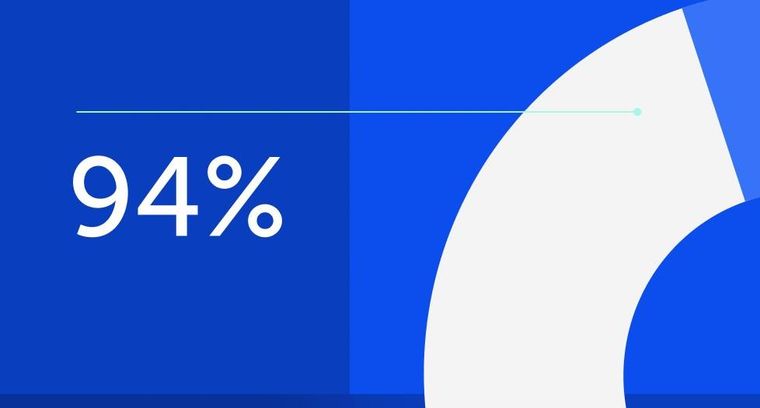
94% of researchers rate our articles as excellent or good
Learn more about the work of our research integrity team to safeguard the quality of each article we publish.
Find out more
ORIGINAL RESEARCH article
Front. Psychiatry, 30 November 2021
Sec. Neuroimaging
Volume 12 - 2021 | https://doi.org/10.3389/fpsyt.2021.735623
Purpose: Comprehensive and longitudinal brain analysis is of great significance for understanding the pathological changes of antipsychotic drug treatment in patients with schizophrenia. This study aimed to investigate the changes of structure, function, and network properties in patients with first-episode schizophrenia (FES) after antipsychotic therapy and their relationship with clinical symptoms.
Materials and Methods: A total of 30 patients diagnosed with FES and 30 healthy subjects matched for sex and age were enrolled in our study. Patients at baseline were labeled as antipsychotic-naive first-episode schizophrenia (AN-FES), and patients after antipsychotic treatment were labeled as antipsychotic treatment first-episode schizophrenia (AT-FES). The severity of illness was measured by using the PANSS and CGI score. Structural and functional MRI data were also performed. Differences in GMV, ALFF, and ReHo between the FES group and healthy control group were tested using a voxel-wise two-sample t-test, and the comparison of AN-FES group and AT-FES group was evaluated by paired-sample t-test.
Results: After the 1-year follow-up, the FES patients showed increased GMV in the right cerebellum, right inferior temporal gyrus, left middle frontal gyrus, parahippocampal gyrus, bilateral inferior parietal lobule, and reduced GMV in the left occipital lobe, gyrus rectus, right orbital frontal cortex. The patients also showed increased ALFF in the medial superior frontal gyrus and right precentral gyrus. For network properties, the patients showed reduced characteristic path length and increased global efficiency. The GMV of the right inferior parietal lobule was negatively correlated with the clinical symptoms.
Conclusions: Our study showed that the antipsychotic treatment contributed to the structural alteration and functional improvement, and the GMV alteration may be associated with the improvement of clinical symptoms.
Schizophrenia is a complex disease of the central nervous system, and a great deal of health care resources are devoted to these patients (1, 2). The genetic and environmental factors play an important role in the occurrence and development of schizophrenia. However, its pathogenic mechanism has not been fully defined, and further research is still needed. Functional magnetic resonance imaging (fMRI) has been widely used in the study of schizophrenia (3). The voxel based morphometry analysis is a common method to evaluate the change of gray matter volume (GMV). Resting-state fMRI is more stable and relatively easier to perform than task state fMRI, and is mainly used for brain functional connectivity and brain network analysis. Previous studies have shown structural and functional deficits in patients with schizophrenia, which may provide useful information for understanding the pathophysiology of schizophrenia (4).
Typical antipsychotics control symptoms by reducing the function of dopaminergic neurons (5). In addition to disease progression, many studies have confirmed that antipsychotics may affect the structure, function, and network characteristics of patients with schizophrenia to a certain extent (6, 7). However, previous studies have used a relatively single brain analysis method for cross-sectional design, which may not provide a comprehensive understanding of the effects of antipsychotics on patients with schizophrenia. Longitudinal and comprehensive studies on the effects of antipsychotic medication are necessary.
Therefore, in this study, we aimed to analyze the changes in the structure, function, and network properties of FES after antipsychotic therapy and their correlation with clinical symptoms.
A total of 30 subjects diagnosed with FES were enrolled in our study. The FES patients were defined according to the patient edition of the Structured Clinical Interview for DSM-IV Axis I Disorders (SCID-IV) (8). Patients at baseline were labeled as antipsychotic-naive first-episode schizophrenia (AN-FES), and patients after antipsychotic treatment were labeled as antipsychotic treatment first-episode schizophrenia (AT-FES). Patients were selected from our hospital. The exclusion criteria for all subjects were a history of head injury, organic mental disorder, neurological disorder, serious medical or surgical illness, and a history of substance abuse. The healthy control subjects were recruited from the local community by advertisement. They had no history of psychiatric illness, as determined by the non-patient edition of the SCID, and no history of psychotic disorders among their first-degree relatives. This study was approved by our ethics committee, and all subjects provided fully informed written consent to participate in the study.
The severity of illness was measured by senior clinical psychiatrists from the Department of Psychiatry using the Positive and Negative Syndrome Scale (PANSS) (9) and Clinical Global Impression Scales (CGI). CGI includes CGI for severity (CGI-S) and CGI for improvement (CGI-I). Patients received treatment according to the severity of the condition at baseline. During the follow-up, all patients received second generation antipsychotic drugs, including risperidone, aripiprazole and olanzapine. The PANSS and CGI score were assessed again after 1-year follow-up.
MRI data acquisition was performed on a 3.0T scanner (Signa HDx; GE Medical Systems, Milwaukee, WI, USA) equipped with an 8-channel phased-array brain coil at our hospital. Details regarding the image acquisition protocol and fMRI data preprocessing were presented in the Supplemental Material.
The VBM8 toolbox (http://dbm.neuro.uni-jena.de/vbm.html) was used to estimate GMV. Structural MRI images were segmented into GM, WM, and cerebrospinal fluid using the standard segmentation model. After initial affine registration of the GM concentration map into the Montreal Neurological Institute (MNI) coordinate space, the GM concentration images were non-linearly warped using the DARTEL technique and then resampled to a voxel size of 1.5 × 1.5 × 1.5 mm. The GMV was obtained by multiplying the GM concentration map by the non-linear determinants that were derived from the spatial normalization step. Finally, the GMV images were smoothed using a Gaussian kernel of 6 × 6 × 6 mm full width at half maximum (FWHM).
ALFF and ReHo were calculated using REST software (http://restfmri.net/forum/rest_v18). For standardization purposes, the ALFF of each voxel were divided by the global mean ALFF value within the whole-brain mask. Prior to the calculation of ReHo, the data were bandpass filtered (0.01–0.08 Hz). Subsequently, ReHo was determined on a voxel-by-voxel basis by calculating Kendall's coefficient of concordance of the time courses of a given voxel with those of its nearest 26 neighbors. Similar to the ALFF analysis, the ReHo of each voxel was divided by the global mean ReHo value.
The software Gretna (http://www.nitrc.org/projects/gretna, v1.2.1) was used to reconstruct the functional brain network. The brain was divided into 90 regions by anatomical automatic labeling, and each region was regarded as a node of network. The pearson correlation coefficient between different nodes was calculated and a matrix with 90 × 90 symmetric distribution was established. Then the matrix was transformed into binary connection matrix, and the sparsity of connection matrix was regarded as a set threshold to construct brain functional networks. The whole and local topology parameters were analyzed at each sparsity. The whole topology parameters include small-world parameters and network efficiency. The small-world parameters include clustering coefficient (Cp), characteristic path length (Lp), normalized clustering coefficient (γ), normalized shortest path length (λ), and small-world index (σ). The network efficiency includes local efficiency (Eloc) and global efficiency (Eglob). The local topology parameters include knodal, Enodal, Degree centrality, and Betweenness centrality.
For voxel-based analyses of GMV, ALFF, and ReHo, the parametric test in the SPM8 package was used to test for statistical significance. Statistical inferences were determined with a voxel-level statistical threshold (P < 0.001, uncorrected). Differences in GMV, ALFF, and ReHo between the FES group and healthy control group were tested using a voxel-wise two-sample t-test, and the comparison of AN-FES group and AT-FES group was evaluated by paired-sample t-test.
The network parameters were analyzed by the software Gretna, and took the Sparsity as threshold, the range was 0.05 ≤ S ≤ 0.5, the interval value is 0.05, so the network analysis within this scope. In order to clarify the differences among subjects of the topology properties, the FES and healthy control group was tested by two-sample t-test, AN-FES group and AT-FES group was evaluated by paired-sample t-test. Meanwhile, the edge index of the network was considered qualitatively by using NBS, the threshold was P < 0.05, and the result was presented by BrainNet Viewer software.
Correlations among structural, functional abnormalities, global network properties, and severity of illness in AN-FES and AT-FES group were evaluated by partial correlation analysis. The gender and age were regarded as covariate for subsequent analysis. The correlation between the GMV, ALFF, ReHo, and global network properties in different regions in AT-FES group compared with AN-FES group and the difference value of positive, negative, general psychopathology, total scores on the PANSS, and CGI-S score before and after treatment and CGI-I score after treatment was analyzed. The threshold was P < 0.05.
Table 1 shows the demographic and clinical characteristics of the FES group and healthy control group. The CGI-I scores of the AN-FES group and the AT-FES group were 0 and 1.6 ± 0.7, respectively, indicating a significant therapeutic effect. The scores of CGI and PANSS in AT-FES group were lower than those in AN-FES group, and the difference was statistically significant (Table 2, P < 0.05).
Compared with healthy control group, the AN-FES group showed reduced GMV in the midbrain and left superior temporal gyrus (Supplementary Table 1, P < 0.001).
ALFF in the medial superior frontal gyrus, left middle frontal gyrus, right superior frontal gyrus, and right insula were all decreased in AN-FES group compared with healthy controls (Supplementary Table 1, P < 0.001).
In addition, AN-FES group also showed reduced ReHo in right superior frontal gyrus and right middle frontal gyrus and increased ReHo in the posterior cingulate cortex (Supplementary Table 1, P < 0.001).
As 0.05 ≤ Sparsity ≤ 0.5, both the AN-FES group and healthy control group had higher γ value (all larger than 1) and approximately equal λ value. The σ > 1 of the two groups indicated that the two groups exhibited no difference in the small-world architecture of the functional brain network (P > 0.05). Compared with healthy controls, the Cp, Eglob, and Eloc of AN-FES were lower, and the Lp was higher, with statistically significant differences (Supplementary Table 2, P < 0.05).
There were significant differences in the betweenness centrality and degree centrality between AN-FES and the healthy control group, as shown in Supplementary Table 3.
The AT-FES group showed increased GM volume in the right cerebellum, right inferior temporal gyrus (ITG), left middle frontal gyrus (MFG), parahippocampal gyrus, bilateral IPL, and reduced GMV in the left occipital lobe, gyrus rectus, right orbital frontal cortex with that of the AN-FES group (Supplementary Table 4, Figure 1, P < 0.001).
Figure 1. GMV differences between AN-FES group and AT-FES group. VBM analysis shows gray matter regions with GMV differences (P < 0.001, uncorrected) across the two groups (A). A post-hoc ROI analysis shows the comparisons (P < 0.001, uncorrected) (B). Error bars indicate the standard error of the mean. *P < 0.001, uncorrected. AN-FES, antipsychotic-naive first-episode schizophrenia; AT-FES, antipsychotic treatment first-episode schizophrenia; GMV, gray matter volume; L, left; R, right; ITG, inferior temporal gyrus; Occ, occipital lobe; MFG, middle frontal gyrus; Rect, gyrus rectus; PPG, parahippocampal gyrus; IPL, inferior parietal lobule.
The AT-FES patients showed increased ALFF in the medial superior frontal gyrus and right precentral gyrus (Supplementary Table 4, Figure 2, P < 0.001).
Figure 2. ALFF differences between AN-FES group and AT-FES group. Voxel-based analysis shows gray matter regions with ALFF differences (P < 0.001, uncorrected) across the two groups (A). A post hoc ROI analysis shows the comparisons (P < 0.001, uncorrected) (B). Error bars indicate the standard error of the mean. *P < 0.001, uncorrected. ALFF, amplitude of low frequency fluctuations; AN-FES, antipsychotic-naive first-episode schizophrenia; AT-FES, antipsychotic treatment first-episode schizophrenia; SFG, superior frontal gyrus; PCG, precentral gyrus; R, right; Med, medial.
The ReHo in AT-FES group and AN-FES group showed no difference.
As 0.05 ≤ Sparsity ≤ 0.5, both the AN-FES group and AT-FES group had higher γ value (all larger than 1) and approximately equal λ value. The σ > 1 in both groups indicated that the two groups showed no difference in the small-world structure of functional brain networks (Supplementary Table 5, Figure 3, P > 0.05). Compared with the AN-FES group, a lower Lp and higher Eglob were found in the AT-FES group (Supplementary Table 5, Figure 3, P < 0.05).
Figure 3. Comparison of the whole brain attributes between AN-FES group and AT-FES group at different sparsity. AN-FES, antipsychotic-naive first-episode schizophrenia; AT-FES, antipsychotic treatment first-episode schizophrenia.
Multiple brain regions showed significant differences in the betweenness centrality and degree centrality between the AT-FES and AN-FES groups, details were shown in Supplementary Table 6.
The NBS showed the coexisting increased and reduced edge index of the network in AT-FES group and AN-FES group (Figure 4).
Figure 4. The abnormal NBS functional connectivity in AN-FES group and AT-FES group. NBS, network-based statistic; AN-FES, antipsychotic-naive first-episode schizophrenia; AT-FES, antipsychotic treatment first-episode schizophrenia.
Partial correlation analysis showed that GMV of the left parietal lobe was positively correlated with the general psychopathological differences of PANSS before and after treatment (r = 0.519, P = 0.016). The details were shown in Supplementary Table 7.
In this study, we analyzed the differences in brain structure, function, and network topological characteristics between the FES group and healthy controls at baseline, and explored the effect of antipsychotics on FES after 1 year of follow-up. Multiple brain regions showed reduced GMV, ALFF, and ReHo at baseline, and the GMV of FES after treatment showed coexisting reduced and increased brain regions. The partial correlation analysis showed the correlation of GMV, network topological properties, and clinical symptoms. Our study provides comprehensive and valuable longitudinal information on FES.
MRI confirmed a decrease in GMV and white matter volume (WMV), with some investigators noting that the decrease in GMV (2%) was more pronounced than the decrease in WMV (1%) (10, 11). Thus, the study of GMV in schizophrenia patients may helpful for pathogenesis and pathologic changes research. Our results showed reduced GMV in the midbrain and left superior temporal gyrus at baseline. Most previous studies indicated the reduction of GMV usually existed in the prefrontal cortex, temporal lobe and parietal cortex (12–14). Nopoulos et al. (15) also found that the schizophrenia patients had significantly smaller midbrain measures compared with control subjects. Since dopamine disorders are central to the pathophysiology of psychosis, the midbrain contains the extranuclear body of all dopamine neurons in the human brain.
In our study, ALFF and ReHo in some brain regions decreased at baseline, suggesting impaired local brain activity and functional connectivity in FES. The schizophrenia patients showed obvious ALFF reduction in bilateral occipital lobe, motorsensory cortex, and posterior parietal cortex by comparison with healthy subject (16). Some studies pointed out that the FES had reduced ALFF in prefrontal lobe, and this change was more obvious in early stage of the disease (17, 18). The increased or reduced ReHo all indicated the abnormity of synchrony of spontaneous neuron activity and coordination mechanism, and evidence abounds that the ReHo abnormity existed in prefrontal lobe, superior temporal gyrus, and posterior cingulate cortex (19–21). Salvador first used the automated anatomical labeling as a template for brain region segmentation and built a whole brain network in 2005 (22). Some researchers (23–25) indicated the Cp, Eglob, Eloc, and node degree were reduced and Lp was increased in schizophrenia patients, which was consistent with our research results.
Our study showed coexisting reduced and increased brain regions in AT-FES patients, and the increased GMV of the left parietal lobule was negatively correlated with clinical symptoms, indicating that the change of GMV after treatment was correlated with improvement of schizophrenia. The antipsychotics may influence the normal physiological process of brain and the progress of schizophrenia, and then have an effect on GMV change of brain (12). Most studies showed the abnormity of GMV exist mainly in temporal lobe, parietal lobe and occipital lobe, and this change was correlated with positive symptoms of schizophrenia, cognitive, and emotional function impediment (26, 27). In our study, the AT-FES group showed reduced GMV in the left occipital lobe, gyrus rectus, right orbital frontal cortex with that of the AN-FES group. The gyrus rectus, right orbital frontal cortex belong to the prefrontal cortex, which is thought to be an important brain region for processing emotions and cognition, such as working memory, and is associated with self-awareness. The present study found that the occipital lobe GMV decreased in the FES group, which was consistent with the conclusion of the previous study (28). Some studies found that the total dose of antipsychotics may predict the GMV change in FES (29, 30). A recent study identified the relationship between antipsychotics and structural changes and suggested that GMV may have the potential to predict antipsychotic response in patients with FES (31). However, due to the small sample size and other factors, further researches are needed in the future.
Our study showed the increased ALFF in the medial superior frontal gyrus and the right precentral gyrus of FES after treatment. Lui et al. reported the ALFF was increased after treatment in some brain regions such as the prefrontal lobe and parietal lobe (17). The medial superior frontal gyrus participates in task state cognition and motor behavior, and also was a part of the default network. In this study, ALFF in the medial superior frontal gyrus decreased at baseline and increased after treatment, suggesting that antipsychotics may have an impact on the local function of this brain region. The anterior central gyrus is involved in the processing of cognitive information related to motor function, and cognitive abnormalities and intentional control disorders may occur after injury. A previous study showed that antipsychotic drugs ameliorate abnormalities in the anterior central gyrus (32).
There are few studies on the effect of antipsychotics on network properties in patients with schizophrenia. A study indicated that the antipsychotics may improve the reduced Eglob and increased Cp (33). In our study, the brain regions with different node properties before and after treatment in FES were the auditory network, which mainly included the insular lobe, transverse gyrus and supramarginal gyrus; the subcortical network mainly included the amygdala, putamen and pallidum; and the sensorimotor network mainly included the pre-central gyrus and post-central gyrus. Most of the brain regions that reduced betweenness centrality at baseline improved after treatment, and this change was associated with remission of clinical symptoms. Previous studies have also shown the effectiveness of antipsychotics in improving auditory hallucinations (34).
Our study has several limitations. First, the results may be limited by the small sample size. Second, due to the exploratory nature of the study, we used uncorrected instead of corrected, as to not inhibit hypothesis generating research by overcorrection for false discovery (35). Third, a prospective, within-subject, counterbalanced drug/placebo design would be preferable to the naturalistic design of the present study, but such a study would not be feasible or ethically justified (36). However, our study is still meaningful and may provide relatively more useful information for longitudinal design and overall analysis.
In conclusion, our study showed changes in the structure, function, and network properties in FES after the antipsychotic treatment and their correlation with clinical symptoms. Our study may help to provide a more complete understanding of the pathological changes in patients with schizophrenia following antipsychotic medication.
The original contributions presented in the study are included in the article/Supplementary Material, further inquiries can be directed to the corresponding author.
The study was approved by the Ethics Committee of Peking University People's Hospital. Written informed consent to participate in this study was provided by the participants' legal guardian/next of kin.
NH: guarantor of integrity of the entire study and manuscript review. PY and NH: study concepts. PY and CZ: study design, definition of intellectual content, clinical studies, and manuscript editing. PY and YL: literature research and data acquisition. PY and XL: experimental studies and data analysis. PY: statistical analysis. PY, LC, and YL: manuscript preparation. All authors contributed to the article and approved the submitted version.
The authors declare that the research was conducted in the absence of any commercial or financial relationships that could be construed as a potential conflict of interest.
All claims expressed in this article are solely those of the authors and do not necessarily represent those of their affiliated organizations, or those of the publisher, the editors and the reviewers. Any product that may be evaluated in this article, or claim that may be made by its manufacturer, is not guaranteed or endorsed by the publisher.
The Supplementary Material for this article can be found online at: https://www.frontiersin.org/articles/10.3389/fpsyt.2021.735623/full#supplementary-material
2. Ibi D, Gonzalez-Maeso J. Epigenetic signaling in schizophrenia. Cell Signal. (2015) 27:2131–6. doi: 10.1016/j.cellsig.2015.06.003
3. Liu Y, Zhang Y, Lv L, Wu R, Zhao J, Guo W. Abnormal neural activity as a potential biomarker for drug-naive first-episode adolescent-onset schizophrenia with coherence regional homogeneity and support vector machine analyses. Schizophr Res. (2018) 192:408–15. doi: 10.1016/j.schres.2017.04.028
4. Zhao C, Zhu J, Liu X, Pu C, Lai Y, Chen L, et al. Structural and functional brain abnormalities in schizophrenia: a cross-sectional study at different stages of the disease. Prog Neuropsychopharmacol Biol Psychiatry. (2018) 83:27–32. doi: 10.1016/j.pnpbp.2017.12.017
5. Zhuo C, Zhu J, Qin W, Qu H, Ma X, Tian H, et al. Functional connectivity density alterations in schizophrenia. Front Behav Neurosci. (2014) 8:404. doi: 10.3389/fnbeh.2014.00404
6. Tarcijonas G, Sarpal DK. Neuroimaging markers of antipsychotic treatment response in schizophrenia: an overview of magnetic resonance imaging studies. Neurobiol Dis. (2019) 131:104209. doi: 10.1016/j.nbd.2018.06.021
7. Del F L, Delvecchio G, D'Agostino A, Brambilla P. Effects of olanzapine during cognitive and emotional processing in schizophrenia: A review of functional magnetic resonance imaging findings. Hum Psychopharmacol. (2019) 34:e2693. doi: 10.1002/hup.2693
8. Wilens T, Zulauf C, Martelon M, Morrison NR, Simon A, Carrellas NW, et al. Nonmedical stimulant use in college students: association with attention-deficit/hyperactivity disorder and other disorders. J Clin Psychiatry. (2016) 77:940–7. doi: 10.4088/JCP.14m09559
9. Kay SR, Fiszbein A, Opler LA. The positive and negative syndrome scale (PANSS) for schizophrenia. Schizophr Bull. (1987) 13:261–76. doi: 10.1093/schbul/13.2.261
10. Vita A, De Peri L, Deste G, Sacchetti E. Progressive loss of cortical gray matter in schizophrenia: a meta-analysis and meta-regression of longitudinal MRI studies. Transl Psychiatry. (2012) 2:e190. doi: 10.1038/tp.2012.116
11. Wright IC, Rabe-Hesketh S, Woodruff PW, David AS, Murray RM, Bullmore ET. Meta-analysis of regional brain volumes in schizophrenia. Am J Psychiatry. (2000) 157:16–25. doi: 10.1176/ajp.157.1.16
12. Dietsche B, Kircher T, Falkenberg I. Structural brain changes in schizophrenia at different stages of the illness: a selective review of longitudinal magnetic resonance imaging studies. Aust N Z J Psychiatry. (2017) 51:500–8. doi: 10.1177/0004867417699473
13. Zarogianni E, Storkey AJ, Johnstone EC, Owens DG, Lawrie SM. Improved individualized prediction of schizophrenia in subjects at familial high risk, based on neuroanatomical data, schizotypal and neurocognitive features. Schizophrenia Res. (2017) 181:6–12. doi: 10.1016/j.schres.2016.08.027
14. Pina-Camacho L, Del RA, Janssen J, Bioque M, Gonzalez-Pinto A, Arango C, et al. Age at first episode modulates diagnosis-related structural brain abnormalities in psychosis. Schizophr Bull. (2016) 42:344–57. doi: 10.1093/schbul/sbv128
15. Nopoulos PC, Ceilley JW, Gailis EA, Andreasen NC. An MRI study of midbrain morphology in patients with schizophrenia: relationship to psychosis, neuroleptics, and cerebellar neural circuitry. Biol Psychiatry. (2001) 49:13–9. doi: 10.1016/S0006-3223(00)01059-3
16. Yu R, Chien YL, Wang HL, Liu CM, Liu CC, Hwang TJ, et al. Frequency-specific alternations in the amplitude of low-frequency fluctuations in schizophrenia. Human Brain Mapping. (2014) 35:627–37. doi: 10.1002/hbm.22203
17. Lui S, Li T, Deng W, Jiang L, Wu Q, Tang H, et al. Short-term effects of antipsychotic treatment on cerebral function in drug-naive first-episode schizophrenia revealed by “resting state” functional magnetic resonance imaging. Arch Gen Psychiatry. (2010) 67:783–92. doi: 10.1001/archgenpsychiatry.2010.84
18. He Z, Deng W, Li M, Chen Z, Jiang L, Wang Q, et al. Aberrant intrinsic brain activity and cognitive deficit in first-episode treatment-naive patients with schizophrenia. Psychol Med. (2013) 43:769–80. doi: 10.1017/S0033291712001638
19. Kuhn S, Gallinat J. Resting-state brain activity in schizophrenia and major depression: a quantitative meta-analysis. Schizophr Bull. (2013) 39:358–65. doi: 10.1093/schbul/sbr151
20. Li T, Wang Q, Zhang J, Rolls E T, Yang W, Palaniyappan L, et al. Brain-wide analysis of functional connectivity in first-episode and chronic stages of schizophrenia. Schizophr Bull. (2017) 43:436–48. doi: 10.1093/schbul/sbw099
21. Wang S, Zhang Y, Lv L, Wu R, Fan X, Zhao J, et al. Abnormal regional homogeneity as a potential imaging biomarker for adolescent-onset schizophrenia: A resting-state fMRI study and support vector machine analysis. Schizophr Res. (2018) 192:179–84. doi: 10.1016/j.schres.2017.05.038
22. Salvador R, Suckling J, Coleman MR, Pickard JD, Menon D, Bullmore E. Neurophysiological architecture of functional magnetic resonance images of human brain. Cerebral Cortex. (2005) 15:1332–42. doi: 10.1093/cercor/bhi016
23. Liu Y, Liang M, Zhou Y, He Y, Hao Y, Song M, et al. Disrupted small-world networks in schizophrenia. Brain. (2008) 131:945–61. doi: 10.1093/brain/awn018
24. Crossley NA, Mechelli A, Scott J, Carletti F, Fox PT, McGuire P, et al. The hubs of the human connectome are generally implicated in the anatomy of brain disorders. Brain. (2014) 137:2382–95. doi: 10.1093/brain/awu132
25. Zhang R, Wei Q, Kang Z, Zalesky A, Li M, Xu Y, et al. Disrupted brain anatomical connectivity in medication-naive patients with first-episode schizophrenia. Brain Struct Funct. (2015) 220:1145–59. doi: 10.1007/s00429-014-0706-z
26. Greenstein D, Lerch J, Shaw P, Clasen L, Giedd J, Gochman P, et al. Childhood onset schizophrenia: cortical brain abnormalities as young adults. J Child Psychol Psychiatry. (2006) 47:1003–12. doi: 10.1111/j.1469-7610.2006.01658.x
27. Moncrieff J, Leo J. A systematic review of the effects of antipsychotic drugs on brain volume. Psychol Med. (2010) 40:1409–22. doi: 10.1017/S0033291709992297
28. Onitsuka T, McCarley RW, Kuroki N, Dickey CC, Kubicki M, Demeo SS, et al. Occipital lobe gray matter volume in male patients with chronic schizophrenia: a quantitative MRI study. Schizophr Res. (2007) 92:197–206. doi: 10.1016/j.schres.2007.01.027
29. Veijola J, Guo JY, Moilanen JS, Jaaskelainen E, Miettunen J, Kyllonen M, et al. Longitudinal changes in total brain volume in schizophrenia: relation to symptom severity, cognition and antipsychotic medication. PLoS ONE. (2014) 9:e101689. doi: 10.1371/journal.pone.0101689
30. Guo JY, Huhtaniska S, Miettunen J, Jaaskelainen E, Kiviniemi V, Nikkinen J, et al. Longitudinal regional brain volume loss in schizophrenia: relationship to antipsychotic medication and change in social function. Schizophr Res. (2015) 168:297–304. doi: 10.1016/j.schres.2015.06.016
31. Guo F, Zhu YQ, Li C, Wang XR, Wang HN, Liu WM, et al. Gray matter volume changes following antipsychotic therapy in first-episode schizophrenia patients: A longitudinal voxel-based morphometric study. J Psychiatr Res. (2019) 116:126–32. doi: 10.1016/j.jpsychires.2019.06.009
32. Deng MY, McAlonan GM, Cheung C, Chiu CP, Law CW, Cheung V, et al. A naturalistic study of grey matter volume increase after early treatment in anti-psychotic naive, newly diagnosed schizophrenia. Psychopharmacology. (2009) 206:437–46. doi: 10.1007/s00213-009-1619-z
33. Hadley JA, Kraguljac NV, White DM, Ver HL, Tabora J, Lahti AC. Change in brain network topology as a function of treatment response in schizophrenia: a longitudinal resting-state fMRI study using graph theory. NPJ Schizophr. (2016) 2:16014. doi: 10.1038/npjschz.2016.14
35. Tolaymat B, Zheng W, Chen H, Choi S, Li X, Dm H. Sex-specific differences in rim appearance of multiple sclerosis lesions on quantitative susceptibility mapping. Mult Scler Relat Disord. (2020) 45:102317. doi: 10.1016/j.msard.2020.102317
Keywords: schizophrenia, first-episode, antipsychotics, neuroimaging, functional magnetic resonance imaging
Citation: Yin P, Zhao C, Li Y, Liu X, Chen L and Hong N (2021) Changes in Brain Structure, Function, and Network Properties in Patients With First-Episode Schizophrenia Treated With Antipsychotics. Front. Psychiatry 12:735623. doi: 10.3389/fpsyt.2021.735623
Received: 13 July 2021; Accepted: 09 November 2021;
Published: 30 November 2021.
Edited by:
Alireza Mohammadi, Baqiyatallah University of Medical Sciences, IranReviewed by:
Felipe Ortuño, University of Navarra, SpainCopyright © 2021 Yin, Zhao, Li, Liu, Chen and Hong. This is an open-access article distributed under the terms of the Creative Commons Attribution License (CC BY). The use, distribution or reproduction in other forums is permitted, provided the original author(s) and the copyright owner(s) are credited and that the original publication in this journal is cited, in accordance with accepted academic practice. No use, distribution or reproduction is permitted which does not comply with these terms.
*Correspondence: Nan Hong, aG9uZ25hbjE5NjhAMTYzLmNvbQ==
†These authors have contributed equally to this work
Disclaimer: All claims expressed in this article are solely those of the authors and do not necessarily represent those of their affiliated organizations, or those of the publisher, the editors and the reviewers. Any product that may be evaluated in this article or claim that may be made by its manufacturer is not guaranteed or endorsed by the publisher.
Research integrity at Frontiers
Learn more about the work of our research integrity team to safeguard the quality of each article we publish.