- 1Department of Clinical Genetics, Graduate School of Medical Science, University of Yamanashi, Kofu, Japan
- 2Department of Neuropsychiatry, Graduate School of Medical Science, University of Yamanashi, Kofu, Japan
- 3Department of Biology, College of Science and Health, William Paterson University, Wayne, NJ, United States
- 4Department of Psychiatry and Behavioral Sciences, Tokyo Metropolitan Institute of Medical Science, Tokyo, Japan
The endocannabinoid system (ECS) is composed of the two canonical receptor subtypes; type-1 cannabinoid (CB1R) and type 2 receptor (CB2R), endocannabinoids (eCBs) and enzymes responsible for the synthesis and degradation of eCBs. Recently, with the identification of additional lipid mediators, enzymes and receptors, the expanded ECS called the endocannabinoidome (eCBome) has been identified and recognized. Activation of CB1R is associated with a plethora of physiological effects and some central nervous system (CNS) side effects, whereas, CB2R activation is devoid of such effects and hence CB2Rs might be utilized as potential new targets for the treatment of different disorders including neuropsychiatric disorders. Previous studies suggested that CB2Rs were absent in the brain and they were considered as peripheral receptors, however, recent studies confirmed the presence of CB2Rs in different brain regions. Several studies have now focused on the characterization of its physiological and pathological roles. Studies done on the role of CB2Rs as a therapeutic target for treating different disorders revealed important putative role of CB2R in neuropsychiatric disorders that requires further clinical validation. Here we provide current insights and knowledge on the potential role of targeting CB2Rs in neuropsychiatric and neurodegenerative disorders. Its non-psychoactive effect makes the CB2R a potential target for treating CNS disorders; however, a better understanding of the fundamental pharmacology of CB2R activation is essential for the design of novel therapeutic strategies.
Introduction
This is an important update on the molecular framework for the therapeutic potential of targeting CB2 cannabinoid receptor ligands, based on reviews, and previous work by our group (1–3) and others. There has been an explosion of research, and new knowledge on the emerging and evolving endocannabinoid system (ECS) to an expanded endocannabinoidome (eCBome) to include other putative cannabinoid receptors (CBRs), lipid molecules, and previously unknown targets of endocannabinoids (eCBs). Delta-9-tetrahydrocannabinol (Δ9-THC) is the most abundant psychoactive, and most studied cannabinoid among the over 500 different compounds present in the cannabis plant (4). The expanding ECS which includes different protein and lipid mediators is involved in the pathophysiology of neuropsychiatric disorders and targeting this system might be of benefit in the management of a number of CNS diseases (5).
CB1Rs and CB2Rs are G-protein coupled receptors (GPCRs) (6). It turned out that CB1Rs are the most abundant GPCRs in the brain, and are expressed in motor function, reward and cognition (7) whereas CB2Rs was reported to be predominantly found in peripheral tissues of immune origin, (8–10). Our previous understanding was that CB2Rs were not expressed in the brain (10–12), but now there are numerous in vitro and in vivo studies showing neuronal and glial expression of CB2R in many parts of the CNS (1, 13). The expression of CB2Rs in dopamine neurons have been documented (14). Unlike CB1Rs, very little is known about the relevance of functional neuronal CB2Rs as they were previously considered to be absent from the brain, hence the role of CB1Rs have been more established and well-studied in comparison to the CNS effects of CB2Rs. However, as others and we have demonstrated at least in preclinical studies CB2Rs are post-synaptically expressed and just like CB1Rs are expressed in some pre-synaptic terminals (15, 16). The low expression of CB2Rs is now recognized but their expression is increased during injury and inflammation, with their upregulation during CNS disorders is providing a basis and focus of attention for the use of CB2R ligands in the neuroprotective and anti-inflammatory activity associated with neuropsychiatric and neurodegenerative disorders. With the increasing research and use of marijuana, some cannabinoids that lack CNS psychoactive effects have been associated with a role CB2Rs as we reviewed (16, 17). Furthermore, there is increasing association of inflammation and neuro-immune crosstalk in neuroinflammatory and neurodegenerative disorders. This review provides an update (1–3) and our pioneering basic research and those of others on the increasing putative neuro-immuno-ECS-CB2Rs in neuropsychiatric and neurodegenerative disorders using a narrative review methodology from currently used search engines. Therefore, this updated review (see also 1–3), discusses recent advances in the integrated neuro-immuno-cannabinoid network, and summarizes the potential role of CB2Rs in neuropsychiatric and neurodegenerative disorders. There are challenges with a paucity of research on the interaction and contribution of both CB1Rs and CB2Rs both in the periphery and CNS disorders. However, the determination of the crystal structures of CB1R and CB2R that revealed functional profile and relationship of CB2R antagonism versus CB1R agonism, provides insights for developing allosteric modulator and dual steric ligands targeting both receptors.
Endocannabinoidome: Insights Into the Expanding Endocannabinoid System With a Focus on Cannabinoid Type 2 Receptors
The expanding ECS include CB1Rs, CB2Rs, and other putative cannabinoid receptors (CBRs), like GPR-55, TRPV1, PPAR and their lipid molecules, and previously unknown targets of endocannabinoids (eCBs) which are endogenous substances that bind to CBRs to mediate their physiology actions (18, 7). 2-AG and AEA are well-characterized eCBs (19) with reports of opposing actions by differential activation of many signaling pathways that are associated with many biological systems. Other lipid molecules including peptide eCBs, fatty acid amides, ethanolamides, and monoacylglycerol molecules with CBRs provides the platform from the ECS to eCBome. It turned out that the most abundant G-protein coupled receptors (GPCRs) in the human brain is for CB1Rs that has been well characterized compared to the accumulating knowledge on CB2Rs that also belong to this family of GPCRs. While the tissue distribution of both CB1Rs and CB2Rs are mostly different, some overlap exists in brain regions where they may work together or independently using similar or different signaling cascades (3, 6, 20–22) resulting in similar or opposing effects (17). There are abundant distribution of CB1Rs in pre-synaptic terminals not only in brain areas associated with the control of emotionality and motor function, but also in other brain regions. In contrast to the pre-dominantly presynaptic localization of CB1Rs, CB2Rs are mostly in post-synaptic terminals. However, just like CB1Rs that are also expressed in some post-synaptic terminals, CB2Rs are also expressed in some pre-synaptic terminals (1, 2, 4). For example, in the rat brain, using in vitro preparations, there is the presence of postsynaptic intracellular CB1Rs and CB2Rs following activation of TRPC4/5-like channels, and a demonstration of the presence of functional presynaptic CB2Rs inhibiting [3H]-glutamate release at subthalamo-nigral neuron terminals that are involved in motor control (23). Although CB1Rs and CB2Rs are both GPCRs with 44% similar homology and molecular structure, but with known different cellular expression and distribution patterns with functional specificity (Figure 1) (24). First cloned in 1993, CB2R is located on human chromosome 1p36 (8, 25–28) and mouse chromosome 4. Earlier studies could not find CB2Rs in the brain tissue and suggested that CB2Rs were absent in the brain and they were considered as peripheral CBRs, since in situ hybridization (ISH) and Northern blot analysis failed to detect CB2R mRNA in rat, mouse, and human brains (8, 9, 29, 30). However, studies conducted in our laboratory and by others identified the presence of CB2Rs throughout the CNS using different methods including ISH, immunostaining and gene expression (17, 31–38). Emerging evidence also shows that significant CB2R mRNA in the mouse cerebellum (39, 40), cortex (38, 41–43), striatum (9, 41), hippocampus (43), amygdala (16, 41), brainstem (1), and retina (44), and in the globus pallidus of non-human primates (43). In addition, some previous studies reported the discovery and functional characterization of CB2Rs in neural progenitor cells, neurons, glial and endothelial cells (13, 35, 45–47). Furthermore, two CB2R isoforms, CB2A and CB2B, have been characterized in the rodent and human brain, with CB2A, CB2B and CB2C only in the rat brain (Figure 1C) (38) along with a new CB2 transcript that has been found in mouse and monkey B lymphocytes (48). The evidence clearly suggests the expression of CB2R in the brain in addition to their expression in the periphery. With two separate promoters and 4 exons CNR2 gene is almost four times as large as CNR1 in genomic size, with CB2a and CB2b specie specific isoforms for brain and immune differential tissue expression profile, respectively (49) (Figure 1).
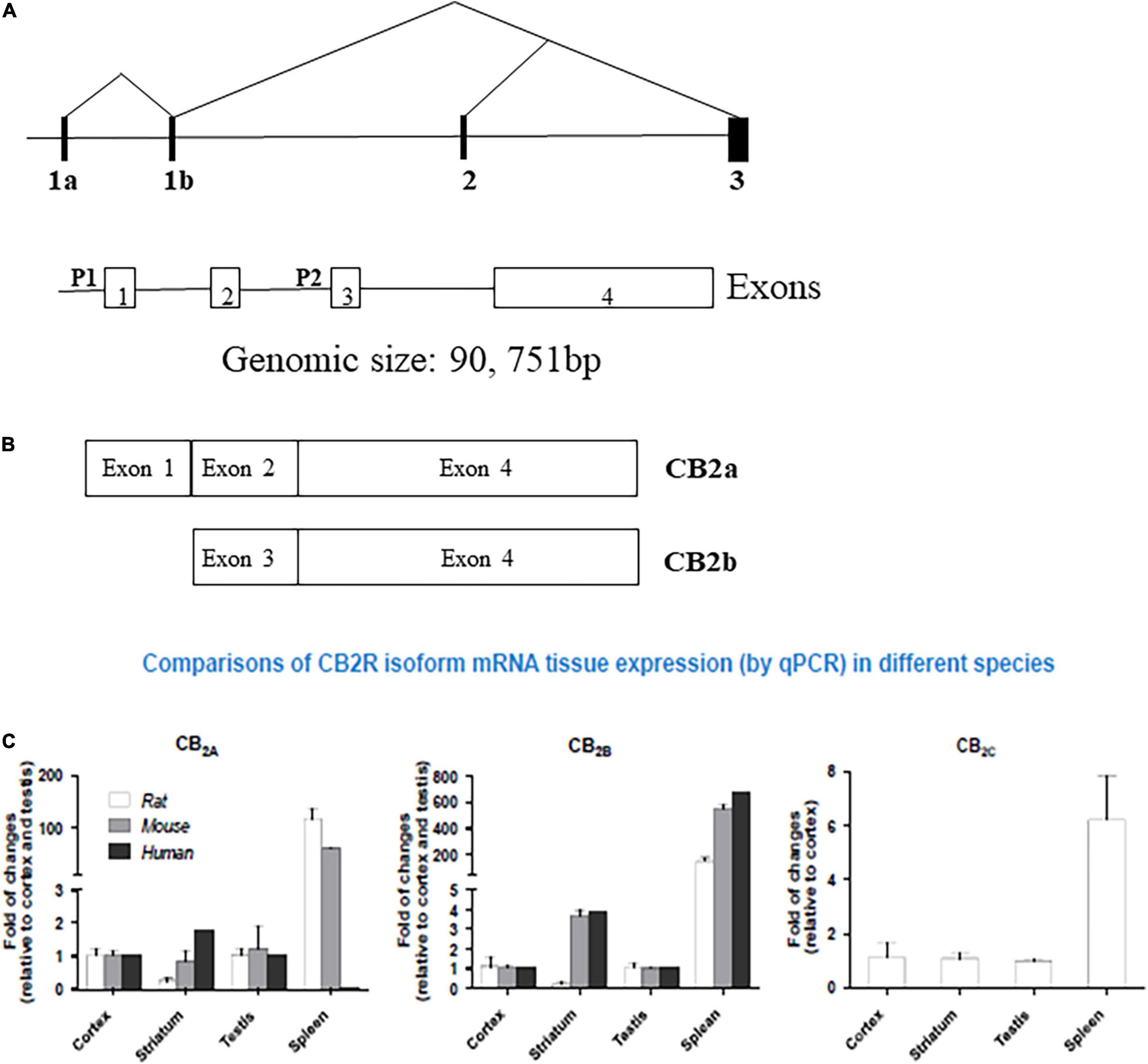
Figure 1. Human hCB2 genomic structure and isoforms: (A) human CNR2 genomic structure. P represent promoters and exons are open boxes with the exon numbers. (B) CB2a and CB2b isoforms and (C) comparison of CB2R isoform mRNA in Human, Rat, and Mouse tissue expression by qPCR. (See also reference Liu et al. (38)).
The activation of CB2Rs inhibits adenylyl cyclase (AC) activity and initiates MAPK and phosphoinositide 3-kinase (PI3K)-Akt pathways (Figure 2) with subsequent activation of the Jun N-terminal protein kinase (JNK), extracellular signal-regulated kinase (ERK)1/2 and p38 (50, 51). In addition, CB2R agonists results in increased synthesis of ceramide, a sphingolipid messenger, particularly in tumor cell lines, which induces apoptotic cell death (52). CB2Rs are mainly expressed post-synaptically and their activation inhibits postsynaptic neuronal function through membrane potential hyperpolarization (53), and also reported presynaptically in some terminals. Thus, CB2Rs are involved in modulating a variety of behavioral effects in the CNS with reports that CB2Rs modulate food intake, body weight (54, 55), depression and anxiety (14, 56), drug addiction (57, 58) and schizophrenia-like behavior (59). Brain CB2Rs are expressed at low levels under physiological conditions; however, in pathological conditions, such as neuropathic pain (60), stroke (61), traumatic brain injury (TBI) (62), neurodegenerative diseases (57, 63, 64) or drug addiction (65, 66), their expression is enhanced and up-regulated. The inducible nature of CB2Rs during events with underlying inflammation can make them a potential therapeutic target and ligands that activate or inhibit the activity of CB2Rs might be used to treat different disorders without causing profound adverse drug and intoxicating effects (67). As our understanding of the involvement of the ECS in different CNS physiologic and pathologic conditions increases, the ECS has gained a special attention as a potential therapeutic target and in designing safe and effective drugs to treat CNS disorders (68). Furthermore, understanding the underlining mechanisms for the involvement of the ECS in different pathological conditions including neuropsychiatric disorders is important in early diagnosis and treatment of these disorders. The expanded ECS, the (eCBome), that has been identified include N-acylethanolamines (NAEs), 2-acylglycerols (2-AcGs), N-acyl-amino acids, N-acyl-dopamine and N-acyl-serotonins (5). As studies unravel the complexity of this system, the interest in utilizing the eCBome system as a potential therapeutic target for the treatment of neuropsychiatric disorders is increasing (24, 5). In addition, previous studies revealed that targeting the CB1R is associated with adverse effects including anxiety, depression and even suicidal ideation (69, 70), and hence the search for a new therapeutic targets with minimal adverse effect is gaining special attention. Recent studies showed that targeting the CB2Rs, unlike the CB1Rs, is safe and effective and that CB2Rs might be new possibilities for safe targeting of the eCBome system (71). Therefore, there is increased research focus on the effects of neuronal function and behaviors induced by CB2R modulation and potential therapeutic applications (3–24). CB2Rs and their variants share an immunological basis that has been associated with endophenotypes of reduced immune function. Specifically, CB2R neuro-immune crosstalk may underlie chronic inflammation that is commonly observed in neuropsychiatric and neurodegenerative disorders. The association of CB2Rs with neuron-microglia crosstalk as target for reduction of the neuroinflammatory process could be considered as a target for the reduction of M1 action and induction of M2 activation. Thus, the molecular features, role and expression of CB2Rs in different neuronal and microglial phenotypes (MO, M1, and M2) will provide structural frame work in designing CB2R ligands in neurological disorders associated with neuro-inflammation. Of note, we have demonstrated and reported the expression of CB2Rs in neuronal, glial, and endothelial cells in the CNS and its function that is associated with neuropsychiatric and neurological disorders with neuroinflammation (17).
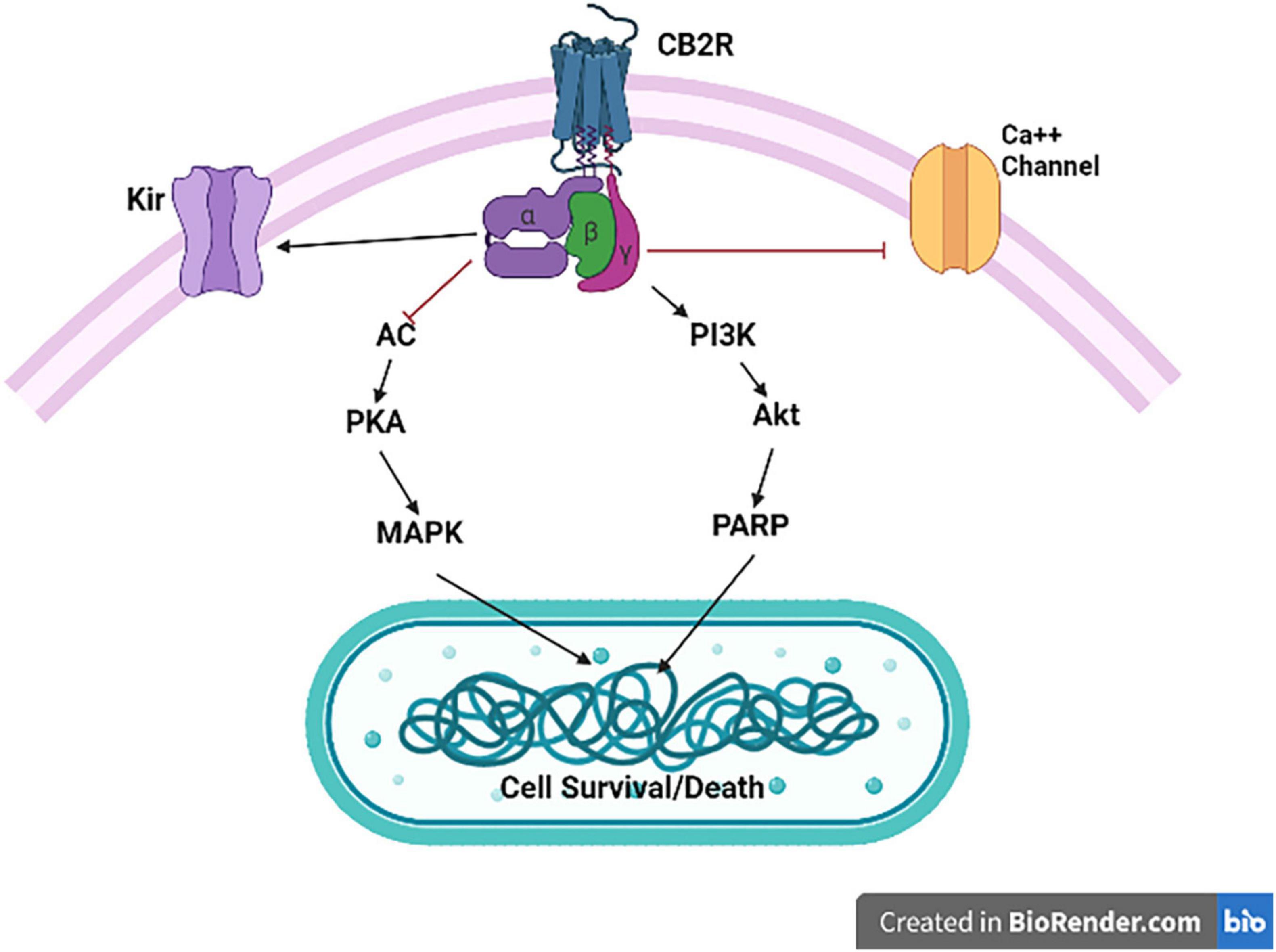
Figure 2. Cannabinoid type 2 receptor signaling. CB2R activation is associated with Gαi/o-dependent inhibition of AC activity and Gβγ-dependent activation of the different MAPK cascades. CB2R activation also results in inhibition of specific calcium channels (Ca++), and enhance opening of inwardly rectifying potassium (Kir) channels.
Cannabinoid Type 2 Receptors in Neuropsychiatric and Neurodegenerative Disorders
Research in animal models using different molecular techniques like immunohistochemistry, Western blotting, gene expression and a battery of behavioral tests have identified the involvement of elements of the ECS particularly CB2Rs (Figure 3) in models of CNS function and dysfunction. Previous studies reported evidence for the involvement of CB2Rs in neuropsychiatry (34–37, 45, 72, 73). The expression of CB2Rs in healthy nervous system is low but inducible with varying perturbation that lead to enhanced and up-regulation associated with neurodegenerative and neuropsychiatric disorders. New lines of evidence now indicate that CB2R upregulation could serve as a neuroprotective mechanisms associated with a number of physical or mental injury. As the clinical and functional implications of neuronal CB2Rs in the brain is gradually becoming clearer, more research is unraveling their contribution in neuropsychiatry and global drug addiction (29). Currently there are no effective therapy and cure for most of neuropsychiatric disorders. Besides, the available conventional therapies are associated with a wide range of unwanted adverse drug effects. Hence there is a need for the search of new therapeutic targets for the development of safe and effective medications for preventing or retarding the disease process in neuropsychiatric disorders (74). Studies reviewed in the previous sections demonstrated the role of CB2Rs in the regulation of physiologic functions and hence they can be utilized as a potential target in neuroinflammatory and neurodegenerative disorders (75, 76), but requires further clinical trials. Furthermore, dissociating CNS psychoactivity and adverse side effects from therapeutic effects is a desirable goal. Below we briefly review evidence supporting the putative role of CB2Rs in various neuropsychiatric and neurodegenerative conditions. The preclinical and clinical role of CB2Rs in these disorders are summarized in Tables 1–4.
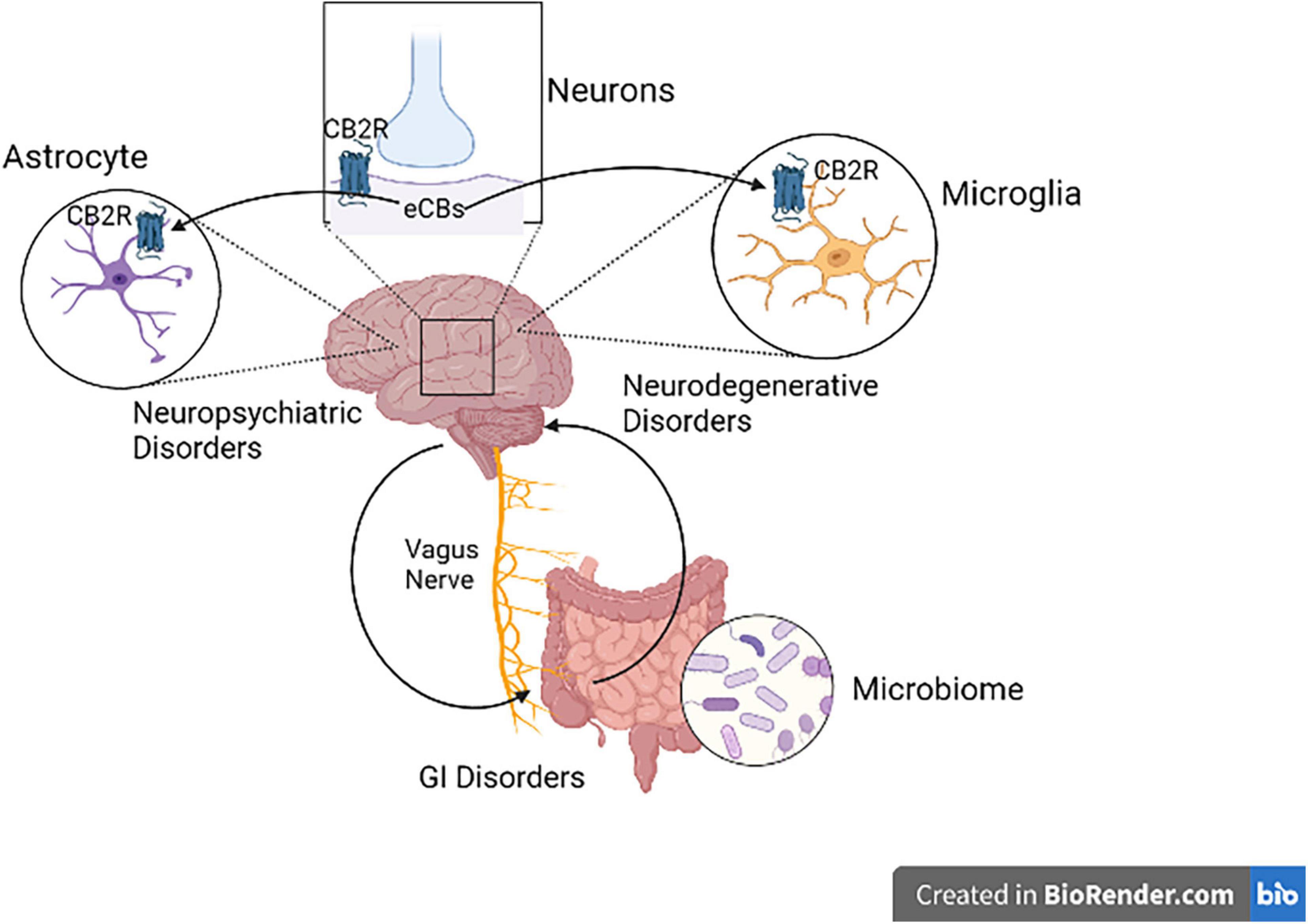
Figure 3. Cannabinoid type 2 receptor neuro-immune crosstalk in neuropsychiatric disorders. Activation of postsynaptic neuronal CB2Rs by cannabinoids alters neuronal network and function. CB2R might be a potential therapeutic targets in neuropsychiatry, since CB2R activation switches microglia to an anti-inflammatory state during injury. CB2Rs found in astrocytes also play a great role in Astrocyte-Neuron-Glia signaling.
Role of Cannabinoid Type 2 Receptors in Psychiatric and Emotionality Disorders
Anxiolysis and Anxiety-Related Disorders
Anxiety is a behavior associated with the anticipation of possible threats that provides coping mechanism to prevent stressful or harmful situations (77). There is increasing preclinical evidence for the involvement of the ECS in controlling mood and emotional behavior, with anecdotal evidence from cannabis users with different subjective effects. The ECS has a pivotal role in the regulation of mood disorders (78) and CB2Rs are expressed in the amygdala, hippocampus, prefrontal cortex and hypothalamus. These brain areas are involved in regulating anxiety like behavior and this suggests the potential role of CB2Rs in regulation of emotional responses (13, 32, 35, 79). Using the a two-compartment black and white chamber- and elevated plus maze test for assessing anxiety-like behavioral responses, we have reported on the effect of CB2R ligands on stress-induced anxiety-related behavior in mice. Our result showed that acute administration of JWH-015 (1–20 mg/kg) which has more affinity for binding to CB2Rs than to CB1Rs dose dependently induced an anxiogenic response in the black and white box but attenuated stress-induced gender-specific aversion to the open arms of the elevated plus maze (34, 35). Using rodent models of acute and chronic anxiety-like behavioral responses, Valenzano et al. (80) demonstrated that administration of GW405833 (100 mg/kg), a CB2R agonist, induced anxiolytic-like effects in the marble burying test that was not reversed by the administration of CB2R antagonist. However, in a mouse stress model, chronic mild stress (CMS) increased anxiety-like behavior responses in Zero maze test paradigm, and treatment with JWH-015 (20 mg/kg) reduced the anxiety-like behavioral effects similar to the effects of the antidepressant, fluvoxamine (81).
The involvement of CB2Rs in anxiety-like behavior was described by studies using selective CB2R antagonists. Acute administration of AM630 (1, 2, or 3 mg/kg) in mice induced anxiogenic-like behavioral effects, whereas with chronic administration anxiolytic-like effects were reported using the light-dark box and the elevated plus maze test (56). Treatment with AM630 (3 mg/kg) worsened the anxiety-like behavioral responses induced by CMS, contrary to the effects observed with JWH-015 treatment as described above (82). Interestingly, studies using transgenic mice overexpressing CB2R in the CNS (CB2xP mice) showed lack of effect against anxiogenic-like stimuli in the light-dark box and the elevated plus-maze behavioral tests (56). In agreement with this, another study using mice lacking the CB2R gene (Cnr2–/– mice) revealed that CB2R gene knock out mice developed higher levels of anxiety-like behavioral responses in both tests (59). We have investigated the involvement of CB2Rs in emotionality test using dopamine neuron cell-type specific CB2R conditional knockout mice (DAT-Cnr2 cKO). The study utilized the elevated plus-maze and the two compartment black/white box behavioral tests (14) and the results demonstrated that the DAT-Cnr2 cKO mice experience significant anxiety-like behavior compared to the wild type mice.
While the mechanism and the involvement of the ECS in mood and emotionality requires more investigation, clinical studies on the role of CB2Rs on anxiety-like behavior are very scarce. But, one study done on children with a primary anxiety disorder investigated whether genetic variation in the ECS explained individual differences in response to cognitive behavioral therapy (83). The study revealed that there is a relationship between the rs2070956 and rs2501431 polymorphisms of CNR2 gene and a reduced treatment outcome in children with anxiety disorders. While rs2070956 and rs2501431 polymorphisms are functionally unknown, however, rs2501431 is located in the main exon of CNR2 gene. The results obtained from animal and clinical studies show a role of CB2Rs in modulating anxiety-like behavior, however, there is a need for further studies in the determination of the therapeutic potential of CB2Rs in anxiety and mood disorders.
Endocannabinoidome-Cannabinoid Type 2 Receptors and Depression
A new approach and targeting the eCBome and psychopathology is increasing not only in basic but also in clinical trials and research with increasing use of cannabis and cannabinoid analogs in children and adult population. There are different types of depression with co-morbidities with mental and neurological disorders. Major depression is characterized by depressed mood and anhedonia – a lack of pleasure, including other symptoms like reduced appetite and libido and disturbed sleep. Preclinical and clinical studies showed that the ECS is responsible in modulating depression with reports that there is a negative correlation between the ECS signaling and depression (84). In animal models of depression and depressed patients, changes have been observed in eCB levels. The withdrawal of rimonabant, a CB1R antagonist, used for the treatment of obesity due to risk of suicide and depression has received increased interest in the role of the CB1R in affecting mood and affective behavior. Some studies have investigated the role of CB1Rs in depression with more basic and clinical components, and factors like stress, interaction with the endocrine system and the use of cannabis and depression with revealing outcomes. Whereas, the involvement of the CB2R in affective disturbances has not received much attention (52). Previous studies indicate that overexpression of CB2R provoked depression-like response. CB2xP mice (overexpressing CB2R) reduced immobility time in acute models of depression (tail suspension and novelty-identification and feeding tests) (80). On the contrary, the results found with the dopamine neuron cell-type specific CB2R conditional knockout (DAT-Cnr2) (14) and Cnr2–/– mutant (59) mice demonstrated depressive-like behavior indicated by enhanced immobility time in the tail suspension and forced swimming tests. This discrepancy might be due to individual and species differences between the strains used and/or differences in the animal model utilized. A pharmacologic study using GW405833, a CB2R agonist, demonstrated that acute administration of the agonist did not alter time spent immobile in the forced swim test (85).
It is important to point out the validity and the mixed and conflicting responses in the use of some of these animal models of depression that have been questioned in the scientific community. Nevertheless, the CMS is commonly used animal model of depression with similar behavioral and physiological effects observed in clinical settings in patients with depressive disorders (86–88). The involvement of CB2R in depression was demonstrated in a study using mice subjected CMS. The results showed that CB2R protein levels measured by immunoblotting in whole brain extract were enhanced in mice subjected to CMS for a period of 4 weeks (36, 45). However, another study showed a reduction in the level of CB2R mRNA in the hippocampus of mice subjected to CMS for 7–8 weeks, compared with non-stressed controls (80). The contradiction might be due to differences in the time of exposure to CMS and variations in the brain regions used to measure the level of CB2R mRNA. The CMS protocol is characterized by reduced intake of sucrose, used as a measure of anhedonia-like response, a hallmark of depression. Studies using transgenic mice that over-express the CB2R showed that there is no change in sucrose consumption and immobility time in mice subjected to tail suspension test, respectively (80). But in another study done to evaluate the effect of CB2R ligands on sucrose consumption, injection of JWH-015, a CB2R agonist, daily or AM630, a CB2R antagonist, did not alter CMS-induced decreases in sucrose consumption (36, 37). Taken together, results from the preclinical studies indicate the possible behavioral and molecular role played by CB2Rs in CMS.
There is limited literature on the role of CB2Rs in depression in humans. In one study done on Japanese patients with depression, the authors found that there is a high incidence of the Q63R polymorphism of CB2R gene, this polymorphism could alter the receptor function and reduces the physiological responsiveness to CB2R ligands (37). Furthermore, a reduction in the expression of CB2R gene was documented in brain regions involved in regulating emotionality like the amygdala and the prefrontal cortex in a postmortem study performed in suicide patients (89) and in patients with major depression (90), supporting a role that CB2Rs could play in depression and the potential of these receptors as a new target for the treatment of depressive disorders, but requires additional modeling and clinical investigations.
Endocannabinoidome-Cannabinoid Type 2 Receptors in Psychosis
There are risks for harm with rising cannabis and cannabinoid medical use, and the widespread availability for recreational consumption creates the potential for psychotic disorders especially in adolescents whose brains are still developing and in those with psychosis. Thus, early exposure can trigger onset of psychosis in such vulnerable adolescents. Schizophrenia a type of psychotic disorder that is characterized by thinking disturbances, hallucinations and delusions is associated with genetic and epigenetic risk factors. Disturbances in the regulation of the ECS with altered levels of eCBs was associated with the development of schizophrenia. Indeed densities of eCB receptors and levels of eCBs have been suggested as possible biomarkers in neuropsychiatric disorders (83). Studies also revealed that the causal disturbances of neural processes in adulthood by early cannabinoid exposure are linked to psychiatric risk like schizophrenia (91). Therefore, the role of cannabinoid self-medication hypothesis, early exposure to cannabis and onset of psychosis in vulnerable adolescents has fueled research interests in changes in the ECS in neuropsychiatric disorders. Recently there is also an increase interest in the use of herbal, synthetic and eCBs for symptomatic management of schizophrenia (92). Studies using animal models of schizophrenia implicated the involvement of CB2R in schizophrenia. Early maternal deprivation in rodents is a model for neurodevelopmental stress and there are some reports indicating that maternal deprivation affects the ECS and that these changes may account for the proposed schizophrenia-like phenotype (93, 94). A study to evaluate the expression of CB1R and CB2R in the hippocampus showed that maternal deprivation induced a significant increase in CB2R immunoreactivity in the hippocampal areas, indicating possible involvement CB2Rs in neurodevelopmental mental illnesses such as schizophrenia (95).
The pre-pulse inhibition (PPI) test is one of the tests used to assess attention deficits associated with schizophrenia (96). Methamphetamine or MK-801, a non-competitive N-methyl-D-aspartate (NMDA) glutamatergic receptor antagonist, are two widely used animal models of schizophrenia that mimic effects observed in schizophrenic individuals (97). Studies showed that AM630, a CB2R antagonist, did not affect PPI on its own, but it did enhance the MK-801- and methamphetamine -induced decrease in PPI and increase in locomotor activity (98). Another study found that administration of JWH015 in doses of 1, 3, and 10 mg/kg enhanced PPI impairment caused by MK-801 (99). CB2R’s role in schizophrenia was investigated in genetically engineered mice. It was reported that Cnr2–/– mice were more susceptible to hyperlocomotion caused by acute cocaine exposure, as well as PPI and cognitive changes. Furthermore, in these mutant Cnr2–/– mice, treatment with the antipsychotic risperidone normalizes PPI readings (59, 100). Interestingly another study showed that CB2R is required for the antipsychotic effects of acetylcholine muscarinic 4 (M4) receptor agonists like UV0467154 (101). This study found that stimulating M4 receptors increased the release of eCBs while inhibiting dopamine release, implying that M4 agonists have a role in antipsychotic efficacy. As a result, inhibiting CB2Rs with the antagonist AM630 would prevent the antipsychotic effects of M4 agonists. Clinical studies have also shown that CB2R is involved in schizophrenia. In Japanese schizophrenia patients, the CNR2 polymorphisms rs12744386 and rs2501432 were found to be significant (98) and the result also showed that both risk alleles of the two polymorphisms associated with schizophrenia were related to the direction of lower functioning of CNR2 and poor responses to CB2R ligands. As there is, yet no major evidence supporting the use of cannabinoids targeting other components of the eCBome, targeting the CB2R may play a critical role in modulating psychotic symptoms. In schizophrenia and some addictive disorders discussed below CB2Rs was associated with comorbidity of schizophrenia and cannabis dependence with polymorphisms in CNR2 gene implicated as a risk factor. Therefore, controlled clinical trials are need to determine the role of CB2Rs in the treatment or as an adjunctive strategy in schizophrenia.
Cannabinoid Type 2 Receptors in Addiction
The efficacy and success of cannabinoid pharmacotherapy in drug addiction is at best with mixed results. Some reports show that cannabis use may be effective in curbing alcohol and opioid addictions (102). Paradoxically, with the increased prevalence of cannabis use globally, and self-medication hypothesis, associations between cannabis use, cannabis use disorder (CUD) and mood disturbances have increased (103). Cannabis use has been linked to psychiatric disorders including manic, psychotic and symptoms of depression, but the underlying mechanism remains unclear, with no currently approved treatment of CUD. Evidence from a number of preclinical and clinical studies show that the ECS is recognized as a neural substrate in the effects of classical drugs of abuse associated with drug addiction in vulnerable individuals. The discovery of this previously unknown but ubiquitous ECS, and that CBRs are encoded in the human genome on chromosome 1 and 6 has led to the changing landscape on cannabinoid research and providing scientific evidence on the potential therapeutic benefits targeting the ECS. However, studies also showed the involvement of the ECS in addictive behavior (104, 105) and the association of early childhood and adolescent exposure to cannabinoids with addictive behavior (91, 106). There are still gaps in our understanding, but the discovery of the expression of CB2Rs in brain regions involved in drug addiction, such as the ventral tegmental area (VTA), nucleus accumbens (NAc), amygdala and hippocampus unraveled and encouraged investigation of the role of CB2Rs and their potential therapeutic target in drug addiction (107, 108). Pharmacologic and genetic studies showed that CB2Rs are involved in the effects of psychostimulants like cocaine, amphetamine and methamphetamine. CB2R is involved in cocaine motor sensitization in studies employing genetically modified mice. Mice overexpressing CB2R in the (CB2xP) showed reduced hyperlocomotor effects in response to acute cocaine administration and were less susceptible to the motor sensitization after repeated cocaine administration (109). Interestingly, mice lacking the CB2R (Cnr2–/–), which have increased sensitivity to cocaine-induced hyperlocomotion, had the opposite effect (59).
A study aimed to evaluate the effects of psychostimulants in dopamine neuron CB2R conditional knockout (DAT-Cnr2 cKO) mice on locomotor activity showed that mice had increased cocaine, amphetamine and methamphetamine-induced hyperactivity, without psychostimulant-induced sensitization when compared to wild type controls. In addition, the authors reported that cocaine, amphetamine, and methamphetamine produced robust conditioned place preference (CPP) in both DAT-Cnr2 cKO and wild type mice (58). In stark contrast, studies using JWH133 revealed systemic and local administration of JWH133 into the NAc blocked cocaine hyperlocomotion in mice (33, 110). In line with this, a study revealed that JWH133 inhibited cocaine and nicotine-induced CPP in wild type mice (58). However, in another investigation AM630 was found to have no effect on the locomotor effects after an acute or repeated cocaine administration in rats (110, 111). These inconsistencies could be explained by differences in animal species and the methods utilized. Since cocaine produced place aversion rather than place preference in CB2xP animals, and these transgenic mice showed an impairment in the acquisition of cocaine self-administration (109), CB2Rs appear to be implicated in the reinforcing features of cocaine. Evidence also showed that intranasally or intra-accumbens administration of JWH133 prevented intravenous cocaine self-administration (33). The involvement of CB2Rs in a model of addictive behavior has been demonstrated by the study using the natural CB2R agonist β-caryophyllene (BCP). The results showed that BCP attenuated methamphetamine self-administration in rats which was partially blocked by AM630 (112). The study also revealed that deletion of CB2Rs blocked low dose BCP-induced reduction in methamphetamine self-administration.
There are also investigations on the involvement of CB2Rs in the modulation of alcohol consumption and reward. The injection of BCP lowered ethanol CPP and ethanol intake in mice, as well as increased voluntary ethanol consumption in the two bottle paradigm and incentive to drink in the oral ethanol self-administration in the mutant Cnr2–/– mice (113, 114). In addition, sub-chronic injection of JWH015 enhanced alcohol intake in mice that had previously been exposed to chronic stress, with no impact in mice that had not been exposed to chronic stress (74, 115). A recent study also demonstrated that alcohol exposure during adolescence induced increases in the amygdala expression of the CB2Rs in rats (116).
A substantial incidence of the single nucleotide polymorphism R63Q in the CNR2 gene locus was observed in a cohort of Japanese alcoholic patients in clinical investigations (74). This single nucleotide polymorphism causes a missense mutation in the first intracellular domain and causes a structural change, which could alter the receptor function and reduces the physiological responsiveness to CB2R ligands (107). More recently, Navarrete et al. extensively complied significant appraisal of CB2R involvement in the treatment of substance use disorders (SUDs), including alcohol, psychostimulants (cocaine and nicotine) with emphasis on the CB2R species differences in the effects of the SUDs covered (117). They concluded that the development of pharmacotherapeutic agents targeting the CB2Rs might offer novel approaches when combined with existing options (118). All the above data support a role of CB2Rs in modulating the addictive effects of alcohol and different psychostimulants, indicating that CB2Rs might be targeted in the treatment of drug addiction. Therefore, there is now increased focus and interest in CB2Rs in drug and alcohol addiction with increasing reports indicating their functional expression not only in reward circuits but also on the CB2R-neuro-immune crosstalk and signaling in neuropsychiatric disorders. With global increase in medicinal and recreational cannabis use, there is potential for increased CUD and therapeutic challenge as the frequency of cannabis use is a risk factor for developing and predicting CUD. Of note, no direct clinical studies have focused on targeting CB2Rs in CUDs.
Role of Cannabinoid Type 2 Receptors in Autism Spectrum Disorder
Autism spectrum disorder (ASD) has early childhood onset with a lifelong progression. It is characterized by poor social skills, deficits in communication as well as by stereotypic behaviors, (119). The ECS plays a significant role in ASD. A review on the role of the ECS in autism found documentation that endocannabinoid signaling plays a key role in many human health and disease conditions of the CNS (120). However, there is limited literature, pre-clinical and clinical, on the role of CB2Rs in ASD. CB2Rs were also identified as a possible target for autism in preliminary research. Indeed, in the cerebellum of BTBR T + tF/J, a mouse model of ASD, there was an increased level CB2A mRNA expression, but not the CB2BR, gene-transcript isoforms (38).
A controlled study of ASD done on autistic children showed that gene expression for CNR2 but not CNR1 was up-regulated in peripheral blood mononuclear cells (PBMCs). The result indicated that CNR2 gene expression was significantly higher in individuals with ASD compared to controls. In addition, the gene expression for one of the enzymes responsible for synthesis of the endocannabinoid anandamide (AEA) (NAPE-PLD) was significantly lower in individuals with ASD. This could have caused an increase in CB2Rs resulting from a decrease in AEA synthesis which may be indicative of decreased ECS tone in ASD (121). While there a multifactorial and putative link of the ECS to ASDs, there are no solid biomarkers, and our investigations using BTBR T + tF/J, and other preclinical mouse models rely on characterization of behavioral alterations (38). So there is a need of a thorough behavioral characterization of mouse models of ASD to demonstrate the therapeutic effects of pharmacological treatments targeting the ECS (122). It is important that more basic and clinical research and trials may unravel etiology of ASDs that involve multigenetic risk factors and environmental neurodevelopment factors in utero including use of drugs, exposure to chemicals, infections, diet and stress (123–125). Further basic and clinical research has also to be done on the role of CB2Rs in ASDs before claiming this receptor as a potential therapeutic target in ASDs.
Eating Disorders
The ECS modulates appetite – a basis for adjunctive cannabinoids use to counter AIDs cachexia and regulates the metabolism of glucose in the pancreas and liver (126–128). It involved in the gaging appetite value, control and associated with weight and obesity supporting data on the modulation cannabinoids in food intake. Food craving often referred to as munchies after smoking or ingesting cannabis has implicated the ECS in food intake. A striking evidence was the approval and use of the CB1R antagonist, acomplia as an antiobesity medication in Europe and was withdrawn because of suicide, and other limiting psychiatric side effects. Now with significant technological advances and progress in eCBome research, it may be feasible to produce ligands with opposing activation profiles of CB1R and CB2R with high degree of selectivity in obesity, neuropsychiatric and neurodegenerative disorders. ‘Diabesity’ refers to the relationship between diabetes and obesity (129, 130) that is in part linked with ECS signaling in adiposity and metabolism. This indicates that dysregulation of the gut ECS and altered levels of eCBs is associated with obesity and metabolic syndrome. The physiological roles of the ECS in the gastrointestinal tract (GIT), adipogenesis and lipogenesis provides an increasing link in dysfunction of the ECS with obesity, metabolic syndrome and other disturbances in the periphery (131, 132). The most common eating disorders are anorexia nervosa (AN) and bulimia nervosa (BN) presents abnormal eating behaviors that generally result in severe food restriction with episodes of binge eating and vomiting without significant changes of body weight in BN and a dramatic loss of body weight in AN (119). The hypothalamic eCBs have been shown to modulate eating behavior in animals and humans, thus indicating the role of the ECS in the pathophysiology of eating disorders (133, 134). A study done on 20 women with AN, 23 women with BN, and 26 healthy women, the levels of CB1R and CB2R expression were examined. The study found no differences in CB2R mRNA levels in the blood of AN and BN patients when compared to controls, with no significant difference between the two groups (135, 136). So far, there is only one study done on human genetic association to identify whether the CNR2 gene is involved in eating disorders (137). 204 individuals with eating disorders (94 AN and 111 BN) and 1876 healthy Japanese volunteers participated in the study. A non-synonymous CNR2 polymorphism, which reduces the physiological responsiveness to CB2R ligands, was found to be linked to both AN and BN. The R allele is substantially more prevalent in people with eating disorders than in controls, according to the findings. Furthermore, there was no change in allele frequency between patients with AN and BN when they were separated. The study demonstrates a link between CB2Rs and eating disorders. There is a paucity of literature on the role of CB2Rs in eating disorders, so there is a need for more research to corroborate these findings.
Role of Cannabinoid Type 2 Receptors in Neurologic and Neurodegenerative Disorders
Alzheimer’s Disease
Alzheimer’s disease (AD) is characterized by abnormal accumulation of β-amyloid (Aβ) in senile plaques in the brain that causes cognitive impairment, memory loss, and behavioral changes due to neurodegeneration and inflammation (138). Studies demonstrate that the ECS is involved in the regulation of memory (139, 140) and ECBs have been shown to decrease Aβ-induced microglia activation and neuroinflammation (141–143). Recently the CB2Rs have attracted attention in AD investigation because of its expression in immune cells and its enhanced expression during inflammation. Studies showed that there is increased expression of CB2Rs in brain tissue in AD patients and mouse models expressing pathogenic variants of amyloid precursor protein (APP) (141, 144, 145). Previous research found that genetic deletion of CB2Rs increased Iba1 staining and exacerbated soluble A42 and plaque deposition (146), implying that CB2Rs play a key role in preventing amyloid plaque pathology in AD (147). In mice, administration of JWH-133 improved cognitive impairment, inhibited neuroinflammation and oxidative stress, decreased tau hyperphosphorylation, induced vasodilation (148, 149), and enhanced glucose uptake, indicating that CB2R agonists could be used as nootropics (150). A recent study assessing the role of AEA analog-N-linoleyltyrosine (NITyr) in APP/PS1 mice mimicking the AD model showed that NITyr protected neurons against Aβ injury which is mainly mediated by the CB2Rs (151). Interestingly Rivas-Santisteban et al. (152) demonstrated CB2R activation blunted NMDA receptor-mediated signaling in primary hippocampal neurons from APPSw/Ind mice model of AD, suggesting a role of CB2Rs in AD pathogenesis. In addition, in AD model mice, CB2R activation by JWH-015 was found to improve new object recognition abilities while also regulating microglia-mediated neuroinflammation and dendritic complexity in a region-specific manner (153). Parenteral administration of 1-((3-benzyl-3-methyl-2,3-dihydro-1-benzofuran-6-yl) carbonyl) piperidine (MDA7), a novel selective CB2R agonist, inhibited the activation of microglial cells and astrocytes, decreased the level of expression of CB2R, enhanced the clearance of Aβ, and improved cognition and memory in rodent AD models (154, 155).
In various in vitro and in vivo AD models, CB2R activation reduced levels of neurotoxic factors and pro- inflammatory mediators produced by reactive astrocytes and microglial cells, stimulated microglial proliferation and migration, and decreased Aβ levels (156–158). Despite a large body of evidence for the involvement of CB2Rs in reducing and processing Aβ in a mouse model of AD, it is difficult to determine the therapeutic value of cannabis-based medicines in AD (159). This might be because of lack sufficient randomized clinical trials and inconsistent and inconclusive evidence. There are also reports that showed that mice with CB2Rs deficiency showed a reduction in microglial cells and macrophages, reduced expression levels of brain pro-inflammatory cytokines, diminished concentrations of soluble Aβ40/42, improved cognitive and learning deficits (141). In few clinical studies, there are contradictory reports on the potential of CB2R ligand in AD and further research is required to determine the potential role of CB2Rs in humans and in animal models of AD.
Parkinson’s Disease
Parkinson’s disease (PD) is a movement disorder characterized by degeneration of dopaminergic neurons that results in motor dysfunction (160, 161). Disease progression is characterized by inflammation through activation of microglia (162) and an increase in cytokines (163, 164). Accumulated evidence suggests that there is a strong potential for the ECS that could provide neuroprotection against acute or chronic neurodegenerative disorders (165–169). CB2R levels were significantly elevated in animal models of PD and postmortem studies of PD patients and this increase correlated significantly with an increase in microglial activation, indicating the possible role of CB2Rs in PD (64, 170). On the other hand, studies showed downregulation of CB2Rs in the substantia nigra and hippocampus 3 weeks after 1-methyl-4-phenyl-1,2,3,6-tetrahydropyridine (MPTP) injection in mice with MPTP-induced parkinsonian syndrome. This discrepancy might be due to differences in the animal model used, duration of treatment and/or brain regions studied. In addition, AM1241, the selective CB2R agonist, has been shown to regenerate DA neurons after the neurotoxic effect of MPTP treatment (165). Furthermore, mice lacking the CB2R showed enhanced activation of microglial cells and much more intense deterioration of tyrosine hydroxylase (TH)-containing nigral neurons in animal models of PD (170), which supported the potential neuroprotective role of CB2Rs.
In animal models of PD, Δ9-THCV, a CB2R agonist, reduced motor inhibition caused by 6-hydroxydopamine (6-OHDA) and the loss of TH–positive neurons caused by 6-OHDA lesion in the substantia nigra after an acute and chronic administrations, respectively. However, CB2Rs were poorly up-regulated in the rat substantia nigra in response to 6-OHDA. By contrast, the substantia nigra of mice that had been injected with lipopolysaccharide (LPS) exhibited a greater up-regulation of CB2Rs. The authors suggest that Δ9-THCV caused preservation of TH–positive neurons probably through the involvement of CB2Rs (171).
In another study the protective effect of JWH-015 against MPTP-induced nigrostriatal degeneration and suppression of microglial activation/infiltration through activation of CB2Rs (172) was implicated. In addition, there are reports showing that BCP reduced oxidative stress and neuroinflammation, blocked gliosis and pro-inflammatory cytokine release, and lowered nigrostriatal degeneration in rotenone (ROT) induced animal model of PD produced (173). Like the pre-clinical studies, clinical studies revealed that the expression of CB2Rs in different brain cells is upregulated in PD patients. One study demonstrated that CB2Rs are elevated in microglial cells recruited and activated at lesioned sites in the substantia nigra of PD patients compared to control subjects (170). In another study the authors observed that CB2R was located in TH-containing neurons in the substantia nigra at levels significantly lower in PD patients than in controls (174). Thus, CB2Rs may be a promising pharmaceutical target for alleviating parkinsonian symptoms and slowing disease development in Parkinson’s disease.
Huntington’s Disease
Huntington’s disease (HD) is a neurodegenerative disease caused by the expansion of CAG triplet repeat (cytosine-adenine-guanine), in the gene encoding the protein huntingtin (Htt), which leads to cognitive decline and abnormal motor movements (chorea) (24, 175). Currently there is no effective treatment for HD and the need for new therapeutic targets is increasing (24). Because the ECS is abundant in the basal ganglia, activation or inhibition of the ECS signaling pathway may have a major impact on motor responses (176), and CB2R is emerging as a new therapeutic target for the treatment and early diagnosis of HD (177). In the transgenic R6/2, CAG repeat length Huntington chorea mouse model, CB2R expression was shown to be elevated in the hippocampus, brain, striatum, and cerebellum (178). Studies also found that mice lacking the CB2Rs were more sensitive to malonate than the control group. In addition, CB2R-defficient mice exhibited an enhanced onset of motor deficits and increased severity (179, 180). There is expression of CB2R that was increased in striatal microglia in a transgenic mouse model of HD and in patients. In addition, the genetic ablation of CB2R exacerbated HD and the administration of CB2R-selective agonists reduced striatal neurodegeneration through microglial activation (181). Taken together, CB2Rs are neuroprotective and might be a potential target and compounds that selectively activate CB2R might be utilized as a potential therapeutic agent in the treatment of HD.
Multiple Sclerosis
Multiple sclerosis (MS) is an autoimmune disorder characterized by inflammation, neurodegeneration, and demyelination of neurons with no effective therapy (182) and studies showed the role of CB2Rs in inflammatory conditions associated with MS (177, 183). Microglial activation was linked to CB2R overexpression in an experimental autoimmune encephalomyelitis (EAE) animal model of MS (184). HU-308, the CB2R-selective agonist, also dramatically reduced EAE symptoms, axonal loss, and microglial activation when given orally (185). O-1966, a CB2R agonist, was also found to reduce immune cell invasion, diminish white cell rolling and adhesion to cerebral microvessels and improve neurologic function following an insult on EAE progression (186). Additional study done using EAE animal models demonstrated that BCP significantly inhibit microglial cells, CD4+ and CD8+ T lymphocytes, cytokines and diminished axonal demyelination and modulated Th1/Treg immune balance through the activation of CB2Rs (187). Maresz et al. (184) also investigated that CB2R expression by encephalitogenic T cells reduced EAE associated inflammation. Furthermore, during EAE, CB2R-deficient T cells in the CNS showed reduced apoptosis, increased proliferation, and increased production of inflammatory cytokines, resulting in severe clinical illness. The Theiler murine encephalomyelitis virus- induced demyelinating disease (TMEV- IDD), which mimics central neuronal demyelination that occurs in MS, is another animal model of MS (188). In TMEV- IDD mice, CB1R and CB2R agonists showed an enhanced clinical benefits through immunomodulatory and anti- inflammatory mechanisms such as activation of CD4+ T cells, increase in regulatory CD4+ T cells in the CNS along with alterations in the cytokine and chemokine milieu (189, 190), which is another evidence for the role of CB2Rs in MS. Postmortem and clinical studies also showed that there is involvement of CB2Rs in MS. Yiangou et al. (191) indicated a higher level of microglia cells in human postmortem spinal cord specimens. Evidenced revealed that T lymphocytes, astrocytes, and both perivascular and reactive microglial cells were also observed to express CB2Rs in postmortem brain tissues from MS donors (192). These findings suggest that CB2Rs play a neuroprotective function in MS pathology, and targeting CB2Rs could aid in the management of symptoms and neurologic issues in MS patients.
Amyotrophic Lateral Sclerosis
Amyotrophic lateral sclerosis (ALS) is a cortical, brain stem and spinal cord motor neuron degenerative disease. Deterioration of both upper and lower motor neurons is the main feature of disease progression (141, 193). The involvement of CB2Rs in ALS has been demonstrated by different studies. A reduction in motor neuron degeneration and preservation of motor function in ALS was observed after selective activation of CB2Rs in animals (194–196). TDP-43 transgenic mice and postmortem specimen studies showed that there is upregulation of CB2Rs in activated microglia cells (191, 197). Studies also showed the survival interval after ALS onset was increased by 56% after the administration of the CB2R agonist AM1241 initiated at symptom onset (194). In addition, Kim et al. (195) reported a reduction in signs of disease progression when AM1241 was administered after onset of signs in an ALS mouse model [hSOD1(G93A)] transgenic mice. All these evidences indicate that CB2R has a role in preventing the progression of disease ALS. Therefore, CB2Rs might be considered a promising target for therapeutic approaches in ALS.
Epilepsy
Epilepsy is a common neurological illness, affecting more than 70 million individuals worldwide. The currently available anti-epileptic drugs are unable to control epileptic seizures in majority of patients (198), hence there is a need for a new therapeutic targets for effective anti-epileptic drugs. There is evidence on the role of the ECS in epilepsy (199, 200) and studies showed that CBD was effective in the treatment of epileptic seizures in preclinical (117) and clinical studies (201, 202). The role of CB2Rs in epileptic seizures has been documented in other studies (71, 203). A study showed that the selective CB2R antagonist AM630 inhibited palmitoyl ethanolamide (PEA) induced increase in the latency of seizure initiation and reduced the duration of seizures in an acute pentylenetetrazol (PTZ) rat seizure model (204). In addition, the administration of BCP was found to improve seizure activity in a mouse model (205). Despite the evidences showing the role of CB2Rs in controlling seizure in animals, few studies didn’t find a possible association between activation of CB2Rs and control of seizure. In a study using a HU-308, a CB2R selective agonist, HU-308 did not show an antiseizure effect and AM630 did increase seizure severity (206). Moreover, CB2R agonist AM1241 increased seizure intensity in a PTZ model (207) and AM630 and SR144528, can increase seizure susceptibility (206). Studies using genetically manipulated mice revealed that CB2R knockout mice show enhanced epileptic susceptibility, and a reduction in CB2R activity was associated with increased susceptibility (208). Taken together, accumulated evidence support the involvement of CB2Rs in the pathophysiology of epileptic seizures and hence CB2Rs might serve as a possible target for the treatment of epilepsy.
Traumatic Brain Injury
Traumatic brain injury is caused by mechanical injury of the brain that results in formation of hematoma that ultimately leads to long-term complications and death (209). Evidence shows the involvement of CB2Rs in modulating the pathophysiology of TBI. Studies using the CB2R agonist, O-1966, in mice with TBI indicated that administration of O-1966 attenuated blood-brain barrier disruption, neuronal degeneration (210) and induced acute neuroprotective effects (211), supporting a role of CB2Rs in the management of TBI. There are also reports showing an increase expression of CB2Rs in mice with TBI associated with edema neurologic deficits. In that study the authors found a positive correlation between the expression of CB2Rs and TBI (62). In another study aimed at evaluating the potential effects of the CB2R agonists, HU-910 and HU-914 in the pathophysiology of TBI in mice, Magid et al. (212) demonstrated an enhanced neuroprotection and neurobehavioral recovery. In a recent study the selective CB2R agonist, JWH133, protected white matter injury via PERK signaling in a rat model of traumatic brain injury (213–218).
Limitations and Future Perspectives
The growing research interests in eCBome, disturbances in its regulation, altered levels of eCBs, determination of the crystal structures of CB1R and CB2R pharmacology, discoveries of associated genetic polymorphisms provides targets for therapeutic intervention in neuropsychiatric and neurodegenerative disorders in the era of global deregulation of cannabis for medical and recreational use. Indeed densities of eCB receptors and levels of eCBs have been suggested as possible biomarkers in neuropsychiatric and neurodegenerative disorders. With the increasing evidence that CB2Rs are involved in brain, the functional role of CB2R neuro-immune axis in pathophysiological signaling in the development of psychiatric disorders warrants further investigation. It is also noteworthy that the determination of the crystal structures of CB1R and CB2R reveals a yin-yang relationship and functional profile of CB2R antagonism verse CB1R agonism for further innovative development of ligands with multiple therapeutic outcomes (2, 18). The relationship shows that some compounds when tested in vitro in the cAMP assay were potent agonists for the CB1R. Conversely, all of these ligands behaved as CB2R inverse agonists or neutral antagonists in the same functional test (17). However, the physiological implications of such opposing activation profiles between CB1R and CB2R should be studied further for their therapeutic potential. This highlights another area where CB1R and CB2R seems likely to work both independently and/or cooperatively that will benefit from critical medical application development. There are limitations and concerns regarding the use of CB2R medication for neurological disorders, as they are abundantly expressed in the periphery, and may have peripheral side effects, while they may be useful in CNS disorders associated with neuroinflammation. Further, research evaluating the numerous compounds in cannabis along with terpenes and flavonoids, will add to our understanding of this natural eCBome in neuropsychiatric and neurodegenerative disorders and contribute to novel biomarkers and therapeutic agents in health and disease.
Conclusion
With more research targeting the eCBome-CB2Rs, the pharmacogenomic basis might be personalized to different disorders that may require combination cannabinoids with drugs affecting other neurobiological targets. We now know that CB2Rs are found in the periphery and the CNS, but the absence of intoxicating effects of these receptors significantly increased attention for the investigation of these receptors as therapeutic targets in neuropsychiatric and neurodegenerative disorders. Currently there are no cures, and only partially effective treatment are available for most of these disorders and therefore, more research is warranted for new therapeutic targets of emerging eCBome using novel strategies including artificial intelligence (AI) that might have better prediction and therapeutic outcomes with minimal adverse effects. The rapid and explosion of cannabinoid research may also transform the current landscape in using in vitro and in vivo technologies that will enhance our understanding of the potential role of CBRs in neuropsychiatric and neurodegenerative disorders. Despite the progress recently, the withdrawal of the FAAH inhibitor during clinical trial and anti-obesity CB1R antagonist due adverse effects engenders cautionary approaches in clinical trials. It is encouraging that clinical trial targeting CB2R, and approval by the Food and Drug Administration (FDA) of nabiximols for epileptic seizures is making targeting components of the CB2R – ECS a pharmacotherapeutic strategy. The findings included in this review showed that CB2Rs are highly expressed in neuropsychiatric and neurodegenerative disorders and selective CB2R ligands have promising effect in symptomatic management of these disorders. Additional studies are required to evaluate the involvement of CB2Rs in these disorders using the full range of tools that are available to study the CB2Rs and their selective ligands in animal models as well as in controlled clinical trials. It is important that such future studies include translational and clinical profiles and in vivo and in vitro models that express human CB2Rs. Furthermore, careful evaluation of the side effects associated with chronic treatment of CB2R ligands will provide further insight into the potential role of CB2Rs in regulating neurophysiological and behavioral function.
Author Contributions
HI and EO conceived the focus of the review. BK searched the literature and wrote the first draft of the manuscript. HI and YH assisted in literature research and writing the manuscript. EO supervised and revised the manuscript. All authors reviewed the manuscript and approved the final version.
Funding
University of Yamanashi and Tokyo Metropolitan Institute of Medical Science support work and collaborative research from HI and YH. Research in EO’s group along with BK was supported by NIAAA-NIH grant AA027909 and William Paterson University and the Dean of COSH, Venkat Sharma. The COSH funds support students and the Animal Laboratory facility.
Conflict of Interest
The authors declare that the research was conducted in the absence of any commercial or financial relationships that could be construed as a potential conflict of interest.
Publisher’s Note
All claims expressed in this article are solely those of the authors and do not necessarily represent those of their affiliated organizations, or those of the publisher, the editors and the reviewers. Any product that may be evaluated in this article, or claim that may be made by its manufacturer, is not guaranteed or endorsed by the publisher.
Abbreviations
6-OHDA, 6-hydroxydopamine; AC, adenylyl cyclase; AD, Alzheimer’s disease; AEA, anandamide; AI, artificial intelligence; ALS, amyotrophic lateral sclerosis; AN, anorexia nervosa; APP, amyloid precursor protein; ASD, autism spectrum disorder; A β, β -amyloid; BCP, β -caryophyllene; BN, bulimia nervosa; CB1R, cannabinoid type 1 receptor; CB2R, cannabinoid type 2 receptor; CMS, chronic mild stress; CNS, central nervous system; CPP, conditioned place preference; D1Rs, dopamine type 1 receptors; EAE, experimental autoimmune encephalomyelitis; eCBome, endocannabinoidome; eCBs, endocannabinoids; ECS, endocannabinoid system; ERK, ½ extracellular signal-regulated kinase; FDA, food and drug administration; GIT, gastrointestinal tract; GPCRs, G-protein coupled receptors; HD, Huntington’s disease; JNK, Jun N-terminal protein kinase; Kir, inwardly rectifying potassium currents; LPS, lipopolysaccharide; MAPK, mitogen-activated protein kinase; MDA7, 1-((3-benzyl-3-methyl-2,3-dihydro-1-benzofuran-6-yl) carbonyl) piperidine; MPTP, 1-methyl-4-phenyl-1,2,3,6-tetrahydropyridine; MS, multiple sclerosis; NAc, nucleus accumbens; NITyr, N-linoleyltyrosine; NMDA, N-methyl -D-aspartate; PD, Parkinson’s disease; PEA, palmitoyl ethanolamide; PI3K, phosphoinositide 3-kinase; PPI, pre-pulse inhibition; PTZ, pentylenetetrazol; ROT, rotenone; TBI, traumatic brain injury; TH, tyrosine hydroxylase; TMEV- IDD, Theiler murine encephalomyelitis virus- induced demyelinating disease; VTA, ventral tegmental area; Δ 9-THC, delta-9-tetrahydrocannabinol.
References
1. Van Sickle MD, Duncan M, Kingsley PJ, Mouihate A, Urbani P, Mackie K, et al. Neuroscience: identification and functional characterization of brainstem cannabinoid CB2 receptors. Science. (2005) 310:329–32. doi: 10.1126/science.1115740
2. Li X, Hua T, Vemuri K, Ho JH, Wu Y, Wu L, et al. Crystal structure of the human cannabinoid receptor CB2. Cell. (2019) 176:459–467.e13. doi: 10.1016/j.cell.2018.12.011
3. Terzian AL, Drago F, Wotjak CT, Micale V. The dopamine and cannabinoid interaction in the modulation of emotions and cognition: assessing the role of cannabinoid CB1 receptor in neurons expressing dopamine D1 receptors. Front Behav Neurosci. (2011) 5:49. doi: 10.3389/fnbeh.2011.00049
4. Micale V, Stepan J, Jurik A, Pamplona FA, Marsch R, Drago F, et al. Extinction of avoidance behavior by safety learning depends on endocannabinoid signaling in the hippocampus. J Psychiatr Res. (2017) 90:46–59. doi: 10.1016/j.jpsychires.2017.02.002
5. Di Marzo V. The endocannabinoidome as a substrate for noneuphoric phytocannabinoid action and gut microbiome dysfunction in neuropsychiatric disorders. Dialogues Clin Neurosci. (2020) 22:259–69. doi: 10.31887/DCNS.2020.22.3/VDIMARZO
6. Pertwee RG, Ross RA. Cannabinoid receptors and their ligands. Prostaglandins Leukot Essent Fat Acids. (2002) 66:101–21. doi: 10.1054/plef.2001.0341
7. Scotter EL, Abood ME, Glass M. The endocannabinoid system as a target for the treatment of neurodegenerative disease. Br J Pharmacol. (2010) 160:480–98. doi: 10.1111/j.1476-5381.2010.00735.x
8. Munro S, Thomas KL, Abu-Shaar M. Molecular characterization of a peripheral receptor for cannabinoids. Nature. (1993) 365:61–5. doi: 10.1038/365061a0
9. Galiègue S, Mary S, Marchand J, Dussossoy D, Carrière D, Carayon P, et al. Expression of central and peripheral cannabinoid receptors in human immune tissues and leukocyte subpopulations. Eur J Biochem. (1995) 232:54–61. doi: 10.1111/j.1432-1033.1995.tb20780.x
10. Brown SM, Wager-Miller J, Mackie K. Cloning and molecular characterization of the rat CB2 cannabinoid receptor. Biochim Biophys Acta. (2002) 1576:255–64. doi: 10.1016/S0167-4781(02)00341-X
11. Griffin G, Wray EJ, Tao Q, McAllister SD, Rorrer WK, Aung M, et al. Evaluation of the cannabinoid CB2 receptor-selective antagonist, SR144528: further evidence for cannabinoid CB2 receptor absence in the rat central nervous system. Eur J Pharmacol. (1999) 377:117–25. doi: 10.1016/S0014-2999(99)00402-1
12. Poso A, Huffman JW. Targeting the cannabinoid CB2 receptor: modelling and structural determinants of CB2 selective ligands. Br J Pharmacol. (2008) 153:335–46. doi: 10.1038/sj.bjp.0707567
13. Brusco A, Tagliaferro P, Saez T, Onaivi ES. Postsynaptic localization of CB2 cannabinoid receptors in the rat hippocampus. Synapse. (2008) 62:944–9. doi: 10.1002/syn.20569
14. Liu QR, Canseco-Alba A, Zhang HY, Tagliaferro P, Chung M, Dennis E, et al. Cannabinoid type 2 receptors in dopamine neurons inhibits psychomotor behaviors, alters anxiety, depression and alcohol preference. Sci Rep. (2017) 7:17410. doi: 10.1038/s41598-017-17796-y
15. Maroso M, Szabo GG, Kim HK, Alexander A, Bui AD, Lee SH, et al. Cannabinoid control of learning and memory through HCN channels. Neuron. (2016) 89:1059–73. doi: 10.1016/j.neuron.2016.01.023
16. Di Chiara G, Bassareo V, Fenu S, De Luca MA, Spina L, Cadoni C, et al. Dopamine and drug addiction: the nucleus accumbens shell connection. Neuropharmacology. (2004) 47:227–41. doi: 10.1016/j.neuropharm.2004.06.032
17. Marsicano G, Lutz B. Expression of the cannabinoid receptor CB1 in distinct neuronal subpopulations in the adult mouse forebrain. Eur J Neurosci. (1999) 11:4213–25. doi: 10.1046/j.1460-9568.1999.00847.x
18. Ogawa G, Tius MA, Zhou H, Nikas SP, Halikhedkar A, Mallipeddi S, et al. 3′-functionalized adamantyl cannabinoid receptor probes. J Med Chem. (2015) 58:3104–16. doi: 10.1021/jm501960u
19. Alhouayek M, Muccioli GG. The endocannabinoid system in inflammatory bowel diseases: from pathophysiology to therapeutic opportunity. Trends Mol Med. (2012) 18:615–25. doi: 10.1016/j.molmed.2012.07.009
20. Howlett AC. Cannabinoid receptor signaling. Handb Exp Pharmacol. (2005) 168:53–79. doi: 10.1007/3-540-26573-2-2
21. Glass M, Felder CC. Concurrent stimulation of cannabinoid CB1 and dopamine D2 receptors augments cAMP accumulation in striatal neurons: evidence for a G(s) linkage to the CB1 receptor. J Neurosci. (1997) 17:5327–33. doi: 10.1523/jneurosci.17-14-05327.1997
22. Turu G, Hunyady L. Signal transduction of the CB1 cannabinoid receptor. J Mol Endocrinol. (2010) 44:75–85. doi: 10.1677/JME-08-0190
23. Sanchez-Zavaleta R, Cotes H, Avalos-Fuentes JA, Garcia U, Vila JS, Erlij D, et al. Presynaptic cannabinoid CB2 receptors modulate [3H]-glutamate release at subthalamo-nigral terminals of the rat. Synapse. (2018) 72:e22061. doi: 10.1002/syn.22061
24. Llorente-Berzal A, Terzian ALB, Di Marzo V, Micale V, Viveros MP, Wotjak CT. 2-AG promotes the expression of conditioned fear via cannabinoid receptor type 1 on GABAergic neurons. Psychopharmacology (Berl). (2015) 232:2811–25. doi: 10.1007/s00213-015-3917-y
25. Onaivi ES, Leonard CM, Ishiguro H, Zhang PW, Lin Z, Akinshola BE, et al. Endocannabinoids and cannabinoid receptor genetics. Prog Neurobiol. (2002) 66:307–44. doi: 10.1016/S0301-0082(02)00007-2
26. Canseco-Alba A, Sanabria B, Hammouda M, Bernadin R, Mina M, Liu QR, et al. Cell-type specific deletion of CB2 cannabinoid receptors in dopamine neurons induced hyperactivity phenotyp: possible relevance to attention-deficit hyperactivity disorder. Front Psychiatry. (2022) 12:803394. doi: 10.3389/fpsyt.2021.803394
27. Joshi N, Onaivi ES. Endocannabinoid system components: overview and tissue distribution. Adv Exp Med Biol. (2019) 1162:1–12. doi: 10.1007/978-3-030-21737-2_1
28. Jordan CJ, Xi ZX. Progress in brain cannabinoid CB 2 receptor research: from genes to behavior. Neurosci Biobehav Rev. (2019) 98:208–20. doi: 10.1016/j.neubiorev.2018.12.026
29. Schatz AR, Lee M, Condie RB, Pulaski JT, Kaminski NE. Cannabinoid receptors CB1 and CB2: a characterization of expression and adenylate cyclase modulation within the immune system. Toxicol Appl Pharmacol. (1997) 142:278–87. doi: 10.1006/taap.1996.8034
30. McCoy KL, Matveyeva M, Carlisle SJ, Cabral GA. Cannabinoid inhibition of the processing of intact lysozyme by macrophages: evidence for CB2 receptor participation. J Pharmacol Exp Ther. (1999) 289:1620–5.
31. Buckley NE, McCoy KL, Mezey É, Bonner T, Zimmer A, Felder CC, et al. Immunomodulation by cannabinoids is absent in mice deficient for the cannabinoid CB2 receptor. Eur J Pharmacol. (2000) 396:141–9. doi: 10.1016/S0014-2999(00)00211-9
32. Gong JP, Onaivi ES, Ishiguro H, Liu QR, Tagliaferro PA, Brusco A, et al. Cannabinoid CB2 receptors: immunohistochemical localization in rat brain. Brain Res. (2006) 1071:10–23. doi: 10.1016/j.brainres.2005.11.035
33. Xi ZX, Peng XQ, Li X, Song R, Zhang HY, Liu QR, et al. Brain cannabinoid CB2 receptors modulate cocaine’s actions in mice. Nat Neurosci. (2011) 14:1160–8. doi: 10.1038/nn.2874
34. Onaivi ES. Neuropsychobiological evidence for the functional presence and expression of cannabinoid CB2 receptors in the brain. Neuropsychobiology. (2007) 54:231–46. doi: 10.1159/000100778
35. Onaivi ES, Ishiguro H, Gong JP, Patel S, Perchuk A, Meozzi PA, et al. Discovery of the presence and functional expression of cannabinoid CB2 receptors in brain. Ann N Y Acad Sci. (2006) 1074:514–36. doi: 10.1196/annals.1369.052
36. Onaivi ES, Ishiguro H, Gong JP, Patel S, Meozzi PA, Myers L, et al. Functional expression of brain neuronal CB2 cannabinoid receptors are involved in the effects of drugs of abuse and in depression. Ann N Y Acad Sci. (2008) 1139:434–49. doi: 10.1196/annals.1432.036
37. Onaivi ES, Ishiguro H, Gong JP, Patel S, Meozzi PA, Myers L, et al. Brain neuronal CB2 cannabinoid receptors in drug abuse and depression: from mice to human subjects. PLoS One. (2008) 3:e1640. doi: 10.1371/journal.pone.0001640
38. Liu QR, Pan CH, Hishimoto A, Li CY, Xi ZX, Llorente-Berzal A, et al. Species differences in cannabinoid receptor 2 (CNR2 gene): identification of novel human and rodent CB2 isoforms, differential tissue expression and regulation by cannabinoid receptor ligands. Genes Brain Behav. (2009) 8:519–30. doi: 10.1111/j.1601-183X.2009.00498.x
39. Skaper SD, Buriani A, Dal Toso R, Petrelii L, Romanello S, Facci L, et al. The ALIAmide palmitoylethanolamide and cannabinoids, but not anandamide, are protective in a delayed postglutamate paradigm of excitotoxic death in cerebellar granule neurons. Proc Natl Acad Sci USA. (1996) 93:3984–9. doi: 10.1073/pnas.93.9.3984
40. Viscomi MT, Oddi S, Latini L, Pasquariello N, Florenzano F, Bernardi G, et al. Selective CB2 receptor agonism protects central neurons from remote axotomy-induced apoptosis through the PI3K/Akt pathway. J Neurosci. (2009) 29:4564–70. doi: 10.1523/JNEUROSCI.0786-09.2009
41. Navarrete F, Pérez-Ortiz JM, Manzanares J. Cannabinoid CB 2 receptor-mediated regulation of impulsive-like behaviour in DBA/2 mice. Br J Pharmacol. (2012) 165:260–73. doi: 10.1111/j.1476-5381.2011.01542.x
42. García-Gutiérrez MS, García-Bueno B, Zoppi S, Leza JC, Manzanares J. Chronic blockade of cannabinoid CB 2 receptors induces anxiolytic-like actions associated with alterations in GABA A receptors. Br J Pharmacol. (2012) 165:951–64. doi: 10.1111/j.1476-5381.2011.01625.x
43. Lanciego JL, Barroso-Chinea P, Rico AJ, Conte-Perales L, Callén L, Roda E, et al. Expression of the mRNA coding the cannabinoid receptor 2 in the pallidal complex of Macaca fascicularis. J Psychopharmacol. (2011) 25:97–104. doi: 10.1177/0269881110367732
44. Lu Q, Straiker A, Lu Q, Maguire G. Expression of CB2 cannabinoid receptor mRNA in adult rat retina. Vis Neurosci. (2000) 17:91–5. doi: 10.1017/S0952523800171093
45. Onaivi ES, Ishiguro H, Sejal P, Meozzi PA, Myers L, Tagliaferro P, et al. Methods to study the behavioral effects and expression of CB2 cannabinoid receptor and its gene transcripts in the chronic mild stress model of depression. Methods Mol Med. (2006) 123:291–8. doi: 10.1385/1-59259-999-0:291
46. Chin CL, Tovcimak AE, Hradil VP, Seifert TR, Hollingsworth PR, Chandran P, et al. Differential effects of cannabinoid receptor agonists on regional brain activity using pharmacological MRI. Br J Pharmacol. (2008) 153:367–79. doi: 10.1038/sj.bjp.0707506
47. Brusco A, Tagliaferro PA, Saez T, Onaivi ES. Ultrastructural localization of neuronal brain CB2 cannabinoid receptors. Ann N Y Acad Sci. (2008) 1139:450–7. doi: 10.1196/annals.1432.037
48. Sherwood TA, Nong L, Agudelo M, Newton C, Widen R, Klein TW. Identification of transcription start sites and preferential expression of select CB2 transcripts in mouse and human B lymphocytes. J Neuroimmune Pharmacol. (2009) 4:476–88. doi: 10.1007/s11481-009-9169-z
49. Liu QR, Aseer KR, Yao Q, Zhong X, Ghosh P, O’Connell JF, et al. Anti-inflammatory and pro-autophagy effects of cannabinoid receptor CB2R: possibility of modulation in type 1 diabetes. Front Pharmacol. (2022) 12:809965. doi: 10.3398/fphar.2021.809965
50. Bosier B, Muccioli GG, Hermans E, Lambert DM. Functionally selective cannabinoid receptor signalling: therapeutic implications and opportunities. Biochem Pharmacol. (2010) 80:1–12. doi: 10.1016/j.bcp.2010.02.013
51. Howlett AC, Barth F, Bonner TI, Cabral G, Casellas P, Devane WA, et al. International union of pharmacology. XXVII. Classification of cannabinoid receptors. Pharmacol Rev. (2002) 54:161–202. doi: 10.1124/pr.54.2.161
52. Roche M, Finn DP. Brain CB2 receptors: implications for neuropsychiatric disorders. Pharmaceutical. (2010) 3:2517–33. doi: 10.3390/ph3082517
53. Zhang HY, Gao M, Liu QR, Bi GH, Li X, Yang HJ, et al. Cannabinoid CB2 receptors modulate midbrain dopamine neuronal activity and dopamine-related behavior in mice. Proc Natl Acad Sci USA. (2014) 111:E5007–15. doi: 10.1073/pnas.1413210111
54. Emadi L, Jonaidi H, Amir Abad EH. The role of central CB2 cannabinoid receptors on food intake in neonatal chicks. J Comp Physiol A Neuroethol Sens Neural Behav Physiol. (2011) 197:1143–7. doi: 10.1007/s00359-011-0676-z
55. Ignatowska-Jankowska B, Jankowski MM, Swiergiel AH. Cannabidiol decreases body weight gain in rats: involvement of CB2 receptors. Neurosci Lett. (2011) 490:82–4. doi: 10.1016/j.neulet.2010.12.031
56. García-Gutiérrez MS, Manzanares J. Overexpression of CB2 cannabinoid receptors decreased vulnerability to anxiety and impaired anxiolytic action of alprazolam in mice. J Psychopharmacol. (2011) 25:111–20. doi: 10.1177/0269881110379507
57. Geresu B, Canseco-Alba A, Sanabria B, Lin Z, Liu QR, Onaivi ES, et al. Involvement of CB2 receptors in the neurobehavioral effects of Catha edulis (Vahl) Endl. (khat) in mice. Molecules. (2019) 24:3164. doi: 10.3390/molecules24173164
58. Canseco-Alba A, Schanz N, Sanabria B, Zhao J, Lin Z, Liu QR, et al. Behavioral effects of psychostimulants in mutant mice with cell-type specific deletion of CB2 cannabinoid receptors in dopamine neurons. Behav Brain Res. (2019) 360:286–97. doi: 10.1016/j.bbr.2018.11.043
59. Ortega-Alvaro A, Aracil-Fernández A, García-Gutiérrez MS, Navarrete F, Manzanares J. Deletion of CB2 cannabinoid receptor induces schizophrenia-related behaviors in mice. Neuropsychopharmacology. (2011) 36:1489–504. doi: 10.1038/npp.2011.34
60. Svíženská IH, Brázda V, Klusáková I, Dubový P. Bilateral changes of cannabinoid receptor type 2 protein and mRNA in the dorsal root ganglia of a rat neuropathic pain model. J Histochem Cytochem. (2013) 61:529–47. doi: 10.1369/0022155413491269
61. Yu SJ, Reiner D, Shen H, Wu KJ, Liu QR, Wang Y. Time-dependent protection of CB2 receptor agonist in stroke. PLoS One. (2015) 10:e0132487. doi: 10.1371/journal.pone.0132487
62. Lopez-Rodriguez AB, Acaz-Fonseca E, Viveros MP, Garcia-Segura LM. Changes in cannabinoid receptors, aquaporin 4 and vimentin expression after traumatic brain injury in adolescent male mice. Association with edema and neurological deficit. PLoS One. (2015) 10:e0128782. doi: 10.1371/journal.pone.0128782
63. Geresu B, Engidawork E. Catha edulis F. (Khat) reverses haloperidol but not morphine induced motor deficits following acute and subacute administration in mice. Ethiop Pharm J. (2012) 28:117–30. doi: 10.4314/epj.v28i2.6
64. Concannon RM, Okine BN, Finn DP, Dowd E. Differential upregulation of the cannabinoid CB2 receptor in neurotoxic and inflammation-driven rat models of Parkinson’s disease. Exp Neurol. (2015) 269:133–41. doi: 10.1016/j.expneurol.2015.04.007
65. Agudelo M, Yndart A, Morrison M, Figueroa G, Muñoz K, Samikkannu T, et al. Differential expression and functional role of cannabinoid genes in alcohol users. Drug Alcohol Depend. (2013) 133:789–93. doi: 10.1016/j.drugalcdep.2013.08.023
66. Rivera P, Miguéns M, Coria SM, Rubio L, Higuera-Matas A, Bermúdez-Silva FJ, et al. Cocaine self-administration differentially modulates the expression of endogenous cannabinoid system-related proteins in the hippocampus of Lewis vs. Fischer 344 rats. Int J Neuropsychopharmacol. (2013) 16:1277–93. doi: 10.1017/S1461145712001186
67. Chen DJ, Gao M, Gao FF, Su QX, Wu J. Brain cannabinoid receptor 2: expression, function and modulation. Acta Pharmacol Sin. (2017) 38:312–6. doi: 10.1038/aps.2016.149
68. Mechoulam R, Parker LA. The endocannabinoid system and the brain. Annu Rev Psychol. (2013) 64:21–47. doi: 10.1146/annurev-psych-113011-143739
69. Nguyen T, Thomas BF, Zhang Y. Overcoming the psychiatric side effects of the cannabinoid CB1 receptor antagonists: current approaches for therapeutics development. Curr Top Med Chem. (2019) 19:1418–35. doi: 10.2174/1568026619666190708164841
70. Moreira FA, Grieb M, Lutz B. Central side-effects of therapies based on CB1 cannabinoid receptor agonists and antagonists: focus on anxiety and depression. Best Pract Res Clin Endocrinol Metab. (2009) 23:133–44. doi: 10.1016/j.beem.2008.09.003
71. Xin Q, Xu F, Taylor DH, Zhao J, Wu J. The impact of cannabinoid type 2 receptors (CB2Rs) in neuroprotection against neurological disorders. Acta Pharmacol Sin. (2020) 41:1507–18. doi: 10.1038/s41401-020-00530-2
72. Cabanero D, Martin-Garcia E, Maldonado R. The CB2 cannabinoid receptor as a potential target in the central nervous system. Expert Opin Ther Targets. (2021) 25:659–76. doi: 10.1080/14728222.2021.1971196
73. Onaivi ES, Carpio O, Ishiguro H, Schanz N, Uhl GR, Benno R. Behavioral effects of CB2 cannabinoid receptor activation and its influence on food and alcohol consumption. Ann N Y Acad Sci. (2008) 1139:426–33. doi: 10.1196/annals.1432.035
74. Ishiguro H, Iwasaki S, Teasenfitz L, Higuchi S, Horiuchi Y, Saito T, et al. Involvement of cannabinoid CB2 receptor in alcohol preference in mice and alcoholism in humans. Pharmacogenomics J. (2007) 7:380–5. doi: 10.1038/sj.tpj.6500431
75. Aso E, Ferrer I. Cannabinoids for treatment of Alzheimer’s disease: moving toward the clinic. Front Pharmacol. (2014) 5:37. doi: 10.3389/fphar.2014.00037
76. Basu S, Dittel BN. Unraveling the complexities of cannabinoid receptor 2 (CB2) immune regulation in health and disease. Immunol Res. (2011) 51:26–38. doi: 10.1007/s12026-011-8210-5
77. Malfitano AM, Basu S, Maresz K, Bifulco M, Dittel BN. What we know and do not know about the cannabinoid receptor 2 (CB2). Semin Immunol. (2014) 26:369–79. doi: 10.1016/j.smim.2014.04.002
78. Lutz B, Marsicano G, Maldonado R, Hillard CJ. The endocannabinoid system in guarding against fear, anxiety and stress. Nat Rev Neurosci. (2015) 16:705–18. doi: 10.1038/nrn4036
79. Micale V, Di Marzo V, Sulcova A, Wotjak CT, Drago F. Endocannabinoid system and mood disorders: priming a target for new therapies. Pharmacol Ther. (2013) 138:18–37. doi: 10.1016/j.pharmthera.2012.12.002
80. García-Gutiérrez MS, Pérez-Ortiz JM, Gutiérrez-Adán A, Manzanares J. Depression-resistant endophenotype in mice overexpressing cannabinoid CB 2 receptors. Br J Pharmacol. (2010) 160:1773–84. doi: 10.1111/j.1476-5381.2010.00819.x
81. Valenzano KJ, Tafesse L, Lee G, Harrison JE, Boulet JM, Gottshall SL, et al. Pharmacological and pharmacokinetic characterization of the cannabinoid receptor 2 agonist, GW405833, utilizing rodent models of acute and chronic pain, anxiety, ataxia and catalepsy. Neuropharmacology. (2005) 48:658–72. doi: 10.1016/j.neuropharm.2004.12.008
82. Ishiguro H, Horiuchi Y, Tabata K, Liu QR, Arinami T, Onaivi ES. Cannabinoid CB2 receptor gene and environmental interaction in the development of psychiatric disorders. Molecules. (2018) 23:1836. doi: 10.3390/molecules23081836
83. Lester KJ, Coleman JRI, Roberts S, Keers R, Breen G, Bögels S, et al. Genetic variation in the endocannabinoid system and response to cognitive behavior therapy for child anxiety disorders. Am J Med Genet Part B Neuropsychiatr Genet. (2017) 174:144–55. doi: 10.1002/ajmg.b.32467
84. Micale V, Tabiova K, Kucerova J, Drago F. Role of the endocannabinoid system in depression: from preclinical to clinical evidence. In: P Campolongo, L Fattore editors. Cannabinoid Modulation of Emotion, Memory, and Motivation. (New York, NY: Springer) (2015). p. 97–129. doi: 10.1007/978-1-4939-2294-9_5
85. Hu B, Doods H, Treede RD, Ceci A. Depression-like behaviour in rats with mononeuropathy is reduced by the CB2-selective agonist GW405833. Pain. (2009) 143:206–12. doi: 10.1016/j.pain.2009.02.018
86. Willner P, Mitchell PJ. The validity of animal models of predisposition to depression. Behav Pharmacol. (2002) 13:169–88. doi: 10.1097/00008877-200205000-00001
87. Willner P. Chronic mild stress (CMS) revisited: consistency and behavioural– neurobiological concordance in the effects of CMS. Neuropsychobiology. (2005) 52:90–110. doi: 10.1159/000087097
88. Papp M, Moryl E, Willner P. Pharmacological validation of the chronic mild stress model of depression. Eur J Pharmacol. (1996) 296:129–36. doi: 10.1016/0014-2999(95)00697-4
89. García-Gutiérrez MS, Navarrete F, Navarro G, Reyes-Resina I, Franco R, Lanciego JL, et al. Alterations in gene and protein expression of cannabinoid CB 2 and GPR55 receptors in the dorsolateral prefrontal cortex of suicide victims. Neurotherapeutics. (2018) 15:796–806. doi: 10.1007/s13311-018-0610-y
90. Salort G, Hernández-Hernández E, García-Fuster MJ, García-Sevilla JA. Regulation of cannabinoid CB1 and CB2 receptors, neuroprotective mTOR and pro-apoptotic JNK1/2 kinases in postmortem prefrontal cortex of subjects with major depressive disorder. J Affect Disord. (2020) 276:626–35. doi: 10.1016/j.jad.2020.07.074
91. Bara A, Ferland JMN, Rompala G, Szutorisz H, Hurd YL. Cannabis and synaptic reprogramming of the developing brain. Nat Rev Neurosci. (2021) 22:423–38. doi: 10.1038/s41583-021-00465-5
92. Kucerova J, Tabiova K, Drago F, Micale V. Therapeutic potential of cannabinoids in schizophrenia. Recent Pat CNS Drug Discov. (2014) 9:13–25. doi: 10.2174/1574889809666140307115532
93. Ellenbroek BA, De Bruin NMWJ, Van Den Kroonenburg PTJM, Van Luijtelaar ELJM, Cools AR. The effects of early maternal deprivation on auditory information processing in adult wistar rats. Biol Psychiatry. (2004) 55:701–7. doi: 10.1016/j.biopsych.2003.10.024
94. Garner B, Wood SJ, Pantelis C, van den Buuse M. Early maternal deprivation reduces prepulse inhibition and impairs spatial learning ability in adulthood: no further effect of post-pubertal chronic corticosterone treatment. Behav Brain Res. (2007) 176:323–32. doi: 10.1016/j.bbr.2006.10.020
95. Suárez J, Llorente R, Romero-Zerbo SY, Mateos B, Bermúdez-Silva FJ, De Fonseca FR, et al. Early maternal deprivation induces gender-dependent changes on the expression of hippocampal CB1 and CB2 cannabinoid receptors of neonatal rats. Hippocampus. (2009) 19:623–32. doi: 10.1002/hipo.20537
96. García-Gutiérrez MS, Navarrete F, Gasparyan A, Manzanares J. Therapeutic potential of the cannabinoid receptor 2 in neuropsychiatry. Explor Neuroprotective Ther. (2021) 1:55–71. doi: 10.37349/ent.2021.00006
97. Errico F, Rossi S, Napolitano F, Catuogno V, Topo E, Fisone G, et al. D-aspartate prevents corticostriatal long-term depression and attenuates schizophrenia-like symptoms induced by amphetamine and MK-801. J Neurosci. (2008) 28:10404–14. doi: 10.1523/JNEUROSCI.1618-08.2008
98. Ishiguro H, Horiuchi Y, Ishikawa M, Koga M, Imai K, Suzuki Y, et al. Brain cannabinoid CB2 receptor in schizophrenia. Biol Psychiatry. (2010) 67:974–82. doi: 10.1016/j.biopsych.2009.09.024
99. Khella R, Short JL, Malone DT. CB2 receptor agonism reverses MK-801-induced disruptions of prepulse inhibition in mice. Psychopharmacology (Berl). (2014) 231:3071–87. doi: 10.1007/s00213-014-3481-x
100. García-Gutiérrez MS, Ortega-Álvaro A, Busquets-García A, Pérez-Ortiz JM, Caltana L, Ricatti MJ, et al. Synaptic plasticity alterations associated with memory impairment induced by deletion of CB2 cannabinoid receptors. Neuropharmacology. (2013) 73:388–96. doi: 10.1016/j.neuropharm.2013.05.034
101. Foster DJ, Wilson JM, Remke DH, Mahmood MS, Uddin MJ, Wess J, et al. Antipsychotic-like effects of M4 positive allosteric modulators are mediated by CB2 receptor-dependent inhibition of dopamine release. Neuron. (2016) 91:1244–52. doi: 10.1016/j.neuron.2016.08.017
102. Hill KP, Gold MS, Nemeroff CB, McDonald W, Grzenda A, Widge AS, et al. Risks and benefits of cannabis and cannabinoids in psychiatry. Am J Psychiatry. (2022) 179:98–109. doi: 10.1176/appi.ajp.2021.21030320
103. Kuhns L, Kroon E, Colyer-Patel K, Cousijn J. Associations between cannabis use disorder, mood disorders: longitudinal, genetic, and neurocognitive evidence. Psychopharmacology. (2021) 239:1231–49. doi: 10.1007/s00213-021-06001-8
104. Plescia F, Brancato A, Marino RAM, Vita C, Navarra M, Cannizzaro C. Effect of acetaldehyde intoxication and withdrawal on NPY expression: focus on endocannabinoidergic system involvement. Front Psychiatry. (2014) 5:138. doi: 10.3389/fpsyt.2014.00138
105. Plescia F, Brancato A, Marino RAM, Cannizzaro C. Acetaldehyde as a drug of abuse: insight into AM281 administration on operant-conflict paradigm in rats. Front Behav Neurosci. (2013) 7:64. doi: 10.3389/fnbeh.2013.00064
106. Brancato A, Castelli V, Lavanco G, Marino RAM, Cannizzaro C. In utero Δ9-tetrahydrocannabinol exposure confers vulnerability towards cognitive impairments and alcohol drinking in the adolescent offspring: is there a role for neuropeptide Y? J Psychopharmacol. (2020) 34:663–79. doi: 10.1177/0269881120916135
107. Manzanares J, Cabañero D, Puente N, García-Gutiérrez MS, Grandes P, Maldonado R. Role of the endocannabinoid system in drug addiction. Biochem Pharmacol. (2018) 157:108–21. doi: 10.1016/j.bcp.2018.09.013
108. Lavanco G, Castelli V, Brancato A, Tringali G, Plescia F, Cannizzaro C. The endocannabinoid-alcohol crosstalk: recent advances on a bi-faceted target. Clin Exp Pharmacol Physiol. (2018) 45:889–96. doi: 10.1111/1440-1681.12967
109. Aracil-Fernández A, Trigo JM, García-Gutiérrez MS, Ortega-Álvaro A, Ternianov A, Maldonado R, et al. Decreased cocaine motor sensitisation and self-administration in mice overexpressing cannabinoid CB2 receptors. Eur Neuropsychopharmacol. (2011) 21:S586–7. doi: 10.1016/s0924-977x(11)70959-2
110. Lopes JB, Bastos JR, Costa RB, Aguiar DC, Moreira FA. The roles of cannabinoid CB1 and CB2 receptors in cocaine-induced behavioral sensitization and conditioned place preference in mice. Psychopharmacology (Berl). (2020) 237:385–94. doi: 10.1007/s00213-019-05370-5
111. Blanco-Calvo E, Rivera P, Arrabal S, Vargas A, Pavón FJ, Serrano A, et al. Pharmacological blockade of either cannabinoid CB1 or CB2 receptors prevents both cocaine-induced conditioned locomotion and cocaine-induced reduction of cell proliferation in the hippocampus of adult male rat. Front Integr Neurosci. (2014) 7:106. doi: 10.3389/fnint.2013.00106
112. He XH, Galaj E, Bi GH, He Y, Hempel B, Wang YL, et al. β-caryophyllene, an FDA-approved food additive, inhibits methamphetamine-taking and methamphetamine-seeking behaviors possibly via CB2 and non-CB2 receptor mechanisms. Front Pharmacol. (2021) 12:722476. doi: 10.3389/fphar.2021.722476
113. Ortega-Álvaro A, Ternianov A, Aracil-Fernández A, Navarrete F, García-Gutiérrez MS, Manzanares J. Role of cannabinoid CB2 receptor in the reinforcing actions of ethanol. Addict Biol. (2015) 20:43–55. doi: 10.1111/adb.12076
114. Al Mansouri S, Ojha S, Al Maamari E, Al Ameri M, Nurulain SM, Bahi A. The cannabinoid receptor 2 agonist, β-caryophyllene, reduced voluntary alcohol intake and attenuated ethanol-induced place preference and sensitivity in mice. Pharmacol Biochem Behav. (2014) 124:260–8. doi: 10.1016/j.pbb.2014.06.025
115. Pradier B, Erxlebe E, Markert A, Rácz I. Interaction of cannabinoid receptor 2 and social environment modulates chronic alcohol consumption. Behav Brain Res. (2015) 287:163–71. doi: 10.1016/j.bbr.2015.03.051
116. Sánchez-Marín L, Flores-López M, Pastor A, Gavito AL, Suárez J, de la Torre R, et al. Acute stress and alcohol exposure during adolescence result in an anxious phenotype in adulthood: role of altered glutamate/endocannabinoid transmission mechanisms. Prog Neuro Psychopharmacol Biol Psychiatry. (2022) 113:110460. doi: 10.1016/j.pnpbp.2021.110460
117. Kaplan JS, Stella N, Catterall WA, Westenbroek RE. Cannabidiol attenuates seizures and social deficits in a mouse model of Dravet syndrome. Proc Natl Acad Sci USA. (2017) 114:11229–34. doi: 10.1073/pnas.1711351114
118. Navarrete F, García-Gutiérrez MS, Gasparyan A, Navarro D, Manzanares J. CB2 receptor involvement in the treatment of substance use disorders. Biomolecules. (2021) 11:1556. doi: 10.3390/biom11111556
119. Do LLTN. American psychiatric association diagnostic and statistical manual of mental disorders (DSM-IV). Encycl Child Behav Dev. (2011) 2011:84–5. doi: 10.1007/978-0-387-79061-9_113
120. Chakrabarti B, Persico A, Battista N, Maccarrone M. Endocannabinoid signaling in Autism. Neurotherapeutics. (2015) 12:837–47. doi: 10.1007/s13311-015-0371-9
121. Siniscalco D, Sapone A, Giordano C, Cirillo A, De Magistris L, Rossi F, et al. Cannabinoid receptor type 2, but not type 1, is up-regulated in peripheral blood mononuclear cells of mystery affected by autistic disorders. J Autism Dev Disord. (2013) 43:2686–95. doi: 10.1007/s10803-013-1824-9
122. Pietropaolo S, Marsicano G. The role of the endocannabinoid system as a therapeutic target for autism spectrum disorder: lessons from behavioral studies on mouse models. Neurosci Biobehav Rev. (2021) 132:664–78. doi: 10.1016/j.neubiorev.2021.11.031
123. Dietert RR, Dietert JM, DeWitt JC. Environmental risk factors for autism. Emerg Health Threats J. (2011) 4:7111. doi: 10.3402/ehtj.v4i0.7111
124. Chaste P, Leboyer M. Autism risk factors: genes, environment, and gene-environment interactions. Dialogues Clin Neurosci. (2012) 14:281–92. doi: 10.31887/dcns.2012.14.3/pchaste
125. Modabbernia A, Velthorst E, Reichenberg A. Environmental risk factors for autism: an evidence-based review of systematic reviews and meta-analyses. Mol Autism. (2017) 8:13. doi: 10.1186/s13229-017-0121-4
126. Foltin RW, Brady JV, Fischman MW. Behavioral analysis of marijuana effects on food intake in humans. Pharmacol Biochem Behav. (1986) 25:577–82. doi: 10.1016/0091-3057(86)90144-9
127. Bedi G, Foltin RW, Gunderson EW, Rabkin J, Hart CL, Comer SD, et al. Efficacy and tolerability of high-dose dronabinol maintenance in HIV-positive marijuana smokers: a controlled laboratory study. Psychopharmacology (Berl). (2010) 212:675–86. doi: 10.1007/s00213-010-1995-4
128. Haney M, Rabkin J, Gunderson E, Foltin RW. Dronabinol and marijuana in HIV+ marijuana smokers: acute effects on caloric intake and mood. Psychopharmacology (Berl). (2005) 181:170–8. doi: 10.1007/s00213-005-2242-2
129. Farag YMK, Gaballa MR. Diabesity: an overview of a rising epidemic. Nephrol Dial Transplant. (2011) 26:28–35. doi: 10.1093/ndt/gfq576
130. Ng ACT, Delgado V, Borlaug BA, Bax JJ. Diabesity: the combined burden of obesity and diabetes on heart disease and the role of imaging. Nat Rev Cardiol. (2021) 18:291–304. doi: 10.1038/s41569-020-00465-5
131. Rumińska A, Dobrzyń A. [The endocannabinoid system and its role in regulation of metabolism in peripheral tissues]. Postepy Biochem. (2012) 58:127–34.
132. Watkins BA, Kim J. The endocannabinoid system: directing eating behavior and macronutrient metabolism. Front Psychol. (2014) 5:1506. doi: 10.3389/fpsyg.2014.01506
133. Di Marzo V, Petrosino S. Endocannabinoids and the regulation of their levels in health and disease. Curr Opin Lipidol. (2007) 18:129–40. doi: 10.1097/MOL.0b013e32803dbdec
134. Schwartz MW, Woods SC, Porte D, Seeley RJ, Baskin DG. Central nervous system control of food intake. Nature. (2000) 404:661–71. doi: 10.1038/35007534
135. Monteleone P, Matias I, Martiadis V, De Petrocellis L, Maj M, Di Marzo V. Blood levels of the endocannabinoid anandamide are increased in anorexia nervosa and in binge-eating disorder, but not in bulimia nervosa. Neuropsychopharmacology. (2005) 30:1216–21. doi: 10.1038/sj.npp.1300695
136. Frieling H, Albrecht H, Jedtberg S, Gozner A, Lenz B, Wilhelm J, et al. Elevated cannabinoid 1 receptor mRNA is linked to eating disorder related behavior and attitudes in females with eating disorders. Psychoneuroendocrinology. (2009) 34:620–4. doi: 10.1016/j.psyneuen.2008.10.014
137. Ishiguro H, Carpio O, Horiuchi Y, Shu A, Higuchi S, Schanz N, et al. A nonsynonymous polymorphism in cannabinoid CB2 receptor gene is associated with eating disorders in humans and food intake is modified in mice by its ligands. Synapse. (2010) 64:92–6. doi: 10.1002/syn.20714
138. Ahmad A, Ali T, Park HY, Badshah H, Rehman SU, Kim MO. Neuroprotective effect of fisetin against amyloid-beta-induced cognitive/synaptic dysfunction, neuroinflammation, and neurodegeneration in adult mice. Mol Neurobiol. (2017) 54:2269–85. doi: 10.1007/s12035-016-9795-4
139. Brancato A, Lavanco G, Cavallaro A, Plescia F, Cannizzaro C. The use of the emotional-object recognition as an assay to assess learning and memory associated to an aversive stimulus in rodents. J Neurosci Methods. (2016) 274:106–15. doi: 10.1016/j.jneumeth.2016.09.010
140. Brancato A, Cavallaro A, Lavanco G, Plescia F, Cannizzaro C. Reward-related limbic memory and stimulation of the cannabinoid system: an upgrade in value attribution? J Psychopharmacol. (2018) 32:204–14. doi: 10.1177/0269881117725683
141. Schmöle AC, Lundt R, Toporowski G, Hansen JN, Beins E, Halle A, et al. Cannabinoid receptor 2-deficiency ameliorates disease symptoms in a mouse model with Alzheimer’s disease-like pathology. J Alzheimers Dis. (2018) 64:379–92. doi: 10.3233/JAD-180230
142. Vázquez C, Tolón RM, Grande MT, Caraza M, Moreno M, Koester EC, et al. Endocannabinoid regulation of amyloid-induced neuroinflammation. Neurobiol Aging. (2015) 36:3008–19. doi: 10.1016/j.neurobiolaging.2015.08.003
143. Van Der Stelt M, Mazzola C, Esposito G, Matias I, Petrosino S, De Filippis D, et al. Endocannabinoids and β-amyloid-induced neurotoxicity in vivo: effect of pharmacological elevation of endocannabinoid levels. Cell Mol Life Sci. (2006) 63:1410–24. doi: 10.1007/s00018-006-6037-3
144. Benito C, Núñez E, Tolón RM, Carrier EJ, Rábano A, Hillard CJ, et al. Cannabinoid CB2 receptors and fatty acid amide hydrolase are selectively overexpressed in neuritic plaque-associated glia in Alzheimer’s disease brains. J Neurosci. (2003) 23:11136–41. doi: 10.1523/jneurosci.23-35-11136.2003
145. Ramírez BG, Blázquez C, Gómez Del Pulgar T, Guzmán M, De Ceballos ML. Prevention of Alzheimer’s disease pathology by cannabinoids: neuroprotection mediated by blockade of microglial activation. J Neurosci. (2005) 25:1904–13. doi: 10.1523/JNEUROSCI.4540-04.2005
146. Solas M, Francis PT, Franco R, Ramirez MJ. CB2 receptor and amyloid pathology in frontal cortex of Alzheimer’s disease patients. Neurobiol Aging. (2013) 34:805–8. doi: 10.1016/j.neurobiolaging.2012.06.005
147. Koppel J, Vingtdeux V, Marambaud P, d’Abramo C, Jimenez H, Stauber M, et al. CB2 receptor deficiency increases amyloid pathology and alters tau processing in a transgenic mouse model of Alzheimer’s disease. Mol Med. (2014) 20:29–36. doi: 10.2119/molmed.2013.00140.revised
148. Aso E, Juvés S, Maldonado R, Ferrer I. CB2 cannabinoid receptor agonist ameliorates Alzheimer-like phenotype in AβPP/PS1 mice. J Alzheimers Dis. (2013) 35:847–58. doi: 10.3233/JAD-130137
149. Navarro-Dorado J, Villalba N, Prieto D, Brera B, Martín-Moreno AM, Tejerina T, et al. Vascular dysfunction in a transgenic model of Alzheimer’s disease: effects of CB1R and CB2R cannabinoid agonists. Front Neurosci. (2016) 10:422. doi: 10.3389/fnins.2016.00422
150. Köfalvi A, Lemos C, Martín-Moreno AM, Pinheiro BS, García-García L, Pozo MA, et al. Stimulation of brain glucose uptake by cannabinoid CB2 receptors and its therapeutic potential in Alzheimer’s disease. Neuropharmacology. (2016) 110:519–29. doi: 10.1016/j.neuropharm.2016.03.015
151. Long C, Zheng Q, Zhou Y, Liu Y, Gong L, Zeng Y, et al. N-linoleyltyrosine exerts neuroprotective effects in APP/PS1 transgenic mice via cannabinoid receptor-mediated autophagy. J Pharmacol Sci. (2021) 147:315–24. doi: 10.1016/j.jphs.2021.08.008
152. Rivas-Santisteban R, Lillo A, Lillo J, Rebassa J-B, Contestí JS, Saura CA, et al. Methyl-D-aspartate (n.d.) and cannabinoid CB2 receptors form functional complexes in cells of the central nervous system: insights into the therapeutic potential of neuronal and microglial NMDA receptors. Alzheimers Res Ther. (2021) 13:184. doi: 10.1186/s13195-021-00920-6
153. Li C, Shi J, Wang B, Li J, Jia H. CB2 cannabinoid receptor agonist ameliorates novel object recognition but not spatial memory in transgenic APP/PS1 mice. Neurosci Lett. (2019) 707:134286. doi: 10.1016/j.neulet.2019.134286
154. Wu J, Bie B, Yang H, Xu JJ, Brown DL, Naguib M. Activation of the CB2 receptor system reverses amyloid-induced memory deficiency. Neurobiol Aging. (2013) 34:791–804. doi: 10.1016/j.neurobiolaging.2012.06.011
155. Wu J, Hocevar M, Foss JF, Bie B, Naguib M. Activation of CB2 receptor system restores cognitive capacity and hippocampal Sox2 expression in a transgenic mouse model of Alzheimer’s disease. Eur J Pharmacol. (2017) 811:12–20. doi: 10.1016/j.ejphar.2017.05.044
156. Sheng WS, Hu S, Min X, Cabral GA, Lokensgard JR, Peterson PK. Synthetic cannabinoid WIN55,212-2 inhibits generation of inflammatory mediators by IL-1β-stimulated human astrocytes. Glia. (2005) 49:211–9. doi: 10.1002/glia.20108
157. Ehrhart J, Obregon D, Mori T, Hou H, Sun N, Bai Y, et al. Stimulation of cannabinoid receptor 2 (CB2) suppresses microglial activation. J Neuroinflammation. (2005) 2:29. doi: 10.1186/1742-2094-2-29
158. Walter L, Franklin A, Witting A, Wade C, Xie Y, Kunos G, et al. Nonpsychotropic cannabinoid receptors regulate microglial cell migration. J Neurosci. (2003) 23:1398–405. doi: 10.1523/jneurosci.23-04-01398.2003
159. Aso E, Andrés-Benito P, Carmona M, Maldonado R, Ferrer I. Cannabinoid receptor 2 participates in amyloid-β processing in a mouse model of Alzheimer’s disease but plays a minor role in the therapeutic properties of a cannabis-based medicine. J Alzheimers Dis. (2016) 51:489–500. doi: 10.3233/JAD-150913
160. de Lau LM, Breteler MM. Epidemiology of Parkinson’s disease. Lancet Neurol. (2006) 5:525–35. doi: 10.1016/S1474-4422(06)70471-9
161. Branchi I, D’Andrea I, Armida M, Cassano T, Pèzzola A, Potenza RL, et al. Nonmotor symptoms in Parkinson’s disease: investigating early-phase onset of behavioral dysfunction in the 6-hydroxydopamine-lesioned rat model. J Neurosci Res. (2008) 86:2050–61. doi: 10.1002/jnr.21642
162. McGeer PL, Itagaki S, Boyes BE, McGeer EG. Reactive microglia are positive for HLA-DR in the: substantia nigra of Parkinson’s and Alzheimer’s disease brains. Neurology. (1988) 38:1285–91. doi: 10.1212/wnl.38.8.1285
163. Ouchi Y, Yoshikawa E, Sekine Y, Futatsubashi M, Kanno T, Ogusu T, et al. Microglial activation and dopamine terminal loss in early Parkinson’s disease. Ann Neurol. (2005) 57:168–75. doi: 10.1002/ana.20338
164. Gerhard A, Pavese N, Hotton G, Turkheimer F, Es M, Hammers A, et al. In vivo imaging of microglial activation with [11C](R)-PK11195 PET in idiopathic Parkinson’s disease. Neurobiol Dis. (2006) 21:404–12. doi: 10.1016/j.nbd.2005.08.002
165. Shi J, Cai Q, Zhang J, He X, Liu Y, Zhu R, et al. AM1241 alleviates MPTP-induced Parkinson’s disease and promotes the regeneration of DA neurons in PD mice. Oncotarget. (2017) 8:67837–50. doi: 10.18632/oncotarget.18871
166. Centonze D, Finazzi-Agrò A, Bernardi G, Maccarrone M. The endocannabinoid system in targeting inflammatory neurodegenerative diseases. Trends Pharmacol Sci. (2007) 28:180–7. doi: 10.1016/j.tips.2007.02.004
167. Shohami E, Cohen-Yeshurun A, Magid L, Algali M, Mechoulam R. Endocannabinoids and traumatic brain injury. Br J Pharmacol. (2011) 163:1402–10. doi: 10.1111/j.1476-5381.2011.01343.x
168. Pertwee RG. Targeting the endocannabinoid system with cannabinoid receptor agonists: pharmacological strategies and therapeutic possibilities. Philos Trans R Soc B Biol Sci. (2012) 367:3353–63. doi: 10.1098/rstb.2011.0381
169. Micale V, Mazzola C, Drago F. Endocannabinoids and neurodegenerative diseases. Pharmacol Res. (2007) 56:382–92. doi: 10.1016/j.phrs.2007.09.008
170. Gómez-Gálvez Y, Palomo-Garo C, Fernández-Ruiz J, García C. Potential of the cannabinoid CB2 receptor as a pharmacological target against inflammation in Parkinson’s disease. Prog Neuro-Psychopharmacology Biol Psychiatry. (2016) 64:200–8. doi: 10.1016/j.pnpbp.2015.03.017
171. García C, Palomo-Garo C, García-Arencibia M, Ramos JA, Pertwee RG, Fernández-Ruiz J. Symptom-relieving and neuroprotective effects of the phytocannabinoid Δ 9-THCV in animal models of Parkinson’s disease. Br J Pharmacol. (2011) 163:1495–506. doi: 10.1111/j.1476-5381.2011.01278.x
172. Price DA, Martinez AA, Seillier A, Koek W, Acosta Y, Fernandez E, et al. WIN55,212-2, a cannabinoid receptor agonist, protects against nigrostriatal cell loss in the 1-methyl-4-phenyl-1,2,3,6-tetrahydropyridine mouse model of Parkinson’s disease. Eur J Neurosci. (2009) 29:2177–86. doi: 10.1111/j.1460-9568.2009.06764.x
173. Javed H, Azimullah S, Haque ME, Ojha SK. Cannabinoid type 2 (CB2) receptors activation protects against oxidative stress and neuroinflammation associated dopaminergic neurodegeneration in rotenone model of Parkinson’s disease. Front Neurosci. (2016) 10:321. doi: 10.3389/fnins.2016.00321
174. García MC, Cinquina V, Palomo-Garo C, Rábano A, Fernández-Ruiz J. Identification of CB2 receptors in human nigral neurons that degenerate in Parkinson’s disease. Neurosci Lett. (2015) 587:1–4. doi: 10.1016/j.neulet.2014.12.003
175. Eddings CR, Arbez N, Akimov S, Geva M, Hayden MR, Ross CA. Pridopidine protects neurons from mutant-huntingtin toxicity via the sigma-1 receptor. Neurobiol Dis. (2019) 129:118–29. doi: 10.1016/j.nbd.2019.05.009
176. Fernández-Ruiz J. The endocannabinoid system as a target for the treatment of motor dysfunction. Br J Pharmacol. (2009) 156:1029–40. doi: 10.1111/j.1476-5381.2008.00088.x
177. Spinelli F, Capparelli E, Abate C, Colabufo NA, Contino M. Perspectives of cannabinoid type 2 receptor (CB2R) ligands in neurodegenerative disorders: structure-affinity relationship (SAfiR) and structure-activity relationship (SAR) studies. J Med Chem. (2017) 60:9913–31. doi: 10.1021/acs.jmedchem.7b00155
178. Haider A, Spinelli F, Herde AM, Mu B, Keller C, Margelisch M, et al. Evaluation of 4-oxo-quinoline-based CB2 PET radioligands in R6/2 chorea huntington mouse model and human ALS spinal cord tissue. Eur J Med Chem. (2018) 145:746–59. doi: 10.1016/j.ejmech.2017.12.097
179. Sagredo O, González S, Aroyo I, Pazos MR, Benito C, Lastres-Becker I, et al. Cannabinoid CB2 receptor agonists protect the striatum against malonate toxicity: relevance for Huntington’s disease. Glia. (2009) 57:1154–67. doi: 10.1002/glia.20838
180. Bouchard J, Truong J, Bouchard K, Dunkelberger D, Desrayaud S, Moussaoui S, et al. Cannabinoid receptor 2 signaling in peripheral immune cells modulates disease onset and severity in mouse models of Huntington’s disease. J Neurosci. (2012) 32:18259–68. doi: 10.1523/JNEUROSCI.4008-12.2012
181. Palazuelos J, Aguado T, Pazos MR, Julien B, Carrasco C, Resel E, et al. Microglial CB2 cannabinoid receptors are neuroprotective in Huntington’s disease excitotoxicity. Brain. (2009) 132:3152–64. doi: 10.1093/brain/awp239
182. Bejargafshe MJ, Hedayati M, Zahabiasli S, Tahmasbpour E, Rahmanzadeh S, Nejad-Moghaddam A. Safety and efficacy of stem cell therapy for treatment of neural damage in patients with multiple sclerosis. Stem Cell Investig. (2019) 6:44. doi: 10.21037/sci.2019.10.06
183. Gonçalves ED, Dutra RC. Cannabinoid receptors as therapeutic targets for autoimmune diseases: where do we stand? Drug Discov Today. (2019) 24:1845–53. doi: 10.1016/j.drudis.2019.05.023
184. Maresz K, Carrier EJ, Ponomarev ED, Hillard CJ, Dittel BN. Modulation of the cannabinoid CB2 receptor in microglial cells in response to inflammatory stimuli. J Neurochem. (2005) 95:437–45. doi: 10.1111/j.1471-4159.2005.03380.x
185. Palazuelos J, Davoust N, Julien B, Hatterer E, Aguado T, Mechoulam R, et al. The CB2 cannabinoid receptor controls myeloid progenitor trafficking: involvement in the pathogenesis of an animal model of multiple sclerosis. J Biol Chem. (2008) 283:13320–4. doi: 10.1074/jbc.M707960200
186. Zhang M, Martin BR, Adler MW, Razdan RJ, Kong W, Ganea D, et al. Modulation of cannabinoid receptor activation as a neuroprotective strategy for EAE and stroke. J Neuroimmune Pharmacol. (2009) 4:249–59. doi: 10.1007/s11481-009-9148-4
187. Alberti TB, Barbosa WLR, Vieira JLF, Raposo NRB, Dutra RC. (–)-β-caryophyllene, a CB2 receptor-selective phytocannabinoid, suppresses motor paralysis and neuroinflammation in a murine model of multiple sclerosis. Int J Mol Sci. (2017) 18:691. doi: 10.3390/ijms18040691
188. Loría F, Petrosino S, Mestre L, Spagnolo A, Correa F, Hernangómez M, et al. Study of the regulation of the endocannabinoid system in a virus model of multiple sclerosis reveals a therapeutic effect of palmitoylethanolamide. Eur J Neurosci. (2008) 28:633–41. doi: 10.1111/j.1460-9568.2008.06377.x
189. Arévalo-Martín Á, Vela JM, Molina-Holgado E, Borrell J, Guaza C. Therapeutic action of cannabinoids in a murine model of multiple sclerosis. J Neurosci. (2003) 23:2511–6. doi: 10.1523/jneurosci.23-07-02511.2003
190. Arevalo-Martin A, Molina-Holgado E, Guaza C. A CB 1/CB 2 receptor agonist, WIN 55,212-2, exerts its therapeutic effect in a viral autoimmune model of multiple sclerosis by restoring self-tolerance to myelin. Neuropharmacology. (2012) 63:385–93. doi: 10.1016/j.neuropharm.2012.04.012
191. Yiangou Y, Facer P, Durrenberger P, Chessell IP, Naylor A, Bountra C, et al. COX-2, CB2 and P2X7-immunoreactivities are increased in activated microglial cells/macrophages of multiple sclerosis and amyotrophic lateral sclerosis spinal cord. BMC Neurol. (2006) 6:12. doi: 10.1186/1471-2377-6-12
192. Benito C, Romero JP, Tolón RM, Clemente D, Docagne F, Hillard CJ, et al. Cannabinoid CB1 and CB2 receptors and fatty acid amide hydrolase are specific markers of plaque cell subtypes in human multiple sclerosis. J Neurosci. (2007) 27:2396–402. doi: 10.1523/JNEUROSCI.4814-06.2007
193. Ludolph AC, Meyer T, Riepe MW. The role of excitotoxicity in ALS what is the evidence? J Neurol. (2000) 247:7–16. doi: 10.1007/s004150050552
194. Shoemaker JL, Seely KA, Reed RL, Crow JP, Prather PL. The CB2 cannabinoid agonist AM-1241 prolongs survival in a transgenic mouse model of amyotrophic lateral sclerosis when initiated at symptom onset. J Neurochem. (2007) 101:87–98. doi: 10.1111/j.1471-4159.2006.04346.x
195. Kim K, Moore DH, Makriyannis A, Abood ME. AM1241, a cannabinoid CB2 receptor selective compound, delays disease progression in a mouse model of amyotrophic lateral sclerosis. Eur J Pharmacol. (2006) 542:100–5. doi: 10.1016/j.ejphar.2006.05.025
196. Espejo-Porras F, García-Toscano L, Rodríguez-Cueto C, Santos-García I, de Lago E, Fernandez-Ruiz J. Targeting glial cannabinoid CB2 receptors to delay the progression of the pathological phenotype in TDP-43 (A315T) transgenic mice, a model of amyotrophic lateral sclerosis. Br J Pharmacol. (2019) 176:1585–600. doi: 10.1111/bph.14216
197. Espejo-Porras F, Piscitelli F, Verde R, Ramos JA, Di Marzo V, de Lago E, et al. Changes in the endocannabinoid signaling system in CNS structures of TDP-43 transgenic mice: relevance for a neuroprotective therapy in TDP-43-related disorders. J Neuroimmune Pharmacol. (2015) 10:233–44. doi: 10.1007/s11481-015-9602-4
198. Ji X, Zeng Y, Wu J. The CB2 receptor as a novel therapeutic target for epilepsy treatment. Int J Mol Sci. (2021) 22:8961. doi: 10.3390/ijms22168961
199. Zhu X, Liu J, Huang S, Zhu W, Wang Y, Chen O, et al. Neuroprotective effects of isoliquiritigenin against cognitive impairment via suppression of synaptic dysfunction, neuronal injury, and neuroinflammation in rats with kainic acid-induced seizures. Int Immunopharmacol. (2019) 72:358–66. doi: 10.1016/j.intimp.2019.04.028
200. Sugaya Y, Yamazaki M, Uchigashima M, Kobayashi K, Watanabe M, Sakimura K, et al. Crucial roles of the endocannabinoid 2-arachidonoylglycerol in the suppression of epileptic seizures. Cell Rep. (2016) 16:1405–15. doi: 10.1016/j.celrep.2016.06.083
201. Devinsky O, Patel AD, Cross JH, Villanueva V, Wirrell EC, Privitera M, et al. Effect of cannabidiol on drop seizures in the lennox–gastaut syndrome. N Engl J Med. (2018) 378:1888–97. doi: 10.1056/nejmoa1714631
202. Bialer M, Perucca E. Does cannabidiol have antiseizure activity independent of its interactions with clobazam? An appraisal of the evidence from randomized controlled trials. Epilepsia. (2020) 61:1082–9. doi: 10.1111/epi.16542
203. Wu J. Cannabis, cannabinoid receptors, and endocannabinoid system: yesterday, today, and tomorrow. Acta Pharmacol Sin. (2019) 40:297–9. doi: 10.1038/s41401-019-0210-3
204. Aghaei I, Rostampour M, Shabani M, Naderi N, Motamedi F, Babaei P, et al. Palmitoylethanolamide attenuates PTZ-induced seizures through CB1 and CB2 receptors. Epilepsy Res. (2015) 117:23–8. doi: 10.1016/j.eplepsyres.2015.08.010
205. da Silva Oliveira GL, da Silva JCCL, dos Santos CL, da Silva AP, Feitosa CM, de Castro Almeida FR. Anticonvulsant, anxiolytic and antidepressant properties of the β-caryophyllene in swiss mice: involvement of benzodiazepine-GABAAergic, serotonergic and nitrergic systems. Curr Mol Pharmacol. (2020) 14:36–51. doi: 10.2174/1874467213666200510004622
206. Huizenga MN, Wicker E, Beck VC, Forcelli PA. Anticonvulsant effect of cannabinoid receptor agonists in models of seizures in developing rats. Epilepsia. (2017) 58:1593–602. doi: 10.1111/epi.13842
207. de Carvalho CR, Hoeller AA, Franco PLC, Martini APS, Soares FMS, Lin K, et al. The cannabinoid CB2 receptor-specific agonist AM1241 increases pentylenetetrazole-induced seizure severity in Wistar rats. Epilepsy Res. (2016) 127:160–7. doi: 10.1016/j.eplepsyres.2016.08.011
208. Shapiro L, Wong JC, Escayg A. Reduced cannabinoid 2 receptor activity increases susceptibility to induced seizures in mice. Epilepsia. (2019) 60:2359–69. doi: 10.1111/epi.16388
209. Chodobski A, Zink BJ, Szmydynger-Chodobska J. Blood-brain barrier pathophysiology in traumatic brain injury. Transl Stroke Res. (2011) 2:492–516. doi: 10.1007/s12975-011-0125-x
210. Amenta PS, Jallo JI, Tuma RF, Elliott MB. A cannabinoid type 2 receptor agonist attenuates blood-brain barrier damage and neurodegeneration in a murine model of traumatic brain injury. J Neurosci Res. (2012) 90:2293–305. doi: 10.1002/jnr.23114
211. Elliott MB, Tuma RF, Amenta PS, Barbe MF, Jallo JI. Acute effects of a selective cannabinoid-2 receptor agonist on neuroinflammation in a model of traumatic brain injury. J Neurotrauma. (2011) 28:973–81. doi: 10.1089/neu.2010.1672
212. Magid L, Heymann S, Elgali M, Avram L, Cohen Y, Liraz-Zaltsman S, et al. Role of CB2 receptor in the recovery of mice after traumatic brain injury. J Neurotrauma. (2019) 36:1836–46. doi: 10.1089/neu.2018.6063
213. Li L, Luo Q, Shang B, Yang X, Zhang Y, Pan Q, et al. Selective activation of cannabinoid receptor-2 reduces white matter injury via PERK signaling in a rat model of traumatic brain injury. Exp Neurol. (2022) 347:113899. doi: 10.1016/j.expneurol.2021.113899
214. Onaivi ES, Ishiguro H, Gu S, Liu QR. CNS effects of CB2 cannabinoid receptors: beyond neuro-immuno-cannabinoid activity. J Psychopharmacol. (2012) 26:92–103. doi: 10.1177/0269881111400652
215. Onaivi ES, Ishiguro H, Liu QR. Future perspectives: cannabinoid CB2 receptor ligands and their therapeutic potential in mental diseases. Cannabinoids Neurol Ment Dis. (2005) 18:425–44. doi: 10.1016/B978-0-12-417041-4.00018-7
216. Kibret BG, Ishiguro H, Horiuchi Y, Onaivi ES. New insights and potential therapeutic targeting of CB2 cannabinoid receptors in CNS disorders. Int J Mol Sci. (2022) 23:975. doi: 10.3390/ijms23020975
217. Basavarajappa BS, Shivakumar M, Joshi V, Subbanna S. Endocannabinoid system in neurodegenerative disorders. J Neurochem. (2017) 142:624–48. doi: 10.1111/jnc.14098
Keywords: cannabinoid CB2 receptors, neuropsychiatric disorders, neurodegenerative (ND) disorders, neuronal and glia activation
Citation: Ishiguro H, Kibret BG, Horiuchi Y and Onaivi ES (2022) Potential Role of Cannabinoid Type 2 Receptors in Neuropsychiatric and Neurodegenerative Disorders. Front. Psychiatry 13:828895. doi: 10.3389/fpsyt.2022.828895
Received: 04 December 2021; Accepted: 02 May 2022;
Published: 14 June 2022.
Edited by:
Michael Kluge, University Hospital Leipzig, GermanyReviewed by:
Anna Brancato, University of Palermo, ItalyArnau Busquets-Garcia, Fundació Institut Mar d’Investigacions Mèdiques (IMIM), Spain
Christina Jennifer Perry, Macquarie University, Australia
Copyright © 2022 Ishiguro, Kibret, Horiuchi and Onaivi. This is an open-access article distributed under the terms of the Creative Commons Attribution License (CC BY). The use, distribution or reproduction in other forums is permitted, provided the original author(s) and the copyright owner(s) are credited and that the original publication in this journal is cited, in accordance with accepted academic practice. No use, distribution or reproduction is permitted which does not comply with these terms.
*Correspondence: Hiroki Ishiguro, hishiguro@yamanashi.ac.jp; Emmanuel S. Onaivi, onaivie@wpunj.edu