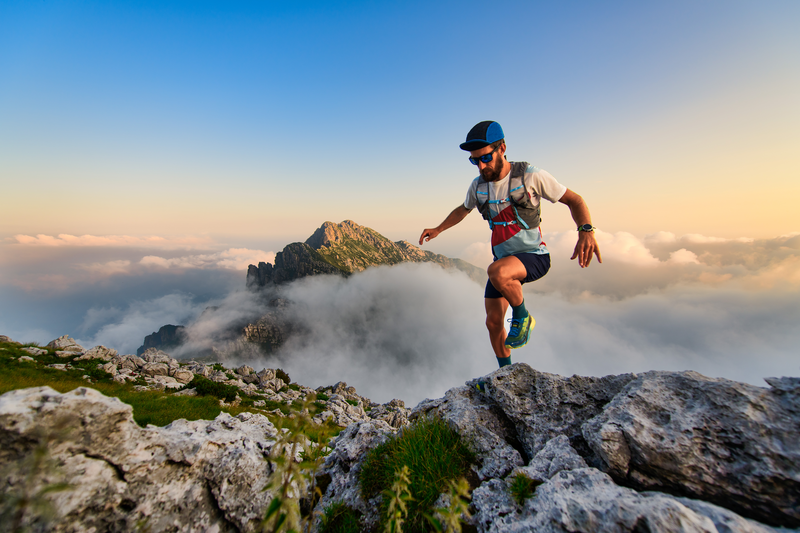
95% of researchers rate our articles as excellent or good
Learn more about the work of our research integrity team to safeguard the quality of each article we publish.
Find out more
MINI REVIEW article
Front. Psychiatry , 10 February 2023
Sec. Psychopharmacology
Volume 13 - 2022 | https://doi.org/10.3389/fpsyt.2022.1076459
This article is part of the Research Topic Alternative Treatments to Classical Antidepressants in Treatment-Resistant Depression View all 6 articles
Introduction: Current treatment options for major depressive disorder (MDD) have limited efficacy and are associated with adverse effects. Recent studies investigating the antidepressant effect of serotonergic psychedelics—also known as classic psychedelics—have promising preliminary results with large effect sizes. In this context, we conducted a review of the putative neurobiological underpinnings of the mechanism of antidepressant action of these drugs.
Methods: A narrative review was conducted using PubMed to identify published articles evaluating the antidepressant mechanism of action of serotonergic psychedelics.
Results: Serotonergic psychedelics have serotonin (5HT)2A agonist or partial agonist effects. Their rapid antidepressant effects may be mediated—in part—by their potent 5HT2A agonism, leading to rapid receptor downregulation. In addition, these psychedelics impact brain derived neurotrophic factor and immunomodulatory responses, both of which may play a role in their antidepressant effect. Several neuroimaging and neurophysiology studies evaluating mechanistic change from a network perspective can help us to further understand their mechanism of action. Some, but not all, data suggest that psychedelics may exert their effects, in part, by disrupting the activity of the default mode network, which is involved in both introspection and self-referential thinking and is over-active in MDD.
Conclusion: The mechanisms of action underlying the antidepressant effect of serotonergic psychedelics remains an active area of research. Several competing theories are being evaluated and more research is needed to determine which ones are supported by the most robust evidence.
Major depressive disorder (MDD) is a chronic and debilitating mood disorder impacting the lives of approximately 300 million people worldwide (1). First-line pharmacotherapy options include selective serotonin reuptake inhibitors (SSRIs) and serotonin and norepinephrine reuptake inhibitors (SNRIs) (2). Other antidepressants, adjunctive pharmacotherapies, psychotherapies, and brain stimulation can be used in patients who do not respond to first- or second-line treatment (2). However, 30–50% of patients do not respond to available treatments (3). Moreover, several weeks are required to determine the efficacy of pharmacotherapies and they are associated with adverse effects (e.g., weight gain, sexual dysfunction) (4, 5). Given the high prevalence of MDD, novel treatments with rapid onset are needed.
Serotonergic (“classic”) psychedelics are a broad category of drugs that includes psilocybin, dimethyltryptamine (DMT, the psychoactive ingredient of ayahuasca), and lysergic acid diethylamide (LSD). These psychedelics have been used as therapeutic agents for thousands of years in various cultures (6). Clinical research into psychedelic treatments began in the 1950s; about 40,000 individuals had been studied by the late 1960s when concerns about their safety and their recreational use led to their classification as Schedule 1 narcotics in the US (7). This precluded human studies until the late 1990s when a “second wave” of modern clinical trials restarted investigating the efficacy and safety of classic psychedelics for major depressive disorder (MDD) and other mental disorders.
Emerging evidence suggests serotonergic psychedelics have antidepressant effects (8–12). Most studies have been conducted using psilocybin for MDD, treatment-resistant depression (TRD), or end-of-life distress (11–15). Psilocybin is primarily administered in conjunction with a form of non-directive and supportive psychotherapy (11–13). Adverse effects are transient and mild, including nausea, anxiety, and minor blood pressure elevations generally resolving within the 8-h dosing session (11–13).
Despite preliminary evidence suggesting serotonergic psychedelics have rapid antidepressant effects, their mechanism of action is not well-understood. This narrative review provides an overview of their putative antidepressant mechanisms of action.
Serotonin influences brain circuits responsible for regulation of mood, reactivity to stress, and cognitive performance. For more than 60 years, alterations of monoamine neurotransmitters, including serotonin, have been hypothesized to underlie depressive symptoms (16). This is indirectly supported by the fact that most traditional antidepressants impact monoamines; for example, SSRIs acutely increase the availability of serotonin in synapses (16). Additionally, depletion of tryptophan an amino acid required for 5-HT synthesis, induces depressive symptoms in previously treated patients (17, 18).
There is robust evidence supporting that the psychoactive effect of serotonergic psychedelics is mediated by their agonism of the 5-HT2A serotonin receptor (5-HT2AR). This effect can be blocked by administering 5-HT2AR antagonists, such as ketanserin or risperidone (19). 5-HT2AR agonism increases serotonin release, impacting excitability of pyramidal neurons in the cortex and inducing glutamate release in the neocortex (20). In MDD, an inverse correlation has been observed between depressive symptoms and the degree of 5-HT2AR stimulation (21). Similarly, psychedelics may exert their effects through their potent 5-HT2AR agonism leading to rapid down regulation of these receptors (22). 5-HT2ARs also play a role in cognitive inflexibility and rumination, core symptoms of depression (22). While it has been proposed that the hallucinogenic experience and associated “mystical effects” produced by 5-HT2AR agonism are responsible for the antidepressant effect (23), there is little evidence to support this. In fact, in pre-clinical models of depression, psilocybin reverses anhedonia even when mice are pre-treated with the 5-HT2AR antagonist ketanserin (24). Other receptors may be involved in psychedelics’ antidepressant effect. LSD is a partial agonist of 5-HT2AR (25, 26) and it exerts its effects mostly through agonism of 5-HT1AR (27, 28). Both LSD and SSRIs impact 5-HT1AR by desensitizing post-synaptic 5-HT1AR resulting in increased serotonin release (28). More research is needed to determine the role of specific 5-HT receptors, with each psychedelic drug requiring discrete investigations.
Psychedelics’ 5-HT2AR agonism indirectly activates glutamate networks that impact prefrontal areas and downstream increases in brain derived neurotropic factor (BDNF), which supports growth and maintenance of neurons and enhances neuroplasticity. Decreased BDNF levels have been observed in MDD and correlate with suicidal behavior (29, 30). Antidepressants (31), sleep, and physical exercise increase BDNF (32, 33). Higher baseline BDNF levels correlate with higher SSRI-associated improvement in depressive symptoms (34). In rats repeated administration of ayahuasca increases hippocampal BDNF levels (35). Similarly, healthy humans and patients with TRD show increased circulating BDNF levels after a single dose of ayahuasca (36), with serum BDNF levels negatively correlated with depressive symptoms (36). Psilocybin also increases peak BDNF levels, regardless of whether patients are pre-treated with the antidepressant, escitalopram or placebo, suggesting that psilocybin has a strong impact on BDNF (37). A study using varying doses of LSD showed acute increases in BDNF only for certain doses (38), suggesting further investigation is needed. Increases in BDNF levels also play a role in neurogenesis. Depressive symptoms have been linked to insufficient neurogenesis and neurotrophic activity (39). SSRIs increase neurogenesis (40) and their behavioral effects can be blocked by its disruption (41). 5-methoxy(MeO)-DMT (42) and psilocybin (43) increase neurogenesis in rats and the study involving psilocybin suggested this might be dose-dependent (43). More research is needed to elucidate the connections among depression, neurogenesis, and psychedelics.
Elevated inflammatory markers signal the body is responding to stressful stimuli and attempting to return to homeostasis (28). Inflammation symptoms are similar to depressive symptoms, including fatigue, low motivation, and irritability (44). Evidence has shown that a subset of patients with MDD have increased inflammatory markers such as Interleukin 6 (IL-6) and 1β, C-reactive protein (CRP), and Tumor Necrosis Factor-alpha (TNF-α) (45, 46). Inflammatory cytokines also increase metabolism of tryptophan which may contribute to depression in some patients (47, 48). A majority of immune cells express 5-HT receptors and it has been hypothesized that serotonergic psychedelics impact immunomodulatory agents through 5-HT2AR agonism (49). Congruent with this hypothesis, several in vitro studies have shown decreases of IL-6 in human cells following administration of DMT (50), 5-MeO-DMT (50), LSD (51), or psilocybin (52). Similar findings are reported for other inflammatory markers such as IL-1β, CRP, and TNF-α (50, 53). Studies with ayahuasca suggest psychedelics may have immunomodulatory effects through decreasing white blood cells (CD4) and elevating natural killer cells (54). Overall, depressive symptoms have been linked to a shift of the immune system toward inflammatory responses. Although further evidence is required, serotonergic psychedelics may, in part, exert their antidepressant effect through activation of anti-inflammatory and immunomodulatory actions.
Table 1 presents a summary of the reviewed neuroimaging findings. Most studies of psychedelics have used functional magnetic resonance imaging (fMRI) with network connections assessed using functional connectivity (FC). FC measures correlations between Blood-Oxygen-Level-Dependent (BOLD) signal fluctuations (55) as a proxy for brain activity (56). Research has focused on the default mode network (DMN), encompassing the medial prefrontal cortex (mPFC), posterior cingulate cortex (PCC), precuneus, and angular gyrus. The DMN is implicated in self-referential thinking, memory, and rumination. Alterations in DMN connectivity may underlie the excessive internal focus and rumination of depression. DMN hyper-connectivity has been reported in MDD patients and correlates positively with rumination (57). Imaging data indicates that psychedelics generally reduces within-network DMN FC. This is evidenced by studies in healthy volunteers showing reductions of within-DMN FC during the acute psychedelic experience or post-treatment with psilocybin, ayahuasca, or LSD (55, 56, 58–65). Positron emission tomography (PET) scans show psilocybin increases in glucose metabolism in the PFC, anterior cingulate, and temporomedial cortex (66). In depressed patients, reductions in within-DMN FC has been observed after treatment with psilocybin (67–69), specifically between the vmPFC and right angular gyrus (69), and between the parahippocampus (PH) and PFC (67). Reduced PH-PFC FC has been associated with improvements in depression scores post-treatment (67), supporting the hypothesis that hyper-connectivity of the DMN underpins depressive symptoms. Thus, administration of a psychedelic could alleviate symptoms by reducing FC in the DMN. Indeed, increased glucose metabolism in the DMN area is positively associated with changes to perception and ego dissolution (66). Also, psychedelic-induced reduction in DMN FC has been correlated with positive changes in psychosocial functioning, attitudes and mood, 4 months post-administration (55) and with decreased mental reflection on one’s past (59).
However, increases in FC have been observed post-psilocybin in depressed patients between the vmPFC and the bilateral inferior lateral parietal cortex (iIPC) (67) and between the ACC and PCC (67, 70). Increased vmPFC-iIPC FC (but not increased ACC-PCC FC) was associated with treatment response at 5-weeks (67). It has been theorized that these discrepancies may result from differences in neural effects immediately following the acute psilocybin experience vs. those that occur later. Like electroconvulsive therapy (ECT), psychedelics may cause acute decreases in network integrity followed by a period of re-integration and subsequent improvements in mood and functioning (67). This interpretation is speculative and more research is needed to elucidate how altered connectivity patterns mediate antidepressant activity of psychedelics.
Emotional processing is also altered by psychedelics through changes in amygdala responsiveness and FC changes between the DMN, amygdala, and visual cortices. Single photon emission tomography (SPECT) in depressed patients administered ayahuasca showed increased blood perfusion in the left nucleus accumbens, right insula, and left subgenual area (9). These areas play a major role in emotional regulation and are less active in depressed patients (9). The amygdala is also hypersensitive to negative stimuli in depressed patient (71, 72) resulting in negative cognitive bias (73) and deficits in emotional regulation (74). SSRIs reduce amygdala responsiveness (75, 76) and a similar mechanism has been proposed for the antidepressant effect of psychedelics. In healthy volunteers, LSD reduce reactivity of the left amygdala to fearful faces (77) and psilocybin reduces response of the right amygdala to negative and neutral stimuli, with these reductions correlating with increases in positive affect (78). In depressed patients, opposite results have been reported: psilocybin induced increased right amygdala BOLD response to emotional faces (with a larger increase with fearful than with happy faces) 1 day post-treatment; larger responses to fearful faces correlated with higher improvements in depressive symptoms (79). This suggests psychedelics may actually restore emotional responsiveness in depressed individuals, in contrast to the emotional blunting associated with SSRIs (79). In patients with TRD, 1 day post-psilocybin, fMRI scans taken while viewing fearful faces also revealed reductions in connectivity between the vmPFC and right amygdala; it was associated with less rumination 1 week later, suggesting that disinhibition of the right amygdala by the vmPFC mediates psilocybin-induced increases in amygdala responsiveness in these depressed individuals (69). Under the same conditions, higher connectivity was also observed between the vmPFC and the right lateral occipital cortex, occipital pole, and fusiform gyrus; it was associated with improvements in depressive symptoms (69). Similar hyper-connectivity between the DMN and the occipital cortex has been reported in healthy volunteers after administration of LSD (64) or psilocybin (65), and between the amygdala and occipital cortex after administration of psilocybin (80). Deficits in down-regulation of the visual cortex in response to negative emotional stimuli has been demonstrated in MDD (81). Thus, psychedelics may help to normalize emotional processing in depressed patients by increasing vmPFC-mediated inhibition of the occipital cortex in response to negative stimuli, thereby reducing negative attentional bias. Taken together, these findings suggest a complex interplay among signals from the DMN, amygdala, and visual cortex may be involved in regulation of emotional response induced by psychedelics in MDD.
Finally, psychedelics increase connectivity between the intrinsic functional networks, i.e., spatially distinct brain regions that are functionally related (82). Brain-wide network disconnectivity is associated with depressive symptoms (83). Thus some antidepressant effects of psychedelics may also result from their ability to increase between-network FC. These increases have been observed in healthy volunteers after administration of psilocybin (56, 61, 84) or LSD (60, 62), particularly in areas rich in 5-HT2AR (60). In depression, psilocybin has been shown to increase global FC (68, 70). In one open-label trial, increase in global FC 1 day after psilocybin dosing was associated with improvement in depressive symptoms 6 months later (68). Specific increases were observed between the DMN and Executive Network (EN), Salience Network (SN). A randomized controlled trial comparing two doses of psilocybin vs. 6 weeks of daily escitalopram for depression saw increased EN dynamic flexibility with psilocybin only–reflecting an increase in the frequency of connectivity changes seen the during fMRI scan. This correlated with symptom improvement 6 weeks post-dose (68). Psilocybin has also been shown to alter connectivity patterns between the task positive network (TPN) (85), which is involved with external or other-processing (82), and the claustrum, or the DMN. Theoretically, the claustrum, known to be volumetrically reduced in depression, may mediate psychedelic-induced network disruptions due to its widespread connectivity (85).
Most psychedelic studies discussed in this section suffer from limitations, in particular difficulties in blinding and small sample sizes. Still, fMRI studies in depression have produced relatively consistent findings and their designs have been rigorous. As summarized in Table 1, of the six fMRI depression studies reviewed, five were preregistered (68–70, 79), all used validated depression scales (67–70, 79) and one was double-blinded and placebo-controlled (68).
As summarized in Table 2, neurophysiology studies provide further support for DMN-related alterations. Electroencephalogram (EEG) recordings in healthy volunteers given LSD or ayahuasca show reductions in broadband oscillatory power and cortical synchrony particularly within areas of the DMN (86–88). Similarly, magnetoencephalography (MEG) shows broadband desynchronization of cortical oscillatory rhythms and decreases in network integrity after ingestion of psilocybin (89), supporting that psychedelics can disorganize spontaneous brain activity (58, 89). In participants who ingested LSD, EEG, and MEG studies have also showed a relationship between decreased alpha power and the hallucinatory experience and subjective reports of ego dissolution (87, 90).
High frequency gamma waves appear when individuals are performing cognitively challenging tasks requiring focus (66). The limited current literature reports increases in gamma power in individuals administered psilocybin. This supports the theory that the DMN is affected by psychedelics as the PFC and hippocampus are activated during tasks requiring concentration in which gamma waves are present (66). However, given the sparsity of relevant human data, this theory remains speculative. Evidence suggests that changes from delta to gamma waves are consistent across different psychedelics (91). Additionally, LSD, ayahuasca, and psilocybin induce alpha power reductions within the occipital and parietal cortices, which are important structures for the interpretation of visual stimuli (88). It is believed this reduction explains subjective reports of visual hallucinations as alpha oscillations have been found to play a role in cortical processing of sensory information (92). Finally, under the influence of ayahuasca and LSD, psychedelic effects such as ego dissolution and spiritual feelings have been reported during moments of global decreases in alpha waves (92). Alpha oscillations are correlated with functions in which the DMN plays a major role such as self-reflection; as discussed above, fMRI studies support this association (93). Psychedelic-induced ego dissolution in patients with MDD is correlated with improvement in depressive symptoms, supporting the theory that ego dissolution is associated with decreased connectivity within the DMN and further establishing a possible link between alpha oscillations and the DMN (92, 93).
Many depressed individuals experience ruminations, with intense self-focus and narrowed thinking. Enhanced neural and cognitive flexibility associated with psychedelics may enhance psychological flexibility, affording them access to a broader frame of mind (68). Thus, it has been hypothesized that psychedelics facilitate transitions away from maladaptive thought patterns, which can be amplified during the “integration” component of psychedelic-assisted psychotherapy (PAP) post-treatment (70). The antidepressant effect of psychedelics has also been postulated to result from their ability to restore emotional responsiveness (86), allowing people to fully experience and accept their emotions (76, 86). This emotional release is thought to be facilitated by disruption of connectivity in regions with high density of 5-HT2ARs (75). Ego dissolution, characterized by a blurring of the distinction between self and other (68) is commonly experienced with psychedelics (63, 67, 68). It has been speculated it allows for an enhanced sense of connection with others and lessen feelings of loneliness. Finally, “mystical experiences” may also play some role in relieving depressive symptoms but this has not yet been evaluated (74). In summary, psychedelics may facilitate some degree of psychological transformation, possibly allowing patients to move beyond their depression.
Overall, available evidence suggests serotonergic psychedelics impact depression, in part through serotonin receptor agonism, neurogenesis, immunomodulation, widespread changes in connectivity within the brain, and psychological effects. To date, neuroimaging studies provide the most robust investigation into the mechanisms of action of psychedelics, but their results remain limited. More studies with larger and more diverse samples are needed to replicate and extend current findings. Future studies should also account for symptom severity and comorbidities while assessing whether and how PAP and social supports contribute to the therapeutic action of psychedelics. Neural correlates of the antidepressant effects of psychedelics and other pharmacotherapies should be compared directly to quantify differences between mechanisms of action.
Understanding the role of the psychedelic experience and its relationship with antidepressant effect should also be a focus of future research. As it stands, alterations in perception induced by psychedelics are a major barrier to their clinical adoption because they require intensive psychological support. As stated above, it is possible that psilocybin’s antidepressant effect is mediated through rapid activation of 5-HT receptors other than those involved in the psychedelic experience. This raises the possibility of blocking the psychedelic experience by co-administering 5-HT2A antagonists, like ketanserin or risperidone, without impeding the antidepressant therapeutic effect. More research is needed to explore this possibility and better understand the relationship among serotonin receptors, psychedelic effect, and antidepressant effect.
As use of, and research on, psychedelics expand, understanding their mechanism of action through molecular science, neuroimaging, and neurophysiology is critical. With several relevant competing theories, well-designed studies need to determine which mechanisms are central to their therapeutic action and which ones are epiphenomenal.
MIH and DC conceived and designed the manuscript. MIH drafted and critically reviewed the manuscript. NL conducted the literature search, reviewed available data, and contributed to the writing, editing, and preparation of the manuscript. EF and JB contributed to the literature search, data review, and writing of the manuscript. JR, DB, BM, and DC drafted the manuscript. All authors approved the manuscript.
MIH received research support from the Brain and Behavior Research Foundation, Canadian Institutes of Health Research (CIHR), CAMH Foundation, Grand Challenges Canada, the PSI Foundation, and the University of Toronto and provided consultancy to Mindset Pharma, PsychEd Therapeutics, and Wake Network. JR received research grant support from the Canadian Institute of Health Research (CIHR), Physician Services Inc (PSI) Foundation, Labatt Brain Health Network, Brain and Cognition Discovery Foundation (BCDF), Canadian Cancer Society, Canadian Psychiatric Association, Academic Scholars Award, American Psychiatric Association, American Society of Psychopharmacology, University of Toronto, University Health Network Centre for Mental Health, Joseph M. West Family Memorial Fund and Timeposters Fellowship and industry funding for speaker/consultation/research fees from iGan, Boehringer Ingelheim, Janssen, Allergan, Lundbeck, Sunovion, and COMPASS. He is the Chief Medical and Scientific Officer of Braxia Scientific and the medical director of the Canadian Rapid Treatment Centre of Excellence (Braxia Health). BM hold and received support from the Labatt Family Chair in Biology of Depression in Late-Life Adults at the University of Toronto and currently receives or has received with the past 5 years research support from Brain Canada, the Canadian Institutes of Health Research, the CAMH Foundation, the Patient-Centered Outcomes Research Institute (PCORI), the US National Institute of Health (NIH), Capital Solution Design LLC (software used in a study founded by CAMH Foundation), and HAPPYneuron (software used in a study founded by Brain Canada); has also been an unpaid consultant to Myriad Neuroscience. DC (past 36 months: September 2022) received grant monies for research from Servier, Boehringer Ingelheim; Travel Support and Honoraria for Talks and Consultancy from Servier, Seqirus, and Lundbeck. He is a founder of the Optimal Health Program (OHP), and holds 50% of the IP for OHP; and is part owner (5%) of Clarity Healthcare. He is an Advisory Board Chair of an Australian not-for-profit institute specializing in psychedelic medicines research. He does not knowingly have stocks or shares in any pharmaceutical company. DB received research support from the Canadian Institutes of Health Research (CIHR), National Institutes of Health—US (NIH), Brain Canada Foundation and the Temerty Family through the CAMH Foundation, and the Campbell Family Research Institute. He received research support and in-kind equipment support for an investigator-initiated study from Brainsway Ltd. and he was the site principal investigator for three sponsor-initiated studies for Brainsway Ltd. He received in-kind equipment support from Magventure for investigator-initiated studies. He received medication supplies for an investigator-initiated trial from Indivior. He participated in an advisory board for Janssen. He participated in an advisory board for Welcony Inc.
The remaining authors declare that the research was conducted in the absence of any commercial or financial relationships that could be construed as a potential conflict of interest.
All claims expressed in this article are solely those of the authors and do not necessarily represent those of their affiliated organizations, or those of the publisher, the editors and the reviewers. Any product that may be evaluated in this article, or claim that may be made by its manufacturer, is not guaranteed or endorsed by the publisher.
1. Hardeveld F, Spijker J, De Graaf R, Nolen WA, Beekman AT. Prevalence and predictors of recurrence of major depressive disorder in the adult population. Acta Psychiatr Scand. (2010) 122:184–91. doi: 10.1111/j.1600-0447.2009.01519.x
2. Kennedy S, Lam R, McIntyre R, Tourjman S, Bhat V, Blier P, et al. Canadian network for mood and anxiety treatments (CANMAT) 2016 clinical guidelines for the management of adults with major depressive disorder: section 3. Pharmacological treatments. Can J Psychiatry. (2016) 61:540–60. doi: 10.1177/0706743716659417
3. Gaynes B, Warden D, Trivedi M, Wisniewski S, Fava M, Rush A. What did STAR*D teach us? Results from a large-scale, practical, clinical trial for patients with depression. Psychiatr Serv. (2009) 60:1439–45. doi: 10.1176/ps.2009.60.11.1439
4. Olfson M, Marcus S. National patterns in antidepressant medication treatment. Arch Gen Psychiatry. (2009) 66:848–56. doi: 10.1001/archgenpsychiatry.2009.81
5. Olfson M, Marcus S, Tedeschi M, Wan G. Continuity of antidepressant treatment for adults with depression in the United States. Am J Psychiatry. (2006) 163:101–8. doi: 10.1176/appi.ajp.163.1.101
6. Carod-Artal F. Hallucinogenic drugs in pre-Columbian mesoamerican cultures. Neurologia. (2015) 30:42–9. doi: 10.1016/j.nrleng.2011.07.010
7. Belouin S, Henningfield J. Psychedelics: where we are now, why we got here, what we must do. Neuropharmacology. (2018) 142:7–19. doi: 10.1016/j.neuropharm.2018.02.018
8. Palhano-Fontes F, Barreto D, Onias H, Andrade K, Novaes M, Pessoa J, et al. Rapid antidepressant effects of the psychedelic ayahuasca in treatment-resistant depression: a randomized placebo-controlled trial. Psychol Med. (2019) 49:655–63. doi: 10.1017/S0033291718001356
9. Sanches RF, de Lima Osório F, Dos Santos RG, Macedo LR, Maia-de-Oliveira JP, Wichert-Ana L, et al. Antidepressant effects of a single dose of ayahuasca in patients with recurrent depression: a SPECT study. J Clin Psychopharmacol. (2016) 36:77–81. doi: 10.1097/JCP.0000000000000436
10. Gasser P, Holstein D, Michel Y, Doblin R, Yazar-Klosinski B, Passie T, et al. Safety and efficacy of lysergic acid diethylamide-assisted psychotherapy for anxiety associated with life-threatening diseases. J Nerv Ment Dis. (2014) 202:513–20. doi: 10.1097/NMD.0000000000000113
11. Carhart-Harris R, Giribaldi B, Watts R, Baker-Jones M, Murphy-Beiner A, Murphy R, et al. Trial of psilocybin versus escitalopram for depression. N Engl J Med. (2021) 384:1402–11. doi: 10.1056/NEJMoa2032994
12. Carhart-Harris RL, Bolstridge M, Rucker J, Day CM, Erritzoe D, Kaelen M, et al. Psilocybin with psychological support for treatment-resistant depression: an open-label feasibility study. Lancet Psychiatry. (2016) 3:619–27. doi: 10.1016/S2215-0366(16)30065-7
13. Davis AK, Barrett FS, May DG, Cosimano MP, Sepeda ND, Johnson MW, et al. Effects of psilocybin-assisted therapy on major depressive disorder: a randomized clinical trial. JAMA Psychiatry. (2021) 78:481–9. doi: 10.1001/jamapsychiatry.2020.3285
14. Griffiths RR, Johnson MW, Carducci MA, Umbricht A, Richards WA, Richards BB, et al. Psilocybin produces substantial and sustained decreases in depression and anxiety in patients with life-threatening cancer: a randomized double-blind trial. J Psychopharmacol. (2016) 30:1181–97. doi: 10.1177/0269881116675513
15. Grob CS, Bossis AP, Griffiths RR. Use of the classic hallucinogen psilocybin for treatment of existential distress associated with cancer. In: Steel J, Carr B editors. Psychological Aspects of Cancer: A Guide to Emotional and Psychological Consequences of Cancer, Their Causes, and Their Management. Cham: Springer International Publishing (2022). p. 69–89. doi: 10.1007/978-3-030-85702-8_5
16. Hamon M, Blier P. Monoamine neurocircuitry in depression and strategies for new treatments. Prog Neuropsychopharmacol Biol Psychiatry. (2013) 45:54–63. doi: 10.1016/j.pnpbp.2013.04.009
17. Shopsin B, Friedman E, Gershon S. Parachlorophenylalanine reversal of tranylcypromine effects in depressed patients. Arch Gen Psychiatry. (1976) 33:811–9. doi: 10.1001/archpsyc.1976.01770070041003
18. Shopsin B, Gershon S, Goldstein M, Friedman E, Wilk S. Use of synthesis inhibitors in defining a role for biogenic amines during imipramine treatment in depressed patients. Psychopharmacol Commun. (1975) 1:239–49.
19. Vollenweider F, Vollenweider-Scherpenhuyzen M, Bäbler A, Vogel H, Hell D. Psilocybin induces schizophrenia-like psychosis in humans via a serotonin-2 agonist action. Neuroreport. (1998) 9:3897–902. doi: 10.1097/00001756-199812010-00024
20. López-Giménez J, González-Maeso J. Hallucinogens and serotonin 5-HT(2A) receptor-mediated signaling pathways. Curr Top Behav Neurosci. (2018) 36:45–73. doi: 10.1007/7854_2017_478
21. Eison A, Mullins U. Regulation of central 5-HT2A receptors: a review of in vivo studies. Behav Brain Res. (1996) 73:177–81. doi: 10.1016/0166-4328(96)00092-7
22. Dos Santos R, Hallak J, Baker G, Dursun S. Hallucinogenic/psychedelic 5HT2A receptor agonists as rapid antidepressant therapeutics: evidence and mechanisms of action. J Psychopharmacol. (2021) 35:453–8. doi: 10.1177/0269881120986422
23. Johnson M, Hendricks P, Barrett F, Griffiths R. Classic psychedelics: an integrative review of epidemiology, therapeutics, mystical experience, and brain network function. Pharmacol Ther. (2019) 197:83–102. doi: 10.1016/j.pharmthera.2018.11.010
24. Hesselgrave N, Troppoli T, Wulff A, Cole A, Thompson S. Harnessing psilocybin: antidepressant-like behavioral and synaptic actions of psilocybin are independent of 5-HT2R activation in mice. Proc Natl Acad Sci USA. (2021) 118:e2022489118. doi: 10.1073/pnas.2022489118
25. Marek G, Aghajanian G. LSD and the phenethylamine hallucinogen DOI are potent partial agonists at 5-HT2A receptors on interneurons in rat piriform cortex. J Pharmacol Exp Ther. (1996) 278:1373–82.
26. De Gregorio D, Comai S, Posa L, Gobbi G. d-Lysergic acid diethylamide (LSD) as a model of psychosis: mechanism of action and pharmacology. Int J Mol Sci. (2016) 17:1953. doi: 10.3390/ijms17111953
27. Rickli A, Moning O, Hoener M, Liechti M. Receptor interaction profiles of novel psychoactive tryptamines compared with classic hallucinogens. Eur Neuropsychopharmacol. (2016) 26:1327–37. doi: 10.1016/j.euroneuro.2016.05.001
28. Inserra A, De Gregorio D, Gobbi G. Psychedelics in psychiatry: neuroplastic, immunomodulatory, and neurotransmitter mechanisms. Pharmacol Rev. (2021) 73:202–77. doi: 10.1124/pharmrev.120.000056
29. Kim Y, Lee H, Won S, Park E, Lee H, Lee B, et al. Low plasma BDNF is associated with suicidal behavior in major depression. Prog Neuropsychopharmacol Biol Psychiatry. (2007) 31:78–85. doi: 10.1016/j.pnpbp.2006.06.024
30. Lee B, Kim H, Park S, Kim Y. Decreased plasma BDNF level in depressive patients. J Affect Disord. (2007) 101:239–44. doi: 10.1016/j.jad.2006.11.005
31. Aydemir O, Deveci A, Taneli F. The effect of chronic antidepressant treatment on serum brain-derived neurotrophic factor levels in depressed patients: a preliminary study. Prog Neuropsychopharmacol Biol Psychiatry. (2005) 29:261–5. doi: 10.1016/j.pnpbp.2004.11.009
32. Shimizu E, Hashimoto K, Okamura N, Koike K, Komatsu N, Kumakiri C, et al. Alterations of serum levels of brain-derived neurotrophic factor (BDNF) in depressed patients with or without antidepressants. Biol Psychiatry. (2003) 54:70–5. doi: 10.1016/S0006-3223(03)00181-1
33. Lu J, Xu Y, Hu W, Gao Y, Ni X, Sheng H, et al. Exercise ameliorates depression-like behavior and increases hippocampal BDNF level in ovariectomized rats. Neurosci Lett. (2014) 573:13–8. doi: 10.1016/j.neulet.2014.04.053
34. Wolkowitz O, Wolf J, Shelly W, Rosser R, Burke H, Lerner G, et al. Serum BDNF levels before treatment predict SSRI response in depression. Prog Neuropsychopharmacol Biol Psychiatry. (2011) 35:1623–30. doi: 10.1016/j.pnpbp.2011.06.013
35. Colaço C, Alves S, Nolli L, Pinheiro W, de Oliveira D, Santos B, et al. Toxicity of ayahuasca after 28 days daily exposure and effects on monoamines and brain-derived neurotrophic factor (BDNF) in brain of Wistar rats. Metab Brain Dis. (2020) 35:739–51. doi: 10.1007/s11011-020-00547-w
36. de Almeida R, Galvão A, da Silva F, Silva E, Palhano-Fontes F, Maia-de-Oliveira J, et al. Modulation of serum brain-derived neurotrophic factor by a single dose of ayahuasca: observation from a randomized controlled trial. Front Psychol. (2019) 10:1234. doi: 10.3389/fpsyg.2019.01234
37. Becker A, Holze F, Grandinetti T, Klaiber A, Toedtli V, Kolaczynska K, et al. Acute effects of psilocybin after escitalopram or placebo pretreatment in a randomized, double-blind, placebo-controlled, crossover study in healthy subjects. Clin Pharmacol Ther. (2022) 111:886–95. doi: 10.1002/cpt.2487
38. Hutten N, Mason N, Dolder P, Theunissen E, Holze F, Liechti M, et al. Low doses of LSD acutely increase BDNF blood plasma levels in healthy volunteers. ACS Pharmacol Transl Sci. (2021) 4:461–6. doi: 10.1021/acsptsci.0c00099
39. Krishnan V, Nestler E. The molecular neurobiology of depression. Nature. (2008) 455:894–902. doi: 10.1038/nature07455
40. Ohira K, Takeuchi R, Shoji H, Miyakawa T. Fluoxetine-induced cortical adult neurogenesis. Neuropsychopharmacology. (2013) 38:909–20. doi: 10.1038/npp.2013.2
41. Santarelli L, Saxe M, Gross C, Surget A, Battaglia F, Dulawa S, et al. Requirement of hippocampal neurogenesis for the behavioral effects of antidepressants. Science. (2003) 301:805–9. doi: 10.1126/science.1083328
42. Lima da Cruz RV, Moulin T, Petiz L, Leão R. A single dose of 5-MeO-DMT stimulates cell proliferation, neuronal survivability, morphological and functional changes in adult mice ventral dentate gyrus. Front Mol Neurosci. (2018) 11:312. doi: 10.3389/fnmol.2018.00312
43. Catlow B, Song S, Paredes D, Kirstein C, Sanchez-Ramos J. Effects of psilocybin on hippocampal neurogenesis and extinction of trace fear conditioning. Exp Brain Res. (2013) 228:481–91. doi: 10.1007/s00221-013-3579-0
44. Dantzer R, O’Connor J, Freund G, Johnson R, Kelley K. From inflammation to sickness and depression: when the immune system subjugates the brain. Nat Rev Neurosci. (2008) 9:46–56. doi: 10.1038/nrn2297
45. Dowlati Y, Herrmann N, Swardfager W, Liu H, Sham L, Reim E, et al. A meta-analysis of cytokines in major depression. Biol Psychiatry. (2010) 67:446–57. doi: 10.1016/j.biopsych.2009.09.033
46. Felger JC, Lotrich F. Inflammatory cytokines in depression: neurobiological mechanisms and therapeutic implications. Neuroscience. (2013) 246:199–229. doi: 10.1016/j.neuroscience.2013.04.060
47. Müller N, Schwarz M. The immune-mediated alteration of serotonin and glutamate: towards an integrated view of depression. Mol Psychiatry. (2007) 12:988–1000. doi: 10.1038/sj.mp.4002006
48. Weiss G, Murr C, Zoller H, Haun M, Widner B, Ludescher C, et al. Modulation of neopterin formation and tryptophan degradation by Th1- and Th2-derived cytokines in human monocytic cells. Clin Exp Immunol. (1999) 116:435–40. doi: 10.1046/j.1365-2249.1999.00910.x
49. Flanagan T, Nichols C. Psychedelics as anti-inflammatory agents. Int Rev Psychiatry. (2018) 30:363–75. doi: 10.1080/09540261.2018.1481827
50. Szabo A, Kovacs A, Frecska E, Rajnavolgyi E. Psychedelic N,N-dimethyltryptamine and 5-methoxy-N,N-dimethyltryptamine modulate innate and adaptive inflammatory responses through the sigma-1 receptor of human monocyte-derived dendritic cells. PLoS One. (2014) 9:e106533. doi: 10.1371/journal.pone.0106533
51. House R, Thomas P, Bhargava H. Immunological consequences of in vitro exposure to lysergic acid diethylamide (LSD). Immunopharmacol Immunotoxicol. (1994) 16:23–40. doi: 10.3109/08923979409029898
52. Nkadimeng S, Steinmann C, Eloff J. Anti-inflammatory effects of four psilocybin-containing magic mushroom water extracts in vitro on 15-lipoxygenase activity and on lipopolysaccharide-induced cyclooxygenase-2 and inflammatory cytokines in human U937 macrophage cells. J Inflamm Res. (2021) 14:3729–38. doi: 10.2147/JIR.S317182
53. Galvão-Coelho N, de Menezes Galvão A, de Almeida R, Palhano-Fontes F, Campos Braga I, Lobão Soares B, et al. Changes in inflammatory biomarkers are related to the antidepressant effects of ayahuasca. J Psychopharmacol. (2020) 34:1125–33. doi: 10.1177/0269881120936486
54. Dos Santos R, Grasa E, Valle M, Ballester M, Bouso J, Nomdedéu J, et al. Pharmacology of ayahuasca administered in two repeated doses. Psychopharmacology (Berl). (2012) 219:1039–53. doi: 10.1007/s00213-011-2434-x
55. Smigielski L, Scheidegger M, Kometer M, Vollenweider F. Psilocybin-assisted mindfulness training modulates self-consciousness and brain default mode network connectivity with lasting effects. Neuroimage. (2019) 196:207–15. doi: 10.1016/j.neuroimage.2019.04.009
56. Carhart-Harris R, Leech R, Erritzoe D, Williams T, Stone J, Evans J, et al. Functional connectivity measures after psilocybin inform a novel hypothesis of early psychosis. Schizophr Bull. (2013) 39:1343–51. doi: 10.1093/schbul/sbs117
57. Zhu X, Zhu Q, Shen H, Liao W, Yuan F. Rumination and default mode network subsystems connectivity in first-episode, drug-naive young patients with major depressive disorder. Sci Rep. (2017) 7:43105. doi: 10.1038/srep43105
58. Carhart-Harris R, Erritzoe D, Williams T, Stone J, Reed L, Colasanti A, et al. Neural correlates of the psychedelic state as determined by fMRI studies with psilocybin. Proc Natl Acad Sci USA. (2012) 109:2138–43. doi: 10.1073/pnas.1119598109
59. Speth J, Speth C, Kaelen M, Schloerscheidt A, Feilding A, Nutt D, et al. Decreased mental time travel to the past correlates with default-mode network disintegration under lysergic acid diethylamide. J Psychopharmacol. (2016) 30:344–53. doi: 10.1177/0269881116628430
60. Tagliazucchi E, Roseman L, Kaelen M, Orban C, Muthukumaraswamy S, Murphy K, et al. Increased global functional connectivity correlates with LSD-induced ego dissolution. Curr Biol. (2016) 26:1043–50. doi: 10.1016/j.cub.2016.02.010
61. Madsen M, Stenbæk D, Arvidsson A, Armand S, Marstrand-Joergensen M, Johansen S, et al. Psilocybin-induced changes in brain network integrity and segregation correlate with plasma psilocin level and psychedelic experience. Eur Neuropsychopharmacol. (2021) 50:121–32. doi: 10.1016/j.euroneuro.2021.06.001
62. Müller F, Dolder P, Schmidt A, Liechti M, Borgwardt S. Altered network hub connectivity after acute LSD administration. Neuroimage Clin. (2018) 18:694–701. doi: 10.1016/j.nicl.2018.03.005
63. Palhano-Fontes F, Andrade K, Tofoli L, Santos A, Crippa J, Hallak J, et al. The psychedelic state induced by ayahuasca modulates the activity and connectivity of the default mode network. PLoS One. (2015) 10:e0118143. doi: 10.1371/journal.pone.0118143
64. Preller K, Burt J, Ji J, Schleifer C, Adkinson B, Stämpfli P, et al. Changes in global and thalamic brain connectivity in LSD-induced altered states of consciousness are attributable to the 5-HT2A receptor. Elife. (2018) 7:e35082. doi: 10.7554/eLife.35082
65. Preller K, Duerler P, Burt J, Ji J, Adkinson B, Stämpfli P, et al. Psilocybin induces time-dependent changes in global functional connectivity. Biol Psychiatry. (2020) 88:197–207. doi: 10.1016/j.biopsych.2019.12.027
66. Smausz R, Neill J, Gigg J. Neural mechanisms underlying psilocybin’s therapeutic potential - the need for preclinical in vivo electrophysiology. J Psychopharmacol. (2022) 36:781–93. doi: 10.1177/02698811221092508
67. Carhart-Harris R, Roseman L, Bolstridge M, Demetriou L, Pannekoek J, Wall M, et al. Psilocybin for treatment-resistant depression: fMRI-measured brain mechanisms. Sci Rep. (2017) 7:13187. doi: 10.1038/s41598-017-13282-7
68. Daws R, Timmermann C, Giribaldi B, Sexton J, Wall M, Erritzoe D, et al. Increased global integration in the brain after psilocybin therapy for depression. Nat Med. (2022) 28:844–51. doi: 10.1038/s41591-022-01744-z
69. Mertens L, Wall M, Roseman L, Demetriou L, Nutt D, Carhart-Harris R. Therapeutic mechanisms of psilocybin: changes in amygdala and prefrontal functional connectivity during emotional processing after psilocybin for treatment-resistant depression. J Psychopharmacol. (2020) 34:167–80. doi: 10.1177/0269881119895520
70. Doss M, Považan M, Rosenberg M, Sepeda N, Davis A, Finan P, et al. Psilocybin therapy increases cognitive and neural flexibility in patients with major depressive disorder. Transl Psychiatry. (2021) 11:574. doi: 10.1038/s41398-021-01706-y
71. Drevets W, Videen T, Price J, Preskorn S, Carmichael S, Raichle M. A functional anatomical study of unipolar depression. J Neurosci. (1992) 12:3628–41. doi: 10.1523/JNEUROSCI.12-09-03628.1992
72. Ma Y. Neuropsychological mechanism underlying antidepressant effect: a systematic meta-analysis. Mol Psychiatry. (2015) 20:311–9. doi: 10.1038/mp.2014.24
73. Mennen A, Norman K, Turk-Browne N. Attentional bias in depression: understanding mechanisms to improve training and treatment. Curr Opin Psychol. (2019) 29:266–73. doi: 10.1016/j.copsyc.2019.07.036
74. Beauregard M, Paquette V, Lévesque J. Dysfunction in the neural circuitry of emotional self-regulation in major depressive disorder. Neuroreport. (2006) 17:843–6. doi: 10.1097/01.wnr.0000220132.32091.9f
75. Godlewska B, Norbury R, Selvaraj S, Cowen P, Harmer C. Short-term SSRI treatment normalises amygdala hyperactivity in depressed patients. Psychol Med. (2012) 42:2609–17. doi: 10.1017/S0033291712000591
76. Harmer C, Duman R, Cowen P. How do antidepressants work? New perspectives for refining future treatment approaches. Lancet Psychiatry. (2017) 4:409–18. doi: 10.1016/S2215-0366(17)30015-9
77. Mueller F, Lenz C, Dolder P, Harder S, Schmid Y, Lang U, et al. Acute effects of LSD on amygdala activity during processing of fearful stimuli in healthy subjects. Transl Psychiatry. (2017) 7:e1084. doi: 10.1038/tp.2017.54
78. Kraehenmann R, Preller K, Scheidegger M, Pokorny T, Bosch O, Seifritz E, et al. Psilocybin-induced decrease in amygdala reactivity correlates with enhanced positive mood in healthy volunteers. Biol Psychiatry. (2015) 78:572–81. doi: 10.1016/j.biopsych.2014.04.010
79. Roseman L, Demetriou L, Wall M, Nutt D, Carhart-Harris R. Increased amygdala responses to emotional faces after psilocybin for treatment-resistant depression. Neuropharmacology. (2018) 142:263–9. doi: 10.1016/j.neuropharm.2017.12.041
80. Grimm O, Kraehenmann R, Preller K, Seifritz E, Vollenweider F. Psilocybin modulates functional connectivity of the amygdala during emotional face discrimination. Eur Neuropsychopharmacol. (2018) 28:691–700. doi: 10.1016/j.euroneuro.2018.03.016
81. van Dam W, Chrysikou E. Effects of unilateral tDCS over left prefrontal cortex on emotion regulation in depression: evidence from concurrent functional magnetic resonance imaging. Cogn Affect Behav Neurosci. (2021) 21:14–34. doi: 10.3758/s13415-020-00830-4
82. Knyazev G, Savostyanov A, Bocharov A, Levin E, Rudych P. Intrinsic connectivity networks in the self- and other-referential processing. Front Hum Neurosci. (2020) 14:579703. doi: 10.3389/fnhum.2020.579703
83. Li B, Friston K, Mody M, Wang H, Lu H, Hu D. A brain network model for depression: from symptom understanding to disease intervention. CNS Neurosci Ther. (2018) 24:1004–19. doi: 10.1111/cns.12998
84. McCulloch D, Madsen M, Stenbæk D, Kristiansen S, Ozenne B, Jensen P, et al. Lasting effects of a single psilocybin dose on resting-state functional connectivity in healthy individuals. J Psychopharmacol. (2022) 36:74–84. doi: 10.1177/02698811211026454
85. Barrett F, Krimmel S, Griffiths R, Seminowicz D, Mathur B. Psilocybin acutely alters the functional connectivity of the claustrum with brain networks that support perception, memory, and attention. Neuroimage. (2020) 218:116980. doi: 10.1016/j.neuroimage.2020.116980
86. Riba J, Anderer P, Morte A, Urbano G, Jané F, Saletu B, et al. Topographic pharmaco-EEG mapping of the effects of the South American psychoactive beverage ayahuasca in healthy volunteers. Br J Clin Pharmacol. (2002) 53:613–28. doi: 10.1046/j.1365-2125.2002.01609.x
87. Murray C, Tare I, Perry C, Malina M, Lee R, de Wit H. Low doses of LSD reduce broadband oscillatory power and modulate event-related potentials in healthy adults. Psychopharmacology. (2022) 239:1735–47. doi: 10.1007/s00213-021-05991-9
88. Kometer M, Schmidt A, Jäncke L, Vollenweider F. Activation of serotonin 2A receptors underlies the psilocybin-induced effects on α oscillations, N170 visual-evoked potentials, and visual hallucinations. J Neurosci. (2013) 33:10544–51. doi: 10.1523/JNEUROSCI.3007-12.2013
89. Muthukumaraswamy S, Carhart-Harris R, Moran R, Brookes M, Williams T, Errtizoe D, et al. Broadband cortical desynchronization underlies the human psychedelic state. J Neurosci. (2013) 33:15171–83. doi: 10.1523/JNEUROSCI.2063-13.2013
90. Carhart-Harris R, Muthukumaraswamy S, Roseman L, Kaelen M, Droog W, Murphy K, et al. Neural correlates of the LSD experience revealed by multimodal neuroimaging. Proc Natl Acad Sci USA. (2016) 113:4853–8. doi: 10.1073/pnas.1518377113
91. Vejmola Č, Tylš F, Piorecká V, Koudelka V, Kadeřábek L, Novák T, et al. Psilocin, LSD, mescaline, and DOB all induce broadband desynchronization of EEG and disconnection in rats with robust translational validity. Transl Psychiatry. (2021) 11:506. doi: 10.1038/s41398-021-01603-4
92. Swanson L. Unifying theories of psychedelic drug effects. Front Pharmacol. (2018) 9:172. doi: 10.3389/fphar.2018.00172
Keywords: psychedelics, hallucinogen, depression, psilocybin, connectivity, LSD, ayahuasca, neurobiology
Citation: Husain MI, Ledwos N, Fellows E, Baer J, Rosenblat JD, Blumberger DM, Mulsant BH and Castle DJ (2023) Serotonergic psychedelics for depression: What do we know about neurobiological mechanisms of action? Front. Psychiatry 13:1076459. doi: 10.3389/fpsyt.2022.1076459
Received: 21 October 2022; Accepted: 19 December 2022;
Published: 10 February 2023.
Edited by:
Georgios Mikellides, University of Nicosia, CyprusReviewed by:
Dhakshin Ramanathan, University of California, San Diego, United StatesCopyright © 2023 Husain, Ledwos, Fellows, Baer, Rosenblat, Blumberger, Mulsant and Castle. This is an open-access article distributed under the terms of the Creative Commons Attribution License (CC BY). The use, distribution or reproduction in other forums is permitted, provided the original author(s) and the copyright owner(s) are credited and that the original publication in this journal is cited, in accordance with accepted academic practice. No use, distribution or reproduction is permitted which does not comply with these terms.
*Correspondence: Muhammad Ishrat Husain, aXNocmF0Lmh1c2FpbkBjYW1oLmNh
Disclaimer: All claims expressed in this article are solely those of the authors and do not necessarily represent those of their affiliated organizations, or those of the publisher, the editors and the reviewers. Any product that may be evaluated in this article or claim that may be made by its manufacturer is not guaranteed or endorsed by the publisher.
Research integrity at Frontiers
Learn more about the work of our research integrity team to safeguard the quality of each article we publish.