- 1Department of Pathophysiology and Transplantation, University of Milan, Milan, Italy
- 2Department of Neurosciences and Mental Health, Fondazione Istituto di Ricovero e Cura a Carattere Scientifico (IRCCS) Ca' Granda, Ospedale Maggiore Policlinico, Milan, Italy
- 3Neuroradiology Unit, Fondazione IRCCS Ca' Granda, Ospedale Maggiore Policlinico, Milan, Italy
- 4Department of Psychiatry and Behavioral Neurosciences, Wayne State University School of Medicine, Detroit, MI, United States
- 5Department of Medical Biotechnology and Translational Medicine, Università degli Studi di Milano, Segrate, Italy
Major Depressive Disorder (MDD) is a disabling illness affecting more than 5% of the elderly population. Higher female prevalence and sex-specific symptomatology have been observed, suggesting that biologically-determined dimensions might affect the disease onset and outcome. Rumination and executive dysfunction characterize adult-onset MDD, but sex differences in these domains and in the related brain mechanisms are still largely unexplored. The present pilot study aimed to explore any interactions between adult-onset MDD and sex on brain morphology and brain function during a Go/No-Go paradigm. We hypothesized to detect diagnosis by sex effects on brain regions involved in self-referential processes and cognitive control. Twenty-four subjects, 12 healthy (HC) (mean age 68.7 y, 7 females and 5 males) and 12 affected by adult-onset MDD (mean age 66.5 y, 5 females and 7 males), underwent clinical evaluations and a 3T magnetic resonance imaging (MRI) session. Diagnosis and diagnosis by sex effects were assessed on regional gray matter (GM) volumes and task-related functional MRI (fMRI) activations. The GM volume analyses showed diagnosis effects in left mid frontal cortex (p < 0.01), and diagnosis by sex effects in orbitofrontal, olfactory, and calcarine regions (p < 0.05). The Go/No-Go fMRI analyses showed MDD effects on fMRI activations in left precuneus and right lingual gyrus, and diagnosis by sex effects on fMRI activations in right parahippocampal gyrus and right calcarine cortex (p < 0.001, ≥ 40 voxels). Our exploratory results suggest the presence of sex-specific brain correlates of adult-onset MDD–especially in regions involved in attention processing and in the brain default mode–potentially supporting cognitive and symptom differences between sexes.
Introduction
Major depressive disorder (MDD) is a disabling psychiatric illness affecting an increasing proportion of the global population, reaching a peak of 5.74% in late adulthood, higher among females (6.75%) compared to males (4.60%) (1, 2).
The growing burden of disease in the elderly population is partly attributable to social risk factors, including stressful life events, which have been shown to facilitate MDD onset during adulthood (3–7). Of note, adult-onset MDD seems to have worse prognosis than MDD occurring at younger ages, as findings suggest that the course of illness is more severe, the recurrence rate is higher as well as is the suicide rate, which appears to be the 13th cause of death in this clinical group (3, 8–11). Executive dysfunction–including aberrant interference resolution and inhibitory control–is another core trait of late life depression, suggesting a cumulative burden of disease (12).
The higher prevalence of adult-onset MDD in females relative to males is in line with the documented sex gap in lifetime MDD rates (4, 13) and supports sex-specific models of MDD vulnerability, neurobiology, and psychopathology (14).
Notably, in MDD in general, sex differences are not limited to the rates of disease, but also involve its clinical presentation, with females developing more somatic symptoms, comorbid anxiety disorders and atypical depression, and males having higher mortality rates, both from suicide and from somatic comorbidity or substance abuse (4, 5, 15). Differences in the cognitive correlates of MDD between females and males have also been observed, even if the results appear fragmented (16, 17).
Up to now, fewer studies have attempted to disambiguate the role of sex in adult-onset MDD (18–20), leaving a number of questions either undiscussed or unresolved. Of note, sex differences in disease susceptibility do not appear to be merely due to sex-specific responsivity to stressors (7).
Overall, a more complex interplay among genetic loading, stress susceptibility, and life events might contribute to sex-specific risk factors for adult-onset MDD, which in turn might result in neurobiological and clinical features that are at least partly different between sexes.
Nevertheless, most of the available knowledge on the topic regards MDD in general. From a neurobiological perspective, like in healthy individuals who show numerous sex-related brain differences throughout the lifespan (21–25),
there is evidence of peculiar patterns of brain alterations in males and females affected by MDD (26). Sex-specific deficits in GM volume have been observed in regions within the frontal and temporal cortices and the prefrontal-striatal circuit in MDD males, and in areas of the prefrontal-limbic circuit and the lingual gyrus in MDD females (27–30). Interaction effects of late life depression and sex on frontal regional brain volumes were suggested as well (19).
Functional Magnetic Resonance Imaging (fMRI) paradigms in MDD reported sex differences in the fMRI correlates of facial emotion processing and autobiographical memory recall (31, 32).
Despite the wide profile of cognitive dysfunctions characterizing MDD, sex differences in cognitive performances and in the underlying functional brain networks remain largely unexplored. The cognitive domain of inhibitory control is receiving special interest, since it appears to be greatly affected by the MDD pathology and to impact on the therapeutical and functional outcomes (33–37).
This domain is often assessed with the Go/No-Go paradigm, which is a well-established tool also used to measure sustained attention (38, 39). Adapted versions of the Go/No-Go task, especially those with affective valences, have been used to study brain activations in MDD patients compared to healthy controls, but evidence of sexual dimorphisms remains scarce. On this regard, Chuang et al. (40) reported group by sex effects on the fMRI response to the affective task in the supramarginal gyrus and in the posterior cingulate cortex (PCC), but not on task performances (40). Additionally, van Deurzen et al. (41) proposed that inhibitory control impairments may predict the onset of affective disorders, including depression, in adolescent females (41). Moreover, evidence from preclinical studies points at a possible sexual dimorphism in the mechanisms that underlie stress-induced alterations in structural brain plasticity and sustained attention disruption in MDD (41).
Sex dimorphisms in inhibitory control have been reported, but mostly in healthy individuals. Concerning the Go/No-Go paradigm, females showed slightly lower accuracy while processing the Go vs. No-Go contrast, and also longer processing times for the monitoring of response conflict, motor response execution, and outcome inhibition (42, 43). In addition, concerning patterns of brain activation, it has been shown that, during impulse inhibition, males tend to show more activity in the anterior cingulate cortex (ACC), while females in the middle temporal cortex (44). Conversely, sex differences in pure inhibitory control and related brain mechanisms are more poorly documented in MDD. In fact, the only evidence available indicates that deficits in this domain may predict the onset of affective disorders, including depression, in adolescent females (41). Adapted versions of the Go/No-Go task, especially those with affective valences, have been used to study the neural bases of emotion-cognitive interference in MDD. On this regard, Chuang et al. (40) reported group by sex effects on the fMRI response to the affective task in the supramarginal gyrus and in the posterior cingulate cortex (PCC), but not on task performances (40). Of note, evidence from preclinical studies points at a possible sexual dimorphism in the mechanisms that underlie stress-induced alterations in structural brain plasticity and sustained attention disruption in MDD (41).
To summarize, despite the remarkable sex differences in MDD and the increasing evidence that its clinical heterogeneity is biologically-determined, the impact of sex on MDD clinical presentation and outcome and on the underlying brain processes has been often underestimated and remains unclear (45).
In this context, very little is still known about sex-related brain mechanisms responsible for inhibitory control and sustained attention in adult-onset MDD. Investigating sexual dimorphisms in these domains may be particularly important for choosing the most appropriate treatment plan, since the functional connectivity of the cognitive control network has been demonstrated to be a good predictor of antidepressant treatment response and to be as well correlated to symptom improvement (46).
On account of these premises, the present work aimed to preliminarily explore the impact of sex and adult-onset MDD on brain morphology and function, especially in the brain regions involved in response inhibition and sustained attention. To this end, we carried out a pilot 3T structural and functional MRI study on subjects with adult-onset MDD and healthy controls and assessed the putative role of sex on brain changes in MDD, in terms of both regional GM volumes and activation patterns during a standard parametric Go/No-Go task.
Materials and Methods
Study Population
Twenty-four subjects, 12 affected by MDD and 12 healthy controls (HC), aged between 50 and 90 years were enrolled in the study. Clinical diagnoses were assessed using the Italian version of the Structured Clinical Interview for DSM-5 (SCID): only subjects with adult-onset MDD (≥ 45 years) were included in the patient group, and only subjects without psychiatric diagnoses were included in the control group. Exclusion criteria for patients were lifetime psychotic symptoms and current DSM-5 comorbid disorders. Exclusion criteria for all participants comprised lifetime alcohol or substance abuse, intellectual disability, history of head trauma with loss of consciousness, neurological or neurodegenerative illnesses.
All subjects provided a written informed consent to the study protocol, which was conducted in accordance with the Declaration of Helsinki and approved by the Ethical Committee of the Fondazione IRCCS Ca' Granda Ospedale Maggiore Policlinico, Milan, Italy.
Psychopathological and Cognitive Assessment
In MDD patients, information on age of onset and current pharmacological treatment were collected. All participants underwent a comprehensive psychopathological assessment including the Hamilton Depression Rating Scale (HDRS) (47), the Depression Anxiety and Stress Scales-21 (DASS-21) (48), and the Traumatic Experience Checklist (TEC) (49). The participants' cognitive performances were rated using the Repeatable Battery for the Assessment of Neuropsychological Status (RBANS) (50).
Multimodal Neuroimaging Acquisition
Structural and functional MRI data were acquired on a 3T Philips Achieva DStream scanner (Philips, Best, The Netherlands) equipped with a 32-channel head coil. Structural T1-weighted images were collected using a 3D turbo field echo (TFE) SENSE sequence (field of view: 250 mm (FH) × 240 mm (AP) × 180 mm (RL), voxel size: 1 mm3, echo time (TE): 4 ms, repetition time (TR): 8 ms, flip angle: 8°). Two hundred (plus two dummy) functional MRI volumes were collected during a visuomotor task (described in section the following) using a multi-transmit T2*-weighted echo planar imaging (EPI) sequence (field of view: 120 mm (FH) × 256 mm (AP) × 256 mm (RL), voxel size: 2 × 2 × 2 mm, echo time (TE): 30 ms, repetition time (TR): 2,000 ms, flip angle: 90°). Five additional (plus two dummy) fMRI scans acquired with the same parameters but opposite phase encoding direction were used to estimate and correct for susceptibility induced distortions in the fMRI volumes.
fMRI Experimental Protocol
The fMRI experiment was a visuomotor Go/No-Go task implemented using the Presentation® software (Neurobehavioral Systems, Inc., Berkeley, CA, USA). Visual stimuli were delivered in the MR scanner through the MR-compatible NordicNeuroLab VisualSystem HD (NNL, Bergen, Norway) and synchronized to the fMRI pulses using the NNL SyncBox. The participants' responses were collected using the MR-compatible NNL ResponseGrip device (composed of thumb and index finger buttons). Prior to the fMRI session, all subjects were trained to perform the task outside the MRI environment.
The Go/No-Go task combined blocks with only target stimuli (“Go” blocks) with blocks with both target and non-target stimuli (“Go/No-Go” blocks). Consecutive stimuli were delivered either at 1 s intervals (“Periodic” blocks) or at random intervals around 1 s (“Random” blocks). In the “Go” blocks, the subject was asked to push the thumb button every time a red or green flashing square appeared on the screen (excitation mode). In the “Go/No-Go” blocks, the participants were asked to push every time a green square flashed on the screen, and to not push when a red square flashed on the screen (inhibition mode). All responses were collected using the dominant thumb, which was in the right hand for all subjects. The experiment consisted of eight task blocks (two “Periodic Go”, two “Periodic Go/No-Go”, two “Random Go”, two “Random Go/No-Go”, lasting 32 s each) alternated with resting state 16 s intervals, during which the subjects had to focus on a fixation cross at the center of the screen and to not think of anything specific.
Data Processing
The processing of clinical, behavioral, and neuroimaging data was performed on Matlab R2019a Update 4 (The Mathworks, Inc.). Neuroimaging analyses were conducted using the Matlab-based Statistical Parametric Mapping (SPM) software (version 12, https://www.fil.ion.ucl.ac.uk/spm) (51), its CAT12 toolbox (http://141.35.69.218/cat/) (52), and Matlab in-house scripts. The FMRIB Software Library (FSL) was also used for fMRI pre-processing (53). Statistical comparisons were performed using SPM12 statistical tools and the Statistics and Machine Learning Toolbox™.
Extraction of Brain Morphological Features
Pre-processing
The participants' T1-weighted images were visually inspected and manually reoriented as the SPM tissue probability maps (TPMs). Brain tissue segmentation was performed using the CAT12 segmentation tool. The pipeline included the SPM bias inhomogeneity correction, initial affine registration to SPM12 TPMs, SPM unified segmentation, skull stripping, local adaptive segmentation, adaptive maximum a posterior (AMAP) segmentation, and optimized shooting registration to the CAT12 default template in the Montreal Neurological Institute (MNI) space. Spatially normalized and modulated GM images were resampled (voxel size: 1.5 × 1.5 × 1.5 mm) and smoothed with a 3D 6 mm Full Width Half Maximum (FWHM) Gaussian kernel. Total intracranial volumes (ITV) were extracted from the subjects' CAT12 report files. The processing quality was checked by displaying the normalized and modulated GM images (one slice per subject) and by checking their correlation among subjects to identify outliers. The quality of the pre-processed GM maps was reasonable for all subjects; therefore no subjects were excluded.
Region-Based Volume Estimation
Region of interest (ROI) analyses were performed using in-house Matlab scripts. The normalized, modulated, and smoothed GM images were used to extract region of interest (ROI) GM volumes in the 116 Automated Anatomical Labeling (AAL) atlas regions (54).
Extraction of Brain Functional Features
Pre-processing
For each subject, the pre-processing of the fMRI volumes recorded during the Go/No-Go task was performed using SPM12 and FSL tools. In SPM12, head motion artifacts were estimated and used to realign all fMRI volumes to the first reference fMRI volume. For all but one subjects, the movement parameters felt within the pre-specified limits (translation < 2 mm, rotation < 3°). The realigned fMRI volumes (and the additional fMRI data with inverse phase-encoding polarity) were imported in FSL, in which the TOPUP tool was used to estimate and correct for susceptibility-induced distortions. This correction step could not be applied to the fMRI volumes from two subjects due to the absence of the inverse polarity data. In SPM12, the subject's structural image was co-registered to the mean fMRI image and used to estimate the deformation fields for spatial normalization. The fMRI volumes were then normalized to the MNI space and spatially smoothed using a 3D 4 mm FWHM Gaussian kernel. The post-processing fMRI data quality in the subject with 3.7 mm translation was carefully verified and considered sufficient for inclusion in the analyses.
Subject-Level fMRI Statistics
The pre-processed fMRI volumes from each subject were entered in a voxel-based block-related GLM activation analysis. The BOLD signal from each voxel was modeled as a function of both task-related and confounding factors (regressors). The GLM design matrix included one regressor for each experimental condition (1. “Periodic Go”, 2. “Periodic Go/No-Go”, 3. “Random Go”, 4. “Random Go/No-Go”) obtained by the block convolution with the canonical hemodynamic response function (HRF), and head translation (n=3) and rotation (n=3) parameters as confounding regressors.
After estimation of the GLM coefficients, inference on the experimental effects was performed with appropriate t-contrasts (t-tests on linear combinations of the coefficients). The following subject-level t-contrasts were considered for the group-level statistics: 1. task vs. rest, 2. “Go” vs. rest, 3. “Go/No-Go” vs. rest, 4. “Go” vs. “Go/No-Go”.
Statistical Analyses
The statistical comparisons of demographic, clinical, cognitive, and brain morphological data were performed using in-house Matlab scripts based on the Statistics and Machine Learning Toolbox™ functions. The effects of diagnosis, sex, and their interaction on clinical (HDRS, DASS-21, TEC) and cognitive (RBANS, fMRI performance) measures were assessed using linear regression models where the selected variable was explained in terms of diagnosis and sex, in interaction, and age. Inference on the GLM coefficients was performed using two-sided t-tests, with significance threshold placed at p = 0.05.
In the brain morphological analyses, the AAL ROI volumes were used as dependent variables in univariate General Linear Model (GLM) designs, in which they were modeled as a function of diagnosis, sex, age, and ITV, with diagnosis and sex interacting. After GLM least-squares fitting estimation, diagnosis, sex, and diagnosis by sex effects were assessed via two-sided t-tests on the GLM coefficients. Significance threshold was set to p = 0.05 after multiple comparison correction using Bonferroni's method (n=116). Due to the conservativeness of such correction, tendencies are also reported (p < 0.01 for main factor effects, p < 0.05 for interaction effects).
Group-level statistics on the fMRI activations were extracted using SPM12 functions. The subject-level fMRI contrast maps were entered in second-level random-effects GLM analyses. For each contrast, we employed a full-factorial GLM design with diagnosis and sex factors in interaction, and age and “Go/No-Go” task performances (1. Percent of responses to target stimuli, 2. Time of response to target stimuli, 3. Percent of responses to non-target stimuli) as covariates of no interest. After GLM estimation, we assessed diagnosis, sex, and diagnosis by sex effects on the subject-level fMRI activations using positive and negative one-sided t-tests enabled by SPM12 (p < 0.001, > 40 voxels). The significant clusters were localized based on the AAL atlas and the subjects' peak contrast values were illustrated as a function of diagnosis, sex, or their combination, as appropriate.
Results
Demographic, Clinical, and Cognitive Information
The sample characteristics are summarized in Table 1. Further details on the patients' therapies and dosages and disease characteristics can be found in Supplementary Tables S2, S3. Sex distribution was comparable between MDD and HC groups. Age was comparable between MDD subjects and HC, females and males, and diagnosis by sex subsets. Total HDRS scores were higher in MDD subjects compared to HC and in females compared to males, but no diagnosis by sex effects emerged. Females also showed higher DASS-21 stress and depression scores than males, regardless of the diagnostic group. No effects of diagnosis, sex nor their interaction emerged on DASS-21 anxiety and TEC scores.
Regarding cognitive performances, total RBANS scores were comparable across diagnosis and sex groups, whereas the fMRI task performances were more influenced by these factors. In the “Go/No-Go” blocks (inhibition mode), the percentage of correct responses to target stimuli (hits) was slightly higher in HC compared to MDD and in females compared to males, with stronger diagnosis by sex effects. Specifically, hit rates were higher in healthy females than in healthy males, lower in MDD females than in MDD males. The times of response to target stimuli were higher in MDD patients than in HC, but no sex or group by sex effects emerged. No influences of diagnosis and sex emerged on the percentage of commission errors (i.e., responses to non-target stimuli) and on the delay of such incorrect responses. Similarly, the performance rates in the “Go” blocks (excitation mode) were comparable across diagnoses and sexes.
Diagnosis and Sex Effects on Brain ROI Morphology
Regional GM volumes in the AAL ROIs were comparable between MDD and HC groups, females and males, and diagnosis by sex subsets (p < 0.05, Bonferroni corrected), but some tendencies emerged. Compared to HC, MDD subjects showed lower GM volume in the left mid frontal cortex (T = 3.19, p = 0.005). Diagnosis by sex effects emerged also in the orbitofrontal gyrus [left middle (T = 2.18, p = 0.043) and superior (T = 2.79, p = 0.012) portions, right superior portion (T = 2.25, p = 0.037)], in the left olfactory cortex (T = 2.56, p = 0.019), and in the bilateral calcarine cortex (left: T = 2.30, p = 0.033; right: T = 2.21, p = 0.040). As shown in Figure 1, the normalized GM volume in all these ROIs was higher in healthy females than in healthy males, but lower in MDD females compared to MDD males. The pairwise differences between group by sex subsets are marked in the boxplots (p < 0.05).
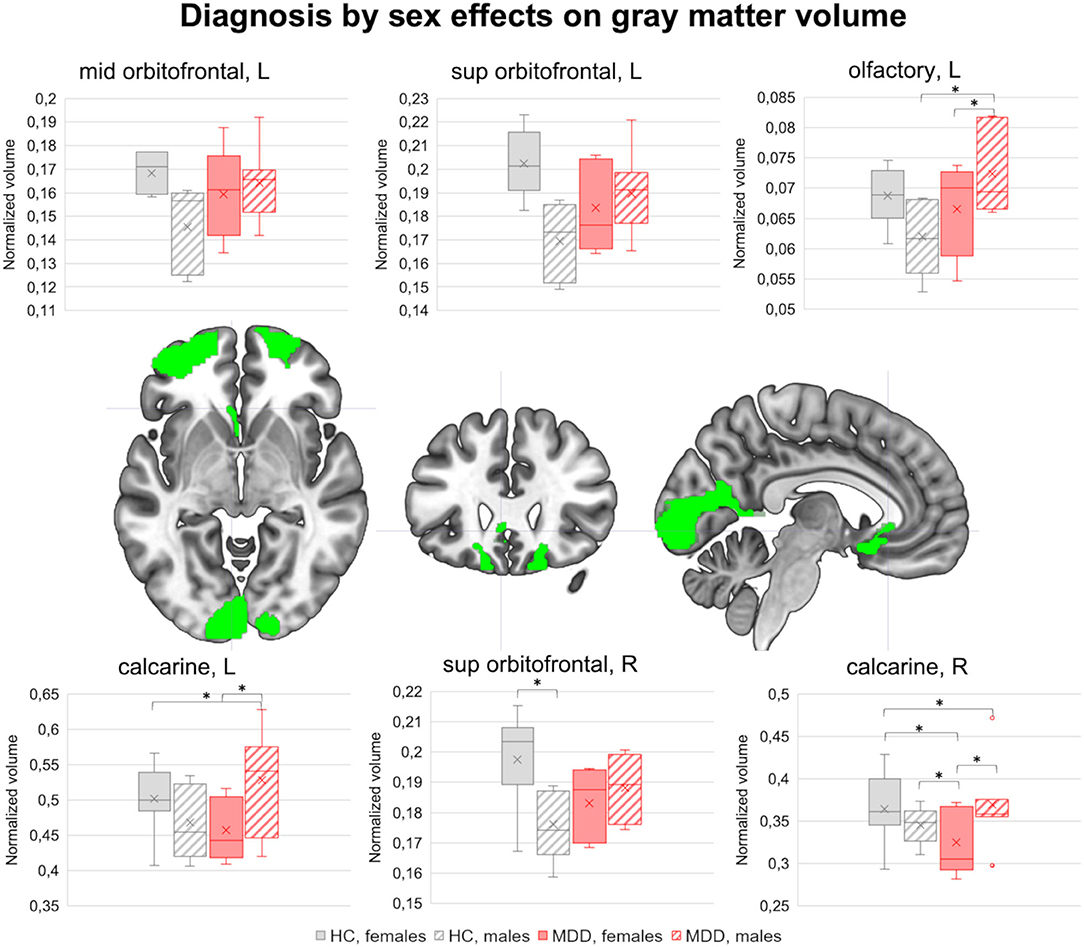
Figure 1. Diagnosis by sex effects on brain morphology. The brain clusters showing sexually dimorphic GM volume differences between MDD and HC groups are highlighted in green (p < 0.05). In the top and bottom rows, the boxplots represent the normalized GM volume distribution in HC females, HC males, MDD females, and MDD males. Pairwise differences between diagnosis by sex subsets (net of age and ITV) are highlighted (p < 0.05). GM, gray matter; MDD, major depressive disorder; HC, healthy controls; L, left; R, right. *significant pairwise differences (p < 0.05).
Diagnosis and Sex Effects on Task-Related fMRI Activations
This section is dedicated to the effects of diagnosis and sex on the fMRI activations induced by the Go/No-Go task, which are detailed in Table 2 and illustrated in Figure 2. The fMRI activations emerged in the entire group, described in the Supplementary Material, are used as reference to interpret the influences of diagnosis and sex.
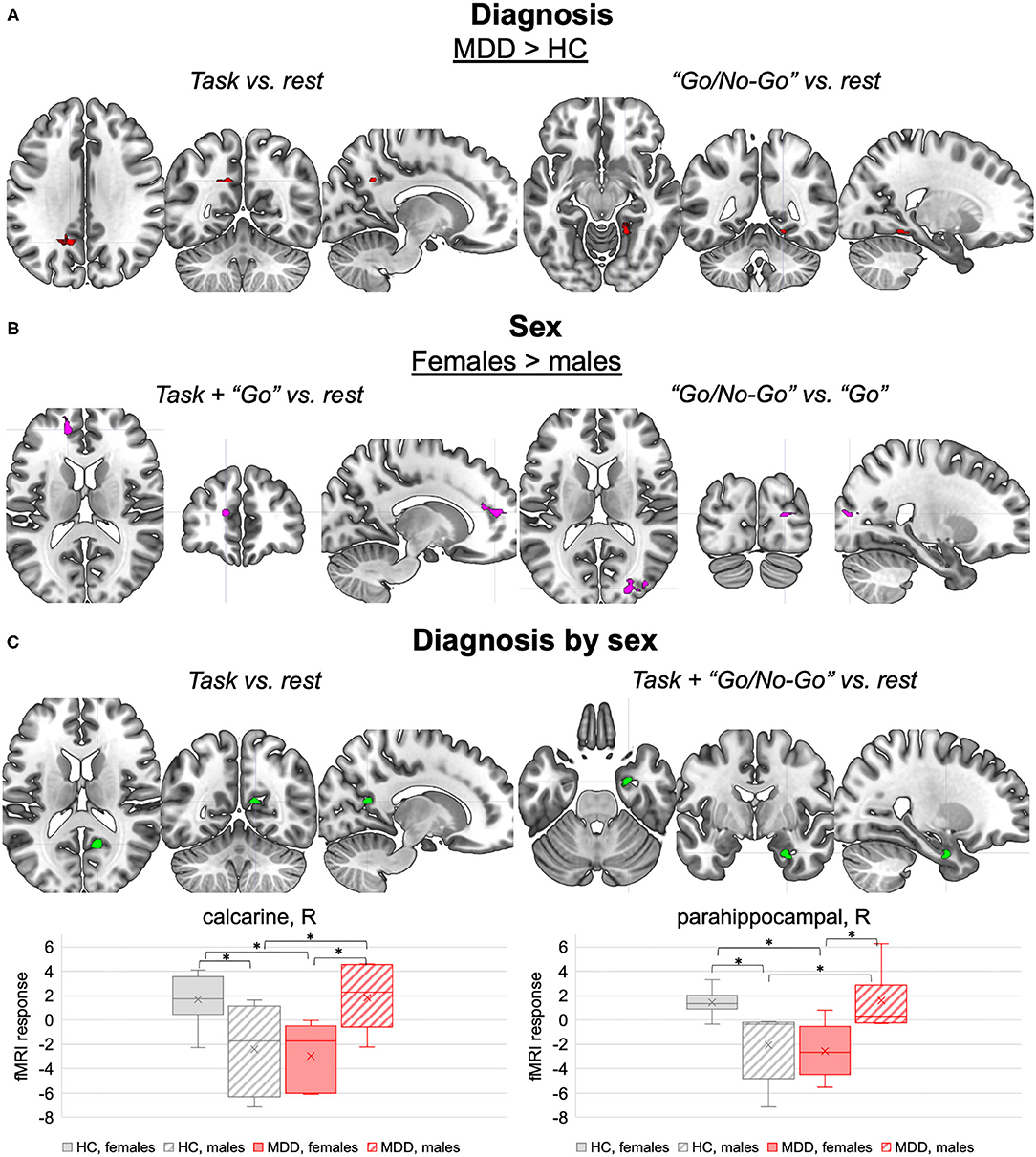
Figure 2. Diagnosis, sex, and interaction effects on task-related fMRI activations. (A) Diagnosis effects. Brain clusters with different fMRI responses to the task (left panel) and to the “Go/No-Go” inhibitory blocks (right panel) in MDD compared to HC (p < 0.001, ≥ 40 voxels). (B) Sex effects. Brain clusters with different fMRI responses to the task and “Go” excitatory blocks (left panel), and the “Go/No-Go” inhibitory blocks vs. “Go” excitatory blocks (right panel) in females compared to males (p < 0.001, ≥ 40 voxels). (C) Diagnosis by sex effects. Brain clusters with interaction effects on fMRI responses to the task (left panel) and the “Go/No-Go” inhibitory blocks (right panel) (p < 0.001, ≥ 40 voxels). The boxplots in the lower panel represent the corresponding normalized peak fMRI response distributions in HC females, HC males, MDD females, and MDD males. Pairwise differences in the peak fMRI response between diagnosis by sex subsets (net of age) are highlighted (p < 0.05). MDD, major depressive disorder; HC, healthy controls; L, left; R, right. *significant pairwise differences (p < 0.05).
Diagnosis Modulation on fMRI Activations
Significant effects of MDD diagnosis were observed on the fMRI response to the task in general and to the “Go/No-Go” condition (p < 0.001, ≥ 40 voxels) (Table 2, section “Main effect of diagnosis”, Figure 2A). Indeed, when compared to HC, MDD subjects showed higher fMRI response to the task (vs. rest) in a portion of the left precuneus, and higher fMRI response to the “Go/No-Go” condition (vs. rest) in a portion of the right lingual gyrus. Both clusters were proximal to DMN nodes with negative BOLD response to the task, suggesting that MDD subjects might fail to deactivate these regions. No differences between MDD and HC groups were observed in the fMRI response to the “Go” vs. rest and the “Go/No-Go” vs. “Go” conditions.
Sex Modulation on fMRI Activations
Females and males were characterized by different fMRI responses to the task in general and to both “Go” and “Go/No-Go” conditions (p < 0.001, ≥ 40 voxels) (Table 2, section “Main effect of sex”, Figure 2B). When compared to males, females showed enhanced fMRI activation during the task, especially in the “Go” condition, in a cluster in the left hemisphere at the interface between anterior cingulate cortex and superior medial frontal cortex. Females also showed higher fMRI response than males to “Go” vs. “Go/No-Go” conditions in a cluster in the right middle and superior occipital cortex. Conversely, the fMRI activations induced by the “Go/No-Go” condition were not influenced by sex.
Diagnosis by Sex Modulation on fMRI Activations
Interaction effects were observed in the fMRI responses to the task in general and to its “Go/No-Go” condition (p < 0.001, ≥ 40 voxels) (Table 2, section “Diagnosis by sex effects”, Figure 2C). The fMRI response to the task and to the “Go/No-Go” condition in a portion of the right parahippocampal gyrus showed diagnosis by sex effects. As illustrated in Figure 2C, such response was more negative in MDD females compared to MDD males, whereas an opposite tendency emerged in the HC group. Similar interaction effects were observed in the fMRI response to the task in a cluster in the right calcarine cortex, which was significantly deactivated by the task at the group level (see Supplementary Material). The fMRI activations concerning the “Go” vs. rest and “Go” vs. “Go/No-Go” conditions did not exhibit interaction effects.
Discussion
The focus of this study was to explore brain morphology and functional activations during a Go/No-Go task in a pilot sample of adult-onset MDD patients and HC, while paying attention to possible differences between females and males. To our knowledge, this is the first attempt to assess sex differences in the brain morphological and cognitive-related functional features of adult-onset MDD. Despite being preliminary, the obtained results suggest reduced GM volume in adult-onset MDD compared to HC in the left mid frontal cortex, coupled with diagnosis by sex effects on GM volumes within the orbitofrontal gyrus, the left olfactory cortex, and the bilateral calcarine cortex. In the same line, the fMRI analyses showed interaction effects on the Go/No-Go activation patterns in the right parahippocampal gyrus and in the right calcarine cortex, as well as higher fMRI responses in the left precuneus and in the right lingual gyrus in adult-onset MDD compared to HC.
This pilot evidence, which needs to be reproduced on larger samples, confirms the importance of considering sex as a relevant neurobiological factor in studies on MDD, including adult-onset disease, paving the way for future patient-centered investigations. A better knowledge of sexual dimorphism in adult-onset MDD could be particularly helpful in predicting the disease vulnerability over lifetime and in understanding the differential sex response to the available antidepressant treatments (55), allowing the identification of new targets for personalized interventions.
Diagnosis by Sex Effects on Brain Morphology
Overall, the majority of the areas emerged from the structural MRI comparison between adult-onset MDD and HC are involved in different aspects of emotional processing and regulation and included the left middle frontal cortex, the orbitofrontal cortex, and the left olfactory cortex. Additionally, selective deficits in the calcarine cortex, a region involved in diverse cognitive processes, were also observed.
Diagnosis Modulation on ROI Morphology
The structural MRI comparison showed that adult-onset MDD patients, regardless of sex, had lower GM volumes in the left mid frontal cortex, an area that in the healthy brain is known to have a key role in several cognitive (56–59) and emotional processes (60, 61). Notably, our result is in line with evidence from previous studies on MDD patients (30, 62, 63).
Moreover, in depression, reduced GM volumes (63) in this area have been associated with impaired attention, psychomotor retardation and cognitive impairments as well as with pathological negative affectivity in response to negative emotional stimuli (60, 61, 64). Therefore, our results together with previous evidence support the key role of this region in MDD and further highlight the putative role of this structure in the development of clinical and cognitive manifestations of MDD that involve both males and females.
Diagnosis by Sex Modulation on ROI Morphology
A diagnosis by sex interaction effect was observed in three different areas, including the orbitofrontal cortex, the olfactory cortex, and the calcarine cortex, where healthy females showed higher GM volumes compared to healthy males, whereas adult-onset MDD patients reported an opposite pattern.
With regards to the orbitofrontal cortex, this brain region is involved in the behavioral modulation through the integration of sensory and visceral motor information, and has a key role in emotional processing (65). Although some studies reported the presence of structural differences between healthy males and females in this area (24, 25, 66, 67), the results are not univocal, possibly due to the different study characteristics. In contrast, the GM reduction in the orbitofrontal cortex in MDD was already observed by our and other research groups (68–72). Some studies also reported a correlation between deficits in this area and illness severity (72–74). Therefore, the increased GM deficits in the orbitofrontal cortex observed in adult-onset MDD females may explain the higher rates of internalizing problems and rumination often observed in the female sex, which could also be explained by the presence of different illness-related genes that in turn contribute to the different symptomatology observed in males and females with MDD (14, 75–77).
The same pattern of diagnosis by sex effects was observed in the olfactory cortex. Besides its key role in olfaction, this structure was found to be involved in emotional regulation (78). The presence of physiological sexual dimorphism on the area is not surprising, since literature evidence reports sex-related differences in olfactory ability and related pathways (79, 80), although there is no specific evidence regarding the olfactory cortex itself (79, 81).
With regards to the opposite sexually dimorphic pattern observed in adult-onset MDD, there is evidence supporting the fact that in depression the hedonic features of olfaction are impaired together with the sensitivity and identification of odors (82), which are also correlated to the severity of disease and emotional dysregulation (83, 84), ultimately suggesting a role of this structure in the pathogenesis (83, 84). Therefore, based on this evidence, lower GM volumes observed in the olfactory cortex in female adult-onset MDD patients are possibly correlated to worse affectivity and emotional dysregulation compared to male MDD patients.
Finally, healthy females showed higher GM volumes than healthy males in the calcarine cortex, a key region involved in processing visual information (85). Several studies reported the presence of different visuospatial information processing in the two sexes and of sex-related structural differences, although not consistently (86, 87). Notably, our results are in line with previous evidence that found higher GM volumes in females (88, 89), ultimately supporting the hypothesis that this structure can be considered a possible neuroanatomical classifier of biological sex. Conversely, sex-related differences in this area have not been previously observed in MDD. However, some evidence reported GM volume reduction and connectivity alterations in this area in MDD (90, 91), which were correlated to the presence of negative cognitive models (92, 93) and impairments in other cognitive domains, such as attention and working memory (94–96). Therefore, the reduced GM volumes observed in females with adult-onset MDD compared to their male counterpart may determine higher impairments in those cognitive domains, as preliminarily observed in our sample in relation to the fMRI task performances (hit rates). However, further studies are needed to corroborate this finding.
Sex by Diagnosis Effects on Task-Related fMRI Activations
Diagnosis Modulation of fMRI Activations
Our results showed significant effects of adult-onset MDD diagnosis on fMRI response to the task in general and to the inhibitory condition, with adult-onset MDD patients showing reduced deactivation in the left precuneus and in the right lingual gyrus compared to HC.
Specifically, the precuneus is well known to be a functional core of the DMN (97, 98), which is a large-scale brain network encompassing different brain areas, creating a system for self-related cognitive activity (99). In healthy individuals, this network is normally active during internally oriented processes, and deactivates during external attention-driven cognitive tasks (94, 99, 100). In MDD, the DMN is known to be dysregulated and to display a decrease in task-related deactivation compared to HC that might contribute to the reduced response to external stimuli characteristic of MDD (74, 101–103). This characteristic might have been enhanced by the Go/No-Go task, which requires a shift of the subject's attention from internally-oriented thinking to external cues.
Our evidence of higher precuneus activity in adult-onset MDD patients compared to HC is concordant with the symptomatology of emotional and attentional dysregulation and with previous fMRI findings (104–106). Moreover, the abnormalities found in this area are also postulated to underline other specific characteristics of MDD, such as the negative representation of the self (107, 108) and rumination (109, 110). Thus, our results support the hypothesis that the DMN represents a putative biomarker of depression also in the adult-onset population, which can explain depressive symptoms like the ones that rely on self-referential processes.
Our sample of adult-onset MDD patients also showed higher fMRI responses to the “Go/No-Go” condition (vs. rest) in the right lingual gyrus. This area is known to regulate visual processing and is in close relation to the primary visual cortex (calcarine fissure) (111). In MDD, activity and connectivity alterations in this structure (108, 112) have been observed. Overall, these results support the involvement of this area in working memory and response inhibition, two key cognitive domains known to be impaired in depression and that are mostly tested during the “Go/No-Go” condition (113–116).
Diagnosis by Sex Modulation of fMRI Activations
An interaction between diagnosis and sex was observed in the fMRI activation patterns associated with the task and especially the inhibitory “Go/No-Go” condition. Indeed, opposite differences between sexes and groups were observed in the right calcarine cortex and the right parahippocampal gyrus. The fMRI response in these regions was more negative, reflecting a higher deactivation, in healthy males vs. healthy females and in adult-onset MDD females vs. adult-onset MDD males.
Notably, regarding the right calcarine cortex, a similar pattern of diagnosis by sex effects was observed on its regional GM volume, providing a neuroanatomical basis to the fMRI results. This region is housing the primary visual cortex (85) and has an important role in targeting attention (117). As already mentioned, sexual dimorphism in this area has been previously documented in healthy brains, supporting the existence of different visual processes in the two sexes depending on the task. The interactions with diagnosis may further suggest sex-specific attentional processes elicited by the Go/No-Go paradigm in MDD individuals, but this result needs to be confirmed on larger samples.
The opposite sexually dimorphic pattern observed in adult-onset MDD patients, with females showing a more negative response than males, might reflect the role of calcarine gyrus in MDD symptomatology. In literature a plethora of alterations have been documented (92, 93), and have been associated both to resistance to treatment (118) and pathological cognitive processes, such as self-isolation, social avoidance, and impaired attentional performances (119).
In light of this evidence, the more negative fMRI response that we observed in adult-onset MDD females can explain the presence of a more severe symptomatology, documented by higher HDRS scores, and to the worse performance in the Go/No-Go task, maybe due to attentional impairments, observed in this subgroup of participants compared to the male counterpart. This evidence, if confirmed on larger samples, lets us hypothesize a role of the calcarine cortex in the pathological cognitive models documented to be more frequent in females than in males also in adult-onset MDD (4, 13, 120).
With regards to the right parahippocampal gyrus, this region is part of the limbic system and is primarily involved in spatial memory and retrieval of associative contextual memory (121, 122). Interestingly, sexual dimorphism in this area in healthy brains, from both structural and functional perspectives, has been documented (25, 123). From the functional perspective, healthy males have been characterized by lower functional connectivity and higher efficiency than healthy females, possibly associated with higher flexibility and better adaptation to stress (124), but differences in the mechanisms of autobiographical memory retrieval have been proposed as well (125). In contrast, meta-analytic evidence supports an increased right-sided activation of this region during working memory tasks in females than in males (126).
Notably, an opposite sexually dimorphic pattern of parahippocampal activation was observed in adult-onset MDD patients, with females having a more negative response compared to males. Several strands of evidence reported the key role of the parahippocampal gyrus in the pathophysiology of depression. Indeed, the region is considered to be a component of the DMN (96, 127, 128) and deficits in this region have been associated with clinical symptoms often observed in MDD, including rumination, abnormal retrieval of autobiographical memory (109), and generation of contextual associates (129). In addition, there is evidence of its role in emotion-mediated memory formation (96, 128) and emotional regulation (128, 130, 131), possibly contributing to impairments in reward and in the development of happy feelings (108). Nevertheless, our preliminary evidence is new in the literature. If reproduced on larger samples, the parahippocampal deactivation that we observed in MDD females during response inhibition might either contribute to and/or result from the female-specific symptomatology (4, 13, 120) characterized by detrimentally high stress levels (4, 13, 75, 132).
Limitations
The main limitation of our study resides in the small number of participants, which significantly limited the statistical power of the results, enabling the extraction of MDD and sex effects at a trend level. Despite our focus on middle and late adulthood, the large age range of the participants might have introduced aging-related confounding effects. Moreover, we did not perform diagnosis or sex by age analyses as well as connectivity analyses, which should be implemented in future replications. The reliability and specificity of our results might have also been affected by the patients' pharmacological therapy, which was characterized by diverse substances and dosages among subjects, and by the females' fertility phase and menstrual cycle, whose status was not assessed during the clinical evaluation. Finally, it should be noticed that our pilot evidence was obtained on a sample of patients with MDD onset during adulthood. While this choice represents the main asset of our study, future replications on larger independent samples and on subjects with earlier illness onset are highly encouraged to confirm and better characterize our results.
Conclusions
Our pilot investigation of sex differences in brain morphology and in the neural bases of inhibitory control in adult-onset MDD showed promising results that, if confirmed, might underly the different disease presentations that have been extensively documented in the two sexes. Our findings strengthen the idea that sex is a relevant factor shaping the neurobiological correlates of depression, and can serve as an incentive to future investigations.
Data Availability Statement
The raw data supporting the conclusions of this article will be made available by the authors, without undue reservation.
Ethics Statement
The studies involving human participants were reviewed and approved by Comitato Etico Milano Area 2, Fondazione IRCCS Ca' Granda Ospedale Maggiore Policlinico, Milan. The patients/participants provided their written informed consent to participate in this study.
Author Contributions
MP: formal analysis and writing–original draft. EM: conceptualization, data curation, methodology, software, formal analysis, and writing–original draft. GD: data curation and writing–original draft. AF and SP: data curation, supervision, and writing–review and editing. DG, EF, PE, CC, and FT: data curation and writing–review and editing. JS: methodology, software, and writing–review and editing. EB: conceptualization, supervision, and writing–review and editing. PB: conceptualization, project administration, supervision, and writing–review and editing. All authors contributed to the article and approved the submitted version.
Funding
The experimental research work was partially supported by the Fondazione Cariplo (Grant no. 2016-0908). Grant support to the authors was also received by the Italian Ministry of Health (Grant no. 2018-12367789 to EM).
Conflict of Interest
The authors declare that the research was conducted in the absence of any commercial or financial relationships that could be construed as a potential conflict of interest.
Publisher's Note
All claims expressed in this article are solely those of the authors and do not necessarily represent those of their affiliated organizations, or those of the publisher, the editors and the reviewers. Any product that may be evaluated in this article, or claim that may be made by its manufacturer, is not guaranteed or endorsed by the publisher.
Acknowledgments
We wish to acknowledge Francesca Bottinelli and Ilenia Pasini for their help in the initial screening of participants.
Supplementary Material
The Supplementary Material for this article can be found online at: https://www.frontiersin.org/articles/10.3389/fpsyt.2021.683912/full#supplementary-material
References
1. WHO. (2021). Depression [WWW Document]. Available online at: https://www.who.int/news-room/fact-sheets/detail/depression (accessed 20, Oct 2021).
2. Institute Institute of Health Metrics Evaluation n. d. (n.d.). Global Health Data Exchange (GHDx) [WWW Document]. Available online at: http://ghdx.healthdata.org/gbd-results-tool?params=gbd-api-2019-permalink/d780dffbe8a381b25e1416884959e88b (accessed 20, Oct 2021).
3. Aziz R, Steffens DC. What are the causes of late-life depression? Psychiatr Clin North Am. (2013) 36:497–516. doi: 10.1016/j.psc.2013.08.001
4. Kuehner C. Why is depression more common among women than among men? Lancet Psychiat. (2017) 4:146–58. doi: 10.1016/S2215-0366(16)30263-2
5. Labaka A, Goñi-Balentziaga O, Lebeña A, Pérez-Tejada J. Biological sex differences in depression: A systematic review. Biol Res Nurs. (2018) 20:383–92. doi: 10.1177/1099800418776082
6. Slavich GM, Sacher J. Stress, sex hormones, inflammation, and major depressive disorder: Extending social signal transduction theory of depression to account for sex differences in mood disorders. Psychopharmacology. (2019) 236:3063–79. doi: 10.1007/s00213-019-05326-9
7. Slopen N, Williams DR, Fitzmaurice GM, Gilman SE. Sex, stressful life events, and adult onset depression and alcohol dependence: Are men and women equally vulnerable? Soc Sci Med. (2011) 73:615–22. doi: 10.1016/j.socscimed.2011.06.022
8. Beekman ATF, Geerlings SW, Deeg DJH, Smit JH, Schoevers RS, de Beurs E, et al. The Natural History of Late-Life Depression. Arch Gen Psychia. (2002) 59:605–11. doi: 10.1001/archpsyc.59.7.605
9. Birrer RB, Vemuri SP. Depression in later life: a diagnostic and therapeutic challenge. Am Fam Physician. (2004) 69:2375–82.
10. Blazer DG. Depression in late life: review and commentary. J Gerontol. (2003) 58A:249–65. doi: 10.1093/gerona/58.3.M249
11. Burke WJ, Wengel SP. Late-life mood disorders. Clin Geriatr Med. (2003) 19:777–97. doi: 10.1016/S0749-0690(03)00041-7
12. Bessette KL, Karstens AJ, Crane NA, Peters AT, Stange JP, Elverman KH, et al. A lifespan model of interference resolution and inhibitory control: risk for depression and changes with illness progression. Neuropsychol Rev. (2020) 30:477–98. doi: 10.1007/s11065-019-09424-5
13. Salk RH, Hyde JS, Abramson LY. Gender differences in depression in representative national samples: Meta-analyses of diagnoses and symptoms. Psychol Bull. (2017) 143:783–822. doi: 10.1037/bul0000102
14. Bangasser DA, Cuarenta A. Sex differences in anxiety and depression: circuits and mechanisms. Nat Rev Neurosci. (2021) 22:674–84. doi: 10.1038/s41583-021-00513-0
15. Marcus SM, Young EA, Kerber KB, Kornstein S, Farabaugh AH, Mitchell J, et al. Gender differences in depression: Findings from the STAR*D study. J Affect Disord. (2005) 87:141–50. doi: 10.1016/j.jad.2004.09.008
16. Hyde JS, Mezulis AH. Gender differences in depression: biological, affective, cognitive, and sociocultural factors. Harv Rev Psychiatry. (2020) 28:4–13. doi: 10.1097/HRP.0000000000000230
17. Wang L, Xue Y, Cao S, Xie Y, Wu C, Ruffaner-Hanson CD, et al. Sex differences in the cognitive function of first-diagnosed, drug-naïve depressed patients: An observational case-control study. J Affect Disord. (2020) 276:461–6. doi: 10.1016/j.jad.2020.07.114
18. Hinton L, Zweifach M, Tang L, Unützer J, Oishi S. Gender disparities in the treatment of late-life depression: qualitative and quantitative findings from the IMPACT trial. Am J Geriatr Psychiatry. (2006) 14:884–92. doi: 10.1097/01.JGP.0000219282.32915.a4
19. Lavretsky H. Sex differences in brain structure in geriatric depression. Am J Geriatr Psychiatry. (2004) 12:653–7. doi: 10.1097/00019442-200411000-00012
20. Sonnenberg CM, Beekman ATF, Deeg DJH, van Tilburg W. Sex differences in late-life depression. Acta Psychiatr Scand. (2000) 101:286–92. doi: 10.1111/j.1600-0447.2000.tb10927.x
21. Delvecchio G, Maggioni E, Pigoni A, Crespo-Facorro B, Nenadić I, Benedetti F, et al. (2021). Sexual regional dimorphism of post-adolescent and middle age brain maturation. A multi-center 3T MRI study. Front Aging Neurosci. 13. doi: 10.3389/fnagi.2021.622054
22. Giedd JN, Raznahan A, Mills KL, Lenroot RK. Review: magnetic resonance imaging of male/female differences in human adolescent brain anatomy. Biol Sex Differ. (2012) 3:19. doi: 10.1186/2042-6410-3-19
23. Qiu A, Fortier M, Bai J, Zhang X, Chong Y-S, Kwek K, et al. Morphology and microstructure of subcortical structures at birth: A large-scale Asian neonatal neuroimaging study. Neuroimage. (2013) 65:315–23. doi: 10.1016/j.neuroimage.2012.09.032
24. Ritchie SJ, Cox SR, Shen X, Lombardo M, Reus LM, Alloza C, et al. Sex differences in the adult human brain: evidence from 5216 UK biobank participants. Cerebral Cortex. (2018) 28:2959–75. doi: 10.1093/cercor/bhy109
25. Ruigrok ANV, Salimi-Khorshidi G, Lai MC, Baron-Cohen S, Lombardo M, Tait RJ, et al. (2014). A meta-analysis of sex differences in human brain structure. Neurosci Biobehav Rev. 39, 34–50. doi: 10.1016/j.neubiorev.2013.12.004
26. Kakimoto A, Ito S, Okada H, Nishizawa S, Minoshima S, Ouchi Y. Age-related sex-specific changes in brain metabolism and morphology. J Nuclear Med. (2016) 57:221–5. doi: 10.2967/jnumed.115.166439
27. Frodl T, Meisenzahl EM, Zetzsche T, Born C, Groll C, Jäger M, et al. Hippocampal changes in patients with a first episode of major depression. Am J Psychiatry. (2002) 159:1112–8. doi: 10.1176/appi.ajp.159.7.1112
28. Kong L, Chen K, Womer F, Jiang W, Luo X, Driesen N, et al. Sex differences of gray matter morphology in cortico-limbic-striatal neural system in major depressive disorder. J Psychiatr Res. (2013) 47:733–9. doi: 10.1016/j.jpsychires.2013.02.003
29. Videbech P. Hippocampal volume and depression: A meta-analysis of MRI studies. Am J Psychiatry. (2004) 161:1957–66. doi: 10.1176/appi.ajp.161.11.1957
30. Yang X, Peng Z, Ma X, Meng Y, Li M, Zhang J, et al. Sex differences in the clinical characteristics and brain gray matter volume alterations in unmedicated patients with major depressive disorder. Sci Rep. (2017) 7:2515. doi: 10.1038/s41598-017-02828-4
31. Jenkins LM, Kendall AD, Kassel MT, Patrón VG, Gowins JR, Dion C, et al. Considering sex differences clarifies the effects of depression on facial emotion processing during fMRI. J Affect Disord. (2018) 225:129–36. doi: 10.1016/j.jad.2017.08.027
32. Young KD, Bodurka J, Drevets WC. Functional neuroimaging of sex differences in autobiographical memory recall in depression. Psychol Med. (2017) 47:2640–52. doi: 10.1017/S003329171700112X
33. Jaeger J, Berns S, Uzelac S, Davis-Conway S. Neurocognitive deficits and disability in major depressive disorder. Psychiatry Res. (2006) 145:39–48. doi: 10.1016/j.psychres.2005.11.011
34. Keller AS, Leikauf JE, Holt-Gosselin B, Staveland BR, Williams LM. Paying attention to attention in depression. Transl Psychiatry. (2019) 9:279. doi: 10.1038/s41398-019-0616-1
35. Knight MJ, Lyrtzis E, Baune BT. The association of cognitive deficits with mental and physical Quality of Life in Major Depressive Disorder. Compr Psychiatry. (2020) 97:152147. doi: 10.1016/j.comppsych.2019.152147
36. Nilsson J, Thomas AJ, Stevens LH, McAllister-Williams RH, Ferrier IN, Gallagher P. The interrelationship between attentional and executive deficits in major depressive disorder. Acta Psychiatr Scand. (2016) 134:73–82. doi: 10.1111/acps.12570
37. Wang X, Zhou H, Zhu X. Attention deficits in adults with Major depressive disorder: A systematic review and meta-analysis. Asian J Psychiatr. (2020) 53:102359. doi: 10.1016/j.ajp.2020.102359
38. Georgiou G, Essau CA. Go/No-Go Task, in Encyclopedia of Child Behavior and Development. Goldstein S, Naglieri JA, editors. Boston, MA: Springer US. (2011) p. 705–706. doi: 10.1007/978-0-387-79061-9_1267
39. Gomez P, Ratcliff R, Perea M. A model of the go/no-go task. J Exp Psychol Gen. (2007) 136:389–413. doi: 10.1037/0096-3445.136.3.389
40. Chuang J-Y, Hagan CC, Murray GK, Graham JME, Ooi C, Tait R, et al. Adolescent major depressive disorder: neuroimaging evidence of sex difference during an affective Go/No-Go task. Front Psychiatry. (2017) 8:1–12. doi: 10.3389/fpsyt.2017.00119
41. van Deurzen PAM, Buitelaar JK, Agnes Brunnekreef J, Ormel J, Minderaa RB, Hartman CA, et al. Response time variability and response inhibition predict affective problems in adolescent girls, not in boys: the TRAILS study. Eur Child Adolesc Psychiatry. (2012) 21:277–87. doi: 10.1007/s00787-012-0260-2
42. Melynyte S, Ruksenas O, Griskova-Bulanova I. Sex differences in equiprobable auditory Go/NoGo task: effects on N2 and P3. Exp Brain Res. (2017) 235:1565–74. doi: 10.1007/s00221-017-4911-x
43. Omura K, Kusumoto K. Sex differences in neurophysiological responses are modulated by attentional aspects of impulse control. Brain Cogn. (2015) 100:49–59. doi: 10.1016/j.bandc.2015.09.006
44. Liu J, Zubieta J-K, Heitzeg M. Sex differences in anterior cingulate cortex activation during impulse inhibition and behavioral correlates. Psychiat Res: Neuroimag. (2012) 201:54–62. doi: 10.1016/j.pscychresns.2011.05.008
45. Alshammari TK. Sexual dimorphism in pre-clinical studies of depression. Prog Neuropsychopharmacol Biol Psychiatry. (2021) 105:110120. doi: 10.1016/j.pnpbp.2020.110120
46. Tozzi L, Goldstein-Piekarski AN, Korgaonkar MS, Williams LM. Connectivity of the Cognitive Control Network During Response Inhibition as a Predictive and Response Biomarker in Major Depression: Evidence From a Randomized Clinical Trial. Biol Psychiatry. (2020) 87:462–72. doi: 10.1016/j.biopsych.2019.08.005
48. Lovibond PF, Lovibond SH. The structure of negative emotional states: comparison of the Depression Anxiety Stress Scales (DASS) with the Beck Depression and Anxiety Inventories. Behav Res Ther. (1995) 33:335–43. doi: 10.1016/0005-7967(94)00075-u
49. Nijenhuis ERS, Van der Hart O, Kruger K. The psychometric characteristics of the Traumatic Experiences Checklist (TEC): First findings among psychiatric outpatients. Clin Psychol Psychother. (2002)9:200–10. doi: 10.1002/cpp.332
50. Randolph C, Tierney MC, Mohr E, Chase TN. The Repeatable Battery for the Assessment of Neuropsychological Status (RBANS): preliminary clinical validity. J Clin Exp Neuropsychol. (1998) 20:310–9. doi: 10.1076/jcen.20.3.310.823
51. Friston K. Statistical Parametric Mapping. Neuroscience Databases. (2003) 85:237–250. doi: 10.1007/978-1-4615-1079-6_16
52. Gaser C, Robert D. CAT-A Computational Anatomy Toolbox for the Analysis of Structural MRI Data. (2016). Available Online at: http://www.neuro.uni-jena.de/hbm2016/GaserHBM2016.pdf
53. Jenkinson M, Beckmann CF, Behrens TE, Woolrich MW, Smith SM. FSL. Neuroimage. (2012) 62:782–90. doi: 10.1016/j.neuroimage.2011.09.015
54. Tzourio-Mazoyer N, Landeau B, Papathanassiou D, Crivello F, Etard O, Delcroix N, et al. Automated anatomical labeling of activations in SPM using a macroscopic anatomical parcellation of the MNI MRI single-subject brain. Neuroimage. (2002) 15:273–89. doi: 10.1006/nimg.2001.0978
55. Sramek JJ, Murphy MF, Cutler NR. Sex differences in the psychopharmacological treatment of depression. Dialogues Clin Neurosci. (2016) 18:447-457. doi: 10.31887/DCNS.2016.18.4/ncutler
56. El-Baba RM, Schury MP. Neuroanatomy, frontal cortex. StatPearls. Treasure Island (FL): StatPearls Publishing. (2020).
57. Euston DR, Gruber AJ, McNaughton BL. The Role of Medial Prefrontal Cortex in Memory and Decision Making. Neuron. (2012) 76:1057–70. doi: 10.1016/j.neuron.2012.12.002
58. Gabrieli JDE, Poldrack RA, Desmond JE. The role of left prefrontal cortex in language and memory. Proc Nat Acad Sci. (1998) 95:906–13. doi: 10.1073/pnas.95.3.906
59. Tekin S, Cummings JL. Frontal-subcortical neuronal circuits and clinical neuropsychiatry An update. J Psychosom Res. (2002) 53:647–54. doi: 10.1016/S0022-3999(02)00428-2
60. Drevets WC, Savitz J, Trimble M. The Subgenual Anterior Cingulate Cortex in Mood Disorders. CNS Spectr. (2008) 13:663–81. doi: 10.1017/S1092852900013754
61. Ochsner KN, Knierim K, Ludlow DH, Hanelin J, Ramachandran T, Glover G, et al. Reflecting upon feelings: An fMRI study of neural systems supporting the attribution of emotion to self and other. J Cogn Neurosci. (2004) 16:1746–72. doi: 10.1162/0898929042947829
62. Steele JD, Currie J, Lawrie SM, Reid I. Prefrontal cortical functional abnormality in major depressive disorder: A stereotactic meta-analysis. J Affect Disord. (2007) 101:1–11. doi: 10.1016/j.jad.2006.11.009
63. Zhang F-F, Peng W, Sweeney JA, Jia Z-Y, Gong Q-Y. Brain structure alterations in depression: Psychoradiological evidence. CNS Neurosci Therapeut. (2018) 24:994–1003. doi: 10.1111/cns.12835
64. Fitzgerald PB, Laird AR, Maller J, Daskalakis ZJ. A meta-analytic study of changes in brain activation in depression. Hum Brain Mapp. (2008) 29:683–95. doi: 10.1002/hbm.20426
65. Kringelbach M. The functional neuroanatomy of the human orbitofrontal cortex: evidence from neuroimaging and neuropsychology. Progress in Neurobiol. (2004) 72:341–72. doi: 10.1016/j.pneurobio.2004.03.006
66. Feis D-L, Brodersen KH, von Cramon DY, Luders E, Tittgemeyer M. Decoding gender dimorphism of the human brain using multimodal anatomical and diffusion MRI data. Neuroimage. (2013) 70:250–7. doi: 10.1016/j.neuroimage.2012.12.068
67. Im K, Lee JM, Lee J, Shin YW, Kim IY, Kwon JS, et al. Gender difference analysis of cortical thickness in healthy young adults with surface-based methods. Neuroimage. (2006) 31:31–8. doi: 10.1016/j.neuroimage.2005.11.042
68. Bremner JD, Vythilingam M, Vermetten E, Nazeer A, Adil J, Khan S, et al. Reduced volume of orbitofrontal cortex in major depression. Biol Psychiatry. (2002) 51:273–9. doi: 10.1016/S0006-3223(01)01336-1
69. Eleonora M, Giuseppe D, Marika G, Marco G, Sara P, Carolina B, et al. Common and different neural markers in major depression and anxiety disorders: A pilot structural magnetic resonance imaging study. Psychiatry Research - Neuroimag. (2019) 290:42–50. doi: 10.1016/j.pscychresns.2019.06.006
70. Otte C, Gold SM, Penninx BW, Pariante CM, Etkin A, Fava M, et al. Major depressive disorder. Nature Reviews. Disease Primers. (2016) 2:16065. doi: 10.1038/nrdp.2016.65
71. Pandya M, Altinay M, Malone DA, Anand A. Where in the Brain Is Depression? Curr Psychiatry Rep. (2012) 14:634–42. doi: 10.1007/s11920-012-0322-7
72. Ribeiz SRI, Duran F, Oliveira MC, Bezerra D, Castro CC, Steffens DC, et al. Structural brain changes as biomarkers and outcome predictors in patients with late-life depression: a cross-sectional and prospective study. PLoS ONE. (2013) 8:e80049. doi: 10.1371/journal.pone.0080049
73. Lorenzetti V, Allen NB, Fornito A, Yücel M. Structural brain abnormalities in major depressive disorder: A selective review of recent MRI studies. J Affect Disord. (2009) 117:1–17. doi: 10.1016/j.jad.2008.11.021
74. Sexton CE, Allan C. L., le Masurier M., McDermott L. M., Kalu U. G., Herrmann L. L., et al. (2012). Magnetic resonance imaging in late-life depression. Arch Gen Psychiatry. 69, 680–689. doi: 10.1001/archgenpsychiatry.2011.1862
75. Duman RS. Sex-specific disease-associated modules for depression. Nat Med. (2017) 23:1015–7. doi: 10.1038/nm.4391
76. Labonté B, Engmann O, Purushothaman I, Menard C, Wang J, Tan C, et al. Sex-specific transcriptional signatures in human depression. Nat Med. (2017) 23:1102–11. doi: 10.1038/nm.4386
77. Seney ML, Huo Z, Cahill K, French L, Puralewski R, Zhang J, et al. Opposite Molecular Signatures of Depression in Men and Women. Biol Psychiatry. (2018) 84:18–27. doi: 10.1016/j.biopsych.2018.01.017
78. Soudry Y, Lemogne C, Malinvaud D, Consoli SM, Bonfils P. Olfactory system and emotion: Common substrates. Eur Ann Otorhinolaryngol Head Neck Dis. (2011) 128:18–23. doi: 10.1016/j.anorl.2010.09.007
79. Garcia-Falgueras A., Junque C., Giménez M., Caldú X., Segovia S., Guillamon A. (2006). Sex differences in the human olfactory system. Brain Research (1116). 103–111. doi: 10.1016/j.brainres.2006.07.115
80. Sorokowski P, Karwowski M, Misiak M, Marczak MK, Dziekan M, Hummel T, et al. Sex differences in human olfaction: a meta-analysis. Front Psychol. (2019) 10. doi: 10.3389/fpsyg.2019.00242
81. Price JL. Olfactory Higher Centers Anatomy. In: Squire LR, editors. Encyclopedia of Neuroscience. Amsterdam; Oxford: Elsevier Ltd; Academic Press. (2009) p. 129–136. doi: 10.1016/B978-008045046-9.01692-2
82. Atanasova B, Graux J, el Hage W, Hommet C, Camus V, Belzung C. Olfaction: A potential cognitive marker of psychiatric disorders. Neurosci Biobehav Rev. (2008) 32:1315–25. doi: 10.1016/j.neubiorev.2008.05.003
83. Takahashi T, Nishikawa Y, Yücel M, Whittle S, Lorenzetti V, Walterfang M, et al. Olfactory sulcus morphology in patients with current and past major depression. Psychiat Res Neuroimag. (2016) 255:60–5. doi: 10.1016/j.pscychresns.2016.07.008
84. Yuan T-F, Slotnick BM. Roles of olfactory system dysfunction in depression. Prog Neuropsychopharmacol Biol Psychiatry. (2014) 54:26–30. doi: 10.1016/j.pnpbp.2014.05.013
85. Meadows M-E. Calcarine Cortex, in: Encyclopedia of Clinical Neuropsychology. New York, NY: Springer New York. (2011). doi: 10.1007/978-0-387-79948-3_1348
86. Amunts K, Armstrong E, Malikovic A, Homke L, Mohlberg H, Schleicher A, et al. Gender-Specific Left-Right Asymmetries in Human Visual Cortex. J Neurosci. (2007) 27:1356–64. doi: 10.1523/JNEUROSCI.4753-06.2007
87. Vanston JE, Strother L. Sex differences in the human visual system. J Neurosci Res. (2017) 95:617–25. doi: 10.1002/jnr.23895
88. Baldinger-Melich P, Urquijo Castro MF, Seiger R, Ruef A, Dwyer DB, Kranz GS, et al. Sex matters: a multivariate pattern analysis of sex- and gender-related neuroanatomical differences in cis- and transgender individuals using structural magnetic resonance imaging. Cerebral Cortex. (2020) 30:1345–56. doi: 10.1093/cercor/bhz170
89. Goldstein JM. Normal sexual dimorphism of the adult human brain assessed by in vivo magnetic resonance imaging. Cerebral Cortex. (2001) 11:490–7. doi: 10.1093/cercor/11.6.490
90. Grieve SM, Korgaonkar MS, Koslow SH, Gordon E, Williams LM. Widespread reductions in gray matter volume in depression. NeuroImage: Clinical. (2013) 3:332–9. doi: 10.1016/j.nicl.2013.08.016
91. Li Q, Zhao Y, Chen Z, Long J, Dai J, Huang X, et al. Meta-analysis of cortical thickness abnormalities in medication-free patients with major depressive disorder. Neuropsychopharmacology. (2020) 45:703–12. doi: 10.1038/s41386-019-0563-9
92. Cui X, Liu F, Chen J, Xie G, Wu R, Zhang Z, et al. Voxel-wise brain-wide functional connectivity abnormalities in first-episode, drug-naive patients with major depressive disorder. Am J Med Genet B Neuropsychiatr Genet. (2018) 177:447–53. doi: 10.1002/ajmg.b.32633
93. Ries A, Hollander M, Glim S, Meng C, Sorg C, Wohlschläger A. Frequency-Dependent Spatial Distribution of Functional Hubs in the Human Brain and Alterations in Major Depressive Disorder. Front Hum Neurosci. (2019) 13. doi: 10.3389/fnhum.2019.00146
94. Epstein J, Perez DL, Ervin K, Pan H, Kocsis JH, Butler T, et al. Failure to segregate emotional processing from cognitive and sensorimotor processing in major depression. Psychiat Res: Neuroimag. (2011) 193:144–50. doi: 10.1016/j.pscychresns.2011.01.012
95. Xia M, Si T, Sun X, Ma Q, Liu B, Wang L, et al. Reproducibility of functional brain alterations in major depressive disorder: Evidence from a multisite resting-state functional MRI study with 1,434 individuals. Neuroimage. (2019) 189:700–14. doi: 10.1016/j.neuroimage.2019.01.074
96. Zeng L-L, Shen H, Liu L, Wang L, Li B, Fang P, et al. Identifying major depression using whole-brain functional connectivity: a multivariate pattern analysis. Brain. (2012) 135:1498–507. doi: 10.1093/brain/aws059
97. Alves PN, Foulon C, Karolis V, Bzdok D, Margulies DS, Volle E, et al. An improved neuroanatomical model of the default-mode network reconciles previous neuroimaging and neuropathological findings. Communicat Biol. (2019) 2:370. doi: 10.1038/s42003-019-0611-3
98. Utevsky A, Smith D, Huettel SA. Precuneus is a functional core of the default-mode network. J Neurosci. (2014) 34:932–40. doi: 10.1523/JNEUROSCI.4227-13.2014
99. Menon V. Large-scale brain networks and psychopathology: A unifying triple network model. Trends Cogn Sci. (2011) 15:483–506. doi: 10.1016/j.tics.2011.08.003
100. Buckner RL, DiNicola LM. The brain's default network: updated anatomy, physiology and evolving insights. Nat Rev Neurosci. (2019) 20:593–608. doi: 10.1038/s41583-019-0212-7
101. Knyazev GG, Savostyanov AN, Bocharov A, Brak I, Osipov EA, Filimonova EA, et al. Task-positive and task-negative networks in major depressive disorder: A combined fMRI and EEG study. J Affect Disord. (2018) 235:211–9. doi: 10.1016/j.jad.2018.04.003
102. Sheline YI, Barch DM, Price JL, Rundle MM, Vaishnavi SN, Snyder AZ, et al. The default mode network and self-referential processes in depression. Proc Nat Acad Sci. (2009) 106:1942–7. doi: 10.1073/pnas.0812686106
103. Tadayonnejad R, Yang S, Kumar A, Ajilore O. Multimodal Brain Connectivity Analysis in Unmedicated Late-Life Depression. PLoS ONE. (2014) 9:e96033. doi: 10.1371/journal.pone.0096033
104. Gong Q, He Y. Depression, neuroimaging and connectomics: a selective overview. Biol Psychiatry. (2015) 77:223–35. doi: 10.1016/j.biopsych.2014.08.009
105. Kaiser RH, Andrews-Hanna JR, Wager TD, Pizzagalli DA. Large-scale network dysfunction in major depressive disorder. JAMA Psychiatry. (2015) 72:603. doi: 10.1001/jamapsychiatry.2015.0071
106. Richard-Devantoy S, Ding Y, Lepage M, Turecki G, Jollant F. Cognitive inhibition in depression and suicidal behavior: A neuroimaging study. Psychol Med. (2016) 46:933–44. doi: 10.1017/S0033291715002421
107. Cavanna AE, Trimble MR. The precuneus: a review of its functional anatomy and behavioural correlates. Brain. (2006) 129:564–83. doi: 10.1093/brain/awl004
108. Rolls ET, Cheng W, Gilson M, Qiu J, Hu Z, Ruan H, et al. Effective connectivity in depression. Biol Psychiatry Cogn Neurosci Neuroimaging. (2018) 3:187–97. doi: 10.1016/j.bpsc.2017.10.004
109. Cooney RE, Joormann J, Eugène F, Dennis EL, Gotlib IH. Neural correlates of rumination in depression. Cogn Affect Behav Neurosci. (2010) 10:470–8. doi: 10.3758/CABN.10.4.470
110. Zhou H. X., Chen X., Shen Y. Q., Li L., Chen N. X., Zhu Z. C., et al. (2020). Rumination and the default mode network: Meta-analysis of brain imaging studies and implications for depression. NeuroImage. doi: 10.1016/j.neuroimage.2019.116287
111. Mendoza JE. (2011). Lingual Gyrus. In: Kreutzer J, DeLuca J, Caplan B, editors. Encyclopedia of Clinical Neuropsychology. New York: Springer New York. p. 1468. doi: 10.1007/978-0-387-79948-3_747
112. Gong J, Wang J, Qiu S, Chen P, Luo Z, Wang J, et al. Common and distinct patterns of intrinsic brain activity alterations in major depression and bipolar disorder: voxel-based meta-analysis. Transl Psychiatry. (2020) 10:353. doi: 10.1038/s41398-020-01036-5
113. Gohier B., Ferracci L, Surguladze SA, Lawrence E, el Hage W, Kefi MZ, et al. (2009). Cognitive inhibition and working memory in unipolar depression. J Affect Disord. 116:100–5. doi: 10.1016/j.jad.2008.10.028
114. Kozlovskiy SA, Pyasik M, Korotkova A, Vartanov AV, Kiselnikov A, Glozman J. Selective involvement of lingual gyrus in working memory and perception of different types of visual stimuli. J Int Neuropsychol Soc. (2014) 20. doi: 10.1017/S1355617714000915
115. Monnart A, Kornreich C, Verbanck P, Campanella S. Just swap out of negative vibes? Rumination and inhibition deficits in major depressive disorder: data from event-related potentials studies. Front Psychol. (2016) 7. doi: 10.3389/fpsyg.2016.01019
116. Sahib AK, Loureiro JRA, Vasavada MM, Kubicki A, Wade B, Joshi SH, et al. Modulation of inhibitory control networks relate to clinical response following ketamine therapy in major depression. Transl Psychiatry. (2020) 10:260. doi: 10.1038/s41398-020-00947-7
117. Liu T, Slotnick SD, Serences JT, Yantis S. Cortical mechanisms of feature-based attentional control. Cerebral Cortex. (2003) 13:1334–43. doi: 10.1093/cercor/bhg080
118. Guo W, Liu F, Xue Z, Gao K, Liu Z, Xiao C, et al. Decreased interhemispheric coordination in treatment-resistant depression: a resting-state fMRI study. PLoS ONE. (2013) 8:e71368. doi: 10.1371/journal.pone.0071368
119. Desseilles M, Balteau E, Sterpenich V, Dang-Vu TT, Darsaud A, Vandewalle G, et al. Abnormal Neural Filtering of Irrelevant Visual Information in Depression. J Neurosci. (2009) 29:1395–403. doi: 10.1523/JNEUROSCI.3341-08.2009
120. Thériault R-K, Perreault ML. Hormonal regulation of circuit function: sex, systems and depression. Biol Sex Differ. (2019) 10:12. doi: 10.1186/s13293-019-0226-x
121. Baumann O, Mattingley JB. Functional organization of the parahippocampal cortex: dissociable roles for context representations and the perception of visual scenes. J Neurosci. (2016) 36:2536–42. doi: 10.1523/JNEUROSCI.3368-15.2016
122. RajMohan V, Mohandas E. The limbic system. Indian J Psychiatry. (2007) 49:132. doi: 10.4103/0019-5545.33264
123. Lui S, Zhou XJ, Sweeney JA, Gong Q. Psychoradiology: the frontier of neuroimaging in psychiatry. Radiology. (2016) 281:357–72. doi: 10.1148/radiol.2016152149
124. Mao N, Zheng H, Long Z, Yao L, Wu X. Gender differences in dynamic functional connectivity based on resting-state fMRI. In: 39th Annual International Conference of the IEEE Engineering in Medicine and Biology Society (EMBC). (2017) 2940–3. doi: 10.1109/EMBC.2017.8037473
125. Piefke M, Weiss PH, Markowitsch HJ, Fink GR. Gender differences in the functional neuroanatomy of emotional episodic autobiographical memory. Hum Brain Mapp. (2005) 24:313–24. doi: 10.1002/hbm.20092
126. Hill AC, Laird AR, Robinson JL. Gender differences in working memory networks: a BrainMap meta-analysis. Biol Psychol. (2014) 102:18–29. doi: 10.1016/j.biopsycho.2014.06.008
127. Andrews-Hanna JR, Smallwood J, Spreng RN. The default network and self-generated thought: component processes, dynamic control, and clinical relevance. Ann N Y Acad Sci. (2014) 1316. doi: 10.1111/nyas.12360
128. Ward AM, Schultz AP, Huijbers W, van Dijk KRA, Hedden T, Sperling RA. The parahippocampal gyrus links the default-mode cortical network with the medial temporal lobe memory system. Hum Brain Mapp. (2014) 35:1061–73. doi: 10.1002/hbm.22234
129. Harel EV, Tennyson RL, Fava M, Bar M. Linking major depression and the neural substrates of associative processing. Cogn Affect Behav. (2016) 16:1017–26. doi: 10.3758/s13415-016-0449-9
130. Olson IR, Plotzker A, Ezzyat Y. The Enigmatic temporal pole: a review of findings on social and emotional processing. Brain. (2007) 130:1718–31. doi: 10.1093/brain/awm052
131. Rempel-Clower NL. Role of Orbitofrontal Cortex Connections in Emotion. Annals of the New York Academy of Sciences. (2007) 1121:72–86. doi: 10.1196/annals.1401.026
Keywords: major depressive disorder, sex differences, brain morphology, brain function, inhibitory control, adult-onset depression
Citation: Piani MC, Maggioni E, Delvecchio G, Ferro A, Gritti D, Pozzoli SM, Fontana E, Enrico P, Cinnante CM, Triulzi FM, Stanley JA, Battaglioli E and Brambilla P (2022) Sexual Dimorphism in the Brain Correlates of Adult-Onset Depression: A Pilot Structural and Functional 3T MRI Study. Front. Psychiatry 12:683912. doi: 10.3389/fpsyt.2021.683912
Received: 26 March 2021; Accepted: 09 December 2021;
Published: 05 January 2022.
Edited by:
Anita Riecher-Rössler, University of Basel, SwitzerlandReviewed by:
Jiajia Zhu, First Affiliated Hospital of Anhui Medical University, ChinaJie-Yu Chuang, University of Cambridge, United Kingdom
Copyright © 2022 Piani, Maggioni, Delvecchio, Ferro, Gritti, Pozzoli, Fontana, Enrico, Cinnante, Triulzi, Stanley, Battaglioli and Brambilla. This is an open-access article distributed under the terms of the Creative Commons Attribution License (CC BY). The use, distribution or reproduction in other forums is permitted, provided the original author(s) and the copyright owner(s) are credited and that the original publication in this journal is cited, in accordance with accepted academic practice. No use, distribution or reproduction is permitted which does not comply with these terms.
*Correspondence: Paolo Brambilla, cGFvbG8uYnJhbWJpbGxhMUB1bmltaS5pdA==; Eleonora Maggioni, ZWxlb25vcmEubWFnZ2lvbmlAcG9saWNsaW5pY28ubWkuaXQ=
†These authors have contributed equally to this work