- 1Department of Psychiatry, The First Hospital of China Medical University, Shenyang, China
- 2Department of Radiology, The First Hospital of China Medical University, Shenyang, China
- 3Brain Function Research Section, The First Hospital of China Medical University, Shenyang, China
- 4Department of Gerontology, The First Hospital of China Medical University, Shenyang, China
Background: Major depressive disorder (MDD) has a high disability rate and brings a large disease burden to patients and the country. Significant sex differences exist in both the epidemiological and clinical features in MDD. The effect of sex on brain function in MDD is not clear now. Regional homogeneity (ReHo) and ALFF are widely used research method in the study of brain function. This research aimed to use ReHo and ALFF to explore gender differences in brain function images in MDD.
Methods: Eighty first-episode drug-naive patients (47 women and 30 men) with MDD and 85 age, education matched healthy volunteers (47 women and 31 men) were recruited in our study and participated in resting-state functional magnetic resonance imaging scans. ReHo and ALFF were used to assess brain activity, two-way ANOVA and post hoc analysis was conducted to explore the sex difference in MDD. Correlation analysis was used to explore the relationship between abnormal brain functioning and clinical symptoms.
Results: We observed sex-specific patterns and diagnostic differences in MDD Patients, further post hoc comparisons indicated that women with MDD showed decreased ALFF value in the right superior occipital gyrus and decreased ReHo value in the left calcarine and left dorsolateral superior frontal gyrus compared with HC females and men with MDD. Men with MDD showed decreased ReHo value in the right median cingulate gyrus compared with HC males and increased ReHo value in the left dorsolateral superior frontal gyrus compared with HC males, we also found that HC males showed higher ReHo value in the right median cingulate gyrus than HC females.
Conclusions: Men and women do have sex differences in brain function, the occipital lobe, calcarine, DLPFC, and DCG were the main different brain regions found between male and female in MDD, which may be the biomarker brain regions that can help diagnose and treat MDD in men and women.
Introduction
Major depressive disorder (MDD) is a multifactorial disease of unknown etiology involving genetics, endocrine factors, chronic inflammation and other aspects. It is mainly characterized by a loss of interest, anhedonia, and decreased energy and may be accompanied by sleep disorders, eating disorders, self-injury, and suicidal thoughts and behaviors (1). According to the World Health Organization (WHO), depression accounts for 10% of the world's total non-fatal disease burden (2). A Chinese epidemiological study found that the incidence of major depression in various regions of China has increased each year, with a lifetime prevalence rate of 3.9% (3). Epidemiological data suggest a significant sex difference in the prevalence of MDD (4–6). A previous meta-analysis found that the incidence of MDD in women was approximately twice as high as that in men (7), but the reason for this difference is currently unclear. Nevertheless, genetic factors, sex hormones, and heightened exposure to severe adversity may be responsible for this difference (8). The clinical manifestations of depression also differ by sex. Women with MDD are more likely to report anxiety symptoms, guilt, atypical depression and somatic symptoms (9, 10), whereas men are more likely to experience suicidal and addictive behaviors (11). These differences in clinical manifestations affect the correct diagnosis and treatment of MDD in different sexes. Therefore, exploring the sex differences in MDD and finding objective indicator differences can contribute to further identification and treatment of MDD.
Previous studies (12, 13) have found that MDD may lead to biological changes, including neurotransmitter imbalance, impaired neurogenesis, decreased neuroplasticity, and abnormal neural circuits. These abnormalities suggest a possible physiological indicator of MDD. The development of magnetic resonance technology has allowed magnetic resonance to become an important imaging method to explore neurological diseases with several advantages, such as non-invasiveness, high sensitivity, and high resolution, and has been widely used in the study of various psychiatric disorders such as obsessive-compulsive disorder (OCD) (14, 15), schizophrenia (16), bipolar disorder (17), depression (18, 19) and so on. Relevant studies have shown that various clinical manifestations of MDD are closely related to changes in structural brain imaging and functional brain imaging (13). Specifically, the cortico-limbic-striatal neural system is the main abnormal brain region in patients with MDD (12, 20). Furthermore, sex differences in the cortico-limbic-striatal neural system have been shown to contribute to sex differences in psychiatric disorders. Functional magnetic resonance imaging (FMRI) is an important method for brain functional imaging research. Blood oxygenation level dependence (BOLD) is a commonly used imaging method for FMRI (21). BOLD mainly uses deoxyhemoglobin (deoxyhemoglobin) as a comparison to take signals of cerebral blood oxygen and cerebral blood flow. Different activities of neurons in the brain can cause changes in cerebral oxygen uptake as well as corresponding changes in blood flow and the conversion of deoxyhemoglobin and oxyhemoglobin. FMRI sensitively and accurately captures and detects these changes, showing changes in brain function in real time. Resting-state functional magnetic resonance imaging (rsfMRI) primarily reflects the spontaneous activity of the brain at rest. RsfMRI has the characteristics of high temporal and spatial resolution and repeatability, the interference to the participants is relatively small, and no specific tasks need to be performed, which can shorten the inspection time and improve the compliance of patients, especially suitable for the development of clinical research (22, 23). Previous studies on the brain function of healthy people have found that rsfMRI reflects the differential changes in healthy groups under different emotional and environmental states (24), and can also reflect stable brain function differences between people (25–27). Some studies have examined sex differences in MDD using the rsfMRI method. Mei et al. (28) used ALFF to show that the bilateral caudate nucleus and posterior cingulate gyrus were the primary regions in the brain that differed between the sexes. In the above brain regions, males in MDD have lower ALFF than females in MDD and female HCs, and female in MDD have higher ALFF compared with male HCs. Yao et al. (29) found a wide range of ALFF values differences in brain regions between male in MDD and female in MDD, including in the frontoparietal, auditory, attention and cerebellum networks. These studies suggest brain functional differences between MDD patients of different sexes, and these differences provide neuroimaging evidence for exploring the sex-specific incidence and clinical manifestations of MDD. Due to the influence of sample size and inclusion criteria, the results of each study are different, suggesting that further research on this topic is required. It is of interest to further explore the sex differences in patients with MDD in the method of ALFF. To our knowledge, the influence of sex on brain function has not yet been fully explored using the regional homogeneity (ReHo) method in patients with MDD and studies combining ReHo and ALFF have not been found. In this study we utilized a whole-brain analysis of regional homogeneity and amplitude of low frequency fluctuation to examine sex differences in medication-naïve participants with MDD. ALFF is a commonly used analytical method in resting f-MRI. By calculating amplitudes with frequencies of 0.01–0.080 Hz, the strength of brain activity in this region is reflected. ReHo is a voxel-based method that estimates brain activity for a given voxel and its nearest neighbors by measuring the similarity or synchrony between time series using the Kendall coefficient (30). ReHo also has been widely used to study brain function in MDD. Sun et al. (31) used the ReHo method and found that patients with early-onset MDD and late-onset MDD have abnormal brain function in the default network. Yang et al. (32) studied 27 MDD patients with suicidal ideation (SI) and 24 MDD patients without SI using a whole-brain analysis of ReHo. They found that elevated cingulate activity may be associated with SI.
In this study, we utilized a whole-brain analysis of regional homogeneity and amplitude of low frequency fluctuation to examine sex differences in medication-naïve participants with MDD. Further understanding of the neurophysiological mechanism of brain function in MDD will provide the basis for the diagnosis and treatment of the disease in the future.
Methods
Participants
All patients were recruited from the outpatient department of the Psychiatry Department of the First Hospital of China Medical University. Healthy people were recruited via advertising. All enrolled patients were assessed for the diagnosis of MDD by two trained psychiatrists according to the Structured Clinical Interview for DSM-IV (SCID-I). A total of 77 MDD patients (30 men and 47 women) aged 18–51 years were enrolled. All patients met the following inclusion criteria: (1) the diagnostic criteria of MDD in SCID-1. (2) Hamilton Depression Scale (HAMD-17) score ≥17. (3) Without any other Axis I and Axis II disorders.
A total of 85 healthy people (31 men and 47 women) matched with the patient group in terms of gender, years of education and age were enrolled. All healthy controls met the following criteria: HAMD-17 score <7 points, no history of mental illness, and no family history of mental illness, without any other Axis I and Axis II disorders.
All enrolled people were excluded in the following situations:
(1) History of a Loss of Consciousness due to Head Trauma for 5 min or More or a History of Other Cerebral Organic Diseases. (2) Chronic Diseases, Such as Hypertension and Diabetes, That may Lead to Changes in Brain. (3) Endocrine Diseases That can Lead to Affective Disorders. (4) History of Substance Abuse and Alcohol Addiction. (5) A Contraindication to MRI Scans. (6) The Inability to Complete MRI Scans. (7) Pregnancy.
All enrolled participants had a detailed understanding of the experimental prohibited content, steps, purpose and related contraindications before enrolment. Written informed consent was signed after fully informed of the study to all participants. The study was approved by the Ethics Committee of China Medical University.
Magnetic resonance imaging scan and processing
Imaging data were acquired by the GE Signa HDx 3.0 T MRI scanner at the First Hospital of China Medical University, Shenyang, China. The patient was instructed to rest with their eyes closed and to avoid thinking. Structure images were obtained by 3D-T1 three-dimensional rapid scrambled gradient echo sequence (3D-FSPGR) with the following scan parameters: repetition time (TR) = 7.1 ms, echo time (TE) = 3.15 ms, flip angle (FA) = 13°, number of slices = 176, slice thickness = 1 mm, voxel resolution = 1 × 1 × 1 mm3, image matrix = 240 × 240, field of view (FOV) = 240 × 240 mm, scan time:8 min 22 s. Spin echo planar imaging (EPI) was used to collect rsfMRI imagine under the following acquisition parameters: TR = 2,000 ms, echo time = 40 ms, matrix = 64 × 64; field of view = 240 × 240 mm2; slice thickness = 3 mm; slice interval = 0; slice number = 35 slices; scan time: 6 min 40 s.
The SPM12 software package and the DPABI software package were used to preprocess the data. Considering the stability of the machine and the adaptation of the enrolled population to the environment, the first 10 time points were removed. To correct for the time difference between layers and head movement, the head rotation did not exceed 3°, and the translation did not exceed 3 mm. Frame-wise displacement (FD) were calculated and no statistical difference were found in mean FD with the four groups, then regression of covariates, including brain white matter signal, cerebrospinal fluid signal and head movement parameter. The images underwent spatial normalization in the Montreal Neurological Institute space using DARTEL with a resampling voxel volume of 3 × 3 × 3 mm.
ReHo analyze: the linear detrend method was used to remove the influence of thermal noise, using a temporal bandpass filter retain the BOLD signal in the 0.01–0.08 Hz frequency band. The consistency between a voxel and the surrounding 26 adjacent voxels was calculated according to the Kendall coefficient of concordance (KCC). The KCC value of each voxel in the entire brain was divided by the mean value of the KCC of the voxels of the entire brain to obtain the normalized ReHo diagram. This process was followed by spatial smoothing with a 6 mm full-width at half-maximum Gaussian kernel smoothing of 6 × 6 × 6 mm.
ALFF analyze: Smoothing with a 6-mm full-width at half-maximum Gaussian kernel, linear detrend method, and then a fast Fourier transform (FFT) was performed to obtain the power spectrum. Obtain the mean square root of the ALFF measurement for each voxel in the range of 0.01–0.08 Hz. Finally, physiological high-frequency noise was eliminated using the temporal bandpass (0.01–0.08 Hz) filter.
Statistical analyses
Subjective characteristics were conducted by SPSS 25.0 software (SPSS IBM Corporation, USA). Age, years of education, and total HAMD-17 scores among the four groups were analyzed by one-way analysis of variance (ANOVA). Two-way analysis of variance (ANOVA) between diagnosis (MDD and HC) and gender (male and female) has also been used to compare demographic data (age and education) and HAMD. Correlational analyses were performed to compare the correlation among the ALFF and ReHo values of the dysfunctions in the brain regions with the HAMA-17 and YMRS scores in females with MDD females and males with MDD groups. Independent sample t tests were utilized to compare the duration of illness between MDD women and MDD men. The statistical significance was determined by p < 0.05.
Correlational analyses were performed to find possible clinical correlation, by compare the correlation among the mean ALFF and ReHo values of the significant different brain regions with the total HAMA-17scores, scores of five clinical factors (cognitive disturbance; anxiety/somatization; psychomotor retardation; sleep disturbance; weight loss) in the HAMD and total HAMA scores in females and males with MDD groups.
The imaging data of brain regions in the four groups were subjected to a two-way analysis of variance (ANCOVA) between diagnosis (MDD and HC) and gender (male and female) as the main effects using SPM12. Statistical significance was determined by voxel p < 0.005 and cluster p < 0.05 [Gaussian random field (GRF) correction]. Significant diagnosis gender interactions were interpreted by performing a post hoc test separately for males and females in the HC and MDD groups (Bonferroni correction, P < 0.05).
Results
Demographic and clinical characteristics of participants
The demographics and clinical features of each group are listed in Table 1. Age, sex, or education years did not significantly differ among men with MDD, women with MDD, HC males, and HC females. The two patient subgroups showed significantly higher HAMD-17 scores than HCs, and no significant differences were found between men and women with MDD. There was no significant effect of diagnosis, sex or interaction of diagnosis and sex in age and education, the effect of diagnosis in HAMD-17 was significant, the duration of illness and disease state have no significantly different between the two MDD groups. The HAMD-17 scores were divided into five clinical factors and are listed in Table 2. No significant associations between ALFF/ReHo values and total HAMA-17 scores, clinical factors in the HAMD and total HAMA scores were found in female and male patients with MDD in correlation analyses.
ALFF and ReHo alterations among four groups
Significant different ALFF values in the main effect of diagnosis were observed in several brain regions, including bilateral calcarine, left caudate, left postcentral gyrus, left precentral gyrus, left thalamus and left orbital inferior frontal gyrus. The ALFF values in patients with MDD was significantly increased in the left caudate, left thalamus, left orbital inferior frontal gyrus, and significantly decreased in the bilateral calcarine, left postcentral gyrus, and left precentral gyrus compared with HCs (Table 3, Figure 1). Significant different ALFF values were also observed between the sexes in several brain regions, including the left putamen, left lingual gyrus, left inferior temporal gyrus, bilateral calcarine, bilateral thalamus, bilateral middle occipital gyrus, left superior temporal gyrus, right median cingulate gyrus, right orbital inferior frontal gyrus, right orbital middle frontal gyrus, right orbital medial gyrus. The female participants exhibited significantly increased ALFF in the left putamen, right orbital medial gyrus, and bilateral thalamus and significantly decreased ALFF in the left lingual gyrus, left inferior temporal gyrus, bilateral calcarine, bilateral middle occipital gyrus, left superior temporal gyrus, right median cingulate gyrus, right orbital inferior frontal gyrus, right orbital middle frontal gyrus compared to male participants (Table 3, Figure 2). The right superior occipital gyrus was significantly observed in diagnosis sex interaction. A post hoc test indicated that MDD females had reduced ALFF in right superior occipital gyrus compared with HC females (P < 0.001, Bonferroni correction) and MDD males (P < 0.005, Bonferroni correction) (Table 3, Figure 3).
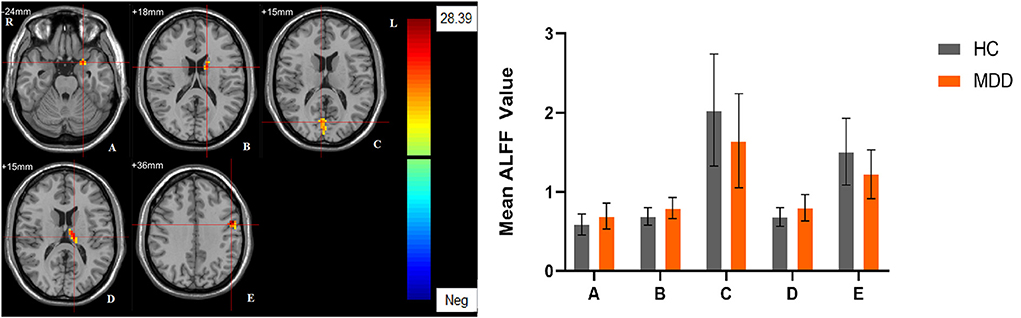
Figure 1. The main effect of diagnostic group. Regions with a main effect of the diagnostic group included (A) The left Olfactory and the left orbital inferior frontal gyrus; (B) The left caudate; (C) The bilateral calcarine; (D) The left thalamus; (E) The left precentral gyrus and the left postcentral gyrus (cluster-level threshold of p < 0.05 after GRF correction). The color bar represents the range of F values. R = right, L = left. The graph shows the ALFF values (mean ± standard deviation) extracted from regions with a main effect of diagnostic group. The Y-axis represents ALFF values. The X-axis represents regions with a main effect of the diagnostic group.
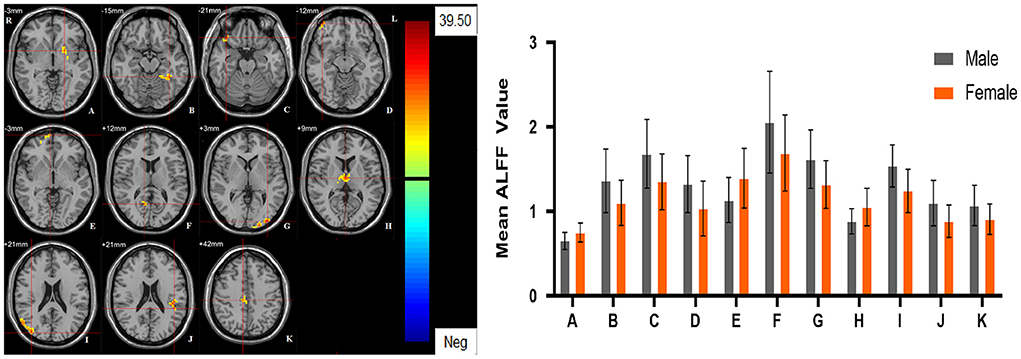
Figure 2. The main effect of sex group. Regions with a main effect of sex group included (A) The left putamen; (B) The left lingual gyrus, the left calcarine and the left inferior temporal gyrus; (C) The right orbital inferior frontal gyrus; (D) The right orbital middle frontal gyrus; (E) The right orbital medial frontal gyrus; (F) The right calcarine; (G) The left middle occipital lobe; (H) The bilateral thalamus; (I) The right middle occipital lobe; (J) The left superior temporal gyrus; (K) The right median cingulate gyrus (cluster-level threshold of p < 0.05 after GRF correction). The color bar represents the range of F values. R = right, L = left. The graph shows the ALFF values (mean ± standard deviation) extracted from regions with a main effect of sex group. The Y-axis represents ALFF values. The X-axis represents regions with a main effect of sex group.
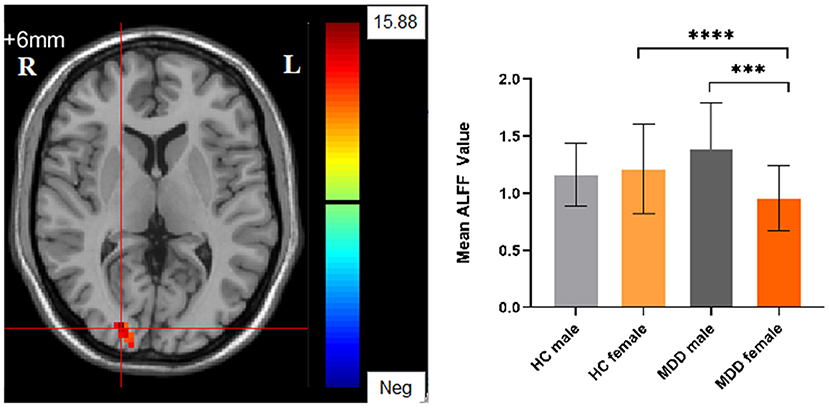
Figure 3. The diagnosis group × gender group interaction. Regions with diagnosis group × sex group interactions include the right superior occipital gyrus (cluster-level threshold of p < 0.05 after GRF correction). The color bar represents the range of F values. R = right, L = left. The graph shows the ALFF values (mean ± standard deviation) extracted from regions with the diagnosis groups × sex group interaction. The Y-axis represents ALFF values. The X-axis represents 4 groups of participants. ****p < 0.001; ***p < 0.005.
Significant different ReHo values in the main effect of diagnosis were observed in the right superior medial frontal gyrus, left postcentral gyrus, right middle temporal gyrus. The ReHo values in patients with MDD was significantly increased in the right superior medial frontal gyrus, right middle temporal gyrus, and significantly decreased in the left postcentral gyrus, compared with HCs (Table 4, Figure 4). In main effect of sex, significant different ReHo value were observed in the bilateral superior temporal gyrus, bilateral lingual gyrus, bilateral calcarine, right orbital inferior frontal gyrus, right orbital middle frontal gyrus, right postcentral gyrus, left middle occipital gyrus, left dorsolateral superior frontal gyrus, left middle temporal gyrus. The female participants exhibited significantly increased ReHo in the left dorsolateral superior frontal gyrus, left middle temporal gyrus, and significantly decreased ReHo in the bilateral superior temporal gyrus, bilateral lingual gyrus, bilateral calcarine, right orbital inferior frontal gyrus, right orbital middle frontal gyrus, right postcentral gyrus, left middle occipital gyrus compared to male participants (Table 4, Figure 5). The left Calcarine, right median cingulate gyrus, left dorsolateral superior frontal gyrus were significantly observed in diagnosis sex interaction. A post hoc test indicated that women with MDD had significantly decreased ReHo value in the left Calcarine compared with HC females (P < 0.005, Bonferroni correction) and men with MDD (P < 0.005, Bonferroni correction). In the left dorsolateral superior frontal gyrus, women with MDD had significantly decreased ReHo value compared with HC females (P < 0.01, Bonferroni correction), while men with MDD had significantly increased ReHo compared with HC males (P < 0.01, Bonferroni correction) and women with MDD (P < 0.001, Bonferroni correction). Furthermore, the ReHo in the right median cingulate gyrus of HC males was higher than that in HC females (P < 0.005, Bonferroni correction), and men with MDD (P < 0.001, Bonferroni correction) (Table 4, Figure 6).
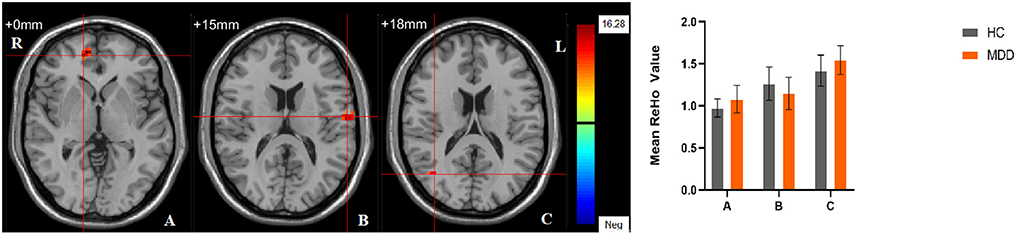
Figure 4. The main effect of diagnostic group. Regions with a main effect of the diagnostic group included (A) The right superior medial frontal gyrus; (B) The left postcentral gyrus; (C) The right middle temporal gyrus (cluster-level threshold of p < 0.05 after GRF correction). The color bar represents the range of F values. R = right, L = left. The graph shows the ReHo values (mean ± standard deviation) extracted from regions with a main effect of diagnostic group. The Y-axis represents ReHo values. The X-axis represents regions with a main effect of the diagnostic group.
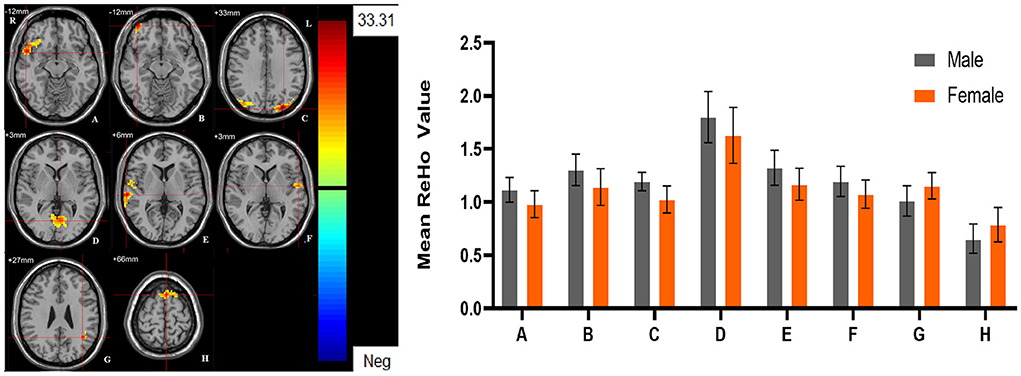
Figure 5. The main effect of sex group. Regions with a main effect of sex group included (A) The right orbital inferior frontal gyrus; (B) The right orbital middle frontal gyrus; (C) The left middle occipital lobe, and the right postcentral gyrus; (D) The bilateral lingual gyrus and the bilateral calcarine; (E) The right superior temporal gyrus; (F) The left superior temporal gyrus; (G) The left middle temporal gyrus; (H) The left dorsolateral Superior frontal gyrus (cluster-level threshold of p < 0.05 after GRF correction). The color bar represents the range of F values. R = right, L = left. The graph shows the ALFF values (mean ± standard deviation) extracted from regions with a main effect of sex group. The Y-axis represents ALFF values. The X-axis represents regions with a main effect of sex group.
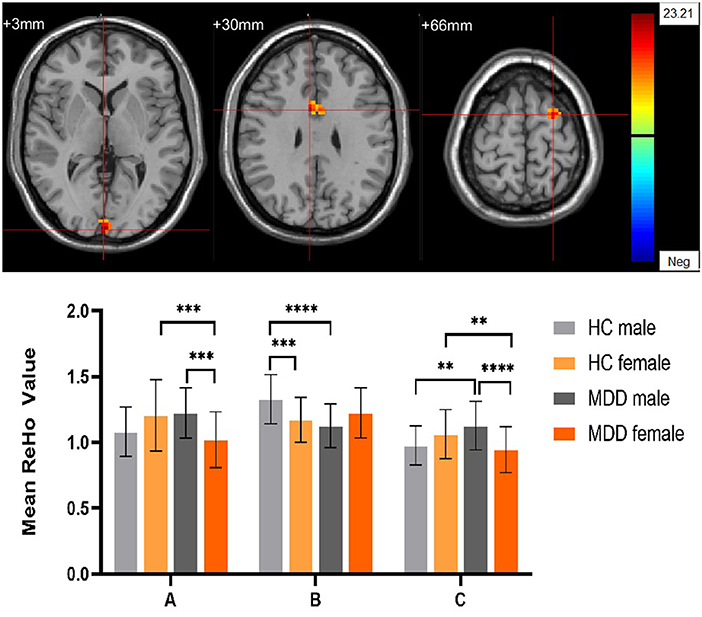
Figure 6. The diagnosis group × gender group interaction. Regions with diagnosis group × sex group interactions include (A) The left Calcarine; (B) The right median cingulate gyrus; (C) The left dorsolateral superior frontal gyrus (cluster-level threshold of p < 0.05 after GRF correction). The color bar represents the range of F values. R = right, L = left. The graph shows the ReHo values (mean ± standard deviation) extracted from regions with the diagnosis groups × sex group interaction. The Y-axis represents ReHo values. The X-axis represents regions with the diagnosis groups × gender group interaction. ****p < 0.001; ***p < 0.005; **p < 0.01.
Discussion
In this study, we observed sex-specific patterns and diagnostic differences in MDD Patients, our further post hoc comparisons indicated that women with MDD showed decreased ALFF value in the right superior occipital gyrus and decreased ReHo value in the left calcarine and left dorsolateral superior frontal gyrus compared with HC females and men with MDD. Men with MDD showed decreased ReHo value in the right median cingulate gyrus compared with HC males and increased ReHo value in the left dorsolateral superior frontal gyrus compared with HC males, we also found that in HC males showed increased ReHo value in the right median cingulate gyrus compared with HC females. The findings revealed that there were sex differences in brain functions in MDD patients and may related to the incidence and clinical features of MDD men and women, this may lead to sex-specific dysfunction in MDD.
The occipital lobe is mainly related to the processing of visual information, and damage to the occipital lobe may lead to a decline in individual cognitive tasks related to vision. Although visual impairment is not included as a core symptom of depressive disorders, studies (33, 34) have found that MDD patients without eye disease have decreased visual function compared with normal people. MRI high-resolution mapping data showed that MDD patients have reductions in myelin in the occipital cortex compared to healthy controls (35). Previous studies have also found abnormal brain structure and function changes in the occipital lobe of MDD patients (36, 37). Calcarine is a concentration of the primary visual cortex of the occipital lobe. Previous research (38) found that calcarine neurological abnormalities may lead to impairment of MDD cognitive control function by affecting visual processing and visual attention. Piani et al. (39) indicted that there was diagnosis by sex effects in the right calcarine cortex by the methods of GMV. The abnormalities found in women with MDD compared with HC women and MDD men in our study suggested that occipital lobe may be one of the neurological biomarkers in MDD women, and may lead to visual impairment and visual cognitive impairment in women with MDD.
The cingulate gyrus is a limbic structure that thought to contribute to motivational aspects of pain and related to responds selection. Previous studies suggested that cingulate gyrus has highly interconnected with amygdala, orbitofrontal cortex (OFC), insular and hippocampus (40). Social pain, error made and conflict between possible responds can active cingulate gyrus (41). Previous studies in MDD were focused more on anterior cingulate gyrus (ACG) and posterior cingulate gyrus (PCG), the median cingulate gyrus (DCG) is also an important limbic structure that participant in salient stimuli processing (42). Studies were found that disfunction of median cingulate gyrus is related to negative emotions (43). Su et al. (44) found that the abnormalities of GMW in bilateral DCG are associated with anorexia nervosa. A meta-analysis has found VBM abnormalities in the DCG in patients with behavior addictions (45). A previous study with a total of 11,246 adult participants found that the symptoms of poor appetite and addictive problem were significantly higher in males compared with MDD women (46), this result has also been confirmed in other studies (11). The decreased ReHo values found in the right DCG in MDD males compared with HC males in our study indicated that the abnormalities of DCG may help to support that MDD males may have more problems in poor appetite and addiction problems.
DLPFC is an important part of PFC that thought to be associated with “cognitive” or “executive” functions (47), receiving input from specific sensory cortices, and regulate emotion through projections to the medial PFC and cingulate cortices (48). Recent studies have found that the imbalance in DLPFC activity may contribute to depression (49, 50). According to the guidelines on the repetitive transcranial magnetic stimulation (rTMS), the level A evidence was reached for high-frequency (HF) rTMS of the left DLPFC for depression (51). Previous studies have found that there were sex differences in the expression of glutamatergic genes and transcription of monoamine in the DLPFC of MDD patients (52, 53). A recent study explored the effects of sex on the neural response to acute psychosocial stress of MDD, and they found that men exhibited higher DLPFC activity than women (54). The above studies supported our results that there are sex differences in DLPFC in MDD patients, with unclear potential neurological mechanism. suggesting that the DLPFC may be the biomarker brain regions that can help diagnose and treat MDD in both men and women, further studies of DLPFC are required.
We also found gender differences of cortical-limbic-thalamus-striatal neural system in ALFF and gender differences of cortical-limbic neural system in ReHo. The orbital frontal gyrus (OFC), temporal gyrus, and occipital gyrus were found to have significantly gender difference in both ALFF and ReHo in all participants.
OFC is an important part of limbic system and have tight connection with with ACC and PCC, Goldstein et al. (55) found that the OFC were activated in response to stress. The temporal lobe is involved in many different functions of the brain, such as gauging distance, recognizing faces, emotion recognition, reading comprehension, etc. A previous study showed that the temporal lobe is involved in the formation of anxiety (56). Kimbrell et al. (57) found that increased regional cerebral blood flow (rCBF) in the left temporal lobe and decreased regional cerebral blood flow in the right temporal lobe were associated with anxiety and anger, as assessed by PET. Sex abnormalities of ALFF also were observed in the left putamen and bilateral thalamus in all participants and HCs; Previous studies have found that patients with MDD show enhanced nodal connectivity in the putamen, which is positively correlated with the number of depressive episodes (58). The thalamus is an important sensory transmission area in the brain and a key relay station that transmits nociceptive messages to the cerebral cortex. Previous fMRI studies found that Painful stimulation can activate the thalamus (59). Sex abnormalities of ReHo were also observed in the postcentral gyrus, which is the sensory center of the body (60), and responds to sensory stimuli, such as touch, pressure, temperature and pain (61). The postcentral gyrus is an important part of the pain perception circuit in the brain, and abnormalities in the central posterior gyrus may lead to the development of chronic pain (62). These above different brain regions found in our study suggest us that there are sex-specific differences in males compared with females, which may influence the incidence and clinical features of MDD men and women.
This study has several limitations. First, this work was limited to a cross-sectional study, where the effects of drugs and disease progression cannot be observed on patients. Future longitudinal studies need to be conducted to provide further guidance for different changes in brain function between men and women with depression. Second, due to the limited number of participants, patients with MDD did not undergo a more detailed subtype classification, future studies will increase sample sizes, different subtypes, conduct a more detailed analysis of differences in brain function in men and women with depression. Finally, our enrolled patients have a large age span of onset, which may have an impact on brain function and structure, and more detailed studies can be conducted to further investigate the effect of MDD onset age on brain function and structure.
Conclusion
In summary, we used the methods of ALFF and ReHo to explore the differences in brain activity between male and female. We found that men and women do have sex differences in brain function, the occipital lobe, calcarine, DLPFC, and DCG were the main different brain regions found in our study between male and female in MDD, which may be the biomarker brain regions that can help diagnose and treat MDD in men and women, these findings revealed that there were sex differences in brain functions in MDD patients and further detailed investigation of sex differences in MDD in the future can make sense.
Data availability statement
The raw data supporting the conclusions of this article will be made available by the corresponding author.
Ethics statement
The studies involving human participants were reviewed and approved by the Medical Scientific Research Ethics Committee of the First Hospital of China Medical University. The patients/participants provided their written informed consent to participate in this study.
Author contributions
YT, LK, FW, and ZT designed the study. LK, FW, and ZT recruited the patients. ZT and XJ acquired the data. ZT and LK analyzed the data. ZT wrote the article. YT was responsible for project administration and funding acquisition. All authors contributed to the article and approved the submitted version.
Funding
This work was supported by grants from the National Key R&D Program of China (Grant Nos. 2018YFC1311600 and 2016YFC1306900 to YT), Liaoning Revitalization Talents Program (Grant No. XLYC1808036 to YT), Natural Science Foundation of Liaoning Province (2020-MS-176 to XJ), National Key R&D Program Science and Technology Winter Olympics (2021YFF0306503 to FW), Joint Fund of National Natural Science Foundation of China (U1808204 to FW), and Natural Science Foundation of Liaoning Province (2019-MS-05 to FW).
Conflict of interest
The authors declare that the research was conducted in the absence of any commercial or financial relationships that could be construed as a potential conflict of interest.
Publisher's note
All claims expressed in this article are solely those of the authors and do not necessarily represent those of their affiliated organizations, or those of the publisher, the editors and the reviewers. Any product that may be evaluated in this article, or claim that may be made by its manufacturer, is not guaranteed or endorsed by the publisher.
References
1. Raison CL, Miller AH. Pathogen-host defense in the evolution of depression: insights into epidemiology, genetics, bioregional differences and female preponderance. Neuropsychopharmacology. (2017) 42:5–27. doi: 10.1038/npp.2016.194
3. Huang Y, Wang Y, Wang H, Liu Z, Yu X, Yan J, et al. Prevalence of mental disorders in China: a cross-sectional epidemiological study. Lancet Psychiatry. (2019) 6:211–24. doi: 10.1016/S2215-0366[18]30511-X
4. Cyranowski JM, Frank E, Young E, Shear MK. Adolescent onset of the gender difference in lifetime rates of major depression: a theoretical model. Arch Gen Psychiatry. (2000) 57:21–7. doi: 10.1001/archpsyc.57.1.21
5. Ford DE, Erlinger TP. Depression and C-reactive protein in US adults: data from the third national health and nutrition examination survey. Arch Int Med. (2004) 164:1010–4. doi: 10.1001/archinte.164.9.1010
6. Mckeever A, Agius M, Mohr P. A review of the epidemiology of major depressive disorder and of its consequences for society and the individual. Psychiatr Danub. (2017) 29:222–31.
7. Salk RH, Hyde JS, Abramson LY. Gender differences in depression in representative national samples: meta-analyses of diagnoses and symptoms. Psychol Bull. (2017) 143:783–822. doi: 10.1037/bul0000102
8. Kuehner C. Why is depression more common among women than among men? Lancet Psychiatry. (2017) 4:146–58. doi: 10.1016/S2215-0366[16]30263-2
9. Vetter JS, Spiller TR, Cathomas F, Robinaugh D, Bruhl A, Boeker H, et al. Kleim B. Sex differences in depressive symptoms and their networks in a treatment-seeking population—a cross-sectional study. J Affect Disord. (2021) 278:357–64. doi: 10.1016/j.jad.2020.08.074
10. Cavanagh A, Wilson CJ, Kavanagh DJ, Caputi P. Differences in the expression of symptoms in men vs. women with depression: a systematic review and meta-analysis. Harv Rev Psychiatry. [2017] 25:29–38. doi: 10.1097/HRP.0000000000000128
11. Shi P, Yang A, Zhao Q, Chen Z, Ren X, Dai Q, et al. Hypothesis of gender differences in self-reporting symptom of depression: implications to solve under-diagnosis and under-treatment of depression in males. Front Psychiatry. (2021) 12:589687. doi: 10.3389/fpsyt.2021.589687
12. Anand A, Li Y, Wang Y, Wu J, Gao S, Bukhari L, et al. Antidepressant effect on connectivity of the mood-regulating circuit: an FMRI study. Neuropsychopharmacology. (2005) 30:1334–44. doi: 10.1038/sj.npp.1300725
13. Patel MJ, Khalaf A, Aizenstein HJ. Studying depression using imaging and machine learning methods. Neuroimage Clin. (2016) 10:115–23. doi: 10.1016/j.nicl.2015.11.003
14. Han S, Xu Y, Guo HR, Fang K, Wei Y, Liu L, et al. Resolving heterogeneity in obsessive-compulsive disorder through individualized differential structural covariance network analysis. Cereb Cortex. (2022). doi: 10.1093/cercor/bhac163. [Epub ahead of print].
15. Han S, Xu Y, Guo HR, Fang K, Wei Y, Liu L, et al. Two distinct subtypes of obsessive compulsive disorder revealed by a framework integrating multimodal neuroimaging information. Hum Brain Mapp. (2022). 43:4254–65. doi: 10.1002/hbm.25951
16. Hoptman MJ, Zuo XN, Butler PD, Javitt DC, D'angelo D, Mauro CJ, et al. Amplitude of low-frequency oscillations in schizophrenia: a resting state fMRI study. Schizophr Res. (2010) 117:13–20. doi: 10.1016/j.schres.2009.09.030
17. Jiang X, Wu F, Zhang Y, Li H, Kang J, Kong L, et al. Gender differences of amplitude of low-frequency fluctuations in bipolar disorder: a resting state fMRI study. J Affect Disord. (2021) 280:189–96. doi: 10.1016/j.jad.2020.11.087
18. Li C, Dong M, Womer FY, Han S, Yin Y, Jiang X, et al. Transdiagnostic time-varying dysconnectivity across major psychiatric disorders. Hum Brain Mapp. (2021) 42:1182–96. doi: 10.1002/hbm.25285
19. Kong L, Chen K, Womer F, Jiang W, Luo X, Driesen N, et al. Sex differences of gray matter morphology in cortico-limbic-striatal neural system in major depressive disorder. J Psychiatr Res. (2013) 47:733–9. doi: 10.1016/j.jpsychires.2013.02.003
20. Anand A, Li Y, Wang Y, Gardner K, Lowe MJ. Reciprocal effects of antidepressant treatment on activity and connectivity of the mood regulating circuit: an FMRI study. J Neuropsychiatry Clin Neurosci. (2007) 19:274–82. doi: 10.1176/jnp.2007.19.3.274
21. Coutanche MN, Thompson-Schill SL, Schultz RT. Multi-voxel pattern analysis of fMRI data predicts clinical symptom severity. Neuroimage. (2011) 57:113–23. doi: 10.1016/j.neuroimage.2011.04.016
22. Biswal BB. Resting state fMRI: a personal history. Neuroimage. (2012) 62:938–44. doi: 10.1016/j.neuroimage.2012.01.090
23. Yang J, Gohel S, Vachha B. Current methods and new directions in resting state fMRI. Clin Imag. (2020) 65:47–53. doi: 10.1016/j.clinimag.2020.04.004
24. Yang H, Long XY, Yang Y, Yan H, Zhu CZ, Zhou XP, et al. Amplitude of low frequency fluctuation within visual areas revealed by resting-state functional MRI. Neuroimage. (2007) 36:144–52. doi: 10.1016/j.neuroimage.2007.01.054
25. Wei L, Duan X, Zheng C, Wang S, Gao Q, Zhang Z, et al. Specific frequency bands of amplitude low-frequency oscillation encodes personality. Hum Brain Mapp. (2014) 35:331–9. doi: 10.1002/hbm.22176
26. Liu H, Liao J, Jiang W, Wang W. Changes in low-frequency fluctuations in patients with antisocial personality disorder revealed by resting-state functional MRI. PLoS ONE. (2014) 9:e89790. doi: 10.1371/journal.pone.0089790
27. Song Y, Huang C, Zhong Y, Wang X, Tao G. Abnormal reginal homogeneity in left anterior cingulum cortex and precentral gyrus as a potential neuroimaging biomarker for first-episode major depressive disorder. Front Psychiatry. (2022) 13:924431. doi: 10.3389/fpsyt.2022.924431
28. Mei L, Wang Y, Liu C, Mou J, Yuan Y, Qiu L, et al. Study of sex differences in unmedicated patients with major depressive disorder by using resting state brain functional magnetic resonance imaging. Front Neurosci. (2022) 16:8144. doi: 10.3389/fnins.2022.814410
29. Yao Z, Yan R, Wei M, Tang H, Qin J, Lu Q. Gender differences in brain activity and the relationship between brain activity and differences in prevalence rates between male and female major depressive disorder patients: a resting-state fMRI study. Clin Neurophysiol. (2014) 125:2232–9. doi: 10.1016/j.clinph.2014.03.006
30. Zang Y, Jiang T, Lu Y, He Y, Tian L. Regional homogeneity approach to fMRI data analysis. Neuroimage. (2004) 22:394–400. doi: 10.1016/j.neuroimage.2003.12.030
31. Sun JF, Chen LM, He JK, Wang Z, Guo CL, Ma Y, et al. A comparative study of regional homogeneity of resting-state fMRI between the early-onset and late-onset recurrent depression in adults. Front Psychol. (2022) 13:849847. doi: 10.3389/fpsyg.2022.849847
32. Yang C, Duan Y, Lei L, Liu P, Zhang A, Li G, et al. Altered cingulum functioning in major depressive disorder patient with suicide attempts: a resting-state functional magnetic resonance imaging study. Front Neurosci. (2022) 16:849158. doi: 10.3389/fnins.2022.849158
33. Friberg TR, Borrero G. Diminished perception of ambient light: a symptom of clinical depression? J Affect Disord. (2000) 61:113–8. doi: 10.1016/S0165-0327(99)00194-9
34. Golomb JD, Mcdavitt JR, Ruf BM, Chen JI, Saricicek A, Maloney KH, et al. Enhanced visual motion perception in major depressive disorder. J Neurosci. (2009) 29:9072–7. doi: 10.1523/JNEUROSCI.1003-09.2009
35. Chandley MJ, Szebeni A, Szebeni K, Wang-Heaton H, Garst J, Stockmeier CA, et al. Markers of elevated oxidative stress in oligodendrocytes captured from the brainstem and occipital cortex in major depressive disorder and suicide. Prog Neuropsychopharmacol Biol Psychiatry. (2022) 117:110559. doi: 10.1016/j.pnpbp.2022.110559
36. Shen Z, Cheng Y, Yang S, Dai N, Ye J, Liu X, et al. Changes of grey matter volume in first-episode drug-naive adult major depressive disorder patients with different age-onset. Neuroimage Clin. (2016) 12:492–8. doi: 10.1016/j.nicl.2016.08.016
37. Zhang B, Li M, Qin W, Demenescu LR, Metzger CD, Bogerts B, et al. Altered functional connectivity density in major depressive disorder at rest. Eur Arch Psychiatry Clin Neurosci. (2016) 266:239–48. doi: 10.1007/s00406-015-0614-0
38. Yang Y, Wei K, Zhang H, Hu H, Yan L, Gui W, et al. Identifying functional brain abnormalities in migraine and depression comorbidity. Quant Imaging Med Surg. (2022) 12:2288–302. doi: 10.21037/qims-21-667
39. Piani MC, Maggioni E, Delvecchio G, Ferro A, Gritti D, Pozzoli SM, et al. Sexual dimorphism in the brain correlates of adult-onset depression: a pilot structural and functional 3T MRI study. Front Psychiatry. (2021) 12:683912. doi: 10.3389/fpsyt.2021.683912
40. Vogt BA. Cingulate cortex in the three limbic subsystems. Handb Clin Neurol. (2019) 166:39–51. doi: 10.1016/B978-0-444-64196-0.00003-0
41. Rolls ET. The cingulate cortex and limbic systems for emotion, action, and memory. Brain Struct Funct. (2019) 224:3001–18. doi: 10.1007/s00429-019-01945-2
42. Small DM, Zatorre RJ, Dagher A, Evans AC, Jones-Gotman M. Changes in brain activity related to eating chocolate: from pleasure to aversion. Brain. (2001) 124:1720–33. doi: 10.1093/brain/124.9.1720
43. Jiang B, He D, Guo Z, Gao Z. Effect-size seed-based d mapping of resting-state fMRI for persistent insomnia disorder. Sleep Breath. (2020) 24:653–9. doi: 10.1007/s11325-019-02001-3
44. Su T, Gong J, Tang G, Qiu S, Chen P, Chen G, et al. Structural and functional brain alterations in anorexia nervosa: a multimodal meta-analysis of neuroimaging studies. Hum Brain Mapp. (2021) 42:5154–69. doi: 10.1002/hbm.25602
45. Starcke K, Antons S, Trotzke P, Brand M. Cue-reactivity in behavioral addictions: a meta-analysis and methodological considerations. J Behav Addict. (2018) 7:227–38. doi: 10.1556/2006.7.2018.39
46. Adewuya AO, Coker OA, Atilola O, Ola BA, Zachariah MP, Adewumi T, et al. Gender difference in the point prevalence, symptoms, comorbidity, and correlates of depression: findings from the Lagos State Mental Health Survey (LSMHS), Nigeria. Arch Womens Ment Health. (2018) 21:591–9. doi: 10.1007/s00737-018-0839-9
47. Koenigs M, Grafman J. The functional neuroanatomy of depression: distinct roles for ventromedial and dorsolateral prefrontal cortex. Behav Brain Res. (2009) 201:239–43. doi: 10.1016/j.bbr.2009.03.004
48. Yang S, Seo H, Wang M, Arnsten AFT. NMDAR neurotransmission needed for persistent neuronal firing: potential roles in mental disorders. Front Psychiatry. (2021) 12:654322. doi: 10.3389/fpsyt.2021.654322
49. Biver F, Goldman S, Delvenne V, Luxen A, De Maertelaer V, Hubain P, et al. Frontal and parietal metabolic disturbances in unipolar depression. Biol Psychiatry. (1994) 36:381–8. doi: 10.1016/0006-3223(94)91213-0
50. Baxter LR, Schwartz JM, Phelps ME, Mazziotta JC, Guze BH, Gerner RH, et al. Reduction of prefrontal cortex glucose metabolism common to three types of depression. Arch Gen Psychiatry. (1989) 46:243–50. doi: 10.1001/archpsyc.1989.01810030049007
51. Lefaucheur JP, Aleman A, Baeken C, Benninger DH, Brunelin J, Di Lazzaro V, et al. Evidence-based guidelines on the therapeutic use of repetitive transcranial magnetic stimulation (rTMS): an update (2014–2018). Clin Neurophysiol. (2020) 131:474–528. doi: 10.1016/j.clinph.2020.02.003
52. Powers B, Joyce C, Kleinman JE, Hyde TM, Ajilore O, Leow A. et al. Sex differences in the transcription of glutamate transporters in major depression and suicide. J Affect Disord. (2020) 277:244–52. doi: 10.1016/j.jad.2020.07.055
53. Bristow GC, Eisenlohr-Moul T, Lotesto K, Sodhi MS. Sex differences in the transcription of monoamine transporters in major depression. J Affect Disord. (2021) 295:1215–9. doi: 10.1016/j.jad.2021.08.124
54. Dong D, Ironside M, Belleau EL, Sun X, Cheng C, Xiong G, et al. Sex-specific neural responses to acute psychosocial stress in depression. Transl Psychiatry. (2022) 12:2. doi: 10.1038/s41398-021-01768-y
55. Goldstein JM, Jerram M, Abbs B, Whitfield-Gabrieli S, Makris N. Sex differences in stress response circuitry activation dependent on female hormonal cycle. J Neurosci. (2010) 30:431–8. doi: 10.1523/JNEUROSCI.3021-09.2010
56. Sawalha J, Yousefnezhad M, Selvitella AM, Cao B, Greenshaw AJ, Greiner R. Predicting pediatric anxiety from the temporal pole using neural responses to emotional faces. Sci Rep. (2021) 11:16723. doi: 10.1038/s41598-021-95987-4
57. Kimbrell TA, George MS, Parekh PI, Ketter TA, Podell DM, Danielson AL, et al. Regional brain activity during transient self-induced anxiety and anger in healthy adults. Biol Psychiatry. (1999) 46:454–65. doi: 10.1016/S0006-3223(99)00103-1
58. Gong Q, He Y. Depression, neuroimaging and connectomics: a selective overview. Biol Psychiatry. (2015) 77:223–35. doi: 10.1016/j.biopsych.2014.08.009
59. Yen CT, Lu PL. Thalamus and pain. Acta Anaesthesiol Taiwan. (2013) 51:73–80. doi: 10.1016/j.aat.2013.06.011
60. Kropf E, Syan SK, Minuzzi L, Frey BN. From anatomy to function: the role of the somatosensory cortex in emotional regulation. Braz J Psychiatry. (2019) 41:261–9. doi: 10.1590/1516-4446-2018-0183
61. Lloyd DM, Mcglone FP, Yosipovitch G. Somatosensory pleasure circuit: from skin to brain and back. Exp Dermatol. (2015) 24:321–4. doi: 10.1111/exd.12639
Keywords: ReHo, gender, magnetic resonance imaging, occipital lobe, ALFF, dorsolateral superior frontal gyrus, median cingulate gyrus
Citation: Tu Z, Wu F, Jiang X, Kong L and Tang Y (2022) Gender differences in major depressive disorders: A resting state fMRI study. Front. Psychiatry 13:1025531. doi: 10.3389/fpsyt.2022.1025531
Received: 23 August 2022; Accepted: 17 October 2022;
Published: 10 November 2022.
Edited by:
Shaoqiang Han, Zhengzhou University, ChinaReviewed by:
Xiaoping Wang, Central South University, ChinaLong-Biao Cui, Fourth Military Medical University, China
Copyright © 2022 Tu, Wu, Jiang, Kong and Tang. This is an open-access article distributed under the terms of the Creative Commons Attribution License (CC BY). The use, distribution or reproduction in other forums is permitted, provided the original author(s) and the copyright owner(s) are credited and that the original publication in this journal is cited, in accordance with accepted academic practice. No use, distribution or reproduction is permitted which does not comply with these terms.
*Correspondence: Lingtao Kong, bHRrb25nQGNtdS5lZHUuY24=; Yanqing Tang, dGFuZ3lhbnFpbmdAY211LmVkdS5jbg==
†These authors have contributed equally to this work