- 1Department of Psychiatry, Chang'an Hospital, Xi'an, China
- 2Department of Psychiatry, Xijing Hospital, Air Force Medical University, Xi'an, China
Lipidomics has become a pivotal tool in biomarker discovery for the diagnosis of psychiatric illnesses. However, the composition and quantitative analysis of peripheral lipids in female patients with bipolar disorder (BD) have been poorly addressed. In this study, plasma samples from 24 female patients with BD and 30 healthy controls (HCs) were analyzed by comprehensive lipid profiling and quantitative validation based on liquid chromatography–mass spectrometry. Clinical characteristics and a correlation between the level of lipid molecules and clinical symptoms were also observed. We found that the quantitative alterations in several lipid classes, including acylcarnitine, lysophosphatidylethanolamine, GM2, sphingomyelin, GD2, triglyceride, monogalactosyldiacylglycerol, phosphatidylinositol phosphate, phosphatidylinositol 4,5-bisphosphate, phosphatidylethanolamine, phosphatidylserine, and lysophosphatidylinositol, were remarkably upregulated or downregulated in patients with BD and were positively or negatively correlated with the severity of psychotic, affective, or mania symptoms. Meanwhile, the composition of different carbon chain lengths and degrees of fatty acid saturation for these lipid classes in BD were also different from those of HCs. Moreover, 55 lipid molecules with significant differences and correlations with the clinical parameters were observed. Finally, a plasma biomarker set comprising nine lipids was identified, and an area under the curve of 0.994 was obtained between patients with BD and the HCs. In conclusion, this study provides a further understanding of abnormal lipid metabolism in the plasma and suggests that specific lipid species can be used as complementary biomarkers for the diagnosis of BD in women.
Introduction
Bipolar disorder (BD) is a severe and episodic mental illness characterized by mood alterations between euthymia, major depression, and mania (1). It affects approximately 3% of the general population (2), and its estimated lifetime prevalence ranges from 0.5 to 2.4% worldwide (3, 4). Moreover, current drug treatments are effective in only 40–60% of cases and cause severe side effects (5, 6). The association of BD with impaired quality of life and the high economic burden have been widely accepted (7, 8). Accurate early detection and intervention during the prodromal stages of BD may help avert the burden by decreasing symptom severity and preventing progression to full disorder status (9, 10). Notably, BD is frequently misdiagnosed as a major depressive disorder (MDD) because of overlapping symptomology, later onset of mania, and frequent depressive episodes (11). The incorrect treatment of BD with antidepressant monotherapy increases the risk of mania and the frequency of episodes, both of which can have detrimental effects on disease prognosis (12, 13). Therefore, the development of specific biomarkers to increase the diagnostic accuracy of BD is required.
Women are nearly twice as likely to develop MDD during their lifetime compared with men (14, 15). Although there is no sex difference in the incidence rate of BD, women are more likely to have precipitous changes between depression and hypomania/mania and episodes of both depressive and manic symptoms (16, 17); therefore, the misdiagnosis of BD in women is more common than in men. Notably, women with BD are at an increased risk of having a serious episode of illness in relation to pregnancy and childbirth (18, 19), and BD is considered the sixth leading cause of disability among women of reproductive age (4). Furthermore, the objective biomarkers for diagnosis have not been clearly identified, and specific medications for the treatment of BD in women are clinically unsatisfactory. Therefore, clarifying the pathological features of BD in women may provide a basis for the development of new diagnostic methods and treatment strategies.
Lipids play an important role in regulating and maintaining neuronal development and function (20–22), and their involvement in modulating synaptic physiology, axonal membranes, and energy metabolism in the brain has been largely demonstrated (23–25). Recently, lipidomic analysis based on liquid chromatography (LC)–mass spectrometry (MS) has allowed for the comprehensive identification of hundreds to thousands of lipid molecular species in tissues and plasma (26, 27), and lipidomics has become established as a pivotal tool for biomarker discovery for the diagnosis of psychiatric diseases (28). Studies have observed the composition of peripheral fatty acid and phospholipid classes in BD (29–31), and a MS-based serum lipidomics study also identified the serum lipid profiles between BD and healthy control (HC) groups (32); however, there is still a need for quantitative analysis of the changes in lipid species. Currently, there is only an indirect and limited understanding of peripheral lipid characteristics in women with BD.
Considering the above-mentioned information, this study sought to determine the differences in lipidomic composition of plasma samples from female patients with BD and the HCs and to analyze the correlation between different lipid profiles and symptoms. These data provide insights into lipidomic alterations in female patients with BD and may guide further studies on the diagnosis of BD.
Methods
Subjects and Plasma Sampling
The study protocol was approved by the Chinese Clinical Trial Ethics Committee (approval no. ChiECRCT20200090) and was registered with the Chinese Clinical Trial Registry (registration no. ChiCTR2000032118). This study was performed in accordance with the Declaration of Helsinki. All participants volunteered to participate in this study and provided written informed consent. Twenty-four female patients (22–58 years of age) who met the criteria for BD of the fifth edition of the Diagnostic and Statistical Manual of Mental Disorders (DSM-5) were recruited from the Department of Psychiatry of Chang'an Hospital, Xi'an, China, along with 30 healthy females (22–53 years of age). All participants underwent physical examination. The Mini-International Neuropsychiatric Interview was used to screen for preexisting psychiatric disorders.
The Hamilton Depression Rating Scale (HAMD), Hamilton Anxiety Scale (HAMA), Positive and Negative Syndrome Scale (PANSS), and Bech–Rafaelsen Mania Rating Scale (BRMS) tests were independently administered by two psychiatrists who were blinded to the clinical status of the participants and had attended a training session on how to administer the tests before the start of the study. The exclusion criteria were diseases of the digestive system; obesity, which was defined as a body mass index (BMI) ≥ 28.0; hypertension; participants with a severely imbalanced diet, such as high-fat diet preferences; pregnancy, lactation, or menstrual period; and presence of other mental disorders according to the DSM-5 criteria. The same exclusion criteria used for the BD group were also applied to the HC group.
Blood samples were collected between 8 and 10 a.m. from all individuals under fasting conditions. The blood was collected in anticoagulant tubes and centrifuged at 1,600 rpm for 15 min. The obtained plasma was aliquoted into sterile cryopreservation tubes and stored in liquid nitrogen until further analysis.
Quantitative Lipidomics
Experiments and data analysis were performed as previously described (33, 34) and were supported by Shanghai Applied Protein Technology Co., Ltd. For the sample preparation, plasma (100 μl) was accurately measured and spiked with appropriate amounts of internal lipid standards (SPLASH® LIPIDOMIX® Mass Spec Standard, methanol solution, AVANTI, 330707-1EA, Merck) and homogenized with 200 μl water and 240 μl methanol. Thereafter, 800 μl methyl tert-butyl ether was added, and the samples were subjected to ultrasonication for 20 min at 4°C and allowed to rest for 30 min at room temperature. The solution was then centrifuged at 10°C for 15 min (14,000 × g), and the supernatant was separated for analysis. For the LC-MS/MS method, the samples were separated using a UHPLC Nexera LC-30A ultra-high-performance LC system with a C18 column (ACQUITY UPLC CSH C18, 1.7 μm, 2.1 × 100 mm, Waters). The column temperature was 45°C, and the flow rate was 300 μl/min. The lipid extracts were redissolved in 200 μl of 90% isopropanol/acetonitrile and centrifuged at 14,000 × g for 15 min, and 3 μl of the sample was injected. Solvent A was acetonitrile–water (6:4, v/v) with 0.1% formic acid and 0.1 mM ammonium formate. Solvent B was acetonitrile–isopropanol (1:9, v/v) with 0.1% formic acid and 0.1 mM ammonium formate. The initial mobile phase was 30% solvent B at a flow rate of 300 μl/min. It was held for 2 min and then linearly increased to 100% solvent B for 23 min, followed by equilibration with 30% solvent B for 25 min. During the whole analysis, the samples were placed in an automatic sampler at 10°C. To avoid the influence caused by the fluctuation of the instrument detection signal, a random sequence was used for the continuous analysis of samples. Mass spectra were acquired using Q-Exactive Plus (Thermo Fisher Scientific, USA) in positive and negative modes. The following electrospray ionization parameters were optimized and preset for all measurements: 300°C, source temperature; 350°C, capillary temperature; 3,000 V, ion spray voltage; 50% S-Lens RF level; and m/z 200–1,800, instrument scan range.
LipidSearch software (Thermo Fisher Scientific) was used for peak recognition, peak extraction, and lipid identification (secondary identification) of lipid molecules and internal standard lipid molecules, which contain more than 30 lipid classes and more than 1,500,000 fragment ions in the database. Single-point internal standard calibrations were used to estimate the absolute concentrations of unique lipids identified by accurate MS, MS/MS spectral matching, and retention times (35). The main parameters were 5 ppm precursor tolerance, 5 ppm product tolerance, and 5% production threshold.
Statistical Analyses
Differences in descriptive data were assessed using the chi-square test for categorical variables, and a non-parametric Mann–Whitney U-test or two-tailed Student's t-test was used for continuous variables. Lipid concentration is presented as mean ± standard deviation and was analyzed using two-tailed Student's t-test. Statistical significance was set at P < 0.05. Different lipid species were screened by fold change analysis, and the correlation between lipid concentrations and clinical parameters was analyzed by Spearman correlation. Potential biochemical markers were further evaluated using receiver operating characteristic (ROC) analysis, including transformation by a logistic regression model to the predicted probability scale. The area under the ROC curve (AUC) was used to assess the sensitivity and specificity of biomarkers.
Results
Clinical Characteristics of the Recruited Participants
A total of 24 women with BD and 30 female HCs were included in this study. No significant differences were found between the BD and HC groups in terms of age (P = 0.069), BMI (P = 0.984), and marital status (P = 0.321). The HAMD, HAMA, PANSS, and BRMS scale scores in the BD group were higher than those in the HC group (Table 1). Supplementary Table 1 shows the detailed clinical and demographic characteristics of the studied samples.
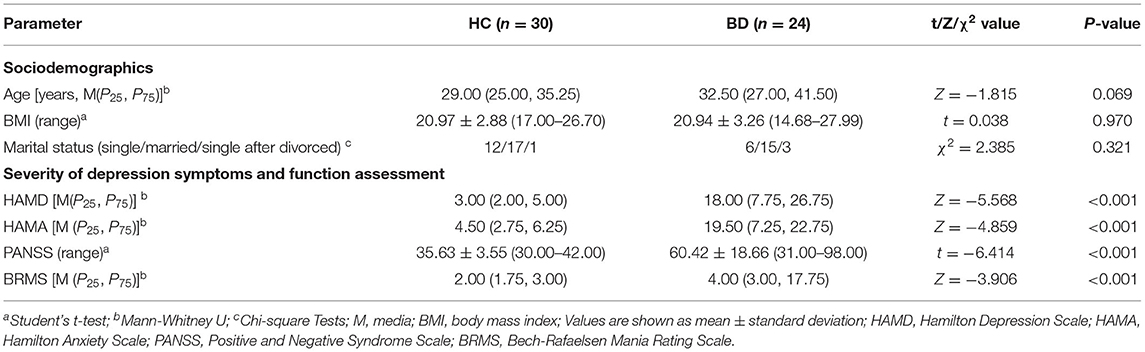
Table 1. Comparison of clinical characteristics data and symptom scale assessment between women with BD and HC.
Different Lipid Classes Between the BD and HC Groups and Their Correlation With Clinical Parameters
A total of 31 lipid classes and 884 lipid species were identified in the samples of each group (Supplementary Table 2). Significant differences were observed in the concentrations of several classes of fatty acids (Figure 1A), saccharolipids, prenol lipids (Figure 1B), glycerolipids (Figure 1C), sphingolipids (Figure 1D), and glycerophospholipids (Figure 1E) between the HC and BD groups. The concentrations of wax esters (WEs) (t = 2.739, P = 0.008), acylcarnitine (AcCa) (t = 6.683, P < 0.001), sphingomyelin (SM) (t = 2.731, P = 0.009), CerG2GNAc1 (t = 2.062, P = 0.045), coenzyme (Co) (t = 2.183, P = 0.034), monogalactosyldiacylglycerol (MGDG) (t = 3.334, P = 0.002), phosphatidylserine (PS) (t = 3.595, P = 0.001), and phosphatidylethanolamine (PE) (t = 3.887, P < 0.001) were significantly lower in the BD group. By contrast, the concentrations of phosphatidylinositol (PI) (t = −2.618, P = 0.012), lysophosphatidylcholine (LPC) (t = −2.116, P = 0.040), lysophosphatidylethanolamine (LPE) (t = −6.675, P < 0.001), lysophosphatidylinositol (LPI) (t = −4.019, P < 0.001), PI phosphate (PIP) (t = −4.001, P < 0.001), PI 4,5-bisphosphate (PIP2) (t = −4.140, P < 0.001), ceramides (Cer) (t = −2.439, P = 0.019), Cer phosphate (CerP) (t = −2.837, P = 0.009), GD2 (t = −3.003, P = 0.005), GM2 (t = −5.060, P < 0.001), triglyceride (TG) (t = −2.891, P = 0.008), and monoglyceride (t = −2.082, P = 0.045) were significantly increased in the BD group.
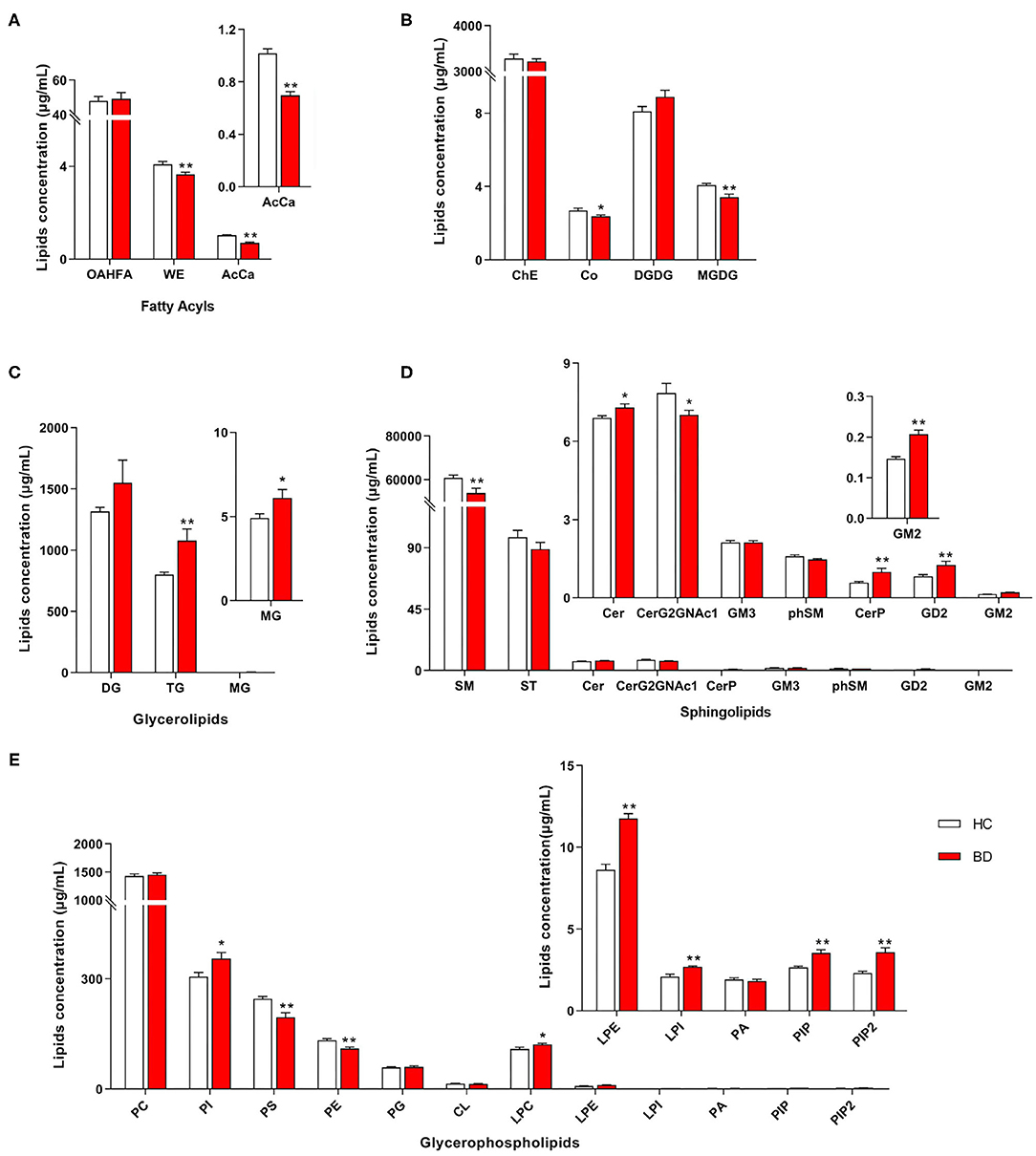
Figure 1. Different concentrations of lipid class between the BD and HC groups. (A) Fatty acyls, (B) ChE, Co, DGDG, and MGDG, (C) glycerolipids, (D) sphingolipids, and (E) glycerophospholipids. HC, healthy controls. Lipids showing low-level results have been enlarged in the insets. BD, bipolar disorder; OAHFA, (O-acyl)-1-hydroxy fatty acid; WE, wax esters; AcCa, acylcarnitine; DG, diglyceride; TG, triglyceride; MG, monoglyceride; SM, sphingomyelin; ST, sulfatide; Cer, ceramides; CerP, ceramides phosphate; phSM, phytosphingomyelin; ChE, cholesterol ester; Co, coenzyme; MGDG, monogalactosyldiacylglycerol; DGDG, digalactosyldiacylglycerol; PC, phosphatidylcholine; PI, phosphatidylinositol; PS, phosphatidylserine; PE, phosphatidylethanolamine; PG, phosphatidylglycerol; CL, cardiolipin; LPC, lysophosphatidylcholine; LPE, lysophosphatidylethanolamine; LPI, lysophosphatidylinositol; PA, phosphatidic acid; PIP, phosphatidylinositol phosphate; PIP2, phosphatidylinositol 4,5-bisphosphate. *P < 0.05 vs. HC; **P < 0.01 vs. HC.
Furthermore, the concentrations of PE, PS, AcCa, MGDG, and Co were negatively correlated with the total scores of PANSS, whereas the concentrations of PIP, PIP2, Cer, CerP, GM2, LPE, PI, and GD2 were positively correlated with the total scores of PANSS (Figure 2A). The concentrations of PE, PS, AcCa, MGDG, SM, phytosphingomyelin, and sulfatide were negatively correlated with the scores of both HAMA and HAMD, whereas the concentrations of PIP, CerP, GM2, LPE, and PI were positively correlated with the scores of both HAMA and HAMD. The concentrations of CerG2GNAc1, WE, and Co were negatively correlated with the scores of HAMD, whereas the concentrations of PIP2 were positively correlated with the scores of HAMD. The concentrations of Co were also negatively correlated with the scores of HAMA. Notably, the concentrations of PE and PS were negatively correlated with the scores of BRMS, whereas the concentrations of GM2, DGDG, and GD2 were positively correlated with the scores of BRMS (detailed data are displayed in Supplementary Table 3). Taken together, the concentrations of 8 and 12 lipids in the BD group, respectively, decreased and increased at the class level compared with those in the HC group. Moreover, changes in these lipid classes were related to the severity of psychotic, affective, and mania symptoms.
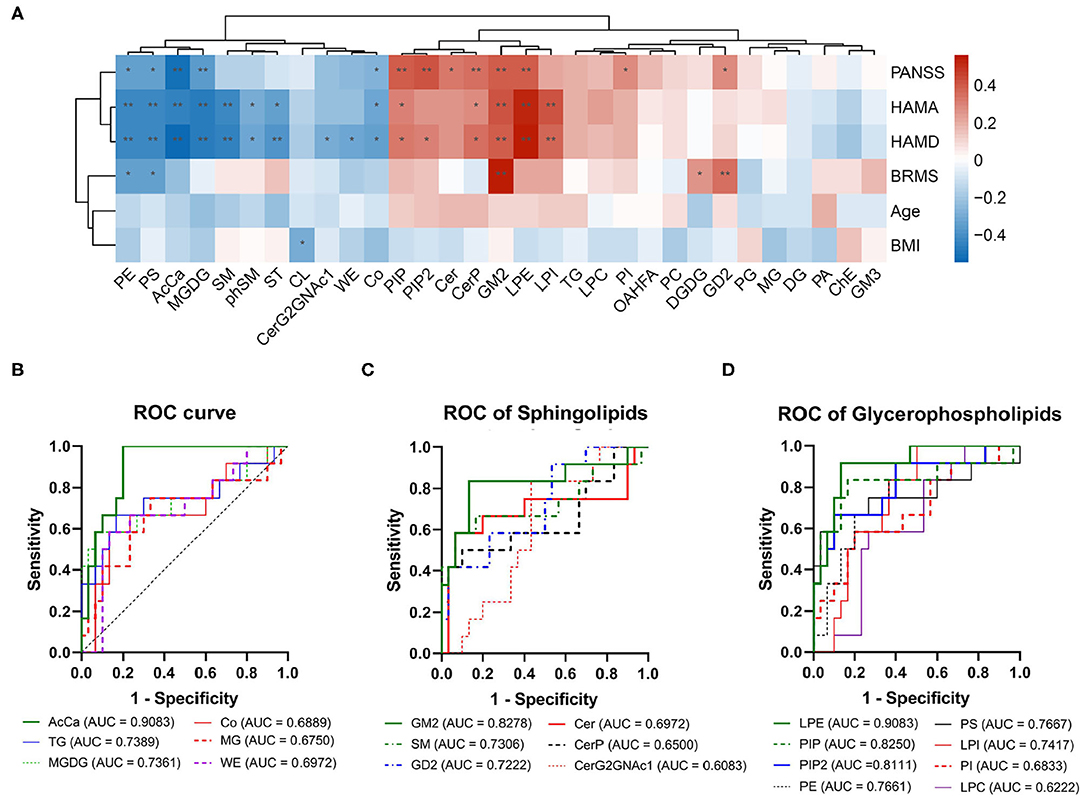
Figure 2. Correlation between clinical parameters and receiver operating characteristic (ROC) analysis for the different lipid classes. (A) Correlation analysis for levels of lipid classes and clinical parameters. The red squares show a positive correlation, and the blue squares show a negative correlation. *P < 0.05; **P < 0.01. (B) ROC of acylcarnitine, triglyceride, monogalactosyldiacylglycerol, coenzyme, monoglyceride, and wax esters. (C) ROC of sphingolipids. (D) ROC of glycerophospholipids.
ROC Analysis for Lipid Classes
ROC test analysis was performed to verify the specificity and predictive accuracy of the different lipid classes, which could be used to distinguish patients with BD from normal controls. As shown in Figure 2, the AUC of both AcCa and LPE was >0.9, thus indicating high reliability (Figures 2B,D). By contrast, GM2, SM, GD2, TG, MGDG, PIP, PIP2, PE, PS, and LPI showed moderate reliability (the AUC value was between 0.7 and 0.9; Figures 2B–D), thus suggesting that changes in AcCa and LPE might be potential markers for BD diagnosis at the class level.
Different Carbon Chain Lengths and Degree of Saturation of Fatty Acid Between the BD and HC Groups
We observed significant alterations in the fatty acid chain profile of lipids between the HC and BD groups (Figure 3). The levels of long-chain fatty acids with 16 carbons, 17 carbons, and more than 44 carbons increased, whereas the levels of long-chain fatty acids with 20 to 44 carbons (20 carbons ≤ carbon length ≤ 44 carbons), except for 25–32 carbons, 34 carbons, 38 carbons, 39 carbons, and 42 carbons, decreased in the BD group (BD vs. HC, P < 0.05) (Figure 3A). Additionally, significant alterations in the degree of carbon chain saturation were observed between the HC and BD groups. In the BD group, the levels of saturated fatty acids, monounsaturated fatty acids, and polyunsaturated fatty acids with five double bonds, eight double bonds, and nine double bonds increased, whereas the levels of polyunsaturated fatty acids with six double bonds and more than nine double bonds decreased (BD vs. HC, P < 0.05) (Figure 3B). Furthermore, alterations in the concentrations of different lipid classes with different carbon chains and unsaturated fatty acids in the plasma of BD were also observed (Supplementary Figures 1, 2). Therefore, changes in plasma lipids in BD affected not only the lipid concentration but also the carbon chain length and degree of saturation of the fatty acid chain.
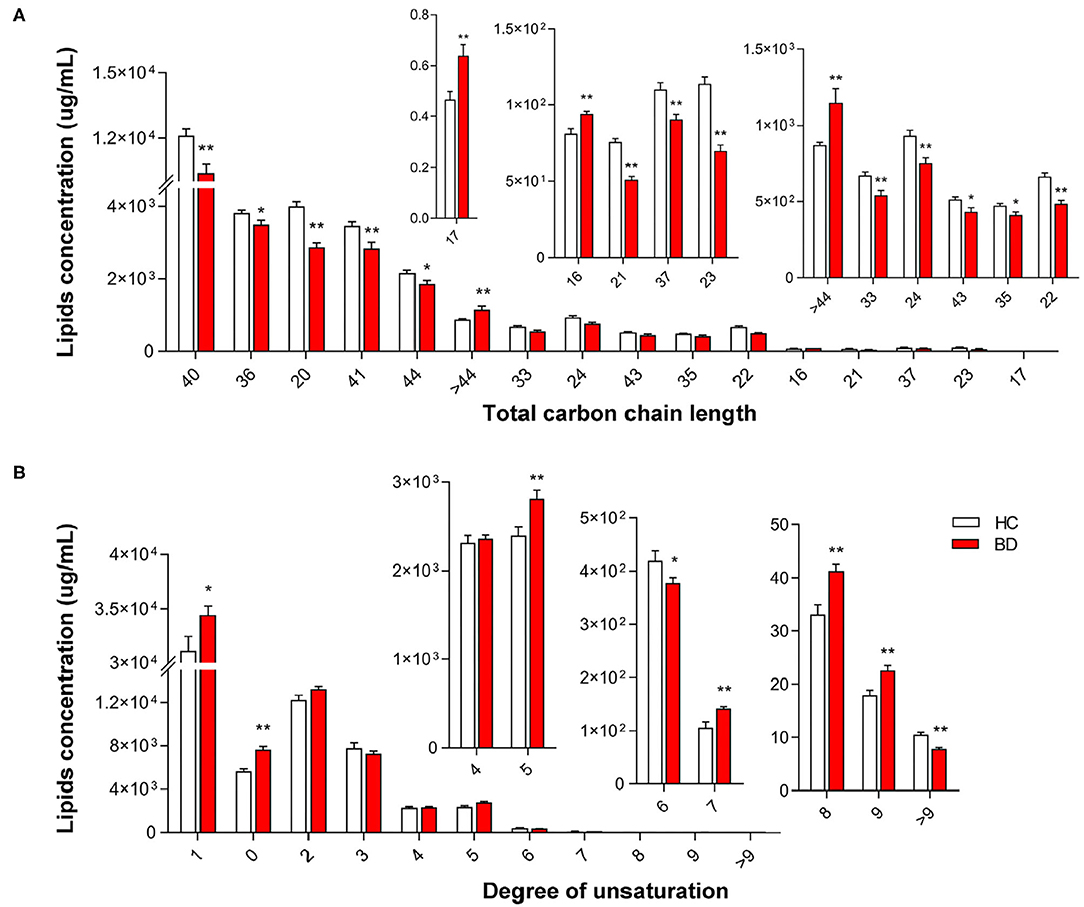
Figure 3. Groupwise alterations in the fatty acid composition of plasma lipids. Results of the analysis of fatty acyl composition by (A) chain length (number of carbons) and (B) degree of unsaturation. Lipids showing low-level results have been enlarged in the insets. *P < 0.05 vs. healthy controls (HC); **P < 0.01 vs. HC.
Characteristic Lipid Species in the BD Group and Its Correlation With Clinical Parameters
Lipidomic profiling further revealed changes in lipid concentrations between the BD and HC groups at the species level (Figure 4A). In general, in the BD group, the concentrations of 12 lipids, such as PC(37:5)+H, PS(16:1e/22:4)-H, and PS(42:9e)-H, decreased; by contrast, the levels of 43 lipids, such as TG(18:0/8:0/20:4)+Na, PI(16:0/16:1)-H, and PC(8:0e/6:0)+Na, increased (Table 2). A correlation analysis further showed that the concentrations of PC(17:1/18:2)+H, PC(36:6e)+H, PS(16:1e/22:4)-H, DG(21:5e)+NH4, PS(42:9e)-H, PC(18:2e/22:5)+H, SM(d43:1)+H, PI(16:1)+H, DG(20:4e)+NH4, AcCa(14:1)+H, and AcCa(14:2)+H were negatively correlated with the PANSS, HAMA, and HAMD scores (Figure 4B). By contrast, the concentrations of PC(8:0e/6:0)+Na, PC(37:4e)+H, TG(20:0/18:1/18:1)+NH4, TG(12:0/18:2/20:4)+NH4, PI(16:0/16:0)-H, PI(16:0/16:1)-H, PI(18:0/18:3)-H, and TG(18:4/20:4/20:5)+H were positively correlated with the PANSS, HAMA, and HAMD scores, thus suggesting that the changes in these lipid species were related to the severity of psychotic and affective symptoms. Notably, the concentrations of PC(17:1/18:2)+H, PC(36:6e)+H, PS(16:1e/22:4)-H, DG(21:5e)+NH4, PS(42:9e)-H, PC(18:2e/22:5)+H, SM(d43:1)+H, and PI(16:1)+H were negatively correlated with the BRMS scores, whereas the concentrations of PC(37:4e)+H, PC(12:0e/10:1)+H, PC(8:0e/6:0)+Na, TG(16:0/20:4/20:5)+NH4, TG(18:1/18:2/22:4)+NH4, TG(16:0/20:4/22:5)+NH4, LPC(20:5)+HCOO, TG(18:3/18:2/20:4)+Na, TG(20:5/18:2/20:4)+NH4, TG(18:3/18:2/20:5)+NH4, and TG(16:1/20:5/22:6)+Na were positively correlated with the BRMS scores (detailed data are displayed in Supplementary Table 4), thus indicating that the changes in these lipid molecules were related to mania symptoms.
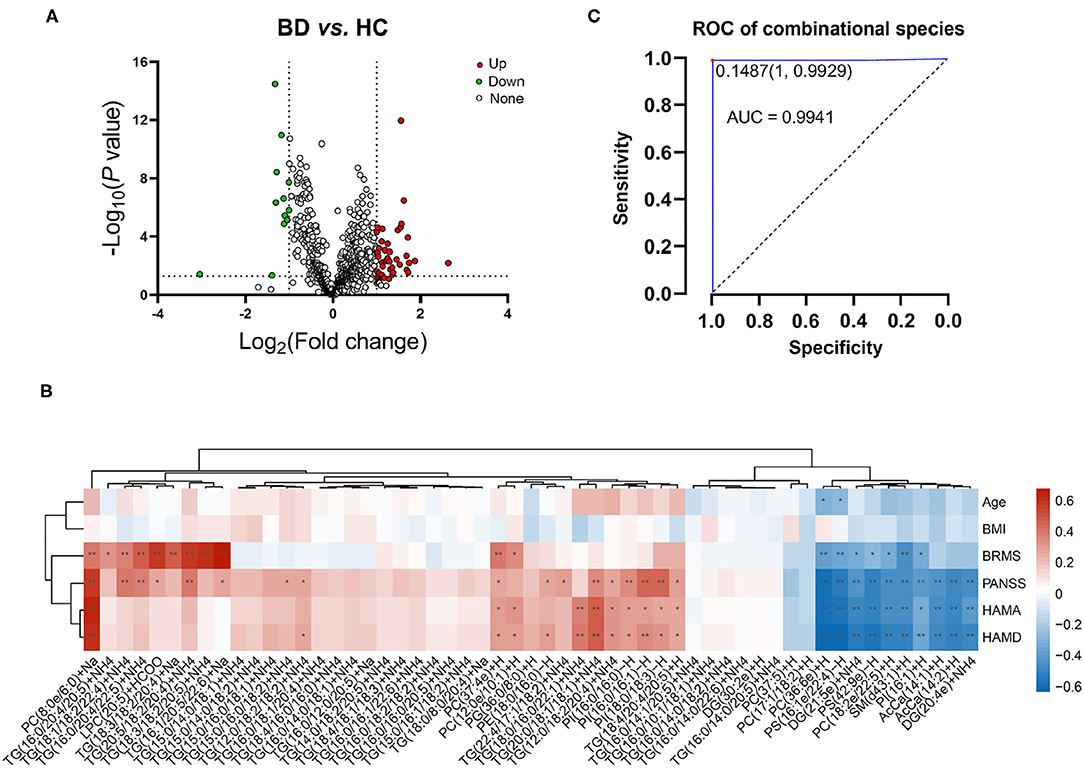
Figure 4. Characterization of lipid species in BD and their correlation with clinical parameters. (A) Volcano map revealing the decrease (green dots) and increase (red dots) in lipid species between patients with BD and the healthy controls. (B) Correlation between the clinical parameters and levels of lipid species in plasma. *P < 0.05; **P < 0.01. (C) Receiver operating characteristic of combinational molecules: PS(42:9e)-H, DG(21:5e)+NH4, PC(36:6e)+H, PC(8:0e/6:0)+Na, PS(16:1e/22:4)-H, TG(16:0/16:1/22:6)+NH4, TG(16:0/20:4/22:5)+NH4, and TG(22:4/17:1/18:2)+NH4, TG(18:0/8:0/20:4)+Na.
Potential Lipid Biomarkers of BD
The ROC analysis revealed that 38 lipid species, such as TG(18:1/18:2/22:4)+NH4, AcCa(14:2)+H, and PC(18:2e/22:5)+H, showed moderate or high reliability (AUC >0.7; Supplementary Table 5). Notably, the AUC of PS(42:9e)-H, DG(21:5e)+NH4, PC(36:6e)+H, PC(8:0e/6:0)+Na, PS(16:1e/22:4)-H, TG(16:0/16:1/22:6)+NH4, TG(16:0/20:4/22:5)+NH4, TG(22:4/17:1/18:2)+NH4, and TG(18:0/8:0/20:4)+Na, respectively, which covered different lipid species and demonstrated different change tendencies in BD subjects, was higher than 0.9 and was defined as a combinational biomarker. We then transformed the concentrations of these nine lipids to the predicted probability scale by using a logistic regression model, and the results showed high sensitivity and specificity (AUC = 0.994, 95% CI: 0.9826–1, P < 0.001) (Figure 4C). Therefore, the characteristic changes in these species may be a peripheral diagnostic biomarker of BD.
Discussion
We investigated the characteristics of peripheral lipid composition and potential lipid biomarkers for female BD patients by comprehensive lipid profiling and quantitative validation based on LC–MS/MS. The lipidomic analysis showed that alterations in several lipid classes, such as AcCa and LPE, were remarkably changed in patients with BD, which were positively or negatively correlated with the severity of psychotic, affective, or mania symptoms. We also found that the composition of different carbon chain lengths and degrees of saturation of fatty acids in BD also differed from those in HCs. Moreover, we screened 55 lipid molecules with significant differences and investigated their correlations with clinical parameters. Finally, we identified a plasma biomarker set that comprised several lipids, including PS(42:9e)-H, DG(21:5e)+NH4, PC(36:6e)+H, PC(8:0e/6:0)+Na, PS(16:1e/22:4)-H, TG(16:0/16:1/22:6)+NH4, TG(16:0/20:4/22:5)+NH4, TG(22:4/17:1/18:2)+NH4, and TG(18:0/8:0/20:4)+Na, which can be used to differentiate female patients with BD from HCs.
The biological functions of lipids are associated with multiple processes, including inflammation, apoptosis, proliferation, and differentiation (36, 37), and disturbances in lipid metabolism have been demonstrated in various neuropsychiatric diseases (38, 39). Although changes in plasma lipids have also been observed in mental disorders, including schizophrenia, depression, and BD (40, 41), we believe that the current study is the first study to quantitatively compare plasma lipid composition in adult women with BD vs. HCs. Herein we found that the levels of SM, AcCa, PE, PS, and Co decreased in BD and were negatively correlated with the severity of psychotic, affective, or mania symptoms. Previous studies also found that plasma SM was negatively correlated with depressive symptoms (42), and lower concentrations of AcCa and PE were also observed in the plasma of BD patients than that of HCs (43–45). Considering that PS biosynthesis via the serine base exchange reaction using PE and PS can be converted to PE by PS decarboxylase in the mitochondria (46), the decrease in PS levels may be related to the reduction of PE. Similarly, although a decrease in plasma CoQ10 has not been reported in BD, adjuvant CoQ10 might be considered a safe and effective strategy for the treatment of patients with BD during their depressive phase (47). On the other hand, we also found that the levels of Cer, CerP, PI, LPE, LPI, PIP, and PIP2 increased in BD and were positively correlated with the severity of psychotic, affective, or mania symptoms. Consistent with this finding, previous studies have found that plasma Cer was substantially increased in patients with major depression and BD irrespective of the severity of symptoms in the current episode (48). PIP2 was increased in the platelet membrane of drug-free depressed bipolar patients, whereas therapeutic doses of lithium significantly decreased the platelet membrane PIP2 levels in vivo in BD subjects (49, 50). Moreover, although changes in plasma LPE and PI have not been reported in BD, a previous work reported that LPE and PI were remarkably increased in MDD and showed pronounced positive relationships with depression severity (51).
Notably, the ROC analysis further found that AcCa and LPE showed high reliability (AUC > 0.9) in distinguishing BD from HCs. AcCa is a class of metabolites that are formed from the transfer of the acyl group of fatty acyl-CoA to carnitine (52) and is involved in mitochondrial function and energy metabolism, antioxidative functions, and neuroprotection (53, 54). LPE is a lyso-type metabolite of PE produced via a phospholipase A-type reaction, and only a limited number of studies have reported that serum LPE was significantly decreased in patients with migraine and non-alcoholic fatty liver disease (55, 56). Furthermore, a decrease in MGDG and an increase in GD2 and GM2 were observed in the plasma of BD patients. Disrupted GM2/GD2 synthase exhibited neurodegeneration in the nervous system (57), and GM2 elevation was associated with glial activation in the injured developing brain (58). MGDGs show strong anti-inflammatory properties (59, 60). Taken together, the composition of plasma lipids was altered in women with BD. Consistent with the pathophysiology of BD, most of these lipid classes are related to oxidative stress, inflammatory responses, neuroprotection, and mitochondrial dysfunction (61–63).
In addition to observing changes in lipid class levels, we also compared the carbon chain lengths and unsaturated bonds of fatty acids in different classes between the two groups. The carbon chain length and degree of saturation of fatty acids influence the biophysical properties of lipids; longer and more fully saturated chains are correlated with more extensive stabilizing lipid–protein and lipid–lipid interactions and a higher free energy barrier for protein dissociation and lipoprotein fusion (64)—for instance, the modulation of the composition of fatty acyl chains and degree of saturation of membrane lipids can potentially affect neuronal functioning, at least in part, via the altered function of membrane-bound proteins (65, 66). The present study found that long-chain and polyunsaturated fatty acids were mainly decreased in BD. Moreover, we compared the concentrations of different lipid classes with different carbon chains and unsaturated fatty acids in the plasma of BD vs. HC. Although the potential mechanism for these differences is not clear, this result sheds a new light on existing data on the differences in lipid class structure and function in women with BD.
In addition to analyzing the composition of lipid classes that are characterized in BD, we further investigated the changes in lipids at the species level and identified which lipid biomarkers could discriminate BD for further development as a potential diagnostic tool. We observed 55 lipid species with significant differences and identified a signature of 9 lipids that could distinguish patients with BD from HCs (AUC = 0.994). We believe that this method has the potential to be used as a different diagnostic tool for distinguishing BD from HC on the basis of the subjects' plasma lipid composition and could fill the clinical need for better quantitative diagnostic tools to optimize the initial treatment approach. However, previous studies on BD and lipids have identified several lipids as potential BD biomarkers—for example, a preliminary study (15 BD patients and 21 healthy subjects) on BD and serum lipids found that PI(40:3), PG(32:4)-OH, and TG(42:3) showed high reliability (AUC > 0.9) in distinguishing BD type I from HCs, whereas PE(42:5), PA(48:8)-OH, and PA(44:4) showed moderate reliability (AUC > 0.8) in distinguishing BD type I from HCs (32). Another large-sample study (67 patients with unipolar disorder or BDs and 405 healthy subjects) used targeted lipidomics, such as C24:1Cer, C24:1GluCer, and C24LacCer, and found that the differences between MDD and BD patients versus controls mainly originated from Cer and their hexosyl metabolites (48). However, we did not measure either of these lipids in our untargeted lipidomics analysis. This discrepancy might be partly due to the different methods for evaluating lipidomics (i.e., different types of lipids were quantified) and the different age/sex distributions. However, the results of lipid composition at the class and species levels were not completely consistent—for example, the TG levels increased in BD with no significant correlation with clinical symptoms, whereas the levels of TG(16:0/16:1/22:6)+NH4, TG(16:0/20:4/22:5)+NH4, TG(22:4/17:1/18:2)+NH4, and TG(18:0/8:0/20:4)+Na were changed in BD, had a significant correlation with the severity of clinical symptoms, and exhibited high reliability. Other species including TG(18:1/18:2/22:4)+NH4, TG(18:4/20:4/20:5)+H, TG(12:0/18:2/18:2)+NH4, TG(16:0/14:1/20:5)+NH4, TG(16:0/20:4/20:5)+NH4, TG(20:0/18:1/18:1)+NH4, and TG(15:0/16:0/18:1)+NH4 also showed moderate reliability for BD diagnosis. Therefore, future studies using consistent methods for a more comprehensive coverage of lipid metabolites with larger sample sizes would be instrumental for clarifying and confirming specific lipid species that may be altered in BD.
Furthermore, although the dysfunction of lipid metabolism in peripheral blood may contribute to the etiopathology of BD (67) and given that the use of nutritional and other treatments aimed at modulating the function and regulation of lipids, such as phospholipids and sphingolipids, represents a means to improve or prevent BD in individuals suffering from mood disorders (68–70), the lipid changes in the brain tissue and plasma of patients with BD are not entirely consistent, and the crosstalk between peripheral and brain lipid homeostasis is still unclear—for example, we found that PE and PS decreased in the plasma of BD patients; however, a previous study showed that there was no significant difference in total PS, PE, and PC in the postmortem hippocampus of patients with BD and the HCs (71). An analysis of brain lipids and associated enzymes in the cerebrospinal fluid might be used to investigate the central characteristics of lipid status and provide further insight into the relationship between lipid homeostasis and the clinical symptoms of BD (37). Moreover, female patients with unipolar depression were excluded from the current study. Whether the potential lipids could distinguish bipolar and unipolar depression remains to be further investigated, and the potential molecular mechanism involved in the pathophysiology of female BD also remains to be determined. Although lipid levels were quantitatively analyzed in this study, the internal mix standards only contained 14 lipids. Considering that the content of the substance was calculated using the response abundance ratio (peak area ratio) between the substance and the internal standard, it does not really reflect the absolute content of all substances and cannot be compared with the clinical reference range of the lipid content of blood samples.
In summary, our study revealed distinct changes in the lipid composition of female BD patients, identified 55 lipids with significant differences, investigated their correlation with clinical parameters, and screened 9 species as complementary biomarkers for the diagnosis of BD. These data provide a further understanding of abnormal lipid metabolism and suggest that specific lipid species can be used to distinguish female patients with BD from normal controls. However, the number of recruited participants in this study was relatively small, and the correlation coefficient between the clinical scale score and the lipid content was low. Although it was consistent with the results of previous studies (42, 51), it suggested that the strength of the relationship was insufficient and that there might be a shortage of lipid markers for the prediction of symptom severity. Furthermore, this study was a cross-sectional study, and the plasma samples were collected only at baseline, thus making it difficult to establish a causal association between changes in lipid composition and disease remission. A discovery and validation cohort study with a larger sample size should be conducted in the future to verify the potential use of these lipid markers.
Data Availability Statement
The original contributions presented in the study are included in the article/Supplementary Materials, further inquiries can be directed to the corresponding authors.
Ethics Statement
The study protocol was approved by Chinese Registered Clinical Trial Ethics Committee (Approval Number: ChiECRCT20200090) and was registered with the Chinese Clinical Trial Registry (Registration number ChiCTR2000032118). The patients/participants provided their written informed consent to participate in this study.
Author Contributions
LG, TZ, RL, JD, and J-bY designed the data collection tool and were responsible for data collection and clinical evaluation. Z-qC was responsible for data management. Z-wP and Q-rT financed and designed the study and supervised the data collection and analysis. Y-HC, FX, and RL analyzed the data. LG and TZ wrote the first draft with Z-wP and Q-rT. All other authors provided the data, reviewed the results, and contributed to the final draft of the report.
Funding
This study was funded by the National Natural Science Foundation of China (Grant Nos. 81630032, 82171512, and 82101594).
Conflict of Interest
The authors declare that the research was conducted in the absence of any commercial or financial relationships that could be construed as a potential conflict of interest.
Publisher's Note
All claims expressed in this article are solely those of the authors and do not necessarily represent those of their affiliated organizations, or those of the publisher, the editors and the reviewers. Any product that may be evaluated in this article, or claim that may be made by its manufacturer, is not guaranteed or endorsed by the publisher.
Acknowledgments
We would like to thank Gene Denovo Co., Ltd. for their technical assistance.
Supplementary Material
The Supplementary Material for this article can be found online at: https://www.frontiersin.org/articles/10.3389/fpsyt.2022.802710/full#supplementary-material
Supplementary Figure 1. Comparison of the concentrations of lipids with different carbon chain lengths from the fatty acyls of the BD and HC groups. Lipids showing low-level results have been enlarged in the insets. (A) AcCa; (B) WE; (C) MGDG; (D) TG; (E) CerP; (F) SM; (G) Cer; (H) CerG2GNAc1; (I) PI, PIP and PIP2; (J) PS; (K) PE; (L) LPE and LPI; and (M) MG. *P < 0.05; **P < 0.01.
Supplementary Figure 2. Comparison of the concentrations of lipids with different degrees of saturation from the fatty acyls of the BD and HC groups. Lipids showing low-level results have been enlarged in the insets. (A) AcCa and WE; (B) SM, CerG2GNAc1, Cer and CerP; (C) PI, PS and PE; (D) MGDG; (E) LPC, LPE, LPI, PIP and PIP2; and (F) TG and MG. *P < 0.05; **P < 0.01.
Supplementary Table 1. Clinical information of enrolled individuals.
Supplementary Table 2. Original data of lipidomics.
Supplementary Table 3. Correlation of clinical parameters and the concentration of lipid class (spearman correlation).
Supplementary Table 4. Correlation of clinical parameters and the concentration of altered lipids at species level (spearman correlation).
Supplementary Table 5. ROC analysis for altered lipids at species level.
Abbreviations
BD, bipolar disorder; LC, liquid chromatography; MS, Mass Spectrometry; DSM-5, fifth edition of the Diagnostic and Statistical Manual of Mental Disorders; HAMD, Hamilton Depression Rating Scale; HAMA, Hamilton Anxiety Scale; BMI, Body Mass Index; PANSS, Positive and Negative Syndrome Scale; BRMS, Bech–Rafaelsen Mania Rating Scale; ROC, Receiver Operating Characteristic; AUC, area under the ROC curve; HC, Healthy Controls; WE, Wax Ester; AcCa, acylcarnitine; DG, diglyceride; TG, triglyceride; MG, monoglyceride; SM, sphingomyelin; Cer, ceramides; CerP, ceramide phosphate; ChE, cholesterol ester; Co, coenzyme; MGDG, monogalactosyldiacylglycerol; DGDG, digalactosyldiacylglycerol; PC, phosphatidylcholine; PI, phosphatidylinositol; PS, phosphatidylserine; PE, phosphatidylethanolamine; PG, phosphatidylglycerol; CL, cardiolipin; LPC, lysophosphatidylcholine; LPE, lysophosphatidylethanolamine; LPI, lysophosphatidylinositol; PA, phosphatidic acid; PIP, phosphatidylinositol phosphate; PIP2, phosphatidylinositol 4, 5-bisphosphate.
References
1. McIntyre RS, Berk M, Brietzke E, Goldstein BI, Lopez-Jaramillo C, Kessing LV, et al. Bipolar disorders. Lancet. (2020) 396:1841–56. doi: 10.1016/S0140-6736(20)31544-0
2. Merikangas KR, Jin R, He JP, Kessler RC, Lee S, Sampson NA, et al. Prevalence and correlates of bipolar spectrum disorder in the world mental health survey initiative. Arch Gen Psychiatry. (2011) 68:241–51. doi: 10.1001/archgenpsychiatry.2011.12
3. Grande I, Berk M, Birmaher B, Vieta E. Bipolar disorder. Lancet. (2016) 387:1561–72. doi: 10.1016/S0140-6736(15)00241-X
4. Merikangas KR, Akiskal HS, Angst J, Greenberg PE, Hirschfeld RM, Petukhova M, et al. Lifetime and 12-month prevalence of bipolar spectrum disorder in the national comorbidity survey replication. Arch Gen Psychiatry. (2007) 64:543–52. doi: 10.1001/archpsyc.64.5.543
5. Jadhav S, Russo S, Cottier S, Schneiter R, Cowart A, Greenberg ML. Valproate induces the unfolded protein response by increasing ceramide levels. J Biol Chem. (2016) 291:22253–61. doi: 10.1074/jbc.M116.752634
6. Henry CA, Zamvil LS, Lam C, Rosenquist KJ, Ghaemi SN. Long-term outcome with divalproex in children and adolescents with bipolar disorder. J Child Adolesc Psychopharmacol. (2003) 13:523–9. doi: 10.1089/104454603322724913
7. Ferrari AJ, Stockings E, Khoo JP, Erskine HE, Degenhardt L, Vos T, et al. The prevalence and burden of bipolar disorder: findings from the global burden of disease study 2013. Bipolar Disord. (2016) 18:440–50. doi: 10.1111/bdi.12423
8. Teneralli RE, Kern DM, Cepeda MS, Gilbert JP, Drevets WC. Exploring real-world evidence to uncover unknown drug benefits and support the discovery of new treatment targets for depressive and bipolar disorders. J Affect Disord. (2021) 290:324–33. doi: 10.1016/j.jad.2021.04.096
9. Salvatore P, Baldessarini RJ, Tohen M, Khalsa HM, Sanchez-Toledo JP, Zarate CA, et al. McLean-harvard international first-episode project: two-year stability of DSM-IV diagnoses in 500 first-episode psychotic disorder patients. J Clin Psychiatry. (2009) 70:458–66. doi: 10.4088/JCP.08m04227
10. Saraf G, Moazen-Zadeh E, Pinto JV, Ziafat K, Torres IJ, Kesavan M, et al. Early intervention for people at high risk of developing bipolar disorder: a systematic review of clinical trials. Lancet Psychiatry. (2021) 8:64–75. doi: 10.1016/S2215-0366(20)30188-7
11. Hashimoto K. Metabolomics of major depressive disorder and bipolar disorder: overview and future perspective. Adv Clin Chem. (2018) 84:81–99. doi: 10.1016/bs.acc.2017.12.005
12. Hashimoto K. Brain-derived neurotrophic factor (BDNF) and its precursor proBDNF as diagnostic biomarkers for major depressive disorder and bipolar disorder. Eur Arch Psychiatry Clin Neurosci. (2015) 265:83–4. doi: 10.1007/s00406-014-0557-x
13. Passos IC, Mwangi B, Vieta E, Berk M, Kapczinski F. Areas of controversy in neuroprogression in bipolar disorder. Acta Psychiatr Scand. (2016) 134:91–103. doi: 10.1111/acps.12581
14. Kuehner C. Why is depression more common among women than among men? Lancet Psychiatry. (2017) 4:146–58. doi: 10.1016/S2215-0366(16)30263-2
15. Sassarini DJ. Depression in midlife women. Maturitas. (2016) 94:149–54. doi: 10.1016/j.maturitas.2016.09.004
16. Clark CT, Wisner KL. Treatment of peripartum bipolar disorder. Obstet Gynecol Clin North Am. (2018) 45:403–17. doi: 10.1016/j.ogc.2018.05.002
17. Huang Y, Wang Y, Wang H, Liu Z, Yu X, Yan J, et al. Prevalence of mental disorders in China: a cross-sectional epidemiological study. Lancet Psychiatry. (2019) 6:211–24. doi: 10.1016/S2215-0366(18)30511-X
18. Di Florio A, Forty L, Gordon-Smith K, Heron J, Jones L, Craddock N, et al. Perinatal episodes across the mood disorder spectrum. JAMA Psychiatry. (2013) 70:168–75. doi: 10.1001/jamapsychiatry.2013.279
19. Wesseloo R, Kamperman AM, Munk-Olsen T, Pop VJ, Kushner SA, Bergink V. Risk of postpartum relapse in bipolar disorder and postpartum psychosis: a systematic review and meta-analysis. Am J Psychiatry. (2016) 173:117–27. doi: 10.1176/appi.ajp.2015.15010124
20. Hashimoto M, Katakura M, Tanabe Y, Al Mamun A, Inoue T, Hossain S, et al. n-3 fatty acids effectively improve the reference memory-related learning ability associated with increased brain docosahexaenoic acid-derived docosanoids in aged rats. Biochim Biophys Acta. (2015) 1851:203–9. doi: 10.1016/j.bbalip.2014.10.009
21. McDougall M, Choi J, Magnusson K, Truong L, Tanguay R, Traber MG. Chronic vitamin E deficiency impairs cognitive function in adult zebrafish via dysregulation of brain lipids and energy metabolism. Free Radic Biol Med. (2017) 112:308–17. doi: 10.1016/j.freeradbiomed.2017.08.002
22. Hussain G, Wang J, Rasul A, Anwar H, Imran A, Qasim M, et al. Role of cholesterol and sphingolipids in brain development and neurological diseases. Lipids Health Dis. (2019) 18:26. doi: 10.1186/s12944-019-0965-z
23. Araque A, Castillo PE, Manzoni OJ, Tonini R. Synaptic functions of endocannabinoid signaling in health and disease. Neuropharmacology. (2017) 124:13–24. doi: 10.1016/j.neuropharm.2017.06.017
24. Egawa J, Pearn ML, Lemkuil BP, Patel PM, Head BP. Membrane lipid rafts and neurobiology: age-related changes in membrane lipids and loss of neuronal function. J Physiol. (2016) 594:4565–79. doi: 10.1113/JP270590
25. Wu L, Zhang X, Zhao L. Human ApoE isoforms differentially modulate brain glucose and ketone body metabolism: implications for Alzheimer's disease risk reduction and early intervention. J Neurosci. (2018) 38:6665–81. doi: 10.1523/JNEUROSCI.2262-17.2018
26. Reichel M, Honig S, Liebisch G, Luth A, Kleuser B, Gulbins E, et al. Alterations of plasma glycerophospholipid and sphingolipid species in male alcohol-dependent patients. Biochim Biophys Acta. (2015) 1851:1501–10. doi: 10.1016/j.bbalip.2015.08.005
27. Zhou C, Cai M, Wang Y, Wu W, Yin Y, Wang X, et al. The effects of repetitive transcranial magnetic stimulation on cognitive impairment and the brain lipidome in a cuprizone-induced mouse model of demyelination. Front Neurosci. (2021) 15:706786. doi: 10.3389/fnins.2021.706786
28. Sethi S, Hayashi MA, Sussulini A, Tasic L, Brietzke E. Analytical approaches for lipidomics and its potential applications in neuropsychiatric disorders. World J Biol Psychiatry. (2017) 18:506–20. doi: 10.3109/15622975.2015.1117656
29. Scola G, Versace A, Metherel AH, Monsalve-Castro LA, Phillips ML, Bazinet RP, et al. Alterations in peripheral fatty acid composition in bipolar and unipolar depression. J Affect Disord. (2018) 233:86–91. doi: 10.1016/j.jad.2017.12.025
30. D'Ambrosio V, Salvi V, Bogetto F, Maina G. Serum lipids, metabolic syndrome and lifetime suicide attempts in patients with bipolar disorder. Prog Neuropsychopharmacol Biol Psychiatry. (2012) 37:136–40. doi: 10.1016/j.pnpbp.2011.12.009
31. Knowles EE, Meikle PJ, Huynh K, Goring HH, Olvera RL, Mathias SR, et al. Serum phosphatidylinositol as a biomarker for bipolar disorder liability. Bipolar Disord. (2017) 19:107–15. doi: 10.1111/bdi.12468
32. Ribeiro HC, Klassen A, Pedrini M, Carvalho MS, Rizzo LB, Noto MN, et al. A preliminary study of bipolar disorder type I by mass spectrometry-based serum lipidomics. Psychiatry Res. (2017) 258:268–73. doi: 10.1016/j.psychres.2017.08.039
33. Fu Y, Chen N, Wang Z, Luo S, Ding Y, Lu B. Degradation of lipid droplets by chimeric autophagy-tethering compounds. Cell Res. (2021). doi: 10.1038/s41422-021-00532-7
34. Sun Y, Song K, Liu L, Sun L, Qin Q, Jiang T, et al. Silencing of sulfoquinovosyl diacylglycerol synthase 1 impairs the glycolipids accumulation and photosynthesis in phosphate-deprived rice. J Exp Bot. (2021) 72:6510–23. doi: 10.1093/jxb/erab300
35. Cajka T, Smilowitz JT, Fiehn O. Validating quantitative untargeted lipidomics across nine liquid chromatography-high-resolution mass spectrometry platforms. Anal Chem. (2017) 89:12360–8. doi: 10.1021/acs.analchem.7b03404
36. Fadok VA, Bratton DL, Rose DM, Pearson A, Ezekewitz RA, Henson PM, et al. receptor for phosphatidylserine-specific clearance of apoptotic cells. Nature. (2000) 405:85–90. doi: 10.1038/35011084
37. Saito K, Hattori K, Hidese S, Sasayama D, Miyakawa T, Matsumura R, et al. Profiling of cerebrospinal fluid lipids and their relationship with plasma lipids in healthy humans. Metabolites. (2021) 11:268. doi: 10.3390/metabo11050268
38. Dong MX, Wei YD, Hu L. The disturbance of lipid metabolism is correlated with neuropsychiatric symptoms in patients with Parkinson's disease. Chem Phys Lipids. (2021) 239:105112. doi: 10.1016/j.chemphyslip.2021.105112
39. Desikan RS, Schork AJ, Wang Y, Thompson WK, Dehghan A, Ridker PM, et al. Polygenic overlap between C-Reactive protein, plasma lipids, and Alzheimer disease. Circulation. (2015) 131:2061–9. doi: 10.1161/CIRCULATIONAHA.115.015489
40. Liu Y, Song X, Liu X, Pu J, Gui S, Xu S, et al. Alteration of lipids and amino acids in plasma distinguish schizophrenia patients from controls: a targeted metabolomics study. Psychiatry Clin Neurosci. (2021) 75:138–44. doi: 10.1111/pcn.13194
41. Enko D, Brandmayr W, Halwachs-Baumann G, Schnedl WJ, Meinitzer A, Kriegshauser G. Prospective plasma lipid profiling in individuals with and without depression. Lipids Health Dis. (2018) 17:149. doi: 10.1186/s12944-018-0796-3
42. Demirkan A, Isaacs A, Ugocsai P, Liebisch G, Struchalin M, Rudan I, et al. Plasma phosphatidylcholine and sphingomyelin concentrations are associated with depression and anxiety symptoms in a Dutch family-based lipidomics study. J Psychiatr Res. (2013) 47:357–62. doi: 10.1016/j.jpsychires.2012.11.001
43. Maldonado C, Guevara N, Queijo C, Gonzalez R, Fagiolino P, Vazquez M. Carnitine and/or acetylcarnitine deficiency as a cause of higher levels of ammonia. Biomed Res Int. (2016) 2016:2920108. doi: 10.1155/2016/2920108
44. Ogawa S, Hattori K, Ota M, Hidese S, Miyakawa T, Matsumura R, et al. Altered ethanolamine plasmalogen and phosphatidylethanolamine levels in blood plasma of patients with bipolar disorder. Psychiatry Clin Neurosci. (2020) 74:204–10. doi: 10.1111/pcn.12967
45. Yokoyama S, Yasui-Furukori N, Nakagami T, Miyazaki K, Ishioka M, Tarakita N, et al. Association between the serum carnitine level and ammonia and valproic acid levels in patients with bipolar disorder. Ther Drug Monit. (2020) 42:766–70. doi: 10.1097/FTD.0000000000000778
46. Vance JE, Steenbergen R. Metabolism and functions of phosphatidylserine. Prog Lipid Res. (2005) 44:207–34. doi: 10.1016/j.plipres.2005.05.001
47. Mehrpooya M, Yasrebifar F, Haghighi M, Mohammadi Y, Jahangard L. Evaluating the effect of coenzyme Q10 augmentation on treatment of bipolar depression: a double-blind controlled clinical trial. J Clin Psychopharmacol. (2018) 38:460–6. doi: 10.1097/JCP.0000000000000938
48. Brunkhorst-Kanaan N, Klatt-Schreiner K, Hackel J, Schroter K, Trautmann S, Hahnefeld L, et al. Targeted lipidomics reveal derangement of ceramides in major depression and bipolar disorder. Metabolism. (2019) 95:65–76. doi: 10.1016/j.metabol.2019.04.002
49. Soares JC, Mallinger AG, Dippold CS, Forster Wells K, Frank E, Kupfer DJ. Effects of lithium on platelet membrane phosphoinositides in bipolar disorder patients: a pilot study. Psychopharmacology. (2000) 149:12–6. doi: 10.1007/s002139900341
50. Soares JC, Dippold CS, Wells KF, Frank E, Kupfer DJ, Mallinger AG. Increased platelet membrane phosphatidylinositol-4,5-bisphosphate in drug-free depressed bipolar patients. Neurosci Lett. (2001) 299:150–2. doi: 10.1016/S0304-3940(00)01775-4
51. Liu X, Li J, Zheng P, Zhao X, Zhou C, Hu C, et al. Plasma lipidomics reveals potential lipid markers of major depressive disorder. Anal Bioanal Chem. (2016) 408:6497–507. doi: 10.1007/s00216-016-9768-5
52. Jones LL, McDonald DA, Borum PR. Acylcarnitines: role in brain. Prog Lipid Res. (2010) 49:61–75. doi: 10.1016/j.plipres.2009.08.004
53. Bjorndal B, Alteras EK, Lindquist C, Svardal A, Skorve J, Berge RK. Associations between fatty acid oxidation, hepatic mitochondrial function, and plasma acylcarnitine levels in mice. Nutr Metab. (2018) 15:10. doi: 10.1186/s12986-018-0241-7
54. Zanelli SA, Solenski NJ, Rosenthal RE, Fiskum G. Mechanisms of ischemic neuroprotection by acetyl-L-carnitine. Ann N Y Acad Sci. (2005) 1053:153–61. doi: 10.1196/annals.1344.013
55. Yamamoto Y, Sakurai T, Chen Z, Furukawa T, Gowda SGB, Wu Y, et al. Analysis of serum lysophosphatidylethanolamine levels in patients with non-alcoholic fatty liver disease by liquid chromatography-tandem mass spectrometry. Anal Bioanal Chem. (2021) 413:245–54. doi: 10.1007/s00216-020-02996-9
56. Ren C, Liu J, Zhou J, Liang H, Wang Y, Sun Y, et al. Lipidomic analysis of serum samples from migraine patients. Lipids Health Dis. (2018) 17:22. doi: 10.1186/s12944-018-0665-0
57. Sheikh KA, Sun J, Liu Y, Kawai H, Crawford TO, Proia RL, et al. Mice lacking complex gangliosides develop Wallerian degeneration and myelination defects. Proc Natl Acad Sci U S A. (1999) 96:7532–7. doi: 10.1073/pnas.96.13.7532
58. Saito M, Wu G, Hui M, Masiello K, Dobrenis K, Ledeen RW, et al. Ganglioside accumulation in activated glia in the developing brain: comparison between WT and GalNAcT KO mice. J Lipid Res. (2015) 56:1434–48. doi: 10.1194/jlr.M056580
59. Lenti M, Gentili C, Pianezzi A, Marcolongo G, Lalli A, Cancedda R, et al. Monogalactosyldiacylglycerol anti-inflammatory activity on adult articular cartilage. Nat Prod Res. (2009) 23:754–62. doi: 10.1080/14786410802456956
60. Ulivi V, Lenti M, Gentili C, Marcolongo G, Cancedda R, Descalzi Cancedda F. Anti-inflammatory activity of monogalactosyldiacylglycerol in human articular cartilage in vitro: activation of an anti-inflammatory cyclooxygenase-2 (COX-2) pathway. Arthritis Res Ther. (2011) 13:R92. doi: 10.1186/ar3367
61. Hornig M, Briese T, Licinio J, Khabbaz RF, Altshuler LL, Potkin SG, et al. Absence of evidence for bornavirus infection in schizophrenia, bipolar disorder and major depressive disorder. Mol Psychiatry. (2012) 17:486–93. doi: 10.1038/mp.2011.179
62. Morris G, Stubbs B, Kohler CA, Walder K, Slyepchenko A, Berk M, et al. The putative role of oxidative stress and inflammation in the pathophysiology of sleep dysfunction across neuropsychiatric disorders: focus on chronic fatigue syndrome, bipolar disorder and multiple sclerosis. Sleep Med Rev. (2018) 41:255–65. doi: 10.1016/j.smrv.2018.03.007
63. Steckert AV, Valvassori SS, Moretti M, Dal-Pizzol F, Quevedo J. Role of oxidative stress in the pathophysiology of bipolar disorder. Neurochem Res. (2010) 35:1295–301. doi: 10.1007/s11064-010-0195-2
64. Guha M, Gantz DL, Gursky O. Effects of acyl chain length, unsaturation, and pH on thermal stability of model discoidal HDLs. J Lipid Res. (2008) 49:1752–61. doi: 10.1194/jlr.M800106-JLR200
65. Carta M, Lanore F, Rebola N, Szabo Z, Da Silva SV, Lourenco J, et al. Membrane lipids tune synaptic transmission by direct modulation of presynaptic potassium channels. Neuron. (2014) 81:787–99. doi: 10.1016/j.neuron.2013.12.028
66. Oliveira TG, Chan RB, Bravo FV, Miranda A, Silva RR, Zhou B, et al. The impact of chronic stress on the rat brain lipidome. Mol Psychiatry. (2016) 21:80–8. doi: 10.1038/mp.2015.14
67. Dolab N, Kamkar MZ, Amiriani T, Yuzugulen J, Marjani M, Marjani A. The association between leptin and adiponectin, and metabolic syndrome components and serum levels of lipid peroxidation in bipolar disorder patients treated with lithium and valproic acid. Heliyon. (2020) 6:e04553. doi: 10.1016/j.heliyon.2020.e04553
68. Schneider M, Levant B, Reichel M, Gulbins E, Kornhuber J, Muller CP. Lipids in psychiatric disorders and preventive medicine. Neurosci Biobehav Rev. (2017) 76:336–62. doi: 10.1016/j.neubiorev.2016.06.002
69. Liao Y, Xie B, Zhang H, He Q, Guo L, Subramanieapillai M, et al. Efficacy of omega-3 PUFAs in depression: a meta-analysis. Transl Psychiatry. (2019) 9:190. doi: 10.1038/s41398-019-0515-5
70. Robinson DG, Gallego JA, John M, Hanna LA, Zhang JP, Birnbaum ML, et al. A potential role for adjunctive omega-3 polyunsaturated fatty acids for depression and anxiety symptoms in recent onset psychosis: results from a 16week randomized placebo-controlled trial for participants concurrently treated with risperidone. Schizophr Res. (2019) 204:295–303. doi: 10.1016/j.schres.2018.09.006
Keywords: lipidomics, women, bipolar disorder, healthy controls, plasma
Citation: Guo L, Zhang T, Li R, Cui Z-q, Du J, Yang J-b, Xue F, Chen Y-H, Tan Q-r and Peng Z-w (2022) Alterations in the Plasma Lipidome of Adult Women With Bipolar Disorder: A Mass Spectrometry-Based Lipidomics Research. Front. Psychiatry 13:802710. doi: 10.3389/fpsyt.2022.802710
Received: 27 October 2021; Accepted: 07 February 2022;
Published: 21 March 2022.
Edited by:
Gilberto Pérez Sánchez, Instituto Nacional de Psiquiatría Ramón de la Fuente Muñiz, MexicoReviewed by:
Haiyun Xu, Wenzhou Medical University, ChinaAdolfo Lopez-Torres, Universidad del Papaloapan, Mexico
Copyright © 2022 Guo, Zhang, Li, Cui, Du, Yang, Xue, Chen, Tan and Peng. This is an open-access article distributed under the terms of the Creative Commons Attribution License (CC BY). The use, distribution or reproduction in other forums is permitted, provided the original author(s) and the copyright owner(s) are credited and that the original publication in this journal is cited, in accordance with accepted academic practice. No use, distribution or reproduction is permitted which does not comply with these terms.
*Correspondence: Zheng-wu Peng, pengzw@fmmu.edu.cn; Qing-rong Tan, tanqingr@fmmu.edu.cn
†These authors have contributed equally to this work