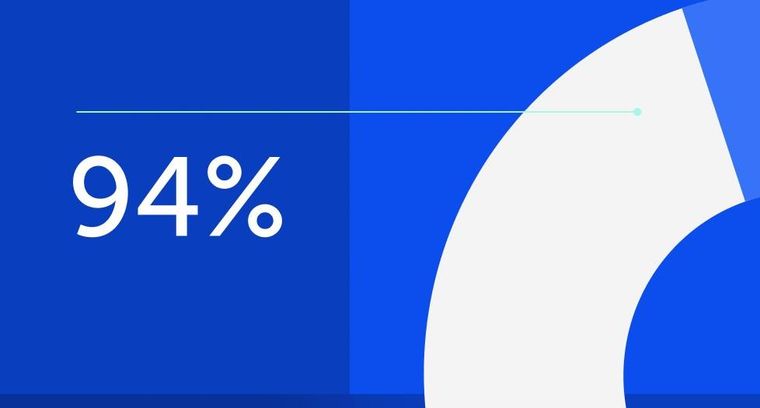
94% of researchers rate our articles as excellent or good
Learn more about the work of our research integrity team to safeguard the quality of each article we publish.
Find out more
MINI REVIEW article
Front. Psychiatry, 17 February 2022
Sec. Neuroimaging
Volume 13 - 2022 | https://doi.org/10.3389/fpsyt.2022.817302
This article is part of the Research TopicThe Habenula and Its Role in Neuropsychiatric SymptomsView all 13 articles
Over the past decades, an ever growing body of literature has explored the anatomy, connections, and functions of the habenula (Hb). It has been postulated that the Hb plays a central role in the control of the monoaminergic system, thus influencing a wide range of behavioral responses, and participating in the pathophysiology of a number of psychiatric disorders and neuropsychiatric symptoms, such as aggressive behaviors. Aggressive behaviors are frequently accompanied by restlessness and agitation, and are commonly observed in patients with psychiatric disorders, intellectual disabilities, and neurodegenerative diseases of aging. Recently, the Hb has been explored as a new target for neuromodulation therapies, such as deep brain stimulation, with promising results. Here we review the anatomical organization of the habenula and discuss several distinct mechanisms by which the Hb is involved in the modulation of aggressive behaviors, and propose new investigations for the development of novel treatments targeting the habenula to reduce aggressive behaviors.
The habenula (Hb) is an epithalamic structure that presents rich connections with several cortical and subcortical structures, including the limbic system, and areas responsible for the production and regulation of monoamines (i.e., raphe nuclei for serotonin, ventral tegmental area and substantia nigra for dopamine, and locus coeruleus for noradrenaline) (1–5). These connections place the Hb in a central position for the regulation of motivated behaviors, and thus has been implicated in the pathophysiology of several disorders, such as autism spectrum disorder (ASD) (6), depression (7, 8), bipolar disorder (8–10), and schizophrenia (10, 11), as well as neuropsychiatric symptoms, such as aggressive behaviors (12, 13).
Aggressive behaviors can be verbal and physical insults directed toward oneself (i.e., self-injury behavior), others, or objects (14), and are highly correlated with restlessness and excessive motor agitation (15, 16). Several distinct classifications of human aggressive behavior have been proposed, with the classification in proactive (also known as premeditated aggression) or reactive (also known as impulsive aggression) being widely accepted (17–21). While proactive aggression is believed to involve planned behaviors to achieve a specific goal, reactive aggression is unrelated to a specific goal, being mainly associated with frustration, provocation or stress. Another important difference between these two types of aggressive behavior, is the association with high levels of autonomic arousal and impulsivity in subjects presenting with reactive aggression, that is absence in the proactive aggression (17–21). Aggressive behaviors, mainly reactive aggression, are frequent among patients with psychiatric conditions, especially in those suffering from intermittent explosive disorder, borderline/antisocial personality disorders, patients with neurodevelopmental conditions, such as ASD (17, 22), and those with neurodegenerative diseases of aging (e.g., Alzheimer's disease) (23–25).
The neurocircuitry underlying aggressive behaviors include prefrontal cortical regions and areas of the mesolimbic system, especially the hypothalamus, amygdala and periaqueductal gray matter (14–17, 26). It is believed that decreased serotonergic transmission in the prefrontal cortex reduces the top-down inhibitory control over the limbic system, resulting in motor activation and hormonal production, preparing the organism for a fight-or-flight situation (17, 21, 26). However, simplistic this mechanism might seem, there are several distinct neural-pathways involved in the association of external and internal stimuli that will result in the expression of an appropriate or inappropriate aggressive behavioral response. As such, the mechanisms by which the Hb is involved in the modulation of aggressive behaviors are numerous and still not fully understood.
In this review, we explore the anatomical organization of the habenula, describe the relevant literature on the involvement of the Hb in the modulation of aggressive behaviors and discuss future perspectives and novel therapies.
The Hb is a bilateral, phylogenetically old, epithalamic structure surrounded by the third ventricle and the thalamus (lateral and dorsal borders), the posterior commissure (ventral and posterior borders), and the stria medullaris of the thalamus (anterior limit, Figure 1A) (1, 4, 9). In mammals, the Hb is divided into two sub-regions—the medial habenula (MHb) and the lateral habenula (LHb)—based on their cellular and genetic profiles, neuroanatomical connectivity, and associated functions (Figure 1B) (1, 4, 5). Through the fasciculus retroflexus, both MHb and LHb project to distinct brain areas. MHb efferents form the core of the fiber bundle that reaches the interpeduncular nucleus (IP) in a 90° rotation pattern, with dorsal projections reaching the lateral aspect of the IP, medial projections to the ventral aspect of IP and the lateral projections ending on the dorsal aspect of IP (2). Projections from the IP then reaches the periaqueductal gray matter (27), an area critically involved in the neural network of aggressive behavior (17, 21, 26). Discrete projections from the MHb can also be found in the LHb, supra-commissural septum and median raphe nucleus (2, 28). Inputs form the medial, lateral and triangular septal nuclei and septofimbrial nucleus, via the medial stria medullaris comprise the main afferent projections to the MHb (2, 5, 29). LHb efferents forms the mantle portion of the fasciculus retroflexus, that reaches the ventral tegmental area, hypothalamus (i.e., lateral, posterior and dorsomedial hypothalamic nuclei, lateral preoptic area), ventromedial thalamic nucleus, substantia innominata, ventrolateral septum, substantia nigra pars compacta, medial and dorsal raphe nuclei, and tegmental reticular formation (2). Discrete additional projections can be found in the pretectal area, superior colliculus, nucleus reticularis tegmenti pontis, parabrachial nuclei, and locus coeruleus (2). Afferent projections from limbic regions are mainly found in the more medial aspect of the LHb while projections from the globus pallidus reach the lateral aspect of the LHb (Figure 1C) (2).
Figure 1. Anatomical organization of the habenula. (A) Magnetic resonance imaging (coronal plane) showing the human and mouse habenula (https://openneuro.org/datasets/ds002179/versions/1.1.0). (B) Anatomical parcellation of the medial (MHb) and lateral habenula (LHb). (C) Main habenula connections. MHbS, medial habenula superior part; MHbI, medial habenula inferior part; MHbC, medial habenula central part; MHbL, medial habenula lateral part; MHbCo, medial habenula commissural part; LHbMA, lateral habenula medial part anterior subregion; LHbMS, lateral habenula medial part superior subregion; LHbMPc, lateral habenula medial part parvocellular subregion; LHbMC, lateral habenula medial part central subregion; LHbMMg, lateral habenula medial part marginal subregion; LHbLPc, lateral habenula lateral part parvocellular subregion; LHbLMc, lateral habenula lateral part magnocellular subregion; LHbLO, lateral habenula lateral part oval subregion; LHbLB, lateral habenula lateral part basal subregion; LHbLMg, lateral habenula lateral part marginal subregion; PAG, periaqueductal gray matter; VTA, ventral tegmental area.
The MHb can be further divided into five subregions, namely superior (MHbS), inferior (MHbI), central (MHbC), lateral (MHbL), and commissural (MHbCo) parts (Figure 1B). The MHbS consists exclusively of densely packed glutamatergic neurons that strongly express interleukin-18, with nuclei on a typical triangular appearance, thin dendrites and tightly packed synaptic vesicles in axon terminals (3, 30). The cell characteristics of neurons in the MHbI are similar to the one in the MHbS, however the nuclei are typically round and the proximal dendrites are thicker. Also, these neurons are not exclusively glutamatergic as they co-transmits acetylcholine from the axonal terminals (3, 30). Likewise, the MHbL is also composed of cholinergic and glutamatergic neurons, however these are smaller, with oval nuclei and nuclear membrane surrounded by nucleoli or chromatin plaques (3, 30). The MHbC can be viewed as a transition area, as it is composed of a combination of cell clusters composed of the diverse cell types observed in the adjacent regions, that are separated from each-other by the terminal fiber bundles of the stria medullaris. While the dorsal region of the MHbC is composed of neurons that co-express substance-P and glutamate, the ventral region is both cholinergic and glutamatergic (3, 30). Finally, the MHbCo displays the largest glutamatergic neurons in the MHb area, with unusual nuclei in a semilunar shape (3, 30).
The LHb is significantly larger than the MHb and is subdivided into medial (LHbM) and lateral (LHbL) parts (Figure 1B). Each of these aspects are further subdivided resulting in a total of ten LHb subregions (3). Irrespective of cell morphology and location, neurons in the LHb are predominantly glutamatergic (30), however it has recently been shown that a very discrete population of GABAergic neurons are present in the medial part of the LHb (31, 32). The LHbM is divided in anterior (LHbMA), superior (LHbMS), parvocellular (LHbMPc), central (LHbMC), and marginal (LHbMMg) subregions. Small neurons are found in the LHbMA, LHbMMg and LHbMPc parts, with LHbMA showing round multipolar cells with heavily folded nuclei, LHbMMg having an elongated version of the LHbMA cells, and LHbMPc presenting a spindle-shaped cell with deeply invaginated nucleus. Greatly larger neurons are found in the LHbMS and LHbMC subregions, however LHbMS neurons are characterized by an oval perikarya, and LHbMC cell nuclei are often invaginated and display a brighter and finer karyoplasm (3). The LHbL is divided into parvocellular (LHbLPc), magnocellular (LHbLMc), oval (LHbLO), basal (LHbLB), and marginal (LHbLMg) subregions (3). Neurons in the LHbLPc and LHbLMg parts are predominantly small to medium-sized. Cells in the LHbLMc, and LHbLO are large, and while neurons in the LHbLMc and LHbLB parts present thick and long dendrites, neurons in the LHbLO extend thin ones (3).
Several clinical and preclinical studies have investigated the involvement of the Hb in the modulation of aggressive behaviors. Studies using transgenic models have provided further evidence of the involvement of the Hb in the regulation of aggressive behaviors. Using double and triple transgenic zebrafish, Chou et al. (33) demonstrated that the dorsal habenula–interpeduncular nucleus pathway [homologous to the mammalian MHb-IP pathway (34)], is key for the modulation of aggressive behaviors, with connectivity between the medial subregion of dHb and IP being associated with increase aggression (33). GPR3 is an G-protein-coupled-receptor broadly expressed in the central nervous system, with maximal expression in the Hb, that has been implicated in the regulation of cAMP signaling and, consequently, modulation of emotional behavior. Knockout Gpr3−/− mice present null expression of GPR3 in the Hb, high levels of aggressive behavior and accentuated reduction of serotonin, noradrenaline and its metabolites in the hypothalamus and frontal cortex (35). The Disrupted-In-Schizophrenia-1 (DISC1-Q31L) mouse model of depression, bipolar disorder and schizophrenia, presents heightened inter-male aggressive behavior along with increased neuronal density in both the LHb and MHb (36). On the other hand, male Mecp2− mice (i.e., knockout of the X-linked methyl-CpG-binding protein 2, gene associated to Rett syndrome) present absence of aggressive behavior and accentuated reduction in oxytocinergic innervation in the lateral habenula (37). It is important to highlight, however, that transgenic animals may also present with additional brain alterations in function and connection and, thus, the altered behavior observed in these studies may be the result of the sum of all these changes and not rely solely on alterations observed in the habenula.
In lactating females, aggressive behaviors toward an intruder are mediated by the medial prefrontal cortex-LHb-dorsal raphe nucleus pathway, as demonstrated by increased co-labeling of c-Fos- and Fluorogold-positive neurons in the mPFC and LHb following aggressive encounters (38). Pharmacological manipulation of NMDA and AMPA/kainate receptors via microinjection of receptor antagonists in the dorsal raphe nucleus of lactating females is capable of inhibiting this behavior (38). Interestingly, injection of arginine-vasopressin V1a receptor antagonists in the LHb or dorsal raphe nucleus, of both male and female mice, is not sufficient to alter aggressive behaviors, suggesting that the arginine-vasopressin system does not play a crucial role in this neurocircuitry (39). Tear fluid is rich in pheromones capable of eliciting several context-specific behavioral responses in both males and females rodents (40). It has been shown that female mouse tears suppress aggressive behaviors in males and induce a great increase in c-Fos immunoreactivity in the medial aspect of the LHb (41).
A study investigating habenula resting-state functional connectivity in highly reactive aggressive men showed an association between high levels of trait aggression to lower global efficiency of the left habenula and atypical habenula-prefrontal connectivity (42). In a recent work from our group, we showed that the Hb—along with the dorsal raphe nuclei, substantia nigra, ventral tegmental area, and locus coeruleus—is part of the functional connectivity map associated with symptom alleviation in a patient treated with deep brain stimulation of the posterior hypothalamus for reduction of severe and treatment refractory aggressive behavior (43).
Another line of evidence is based on the well-known involvement of the LHb in reward-related behaviors. The suppression of midbrain dopaminergic neurons, via GABAergic indirect connections, is thought to be the main mechanism by which the LHb drives reinforcement learning (1). The LHb is active in response to the negative value of a stimulus, unexpected reward omissions, and cues associated with these stimuli (44, 45), such as in situations of drug withdrawal (46–48). Moreover, the functional integrity of the LHb is necessary to integrate proactive and retroactive information to guide behavioral flexibility when the reward contingencies change (49). Golden et al. (50) investigated the involvement of basal forebrain projections to the LHb in the modulation of aggression reward. Using a conditioned place preference (CPP) or place aversion (CPA) paradigm, the authors have shown that aggressive mice presented CPP for the chamber where aggressive encounters occurred, while non-aggressive mice developed a CPA to the same chamber. Using optogenetic techniques to stimulate or inhibit the basal forebrain projections to the LHb, it was found that the stimulation of these terminals promotes CPP and reduces LHb firing, and the inhibition results in CPA and increases LHb firing (50). These results are in line with previous findings indicating that decreased LHb activity is associated with rewarding components of behavior. Flanigan et al. (32) showed that optogenetic stimulation of orexin terminals located in GABAergic neurons within the LHb promotes inter-male aggressive behavior and CPP for aggression-paired contexts.
The reward value of aggressive behaviors is also mediated via the LHb-ventral tegmental area (VTA)-nucleus accumbens (nAcc) network that results in increased dopamine release in the nAcc when animals are expecting a conditioned aggressive encounter (51). Moreover, antagonism of both dopamine receptor types 1 and 2 in the nAcc have been described as reducing the rewarding value of aggressive behaviors (52–54). In line with these findings, two case reports (55, 56) and one case series (57) reporting on patients treated with deep brain stimulation of the nAcc for severe refractory aggressive behaviors, have shown long lasting positive results (58).
There is a strong body of evidence on the role of the Hb in regulating the circadian cycle, thus influencing internal physiology, brain activity patterns and day-night behavioral rhythm (59–62). Anatomically, the Hb shares the epithalamus with the pineal gland, a brain structure responsible for the production of the hormone melatonin, that serves among others, as a major regulator of the sleep-wake cycle (5, 63). Similar to the pineal gland, the Hb expresses mRNA for arylalkylamine-N-acetyltransferase, the enzyme responsible for melatonin synthesis, thus being implicated as a supplementary location for melatonin biosynthesis (63). Dysregulations of the circadian cycle are known to negatively influence cognition, emotions and behavior, and is associated with worsening of symptoms in several neuropsychiatric disorders (64, 65). Poor sleep routines (i.e., short sleep duration, inadequate sleep quality) in children and adolescents is associated with increased irritability, conduct problems, anxiety, and hyperactivity (66–68), and in adults, is associated with increased hostility, anger, aggression and suicidal ideation (49–52). However, it is important to highlight that the Hb circadian clock is independent of the suprachiasmatic nucleus (60), and although disruption of the Hb circadian clock does not disrupt the sleep cycle, it is capable of altering the subjects response to stressors, suggesting that a sleep-independent effect on aggression may exist (69).
Among the various neurotransmitters, neuropeptides, and neurohormones involved in the modulation of the circadian cycle, dysfunctions in serotonin transmission are thought to be central in the association between poor sleep and aggressiveness. Via GABAergic interneurons, the LHb modulates the activity of serotonergic neurons in the dorsal raphe nucleus (70, 71), that have an increased activity during wakefulness, along with brain-wide increase in serotonin levels (72, 73). The prolonged exposure to high serotonin levels caused by reduced sleep time causes gradual desensitization of serotonin receptors (74), thus contributing to a reduced serotonergic effective transmission in the prefrontal cortex and consequently reduction in the top-down inhibitory control of emotions described above.
Excessive aggressive behaviors are highly prevalent, particularly among patients with psychiatric disorders and presents a major obstacle for patient care, increasing institutionalization rates and reducing patients' quality of life (15, 17). The clinical and preclinical studies described here show evidence of the involvement of the habenula in the neuro-circuitry of aggressive behavior, and suggest that the modulation of neurons in this area could result in symptom alleviation in patients presenting psychiatric disorders associated with aggressive behavior. Furthermore, typical and atypical antipsychotics and antidepressants are commonly used for the treatment of aggressive behaviors via antagonizing dopaminergic receptors or selectively inhibiting serotonin reuptake enzymes (17). As described above, the Hb is highly connected with areas responsible for the production and regulation of monoamines (e.g., dopamine and serotonin) and thus, involved in the response to standard treatments (1–5). However, the chronic systemic exposure to these compounds is associated with refractoriness to treatment, and may produce severe side effects that can escalate to the point of being impeditive of treatment (14, 16, 75, 76). Thus, further studies are necessary to better understand the brain mechanisms associated with aggressive behaviors and develop novel treatments that are tailored to safely and effectively improve patient outcomes.
Focused ultrasound is being intensively investigated as a novel non-invasive tool for neuromodulation that could be used to deliver pharmacological agents to localized brain areas, without the need of a systemic distribution (77). By repurposing a commercially available ultrasound contrast, Lea-Banks and colleagues fabricated an ultrasound-sensitive nanodroplet loaded with or without anesthetic drug, that was injected intravenously and then vaporized in a discrete brain target using focused ultrasound (78, 79). The authors showed that the use of the unloaded nanodroplets increased local neuronal activity, while drug-loaded nanodroplets suppressed (78, 79). Considering that the Hb is involved in the regulation of monoamines, such as those modulated by drug therapy, and the current evidence on the possibility of reducing the reward value of aggressive behavior by increasing neuronal firing in the LHb, one could envision further exploring this innovative technique to selectively deliver nanodroplets to the LHb and modulate its activity, to reduce aggressive behavior in patients with psychiatric disorders that do not present adequate response to conventional therapy.
Deep brain stimulation is a neuromodulation therapy that involves the precise placement of electrodes into deep brain structures to modulate neuronal activity via the application of an electrical current that can be precisely titrated (80). Although only a few case studies on Hb deep brain stimulation have been published, they report beneficial outcomes in patients suffering from schizophrenia (81), depression (82, 83), obsessive-compulsive disorder (84), and bipolar disorder (85). New clinical trials are currently being performed, demonstrating a growing interest to target this region for the treatment of psychiatric disorders [for a review on deep brain stimulation of the habenula see (86)]. Considering the strong evidence on the involvement of the Hb in the pathophysiology of aggressive behaviors and the possibility of safely targeting this area with deep brain stimulation, it would be interesting to investigate Hb deep brain stimulation in the context of aggressive behaviors.
In this article we provide a detailed review of the anatomical organization of the Hb, by describing cell characteristics and connections of the lateral and medial aspects of the Hb and its subdivisions. We discussed several distinct mechanisms by which the Hb modulates aggressive behavior, detailing studies investigating transgenic models, neuronal modulation and neuroimaging, and the literature about the involvement of the Hb in reward-related behaviors and regulation of circadian cycle. We concluded this review discussing how innovative neuromodulatory techniques could be investigated in the context of Hb and aggressive behaviors to improve patient outcome.
Both authors listed have made a substantial, direct, and intellectual contribution to the work and approved it for publication.
The authors declare that the research was conducted in the absence of any commercial or financial relationships that could be construed as a potential conflict of interest.
All claims expressed in this article are solely those of the authors and do not necessarily represent those of their affiliated organizations, or those of the publisher, the editors and the reviewers. Any product that may be evaluated in this article, or claim that may be made by its manufacturer, is not guaranteed or endorsed by the publisher.
1. Hikosaka O. The habenula: from stress evasion to value-based decision-making. Nat Rev Neurosci. (2010) 11:503–13. doi: 10.1038/nrn2866
2. Herkenham M, Nauta WJ. Efferent connections of the habenular nuclei in the rat. J Comp Neurol. (1979) 187:19–47. doi: 10.1007/978-1-4684-7920-1_17
3. Andres KH, von Düring M, Veh RW. Subnuclear organization of the rat habenular complexes. J Comp Neurol. (1999) 407:130–50. doi: 10.1002/(sici)1096-9861(19990428)407:1<130::aid-cne10>3.0.co;2-8
4. Hikosaka O, Sesack SR, Lecourtier L, Shepard PD. Habenula: crossroad between the basal ganglia and the limbic system. J Neurosci. (2008) 28:11825–9. doi: 10.1523/JNEUROSCI.3463-08.2008
5. Namboodiri VMK, Rodriguez-Romaguera J, Stuber GD. The habenula. Curr Biol. (2016) 26:R873–7. doi: 10.1016/j.cub.2016.08.051
6. Germann J, Gouveia FV, Brentani H, Bedford SA, Tullo S, Chakravarty MM, et al. Involvement of the habenula in the pathophysiology of autism spectrum disorder. Sci Rep. (2021) 11:21168. doi: 10.1038/s41598-021-00603-0
7. Sartorius A, Henn FA. Deep brain stimulation of the lateral habenula in treatment resistant major depression. Med Hypotheses. (2007) 69:1305–8. doi: 10.1016/j.mehy.2007.03.021
8. Savitz JB, Nugent AC, Bogers W, Roiser JP, Bain EE, Neumeister A, et al. Habenula volume in bipolar disorder and major depressive disorder: a high-resolution magnetic resonance imaging study. Biol Psychiatry. (2011) 69:336–43. doi: 10.1016/j.biopsych.2010.09.027
9. Germann J, Gouveia FV, Martinez RCR, Zanetti MV, de Souza Duran FL, Chaim-Avancini TM, et al. Fully automated habenula segmentation provides robust and reliable volume estimation across large magnetic resonance imaging datasets, suggesting intriguing developmental trajectories in psychiatric disease. Biol Psychiatry Cogn Neurosci Neuroimaging. (2020) 5:923–9. doi: 10.1016/j.bpsc.2020.01.004
10. Schafer M, Kim J-W, Joseph J, Xu J, Frangou S, Doucet GE. Imaging habenula volume in schizophrenia and bipolar disorder. Front Psychiatry. (2018) 9:456. doi: 10.3389/fpsyt.2018.00456
11. Zhang L, Wang H, Luan S, Yang S, Wang Z, Wang J, et al. Altered volume and functional connectivity of the habenula in schizophrenia. Front Hum Neurosci. (2017) 11:636. doi: 10.3389/fnhum.2017.00636
12. Flanigan M, Aleyasin H, Takahashi A, Golden SA, Russo SJ. An emerging role for the lateral habenula in aggressive behavior. Pharmacol Biochem Behav. (2017) 162:79–86. doi: 10.1016/j.pbb.2017.05.003
13. Webster JF, Wozny C. Behavior: local lateral habenula interneurons mediate aggression. Curr Biol. (2020) 30:R954–6. doi: 10.1016/j.cub.2020.06.065
14. Gouveia FV, Germann J, Devenyi GA, Fonoff ET, Morais RMCB, Brentani H, et al. Bilateral amygdala radio-frequency ablation for refractory aggressive behavior alters local cortical thickness to a pattern found in non-refractory patients. Front Hum Neurosci. (2021) 15:653631. doi: 10.3389/fnhum.2021.653631
15. Gouveia FV, Germann J, de Morais R, Fonoff ET, Hamani C, Alho EJ, et al. Longitudinal changes after amygdala surgery for intractable aggressive behavior: clinical, imaging genetics, and deformation-based morphometry study-a case series. Neurosurgery. (2021) 88:E158–69. doi: 10.1093/neuros/nyaa378
16. Gouveia FV, Germann J, Devenyi GA, Morais RMCB, Santos APM, Fonoff ET, et al. Refractoriness of aggressive behaviour to pharmacological treatment: cortical thickness analysis in autism spectrum disorder. BJPsych Open. (2020) 6:e85. doi: 10.1192/bjo.2020.71
17. Gouveia FV, Hamani C, Fonoff ET, Brentani H, Alho EJL, de Morais RMCB, et al. Amygdala and hypothalamus: historical overview with focus on aggression. Neurosurgery. (2019) 85:11–30. doi: 10.1093/neuros/nyy635
18. Batrinos ML. Testosterone and aggressive behavior in man. Int J Endocrinol Metab. (2012) 10:563–8. doi: 10.5812/ijem.3661
19. Miczek KA, de Almeida RMM, Kravitz EA, Rissman EF, de Boer SF, Raine A. Neurobiology of escalated aggression and violence. J Neurosci. (2007) 27:11803–6. doi: 10.1523/JNEUROSCI.3500-07.2007
20. Rosell DR, Siever LJ. The neurobiology of aggression and violence. CNS Spectr. (2015) 20:254–79. doi: 10.1017/S109285291500019X
21. Siever LJ. Neurobiology of aggression and violence. Am J Psychiatry. (2008) 165:429–42. doi: 10.1176/appi.ajp.2008.07111774
22. Brentani H, de Paula CS, Bordini D, Rolim D, Sato F, Portolese J, et al. Autism spectrum disorders: an overview on diagnosis and treatment. Braz J Psychaitry. (2013) 35:S62–72. doi: 10.1590/1516-4446-2013-S104
23. Lanctôt KL, Herrmann N. Mirtazapine for agitation in dementia: moving forward. Lancet. (2021) 398:1462–3. doi: 10.1016/S0140-6736(21)01421-5
24. Ruthirakuhan M, Herrmann N, Andreazza AC, Verhoeff NPLG, Gallagher D, Black SE, et al. Agitation, oxidative stress, and cytokines in alzheimer disease: biomarker analyses from a clinical trial with nabilone for agitation. J Geriatr Psychiatry Neurol. (2020) 33:175–84. doi: 10.1177/0891988719874118
25. Ruthirakuhan M, Lanctôt KL, Di Scipio M, Ahmed M, Herrmann N. Biomarkers of agitation and aggression in Alzheimer's disease: a systematic review. Alzheimers Dement. (2018) 14:1344–76. doi: 10.1016/j.jalz.2018.04.013
26. Blair RJR. The neurobiology of impulsive aggression. J Child Adolesc Psychopharmacol. (2016) 26:4–9. doi: 10.1089/cap.2015.0088
27. Agetsuma M, Aizawa H, Aoki T, Nakayama R, Takahoko M, Goto M, et al. The habenula is crucial for experience-dependent modification of fear responses in zebrafish. Nat Neurosci. (2010) 13:1354–6. doi: 10.1038/nn.2654
28. Kim U, Chang S-Y. Dendritic morphology, local circuitry, and intrinsic electrophysiology of neurons in the rat medial and lateral habenular nuclei of the epithalamus. J Comp Neurol. (2005) 483:236–50. doi: 10.1002/cne.20410
29. Viswanath H, Carter AQ, Baldwin PR, Molfese DL, Salas R. The medial habenula: still neglected. Front Hum Neurosci. (2013) 7:931. doi: 10.3389/fnhum.2013.00931
30. Aizawa H, Kobayashi M, Tanaka S, Fukai T, Okamoto H. Molecular characterization of the subnuclei in rat habenula. J Comp Neurol. (2012) 520:4051–66. doi: 10.1002/cne.23167
31. Zhang L, Hernández VS, Swinny JD, Verma AK, Giesecke T, Emery AC, et al. A GABAergic cell type in the lateral habenula links hypothalamic homeostatic and midbrain motivation circuits with sex steroid signaling. Transl Psychiatry. (2018) 8:50. doi: 10.1038/s41398-018-0099-5
32. Flanigan ME, Aleyasin H, Li L, Burnett CJ, Chan KL, LeClair KB, et al. Orexin signaling in GABAergic lateral habenula neurons modulates aggressive behavior in male mice. Nat Neurosci. (2020) 23:638–50. doi: 10.1038/s41593-020-0617-7
33. Chou M-Y, Amo R, Kinoshita M, Cherng B-W, Shimazaki H, Agetsuma M, et al. Social conflict resolution regulated by two dorsal habenular subregions in zebrafish. Science. (2016) 352:87–90. doi: 10.1126/science.aac9508
34. Concha M. An evolutionary perspective on habenular asymmetry in humans. J Neurol Neuromed. (2016) 1:44–50. doi: 10.29245/2572.942X/2016/8.1094
35. Valverde O, Célérier E, Baranyi M, Vanderhaeghen P, Maldonado R, Sperlagh B, e al. GPR3 receptor, a novel actor in the emotional-like responses. PLoS ONE. (2009) 4:e4704. doi: 10.1371/journal.pone.0004704
36. Serykh A, Khrapova MV, Dubrovina NI, Petrova ES, Mikhnevich N, Starostina MV, et al. The increased density of the habenular neurons, high impulsivity, aggression and resistant fear memory in Disc1-Q31L genetic mouse model of depression. Behav Brain Res. (2020) 392:112693. doi: 10.1016/j.bbr.2020.112693
37. Martínez-Rodríguez E, Martín-Sánchez A, Kul E, Bose A, Martínez-Martínez FJ, Stork O, et al. Male-specific features are reduced in Mecp2-null mice: analyses of vasopressinergic innervation, pheromone production and social behaviour. Brain Struct Funct. (2020) 225:2219–38. doi: 10.1007/s00429-020-02122-6
38. Muroi Y, Ishii T. Glutamatergic signals in the dorsal raphe nucleus regulate maternal aggression and care in an opposing manner in mice. Neuroscience. (2019) 400:33–47. doi: 10.1016/j.neuroscience.2018.12.034
39. Rigney N, Beaumont R, Petrulis A. Sex differences in vasopressin 1a receptor regulation of social communication within the lateral habenula and dorsal raphe of mice. Horm Behav. (2020) 121:104715. doi: 10.1016/j.yhbeh.2020.104715
40. Tsunoda M, Miyamichi K, Eguchi R, Sakuma Y, Yoshihara Y, Kikusui T, et al. Identification of an intra- and inter-specific tear protein signal in rodents. Curr Biol. (2018) 28:1213–23.e6. doi: 10.1016/j.cub.2018.02.060
41. Cavaliere RM, Silvotti L, Percudani R, Tirindelli R. Female mouse tears contain an anti-aggression pheromone. Sci Rep. (2020) 10:2510. doi: 10.1038/s41598-020-59293-9
42. Gan G, Zilverstand A, Parvaz MA, Preston-Campbell RN, d'Oleire Uquillas F, Moeller SJ, et al. Habenula-prefrontal resting-state connectivity in reactive aggressive men—a pilot study. Neuropharmacology. (2019) 156:107396. doi: 10.1016/j.neuropharm.2018.10.025
43. Gouveia FV, Germann J, Elias GJ, Hamani C, Fonoff ET, Martinez RCR. Case report: 5 Years follow-up on posterior hypothalamus deep brain stimulation for intractable aggressive behaviour associated with drug-resistant epilepsy. Brain Stimul. (2021) 14:1201–4. doi: 10.1016/j.brs.2021.07.062
44. Baker PM, Jhou T, Li B, Matsumoto M, Mizumori SJY, Stephenson-Jones M, et al. The lateral habenula circuitry: reward processing and cognitive control. J Neurosci. (2016) 36:11482–8. doi: 10.1523/JNEUROSCI.2350-16.2016
45. Congiu M, Trusel M, Pistis M, Mameli M, Lecca S. Opposite responses to aversive stimuli in lateral habenula neurons. Eur J Neurosci. (2019) 50:2921–30. doi: 10.1111/ejn.14400
46. Valentinova K, Tchenio A, Trusel M, Clerke JA, Lalive AL, Tzanoulinou S, et al. Morphine withdrawal recruits lateral habenula cytokine signaling to reduce synaptic excitation and sociability. Nat Neurosci. (2019) 22:1053–6. doi: 10.1038/s41593-019-0421-4
47. Tan D, Nuno-Perez A, Mameli M, Meye FJ. Cocaine withdrawal reduces GABA R transmission at entopeduncular nucleus—lateral habenula synapses. Eur J Neurosci. (2019) 50:2124–33. doi: 10.1111/ejn.14120
48. Meye FJ, Trusel M, Soiza-Reilly M, Mameli M. Neural circuit adaptations during drug withdrawal—Spotlight on the lateral habenula. Pharmacol Biochem Behav. (2017) 162:87–93. doi: 10.1016/j.pbb.2017.08.007
49. Baker PM, Raynor SA, Francis NT, Mizumori SJY. Lateral habenula integration of proactive and retroactive information mediates behavioral flexibility. Neuroscience. (2017) 345:89–98. doi: 10.1016/j.neuroscience.2016.02.010
50. Golden SA, Heshmati M, Flanigan M, Christoffel DJ, Guise K, Pfau ML, et al. Basal forebrain projections to the lateral habenula modulate aggression reward. Nature. (2016) 534:688–92. doi: 10.1038/nature18601
51. Ferrari PF, van Erp AMM, Tornatzky W, Miczek KA. Accumbal dopamine and serotonin in anticipation of the next aggressive episode in rats. Eur J Neurosci. (2003) 17:371–8. doi: 10.1046/j.1460-9568.2003.02447.x
52. Beiderbeck DI, Reber SO, Havasi A, Bredewold R, Veenema AH, Neumann ID. High and abnormal forms of aggression in rats with extremes in trait anxiety–involvement of the dopamine system in the nucleus accumbens. Psychoneuroendocrinology. (2012) 37:1969–80. doi: 10.1016/j.psyneuen.2012.04.011
53. Couppis MH, Kennedy CH. The rewarding effect of aggression is reduced by nucleus accumbens dopamine receptor antagonism in mice. Psychopharmacology. (2008) 197:449–56. doi: 10.1007/s00213-007-1054-y
54. Becker EA, Marler CA. Postcontest blockade of dopamine receptors inhibits development of the winner effect in the California mouse. (Peromyscus californicus). Behav Neurosci. (2015) 129:205–13. doi: 10.1037/bne0000043
55. Harat M, Rudaś M, Zieliński P, Birska J, Sokal P. Deep brain stimulation in pathological aggression. Stereotact Funct Neurosurg. (2015) 93:310–5. doi: 10.1159/000431373
56. Park HR, Kim IH, Kang H, Lee DS, Kim B-N, Kim DG, et al. Nucleus accumbens deep brain stimulation for a patient with self-injurious behavior and autism spectrum disorder: functional and structural changes of the brain: report of a case and review of literature. Acta Neurochir. (2017) 159:137–43. doi: 10.1007/s00701-016-3002-2
57. Harat M, Kiec M, Rudaś M, Birski M, Furtak J. Treating aggression and self-destructive behaviors by stimulating the nucleus accumbens: a case series. Front Neurol. (2021) 12:706166. doi: 10.3389/fneur.2021.706166
58. Yan H, Elkaim LM, Venetucci Gouveia F, Huber JF, Germann J, Loh A, et al. Deep brain stimulation for extreme behaviors associated with autism spectrum disorder converges on a common pathway: a systematic review and connectomic analysis. J Neurosurg. (2022) 1–10. doi: 10.3171/2021.11.JNS21928. [Epub ahead of print].
59. Baño-Otálora B, Piggins HD. Contributions of the lateral habenula to circadian timekeeping. Pharmacol Biochem Behav. (2017) 162:46–54. doi: 10.1016/j.pbb.2017.06.007
60. Salaberry NL, Hamm H, Felder-Schmittbuhl M-P, Mendoza J. A suprachiasmatic-independent circadian clock(s) in the habenula is affected by Per gene mutations and housing light conditions in mice. Brain Struct Funct. (2019) 224:19–31. doi: 10.1007/s00429-018-1756-4
61. Shuboni-Mulligan DD, Cavanaugh BL, Tonson A, Shapiro EM, Gall AJ. Functional and anatomical variations in retinorecipient brain areas in and: implications for the circadian and masking systems. Chronobiol Int. (2019) 36:1464–81. doi: 10.1080/07420528.2019.1651325
62. Sakhi K, Belle MDC, Gossan N, Delagrange P, Piggins HD. Daily variation in the electrophysiological activity of mouse medial habenula neurones. J Physiol. (2014) 592:587–603. doi: 10.1113/jphysiol.2013.263319
63. Yu EZ, Hallenbeck JM, Cai D, McCarron RM. Elevated arylalkylamine-N-acetyltransferase. (AA-NAT) gene expression in medial habenular and suprachiasmatic nuclei of hibernating ground squirrels. Brain Res Mol Brain Res. (2002) 102:9–17. doi: 10.1016/S0169-328X(02)00138-9
64. Bronsard G, Bartolomei F. Rhythms, rhythmicity and aggression. J Physiol Paris. (2013) 107:327–34. doi: 10.1016/j.jphysparis.2013.03.002
65. Benca R, Duncan MJ, Frank E, McClung C, Nelson RJ, Vicentic A. Biological rhythms, higher brain function, and behavior: gaps, opportunities, and challenges. Brain Res Rev. (2009) 62:57–70. doi: 10.1016/j.brainresrev.2009.09.005
66. O'Brien LM. The neurocognitive effects of sleep disruption in children and adolescents. Child Adolesc Psychiatr Clin N Am. (2009) 18:813–23. doi: 10.1016/j.chc.2009.04.008
67. Dahl RE. Sleeplessness and aggression in youth. J Adolesc Health Care. (2006) 38:641–2. doi: 10.1016/j.jadohealth.2006.03.013
68. Reid GJ, Hong RY, Wade TJ. The relation between common sleep problems and emotional and behavioral problems among 2- and 3-year-olds in the context of known risk factors for psychopathology. J Sleep Res. (2009) 18:49–59. doi: 10.1111/j.1365-2869.2008.00692.x
69. Basnakova A, Cheng R-K, Chia JSM, D'Agostino G, Suryadi Tan GJH, et al. The habenula clock influences response to a stressor. Neurobiol Stress. (2021) 15:100403. doi: 10.1016/j.ynstr.2021.100403
70. Wang RY, Aghajanian GK. Physiological evidence for habenula as major link between forebrain and midbrain raphe. Science. (1977) 197:89–91. doi: 10.1126/science.194312
71. Varga V, Kocsis B, Sharp T. Electrophysiological evidence for convergence of inputs from the medial prefrontal cortex and lateral habenula on single neurons in the dorsal raphe nucleus. Eur J Neurosci. (2003) 17:280–6. doi: 10.1046/j.1460-9568.2003.02465.x
72. Portas CM, Bjorvatn B, Ursin R. Serotonin and the sleep/wake cycle: special emphasis on microdialysis studies. Prog Neurobiol. (2000) 60:13–35. doi: 10.1016/S0301-0082(98)00097-5
73. Monti JM. Serotonin control of sleep-wake behavior. Sleep Med Rev. (2011) 15:269–81. doi: 10.1016/j.smrv.2010.11.003
74. Roman V, Walstra I, Luiten PGM, Meerlo P. Too little sleep gradually desensitizes the serotonin 1A receptor system. Sleep. (2005) 28:1505–10. doi: 10.1093/sleep/28.12.1505
75. Edelsohn GA, Abright AR. Editorial: Safer Use of Antipsychotics in Youth (SUAY): should treatment be guided by symptoms? J Am Acad Child Adolesc Psychiatry. (2021) 61:34–36. doi: 10.1016/j.jaac.2021.07.009
76. Vaudreuil C, Farrell A, Wozniak J. Psychopharmacology of treating explosive behavior. Child Adolesc Psychiatr Clin N Am. (2021) 30:537–60. doi: 10.1016/j.chc.2021.04.006
77. Lea-Banks H, O'Reilly MA, Hynynen K. Ultrasound-responsive droplets for therapy: a review. J Control Release. (2019) 293:144–54. doi: 10.1016/j.jconrel.2018.11.028
78. Lea-Banks H, Meng Y, Wu S-K, Belhadjhamida R, Hamani C, Hynynen K. Ultrasound-sensitive nanodroplets achieve targeted neuromodulation. J Control Release. (2021) 332:30–9. doi: 10.1016/j.jconrel.2021.02.010
79. Lea-Banks H, O'Reilly MA, Hamani C, Hynynen K. Localized anesthesia of a specific brain region using ultrasound-responsive barbiturate nanodroplets. Theranostics. (2020) 10:2849–58. doi: 10.7150/thno.41566
80. Lozano AM, Lipsman N. Probing and regulating dysfunctional circuits using deep brain stimulation. Neuron. (2013) 77:406–24. doi: 10.1016/j.neuron.2013.01.020
81. Wang Y, Zhang C, Zhang Y, Gong H, Li J, Jin H, et al. Habenula deep brain stimulation for intractable schizophrenia: a pilot study. Neurosurg Focus. (2020) 49:E9. doi: 10.3171/2020.4.FOCUS20174
82. Sartorius A, Kiening KL, Kirsch P, von Gall CC, Haberkorn U, Unterberg AW, et al. Remission of major depression under deep brain stimulation of the lateral habenula in a therapy-refractory patient. Biol Psychiatry. (2010) 67:e9–11. doi: 10.1016/j.biopsych.2009.08.027
83. Wang Z, Cai X, Qiu R, Yao C, Tian Y, Gong C, et al. Case report: lateral habenula deep brain stimulation for treatment-resistant depression. Front Psychiatry. (2020) 11:616501. doi: 10.3389/fpsyt.2020.616501
84. Zhang C, Zhang Y, Li D, Deng Z, Nuttin B, Voon V, et al. Habenular stimulation for neurosurgery resistant obsessive-compulsive disorder: a case report. Front Psychiatry. (2020) 11:29. doi: 10.3389/fpsyt.2020.00029
85. Zhang C, Kim S-G, Li D, Zhang Y, Li Y, Husch A, et al. Habenula deep brain stimulation for refractory bipolar disorder. Brain Stimul. (2019) 12:1298–300. doi: 10.1016/j.brs.2019.05.010
Keywords: habenula, aggressive behavior (AB), neuropsychiatric symptoms, preclinical studies, review
Citation: Gouveia FV and Ibrahim GM (2022) Habenula as a Neural Substrate for Aggressive Behavior. Front. Psychiatry 13:817302. doi: 10.3389/fpsyt.2022.817302
Received: 17 November 2021; Accepted: 25 January 2022;
Published: 17 February 2022.
Edited by:
Ali Saffet Gonul, Ege University, TurkeyReviewed by:
Yukiori Goto, Kyoto University, JapanCopyright © 2022 Gouveia and Ibrahim. This is an open-access article distributed under the terms of the Creative Commons Attribution License (CC BY). The use, distribution or reproduction in other forums is permitted, provided the original author(s) and the copyright owner(s) are credited and that the original publication in this journal is cited, in accordance with accepted academic practice. No use, distribution or reproduction is permitted which does not comply with these terms.
*Correspondence: Flavia Venetucci Gouveia, ZnZlbmV0dWNjaUBnbWFpbC5jb20=
Disclaimer: All claims expressed in this article are solely those of the authors and do not necessarily represent those of their affiliated organizations, or those of the publisher, the editors and the reviewers. Any product that may be evaluated in this article or claim that may be made by its manufacturer is not guaranteed or endorsed by the publisher.
Research integrity at Frontiers
Learn more about the work of our research integrity team to safeguard the quality of each article we publish.