (44). Therefore, astrocytes are ideally positioned to promote synchronization of large-scale neuronal networks, such as slow wave sleep (SWS), which is a reliable index of sleep homeostasis (45). The role of astrocytes in sleep homeostasis was previously investigated in the dnSNARE (dominant-negative soluble N-ethylmaleimide-sensitive fusion protein attachment protein receptor) mouse model. The authors concluded that defective astrocyte transmission could reduce SWS but did not influence REM sleep or awakening (13). Others reported that blockade of astrocytic CX43 also reduced slow wave amplitude in mice (46). Moreover, the study found that AQP4 was also linked with SWS (22). However, previous reports were mainly based on animal experiments, and this association has not yet been established in CID patients. In the current study, AQP4, CX43, and CX30 levels in subjects with CID all positively correlated with N3 sleep, also known as SWS. Our results support the idea that astrocytes may play an important role in sleep homeostasis. Interestingly, there were no significant correlations between serum astrocyte-related marker levels (AQP4, CX43, and CX30) and subjective sleep parameters (PSQI scores). This suggests that subjective and objective sleep appear to reflect two distinct neurophysiological processes.
Astrocyte-Related Markers Were Associated With Cognitive Impairment
Astrocytes play a significant role in cognitive abilities. They are capable of (1) regulating extracellular glutamate and potassium ion uptake as well as Ca2+ concentration, (2) releasing and taking up neurotransmitters, and (3) controlling energy supply to neurons to facilitate cognition (47). Indeed, a recent study find that AQP4 knockout mice exhibited impaired memory consolidation in the Morris Water Maze used to test learning and memory function (48). In addition, microinjection of TAT-Gap19 (a Cx43-specific hemichannel inhibitor) into the ventricle of mice impaired short-term spatial memory in a delayed spontaneous alternation Y-maze task (49). One study also showed that mice lacking CX30 have impaired memory (50). However, it is not clear whether astrocyte dysfunction is associated with cognitive dysfunction in patients with CID. Our findings showed that serum CX30 and AQP4 levels positively correlated with cognition assessed by MoCA-C and NBMT in patients with CID. Contrary to expectations, this study did not reveal a correlation between CX43 and cognitive impairment. This may be due to the high molecular weight of CX43, which cannot easily pass the blood-brain barrier (19). It is unfortunate that we were only able to assess blood samples; future investigations can expand our findings by measuring AQP4 and CX levels in cerebrospinal fluid (CSF). It is notable that lower AQP4 expression was also observed in CSF from patients with Alzheimer's disease and Optic Neuromyelitis (51, 52). In addition, recent studies also demonstrated that the distribution of cerebrospinal fluid appears to be under a circadian control and that AQP4 seems to support this rhythm (53).
Possible Diagnostic and Prognostic Value of Astrocyte-Related Markers
ROC analysis was conducted to assess these biomarkers' discriminatory power for CID. The results showed that three proteins had AUC values of 0.726–0.823. The optimum cut-off values for serum AQP4, CX43, and CX30 for identifying subjects with CID were 2109.7, 2542.7, and 6348.5 pg/mL, respectively (Table 6). The use of biomarkers as an objective index may help support making a diagnosis of CID. Combining these three indicators may provide a sensitive and specific approach to distinguish between the CID and healthy people.
Limitations
Our findings should be considered in the context of several limitations. Our study is limited by its cross-sectional study design, we did follow up subjects with CID to assess patient outcomes. Therefore, it cannot be determined whether astrocyte-related markers are causally related to CID pathogenesis. In addition, the sample size was too small to accurately evaluate the different contributions of each biomarker to CID, and the results need to be validated in a larger sample. Finally, our research was limited to peripheral blood; future investigations should be performed with CSF samples.
Conclusions
The patients with CID had decreased concentrations of serum AQP4, CX43, and CX30, suggesting astrocytic pathological damages, which were linked to the decline of objective sleep quality and/or cognition ability.
Data Availability Statement
The raw data supporting the conclusions of this article will be made available by the authors, without undue reservation.
Ethics Statement
The studies involving human participants were reviewed and approved by Ethics Committee of The Affiliated Chaohu Hospitals of Anhui Medical University approved the study (Number KYXM-202108-005). The patients/participants provided their written informed consent to participate in this study.
Author Contributions
G-HC: involved in the concept generation, study design, application for funding and ethical committee, conduction of the study, and data acquisition. SY and PZ: data acquisition, analysis and interpretation of the PSG data, data entry, statistical analysis and interpretation, and preparation of the working draft of the manuscript. TH and X-YK: data acquisition. Y-JG, X-YL, J-TC, and SH: data acquisition, storage, and retrieval. All authors approved the final version of the manuscript prior to the submission.
Funding
This work was financially supported by the National Natural Science Foundation of China (81671316) and Anhui Medical University Foundation (2020xkj053).
Conflict of Interest
The authors declare that the research was conducted in the absence of any commercial or financial relationships that could be construed as a potential conflict of interest.
Publisher's Note
All claims expressed in this article are solely those of the authors and do not necessarily represent those of their affiliated organizations, or those of the publisher, the editors and the reviewers. Any product that may be evaluated in this article, or claim that may be made by its manufacturer, is not guaranteed or endorsed by the publisher.
Acknowledgments
Thanks to Charlesworth for providing language help.
References
1. Riemann D, Nissen C, Palagini L, Otte A, Perlis ML, Spiegelhalder K. The neurobiology, investigation, and treatment of chronic insomnia. Lancet Neurol. (2015) 14:547–58. doi: 10.1016/S1474-4422(15)00021-6
2. Hillman D, Mitchell S, Streatfeild J, Burns C, Bruck D, Pezzullo L. The economic cost of inadequate sleep. Sleep. (2018) 41:zsy083. doi: 10.1093/sleep/zsy083
3. Lee GB, Kim HC, Jung SJ. Association between sleep duration and augmentation index in post-menopausal women: a moderating role of depressive symptoms. Maturitas. (2021) 149:8–15. doi: 10.1016/j.maturitas.2021.04.007
4. Katzan IL, Thompson NR, Walia HK, Moul DE, Foldvary-Schaefer N. Sleep-related symptoms in patients with mild stroke. J Clin Sleep Med. (2020) 16:55–64. doi: 10.5664/jcsm.8122
5. Morin CM, Vallières A, Guay B, Ivers H, Savard J, Mérette C, et al. Cognitive behavioral therapy, singly and combined with medication, for persistent insomnia: a randomized controlled trial. JAMA. (2009) 301:2005–15. doi: 10.1001/jama.2009.682
6. Altena E, Vrenken H, Van Der Werf YD, van den Heuvel OA, Van Someren EJ. Reduced orbitofrontal and parietal gray matter in chronic insomnia: a voxel-based morphometric study. Biol Psychiatry. (2010) 67:182–5. doi: 10.1016/j.biopsych.2009.08.003
7. Joo EY, Noh HJ, Kim JS, Koo DL, Kim D, Hwang KJ, et al. Brain gray matter deficits in patients with chronic primary insomnia. Sleep. (2013) 36:999–1007. doi: 10.5665/sleep.2796
8. Koo DL, Shin JH, Lim JS, Seong JK, Joo EY. Changes in subcortical shape and cognitive function in patients with chronic insomnia. Sleep Med. (2017) 35:23–6. doi: 10.1016/j.sleep.2017.04.002
9. Jespersen KV, Stevner A, Fernandes H, Sørensen SD, Van Someren E, Kringelbach M, et al. Reduced structural connectivity in Insomnia Disorder. J Sleep Res. (2020) 29:e12901. doi: 10.1111/jsr.12901
10. Botvinik-Nezer R, Holzmeister F, Camerer CF, Dreber A, Huber J, Johannesson M, et al. Variability in the analysis of a single neuroimaging dataset by many teams. Nature. (2020) 582:84–8. doi: 10.1038/s41586-020-2314-9
11. Kay DB, Buysse DJ. Hyperarousal and beyond: new insights to the pathophysiology of insomnia disorder through functional neuroimaging studies. Brain Sci. (2017) 7:23. doi: 10.3390/brainsci7030023
12. Araque A, Carmignoto G, Haydon PG. Dynamic signaling between astrocytes and neurons. Annu Rev Physiol. (2001) 63:795–813. doi: 10.1146/annurev.physiol.63.1.795
13. Halassa MM, Florian C, Fellin T, Munoz JR, Lee SY, Abel T, et al. Astrocytic modulation of sleep homeostasis and cognitive consequences of sleep loss. Neuron. (2009) 61:213–9. doi: 10.1016/j.neuron.2008.11.024
14. Ott CV, Johnson CB, Macoveanu J, Miskowiak K. Structural changes in the hippocampus as a biomarker for cognitive improvements in neuropsychiatric disorders: a systematic review. Eur Neuropsychopharmacol. (2019) 29:319–29. doi: 10.1016/j.euroneuro.2019.01.105
15. Frank MG. The role of glia in sleep regulation and function. Handb Exp Pharmacol. (2019) 253:83–96. doi: 10.1002/cphy.c190022
16. Zhang P, Tan CW, Chen GH, Ge YJ, Xu J, Xia L, et al. Patients with chronic insomnia disorder have increased serum levels of neurofilaments, neuron-specific enolase and S100B: does organic brain damage exist? Sleep Med. (2018) 48:163–71. doi: 10.1016/j.sleep.2017.12.012
17. Zhang P, Li YX, Zhang ZZ, Yang Y, Rao JX, Xia L, et al. Astroglial mechanisms underlying chronic insomnia disorder: a clinical study. Nat Sci Sleep. (2020) 12:693–704. doi: 10.2147/NSS.S263528
18. Charvériat M, Naus CC, Leybaert L, Sáez JC, Giaume C. Connexin-dependent neuroglial networking as a new therapeutic target. Front Cell Neurosci. (2017) 11:174. doi: 10.3389/fncel.2017.00174
19. Giaume C, Naus CC, Sáez JC, Leybaert L. Glial connexins and pannexins in the healthy and diseased brain. Physiol Rev. (2021) 101:93–145. doi: 10.1152/physrev.00043.2018
20. Franco-Pérez J, Ballesteros-Zebadúa P, Fernández-Figueroa EA, Ruiz-Olmedo I, Reyes-Grajeda P, Paz C. Sleep deprivation and sleep recovery modifies connexin 36 and connexin 43 protein levels in rat brain. Neuroreport. (2012) 23:103–7. doi: 10.1097/WNR.0b013e32834e8fcb
21. Ali AAH, Stahr A, Ingenwerth M, Theis M, Steinhäuser C, von Gall C. Connexin 30 and connexin 43 show a time-of-day dependent expression in the mouse suprachiasmatic nucleus and modulate rhythmic locomotor activity in the context of chronodisruption. Cell Commun Signal. (2019) 17:61. doi: 10.1186/s12964-019-0370-2
22. Xie L, Kang H, Xu Q, Chen MJ, Liao Y, Thiyagarajan M, et al. Sleep drives metabolite clearance from the adult brain. Science. (2013) 342:373–7. doi: 10.1126/science.1241224
23. Mestre H, Hablitz LM, Xavier AL, Feng W, Zou W, Pu T, et al. Aquaporin-4-dependent glymphatic solute transport in the rodent brain. Elife. (2018) 7:e40070. doi: 10.7554/eLife.40070
24. Zhang R, Liu Y, Chen Y, Li Q, Marshall C, Wu T, et al. Aquaporin 4 deletion exacerbates brain impairments in a mouse model of chronic sleep disruption. CNS Neurosci Ther. (2020) 26:228–39. doi: 10.1111/cns.13194
25. Fujita A, Yamaguchi H, Yamasaki R, Cui Y, Matsuoka Y, Yamada KI, et al. Connexin 30 deficiency attenuates A2 astrocyte responses and induces severe neurodegeneration in a 1-methyl-4-phenyl-1,2,3,6-tetrahydropyridine hydrochloride Parkinson's disease animal model. J Neuroinflammation. (2018) 15:227. doi: 10.1186/s12974-018-1251-0
26. Sato S, Umenishi F, Inamasu G, Sato M, Ishikawa M, Nishizawa M, et al. Expression of water channel mRNA following cerebral ischemia. Acta Neurochir Suppl. (2000) 76:239–41. doi: 10.1007/978-3-7091-6346-7_48
27. Yamamoto N, Yoneda K, Asai K, Sobue K, Tada T, Fujita Y, et al. Alterations in the expression of the AQP family in cultured rat astrocytes during hypoxia and reoxygenation. Brain Res Mol Brain Res. (2001) 90:26–38. doi: 10.1016/s0169-328x(01)00064-x
28. Hirt L, Fukuda AM, Ambadipudi K, Rashid F, Binder D, Verkman A, et al. Improved long-term outcome after transient cerebral ischemia in aquaporin-4 knockout mice. J Cereb Blood Flow Metab. (2017) 37:277–90. doi: 10.1177/0271678X15623290
29. Ramiro L, Simats A, Penalba A, Garcia-Tornel A, Rovira A, Mancha F, et al. Circulating aquaporin-4 as a biomarker of early neurological improvement in stroke patients: a pilot study. Neurosci Lett. (2020) 714:134580. doi: 10.1016/j.neulet.2019.134580
30. Lien CF, Mohanta SK, Frontczak-Baniewicz M, Swinny JD, Zablocka B, Górecki DC. Absence of glial α-dystrobrevin causes abnormalities of the blood-brain barrier and progressive brain edema. J Biol Chem. (2012) 287:41374–85. doi: 10.1074/jbc.M112.400044
31. Boulay AC, Cisternino S, Cohen-Salmon M. Immunoregulation at the gliovascular unit in the healthy brain: a focus on connexin 43. Brain Behav Immun. (2016) 56:1–9. doi: 10.1016/j.bbi.2015.11.017
32. Marazuela P, Bonaterra-Pastra A, Faura J, Penalba A, Pizarro J, Pancorbo O, et al. Circulating AQP4 levels in patients with cerebral amyloid angiopathy-associated intracerebral hemorrhage. J Clin Med. (2021) 10:989. doi: 10.3390/jcm10050989
33. Morin CM, Drake CL, Harvey AG, Krystal AD, Manber R, Riemann D, et al. Insomnia disorder. Nat Rev Dis Primers. (2015) 1:15026. doi: 10.1038/nrdp.2015.26
34. Pilz LK, Keller LK, Lenssen D, Roenneberg T. Time to rethink sleep quality: PSQI scores reflect sleep quality on workdays. Sleep. (2018) 41:zsy029. doi: 10.1093/sleep/zsy029
35. Hamilton M. A rating scale for depression. J Neurol Neurosurg Psychiatry. (1960) 23:56–62. doi: 10.1136/jnnp.23.1.56
36. Maier W, Buller R, Philipp M, Heuser I. The Hamilton Anxiety Scale: reliability, validity and sensitivity to change in anxiety and depressive disorders. J Affect Disord. (1988) 14:61–8. doi: 10.1016/0165-0327(88)90072-9
37. Berry RB, Budhiraja R, Gottlieb DJ, Gozal D, Iber C, Kapur VK, et al. Rules for scoring respiratory events in sleep: update of the 2007 AASM Manual for the Scoring of Sleep and Associated Events. J Clin Sleep Med. (2012) 8:597–619. doi: 10.5664/jcsm.2172
38. Li X, Jia S, Zhou Z, Jin Y, Zhang X, Hou C, et al. The role of the Montreal Cognitive Assessment (MoCA) and its memory tasks for detecting mild cognitive impairment. Neurol Sci. (2018) 39:1029–34. doi: 10.1007/s10072-018-3319-0
39. Abrahams S, Pickering A, Polkey CE, Morris RG. Spatial memory deficits in patients with unilateral damage to the right hippocampal formation. Neuropsychologia. (1997) 35:11–24. doi: 10.1016/s0028-3932(96)00051-6
40. Chen GH, Xia L, Wang F, Li XW, Jiao CA. Patients with chronic insomnia have selective impairments in memory that are modulated by cortisol. Psychophysiology. (2016) 53:1567–76. doi: 10.1111/psyp.12700
41. Sweetman A, Lack L, McEvoy RD, Smith S, Eckert DJ, Osman A, et al. Bi-directional relationships between co-morbid insomnia and sleep apnea (COMISA). Sleep Med Rev. (2021) 60:101519. doi: 10.1016/j.smrv.2021.101519
42. Olaithe M, Bucks RS, Hillman DR, Eastwood PR. Cognitive deficits in obstructive sleep apnea: insights from a meta-review and comparison with deficits observed in COPD, insomnia, and sleep deprivation. Sleep Med Rev. (2018) 38:39–49. doi: 10.1016/j.smrv.2017.03.005
43. Wang B, Li W, Jin H, Nie X, Shen H, Li E, et al. Curcumin attenuates chronic intermittent hypoxia-induced brain injuries by inhibiting AQP4 and p38 MAPK pathway. Respir Physiol Neurobiol. (2018) 255: 50–7. doi: 10.1016/j.resp.2018.05.006
44. Tewari SG, Parpura V. Astrocytes modulate local field potential rhythm. Front Integr Neurosci. (2016) 9:69. doi: 10.3389/fnint.2015.00069
45. Greene RW, Bjorness TE, Suzuki A. The adenosine-mediated, neuronal-glial, homeostatic sleep response. Curr Opin Neurobiol. (2017) 44:236–42. doi: 10.1016/j.conb.2017.05.015
46. Szabó Z, Héja L, Szalay G, Kékesi O, Füredi A, Szebényi K, et al. Extensive astrocyte synchronization advances neuronal coupling in slow wave activity in vivo. Sci Rep. (2017) 7:6018. doi: 10.1038/s41598-017-06073-7
47. Santello M, Toni N, Volterra A. Astrocyte function from information processing to cognition and cognitive impairment. Nat Neurosci. (2019) 22:154–66. doi: 10.1038/s41593-018-0325-8
48. Fan Y, Liu M, Wu X, Wang F, Ding J, Chen J, et al. Aquaporin-4 promotes memory consolidation in Morris water maze. Brain Struct Funct. (2013) 218:39–50. doi: 10.1007/s00429-011-0373-2
49. Walrave L, Vinken M, Albertini G, De Bundel D, Leybaert L, Smolders IJ. Inhibition of connexin 43 hemichannels impairs Spatial Short-Term Memory without Affecting Spatial Working Memory. Front Cell Neurosci. (2016) 10:288. doi: 10.3389/fncel.2016.00288
50. Pannasch U, Freche D, Dallérac G, Ghézali G, Escartin C, Ezan P, et al. Connexin 30 sets synaptic strength by controlling astroglial synapse invasion. Nat Neurosci. (2014) 17:549–58. doi: 10.1038/nn.3662
51. Arighi A, Di Cristofori A, Fenoglio C, Borsa S, D'Anca M, Fumagalli GG, et al. Cerebrospinal fluid level of aquaporin 4: a new window on glymphatic system involvement in neurodegenerative disease? J Alzheimers Dis. (2019) 69:663–9. doi: 10.3233/JAD-190119
52. Wu Y, Zhong L, Geng J. Neuromyelitis optica spectrum disorder: Pathogenesis, treatment, and experimental models. Mult Scler Relat Disord. (2019) 27:412–8. doi: 10.1016/j.msard.2018.12.002
Keywords: aquaporin-4, astrocyte, cognition, connexin-30, connexin-43, insomnia disorder
Citation: Yang S, Kong X-Y, Hu T, Ge Y-J, Li X-Y, Chen J-T, He S, Zhang P and Chen G-H (2022) Aquaporin-4, Connexin-30, and Connexin-43 as Biomarkers for Decreased Objective Sleep Quality and/or Cognition Dysfunction in Patients With Chronic Insomnia Disorder. Front. Psychiatry 13:856867. doi: 10.3389/fpsyt.2022.856867
Received: 17 January 2022; Accepted: 21 February 2022;
Published: 25 March 2022.
Edited by:
Luigi De Gennaro, Sapienza University of Rome, ItalyReviewed by:
Maria Paola Mogavero, Scientific Clinical Institute Maugeri (ICS Maugeri), ItalyAxel Steiger, Ludwig Maximilian University of Munich, Germany
Copyright © 2022 Yang, Kong, Hu, Ge, Li, Chen, He, Zhang and Chen. This is an open-access article distributed under the terms of the Creative Commons Attribution License (CC BY). The use, distribution or reproduction in other forums is permitted, provided the original author(s) and the copyright owner(s) are credited and that the original publication in this journal is cited, in accordance with accepted academic practice. No use, distribution or reproduction is permitted which does not comply with these terms.
*Correspondence: Gui-Hai Chen, doctorcgh@163.com; Ping Zhang, 932247811@qq.com