- 1Institute of Innovation and Applied Research in Chinese Medicine, Hunan University of Chinese Medicine, Changsha, China
- 2Department of Scientific Research, The First Hospital of Hunan University of Chinese Medicine, Changsha, China
- 3Hunan Provincial Key Laboratory for the Prevention and Treatment of Depressive Diseases with Traditional Chinese Medicine, Changsha, China
- 4Hunan Key Laboratory of Power and Innovative Drugs State Key Laboratory of Ministry Training Bases, Changsha, China
Depressive Disorder is a common mood disorder or affective disorder that is dominated by depressed mood. It is characterized by a high incidence and recurrence. The onset of depression is related to genetic, biological and psychosocial factors. However, the pathogenesis is still unclear. In recent years, there has been an increasing amount of research on the inflammatory hypothesis of depression, in which cyclo-oxygen-ase 2 (COX-2), a pro-inflammatory cytokine, is closely associated with depression. A variety of chemical drugs and natural products have been found to exert therapeutic effects by modulating COX-2 levels. This paper summarizes the relationship between COX-2 and depression in terms of neuroinflammation, intestinal flora, neurotransmitters, HPA axis, mitochondrial dysfunction and hippocampal neuronal damage, which can provide a reference for further preventive control, clinical treatment and scientific research on depression.
Introduction
Depression is a mental disorder. It is often characterized by depressed mood, loss of interest, decreased energy, self-denial, diminished ability to think, and sleep disturbances, etc. According to statistics, 350 million people worldwide suffer from varying degrees of depression, and more than 1 million people commit suicide each year because of depression (1). The pathogenesis of depression is complex, and current pathogenesis includes the monoamine hypothesis, changes in the hypothalamic-pituitary-adrenal axis, the inflammation hypothesis, neuroplasticity and neurogenesis, changes in brain structure and function, and the interaction and influence of genes and the environment (2). Recent studies have revealed the important role of the inflammation hypothesis, especially neuroinflammation, in the pathogenesis of depression (3).
Cyclo-oxygen-ase (COX) is an inflammatory mediator that regulates the inflammatory response and a key rate-limiting enzyme in the initial steps of prostaglandin (PGs) synthesis (4). PGs have a central role in the induction of the inflammatory response and can be synthesized in inflamed and damaged tissues, thus they are associated with the development of acute inflammatory swelling and painful symptoms (5). Among them, PGI2 and PGE2 are involved in vascular permeability, tissue swelling and gastric mucus secretion, which are typical symptoms of inflammation (6). COX has three isoforms, COX-1, COX-2 and COX-3 (6), of which COX-2 is usually induced by inflammatory stimuli in most tissues and is the only isoform responsible for propagating the inflammatory response. Current studies have demonstrated that COX-2 activation is an important factor mediating the development of depression (7, 8). Here, the present study summarizes recent research advances to further describe the link between depression and COX-2, aiming to provide new therapeutic ideas for the treatment of depression.
Cyclo-oxygen-ase-2 roles in the pathogenesis of depression
Neuroinflammation
Inflammation is a defense response of the immune system against tissue damage caused by inflammatory factors such as biological, physical, or chemical factors, foreign bodies, or necrotic tissue. One of the inflammatory responses in the brain is called “neuroinflammation.” Neuroinflammation is a protective response of the central nervous system (CNS), which is composed of neurons, microglia, oligodendrocytes and astrocytes. The entry of any foreign pathogen activates glial cells (astrocytes and microglia) and the over-activation of these cells triggers the release of various neuroinflammatory markers (NM), such as tumor necrosis factor-α (TNF-α), interleukin-1β (IL- 1β), interleukin-10 (IL-10), nitric oxide (NO), COX-2, etc. Various studies have shown the importance of neuroinflammatory markers in the development, diagnosis and treatment of depression (9–11).
In recent years, an increasing number of scholars have found that neuroinflammation is an important pathological factor triggering central neurological disorders such as depression, and inhibiting central neuroinflammation is a key way to effectively alleviate the onset and progression of depression (12–16). It was found that upregulation of pro-inflammatory cytokines (IL-6 and TNF-α) was seen in the hippocampus and cortex of Lipopolysaccharide (LSP)-induced depressed mice (17). Inflammatory cytokines in the blood can activate the hypothalamic-pituitary-adrenal (HPA) axis, and HPA dysfunction leads to corticotropin releasing hormone (CRH), adrenocorticotropic hormone (ACTH), increased secretion of corticosterone (CORT) and its negative feedback dysfunction, upregulate the release of glucocorticoids(GC) and cortisol in the adrenal cortex as well as cause dysregulation of monoamine transmitters, while inflammatory factors decrease the release of glucocorticoids and cortisol through activation of indoleamine-2,3-dioxygenase (IDO) enzyme, IDO activation reduces tryptophan and increases toxic metabolites of the kynurenine pathway, which leads to damage to astrocytes, microglia, and neurons, triggering neuroinflammation, which is positively associated with Major Depressive Disorder (MDD) (18–20). In addition, inflammatory factors can also induce damage to the blood-brain barrier (BBB) and enter the brain (21, 22). Damage to the hippocampal BBB, an important portal for maintaining a homeostatic environment for neurons and glial cells, would allow increased permeability and trigger neuroinflammation (23), which may be an important pathological factor in triggering depression (24). COX-2 is expressed in the central nervous system and is associated with neuroinflammation (25). Prabhakaran et al. used PET imaging to directly measure brain COX-2 levels, which showed that COX-2 can be induced to be upregulated during neuroinflammation, causing elevated COX-2 levels in vivo (26). The anti-neuroinflammatory effect is mediated by modulation of COX-2 levels to produce PGE2. The novel GPR55 receptor antagonist KIT10 may reduce neuroinflammation in microglia by inhibiting COX-2/PGE2. It has been shown that KIT10 inhibits the onset of depression by inhibiting protein synthesis of mPGES-1 and COX-2 and reducing PGE2 levels (26, 27). Neuroinflammation can mediate the onset of depression by affecting the reduction of monoamine neurotransmitters or changes in the number and sensitivity of their receptors. Venlafaxine, a serotonin-norepinephrine reuptake inhibitor, is a clinical agent used in the treatment of depression. It has anti-injurious and anti-inflammatory activity by inhibiting pro-inflammatory cytokines, and studies have shown that venlafaxine significantly reduced the mRNA expression of TNF-α, IL-6, IL-1β, and COX-2 (28) (Figure 1).
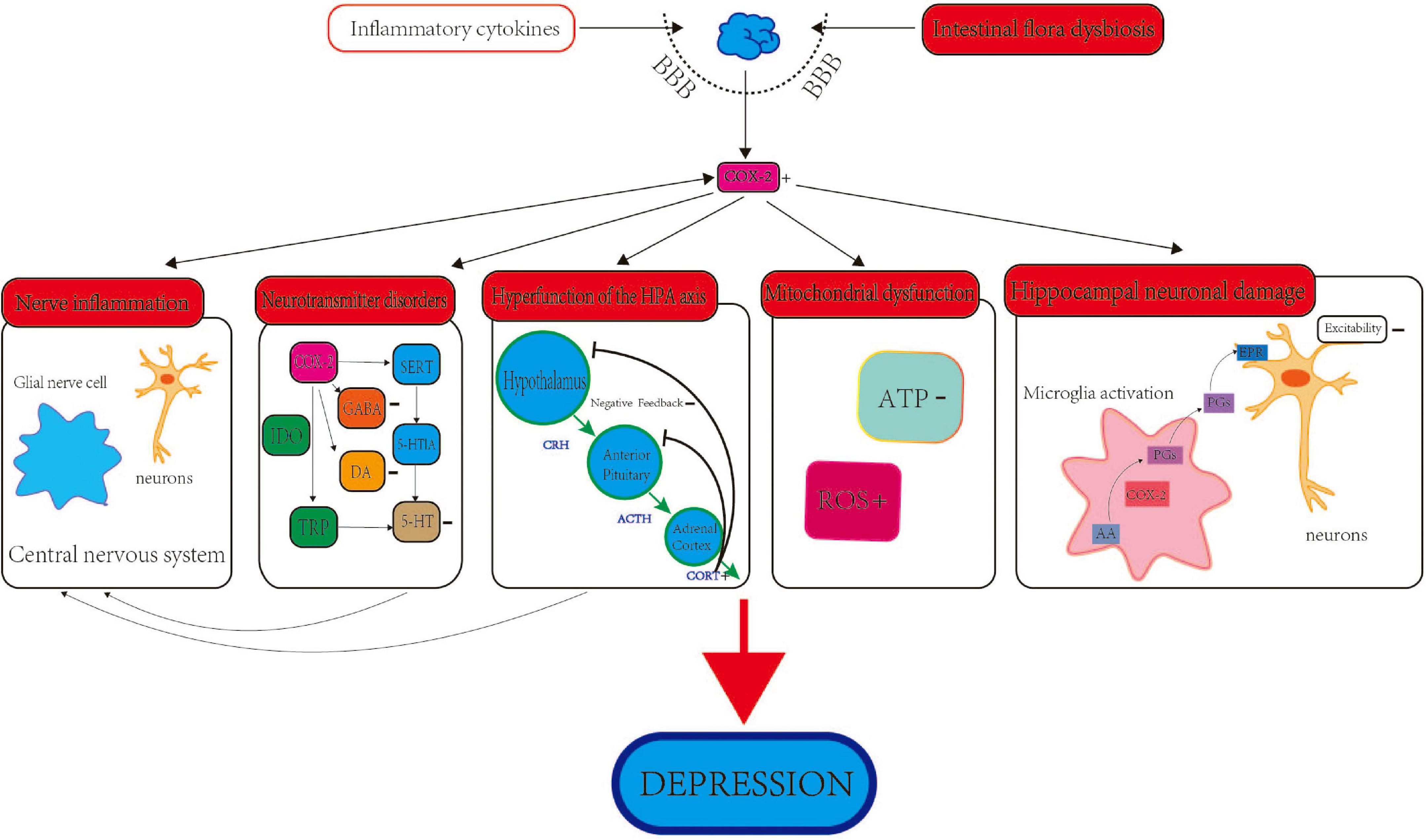
Figure 1. The role and mechanism of COX-2 in depression. The role of COX-2 and depression involves several aspects, including neuroinflammation, intestinal flora, neurotransmitters, HPA axis, deregulation of mitochondrial functions and neuronal damage of hippocampus.
Intestinal flora dysbiosis
Intestinal flora are normal microorganisms that reside in the human gastrointestinal tract for a long time and exist on the surface of the intestinal mucosal layer and in the intestinal lumen. These symbiotic flora synthesize a variety of vitamins essential for human growth and development, and also use protein residues to synthesize essential amino acids and participate in the metabolism of sugars and proteins, as well as promote the absorption of minerals such as iron, magnesium, and zinc and metabolize toxins (29). The brain and the gut are closely linked and various aspects of brain development, function, mood and cognition may be influenced by the symbiotic flora (30). The gut microbiota and CNS are connected through multiple bidirectional pathways involving neural, endocrine, and immune signaling, and dysbiosis of the gut flora can cause psychiatric disorders, such as depression (31–33). Current studies suggest that intestinal flora dysbiosis can mediate depression by causing an inflammatory response (34, 35). Inflammatory bowel syndrome (IBS) is a chronic, debilitating functional gastrointestinal disorder in which patients have impaired intestinal blood barrier integrity and leaky gut occurs, leading to the entry of intestinal contents such as immune cells and microbiota into the bloodstream, resulting in low-grade systemic inflammation, and circulating inflammation-related cytokines disrupt the blood-brain barrier, permitting infiltration of peripheral immune cells into the brain, leading to central inflammation in the brain, which central inflammation is associated with depression (36). Liu et al. (37) gave Chronic Unpredicted Mild Stress (CUMS) model rats Zhi Zhi Tang (ZZCD) for intervention and found that ZZCD promotes butyrate production by modulating the gut microbiota, and butyrate acts on the gut-brain axis, thereby It was found that ZZCD promotes butyrate production by regulating intestinal microbiota, and butyrate acts on the gut-brain axis, thus further regulating inflammation, neurotransmitters, endocrine and brain-derived neurotrophic factor (BDNF) to achieve antidepressant effects.
Intestinal barrier dysfunction is accompanied by increased intestinal permeability, permitting translocation of bacteria or their cell wall component LPS to the bloodstream, leading to persistent systemic inflammation (38). LPS is commonly used for modeling animal models of depression (17, 39). Rubab et al. (40) developed curcumin- nanostructured lipid carriers CUR-NLCs carriers (CUR-NLCs) and replicated the depression and anxiety model with LPS to study their neuroprotective effects in this model, and from histological and immunohistochemical analyses, CUR-NLCs improved brain tissue structure, inhibited the expression of p-NF-κB, TNF-α, and COX-2 in brain tissue, and enhanced the neuroprotective effects of curcumin, which could be used as a treatment for depression and anxiety as a potential drug. Natural drug substances can improve the levels of oxidative stress and neuroinflammation in depression, Muhammad et al. (41) used carvacrol to treat rats with depressive-like behavior induced by LPS, and significant neuronal alterations as well as elevated levels of inflammatory cytokines, such as TNF-α, COX-2 and c-Jun N-terminal kinase (p JNK). lPS stimulates microglia activation and inflammatory responses and upregulates mRNA and protein expression of inflammatory mediators such as COX-2 (42, 43). These inflammatory mediators can further mediate changes in the intestinal flora and disturbances in their metabolites, affecting brain function and depression. Estrogen deficiency can cause depression-like behavior, and Huang et al. (44) found in their experiments that estrogen deficiency-induced depression was associated with intestinal flora imbalance and inflammatory response in mice undergoing ovariectomy (OVX), where intestinal flora imbalance caused leaky gut and central inflammation (downregulation of COX-2 expression) to suppress BDNF expression in hippocampal neurogenesis, resulting in depressive behavior in OVX mice. BDNF is a neuroprotective molecule that plays a key role in neuroplasticity, neuroinflammation, learning and memory (45). There is growing evidence that modulation of BDNF expression levels can exert antidepressant effects (46–48), where BDNF-cAMP response element binding protein (CREB) signaling is one of the most attractive signaling pathways for the treatment of depression (49, 50). In summary, gut flora and COX-2-mediated inflammatory responses are strongly correlated, but there are fewer studies on how COX-2-mediated pathways and gut flora-brain centers interact and thus mediate depression, and further research is needed (Figure 1).
Neurotransmitter disorders
To date, neurotransmitter dysregulation has an important role in the pathogenesis of depression. Among these, the traditional monoaminergic theory (serotonergic, noradrenergic and dopaminergic dysregulation) has received the most attention in the treatment of depression, and most of the available antidepressants target these monoaminergic systems, such as serotonin and/or noradrenergic reuptake inhibitors and antipsychotic compounds that antagonize dopamine D2 (51). However, the exact pathophysiological mechanisms underlying serotonergic, noradrenergic, and dopaminergic dysfunction in depression remain unclear, and it has been hypothesized that inflammatory mechanisms may be involved in the pathogenesis of depression.
5-hydroxytryptamine (5-HT) is a monoamine neurotransmitter, which is mainly distributed in the pineal gland and hypothalamus, and abnormalities in 5-HT levels and functions in the central nervous system may be associated with the development of various disorders such as psychosis and migraine. Studies have shown that 5-HT and the onset of depression are closely related (52, 53). Overproduction of inflammatory cytokines affects the function of the 5-HT system by inhibiting cellular inflammatory factors thereby decreasing IDO activity. IDO degrades tryptophan (TRP) in the blood, a precursor of 5-HT, and its decrease reduces 5-HT synthesis, therefore, increasing 5-HT synthesis by inhibiting the IDO/5-HT/TRP signaling pathway can promote and improve hippocampal cognitive dysfunction and exert anti-inflammatory and antidepressant effects (54, 55). COX-2, as an inflammatory cytokine, inhibition of COX-2 expression reduces oxidative stress, inflammatory factor release, upregulates 5-HTlA receptors in hippocampal tissue, ameliorates cortical inflammatory response damage, reestablishes monoamine transmitter system homeostasis, and attenuates depression-like behavior (56). COX-2 overexpression leads to an increase in the pro-inflammatory cytokines IL-1β, IL-6 and TNF, which increases the activity of the 5-HT transporter protein SERT, increases the affinity of the 5-HT receptor 5-HT1A, and mediates depression through an IDO mechanism that alters tryptophan metabolism (57). Su et al. (58) found that the acidic sphingomyelinase inhibitor acidic sphingomyelinase inhibitors blocked interferon-α-induced 5-HT uptake in T cells through a COX-2/Akt/ERK/stat-dependent pathway. Clinical trials and experimental studies have demonstrated that COX-2 inhibitors can produce adjuvant antidepressant therapeutic effects against selective 5-HT reuptake inhibitors (59), while chronic selective 5-HT reuptake inhibition improves the endothelium-dependent hyperpolarization-like pathway of the small arteries in the fraction of endothelial function and COX-2 levels, modulating endothelial dysfunction and oxidative status in a chronic mild stress model in depressed rats (60).
In addition to the monoamine transmitter 5-HT, the functions of other brain neurotransmitters such as histamine (HA), dopamine (DA), acetylcholine (ACh), gamma-aminobutyric acid (GABA), and glutamate are thought to be closely related to various brain disorders such as depression, schizophrenia, and anxiety disorders. Venkatachalam et al. (61) found that histamine H3R and dopamine D2R/D3R antagonist, ST-713, improved autistic-like behavior in BTBR T + tf/J mice by attenuating the protein expression levels of NF-κB p65, COX-2 and iNOS in the hippocampus and cerebellum of BTBR mice. Klawonn et al. (62) found that the COX-2/activation of the PGs/EPR pathway increased neuronal peak potential spacing, decreased membrane electrical resistance and posterior hyperpolarization (fAHP), and simultaneously inhibited the GABA and DA signaling pathways, which in turn inhibited DA transmitter production and transmission, thereby triggering negative mood and depression (61). Emerging preclinical and clinical findings suggest that maladaptive glutamatergic neurotransmission may underlie the pathophysiology of MDD and that the neurobiological mechanisms of stress-induced impairment of AMPA (α-amino-3-hydroxy-5-methyl-4-isoxazolepropionic acid)-glutamatergic neurotransmission in the brain may provide new insights into the pathogenesis of MDD (51). In summary, inhibition of the pro-inflammatory cytokine COX-2 in the inflammatory response has an effect on CNS and 5-HT, HA, GABA, DA and glutamatergic neurotransmission, further affecting the onset of depression, and this effect needs to be further evaluated, which should include clinical trials in a larger number of patients (Figure 1).
Hyperfunction of the hypothalamic-pituitary-adrenal axis
The hypothalamic-pituitary-adrenal axis (HPA) axis is an important component of neuroendocrinology, and patients with depression often show hyperfunction of the HPA axis (63). Stress leads to hyperactivation of the HPA axis and disruption of the end product of HPA axis secretion, GC, which acts on glucocorticoid receptors (GR) in the hippocampus and hypothalamus, among other sites, after crossing the blood-brain barrier, causing disruption of the negative feedback regulation of the HPA axis, which in turn mediates the onset of depression (64–67). It has been shown that glucocorticoids and inflammatory responses are closely related and synthetic glucocorticoids (GCs) are widely used in a variety of inflammatory diseases, and the anti-inflammatory effects of GCs are achieved in part through the inhibition of a large number of pro-inflammatory cytokines (68, 69). Among them, GC correlate with the expression levels of the pro-inflammatory cytokine COX-2 (70, 71). The HPA axis acts as a negative feedback loop in which: stressors cause CRH production in the hypothalamus; CRH causes ACTH secretion in the pituitary; ACTH stimulates cortisol secretion in the adrenal cortex; and cortisol reversibly inhibits corticosteroid secretion (72). It has been shown that the HPA axis maintains the balance between cortisol and inflammation by regulating GR, and stress activates pro-inflammatory cytokines such as COX-2, which further stimulates the HPA axis to secrete cortisol (73), leading to depression (74, 75). overexpression of COX-2 leads to increased synthesis of prostaglandins, which in turn increases tissue sensitivity to catecholamines. stimulates the activity of the HPA axis through pro-adrenocorticotropin-releasing factors and leads to a surge in the production of pro-inflammatory cytokines (57). Acupuncture can exert anti-inflammatory effects through the HPA axis in order to reduce COX-2) and PGE2) levels and enhance the sympathetic nervous system (76). Chronic administration of corticosterone (CORT) in rodents is used to mimic stress-related dysregulation of the HPA axis and is a well-recognized approach in depression modeling, and Kv et al. (77) administered corticosterone to mice to replicate a depression model in which the pathogenesis of depression is increased levels of pro-inflammatory cytokines such as COX-2, which promotes GC resistance due to an overactive HPA axis, thereby increasing susceptibility to inflammatory responses that further leading to the development of depression. Therefore, COX-2 overexpression can affect the level of glucocorticoid and its receptor-mediated HPA axis function, which leads to the development of depression (Figure 1).
Mitochondrial dysfunction
As an energy-supplying organelle of the body, mitochondria have numerous physiological functions such as synthesizing ATP, providing energy to cells, participating in the tricarboxylic acid cycle, oxidizing phosphate, and storing calcium ions. Studies have reported that inflammation negatively affects mitochondria, leading to excitotoxicity, oxidative stress, and energy deficit, and that dysfunctional mitochondria may drive or propagate the inflammatory response, thus creating a vicious cycle (78). Numerous basic studies in recent years have found that the onset of depression is associated with mitochondrial dysfunction in brain regions (79, 80). Mitochondrial dysfunction includes reduced ATP synthesis, oxidative respiratory chain dysfunction, abnormal mitochondrial structure and excessive occurrence of apoptosis (81).
It has been found that reduced mitochondrial function as well as impaired oxidative metabolism and COX expression levels in hippocampal tissue are closely linked, and the mechanism of action may be related to reduced mitochondrial membrane potential and cell death due to reduced energy production (82, 83). Metabolomic and proteomic analyses further revealed that disruption of mitochondrial energy metabolism is one of the pathophysiological symptoms of depression, and that changes in subunit proteins of cytochrome c, ATP synthase or NADH dehydrogenase and a decrease in oxidative phosphorylation due to reduced electron transport chain activity in chronic mild stress (CMS) model rats stimulate biochemical dysregulation in the process of ATP production (84). Wang et al. (85), based on the theory that mitochondrial dysfunction mediates the generation of depression, hypothesized that healthy mitochondrial transplantation could improve depressive symptoms, and their study demonstrated for the first time that mitochondrial transplantation produces antidepressant-like effects in lipopolysaccharide-induced depression. Isolated mitochondria significantly reduced hippocampal astrocyte and microglia activation and neuroinflammation (i.e., 1L-1β, TNF-α, and COX-2), increased BDNF expression, and restored ATP production dysfunction and oxidative stress in LPS-induced depression. Furthermore, impaired synaptic mitochondrial function in the hippocampus and amygdala has a key role in the pathophysiological impairment of depression, and scholars have studied the potential dysregulation of synaptic mitochondrial proteins in the hippocampus and amygdala of CMS rats based on proteomics coupled with mass spectrometry, respectively, and these proteins are associated with impaired oxidative phosphorylation of synaptic mitochondria in the hippocampus and impaired glutamatergic transmission in the amygdala, which can lead to synaptic morphological and functional abnormalities and, therefore, synaptic mitochondria may be a key therapeutic target during depression (86, 87). Studies have shown that non-ylphenol (NP) exposure can induce altered synaptic morphology, reduce learning and memory capacity, and increase the incidence of depression in fetal rats. Xu Weihong et al. (88) and Angrand et al. (89) studied depression-like behavior in rats by NPonly or NP + high-fat diets and showed that exposure to NP only or both NP and high-fat diets can lead to mitochondrial damage and dysfunction in hippocampal neurons. affecting synaptic morphological plasticity and COX-2 expression in the hippocampus, further leading to the development of depression. Therefore, disruption of mitochondrial energy metabolism, impairment of synaptic plasticity and altered COX-2 levels are key causes of the development of depression.
The occurrence of depression is associated with stressful stressful events, and stress leads to a disturbance in the redox homeostasis of the body (brain tissue is the main target), producing a state of oxidative stress, which is manifested by increased levels of reactive oxygen species (ROS) clusters in the body. Mitochondria are the main organelle for ROS production (90, 91) and an antioxidant defense system exists in mitochondria to repair oxidative damage, which helps to maintain cellular integrity. Under normal conditions, several antioxidant enzymes such as superoxide dismutase (SOD), catalase (CAT), glutathione peroxidise (GPx), and glutathione reductase (GR) maintain the physiological levels of ROS. However, when stimulated by external stresses such as stress, the mitochondrial redox buffering system is disrupted and oxidative stress occurs (92, 93). High levels of ROS are one of the hallmarks of mitochondrial oxidative stress. NADPH oxidase is an electron transport membrane protein that is mainly responsible for ROS production (94) and contributes to ROS production and COX/PGE expression in neuropsychiatric disorders (95). Inhibition of microglia NADPH oxidase activation and nitric oxide synthase reduces ROS and RNS production and exerts neuroprotective effects by ameliorating oxidative stress thereby achieving a therapeutic effect on depression (93). And studies have found that neuroinflammation, common in the brains of depressed patients, has a strong impact on ROS production, and there is evidence that inflammatory factors increase the risk of depression (96). Among these, there is a positive correlation between the expression level of the inflammatory factor COX-2 and ROS concentration (97–99). An increasing number of studies have shown that inflammation leads to increased ROS production and that mitochondrial oxidative stress plays an important role in the pathophysiology of depression (78, 100). In summary, mitochondrial dysfunction is one of the pathogenic mechanisms of depression, and mitochondrial dysfunction can affect COX-2 levels, which in turn affects depression by mediating neuroinflammation in the brain. In addition, studying the interaction between COX-2 and mitochondria facilitates the study of the mechanisms of neuroinflammatory response to depression (Figure 1).
Hippocampal neuronal damage
Hippocampal microglia (MG) are the first line of defense of the CNS and are intrinsic immune cells in the brain, accounting for 5–12% of CNS cells, which regulate neuronal cell viability and dendritic spine density (101), provide neurological protection, and address inflammation through phagocytosis and tissue repair (102). Under normal conditions, MG is in a “resting” state and performs its immunosurveillance role. In contrast, changes in the cellular environment (e.g., oxidative stress leading to MG activation) alter its cellular morphology and function, and affect neuronal cell viability, function, and synaptic plasticity through exocytosis of inflammatory and other cytokines (103). MG activation is an important marker of hippocampal neuronal injury in depression.
Hippocampal neuronal damage is an important etiology mediating cognitive dysfunction and depression-like behavior (104). It has been reported that inflammation can induce neuronal apoptosis, impairment of synaptic plasticity and reduced excitability, among other impairments. As a typical pro-inflammatory factor, IL-1β induces neuronal apoptosis through the STAT3 pathway (105), while TNF-α and NO cause impaired neuronal viability through activation of the NF-κB pathway (106). Conversely, inhibiting the production of inflammatory factors or increasing the secretion of anti-inflammatory factors helps to maintain neuronal cell activity (107). In addition, inflammatory factors impair neuronal synaptic plasticity and thus cause abnormal neuronal function (108), e.g., IL-6 reduces neuronal synaptic plasticity and accelerates depression (109). MG is an important source of COX-2, and Strekalova et al. (57) found an increase in the number of iba-1 positive cells in the hippocampal region and a decrease in the number of ki67 positive cells in the granular subregion of depressed mice. positive cells, suggesting that MG activation in depressed mice inhibits cell proliferation, which in turn leads to overexpression of COX-2 in hippocampal CA1 areas as well as in dentate gyrus neurons, and that these changes mediate susceptibility to stress-induced lack of pleasure (a core symptom of depression). Recent studies have shown that activation of MG promotes the synthesis of more PGs by COX-2, which can act as a direct messenger factor between MG cells and neuronal cells (62). These PGs are secreted extracellularly by MG and further bind to prostaglandin receptors (EPR) on neuronal cell membranes, which in turn leads to decreased neuronal excitability and induces depression (61, 62, 110). It is thus evident that the inflammatory mediator CO can cause a decrease in neuronal excitability by mediating the synthesis of PGs, thus suggesting that inflammation is extensively involved in neuronal injury. Therefore, MG activation mediates COX-2/PGs pathway to decrease hippocampal neuronal excitability may be an important mechanism to trigger depression (62) (Figure 1).
Cyclo-oxygen-ase-2 inhibitors
Cyclo-oxygen-ase-2 (COX-2) can regulate the occurrence and development of depression. In recent years, COX-2 inhibitors have been widely used in the treatment of depressed patients, and the drugs commonly used in clinical practice as COX-2 inhibitors are non-steroidal anti-inflammatory drugs (NSAIDs), all of which have varying degrees of efficacy in depression (111–113). Some natural compounds such as xanthines, alkaloids, astragals, flavonoids, terpenoids and quinones also have the ability to inhibit COX-2 activity.
Non-steroidal anti-inflammatory agents
Cyclo-oxygen-ase-2 (COX-2) is widely involved in the inflammatory response and plays a crucial role in the inflammatory pathway, which can be improved by inhibiting it with NSAIDS, which can reduce the production of key pro-inflammatory mediators PG by inhibiting COX-1 and COX-2 (114). Long-term use of NSAIDS predisposes to gastrointestinal and cardiovascular diseases, which may be caused by gastric, renal, and platelet cell dysfunction due to COX-1 inhibition (115). Through research experts have found that selective COX-2 inhibitors have less side effects than non-selective COX-2 inhibitors. Among them, celecoxib is a selective COX-2 inhibitor, which is clinically the first-line drug in NSAID, Song et al. (116) found that CUMS exposure leads to increased COX-2 levels and that excess COX-2 converts arachidonic acid to PEG2 thereby leading to increased PEG2 expression, and that PEG2 production is associated with increased expression of the pro-inflammatory cytokines IL-1β, TNF a and IFN-γ (8), and celecoxib downregulates the production of these pro-inflammatory cytokines in the DG region, in addition to effectively attenuating mitochondrial ROS production and attenuating oxidative DNA oxidative damage, thereby potentially ameliorating the depressive phenotype resulting from neuroinflammation and apoptosis in the DG region of the hippocampus. Celecoxib also modulates COX-2 to improve indoleamine 2,3-dioxygenase activity, an enzyme that drives the metabolism of tryptophan and kynurenine in the CNS and degrades 5-HT (112, 117). The non-steroidal anti-inflammatory agent NS-398 is neuroprotective against excitotoxicity and neuroinflammatory injury, and it was found that NS-398 blocked the neuroinflammation-induced decrease in small albumin PVB in the prefrontal cortex reducing depressive effects (118). 5-LO, like COX-2, is a key enzyme in AA metabolism, and elevated levels of 5-LO and COX-2 expression accelerate inflammatory Inhibiting the inflammatory pathway arachidonic acid-cyclooxygenase-2/lipoxygenase (AA-COX-2/5-LO) pathway can regulate neurotransmitter metabolism, and the selective COX-2 inhibitor meloxicam is an inhibitor of this pathway, and Huang (119) gave meloxicam to CUMS rats, which improved cortical and hippocampal elevation of the biogenic amine neurotransmitters 5-HIAA, DOPAC and HVA in CUMS rats, which exert neuronal protective effects and inhibit inflammatory responses through inhibition of the AA/COX-2/5-LO pathway to achieve therapeutic effects in depression.
Diaryl-substituted heterocyclic compounds (e.g., thiazole, imidazole, triazole, oxazole, oxadiazole, pyrrole, thiophene) have been extensively studied as selective COX-2 inhibitors, and researchers have synthesized a series of COX-2 inhibitors with higher selectivity by improving the compounds, and thiazole derivatives can exert anti-inflammatory effects well as dual COX-2 and 5-LOX inhibitors. Zhang (120) synthesized a novel 5-aminothiazole ring-containing nuclear COX-2 inhibitor with higher anti-inflammatory effect than meloxicam. Hamoud MMS and other researchers (121) synthesized 1,3,4-oxadiazolyl derivatives (8a-g) and 1,2,4-triazolyl derivatives (10a,b and 11a-g), and through further experiments the newly designed compounds were found to have potent COX-2 inhibitory effects, possessing powerful with potent anti-inflammatory and antioxidant activities, capable of inhibiting TNF-α, NO and ROS production, and have the potential to be promising therapeutic agents for the treatment of inflammation-related psychiatric disorders. Novel chalcone compounds have excellent COX-2 inhibitory activity, can effectively suppress EGFR expression levels, and can overcome gastrointestinal side effects (122, 123). Novel COX-2 inhibitors 5-diaryl pyrrole nitrooxyethyl sulfide and related compounds, which could achieve potent anti-inflammatory effects with an overall safety profile and reduced gastrointestinal toxicity (124).
New targeted preparation
In addition to improving the structure of compounds, experts have also enhanced the effectiveness of drugs as well as attenuated their side effects by creating novel formulations. Schmied et al. (125) used novel cellulose-based particles as adsorbent carriers to prepare celecoxib solid self-nanoemulsifying drug delivery system (SNEDDS), SNEDDS can improve the oral bioavailability and stability of low-soluble lipophilic drugs, enhance the release of their active ingredients, and further develop controlled release dosage forms. Sipos et al. (126) combined the NSAIDs meloxicam with novel nanomedicines to develop mucosal adherent nanoemulsions containing meloxicam, which has advanced drug transportability and stability to further increase the absorption and bioavailability of meloxicam in humans to systemic administration and nasal-to-brain delivery. They performed cytotoxic effects of the formulation on an NIH/3T3 mouse embryonic fibroblast cell line and the results supported the safe nasal suitability of the novel agent. Long-term administration of NSAIDs has adverse effects such as gastrointestinal, cardiovascular function and to ameliorate this side effect, some researchers designed celecoxib into a celecoxib a microsphere-microcrystal-gel delivery system for intra-articular injection using an ultrasound approach (127). Thus, the use of novel agents can improve the bioavailability and stability of NSAIDs and also attenuate their toxic side effects, but there are fewer studies on the use of modified new dosage forms of NSAIDs for the treatment of depression and further in-depth studies are needed.
Natural products and their derivatives
Bangpungtongsung-san (BTS) is a Korean medicine with antidepressant effects consisting of 18 herbs. Park et al. (128) evaluated the antidepressant and anti-neuroinflammatory effects of BTS using an in vivo model of lisinopine-induced depression and an in vitro model of LPS-stimulated BV2 microglia. The results showed that BTS may exert antidepressant effects by affecting mood-related hormones, neuronal activity and neuroinflammation in lisinopine-induced depression in mice. In vitro experiments showed that BTS may achieve depression-inducing effects by downregulating Nos-2 and Cox-2 to inhibit NO production, stimulating pro-inflammatory cytokine production and inducing neuroinflammatory responses in microglia. Kaixin San (KXS) is a well-known herbal formula for treating depressed mood and improving various learning and memory disorders, which was found to have antidepressant effects in a previous study by Dong et al. (129). Further studies found that KXS can reduce glucocorticoid secretion by regulating the levels of serum cytokines (such as COX, IL-2, and TNF-α) while inhibiting HPA axis hyperactivity and inhibiting apoptosis and thus treating depression.
Studies have shown that herbal monomeric components have therapeutic effects on depression, and these monomeric components are the direct material basis for the efficacy of herbal medicines. Flavoprotein (FX) is an edible brown seaweed rich in natural carotenoids, and FX can prevent LPS-induced depression-like behavior in mice by modulating the AMPK-NF-κB signaling pathway and downregulating pro-inflammatory cytokines (IL-1β, IL-6, and TNF-α) as well as overexpression of iNOS and COX-2 in the hippocampus, frontal cortex, and hypothalamus (130). Dihydromyricetin (DMY) is an important flavonoid extracted from Daphyllostachys, and Wei et al. (131) found that DMY could inhibit neuroinflammation and improve LPS-induced depression-like behavior in mice by decreasing the secretion of pro-inflammatory factors TNFα, IL-6, IL-1β, COX-2, and iNOS in vivo. Trans-cinnamaldehyde (TCA) is an excellent CNS COX-2 inhibitor and is the main component of the traditional Chinese medicine Cinnamomum cassia. Lin et al. (132) constructed a depression model in BALB/c mice and gave TCA intervention treatment. TCA significantly decreased COX-2, TRPV1 and CB1 protein levels in the hippocampus, increased 5-HT levels in the hippocampus and decreased the Glu/GABA ratio. Studies have demonstrated that TCA has some effect in governing depression-like behavior, and these findings suggest that TCA treatment has antidepressant effects and that it mediates depression levels by mediating COX-2 levels and regulating neurotransmitters. Tao et al. (133) showed that luteolin containing PA methanolic extract mediates depression levels by balancing excitatory (glutamate) and inhibitory (GABA) brain monoamines, the voltage-gated ion channels (NaK/Ca-ATPase) and inhibition of NF-κB/TLR-4 pathway ameliorated neuroinflammation (TNF-α, IL-1β and COX-2) and improved seizure-complicated depression-like behavior in experimental epileptic mice. Catalpol, a highly active cyclic enol ether terpene glycoside, is a major constituent of Dictyostelium, which is rich, nutritious, and has excellent medicinal and nutritional value, and is reflected in classical clinical antidepressant formulas in Chinese medicine (134, 135). The antidepressant mechanism of catalpol involves ERK1/2/Nrf2 upregulation due to HO-1 activation and downregulation of factors related to the COX-2/iNOS/NO pathway suppressing neuroinflammation and triggering upregulation of the BDNF/TrkB pathway to enhance neurotrophy (136).
Conclusion and future directions
The activation of the inflammatory response system is an important mechanism in the pathogenesis of depression, and patients with depression are associated with increased levels of central or peripheral inflammatory factors. COX is inextricably linked to the above hypothesis and pathological manifestations. However, the current research on COX-2 is mainly focused on tumor and cancer-related aspects, and the pathophysiological studies in central nervous system diseases such as depression are relatively weak. The evidence emphasizes that the inflammatory response mediated by COX-2 activation is a key pathological component of depression, and the use of COX-2 as a therapeutic target will become a new strategy for the prevention and treatment of depression in the future. Significantly improved depression in rats and mice, mainly by reducing the pro-inflammatory mediator PG and other pro-inflammatory factors associated with inflammation, thereby ameliorating neuroinflammation, intestinal flora disorders, neurotransmitter imbalance, mitochondrial dysfunction, and neuronal damage. In the pathogenesis of depression, these pathological links can in turn interact with each other to exacerbate the pathogenic process. Although animal and clinical experiments have shown positive effects of COX-2 inhibitors on these links, further experimental studies are needed to validate them in large clinical samples and to determine whether COX-2 inhibitors also exert antidepressant effects through other pathways. At present, the development of antidepressant applications of COX-2 inhibitors is still relatively weak. Clinically, some common COX-2 inhibitors can have side effects such as cardiovascular and gastrointestinal diseases after long-term use, thus, it is important to fully understand the role of COX-2 and its related pathways in the development of depression, and to use COX-2 inhibitors as lead compounds to synthesize compounds with high bioavailability, good stability performance, safety, and significant biological activity. This will provide a reliable basis for the development of antidepressant drugs by using COX-2 inhibitors as lead compounds to synthesize excellent compounds and dosage forms with high bioavailability, good stability, safety, and significant biological activity.
In recent years, scholars have discovered another novel isozyme of COX, which is a new structural isoform of COX derived from COX-1. Unlike COX-1, which is mainly found in vascular, gastric and renal tissues, COX-3 is expressed in the heart and brain, more in the brain, and it is widely involved in the anti-inflammatory pathway. the site of inhibition of inflammatory mediator PG by COX-3 is different, it mainly inhibits central nervous system sites and weakly inhibits peripheral PG synthesis, and one study found that COX-1 and COX-3 mRNA levels can induce depression by leading to impaired oxidative metabolism and reduced mitochondrial function (83). Thus, it is clear that COX-3 may be an important target for anti-inflammatory and antidepressant effects, but the related literature is poorly reported and needs to be explored in depth, and anti-inflammatory therapy against the target COX-3 would be another new avenue for depression treatment. In addition, different sites have different sensitivity to COX inhibitors. Hippocampus plays an important role in the development of depression, and hippocampal region is an important anatomical region associated with depression, while MG is the main source of COX, so the study of COX inhibitors in hippocampal MG may provide further new ideas and targets for the prevention and treatment of depression. Furthermore, the amygdala plays an important role in stress-related psychiatric disorders and may be affected in chronic stress (137, 138). Surprisingly, there is no information on the role of COX-2 in the amygdala in rodent studies, so further attention to COX-2 changes in the amygdala in rodent models could provide another new idea and target for the prevention and treatment of depression.
Author contributions
YH drafted the manuscript and collected important background information. YSH and XL analyzed data and document. MZ and YW reviewed and revised manuscript. All authors contributed to the article and approved the submitted version.
Funding
This work was supported by the National Natural Science Foundation of China (No. 82104846), the Natural Science Foundation of Hunan Province (Nos. 2022JJ40323 and 2022JJ80092), the Natural Science Foundation of Changsha (No. Kq2202266), and the Hunan Postgraduate Scientific Research Innovation Project (No. CX20220796).
Conflict of interest
The authors declare that the research was conducted in the absence of any commercial or financial relationships that could be construed as a potential conflict of interest.
Publisher’s note
All claims expressed in this article are solely those of the authors and do not necessarily represent those of their affiliated organizations, or those of the publisher, the editors and the reviewers. Any product that may be evaluated in this article, or claim that may be made by its manufacturer, is not guaranteed or endorsed by the publisher.
References
1. Bayes J, Schloss J, Sibbritt D. Effects of polyphenols in a mediterranean diet on symptoms of depression: a systematic literature review. Adv Nutr. (2020) 11:602–15. doi: 10.1093/advances/nmz117
3. Ali T, Rahman SU, Hao Q, Li W, Liu Z, Ali Shah F, et al. Melatonin prevents neuroinflammation and relieves depression by attenuating autophagy impairment through FOXO3a regulation. J Pineal Res. (2020) 69:e12667. doi: 10.1111/jpi.12667
4. Dagallier C, Avry F, Touchefeu Y, Buron F, Routier S, Chérel M, et al. Development of PET radioligands targeting COX-2 for colorectal cancer staging, a review of in vitro and preclinical imaging studies. Front Med. (2021) 8:675209. doi: 10.3389/fmed.2021.675209
5. Aryal B, Raut BK, Bhattarai S, Bhandari S, Tandan P, Gyawali K, et al. Potential therapeutic applications of plant-derived alkaloids against inflammatory and neurodegenerative diseases. Evid Based Complement Alternat Med. (2022) 2022:7299778. doi: 10.1155/2022/7299778
6. Ahmadi M, Bekeschus S, Weltmann KD, von Woedtke T, Wende K. Non-steroidal anti-inflammatory drugs: recent advances in the use of synthetic COX-2 inhibitors. RSC Med Chem. (2022) 13:471–96. doi: 10.1039/D1MD00280E
7. Fresta CG, Fidilio A, Lazzarino G, Musso N, Grasso M, Merlo S, et al. Modulation of pro-oxidant and pro-inflammatory activities of M1 macrophages by the natural dipeptide carnosine. Int J Mol Sci. (2020) 21:776. doi: 10.3390/ijms21030776
8. Halaris A, Sohl E, Whitham EA. Treatment-resistant depression revisited: a glimmer of hope. J Pers Med. (2021) 11:155. doi: 10.3390/jpm11020155
9. Apweiler M, Streyczek J, Saliba SW, Ditrich J, Muñoz E, Fiebich BL. Anti-inflammatory and anti-oxidative effects of AM404 in IL-1β-Stimulated SK-N-SH Neuroblastoma Cells. Front Pharmacol. (2021) 12:789074. doi: 10.3389/fphar.2021.789074
10. de Souza AG, Lopes IS, Filho A, Cavalcante TMB, Oliveira JVS, de Carvalho MAJ, et al. Neuroprotective effects of dimethyl fumarate against depression-like behaviors via astrocytes and microglia modulation in mice: possible involvement of the HCAR2/Nrf2 signaling pathway. Naunyn Schmiedebergs Arch Pharmacol. (2022) 395:1029–45. doi: 10.1007/s00210-022-02247-x
11. Sørensen NV, Orlovska-Waast S, Jeppesen R, Klein-Petersen AW, Christensen R, Benros ME. Neuroinflammatory biomarkers in cerebrospinal fluid from 106 patients with recent-onset depression compared with 106 individually matched healthy control subjects. Biol Psychiatry. (2022) 92:563–72. doi: 10.1016/j.biopsych.2022.04.002
12. Beurel E, Toups M, Nemeroff CB. The bidirectional relationship of depression and inflammation: double trouble. Neuron. (2020) 107:234–56. doi: 10.1016/j.neuron.2020.06.002
13. Benedetti F, Zanardi R, Mazza MG. Antidepressant psychopharmacology: is inflammation a future target. Int Clin Psychopharmacol. (2022) 37:79–81. doi: 10.1097/YIC.0000000000000403
14. Franklyn SI, Stewart J, Beaurepaire C, Thaw E, McQuaid RJ. Developing symptom clusters: linking inflammatory biomarkers to depressive symptom profiles. Transl Psychiatry. (2022) 12:133. doi: 10.1038/s41398-022-01900-6
15. Tayab MA, Islam MN, Chowdhury K, Tasnim FM. Targeting neuroinflammation by polyphenols: a promising therapeutic approach against inflammation-associated depression. Biomed Pharmacother. (2022) 147:112668. doi: 10.1016/j.biopha.2022.112668
16. Wan T, Li X, Fu M, Gao X, Li P, Guo W. NLRP3-dependent pyroptosis: a candidate therapeutic target for depression. Front Cell Neurosci. (2022) 16:863426. doi: 10.3389/fncel.2022.863426
17. Zhuo R, Cheng X, Luo L, Yang L, Zhao Y, Zhou Y, et al. Cinnamic acid improved lipopolysaccharide-induced depressive-like behaviors by inhibiting neuroinflammation and oxidative stress in mice. Pharmacology. (2022) 107:281–9. doi: 10.1159/000520990
18. Mingoti M, Bertollo AG, Simões J, Francisco GR, Bagatini MD, Ignácio ZM. COVID-19, oxidative stress, and neuroinflammation in the depression route. J Mol Neurosci. (2022) 72:1166–81. doi: 10.1007/s12031-022-02004-y
19. Pan X, Chen K, Han S, Luo X, Zhang D, Zhang H, et al. Total triterpenes of Wolfiporia cocos (Schwein.) ryvarden & gilb exerts antidepressant-like effects in a chronic unpredictable mild stress rat model and regulates the levels of neurotransmitters, HPA axis and NLRP3 pathway. Front Pharmacol. (2022) 13:793525. doi: 10.3389/fphar.2022.793525
20. Fischer S, Macare C, Cleare AJ. Hypothalamic-pituitary-adrenal (HPA) axis functioning as predictor of antidepressant response-Meta-analysis. Neurosci Biobehav Rev. (2017) 83:200–11. doi: 10.1016/j.neubiorev.2017.10.012
21. Zong C, Hasegawa R, Urushitani M, Zhang L, Nagashima D, Sakurai T, et al. Role of microglial activation and neuroinflammation in neurotoxicity of acrylamide in vivo and in vitro. Arch Toxicol. (2019) 93:2007–19. doi: 10.1007/s00204-019-02471-0
22. Hu J, Wang X, Chen X, Fang Y, Chen K, Peng W, et al. Hydroxychloroquine attenuates neuroinflammation following traumatic brain injury by regulating the TLR4/NF-κB signaling pathway. J Neuroinflammation. (2022) 19:71. doi: 10.1186/s12974-022-02430-0
23. Erickson MA, Dohi K, Banks WA. Neuroinflammation: a common pathway in CNS diseases as mediated at the blood-brain barrier. Neuroimmunomodulation. (2012) 19:121–30. doi: 10.1159/000330247
24. Greene C, Hanley N, Campbell M. Blood-brain barrier associated tight junction disruption is a hallmark feature of major psychiatric disorders. Transl Psychiatry. (2020) 10:373. doi: 10.1038/s41398-020-01054-3
25. Song Q, Fan C, Wang P, Li Y, Yang M, Yu SY. Hippocampal CA1 βCaMKII mediates neuroinflammatory responses via COX-2/PGE2 signaling pathways in depression. J Neuroinflammation. (2018) 15:338. doi: 10.1186/s12974-018-1377-0
26. Prabhakaran J, Molotkov A, Mintz A, Mann JJ. Progress in PET imaging of neuroinflammation targeting COX-2 enzyme. Molecules. (2021) 26:3208. doi: 10.3390/molecules26113208
27. Saliba SW, Gläser F, Deckers A, Keil A, Hurrle T, Apweiler M, et al. Effects of a novel GPR55 antagonist on the arachidonic acid cascade in LPS-activated primary microglial cells. Int J Mol Sci. (2021) 22:2503. doi: 10.3390/ijms22052503
28. Mahnashi MH, Jabbar Z, Alamgeer, Irfan HM, Asim MH, Akram M, et al. Venlafaxine demonstrated anti-arthritic activity possibly through down regulation of TNF-α, IL-6, IL-1β, and COX-2. Inflammopharmacology. (2021) 29:1413–25. doi: 10.1007/s10787-021-00849-0
29. Rinninella E, Raoul P, Cintoni M, Franceschi F, Miggiano GAD, Gasbarrini A, et al. What is the healthy gut microbiota composition? A changing ecosystem across age, environment, diet, and diseases. Microorganisms. (2019) 7:14. doi: 10.3390/microorganisms7010014
30. He Q, Si C, Sun Z, Chen Y, Zhang X. The intervention of prebiotics on depression via the gut-brain axis. Molecules. (2022) 273671. doi: 10.3390/molecules27123671
31. Cox LM, Weiner HL. Microbiota signaling pathways that influence neurologic disease. Neurotherapeutics. (2018) 15:135–45. doi: 10.1007/s13311-017-0598-8
32. Bhatt S, Kanoujia J, Mohanalakshmi S, Patil CR, Gupta G, Dua K, et al. Role of brain-gut-microbiota axis in depression: emerging therapeutic avenues. CNS Neurol Disord Drug Targets. (2022) [Online ahead of print]. doi: 10.2174/1871527321666220329140804
33. Wang P, Guo P, Wang Y, Teng X, Zhang H, Sun L, et al. Propolis ameliorates alcohol-induced depressive symptoms in C57BL/6J mice by regulating intestinal mucosal barrier function and inflammatory reaction. Nutrients. (2022) 14:1213. doi: 10.3390/nu14061213
34. Kang Y, Yang Y, Wang J, Ma Y, Cheng H, Wan D. Correlation between intestinal flora and serum inflammatory factors in post-stroke depression in ischemic stroke. J Coll Phys Surg Pak. (2021) 31:1224–7. doi: 10.29271/jcpsp.2021.10.1224
35. Bai S, Bai H, Li D, Zhong Q, Xie J, Chen JJ. Gut microbiota-related inflammation factors as a potential biomarker for diagnosing major depressive disorder. Front Cell Infect Microbiol. (2022) 12:831186. doi: 10.3389/fcimb.2022.831186
36. El-Hakim Y, Bake S, Mani KK, Sohrabji F. Impact of intestinal disorders on central and peripheral nervous system diseases. Neurobiol Dis. (2022) 165:105627. doi: 10.1016/j.nbd.2022.105627
37. Liu J, Fang Y, Cui L, Wang Z, Luo Y, Gao C, et al. Butyrate emerges as a crucial effector of Zhi-Zi-Chi decoctions to ameliorate depression via multiple pathways of brain-gut axis. Biomed Pharmacother. (2022) 149:112861. doi: 10.1016/j.biopha.2022.112861
38. de Waal GM, de Villiers W, Forgan T, Roberts T, Pretorius E. Colorectal cancer is associated with increased circulating lipopolysaccharide, inflammation and hypercoagulability. Sci Rep. (2020) 10:8777. doi: 10.1038/s41598-020-65324-2
39. Ramalho JB, Spiazzi CC, Bicca DF, Rodrigues JF, Sehn CP, da Silva WP, et al. Beneficial effects of Lactococcus lactis subsp. cremoris LL95 treatment in an LPS-induced depression-like model in mice. Behav Brain Res. (2022) 426:113847. doi: 10.1016/j.bbr.2022.113847
40. Rubab S, Naeem K, Rana I, Khan A, Afridi M, Ullah I, et al. Enhanced neuroprotective and antidepressant activity of curcumin-loaded nanostructured lipid carriers in lipopolysaccharide-induced depression and anxiety rat model. Int J Pharm. (2021) 603:120670. doi: 10.1016/j.ijpharm.2021.120670
41. Muhammad AJ, Hao L, Al Kury LT, Rehman NU, Alvi AM, Badshah H, et al. Carveol Promotes Nrf2 contribution in depressive disorders through an anti-inflammatory mechanism. Oxid Med Cell Longev. (2022) 2022:4509204. doi: 10.1155/2022/4509204
42. Dong J, Fu T, Yang Y, Mu Z, Li X. Long Noncoding RNA SNHG1 promotes lipopolysaccharide-induced activation and inflammation in microglia via targeting miR-181b. Neuroimmunomodulation. (2021) 28:255–65. doi: 10.1159/000514549
43. Guo C, Guo D, Fang L, Sang T, Wu J, Guo C, et al. Ganoderma lucidum polysaccharide modulates gut microbiota and immune cell function to inhibit inflammation and tumorigenesis in colon. Carbohydr Polym. (2021) 267:118231. doi: 10.1016/j.carbpol.2021.118231
44. Huang F, Liu X, Xu S, Hu S, Wang S, Shi D, et al. Prevotella histicola mitigated estrogen deficiency-induced depression via gut microbiota-dependent modulation of inflammation in Ovariectomized Mice. Front Nutr. (2021) 8:805465. doi: 10.3389/fnut.2021.805465
45. Keeler JL, Patsalos O, Chung R, Schmidt U, Breen G, Treasure J, et al. Short communication: serum levels of brain-derived neurotrophic factor and association with pro-inflammatory cytokines in acute and recovered anorexia nervosa. J Psychiatr Res. (2022) 150:34–9. doi: 10.1016/j.jpsychires.2022.03.031
46. Shi LS, Ji CH, Tang WQ, Liu Y, Zhang W, Guan W. Hippocampal miR-124 participates in the pathogenesis of depression via regulating the expression of BDNF in a Chronic Social Defeat Stress model of depression. Curr Neurovasc Res. (2022) [Online ahead of print]. doi: 10.2174/1567202619666220713105306
47. Xu J, Tang M, Wu X, Kong X, Liu Y, Xu X. Lactobacillus rhamnosus zz-1 exerts preventive effects on chronic unpredictable mild stress-induced depression in mice via regulating the intestinal microenvironment. Food Funct. (2022) 13:4331–43. doi: 10.1039/D1FO03804D
48. Zhang R, Li D, Mao H, Wei X, Xu MD, Zhang S, et al. Disruption of 5-hydroxytryptamine 1A receptor and orexin receptor 1 heterodimer formation affects novel G protein-dependent signaling pathways and has antidepressant effects in vivo. Transl Psychiatry. (2022) 12:122. doi: 10.1038/s41398-022-01886-1
49. Chen YM, Fan H, Huang J, Shi TS, Li WY, Wang CN, et al. Hippocampal F3/Contactin plays a role in chronic stress-induced depressive-like effects and the antidepressant actions of vortioxetine in mice. Biochem Pharmacol. (2022) 202:115097. doi: 10.1016/j.bcp.2022.115097
50. Ji CH, Gu JH, Liu Y, Tang WQ, Guan W, Huang J, et al. Hippocampal MSK1 regulates the behavioral and biological responses of mice to chronic social defeat stress: involving of the BDNF-CREB signaling and neurogenesis. Biochem Pharmacol. (2022) 195:114836. doi: 10.1016/j.bcp.2021.114836
51. Lee MT, Peng WH, Kan HW, Wu CC, Wang DW, Ho YC. Neurobiology of depression: chronic stress alters the glutamatergic system in the brain-focusing on AMPA receptor. Biomedicines. (2022) 10:1005. doi: 10.3390/biomedicines10051005
52. Tan Y, Xu P, Huang S, Yang G, Zhou F, He X, et al. Structural insights into the ligand binding and G(i) coupling of serotonin receptor 5-HT(5A). Cell Discov. (2022) 8:50. doi: 10.1038/s41421-022-00412-3
53. Venkatachalam K, Zhong S, Dubiel M, Satała G, Sadek B, Stark H. The novel pimavanserin derivative ST-2300 with histamine H(3) receptor affinity shows reduced 5-HT(2A) binding, but maintains antidepressant- and anxiolytic-like properties in mice. Biomolecules. (2022) 12:683. doi: 10.3390/biom12050683
54. Lv Z, Yan L, Yang S, Yang Y. Effect of electroacupuncture “Xie Yu Fang” on serum levels of indoleamine 2,3 dioxygenase, 5-hydroxytryptamine and interleukin 10 in a rat model of depression. J Shanxi Univ Tradit Chin Med. (2020) 21:172–5.
55. Liu RL, Qu HL. Aerobic exercise intervenes in hippocampal neuroinflammation and improves the regulatory mechanism of NF-κB and TNF-α/IDO/5-HT signaling pathways in CUMS mice. Chin J Immunol. (2021) 37:1563–70.
56. Kuang S-N, Luo Y, Tian S-Y, Zhang L, Yang Y, Yang J-Q. A preliminary investigation on the mechanism of meloxicam to improve depressive behavior in chronically stressed rats. Chin Pharmacol Bull. (2016) :263–7.
57. Strekalova T, Pavlov D, Trofimov A, Anthony DC, Svistunov A, Proshin A, et al. Hippocampal over-expression of cyclooxygenase-2 (COX-2) is associated with susceptibility to stress-induced anhedonia in mice. Int J Mol Sci. (2022) 23:2061. doi: 10.3390/ijms23042061
58. Su HC, Ma CT, Lin CF, Wu HT, Chuang YH, Chen LJ, et al. The acid sphingomyelinase inhibitors block interferon-α-induced serotonin uptake via a COX-2/Akt/ERK/STAT-dependent pathway in T cells. Int Immunopharmacol. (2011) 11:1823–31. doi: 10.1016/j.intimp.2011.07.011
59. Köhler O, Petersen L, Mors O, Gasse C. Inflammation and depression: combined use of selective serotonin reuptake inhibitors and NSAIDs or paracetamol and psychiatric outcomes. Brain Behav. (2015) 5:e00338. doi: 10.1002/brb3.338
60. Matchkov VV, Kravtsova VV, Wiborg O, Aalkjaer C, Bouzinova EV. Chronic selective serotonin reuptake inhibition modulates endothelial dysfunction and oxidative state in rat chronic mild stress model of depression. Am J Physiol Regul Integr Comp Physiol. (2015) 309:R814–23. doi: 10.1152/ajpregu.00337.2014
61. Fritz M, Klawonn AM, Nilsson A, Singh AK, Zajdel J, Wilhelms DB, et al. Prostaglandin-dependent modulation of dopaminergic neurotransmission elicits inflammation-induced aversion in mice. J Clin Invest. (2016) 126:695–705. doi: 10.1172/JCI83844
62. Klawonn AM, Fritz M, Castany S, Pignatelli M, Canal C, Similä F, et al. Microglial activation elicits a negative affective state through prostaglandin-mediated modulation of striatal neurons. Immunity. (2021) 54:225–234.e6. doi: 10.1016/j.immuni.2020.12.016
63. Liu Z, Zou Y, He M, Yang P, Qu X, Xu L. Hydroxysafflor yellow A can improve depressive behavior by inhibiting hippocampal inflammation and oxidative stress through regulating HPA axis. J Biosci. (2022) 47:7. doi: 10.1007/s12038-021-00246-3
64. Juruena MF, Bourne M, Young AH, Cleare AJ. Hypothalamic-pituitary-adrenal axis dysfunction by early life stress. Neurosci Lett. (2021) 759:136037. doi: 10.1016/j.neulet.2021.136037
65. Chen S, Tang Y, Gao Y, Nie X, Wang H, Su H, et al. Antidepressant potential of quercetin and its glycoside derivatives: a comprehensive review and update. Front Pharmacol. (2022) 13:865376. doi: 10.3389/fphar.2022.865376
66. Kyle CJ, Nixon M, Homer N, Morgan RA, Andrew R, Stimson RH, et al. ABCC1 modulates negative feedback control of the hypothalamic-pituitary-adrenal axis in vivo in humans. Metabolism. (2022) 128:155118. doi: 10.1016/j.metabol.2021.155118
67. Lupien SJ, Leclaire S, Majeur D, Raymond C, Jean Baptiste F, Giguère CE. ‘Doctor, I am so stressed out!’ A descriptive study of biological, psychological, and socioemotional markers of stress in individuals who self-identify as being ‘very stressed out’ or ‘zen’. Neurobiol Stress. (2022) 18:100454. doi: 10.1016/j.ynstr.2022.100454
68. Lunin SM, Novoselova EG, Glushkova OV, Parfenyuk SB, Novoselova TV, Khrenov MO. Cell senescence and central regulators of immune response. Int J Mol Sci. (2022) 23:4109. doi: 10.3390/ijms23084109
69. Pang JP, Hu XP, Wang YX, Liao JN, Chai X, Wang XW, et al. Discovery of a novel nonsteroidal selective glucocorticoid receptor modulator by virtual screening and bioassays. Acta Pharmacol Sin. (2022) 43:2429–38. doi: 10.1038/s41401-021-00855-6
70. Abul M, Al-Bader MD, Mouihate A. Prenatal activation of glucocorticoid receptors induces memory impairment in a sex-dependent manner: role of cyclooxygenase-2. Mol Neurobiol. (2022) 59:3767–77. doi: 10.1007/s12035-022-02820-8
71. Raafat M, Kamel AA, Shehata AH, Ahmed AF, Bayoumi AMA, Moussa RA, et al. Aescin protects against experimental benign prostatic hyperplasia and preserves prostate histomorphology in rats via suppression of inflammatory cytokines and COX-2. Pharmaceuticals. (2022) 15:130. doi: 10.3390/ph15020130
72. Fijałkowska A, Jȩdrejko K, Sułkowska-Ziaja K, Ziaja M, Kała K, Muszyńska B. Edible mushrooms as a potential component of dietary interventions for major depressive disorder. Foods. (2022) 11:1489. doi: 10.3390/foods11101489
73. Yang L, Wang J, Wang D, Hu G, Liu Z, Yan D, et al. Delayed behavioral and genomic responses to acute combined stress in zebrafish, potentially relevant to PTSD and other stress-related disorders: focus on neuroglia, neuroinflammation, apoptosis and epigenetic modulation. Behav Brain Res. (2020) 389:112644. doi: 10.1016/j.bbr.2020.112644
74. Rothe N, Steffen J, Penz M, Kirschbaum C, Walther A. Examination of peripheral basal and reactive cortisol levels in major depressive disorder and the burnout syndrome: a systematic review. Neurosci Biobehav Rev. (2020) 114:232–70. doi: 10.1016/j.neubiorev.2020.02.024
75. Lipner E, Murphy SK, Breen EC, Cohn BA, Krigbaum NY, Cirillo PM, et al. Infection and higher cortisol during pregnancy and risk for depressive symptoms in adolescent offspring. Psychoneuroendocrinology. (2022) 141:105755. doi: 10.1016/j.psyneuen.2022.105755
76. Lin JG, Kotha P, Chen YH. Understandings of acupuncture application and mechanisms. Am J Transl Res. (2022) 14:1469–81.
77. Kv A, Madhana RM, Js IC, Lahkar M, Sinha S, Naidu V. Antidepressant activity of vorinostat is associated with amelioration of oxidative stress and inflammation in a corticosterone-induced chronic stress model in mice. Behav Brain Res. (2018) 344:73–84. doi: 10.1016/j.bbr.2018.02.009
78. Casaril AM, Dantzer R, Bas-Orth C. Neuronal mitochondrial dysfunction and bioenergetic failure in inflammation-associated depression. Front Neurosci. (2021) 15:725547. doi: 10.3389/fnins.2021.725547
79. Hollis F, Pope BS, Gorman-Sandler E, Wood SK. Neuroinflammation and mitochondrial dysfunction link social stress to depression. Curr Top Behav Neurosci. (2022) 54:59–93. doi: 10.1007/7854_2021_300
80. Li J, Gao W, Zhao Z, Li Y, Yang L, Wei W, et al. Ginsenoside Rg1 reduced microglial activation and mitochondrial dysfunction to alleviate depression-like behaviour via the GAS5/EZH2/SOCS3/NRF2 Axis. Mol Neurobiol. (2022) 59:2855–73. doi: 10.1007/s12035-022-02740-7
81. Xu J, Du W, Zhao Y, Lim K, Lu L, Zhang C, et al. Mitochondria targeting drugs for neurodegenerative diseases-Design, mechanism and application. Acta Pharm Sin B. (2022) 12:2778–89. doi: 10.1016/j.apsb.2022.03.001
82. Brkic Z, Milosavljevic M, Glavonic E, Adzic M. Mitochondrial signaling in inflammation-induced depressive behavior in female and male rats: the role of glucocorticoid receptor. Brain Res Bull. (2019) 150:317–27. doi: 10.1016/j.brainresbull.2019.06.016
83. Brkic Z, Zivanovic A, Adzic M. Sex-specific effects of lipopolysaccharide on hippocampal mitochondrial processes in neuroinflammatory model of depression. Neuroscience. (2020) 451:174–83. doi: 10.1016/j.neuroscience.2020.09.059
84. Kahl KG, Stapel B, Frieling H. Link between depression and cardiovascular diseases due to epigenomics and proteomics: focus on energy metabolism. Prog Neuropsychopharmacol Biol Psychiatry. (2019) 89:146–57. doi: 10.1016/j.pnpbp.2018.09.004
85. Wang Y, Ni J, Gao C, Xie L, Zhai L, Cui G, et al. Mitochondrial transplantation attenuates lipopolysaccharide- induced depression-like behaviors. Prog Neuropsychopharmacol Biol Psychiatry. (2019) 93:240–9. doi: 10.1016/j.pnpbp.2019.04.010
86. Xie H, Huang H, Tang M, Wu Y, Huang R, Liu Z, et al. iTRAQ-based quantitative proteomics suggests synaptic mitochondrial dysfunction in the hippocampus of rats susceptible to chronic mild stress. Neurochem Res. (2018) 43:2372–83. doi: 10.1007/s11064-018-2664-y
87. Zhou M, Liu Z, Yu J, Li S, Tang M, Zeng L, et al. Quantitative proteomic analysis reveals synaptic dysfunction in the amygdala of rats susceptible to chronic mild stress. Neuroscience. (2018) 376:24–39. doi: 10.1016/j.neuroscience.2018.02.010
88. Xu W, Yu J, Jiang Z, Yan W, Li S, Luo Y, et al. The impact of subchronic low-dose exposure to nonylphenol on depression-like behaviors in high-sucrose and high-fat diet induced rats. Toxicology. (2019) 414:27–34. doi: 10.1016/j.tox.2019.01.003
89. Angrand L, Boukouaci W, Lajnef M, Richard JR, Andreazza A, Wu CL, et al. Low peripheral mitochondrial DNA copy number during manic episodes of bipolar disorders is associated with disease severity and inflammation. Brain Behav Immun. (2021) 98:349–56. doi: 10.1016/j.bbi.2021.09.003
90. Chenna S, Koopman W, Prehn J, Connolly N. Mechanisms and mathematical modeling of ROS production by the mitochondrial electron transport chain. Am J Physiol Cell Physiol. (2022) 323:C69–83. doi: 10.1152/ajpcell.00455.2021
91. Teng H, Hong Y, Cao J, Li H, Tian F, Sun J, et al. Senescence marker protein30 protects lens epithelial cells against oxidative damage by restoring mitochondrial function. Bioengineered. (2022) 13:12955–71. doi: 10.1080/21655979.2022.2079270
92. Rajasekaran A, Venkatasubramanian G, Berk M, Debnath M. Mitochondrial dysfunction in schizophrenia: pathways, mechanisms and implications. Neurosci Biobehav Rev. (2015) 48:10–21. doi: 10.1016/j.neubiorev.2014.11.005
93. Perić I, Stanisavljević A, Gass P, Filipović D. Fluoxetine reverses behavior changes in socially isolated rats: role of the hippocampal GSH-dependent defense system and proinflammatory cytokines. Eur Arch Psychiatry Clin Neurosci. (2017) 267:737–49. doi: 10.1007/s00406-017-0807-9
94. Ibi M, Liu J, Arakawa N, Kitaoka S, Kawaji A, Matsuda KI, et al. Depressive-like behaviors are regulated by NOX1/NADPH oxidase by redox modification of NMDA receptor 1. J Neurosci. (2017) 37:4200–12. doi: 10.1523/JNEUROSCI.2988-16.2017
95. Morris G, Fernandes BS, Puri BK, Walker AJ, Carvalho AF, Berk M. Leaky brain in neurological and psychiatric disorders: drivers and consequences. Aust N Z J Psychiatry. (2018) 52:924–48. doi: 10.1177/0004867418796955
96. Riveros ME, Ávila A, Schruers K, Ezquer F. Antioxidant biomolecules and their potential for the treatment of difficult-to-treat depression and conventional treatment-resistant depression. Antioxidants. (2022) 11:540. doi: 10.3390/antiox11030540
97. Hsu CY, Vo T, Lee CW, Chen YL, Lin WN, Cheng HC, et al. Carbon monoxide releasing molecule-2 attenuates angiotensin II-induced IL-6/Jak2/Stat3-associated inflammation by inhibiting NADPH oxidase- and mitochondria-derived ROS in human aortic smooth muscle cells. Biochem Pharmacol. (2022) 198:114978. doi: 10.1016/j.bcp.2022.114978
98. Nguyen TD, Nguyen TH, Do TH, Tran VT, Nguyen HA, Pham DV. Anti-inflammatory effect of a triterpenoid from Balanophora laxiflora: results of bioactivity-guided isolation. Heliyon. (2022) 8:e09070. doi: 10.1016/j.heliyon.2022.e09070
99. Zhang X, Xu H, Hua J, Zhu Z, Wang M. Protective effects of grapefruit essential oil against staphylococcus aureus-induced inflammation and cell damage in human epidermal keratinocytes. Chem Biodivers. (2022) 19:e202200205. doi: 10.1002/cbdv.202200205
100. Sun H, Ma F, Chen W, Yang X. Adipokine ZAG alters depression-like behavior by regulating oxidative stress in hippocampus. Horm Metab Res. (2022) 54:259–67. doi: 10.1055/a-1759-3554
101. Tremblay MÈ, Stevens B, Sierra A, Wake H, Bessis A, Nimmerjahn A. The role of microglia in the healthy brain. J Neurosci. (2011) 31:16064–9. doi: 10.1523/JNEUROSCI.4158-11.2011
102. Hambali A, Kumar J, Hashim N, Maniam S, Cheema MS, Mustapha M, et al. Hypoxia-induced neuroinflammation in Alzheimer’s disease: potential neuroprotective effects of Centella asiatica. Front Physiol. (2021) 12:712317. doi: 10.3389/fphys.2021.712317
103. Wohleb ES. Neuron-microglia interactions in mental health disorders: “for better, and for worse”. Front Immunol. (2016) 7:544. doi: 10.3389/fimmu.2016.00544
104. Takechi R, Lam V, Brook E, Giles C, Fimognari N, Mooranian A, et al. Blood-brain barrier dysfunction precedes cognitive decline and neurodegeneration in diabetic insulin resistant mouse model: an implication for causal link. Front Aging Neurosci. (2017) 9:399. doi: 10.3389/fnagi.2017.00399
105. Mao J, Yang J, Zhang Y, Li T, Wang C, Xu L, et al. Arsenic trioxide mediates HAPI microglia inflammatory response and subsequent neuron apoptosis through p38/JNK MAPK/STAT3 pathway. Toxicol Appl Pharmacol. (2016) 303:79–89. doi: 10.1016/j.taap.2016.05.003
106. Zhi SM, Fang GX, Xie XM, Liu LH, Yan J, Liu DB, et al. Melatonin reduces OGD/R-induced neuron injury by regulating redox/inflammation/apoptosis signaling. Eur Rev Med Pharmacol Sci. (2020) 24:1524–36.
107. Xu D, Lian D, Wu J, Liu Y, Zhu M, Sun J, et al. Brain-derived neurotrophic factor reduces inflammation and hippocampal apoptosis in experimental Streptococcus pneumoniae meningitis. J Neuroinflammation. (2017) 14:156. doi: 10.1186/s12974-017-0930-6
108. Stampanoni Bassi M, Buttari F, Nicoletti CG, Mori F, Gilio L, Simonelli I, et al. Interleukin-1β alters hebbian synaptic plasticity in multiple sclerosis. Int J Mol Sci. (2020) 21:6982. doi: 10.3390/ijms21196982
109. Wang J, Hodes GE, Zhang H, Zhang S, Zhao W, Golden SA, et al. Epigenetic modulation of inflammation and synaptic plasticity promotes resilience against stress in mice. Nat Commun. (2018) 9:477. doi: 10.1038/s41467-017-02794-5
110. Singh AK, Zajdel J, Mirrasekhian E, Almoosawi N, Frisch I, Klawonn AM, et al. Prostaglandin-mediated inhibition of serotonin signaling controls the affective component of inflammatory pain. J Clin Invest. (2017) 127:1370–4. doi: 10.1172/JCI90678
111. Ebada ME. Comment on ‘New drug candidates for depression - a nationwide population-based study’. Acta Psychiatr Scand. (2019) 139:97–8. doi: 10.1111/acps.12984
112. Mesripour A, Shahnooshi S, Hajhashemi V. Celecoxib, ibuprofen, and indomethacin alleviate depression-like behavior induced by interferon-alfa in mice. J Complement Integr Med. (2019) 17:6982. doi: 10.1515/jcim-2019-0016
113. Elnazer HY, Sampson AP, Baldwin DS. Effects of celecoxib augmentation of antidepressant or anxiolytic treatment on affective symptoms and inflammatory markers in patients with anxiety disorders: exploratory study. Int Clin Psychopharmacol. (2021) 36:126–32. doi: 10.1097/YIC.0000000000000356
114. Bay-Richter C, Wegener G. Antidepressant effects of NSAIDs in rodent models of depression-a systematic review. Front Pharmacol. (2022) 13:909981. doi: 10.3389/fphar.2022.909981
115. Badawy M, Abdelall E, El-Nahass ES, Abdellatif K, Abdel-Rahman HM. Design, synthesis, biological assessment and in silico ADME prediction of new 2-(4-(methylsulfonyl) phenyl) benzimidazoles as selective cyclooxygenase-2 inhibitors. RSC Adv. (2021) 11:27659–73. doi: 10.1039/D1RA04756F
116. Song Q, Feng YB, Wang L, Shen J, Li Y, Fan C, et al. COX-2 inhibition rescues depression-like behaviors via suppressing glial activation, oxidative stress and neuronal apoptosis in rats. Neuropharmacology. (2019) 160:107779. doi: 10.1016/j.neuropharm.2019.107779
117. Müller N. Immunological aspects of the treatment of depression and schizophrenia. Dialogues Clin Neurosci. (2017) 19:55–63. doi: 10.31887/DCNS.2017.19.1/nmueller
118. Lukkes JL, Meda S, Thompson BS, Freund N, Andersen SL. Early life stress and later peer distress on depressive behavior in adolescent female rats: effects of a novel intervention on GABA and D2 receptors. Behav Brain Res. (2017) 330:37–45. doi: 10.1016/j.bbr.2017.04.053
119. Huang D, Zhang L, Yang JQ, Luo Y, Cui T, Du TT, et al. Evaluation on monoamine neurotransmitters changes in depression rats given with sertraline, meloxicam or/and caffeic acid. Genes Dis. (2019) 6:167–75. doi: 10.1016/j.gendis.2018.05.005
120. Zhang Q. Synthesis and Activity of Novel COX-2 Inhibitors Containing 5-Aminothiazole Ring Nuclei[D]. Hunan: Hunan Normal University (2020).
121. Hamoud M, Osman NA, Rezq S, Hassan A, Abdel-Fattah HA, Romero DG, et al. Design and synthesis of novel 1,3,4-oxadiazole and 1,2,4-triazole derivatives as cyclooxygenase-2 inhibitors with anti-inflammatory and antioxidant activity in lps-stimulated RAW264.7 macrophages. Bioorg Chem. (2022) 124:105808. doi: 10.1016/j.bioorg.2022.105808
122. Abdelall E, Lamie PF, Aboelnaga LS, Hassan RM. Trimethoxyphenyl containing compounds: synthesis, biological evaluation, nitric oxide release, modeling, histochemical and histopathological studies. Bioorg Chem. (2022) 124:105806. doi: 10.1016/j.bioorg.2022.105806
123. Musa A, Mostafa EM, Bukhari S, Alotaibi NH, Farouk A, Nayl AA, et al. EGFR and COX-2 dual inhibitor: the design, synthesis, and biological evaluation of novel chalcones. Molecules. (2022) 27:1158. doi: 10.3390/molecules27041158
124. Saletti M, Maramai S, Reale A, Paolino M, Brogi S, Di Capua A, et al. Novel analgesic/anti-inflammatory agents: 1,5-Diarylpyrrole nitrooxyethyl sulfides and related compounds as Cyclooxygenase-2 inhibitors containing a nitric oxide donor moiety endowed with vasorelaxant properties. Eur J Med Chem. (2022) 241:114615. doi: 10.1016/j.ejmech.2022.114615
125. Schmied FP, Bernhardt A, Baudron V, Beine B, Klein S. Development and characterization of celecoxib solid self-nanoemulsifying Drug Delivery Systems (S-SNEDDS) prepared using novel cellulose-based microparticles as adsorptive carriers. AAPS PharmSciTech. (2022) 23:213. doi: 10.1208/s12249-022-02347-0
126. Sipos B, Csóka I, Szivacski N, Budai-Szűcs M, Schelcz Z, Zupkó I, et al. Mucoadhesive meloxicam-loaded nanoemulsions: development, characterization and nasal applicability studies. Eur J Pharm Sci. (2022) 175:106229. doi: 10.1016/j.ejps.2022.106229
127. Fang W, Yang F, Li W, Hu Q, Chen W, Yang M, et al. Dexamethasone microspheres and celecoxib microcrystals loaded into injectable gels for enhanced knee osteoarthritis therapy. Int J Pharm. (2022) 622:121802. doi: 10.1016/j.ijpharm.2022.121802
128. Park BK, Kim NS, Kim YR, Yang C, Jung IC, Jang IS, et al. Antidepressant and anti-neuroinflammatory effects of bangpungtongsung-san. Front Pharmacol. (2020) 11:958. doi: 10.3389/fphar.2020.00958
129. Dong XZ, Wang DX, Lu YP, Yuan S, Liu P, Hu Y. Antidepressant effects of Kai-Xin-San in fluoxetine-resistant depression rats. Braz J Med Biol Res. (2017) 50:e6161. doi: 10.1590/1414-431x20176161
130. Jiang X, Wang G, Lin Q, Tang Z, Yan Q, Yu X. Fucoxanthin prevents lipopolysaccharide-induced depressive-like behavior in mice via AMPK- NF-κB pathway. Metab Brain Dis. (2019) 34:431–42. doi: 10.1007/s11011-018-0368-2
131. Wei Y, Hu Y, Qi K, Li Y, Chen J, Wang R. Dihydromyricetin improves LPS-induced sickness and depressive-like behaviors in mice by inhibiting the TLR4/Akt/HIF1a/NLRP3 pathway. Behav Brain Res. (2022) 423:113775. doi: 10.1016/j.bbr.2022.113775
132. Lin J, Song Z, Chen X, Zhao R, Chen J, Chen H, et al. Trans-cinnamaldehyde shows anti-depression effect in the forced swimming test and possible involvement of the endocannabinoid system. Biochem Biophys Res Commun. (2019) 518:351–6. doi: 10.1016/j.bbrc.2019.08.061
133. Tao Z, Chun-Yan H, Hua P, Bin-Bin Y, Xiaoping T. Phyllathin from phyllanthus amarus ameliorates epileptic convulsion and kindling associated post-ictal depression in mice via inhibition of NF-κB/TLR-4 Pathway. Dose Response. (2020) 18:1559325820946914. doi: 10.1177/1559325820946914
134. Wang J, Chen R, Liu C, Wu X, Zhang Y. Antidepressant mechanism of catalpol: involvement of the PI3K/Akt/Nrf2/HO-1 signaling pathway in rat hippocampus. Eur J Pharmacol. (2021) 909:174396. doi: 10.1016/j.ejphar.2021.174396
135. Zhang X, Liu K, Shi M, Xie L, Deng M, Chen H, et al. Therapeutic potential of catalpol and geniposide in Alzheimer’s and Parkinson’s diseases: a snapshot of their underlying mechanisms. Brain Res Bull. (2021) 174:281–95. doi: 10.1016/j.brainresbull.2021.06.020
136. Wu X, Liu C, Wang J, Guan Y, Song L, Chen R, et al. Catalpol exerts antidepressant-like effects by enhancing anti-oxidation and neurotrophy and inhibiting neuroinflammation via activation of HO-1. Neurochem Res. (2022) 47:2975–91. doi: 10.1007/s11064-022-03641-w
137. AbuHasan Q, Reddy V, Siddiqui W. Neuroanatomy, Amygdala. Treasure Island (FL): StatPearls Publishing (2022).
Keywords: COX-2, depression, pro-inflammatory cytokines, mechanism of action, COX-2 inhibitors
Citation: He Y, Han Y, Liao X, Zou M and Wang Y (2022) Biology of cyclooxygenase-2: An application in depression therapeutics. Front. Psychiatry 13:1037588. doi: 10.3389/fpsyt.2022.1037588
Received: 06 September 2022; Accepted: 25 October 2022;
Published: 10 November 2022.
Edited by:
Roberto Ciccocioppo, University of Camerino, ItalyReviewed by:
Raquel Romay-Tallon, University of Illinois at Chicago, United StatesDragana Filipovic, Vinča Institute of Nuclear Science, University of Belgrade, Serbia
Copyright © 2022 He, Han, Liao, Zou and Wang. This is an open-access article distributed under the terms of the Creative Commons Attribution License (CC BY). The use, distribution or reproduction in other forums is permitted, provided the original author(s) and the copyright owner(s) are credited and that the original publication in this journal is cited, in accordance with accepted academic practice. No use, distribution or reproduction is permitted which does not comply with these terms.
*Correspondence: Yuhong Wang, d3loMTA3QGhudWNtLmVkdS5jbg==; Manshu Zou, MDA0NjgwQGhudWNtLmVkdS5jbg==