- Department of Behavioral Neuroscience, Oregon Health & Science University, Portland, OR, United States
Development of better treatments for alcohol use disorder (AUD) is urgently needed. One promising opportunity for this development is the potential of targeting the oxytocin peptide system. Preclinical studies showed that administration of exogenous oxytocin or, more recently, stimulation of neurons expressing endogenous oxytocin lead to a decreased alcohol consumption across several rodent models. Initial clinical studies also showed that administration of oxytocin decreased craving for alcohol and heavy alcohol drinking. However, several more recent clinical studies were not able to replicate these effects. Thus, although targeting the oxytocin system holds promise for the treatment of AUD, more nuanced approaches toward development and application of these treatments are needed. In this mini-review we discuss potential caveats resulting in differential success of attempts to use oxytocin for modulating alcohol use disorder-related behaviors in clinical studies and evaluate three directions in which targeting the oxytocin system could be improved: (1) increasing potency of exogenously administered oxytocin, (2) developing oxytocin receptor agonists, and (3) stimulating components of the endogenous oxytocin system. Both advances and potential pitfalls of these directions are discussed.
Introduction
Alcohol use disorder (AUD) is a devastating condition where the affected individuals continue to engage in drinking despite the negative experience and the harm caused by drinking. Globally, more than 5% of all deaths have been attributed to alcohol consumption (1). In addition to alcohol-related mortality, AUD is associated with a plethora of debilitating health and societal consequences. Harmful alcohol consumption increased during the COVID-19 pandemic (2–4). Better treatments for AUD with better access to these treatments are urgently needed.
Among potential targets for such treatments, recent attention has focused on the oxytocin (OXT) neuropeptide system. OXT has a leading role in coordinating maternal behaviors, and regulates other functions, including social attachment, anxiety, food consumption, learning, body temperature, neuroinflammation, and pain (5, 6). OXT has been suggested to modulate alcohol-related responses including craving, tolerance, abstinence, and withdrawal-induced anxiety and social engagement (7–10). In turn, alcohol can affect OXT levels and underlying neurocircuitry (11–13). These observations suggested that stimulating the OXT system could curb excessive alcohol use by targeting several phases of the addiction cycle: modulating alcohol's effects during the intoxication phase, decreasing alcohol's toxicity during the abstinence/withdrawal phase and/or decreasing craving during the anticipation/preoccupation phase (14–17). Indeed, OXT administration decreased alcohol self-administration in numerous preclinical studies (16, 18–21). Importantly, OXT was effective in translationally relevant animal models, including being administered via intranasal (IN) routes, in models of severe alcohol dependence (22), in prairie voles, which share similarities in neurocircuitry regulating social behaviors (21), and in presence of untreated peers, similarly to medication administration in outpatient clinics (23, 24). Initial clinical studies also indicated that OXT administration in humans could decrease signs of withdrawal and craving and inhibit excessive alcohol drinking (12, 17, 25). However, more recent studies had limited success (26–28), calling for more nuanced approaches for the treatment of AUD with OXT. Moreover, recent basic science studies revised our understanding of organization of the OXT system (29) and exogenous OXT's penetrance into the brain (30, 31). With these developments, the goal of this minireview is to critically analyze the potential pitfalls associated with clinical studies testing effects of OXT on signs of AUD (Table 1) and to evaluate different directions of overcoming these pitfalls: by increasing potency of exogenously administered OXT, by developing OXT receptor (OXTR) agonists, and by stimulating the endogenous OXT system (Figure 1).
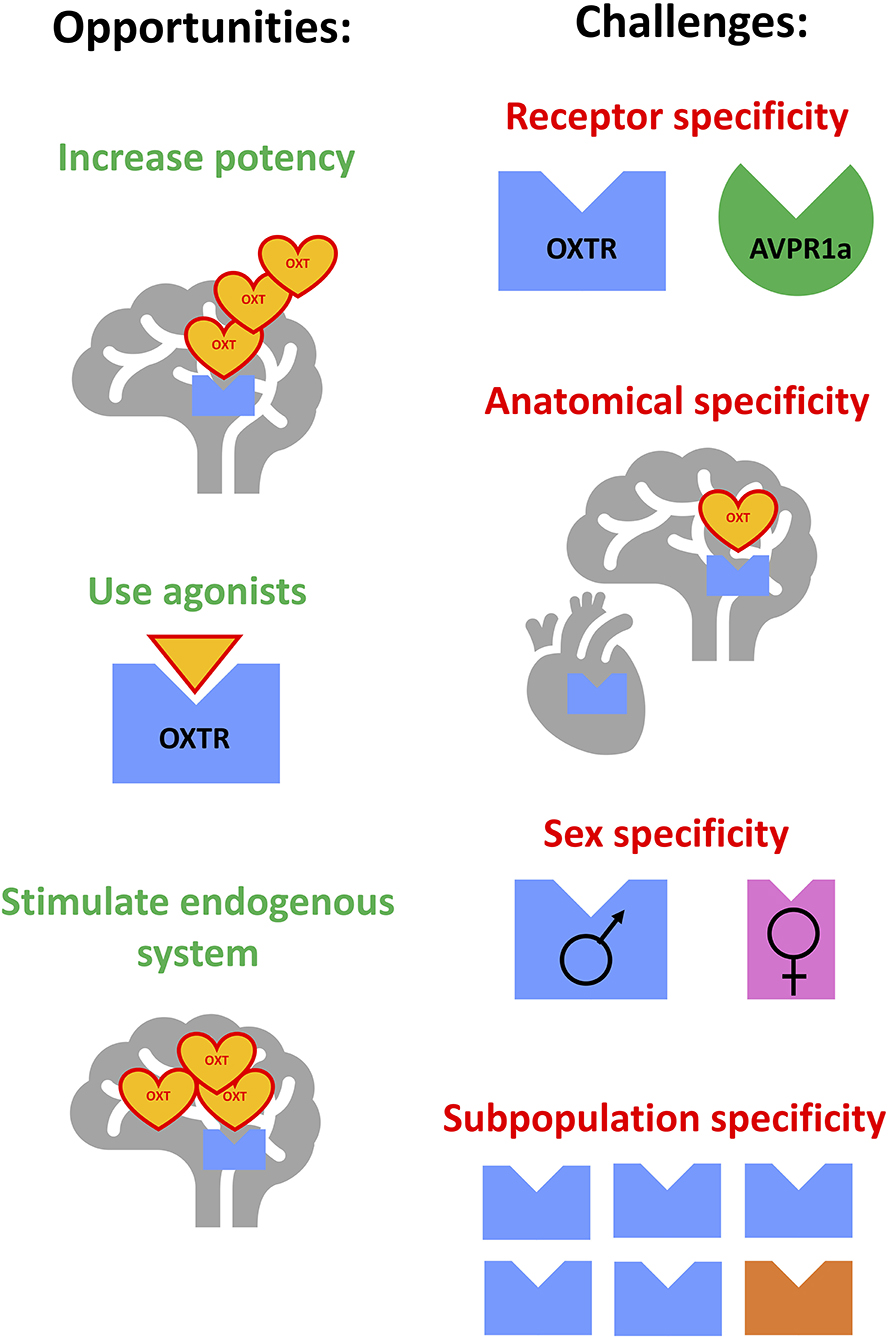
Figure 1. Opportunities and challenges for improving the targeting of the oxytocin system for the treatment of alcohol use disorder. Opportunities: (1) increasing potency of exogenously-administered oxytocin, (2) development and use of oxytocin receptor agonists, (3) stimulating components of the endogenous oxytocin system. Challenges: (1) achieving specificity to oxytocin receptor vs. other related receptors, (2) achieving anatomical specificity (central vs. peripheral, or targeting specific subpopulations of neurons), (3) overcoming or focusing on potential sex-dependent effects, (4) identifying subpopulations sensitive to treatments due to genetic or environment-induced differences.
Clinical Studies on OXT Administration and Motivation to Consume Alcohol
So far, 11 clinical studies have been published on effects of IN OXT on alcohol drinking-related behaviors, and one study on social stress response but not drinking-related behaviors in individuals with AUD (38) (Table 1). These studies assessed effects of OXT treatments ranging from single to 12 week-long daily administrations across populations in three different countries, in subjects characterized as social drinkers, heavy drinkers or patients with post-traumatic stress disorder/AUD comorbidity, with modest sample sizes ranging from 11 to 84. Only half of the studies evaluated effects of OXT in both sexes, and most studies focused on males only. Evaluating Table 1, one can conclude that effectiveness of OXT to decrease relevant outcomes was not overwhelming, revealing the following potential pitfalls.
Insufficient Treatment Period
The majority of studies that looked at short-term outcomes failed to observe decrease in drinking. The only study that reported decreased drinking administered OXT for the longest time (25). Importantly, decreased measures of intake in this study only started to emerge on the second week of treatment. Therefore, the usual duration of OXT treatment and observation in most current studies might be insufficient to show its effect. On the other hand, effects of OXT on measures of heavy drinking in this study appeared to be subsiding in the last weeks of treatment, suggesting either a desensitization to repeated treatment or a “floor effect” of OXT.
Insufficient and Inconsistent Dosage
It appears that more recent studies, showing less consistent effects, used slightly lower doses of OXT per day. One study relied on subjects' self-administration of OXT, which might have not achieved effective uptake (27). It is important to note that in some of the studies, OXT did not decrease measures of alcohol craving or drinking despite having significant effects on measures of stress or anxiety, arguing against insufficient OXT uptake (26, 27). On the other hand, it could be that higher doses are needed to decrease alcohol drinking than doses that can affect stress or anxiety.
Individual Variance in the Population
Another consideration is that OXT could be effective only in a subset of patients. One study indicated that OXT can even increase craving in subjects with low attachment anxiety (32). Thus, subpopulations of subjects can be differentially sensitive to OXT treatment, requiring bigger sample sizes to achieve the power necessary to detect effects of these treatments.
In studies listed in Table 1, five studies observed decreases in craving, alcohol cue response or heavy alcohol use (12, 17, 25, 33, 37), and the other five did not observe significant effects (26–28, 34–36). Since only one study reported that OXT produced opposite from hypothesized effects in a subpopulation of subjects (32), we conservatively interpret this analysis that OXT can be effective in decreasing alcohol craving or intake, but its potency needs to be enhanced.
Enhancing Exogenous OXT's Ability to Decrease Alcohol Drinking
While observations of positive effects of OXT on alcohol drinking in clinical studies are not consistent, this contrasts with a large body of preclinical studies showing effects. Decreased alcohol intake following OXT administration has been observed in mice, rats, and prairie voles. These studies have been previously reviewed (8, 9, 14, 15, 25, 39–41). The success of preclinical studies may offer clues and opportunities to potentiate the effect of OXT treatment in humans.
Non-human studies have explored both peripheral (e.g., subcutaneous, intraperitoneal, and IN) and central routes of administration of OXT to understand its mechanisms of action. Since OXT's half-life in plasma is estimated to be on the order of minutes (42, 43), the observation that peripherally administered OXT had longer effects than expected from its half-life was attributed to the potential feed-forward release of OXT from magnocellular neurons (44, 45).
To overcome the difficulty interpreting results from peripherally administered OXT, some investigators resorted to study its effects following intracranial administration (12, 46, 47). While these studies demonstrated OXT's central modulation of alcohol-related behaviors, they did not clarify how the peptide reaches the relevant intracranial sites. More recent studies indicated that exogenously administered OXT indeed reaches selected brain regions in mice, rats, monkeys and humans (30, 48–52). Since OXT has additional affinity to vasopressin (AVP) receptors, it is worth noting that the exogenously administered and centrally active OXT could also exert its functions via actions that do not involve OXTRs (29, 53, 54).
The IN route results in penetration of a portion of total administered OXT to the brain via several pathways: transmission along the olfactory and trigeminal neural bundles, absorption from basal epithelia directly into cerebrospinal fluid, crossing the blood-brain barrier, and through circumventricular organs (6, 52). The different relative size of the neural bundles vs. whole brain across species might contribute to differences in the access to specific brain regions in rodents vs. humans. Nevertheless, attempts to increase penetration of IN OXT are underway, including the use of mucoadhesives, nanoemulsions and liposomal formulations (6, 55). Another development is the recent recognition that brain penetrance of OXT is aided by the participation of the Receptor for Advanced Glycation End-products (RAGE) (31, 56). Development of allosteric modulators of RAGE could be an interesting approach to enhance the potency of exogenous OXT.
Finally, as mentioned, OXT could be differentially effective across different populations of subjects. OXT was found to differentially affect alcohol craving in subjects with low and high attachment anxiety (32). Several indicators suggest that it could be more effective in males vs. females (57). Moreover, subjects carrying polymorphisms in OXTR and OXT show differences in alcohol consumption patterns (58, 59). Carriers of these polymorphisms could be differentially sensitive to OXT treatments. Studies targeting these subpopulations could be a step toward development of individualized AUD treatments.
Development of More Potent and Efficacious OXTR Agonists
OXT's lack of stability, low brain penetrance and non-specific effects on AVP receptors prompted researchers to consider developing OXTR agonists (14, 60). The majority of developed and tested OXTR agonist are peptides, with presumably minimal penetrance of the blood-brain barrier. Because of this, peptide-based OXTR agonist PF-06655075 was used to test whether effects from manipulating the OXT system are through central or peripheral OXTRs (22). This is an important consideration as stimulation of peripheral OXTR can affect certain behaviors, for example fear conditioning (61). However, central, but not peripheral administration of PF-06655075 decreased alcohol drinking in dependent rats, indicating that stimulation of the central OXT system is crucial for moderating alcohol consumption (22).
Carbetocin is a highly stable OXT analog used in clinics to prevent postpartum hemorrhage. It has been demonstrated to modulate central effects of morphine (62, 63). In relation to alcohol, however, reported effects are mixed. While the same dose of repeated carbetocin inhibited acquisition, enhanced extinction and suppressed reinstatement of ethanol-induced conditioned place preference in a mouse study (64), it enhanced ethanol-induced conditioned place preference in another study (65). We are not aware of any reports on carbetocin's effects on alcohol drinking. Other peptide OXTR agonists include Lipo-oxytocin-1 and [Thr4,Gly7]OXT (66– 68). Their effect on alcohol-related behaviors have not been reported, but the strong possibility of effects on various peripheral systems could be a matter of concern.
Another possibility is to explore the effects of OXT metabolites—OXT fragments composed of shorter chained peptides. As smaller molecules, they should have better penetrance into the brain and, therefore, higher potency. In contrast to carbetocin, OXT (4–9) improved social preference in the BALBc/J model of autism spectrum disorder-like social deficits (69). In early studies, the tripeptide OXT (6–9) was shown to attenuate tolerance to hypothermic effects of repeated ethanol injections in mice (70). However, whether OXT metabolites can affect alcohol drinking or alcohol reward is still to be discovered.
Development of non-peptide OXTR agonists continues to progress. Three small-molecule agonists have been reported (14, 60, 71, 72). TC-OT-39 has an EC50 of ~100 nM at OXTR as an agonist, but also a moderate affinity at V1a AVP receptors as an antagonist. Therefore, its neurochemical mode of action in vivo is difficult to estimate. In any case, it was not effective in studies of BALBc/J model of autism spectrum disorder-like social deficits (69). Comparing to TC-OT-39, WAY-267464 is a level of magnitude more potent OXTR agonist, but with a similar affinity to V1a AVP receptors (71), again making it difficult to evaluate specificity of its effects after systemic administration. Promisingly, however, administration of this compound into amygdala facilitated extinction of fear conditioning (73) whereas systemic injection of this compound rescued not only social deficits in Shank3b mutant mice (74), but also social deficits caused by adolescent alcohol exposure in rats (75).
LIT-001, the most recently developed small molecule, has an agonist activity on OXTR similar to that of WAY-267464 in in vitro studies, but several magnitudes lower affinity to AVP receptors, for the first time allowing receptor-specific systemic pharmacology of OXTR (71). Systemic administration of LIT-001 rescued deficits in social interaction in the Oprm1 knockout model of autism spectrum disorder (71) and inhibited inflammation-induced hyperalgesia in rats (76). Studies testing LIT-001 in models of AUD are underway.
Development of small-molecule OXTR agonists is a promising avenue for future research. Though striving to develop compounds with more specific and potent effects than OXT, one has to keep in mind the potential side effects. For example, a compound that has potent anxiolytic and analgesic properties must be evaluated for its own potential addictive properties.
Harnessing the Endogenous OXT System
OXT is an evolutionary old peptide. Therefore, its mixed affinities toward OXTR and AVP receptors, as well as its poor brain-penetrance, could have advantages for fitness. It might be, therefore, more prudent to stimulate the endogenous OXT system to produce beneficial effects instead of manipulating the system exogenously. As a proof of this concept, a recent study by King et al. used a chemogenetic approach to activate OXT neurons of the mouse paraventricular nucleus of hypothalamus (PVN) (77). This activation significantly decreased alcohol consumption in a mouse model of binge drinking. This finding implicates the need to explore the feasibility to develop effective means of stimulating the endogenous central OXT system for AUD treatment.
The importance of OXT in regulating social attachments is well-demonstrated across vertebrate species (78–83). Positive social interactions are known to stimulate OXT neurons (84–87). Therefore, social interactions could stimulate the OXT system and hence, decrease alcohol consumption. However, the relationship between social interaction and activity of OXT system is more complex. OXT neurons are also activated during social isolation and after exposure to other stressors (88–90). Stressful experiences are well-known to increase alcohol consumption (7, 91, 92). Therefore, it would be more relevant to selectively stimulate OXT system in a way that mimics the effect of positive social interactions to regulate AUD-related phenotypes.
The latter task is extremely difficult because there are currently no methods allowing to evaluate when specific components of the central OXT system are activated in humans. Our understanding of the relationship between the activation of subpopulations of OXT neurons and OXT's peripheral levels continues to change, and knowledge of projections sites of parvocellular and magnocellular OXT neurons are being revised (29). Additionally, it is difficult to estimate the relationship between levels of OXT in plasma vs. levels in the brain. A meta-analysis across 17 studies found no significant associations between peripheral and central—in either cerebrospinal fluid or specific brain regions—OXT levels under basal conditions in both humans and non-human animals (93). On the other hand, this meta-analysis confirmed significant positive associations between these levels after IN OXT in humans and rodents (49, 94, 95) and after experimental stress in rodents (93, 96–98), indicating that correspondence in OXT levels can be established. Recent studies also complicate the interpretation of previous studies showing that alcohol consumption decreases OXT levels (9). For instance, while one study showed significantly higher OXT levels in blood of patients with AUD (99), another study found significantly lower OXT levels in AUD patients (100). The reason for such opposite results needs to be further studied.
Taken together, stimulation of endogenous OXT system could contribute to treatments of AUD by modulating social relationships or adherence to therapy. This potential could be especially relevant to group-based approaches to treatment. However, direct behavioral manipulation of this system to moderate excessive alcohol intake in AUD patients at this point is still a theoretical goal. Its realization relies on better understanding of the OXT system.
Conclusions
While recent clinical studies show mixed results in the effectiveness of IN OXT to decrease excessive drinking, more sophisticated approaches targeting the OXT system are needed. One possibility is to enhance access of exogenously administered OXT to relevant brain regions either through development of better delivery systems or through enhancement of transporters. Another possibility is to further explore the development of OXTR agonists. The potential of behavioral stimulation of parts of the endogenous OXT system should also be investigated. The better we understand the organization of the OXT system, the greater chance we have of harnessing it for the treatment of AUD.
Author Contributions
AR and YZ conceptualized and wrote the manuscript. All authors contributed to the article and approved the submitted version.
Funding
This work was supported by NIH R01 grants (AA019793, AA025024, and AA028680) to AR.
Conflict of Interest
The authors declare that the research was conducted in the absence of any commercial or financial relationships that could be construed as a potential conflict of interest.
Publisher's Note
All claims expressed in this article are solely those of the authors and do not necessarily represent those of their affiliated organizations, or those of the publisher, the editors and the reviewers. Any product that may be evaluated in this article, or claim that may be made by its manufacturer, is not guaranteed or endorsed by the publisher.
References
1. World Health Organization. Global Status Report on Alcohol and Health 2018. World Health Organization (2018). Available online at: https://apps.who.int/iris/handle/10665/274603
2. Acuff SF, Strickland JC, Tucker JA, Murphy JG. Changes in alcohol use during COVID-19 and associations with contextual and individual difference variables: a systematic review and meta-analysis. Psychol Addict Behav. (2021) 36:1–19. doi: 10.1037/adb0000796
3. Gonzalez-Monroy C, Gomez-Gomez I, Olarte-Sanchez CM, Motrico E. Eating behaviour changes during the COVID-19 pandemic: a systematic review of longitudinal studies. Int J Environ Res Public Health. (2021) 18:11130. doi: 10.3390/ijerph182111130
4. Vogel EA, Chieng A, Robinson A, Pajarito S, Prochaska JJ. Associations between substance use problems and stress during COVID-19. J Stud Alcohol Drugs. (2021) 82:776–81. doi: 10.15288/jsad.2021.82.776
5. Carter CS, Kenkel WM, MacLean EL, Wilson SR, Perkeybile AM, Yee JR, et al. Is oxytocin “nature's medicine”? Pharmacol Rev. (2020) 72:829–61. doi: 10.1124/pr.120.019398
6. Grinevich V, Neumann ID. Brain oxytocin: how puzzle stones from animal studies translate into psychiatry. Mol Psychiatry. (2021) 26:265–79. doi: 10.1038/s41380-020-0802-9
7. Koob GF. Drug addiction: hyperkatifeia/negative reinforcement as a framework for medications development. Pharmacol Rev. (2021) 73:163–201. doi: 10.1124/pharmrev.120.000083
8. Lee MR, Weerts EM. Oxytocin for the treatment of drug and alcohol use disorders. Behav Pharmacol. (2016) 27:640–8. doi: 10.1097/FBP.0000000000000258
9. Ryabinin AE, Fulenwider HD. Alcohol and oxytocin: scrutinizing the relationship. Neurosci Biobehav Rev. (2021) 127:852–64. doi: 10.1016/j.neubiorev.2021.06.009
10. Szabo G, Kovacs GL, Szekeli S, Telegdy G. The effects of neurohypophyseal hormones on tolerance to the hypothermic effect of ethanol. Alcohol. (1985) 2:567–74. doi: 10.1016/0741-8329(85)90082-5
11. Fuchs AR. The inhibitory effect of ethanol on the release of oxytocin during parturition in the rabbit. J Endocrinol. (1966) 35:125–34. doi: 10.1677/joe.0.0350125
12. Hansson AC, Koopmann A, Uhrig S, Buhler S, Domi E, Kiessling E, et al. Oxytocin reduces alcohol cue-reactivity in alcohol-dependent rats and humans. Neuropsychopharmacology. (2018) 43:1235–46. doi: 10.1038/npp.2017.257
13. Stevenson JR, Young KA, Bohidar AE, Francomacaro LM, Fasold TR, Buirkle JM, et al. Alcohol consumption decreases oxytocin neurons in the anterior paraventricular nucleus of the hypothalamus in prairie voles. Alcohol Clin Exp Res. (2017) 41:1444–51. doi: 10.1111/acer.13430
14. Che X, Cai J, Liu Y, Xu T, Yang J, Wu C. Oxytocin signaling in the treatment of drug addiction: therapeutic opportunities and challenges. Pharmacol Ther. (2021) 223:107820. doi: 10.1016/j.pharmthera.2021.107820
15. Leong KC, Cox S, King C, Becker H, Reichel CM. Oxytocin and rodent models of addiction. Int Rev Neurobiol. (2018) 140:201–47. doi: 10.1016/bs.irn.2018.07.007
16. McGregor IS, Bowen MT. Breaking the loop: oxytocin as a potential treatment for drug addiction. Horm Behav. (2012) 61:331–9. doi: 10.1016/j.yhbeh.2011.12.001
17. Pedersen CA, Smedley KL, Leserman J, Jarskog LF, Rau SW, Kampov-Polevoi A, et al. Intranasal oxytocin blocks alcohol withdrawal in human subjects. Alcohol Clin Exp Res. (2013) 37:484–9. doi: 10.1111/j.1530-0277.2012.01958.x
18. King CE, Griffin WC, Luderman LN, Kates MM, McGinty JF, Becker HC. Oxytocin reduces ethanol self-administration in mice. Alcohol Clin Exp Res. (2017) 41:955–64. doi: 10.1111/acer.13359
19. MacFadyen K, Loveless R, DeLucca B, Wardley K, Deogan S, Thomas C, et al. Peripheral oxytocin administration reduces ethanol consumption in rats. Pharmacol Biochem Behav. (2016) 140:27–32. doi: 10.1016/j.pbb.2015.10.014
20. Peters S, Slattery DA, Flor PJ, Neumann ID, Reber SO. Differential effects of baclofen and oxytocin on the increased ethanol consumption following chronic psychosocial stress in mice. Addict Biol. (2013) 18:66–77. doi: 10.1111/adb.12001
21. Stevenson JR, Wenner SM, Freestone DM, Romaine CC, Parian MC, Christian SM, et al. Oxytocin reduces alcohol consumption in prairie voles. Physiol Behav. (2017) 179:411–21. doi: 10.1016/j.physbeh.2017.07.021
22. Tunstall BJ, Kirson D, Zallar LJ, McConnell SA, Vendruscolo JCM, Ho CP, et al. Oxytocin blocks enhanced motivation for alcohol in alcohol dependence and blocks alcohol effects on GABAergic transmission in the central amygdala. PLoS Biol. (2019) 17:e2006421. doi: 10.1371/journal.pbio.2006421
23. Caruso MA, Robins MT, Fulenwider HD, Ryabinin AE. Temporal analysis of individual ethanol consumption in socially housed mice and the effects of oxytocin. Psychopharmacology. (2021) 238:899–911. doi: 10.1007/s00213-020-05741-3
24. Walcott AT, Ryabinin AE. Assessing effects of oxytocin on alcohol consumption in socially housed prairie voles using radio frequency tracking. Addict Biol. (2021) 26:e12893. doi: 10.1111/adb.12893
25. Pedersen CA. Oxytocin, tolerance, and the dark side of addiction. Int Rev Neurobiol. (2017) 136:239–74. doi: 10.1016/bs.irn.2017.08.003
26. Flanagan JC, Allan NP, Calhoun CD, Badour CL, Moran-Santa Maria M, Brady KT, et al. Effects of oxytocin on stress reactivity and craving in veterans with co-occurring PTSD and alcohol use disorder. Exp Clin Psychopharmacol. (2019) 27:45–54. doi: 10.1037/pha0000232
27. Melby K, Grawe RW, Aamo TO, Skovlund E, Spigset O. Efficacy of self-administered intranasal oxytocin on alcohol use and craving after detoxification in patients with alcohol dependence. A double-blind placebo-controlled trial. Alcohol Alcohol. (2021) 56:565–72. doi: 10.1093/alcalc/agaa133
28. Vena A, King A, Lee R, de Wit H. Intranasal oxytocin does not modulate responses to alcohol in social drinkers. Alcohol Clin Exp Res. (2018) 42:1725–34. doi: 10.1111/acer.13814
29. Grinevich V, Ludwig M. The multiple faces of the oxytocin and vasopressin systems in the brain J Neuroendocrinol. (2021) 2021:13004. doi: 10.1111/jne.13004
30. Lee MR, Shnitko TA, Blue SW, Kaucher AV, Winchell AJ, Erikson DW, et al. Labeled oxytocin administered via the intranasal route reaches the brain in rhesus macaques. Nat Commun. (2020) 11:2783. doi: 10.1038/s41467-020-15942-1
31. Yamamoto Y, Higashida H. RAGE regulates oxytocin transport into the brain. Commun Biol. (2020) 3:70. doi: 10.1038/s42003-020-0799-2
32. Mitchell JM, Arcuni PA, Weinstein D, Woolley JD. Intranasal oxytocin selectively modulates social perception, craving, and approach behavior in subjects with alcohol use disorder. J Addict Med. (2016) 10:182–9. doi: 10.1097/ADM.0000000000000213
33. Bach P, Reinhard I, Buhler S, Vollstadt-Klein S, Kiefer F, Koopmann A. Oxytocin modulates alcohol-cue induced functional connectivity in the nucleus accumbens of social drinkers. Psychoneuroendocrinology. (2019) 109:104385. doi: 10.1016/j.psyneuen.2019.104385
34. Stauffer CS, Moschetto JM, McKernan SM, Hsiang E, Borsari B, Woolley JD. Oxytocin-enhanced motivational interviewing group therapy for methamphetamine use disorder in men who have sex with men: study protocol for a randomized controlled trial. Trials. (2019) 20:145. doi: 10.1186/s13063-019-3225-7
35. Melby K, Grawe RW, Aamo TO, Salvesen O, Spigset O. Effect of intranasal oxytocin on alcohol withdrawal syndrome: a randomized placebo-controlled double-blind clinical trial. Drug Alcohol Depend. (2019) 197:95–101. doi: 10.1016/j.drugalcdep.2019.01.003
36. Melby K, Fasmer OB, Henriksen TE, Grawe RW, Aamo TO, Spigset O. Actigraphy assessment of motor activity and sleep in patients with alcohol withdrawal syndrome and the effects of intranasal oxytocin. PLoS ONE. (2020) 15:e0228700. doi: 10.1371/journal.pone.0228700
37. Bach P, Koopmann A, Bumb JM, Zimmermann S, Buhler S, Reinhard I, et al. Oxytocin attenuates neural response to emotional faces in social drinkers: an fMRI study. Eur Arch Psychiatry Clin Neurosci. (2020) 271:873–82. doi: 10.1007/s00406-020-01115-0
38. Melkonian AJ, Flanagan JC, Calhoun CD, Hogan JN, Back SE. Craving moderates the effects of intranasal oxytocin on anger in response to social stress among veterans with co-occurring posttraumatic stress disorder and alcohol use disorder. J Clin Psychopharmacol. (2021) 41:465–9. doi: 10.1097/JCP.0000000000001434
39. Bowen MT, Neumann ID. Rebalancing the addicted brain: oxytocin interference with the neural substrates of addiction. Trends Neurosci. (2017) 40:691–708. doi: 10.1016/j.tins.2017.10.003
40. Ferrer-Perez C, Reguilon MD, Minarro J, Rodriguez-Arias M. Oxytocin signaling as a target to block social defeat-induced increases in drug abuse reward. Int J Mol Sci. (2021) 22:2372. doi: 10.3390/ijms22052372
41. Sanna F, De Luca MA. The potential role of oxytocin in addiction: what is the target process? Curr Opin Pharmacol. (2021) 58:8–20. doi: 10.1016/j.coph.2021.03.002
42. Mens WB, Witter A, van Wimersma Greidanus TB. Penetration of neurohypophyseal hormones from plasma into cerebrospinal fluid (CSF): half-times of disappearance of these neuropeptides from CSF. Brain Res. (1983) 262:143–9. doi: 10.1016/0006-8993(83)90478-X
43. Ryden G, Sjoholm I. Half-life of oxytoxin in blood of pregnant and non-pregnant woman. Acta Obstet Gynecol Scand. (1969) 48:139–40. doi: 10.3109/00016346909157733
44. Bowen MT, Carson DS, Spiro A, Arnold JC, McGregor IS. Adolescent oxytocin exposure causes persistent reductions in anxiety and alcohol consumption and enhances sociability in rats. PLoS ONE. (2011) 6:e27237. doi: 10.1371/journal.pone.0027237
45. Richard P, Moos F, Freund-Mercier MJ. Central effects of oxytocin. Physiol Rev. (1991) 71:331–70. doi: 10.1152/physrev.1991.71.2.331
46. King CE, Becker HC. Oxytocin attenuates stress-induced reinstatement of alcohol seeking behavior in male and female mice. Psychopharmacology. (2019) 236:2613–22. doi: 10.1007/s00213-019-05233-z
47. Peters ST, Bowen MT, Bohrer K, McGregor IS, Neumann ID. Oxytocin inhibits ethanol consumption and ethanol-induced dopamine release in the nucleus accumbens. Addict Biol. (2017) 22:702–11. doi: 10.1111/adb.12362
48. Lee MR, Scheidweiler KB, Diao XX, Akhlaghi F, Cummins A, Huestis MA, et al. Oxytocin by intranasal and intravenous routes reaches the cerebrospinal fluid in rhesus macaques: determination using a novel oxytocin assay. Mol Psychiatry. (2018) 23:115–22. doi: 10.1038/mp.2017.27
49. Neumann ID, Maloumby R, Beiderbeck DI, Lukas M, Landgraf R. Increased brain and plasma oxytocin after nasal and peripheral administration in rats and mice. Psychoneuroendocrinology. (2013) 38:1985–93. doi: 10.1016/j.psyneuen.2013.03.003
50. Paloyelis Y, Doyle OM, Zelaya FO, Maltezos S, Williams SC, Fotopoulou A, et al. A spatiotemporal profile of in vivo cerebral blood flow changes following intranasal oxytocin in humans. Biol Psychiatry. (2016) 79:693–705. doi: 10.1016/j.biopsych.2014.10.005
51. Smith AS, Korgan AC, Young WS. Oxytocin delivered nasally or intraperitoneally reaches the brain and plasma of normal and oxytocin knockout mice. Pharmacol Res. (2019) 146:104324. doi: 10.1016/j.phrs.2019.104324
52. Yeomans DC, Hanson LR, Carson DS, Tunstall BJ, Lee MR, Tzabazis AZ, et al. Nasal oxytocin for the treatment of psychiatric disorders and pain: achieving meaningful brain concentrations. Transl Psychiatry. (2021) 11:388. doi: 10.1038/s41398-021-01511-7
53. Bowen MT, Peters ST, Absalom N, Chebib M, Neumann ID, McGregor IS. Oxytocin prevents ethanol actions at delta subunit-containing GABAA receptors and attenuates ethanol-induced motor impairment in rats. Proc Natl Acad Sci USA. (2015) 112:3104–9. doi: 10.1073/pnas.1416900112
54. Freeman SM, Young LJ. Comparative perspectives on oxytocin and vasopressin receptor research in rodents and primates: translational implications. J Neuroendocrinol. (2016) 28. doi: 10.1111/jne.12382
55. Bharadwaj VN, Tzabazis AZ, Klukinov M, Manering NA, Yeomans DC. Intranasal administration for pain: oxytocin and other polypeptides. Pharmaceutics. (2021) 13:1088. doi: 10.3390/pharmaceutics13071088
56. Yamamoto Y, Liang M, Munesue S, Deguchi K, Harashima A, Furuhara K, et al. Vascular RAGE transports oxytocin into the brain to elicit its maternal bonding behaviour in mice. Commun Biol. (2019) 2:76. doi: 10.1038/s42003-019-0325-6
57. Hansson AC, Spanagel R. No changes in the oxytocin system in alcohol-dependent female rodents and humans: towards a sex-specific psychopharmacology in alcoholism. Addict Biol. (2021) 26:e12945. doi: 10.1111/adb.12945
58. Vaht M, Kurrikoff T, Laas K, Veidebaum T, Harro J. Oxytocin receptor gene variation rs53576 and alcohol abuse in a longitudinal population representative study. Psychoneuroendocrinology. (2016) 74:333–41. doi: 10.1016/j.psyneuen.2016.09.018
59. Yang L, Wang F, Wang M, Han M, Hu L, Zheng M, et al. Association between oxytocin and receptor genetic polymorphisms and aggression in a northern Chinese Han population with alcohol dependence. Neurosci Lett. (2017) 636:140–4. doi: 10.1016/j.neulet.2016.10.066
60. Cid-Jofre V, Moreno M, Reyes-Parada M, Renard GM. Role of oxytocin and vasopressin in neuropsychiatric disorders: therapeutic potential of agonists and antagonists. Int J Mol Sci. (2021) 22:12077. doi: 10.3390/ijms222112077
61. Modi ME, Majchrzak MJ, Fonseca KR, Doran A, Osgood S, Vanase-Frawley M, et al. Peripheral administration of a long-acting peptide oxytocin receptor agonist inhibits fear-induced freezing. J Pharmacol Exp Ther. (2016) 358:164–72. doi: 10.1124/jpet.116.232702
62. Georgiou P, Zanos P, Garcia-Carmona JA, Hourani S, Kitchen I, Kieffer BL, et al. The oxytocin analogue carbetocin prevents priming-induced reinstatement of morphine-seeking: involvement of dopaminergic, noradrenergic and MOPr systems. Eur Neuropsychopharmacol. (2015) 25:2459–64. doi: 10.1016/j.euroneuro.2015.09.015
63. Zanos P, Georgiou P, Wright SR, Hourani SM, Kitchen I, Winsky-Sommerer R, et al. The oxytocin analogue carbetocin prevents emotional impairment and stress-induced reinstatement of opioid-seeking in morphine-abstinent mice. Neuropsychopharmacology. (2014) 39:855–65. doi: 10.1038/npp.2013.285
64. Bahi A. The oxytocin receptor impairs ethanol reward in mice. Physiol Behav. (2015) 139:321–7. doi: 10.1016/j.physbeh.2014.11.046
65. Rae M, Zanos P, Georgiou P, Chivers P, Bailey A, Camarini R. Environmental enrichment enhances conditioned place preference to ethanol via an oxytocinergic-dependent mechanism in male mice. Neuropharmacology. (2018) 138:267–74. doi: 10.1016/j.neuropharm.2018.06.013
66. Busnelli M, Bulgheroni E, Manning M, Kleinau G, Chini B. Selective and potent agonists and antagonists for investigating the role of mouse oxytocin receptors. J Pharmacol Exp Ther. (2013) 346:318–27. doi: 10.1124/jpet.113.202994
67. Cherepanov SM, Akther S, Nishimura T, Shabalova AA, Mizuno A, Ichinose W, et al. Effects of three lipidated oxytocin analogs on behavioral deficits in CD38 knockout mice. Brain Sci. (2017) 7:132. doi: 10.3390/brainsci7100132
68. Sala M, Braida D, Donzelli A, Martucci R, Busnelli M, Bulgheroni E, et al. Mice heterozygous for the oxytocin receptor gene (Oxtr(+/-)) show impaired social behaviour but not increased aggression or cognitive inflexibility: evidence of a selective haploinsufficiency gene effect. J Neuroendocrinol. (2013) 25:107–18. doi: 10.1111/j.1365-2826.2012.02385.x
69. Moy SS, Teng BL, Nikolova VD, Riddick NV, Simpson CD, Van Deusen A, et al. Prosocial effects of an oxytocin metabolite, but not synthetic oxytocin receptor agonists, in a mouse model of autism. Neuropharmacology. (2019) 144:301–11. doi: 10.1016/j.neuropharm.2018.10.036
70. Szabo G, Kovacs GL, Szekeli S, Balaspiri L, Telegdy G. C-terminal fragments of oxytocin (prolyl-leucyl-glycinamide and Z-prolyl-D-leucine) attenuate the development of tolerance to ethanol. Acta Physiol Hung. (1987) 69:115–22.
71. Frantz MC, Pellissier LP, Pflimlin E, Loison S, Gandia J, Marsol C, et al. LIT-001, the first nonpeptide oxytocin receptor agonist that improves social interaction in a mouse model of autism. J Med Chem. (2018) 61:8670–92. doi: 10.1021/acs.jmedchem.8b00697
72. Pitt GR, Batt AR, Haigh RM, Penson AM, Robson PA, Rooker DP, et al. Non-peptide oxytocin agonists. Bioorg Med Chem Lett. (2004) 14:4585–9. doi: 10.1016/j.bmcl.2004.04.107
73. Lahoud N, Maroun M. Oxytocinergic manipulations in corticolimbic circuit differentially affect fear acquisition and extinction. Psychoneuroendocrinology. (2013) 38:2184–95. doi: 10.1016/j.psyneuen.2013.04.006
74. Resendez SL, Namboodiri VMK, Otis JM, Eckman LEH, Rodriguez-Romaguera J, Ung RL, et al. Social stimuli induce activation of oxytocin neurons within the paraventricular nucleus of the hypothalamus to promote social behavior in male mice. J Neurosci. (2020) 40:2282–95. doi: 10.1523/JNEUROSCI.1515-18.2020
75. Dannenhoffer CA, Kim EU, Saalfield J, Werner DF, Varlinskaya EI, Spear LP. Oxytocin and vasopressin modulation of social anxiety following adolescent intermittent ethanol exposure. Psychopharmacology. (2018) 235:3065–77. doi: 10.1007/s00213-018-5003-8
76. Hilfiger L, Zhao Q, Kerspern D, Inquimbert P, Andry V, Goumon Y, et al. A nonpeptide oxytocin receptor agonist for a durable relief of inflammatory pain. Sci Rep. (2020) 10:3017. doi: 10.1038/s41598-020-59929-w
77. King CE, Griffin WC, Lopez MF, Becker HC. Activation of hypothalamic oxytocin neurons reduces binge-like alcohol drinking through signaling at central oxytocin receptors. Neuropsychopharmacology. (2021) 46:1950–7. doi: 10.1038/s41386-021-01046-x
78. Anacker AM, Beery AK. Life in groups: the roles of oxytocin in mammalian sociality. Front Behav Neurosci. (2013) 7:185. doi: 10.3389/fnbeh.2013.00185
79. Bales KL, Arias Del Razo R, Conklin QA, Hartman S, Mayer HS, Rogers FD, et al. Titi monkeys as a novel non-human primate model for the neurobiology of pair bonding. Yale J Biol Med. (2017) 90:373–87.
80. Bosch OJ, Young LJ. Oxytocin and social relationships: from attachment to bond disruption. Curr Top Behav Neurosci. (2018) 35:97–117. doi: 10.1007/7854_2017_10
81. Donaldson ZR, Young LJ. Oxytocin, vasopressin, and the neurogenetics of sociality. Science. (2008) 322:900–4. doi: 10.1126/science.1158668
82. Goodson JL, Kelly AM, Kingsbury MA. Evolving nonapeptide mechanisms of gregariousness and social diversity in birds. Horm Behav. (2012) 61:239–50. doi: 10.1016/j.yhbeh.2012.01.005
83. Young LJ, Wang Z. The neurobiology of pair bonding. Nat Neurosci. (2004) 7:1048–54. doi: 10.1038/nn1327
84. Bosch OJ, Dabrowska J, Modi ME, Johnson ZV, Keebaugh AC, Barrett CE, et al. Oxytocin in the nucleus accumbens shell reverses CRFR2-evoked passive stress-coping after partner loss in monogamous male prairie voles. Psychoneuroendocrinology. (2016) 64:66–78. doi: 10.1016/j.psyneuen.2015.11.011
85. Cushing BS, Yamamoto Y, Hoffman GE, Carter CS. Central expression of c-Fos in neonatal male and female prairie voles in response to treatment with oxytocin. Brain Res Dev Brain Res. (2003) 143:129–36. doi: 10.1016/S0165-3806(03)00105-6
86. Thompson MR, Callaghan PD, Hunt GE, Cornish JL, McGregor IS. A role for oxytocin and 5-HT(1A) receptors in the prosocial effects of 3,4 methylenedioxymethamphetamine (“ecstasy”). Neuroscience. (2007) 146:509–14. doi: 10.1016/j.neuroscience.2007.02.032
87. Uvnas-Moberg K. Physiological and endocrine effects of social contact. Ann NY Acad Sci. (1997) 807:146–63. doi: 10.1111/j.1749-6632.1997.tb51917.x
88. Grippo AJ, Gerena D, Huang J, Kumar N, Shah M, Ughreja R, et al. Social isolation induces behavioral and neuroendocrine disturbances relevant to depression in female and male prairie voles. Psychoneuroendocrinology. (2007) 32:966–80. doi: 10.1016/j.psyneuen.2007.07.004
89. Pan Y, Liu Y, Young KA, Zhang Z, Wang Z. Post-weaning social isolation alters anxiety-related behavior and neurochemical gene expression in the brain of male prairie voles. Neurosci Lett. (2009) 454:67–71. doi: 10.1016/j.neulet.2009.02.064
90. Torner L, Plotsky PM, Neumann ID, de Jong TR. Forced swimming-induced oxytocin release into blood and brain: effects of adrenalectomy and corticosterone treatment. Psychoneuroendocrinology. (2017) 77:165–74. doi: 10.1016/j.psyneuen.2016.12.006
91. Becker HC. Influence of stress associated with chronic alcohol exposure on drinking. Neuropharmacology. (2017) 122:115–26. doi: 10.1016/j.neuropharm.2017.04.028
92. Pohorecky LA. Interaction of ethanol and stress: research with experimental animals–an update. Alcohol Alcohol. (1990) 25:263–76. doi: 10.1093/oxfordjournals.alcalc.a045000
93. Valstad M, Alvares GA, Egknud M, Matziorinis AM, Andreassen OA, Westlye LT, et al. The correlation between central and peripheral oxytocin concentrations: a systematic review and meta-analysis. Neurosci Biobehav Rev. (2017) 78:117–24. doi: 10.1016/j.neubiorev.2017.04.017
94. Striepens N, Kendrick KM, Hanking V, Landgraf R, Wullner U, Maier W, et al. Elevated cerebrospinal fluid and blood concentrations of oxytocin following its intranasal administration in humans. Sci Rep. (2013) 3:3440. doi: 10.1038/srep03440
95. Wang YL, Yuan Y, Yang J, Wang CH, Pan YJ, Lu L, et al. The interaction between the oxytocin and pain modulation in headache patients. Neuropeptides. (2013) 47:93–7. doi: 10.1016/j.npep.2012.12.003
96. Engelmann M, Bull PM, Brown CH, Landgraf R, Horn TF, Singewald N, et al. GABA selectively controls the secretory activity of oxytocin neurons in the rat supraoptic nucleus. Eur J Neurosci. (2004) 19:601–8. doi: 10.1111/j.1460-9568.2004.03151.x
97. Kojima S, Stewart RA, Demas GE, Alberts JR. Maternal contact differentially modulates central and peripheral oxytocin in rat pups during a brief regime of mother-pup interaction that induces a filial huddling preference. J Neuroendocrinol. (2012) 24:831–40. doi: 10.1111/j.1365-2826.2012.02280.x
98. Williams SK, Barber JS, Jamieson-Drake AW, Enns JA, Townsend LB, Walker CH, et al. Chronic cocaine exposure during pregnancy increases postpartum neuroendocrine stress responses. J Neuroendocrinol. (2012) 24:701–11. doi: 10.1111/j.1365-2826.2012.02291.x
99. Lenz B, Weinland C, Bach P, Kiefer F, Grinevich V, Zoicas I, et al. Oxytocin blood concentrations in alcohol use disorder: a cross-sectional, longitudinal, and sex-separated study. Eur Neuropsychopharmacol. (2021) 51:55–67. doi: 10.1016/j.euroneuro.2021.04.015
Keywords: oxytocin, alcohol use disorder, alcoholism, addiction, pharmacotherapy, oxytocin receptor agonist, individualized medicine
Citation: Ryabinin AE and Zhang Y (2022) Barriers and Breakthroughs in Targeting the Oxytocin System to Treat Alcohol Use Disorder. Front. Psychiatry 13:842609. doi: 10.3389/fpsyt.2022.842609
Received: 23 December 2021; Accepted: 04 February 2022;
Published: 28 February 2022.
Edited by:
Roberto Ciccocioppo, University of Camerino, ItalyReviewed by:
Valery Grinevich, University of Heidelberg, GermanyKatie Witkiewitz, University of New Mexico, United States
Copyright © 2022 Ryabinin and Zhang. This is an open-access article distributed under the terms of the Creative Commons Attribution License (CC BY). The use, distribution or reproduction in other forums is permitted, provided the original author(s) and the copyright owner(s) are credited and that the original publication in this journal is cited, in accordance with accepted academic practice. No use, distribution or reproduction is permitted which does not comply with these terms.
*Correspondence: Andrey E. Ryabinin, ryabinin@ohsu.edu