- 1Psychiatry Neuroimaging Branch, Department of Psychiatry and Psychotherapy, University Medical Center Hamburg-Eppendorf, Hamburg, Germany
- 2Psychiatry Neuroimaging Laboratory, Department of Psychiatry, Brigham and Women's Hospital, Boston, MA, United States
- 3Department of Psychiatry, Massachusetts General Hospital Harvard Medical School, Boston, MA, United States
- 4Department of Radiology, Brigham and Women's Hospital Harvard Medical School, Boston, MA, United States
- 5Center for Psychiatry and Psychotherapy, Justus-Liebig-University Giessen, Giessen, Germany
Objective: Sexual dimorphism has been investigated in schizophrenia, although sex-specific differences among individuals who are at clinical high-risk (CHR) for developing psychosis have been inconclusive. This study aims to characterize sexual dimorphism of language areas in the brain by investigating the asymmetry of four white matter tracts relevant to verbal working memory in CHR patients compared to healthy controls (HC). HC typically show a leftward asymmetry of these tracts. Moreover, structural abnormalities in asymmetry and verbal working memory dysfunctions have been associated with neurodevelopmental abnormalities and are considered core features of schizophrenia.
Methods: Twenty-nine subjects with CHR (17 female/12 male) for developing psychosis and twenty-one HC (11 female/10 male) matched for age, sex, and education were included in the study. Two-tensor unscented Kalman filter tractography, followed by an automated, atlas-guided fiber clustering approach, were used to identify four fiber tracts related to verbal working memory: the superior longitudinal fasciculi (SLF) I, II and III, and the superior occipitofrontal fasciculus (SOFF). Using fractional anisotropy (FA) of tissue as the primary measure, we calculated the laterality index for each tract.
Results: There was a significantly greater right>left asymmetry of the SLF-III in CHR females compared to HC females, but no hemispheric difference between CHR vs. HC males. Moreover, the laterality index of SLF-III for CHR females correlated negatively with Backward Digit Span performance, suggesting a greater rightward asymmetry was associated with poorer working memory functioning.
Conclusion: This study suggests increased rightward asymmetry of the SLF-III in CHR females. This finding of sexual dimorphism in white matter asymmetry in a language-related area of the brain in CHR highlights the need for a deeper understanding of the role of sex in the high-risk state. Future work investigating early sex-specific pathophysiological mechanisms, may lead to the development of novel personalized treatment strategies aimed at preventing transition to a more chronic and difficult-to-treat disorder.
Introduction
Sexual dimorphisms in schizophrenia have been well-documented in a range of areas including symptomatology, treatment response, and outcome. However, one of the most robust findings is the earlier onset of illness in males (1). More specifically, age of onset in males tends to occur in late teens to early 20s, whereas females typically show an onset to illness ~3–5 years later (20–30 years of age), with another period of conversion to illness observed just after the onset of menopause (40–50 years of age) (2, 3). Females have also been shown to respond better to treatment, to recover to a greater degree even in the absence of medication (4), and to show a more benign course of illness (1). Since much of this research has been conducted with individuals with established diagnoses, there is less known about sex-specific differences in those individuals who are at clinical high-risk for developing psychosis (CHR). Given the demonstrated impact of sex on illness course and outcome, the investigation of sex-specific pathologies in CHR individuals could lead to the identification of biomarkers which might be crucial for developing more effective sex-specific therapeutic interventions.
A promising indicator of a CHR state for psychosis is working memory impairments (5–7). Specifically, deficits in verbal working memory have been repeatedly shown to be more severe in CHR individuals who later convert to psychosis compared to those who do not (8, 9). Exploring verbal working memory with imaging (10), and lesion studies (11), reveal a core frontoparietal network of slightly left-lateralized activation, which involves primarily dorsolateral prefrontal and posterior parietal cortices (12). These brain regions are connected via two key white matter association fibers, the superior longitudinal fasciculus (SLF) and the superior occipitofrontal fasciculus (SOFF) (13, 14). White matter maturation and white matter microstructure of the SLF, in particular, have been associated with verbal working memory performance in healthy children, adolescents, and adults (15, 16), as well as in patients with recent-onset schizophrenia (17).
Verbal memory impairments in patients with schizophrenia have also been linked to white matter tract asymmetries. Tract asymmetries in this study are defined as the relative ratio of white matter microstructural measurements, such as fractional anisotropy (FA), a measure of the directionality of white matter fiber bundles, in white matter tracts in the left hemisphere compared to the right hemisphere (18). Since the left and right hemispheres are not identical, such asymmetries can provide important functional information. For example, a left > right asymmetry in several tracts, particularly those of the SLF, has been consistently reported in healthy adults (19, 20) and in the developing human brain (21, 22). However, early stage/course patients with schizophrenia tend to show a stronger rightward asymmetry in the SLF (23). Comparable reductions in left > right asymmetry have also been reported in populations of chronic patients for the SLF (24) and for other components of the frontoparietal network underlying verbal working memory, specifically the SOFF (25), and the inferior fronto-occipital fasciculus (IOFF) (26). Very recently, it has been demonstrated that even the emergence of specific symptoms such as auditory-verbal hallucinations in schizophrenia is related to longer left than right-sided arcuate fasciculus fiber tracts (27). Taken together, there is reliable evidence that individuals with schizophrenia tend to show deviations in hemispheric laterality (i.e., cerebral asymmetry, left hemispheric dominance for language, and handedness) from healthy individuals (28). For instance, several studies reported less left-lateralized language functions (29) as well as a higher rate of non-right-handedness (i.e., mixed- and left-handedness) in schizophrenia patients (30, 31) and their healthy relatives (32, 33). Hence, a genetic link has been suggested between atypical laterality and schizophrenia (34).
While white matter tract asymmetries have also been reported to increase with a longer duration of illness (35), it has been suggested that their origins are the result of neurodevelopmental abnormalities in schizophrenia (24, 36). Accordingly, further investigations of these possible neurodevelopmental asymmetries in those at CHR for developing schizophrenia is needed.
Sex has also been shown to be a major influence on the development of white matter tracts (37, 38). For example, males show a later onset in the maturation of white matter tracts than do females (39, 40). Moreover, this finding has been linked to an earlier onset of pubertal maturation in females (~2–3 years earlier) (41). However, the role of sex on white matter tract asymmetries in verbal working memory in CHR individuals is not as yet known. Demonstrating such sex-specific neurodevelopmental disturbances in the working memory-relevant white matter network of CHR individuals may be central to understanding the emergence of psychosis symptomatology.
Given that verbal working memory deficits have been shown in patients with schizophrenia and are a promising symptom criterion for CHR conversion to psychosis, we investigated possible alterations in sexually dimorphic asymmetry in four white matter tracts in CHR for psychosis individuals compared to healthy controls (HC). The tracts of interest were central components of the verbal working memory circuit, including the SLF with its three subcomponents (SLF-I, II and III), in addition to the SOFF. In this study, we employed a novel data-driven fiber clustering approach based on diffusion magnetic resonance imaging (dMRI), and an anatomically curated white matter fiber clustering atlas (42). We hypothesized that: (1) CHR females show a greater rightward asymmetry of SLF, and (2) that these alterations would correlate with verbal working memory dysfunctions.
Materials and Methods
Ethics Statement
The present study was part of a larger ongoing project within the context of the Collaborative Research Center 936 (“multi-site communication in the brain,” www.sfb936.net) investigating functional and structural connectivity in schizophrenia. Parts of this data were already published (43). The study was approved by the Ethics Committee of the Medical Association Hamburg and was performed in accordance with the seventh version of the Declaration of Helsinki ethical standards. All participants provided written informed consent (or in case of minors from their respective legal guardian).
Participants
Our participant sample consisted of 29 right-handed individuals at CHR for psychosis (12 men, mean age 21.00 ± 4.14, 17 women, mean age 20.71 ± 3.29) and 25 HC. The participants' handedness was verified with the empirically validated Edinburgh Handedness Inventory (44). Four HCs were excluded, two as a result of severe signal dropouts, one as a result of presence of a hydrocephalus, and one as a result of sex transformation, resulting in 21 HC (10 men, mean age 23.00 ± 4.85, 11 women, mean age 22.55 ± 3.53). The exclusion criteria for all participants were defined as follows: (1) presence of major neurological disorder or head injury, (2) current substance abuse or dependence, (3) intellectual disability (IQ <70), and (4) left-handedness. Additionally, HCs were excluded if they had a (5) history of psychotic disorders or if they had been (6) previously diagnosed with any psychiatric disorder. The screening for eligibility was carried out in the form of a semi-structured interview by a clinical psychiatrist or psychiatry resident with a minimum of 4 years of clinical experience.
The inclusion criteria for CHR for psychosis were defined in line with the criteria of the Early Detection and Intervention Program of the German Research Network on Schizophrenia (GNRS) (45), including the following: presence of either (1) basic symptoms syndrome (BSS), (2) a schizotypal personality disorder/ plus a decline in global functioning (GRD), (3) attenuated psychotic symptoms (APS), or a brief limited intermittent psychotic syndrome (BLIPS). Sociodemographic and clinical data for 29 CHR individuals and 21 HC are presented for females and males separately in Table 1.
Cognitive and Clinical Assessments
All CHR individuals underwent the Mini Neuropsychiatric Interview (46) to record current psychiatric comorbidities and to ensure that CHR subjects had no schizophrenia spectrum disorder in the past. Psychopathology in CHR was assessed with the Positive and Negative Syndrome Scale (PANSS) (47) and the Scale of Prodromal Symptoms (SOPS) (48). PANSS scores were derived for positive, negative, disorganization, excitement and distress factors from the scale (49). Participants also underwent neuropsychological testing with an extensive battery including tests of memory, attention and executive functioning. Given this study's purpose, we focused on the measures relevant to verbal working memory, such as the Digit Span Backwards Task and the Letter-Number Sequencing Test of the Wechsler Adult Intelligence Scale (50). Neurocognitive performance data were available from 20 HC and 27 CHR (see Table 1).
Diffusion Weighted Images
All images were obtained using a Siemens 3T scanner (Magnetom Trio) at the University Medical Center Hamburg-Eppendorf with a 12-channel head coil. Diffusion-weighted images (DWI) were acquired using an echo-planar imaging sequence (EPI) with the following parameters: TR = 7.7 s, TE = 85 ms, flip angle = 90°, 32 interleaved slices, slice thickness = 2 mm, field of view (FOV) = 216 × 256 mm2 matrix = 108 × 128, 30 non-colinear gradient directions with b = 1,000 s/mm2 collected twice and 10 images with b = 0 s/mm2. The quality control steps comprised visual inspection of the images for movement artifacts, EPI distortions and structural abnormalities using 3D Slicer (software version 4.5; www.slicer.org) (51). Gradient directions that showed severe artifacts or signal dropouts were removed after a careful quality control process. All scans were corrected for motion and eddy currents by affine registration of all volumes to the first B0 of the acquisition using the Linear Image Registration Tool (FLIRT), provided in the Oxford Center for Functional MRI of the Brain Software Library (FSL, http://fsl.fmrib.ox.ac.uk/fsl/). The gradient vector table was updated accordingly. A relative motion parameter was obtained for each scan from the transforms by calculating the average displacement between adjacent volumes. Finally, brain masks for skull stripping were generated automatically within 3D Slicer using Otsu's method and then manually edited to ensure anatomical accuracy. After preprocessing, each gradient was averaged from both acquisitions using an in-house developed script (52).
Unscented Kalman Filter Tractography
Next, whole brain tractography was computed using the unscented Kalman filter tractography method (UKFt), as implemented in the open-source software package (https://github.com/pnlbwh/ukftractography). The UKFt fits a mixture model of compartments to the dMRI data while tracking fibers, taking advantage of information from the previous step along the fiber to help stabilize model fitting (53, 54). UKFt has been shown to be highly consistent in fiber tracking across age, state of health, and image acquisition (42), and superior than standard single-tensor tractography, in particular in the presence of crossing fibers and edema (55). In this study, we used a three-compartment model including two tensor compartments to model the fiber being tracked, and a potential additional fiber crossing it, and a third compartment of an isotropic tensor with diffusivity equal to that of extracellular water. The third compartment is aimed at modeling partial volume with cerebrospinal fluid (CSF) and other freely diffusing extracellular water pools (56, 57). The fibers were quantified using FA of tissue of the principal compartment (the one driving the direction of the UKFt), which is inherently corrected for crossing fibers or for partial volume with free-water. Tractography was seeded with 10 seeds per voxel in all voxels within the binary brain mask where a DTI fit yielded FA >0.08. Tracking stopped where the FA value of the principal compartment fell below 0.08, or the generalized anisotropy fell below 0.08.
Automatic Identification of SOFF, SLF-I, SLF-II, and SLF-III
After performing whole-brain tractography using the UKFt + FW method, automated fiber parcellation was performed using tract registration and clustering methods (58, 59). Subject-specific bilateral SOFF, SLF-I, SLF-II, and SLF-III clusters were automatically identified according to the anatomically curated fiber clustering atlas, provided by the O'Donnell Research Group (ORG) (42) (http://dmri.slicer.org/atlases/). Recent studies have shown that this fiber clustering approach enables reliable fiber quantification and three-dimensional visualization (42, 60–62). The ORG atlas is based on 100 subjects, containing 800 cluster parcellations of the entire brain white matter and an anatomical tract parcellation that defines 58 deep white matter tracts, as well as 198 short and medium range superficial fiber clusters (42, 59, 63). For clustering, each fiber is compared to a variety of fibers in the atlas, giving a feature vector or “fingerprint” that is used to classify the fiber into a cluster. The fiber clustering result of one SLF-III tract is shown in Figure 1. To provide sufficient data quality, we excluded data from subsequent analysis for any tract with fewer than five streamlines. Finally, mean FA values were extracted for each fiber tract and exported to SPSS. All software used is publicly available, including computational tractography, fiber cluster analysis (https://github.com/SlicerDMRI/whitematteranalysis), as well as tractography visualization with anatomical hierarchies in 3D Slicer via the SlicerDMRI project (dmri.slicer.org) (64, 65).
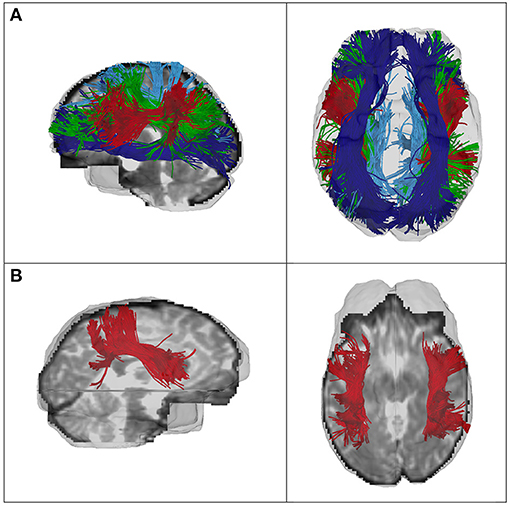
Figure 1. (A) Fiber clustering results of the SLF-I (light blue), SLF-II (green), SLF-III (red), and SOFF (dark blue) of one CHR female, and (B) of the SLF-III of one HC female. Left column, sagittal view; right column, axial view.
Laterality Index of SOFF, SLF-I, SLF-II, and SLF-III
Next, hemispheric asymmetry was calculated for all four tracts of interest using the laterality index (LI) based on the following formula: LI = (Right FA – Left FA)/(Right FA + Left FA) of the average FA across each tract. This index yields positive values for right-lateralized, negative values for left-lateralized white matter tracts, and zero values for absolute symmetry.
Statistical Analysis
Group differences were compared by means of independent t-tests or Mann-Whitney-U-tests (depending on normal distribution and corrected for multiple comparison for demographic, clinical and behavioral data).
A linear mixed-effects (LMEs) model was used to analyse the effects of group, sex, white matter tracts and any group-related interactions on LI. LMEs have several advantages over traditional repeated-measures designs, as they can accommodate departures from assumptions of homogeneity of regression slopes and independence, and thus are better suited to model inter-individual variability (66). Moreover, LMEs are able to handle an unequal number of within-subject data (67), which is particularly important in the current study as we excluded one subject's white matter tract (i.e., SOFF) due to an insufficient number of streamlines. In this study, the model included Group (2 levels: CHR and HC), White Matter Tracts (4 levels: SOFF, SLF-I, SLF-II and SLF-III), and Sex (2 levels: female and male) as the fixed-effects factors, Subject as a random-effects factor (to account for within-subject variability), and FA-based LI as the dependent variable. To capture effects of possible confounding variables, our model also included age, years of education and their respective interaction with Group as fixed-effects. All the analyses were performed with SPSS version 25 (IBM SPSS Statistics for Macintosh).
Results
Sociodemographic and Clinical Variables
Sociodemographic and clinical variables of the sample are shown in Table 1. No differences were observed between CHR and HC in age, sex and years of education (all p > 0.09), nor between the sex-specific subgroups (all p > 0.15). Additionally, there were no significant differences in PANSS sub-scores, nor in SOPS sub-scores, between CHR males and CHR females (all p > 0.13). There were also no significant differences between group (CHR or HC) and gender (male or female) in working memory performances (all p > 0.15).
Sex-Related Differences in Asymmetry of White Matter Tracts
The interaction of Group × Sex had a significant effect on LI [F(1, 48.574) = 4.099, p = 0.048], indicating that there are sex-related differences in terms of hemispheric asymmetry across the two groups (i.e., HC vs. CHR). Specifically, subsequent post-hoc pairwise comparisons within each group (HC or CHR) revealed a greater hemispheric asymmetry in healthy males than healthy females, but that did not reach the significance threshold (p = 0.051, Cohen's d = 0.35). There was no such difference between CHR males and CHR females (p = 0.45). Moreover, post-hoc pairwise comparisons within each sex demonstrated a significantly different LI between HC and CHR females (p = 0.008, Cohen's d = 0.49), but not between HC and CHR males (p = 0.91). To determine which tract was driving the effect in females, post-hoc pairwise comparisons between each white matter tract's LI revealed a significantly greater rightward asymmetry of the SLF-III in CHR females compared to HC females (p = 0.012, Cohen's d = 0.92) (Figure 2). There was no significant difference in any of the other three white matter tracts (all p > 0.1).
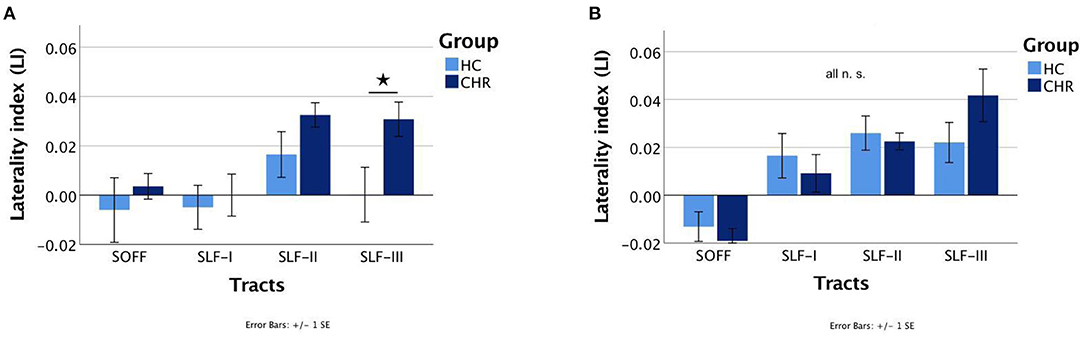
Figure 2. Mean laterality indices of the superior occipitofrontal fasciculi (SOFF) and the superior longitudinal fasciculi (SLF) I, II, and III for healthy controls (HC) and individuals at clinical high-risk (CHR). The significant different laterality index of the SLF-III between HC and CHR females is marked with an asterisk. (A) Females and (B) Males. N.S., not significant.
Associations Between SLF-III Asymmetry and Verbal Working Memory
Correlation analyses were performed for SLF-III, which was the only tract with a significant group effect in hemispheric laterality. In CHR females, the laterality index of SLF-III correlated negatively with Digit Span Backwards performance (two-tailed Spearman's rho = −0.520, p = 0.047), indicating that in females, a greater degree of rightward asymmetry was related to poorer performance (Figure 3). No significant correlations were found in HC males, HC females, or CHR males.
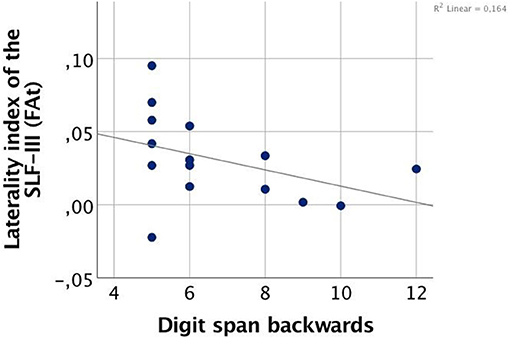
Figure 3. Correlation between FA-based laterality index of the superior longitudinal fasciculus (SLF) III and working memory performance in clinical high-risk females (CHR).
Discussion
In this study, we employed a state-of-the-art fiber clustering method to investigate the impact of sex on hemispheric white matter asymmetries within a verbal working memory circuitry in a population of individuals at CHR and matched HC. We observed a greater right > left asymmetry of the SLF-III in CHR females only. Further, we found that the greater degree of rightward asymmetry in the CHR females was related to poorer performance in the Digit Span Backwards Task of the WAIS. This relationship was not observed in the male CHR group nor in the male or female HC groups. Thus, this study reports an association between a putative sex-specific neurodevelopmental disturbance in the frontoparietal network and verbal working memory in female CHR individuals.
Our primary finding of aberrant white matter asymmetry in the SLF-III in female CHR individuals aligns well with earlier studies reporting decreased left > right asymmetry in first-episode and chronic patients with schizophrenia (23–26, 35). In this study, we chose to focus on white matter tract asymmetry in a frontoparietal network proposed to underlie verbal working memory because both have been previously considered core features of schizophrenia pathology (68, 69). For example, a reduction of the typical left > right asymmetry has been shown for frontoparietal tracts, such as the SLF (23, 24), the SOFF (25), and the IOFF (26) in patients with chronic schizophrenia.
Moreover, abnormalities within the SLF have been the most frequently reported alterations in CHR individuals (17, 70, 71), suggesting that alterations in the SLF precede illness onset. As stated previously, changes in white matter asymmetry have been associated with aberrant white matter development (24). Of further note, the SLF is one of the most dynamically changing white matter tracts from childhood to early adulthood (72). There is also evidence that the FA values of the SLF increase substantially between 8 and 22 years of age (73) and peak on average between 15 and 20 years of age (74) in healthy individuals. As this period overlaps with the onset of puberty, many have investigated the possible role that sex, as well as pubertal hormones, may have on the maturation of white matter (38, 75). Indeed, it has been consistently reported that white matter growth during puberty and adolescence follows a striking sexual dimorphism: age-related increases in white matter occur much more steeply in boys when compared to girls (76, 77). This faster degree of growth has been shown to be driven—at least in part—by testosterone in boys, but not in girls (38). Girls, on the other hand, show a negative relationship between estradiol and FA (37). In our sample, the average age of our CHR females is 21 years of age, placing them past the previously reported maturational peak for the SLF. When taken together, these findings suggest that our finding of a greater right > left asymmetry of the SLF-III in young adult CHR females only, might reflect a sex-specific deviation in white matter maturation and early development.
However, even in the presence of significantly altered white matter asymmetry, the CHR females in our sample still performed at a similar level to the female and male controls in the Digit Span Backwards task. This finding is also particularly interesting in light of the inverse relationship we observed between task performance and the degree of asymmetry in the CHR females. The Digit Span Backwards Task measures verbal working memory, by requiring temporary storage and manipulation of verbal information no longer available in the external environment (78). In the literature, worse performance within the Digit Span Backwards Task has been reported in first-degree relatives (79) and in patients with schizophrenia (80) compared to healthy controls. However, these studies tend not to control for sex, or they have limited power to test for sex differences. When taking sex into account, female patients perform better than males in the digit symbol subtest (81) and show, in general, higher levels of functioning in language, and in executive and memory domains (1).
Our study shows a similar behavioral trend, yet interestingly, we observed that female CHR individuals also exhibit greater structural differences than their male CHR counterparts. Though speculative, our finding of a greater right > left asymmetry of the SLF-III in CHR females may reflect a compensatory mechanism. Other studies have also described potential compensatory mechanisms in the brain of individuals with psychosis or of healthy controls at higher age (i.e., range: 64–78), while few have investigated the role that sex may play. For example, Di Biase and colleagues suggest that increases in FA in early stage psychosis patients may compensate for cortical thinning that occurs with longer durations of illness (82). Similarly, neural and cognitive decline in elderly subjects are thought to be compensated for by recruitment of homologous brain areas in the contralateral hemisphere (83). For example, the typical left-lateralized verbal memory network in young adults begin to appear more bilateral with increased age (84). Accordingly, we propose that the greater right > left asymmetry of the SLF-III of CHR females may be the result of developmental compensation for compromised neural processing in the left hemisphere in the female CHR individuals.
Moreover, we found no significant difference in white matter asymmetry in CHR males compared to HC males, which aligns well with another DTI study which also reports no significant white matter asymmetry differences in ultra-high-risk males who later developed psychosis (85). Thus, overall, hemispheric volume is a sexually dimorphic trait, i.e., the male brain tends to be more asymmetric than the female brains, a pattern replicated in healthy controls in the present study.
Concerning methodological aspects of the study, the present study demonstrates the efficacy and sensitivity of using and automatically annotating fiber clustering method for white matter tract parcellation across different populations. This method has been increasingly and successfully applied to clinical studies (42, 60, 61) and is shown to be superior to cortical-parcellation-based methods (62).
Several potential limitations of our study should be mentioned. Due to difficulty in recruiting CHR individuals, the sample size is not large, especially considering the sex-specific subgroups, thus limiting statistical power. This translates into another limitation concerning our lack of findings with respect to correlations with prodromal symptoms. It would have been promising to find a correlation between altered laterality and specific symptoms. Single shell data is another limitation. Multi-shell data would make the multi-compartmental fit more robust (86). Lastly, while our data are part of a longitudinal study with 1-year follow-up interviews, only four females converted to a first-episode of psychosis, which limits our ability to make predictions about conversion to psychosis.
Finally, one empirical next step to elucidate the association between a putative sex-specific neurodevelopmental disturbance in the frontoparietal network and verbal working memory dysfunction in the CHR state might be the inclusion of a sufficient number of individuals with a schizotypal tendency. In schizophrenia, as well as in individuals with schizotypy, atypical brain laterality is considered a potential underlying basis for the excess of non-right-handedness (i.e., mixed- and left-handedness) (87). Further, it has been shown that higher scores on schizotypy questionnaires have been previously able to predict conversion to psychosis in CHR subjects (88). Although our inclusion criteria involved schizotypal personality disorder, only one male was recruited. Therefore, we are not able to address this question in the present analysis.
In conclusion, the present study demonstrates that CHR females show greater rightward asymmetry of the SLF-III which we believe highlights the underappreciated role of sex in understanding white matter asymmetry and alterations in the pathophysiology of a high-risk state for developing psychosis. Thus, improving our understanding of both the sexual dimorphism of white matter asymmetry and the factors that influence these neurodevelopmental maturational processes, as well as the factors they influence, such as clinical and cognitive symptoms, may help to promote the development of novel interventions aiming to prevent transition into a chronic and difficult-to-treat disorder.
Data Availability Statement
The raw data supporting the conclusions of this article will be made available by the authors, without undue reservation.
Ethics Statement
The studies involving human participants were reviewed and approved by Ethik-Kommission - Ärztekammer Hamburg. The patients/participants provided their written informed consent to participate in this study.
Author Contributions
CM and SS designed the study. JR, FN, and MM collected data. SS and ML analyzed data. TB, YR, and SC-K wrote codes. AL, GL, MK, FZ, LO'D, OP, and MS gave methodological support. SS, CM, and MS supervised the project. SS and AL worte the paper. All authors discussed the results and commented on the manuscript at all stages.
Funding
This research was supported by the SFB 936 C6 (grant holder are GL and CM) of the German Research Foundation, and the Human Connectome Project to MS (2016P000764). SS was supported by a grant from the German Academic Exchange Service (DAAD). AL was supported by the K01 MH108574 as well as by the NARSAD Young Investigator Award. FN was supported by the Claussen-Simon-Stiftung. LO'D and FZ were supported by the NAC (P41 EB015902), R01 MH119222, U01 CA199459, and NCIGT (P41 EB015898).
Conflict of Interest
The authors declare that the research was conducted in the absence of any commercial or financial relationships that could be construed as a potential conflict of interest.
Acknowledgments
The authors wish to thank all the volunteers who participated in the study.
References
1. Ochoa S, Usall J, Cobo J, Labad X, Kulkarni J. Gender differences in schizophrenia and first-episode psychosis: a comprehensive literature review. Schizophr Res Treatment. (2012) 2012:916198. doi: 10.1155/2012/916198
2. Eranti SV, MacCabe JH, Bundy H, Murray RM. Gender difference in age at onset of schizophrenia: a meta-analysis. Psychol Med. (2013) 43:155–67. doi: 10.1017/S003329171200089X
3. Häfner H. Gender differences in schizophrenia. Psychoneuroendocrinology. (2003) 28:17–54. doi: 10.1016/S0306-4530(02)00125-7
4. Wils RS, Gotfredsen DR, Hjorthoj C, Austin SF, Albert N, Secher RG, et al. Antipsychotic medication and remission of psychotic symptoms 10years after a first-episode psychosis. Schizophr Res. (2017) 182:42–8. doi: 10.1016/j.schres.2016.10.030
5. Correll CU, Hauser M, Auther AM, Cornblatt BA. Research in people with psychosis risk syndrome: a review of the current evidence and future directions. J Child Psychol Psychiatry. (2010) 51:390–431. doi: 10.1111/j.1469-7610.2010.02235.x
6. Pukrop R, Ruhrmann S, Schultze-Lutter F, Bechdolf A, Brockhaus-Dumke A, Klosterkotter J. Neurocognitive indicators for a conversion to psychosis: comparison of patients in a potentially initial prodromal state who did or did not convert to a psychosis. Schizophr Res. (2007) 92:116–25. doi: 10.1016/j.schres.2007.01.020
7. Reichenberg A, Caspi A, Harrington H, Houts R, Keefe RS, Murray RM, et al. Static and dynamic cognitive deficits in childhood preceding adult schizophrenia: a 30-year study. Am J Psychiatry. (2010) 167:160–9. doi: 10.1176/appi.ajp.2009.09040574
8. De Herdt A, Wampers M, Vancampfort D, De Hert M, Vanhees L, Demunter H, et al. Neurocognition in clinical high risk young adults who did or did not convert to a first schizophrenic psychosis: a meta-analysis. Schizophr Res. (2013) 149:48–55. doi: 10.1016/j.schres.2013.06.017
9. Giuliano AJ, Li H, Mesholam-Gately RI, Sorenson SM, Woodberry KA, Seidman LJ. Neurocognition in the psychosis risk syndrome: a quantitative and qualitative review. Curr Pharm Des. (2012) 18:399–415. doi: 10.2174/138161212799316019
10. Rottschy C, Langner R, Dogan I, Reetz K, Laird AR, Schulz JB, et al. Modelling neural correlates of working memory: a coordinate-based meta-analysis. Neuroimage. (2012) 60:830–46. doi: 10.1016/j.neuroimage.2011.11.050
11. Muller NG, Knight RT. The functional neuroanatomy of working memory: contributions of human brain lesion studies. Neuroscience. (2006) 139:51–8. doi: 10.1016/j.neuroscience.2005.09.018
12. Cabeza R, Nyberg L. Imaging cognition II: an empirical review of 275 PET and fMRI studies. J Cogn Neurosci. (2000) 12:1–47. doi: 10.1162/08989290051137585
13. Makris N, Kennedy DN, McInerney S, Sorensen AG, Wang R, Caviness S Jr, et al. Segmentation of subcomponents within the superior longitudinal fascicle in humans: a quantitative, in vivo, DT-MRI study. Cereb Cortex. (2005) 15:854–69. doi: 10.1093/cercor/bhh186
14. Makris N, Papadimitriou GM, Sorg S, Kennedy DN, Caviness VS, Pandya DN. The occipitofrontal fascicle in humans: a quantitative, in vivo, DT-MRI study. Neuroimage. (2007) 37:1100–11. doi: 10.1016/j.neuroimage.2007.05.042
15. Burzynska AZ, Nagel IE, Preuschhof C, Li SC, Lindenberger U, Backman L, et al. Microstructure of frontoparietal connections predicts cortical responsivity and working memory performance. Cereb Cortex. (2011) 21:2261–71. doi: 10.1093/cercor/bhq293
16. Ostby Y, Tamnes CK, Fjell AM, Walhovd KB. Morphometry and connectivity of the fronto-parietal verbal working memory network in development. Neuropsychologia. (2011) 49:3854–62. doi: 10.1016/j.neuropsychologia.2011.10.001
17. Karlsgodt KH, van Erp TG, Poldrack RA, Bearden CE, Nuechterlein KH, Cannon TD. Diffusion tensor imaging of the superior longitudinal fasciculus and working memory in recent-onset schizophrenia. Biol Psychiatry. (2008) 63:512–8. doi: 10.1016/j.biopsych.2007.06.017
18. Gomez-Gastiasoro A, Zubiaurre-Elorza L, Pena J, Ibarretxe-Bilbao N, Rilo O, Schretlen DJ, et al. Altered frontal white matter asymmetry and its implications for cognition in schizophrenia: a tractography study. Neuroimage Clin. (2019) 22:101781. doi: 10.1016/j.nicl.2019.101781
19. Powell HW, Parker GJ, Alexander DC, Symms MR, Boulby PA, Wheeler-Kingshott CA, et al. Hemispheric asymmetries in language-related pathways: a combined functional MRI and tractography study. Neuroimage. (2006) 32:388–99. doi: 10.1016/j.neuroimage.2006.03.011
20. Takao H, Hayashi N, Ohtomo K. White matter microstructure asymmetry: effects of volume asymmetry on fractional anisotropy asymmetry. Neuroscience. (2013) 231:1–12. doi: 10.1016/j.neuroscience.2012.11.038
21. Beaulieu C, Plewes C, Paulson LA, Roy D, Snook L, Concha L, et al. Imaging brain connectivity in children with diverse reading ability. Neuroimage. (2005) 25:1266–71. doi: 10.1016/j.neuroimage.2004.12.053
22. Bonekamp D, Nagae LM, Degaonkar M, Matson M, Abdalla WM, Barker PB, et al. Diffusion tensor imaging in children and adolescents: reproducibility, hemispheric, age-related differences. Neuroimage. (2007) 34:733–42. doi: 10.1016/j.neuroimage.2006.09.020
23. Bora E, Fornito A, Radua J, Walterfang M, Seal M, Wood SJ, et al. Neuroanatomical abnormalities in schizophrenia: a multimodal voxelwise meta-analysis and meta-regression analysis. Schizophr Res. (2011) 127:46–57. doi: 10.1016/j.schres.2010.12.020
24. Park HJ, Westin CF, Kubicki M, Maier SE, Niznikiewicz M, Baer A, et al. White matter hemisphere asymmetries in healthy subjects and in schizophrenia: a diffusion tensor MRI study. Neuroimage. (2004) 23:213–23. doi: 10.1016/j.neuroimage.2004.04.036
25. Kunimatsu N, Aoki S, Kunimatsu A, Yoshida M, Abe O, Yamada H, et al. Tract-specific analysis of the superior occipitofrontal fasciculus in schizophrenia. Psychiatry Res. (2008) 164:198–205. doi: 10.1016/j.pscychresns.2008.03.004
26. Miyata J, Sasamoto A, Koelkebeck K, Hirao K, Ueda K, Kawada R, et al. Abnormal asymmetry of white matter integrity in schizophrenia revealed by voxelwise diffusion tensor imaging. Hum Brain Mapp. (2012) 33:1741–9. doi: 10.1002/hbm.21326
27. Falkenberg LE, Westerhausen R, Johnsen E, Kroken R, Loberg EM, Beresniewicz J, et al. Hallucinating schizophrenia patients have longer left arcuate fasciculus fiber tracks: a DTI tractography study. Psychiatry Res Neuroimaging. (2020) 302:111088. doi: 10.1016/j.pscychresns.2020.111088
28. Sommer I, Ramsey N, Kahn R, Aleman A, Bouma A. Handedness, language lateralisation and anatomical asymmetry in schizophrenia: meta-analysis. Br J Psychiatry. (2001) 178:344–51. doi: 10.1192/bjp.178.4.344
29. Ocklenburg S, Gunturkun O, Hugdahl K, Hirnstein M. Laterality and mental disorders in the postgenomic age–A closer look at schizophrenia and language lateralization. Neurosci Biobehav Rev. (2015) 59:100–10. doi: 10.1016/j.neubiorev.2015.08.019
30. Dragovic M, Hammond G. Handedness in schizophrenia: a quantitative review of evidence. Acta Psychiatr Scand. (2005) 111:410–9. doi: 10.1111/j.1600-0447.2005.00519.x
31. Hirnstein M, Hugdahl K, Hausmann M. Cognitive sex differences and hemispheric asymmetry: a critical review of 40 years of research. Laterality. (2019) 24:204–52. doi: 10.1080/1357650X.2018.1497044
32. Orr KG, Cannon M, Gilvarry CM, Jones PB, Murray RM. Schizophrenic patients and their first-degree relatives show an excess of mixed-handedness. Schizophr Res. (1999) 39:167–76. doi: 10.1016/S0920-9964(99)00071-7
33. Shen DM, Zhang F, Brady EJ, Candelore MR, Dallas-Yang Q, Ding VD, et al. Discovery of novel, potent, and orally active spiro-urea human glucagon receptor antagonists. Bioorg Med Chem Lett. (2005) 15:4564–9. doi: 10.1016/j.bmcl.2005.06.101
34. Crow TJ. The XY gene hypothesis of psychosis: origins and current status. Am J Med Genet B Neuropsychiatr Genet B. (2013) 162:800–24. doi: 10.1002/ajmg.b.32202
35. Ribolsi M, Daskalakis ZJ, Siracusano A, Koch G. Abnormal asymmetry of brain connectivity in schizophrenia. Front Hum Neurosci. (2014) 8:1010. doi: 10.3389/fnhum.2014.01010
36. Crow TJ, Paez P, Chance SA. Callosal misconnectivity and the sex difference in psychosis. Int Rev Psychiatry. (2007) 19:449–57. doi: 10.1080/09540260701486282
37. Herting MM, Maxwell EC, Irvine C, Nagel BJ. The impact of sex, puberty, and hormones on white matter microstructure in adolescents. Cereb Cortex. (2012) 22:1979–92. doi: 10.1093/cercor/bhr246
38. Perrin JS, Herve PY, Leonard G, Perron M, Pike GB, Pitiot A, et al. Growth of white matter in the adolescent brain: role of testosterone and androgen receptor. J Neurosci. (2008) 28:9519–24. doi: 10.1523/JNEUROSCI.1212-08.2008
39. Asato MR, Terwilliger R, Woo J, Luna B. White matter development in adolescence: a DTI study. Cereb Cortex. (2010) 20:2122–31. doi: 10.1093/cercor/bhp282
40. Wang Y, Adamson C, Yuan W, Altaye M, Rajagopal A, Byars AW, et al. Sex differences in white matter development during adolescence: a DTI study. Brain Res. (2012) 1478:1–5. doi: 10.1016/j.brainres.2012.08.038
41. Sisk CL, Foster DL. The neural basis of puberty and adolescence. Nat Neurosci. (2004) 7:1040–7. doi: 10.1038/nn1326
42. Zhang F, Wu Y, Norton I, Rigolo L, Rathi Y, Makris N, et al. An anatomically curated fiber clustering white matter atlas for consistent white matter tract parcellation across the lifespan. Neuroimage. (2018) 179:429–47. doi: 10.1016/j.neuroimage.2018.06.027
43. Nagele FL, Pasternak O, Bitzan LV, Mussmann M, Rauh J, Kubicki M, et al. Cellular and extracellular white matter alterations indicate conversion to psychosis among individuals at clinical high-risk for psychosis. World J Biol Psychiatry. (2020) 1–14. doi: 10.1080/15622975.2020.1775890. [Epub ahead of print].
44. Oldfield RC. The assessment and analysis of handedness: the Edinburgh inventory. Neuropsychologia. (1971) 9:97–113. doi: 10.1016/0028-3932(71)90067-4
45. Wolwer W, Baumann A, Bechdolf A, Buchkremer G, Häfner H, Janssen B, et al. The German research network on schizophrenia–impact on the management of schizophrenia. Dialogues Clin Neurosci. (2006) 8:115–21. doi: 10.31887/DCNS.2006.8.1/wwoelwer
46. Sheehan DV, Lecrubier Y, Sheehan KH, Amorim P, Janavs J, Weiller E, et al. The Mini-International Neuropsychiatric Interview (M.I.N.I.): the development and validation of a structured diagnostic psychiatric interview for DSM-IV and ICD-10. J Clin Psychiatry. (1998) 59(Suppl 20):22–33.
47. Kay SR, Fiszbein A, Opleret LA. The positive and negative syndrome scale (PANSS) for schizophrenia. Schizophr Bull. (1987) 13:261–76.
48. Miller TJ, McGlashan TH, Rosen JL, Cadenhead K, Cannon T, Ventura J, et al. Prodromal assessment with the structured interview for prodromal syndromes and the scale of prodromal symptoms: predictive validity, interrater reliability, and training to reliability. Schizophr Bull. (2003) 29:703–15. doi: 10.1093/oxfordjournals.schbul.a007040
49. van der Gaag M, Cuijpers A, Hoffman T, Remijsen M, Hijman R, de Haan L, et al. The five-factor model of the positive and negative syndrome scale I: confirmatory factor analysis fails to confirm 25 published five-factor solutions. Schizophr Res. (2006) 85:273–9. doi: 10.1016/j.schres.2006.04.001
50. Wechsler D. Wechsler Adult Intelligence Scale. 3rd ed. San Antonio, TX: The Psychological Corporation (1997).
51. Fedorov A, Beichel R, Kalpathy-Cramer J, Finet J, Fillion-Robin J-C, Pujol S, et al. 3D Slicer as an image computing platform for the Quantitative Imaging Network. Magn Reson Imaging. (2012) 30:1323–41. doi: 10.1016/j.mri.2012.05.001
52. Billah T, Bouix S, Rathi Y. Various MRI Conversion Tools. Available online at: https://githubcom/pnlbwh/conversion (2019).
53. Chen Z, Tie Y, Olubiyi O, Rigolo L, Mehrtash A, Norton I, et al. Reconstruction of the arcuate fasciculus for surgical planning in the setting of peritumoral edema using two-tensor unscented Kalman filter tractography. Neuroimage Clin. (2015) 7:815–22. doi: 10.1016/j.nicl.2015.03.009
54. Malcolm JG, Shenton ME, Rathi Y. Filtered multitensor tractography. IEEE Trans Med Imaging. (2010) 29:1664–75. doi: 10.1109/TMI.2010.2048121
55. Liao R, Ning L, Chen Z, Rigolo L, Gong S, Pasternak O, et al. Performance of unscented Kalman filter tractography in edema: analysis of the two-tensor model. Neuroimage Clin. (2017) 15:819–31. doi: 10.1016/j.nicl.2017.06.027
56. Pasternak O, Sochen N, Gur Y, Intrator N, Assaf Y. Free water elimination and mapping from diffusion MRI. Magn Reson Med. (2009) 62:717–30. doi: 10.1002/mrm.22055
57. Tang Y, Pasternak O, Kubicki M, Rathi Y, Zhang T, Wang J, et al. Altered cellular white matter but not extracellular free water on diffusion MRI in individuals at clinical high risk for psychosis. Am J Psychiatry. (2019) 176:820–28. doi: 10.1176/appi.ajp.2019.18091044. [Epub ahead of print].
58. O'Donnell LJ, Wells WM III, Golby AJ, Westin CF. Unbiased groupwise registration of white matter tractography. Med Image Comput Comput Assist Interv. (2012) 15:123–30. doi: 10.1007/978-3-642-33454-2_16
59. O'Donnell LJ, Westin CF. Automatic tractography segmentation using a high-dimensional white matter atlas. IEEE Transact Med Imaging. (2007) 26:1562–75. doi: 10.1109/TMI.2007.906785
60. Sydnor VJ, Rivas-Grajales AM, Lyall AE, Zhang F, Bouix S, Karmacharya S, et al. A comparison of three fiber tract delineation methods and their impact on white matter analysis. Neuroimage. (2018) 178:318–31. doi: 10.1016/j.neuroimage.2018.05.044
61. Wu Y, Zhang F, Makris N, Ning Y, Norton I, She S, et al. Investigation into local white matter abnormality in emotional processing and sensorimotor areas using an automatically annotated fiber clustering in major depressive disorder. Neuroimage. (2018) 181:16–29. doi: 10.1016/j.neuroimage.2018.06.019
62. Zhang F, Wu Y, Norton I, Rathi Y, Golby AJ, O'Donnell LJ. Test-retest reproducibility of white matter parcellation using diffusion MRI tractography fiber clustering. Hum Brain Mapp. (2019) 40:3041–57. doi: 10.1002/hbm.24579
63. O'Donnell LJ, Suter Y, Rigolo L, Kahali P, Zhang F, Norton I, et al. Automated white matter fiber tract identification in patients with brain tumors. Neuroimage Clin. (2017) 13:138–53. doi: 10.1016/j.nicl.2016.11.023
64. Norton I, Essayed W, Zhang F, Pujol S, Yarmarkovich A, Golby AJ, et al. SlicerDMRI: open source diffusion MRI software for brain cancer research. Cancer Res. (2017) 77:e101–3. doi: 10.1158/0008-5472.CAN-17-0332
65. Zhang F, Noh T, Juvekar P, Frisken SF, Rigolo L, Norton I, et al. SlicerDMRI: diffusion MRI and tractography research software for brain cancer surgery planning and visualization. JCO Clin Cancer Inform. (2020) 4:299–309. doi: 10.1200/CCI.19.00141
67. Bernal-Rusiel JL, Greve DN, Reuter M, Fischl B, Sabuncu MR, Alzheimer's Disease Neuroimaging I. Statistical analysis of longitudinal neuroimage data with linear mixed effects models. Neuroimage. (2013) 66:249–60. doi: 10.1016/j.neuroimage.2012.10.065
68. Mark W, Toulopoulou T. Cognitive intermediate phenotype and genetic risk for psychosis. Curr Opin Neurobiol. (2016) 36:23–30. doi: 10.1016/j.conb.2015.08.008
69. Park S, Gooding DC. Working memory impairment as an endophenotypic marker of a schizophrenia diathesis. Schizophr Res Cogn. (2014) 1:127–36. doi: 10.1016/j.scog.2014.09.005
70. Bloemen OJ, de Koning MB, Schmitz N, Nieman DH, Becker HE, de Haan L, et al. White-matter markers for psychosis in a prospective ultra-high-risk cohort. Psychol Med. (2010) 40:1297–304. doi: 10.1017/S0033291709991711
71. Carletti F, Woolley JB, Bhattacharyya S, Perez-Iglesias R, Fusar Poli P, Valmaggia L, et al. Alterations in white matter evident before the onset of psychosis. Schizophr Bull. (2012) 38:1170–9. doi: 10.1093/schbul/sbs053
72. Peters BD, Szeszko PR, Radua J, Ikuta T, Gruner P, DeRosse P, et al. White matter development in adolescence: diffusion tensor imaging and meta-analytic results. Schizophr Bull. (2012) 38:1308–17. doi: 10.1093/schbul/sbs054
73. Lebel C, Beaulieu C. Longitudinal development of human brain wiring continues from childhood into adulthood. J Neurosci. (2011) 31:10937–47. doi: 10.1523/JNEUROSCI.5302-10.2011
74. Lebel C, Walker L, Leemans A, Phillips L, Beaulieu C. Microstructural maturation of the human brain from childhood to adulthood. Neuroimage. (2008) 40:1044–55. doi: 10.1016/j.neuroimage.2007.12.053
75. Peper JS, Pol HEH, Crone EA, van Honk J. Sex steroids and brain structure in pubertal boys and girls: a mini-review of neuroimaging studies. Neuroscience. (2011) 191:28–37. doi: 10.1016/j.neuroscience.2011.02.014
76. De Bellis MD, Keshavan MS, Beers SR, Hall J, Frustaci K, Masalehdan A, et al. Sex differences in brain maturation during childhood and adolescence. Cerebral Cortex. (2001) 11:552–7. doi: 10.1093/cercor/11.6.552
77. Lenroot RK, Gogtay N, Greenstein DK, Wells EM, Wallace GL, Clasen LS, et al. Sexual dimorphism of brain developmental trajectories during childhood and adolescence. Neuroimage. (2007) 36:1065–73. doi: 10.1016/j.neuroimage.2007.03.053
79. Conklin HM, Curtis CE, Katsanis J, Iacono WG. Verbal working memory impairment in schizophrenia patients and their first-degree relatives: evidence from the digit span task. Am J Psychiatry. (2000) 157:275–7. doi: 10.1176/appi.ajp.157.2.275
80. Stefansson SB, Jonsdottir TJ. Auditory event-related potentials, auditory digit span, and clinical symptoms in chronic schizophrenic men on neuroleptic medication. Biol Psychiatry. (1996) 40:19–27. doi: 10.1016/0006-3223(95)00351-7
81. Bilder RM, Lipschutz-Broch L, Reiter G, Geisler SH, Mayerhoff DI, Lieberman JA. Intellectual deficits in first-episode schizophrenia: evidence for progressive deterioration. Schizophr Bull. (1992) 18:437–48. doi: 10.1093/schbul/18.3.437
82. Di Biase M, Cropley V, Cocchi L, Fornito A, Calamante F, Ganella E, et al. Linking cortical and connectional pathology in schizophrenia. Schizophr Bull. (2018) 44:S91. doi: 10.1093/schbul/sby015.226
83. Cabeza R, Anderson ND, Locantore JK, McIntosh AR. Aging gracefully: compensatory brain activity in high-performing older adults. Neuroimage. (2002) 17:1394–402. doi: 10.1006/nimg.2002.1280
84. Reuter-Lorenz PA, Jonides J, Smith EE, Hartley A, Miller A, Marshuetz C, et al. Age differences in the frontal lateralization of verbal and spatial working memory revealed by PET. J Cogn Neurosci. (2000) 12:174–87. doi: 10.1162/089892900561814
85. Peters BD, Dingemans PM, Dekker N, Blaas J, Akkerman E, van Amelsvoort TA, et al. White matter connectivity and psychosis in ultra-high-risk subjects: a diffusion tensor fiber tracking study. Psychiatry Res Neuroimaging. (2010) 181:44–50. doi: 10.1016/j.pscychresns.2009.10.008
86. Pasternak O, Shenton ME, Westin CF. Estimation of extracellular volume from regularized multi-shell diffusion MRI. Med Image Comput Comput Assist Interv. (2012) 15:305–12. doi: 10.1007/978-3-642-33418-4_38
87. Somers M, Sommer IE, Boks MP, Kahn RS. Hand-preference and population schizotypy: a meta-analysis. Schizophr Res. (2009) 108:25–32. doi: 10.1016/j.schres.2008.11.010
Keywords: sex differences, white matter asymmetry, high risk of psychosis, working memory, diffusion-tensor-imaging
Citation: Steinmann S, Lyall AE, Langhein M, Nägele FL, Rauh J, Cetin-Karayumak S, Zhang F, Mussmann M, Billah T, Makris N, Pasternak O, O'Donnell LJ, Rathi Y, Kubicki M, Leicht G, Shenton ME and Mulert C (2021) Sex-Related Differences in White Matter Asymmetry and Its Implications for Verbal Working Memory in Psychosis High-Risk State. Front. Psychiatry 12:686967. doi: 10.3389/fpsyt.2021.686967
Received: 28 March 2021; Accepted: 17 May 2021;
Published: 14 June 2021.
Edited by:
Neeltje E. M. Van Haren, Sophia Children's Hospital, NetherlandsReviewed by:
Sebastian Ocklenburg, Ruhr University Bochum, GermanyUner Tan, Çukurova University, Turkey
Copyright © 2021 Steinmann, Lyall, Langhein, Nägele, Rauh, Cetin-Karayumak, Zhang, Mussmann, Billah, Makris, Pasternak, O'Donnell, Rathi, Kubicki, Leicht, Shenton and Mulert. This is an open-access article distributed under the terms of the Creative Commons Attribution License (CC BY). The use, distribution or reproduction in other forums is permitted, provided the original author(s) and the copyright owner(s) are credited and that the original publication in this journal is cited, in accordance with accepted academic practice. No use, distribution or reproduction is permitted which does not comply with these terms.
*Correspondence: Saskia Steinmann, cy5zdGVpbm1hbm5AdWtlLmRl