- Department of Psychiatry, The Affiliated Brain Hospital of Nanjing Medical University, Nanjing, China
Objective: Age of onset is one of the heterogeneous factors in schizophrenia, and an earlier onset of the disease indicated a worse prognosis. The left superior frontal gyrus (SFG) is involved in numerous cognitive and motor control tasks. Hence, we explored the relationship between abnormal changes in SFG resting-state functional connectivity (rsFC) and cognitive function in the peak age of incidence to understand better the pathophysiological mechanism in youth-onset drug-naïve schizophrenia to search for reliable biomarkers.
Methods: About 66 youth-onset drug-naïve schizophrenia patients and 59 healthy controls (HCs) were included in this study. Abnormal connectivity changes in the left SFG and whole brain were measured using the region of interest (ROI) rsFC analysis method. The cognitive function was assessed using the MATRICS Consensus Cognitive Battery (MCCB), and the severity of the clinical symptoms was evaluated by positive and negative syndrome scale (PANSS). Furthermore, we analyzed the relationships among abnormal FC values, cognition scores, and clinical symptoms.
Results: We found decreased FC between left SFG and bilateral precuneus (PCUN), right hippocampus, right parahippocampal gyrus, left thalamus, left caudate, insula, and right superior parietal lobule (SPL), whereas increased FC was seen between the left SFG and right middle frontal gyrus (MFG) in the youth-onset drug-naïve schizophrenia group, compared with HCs. Meanwhile, the T-scores were lower in each cognitive domain than HCs. Moreover, in the youth-onset drug-naive schizophrenia group, the insula was negatively correlated with processing speed. No significant correlations were found between the FC-value and PANSS score.
Conclusions: Our findings suggest widespread FC network abnormalities in the left SFG and widespread cognitive impairments in the early stages of schizophrenia. The dysfunctional connectivity of the left SFG may be a potential pathophysiological mechanism in youth-onset drug-naïve schizophrenia.
Introduction
Schizophrenia is a highly heterogeneous severe mental disorder (1). Age of onset is an important confounding factor, with the peak onset at 16–25 years old. This age group is defined as youth-onset schizophrenia (2–5). A second small peak occurs after the age of 40 years old, referred to as late-onset schizophrenia. Compared with late-onset schizophrenia, youth-onset schizophrenia experiences fewer life changes and stressors essential for the disease's occurrence and development (6). The earlier the age of onset, the more serious the clinical symptoms and cognitive impairments and the heavier the burden to the society and family. Moreover, the neurodevelopment of young schizophrenia patients is incomplete. Hence, the study of schizophrenia at peak ages may provide abundant evidence to understand neurodevelopmental abnormalities in schizophrenia.
Over the past decades, neuroimaging studies have become a hot research area, and the region of interest (ROI) analysis method was widely used in the etiology of schizophrenia (7). Current studies have confirmed that the pathological mechanism of schizophrenia is associated with the abnormality in multiple neural circuits and networks, including default mode network (DMN), salience network (SN), frontoparietal network (FPN), central executive network (CEN), and frontostriatal-thalamic circuit (8–11). However, previous studies mainly focused on chronic schizophrenia patients. Few studies have targeted specific age groups without considering the effects of drugs and disease progression (8–10). Different ages of onset, medication, and disease duration may lead to different brain structures and function damage patterns.
The application of resting-state functional magnetic resonance imaging (rs-fMRI) in elucidating the changes in brain function in specific dorsolateral prefrontal cortex (DLPFC) subareas [left superior frontal gyrus (SFG)] in the high incidence age group has not been fully explored. The SFG is one of the vital brain regions of the DLPFC. The DLPFC is widely interconnected with almost all cortical and subcortical structures, responsible for cognition and mood (12). There is no doubt that the abnormal resting-state functional connectivity (rsFC) of the DLPFC and other brain regions leads to various mental symptoms and cognitive disorders (13–15). Previous studies mainly focused on the global perspective to understand schizophrenia; however, the results were inconsistent or even opposite (8, 11). Zhou et al. (11) reported decreased FC between the bilateral DLPFC and parietal lobes, posterior cingulate cortex (PCC), thalamus, and striatum, while He et al. (8) found increased connectivity between the SFG and thalamus/caudate. These studies' heterogeneity may be due to differences in small sample size, use of drugs or not, course of the disease, age at onset, and selection of seed points.
Numerous studies have confirmed cognitive impairment in schizophrenia patients, involving various cognitive fields (16–18). Cognitive impairments were regarded as core features, existed at each stage of the disease, played a significant role in the prognosis of schizophrenia, and considered a potential treatment target (19–21). Recent studies have shown that the frontal cortex's abnormal functional connectivity was related to cognitive function in schizophrenia, both anatomically and functionally. An anatomical study suggested that PFC–thalamus' decreased connectivity was associated with impaired working memory in schizophrenia but not associated with cognitive flexibility and inhibition (22). Another study found that functional connectivity between the DLPFC and task-related brain regions was significantly impaired. The study also found significant correlations between functional connectivity of the DLPFC and cognitive performance, behavioral disorder, and overall function (23). However, previous studies' sample size was smaller, and the sample homogeneities were lower; hence, the results were unclear. Moreover, there is no study on the relationships between the FC abnormal brain regions of the left SFG and MATRICS Consensus Cognitive Battery (MCCB) in youth-onset drug-naïve schizophrenia.
Hence, based on previous studies' inadequacy, in this study, we used ROI imaging methods and MCCB neurocognitive measurement tools to explore the relationships among abnormal functional connectivity signal value, cognitive impairments, and clinical symptoms. It was hypothesized that (1) compared with a healthy control (HC) group, patients with youth-onset drug-naïve schizophrenia would show abnormal functional connectivity of the left SFG; (2) the cognitive function of the schizophrenia group decreased significantly in various cognitive domains; and (3) we also explored whether the brain areas with abnormal left SFG FC were related to cognitive and clinical symptoms.
Methods
Participants
A total of 66 youth-onset schizophrenia subjects and 59 HCs were involved in this study. All the youth-onset schizophrenia patients did not take any antipsychotic drugs, and the course of the disease was ≤24 months. Neuroimaging data were stemmed from the Affiliated Brain Hospital of Nanjing Medical University, Jiangsu, China, from September 2015 to December 2019 (Table 1). Diagnosis of schizophrenia was confirmed by two experienced psychiatrists using the Structured Clinical Interview according to the DSM-5 criteria (24). Healthy controls (59) were recruited through advertising in the local community. The following inclusion criteria apply to both groups: Han people, right-handed, aged 16–25, and able to understand survey instructions and execute cognitive tests. The general exclusion criteria included intellectual disability, head injury, substance abuse or dependence, pregnancy, modified electroconvulsive therapy (MECT) or transcranial magnetic stimulation therapy (rTMS), other neuropsychiatric disorders, or contraindications of MRI. These research procedures were in line with the provisions of the review committee of the Affiliated Brain Hospital of Nanjing Medical University. Written informed consent was obtained from all participants before the study.
Neuropsychological and Intelligence Quotient
The Chinese version of the MCCB was implemented by two well-experienced psychiatrists to evaluate cognitive function (25, 26). The MCCB is sensitive to the degree of cognitive impairment in seven domains, including speed of processing, attention/vigilance, working memory, verbal learning, visual learning, reasoning, problem solving, and social cognition (25, 26). Each domain's raw scores were corrected by age, gender, and education to get T-scores. The Wechsler Adult Scale of Intelligence (WAIS) was used to evaluate the intelligence quotient (IQ). The severity of mental symptoms was assessed by the positive and negative syndrome scale (PANSS) (27), which includes a positive, negative, and general psychopathology scale (27).
Image Data Acquisition
Neuroimaging was conducted by Germany Siemens 3.0 T signal scanner at the Department of Radiology, Nanjing Brain Hospital, China. All subjects were asked to relax and stay awake and still, and think nothing. Echo planar imaging (EPI) was used to acquire the BOLD-fMRI images. Details of our scanning parameters are provided in Supplementary Table 3.
Data Preprocessing and Processing
Data Processing Assistant for Resting-State fMRI (DPARSF4.4) advanced edition (http://rfmri.org/DPARSF) was used to preprocess all imaging data (28). The imaging data were calculated in an original space warped by diffeomorphic anatomical registration through exponential Lie algebra (DARTEL). The first four images were discarded for each participant to reduce the influence of noise and magnetic field signal instability, and the remaining imaging was corrected for the acquisition time delay between slices. All subjects with head movement translation >2 mm or rotation angle more than 2° in any direction were excluded (29). Then, functional images were normalized to the Montreal Neurological Institute (MNI) standard space, and the data were resampled in voxels of 3 ×3 × 3 mm size. Low-frequency filtering (0.01 Hz < f < 0.08 Hz, TR = 2 s) was employed to remove the high-frequency drift after removing covariate (whole brain signal, cerebrospinal fluid, and movement) interferences. Finally, the resulting imaging underwent spatial smoothing using 4 × 4 × 4 mm as a Gaussian smoothing kernel to improve the signal-to-noise ratio.
ROI Selections
According to our previous study, meta-analysis results of regional homogeneity (ReHo) abnormal brain changes reported MNI coordinates between schizophrenia and HCs. We chose the left SFG (x = 0, y = 36, z = 48; radius: 6 mm) as the seed point and created FC brain maps with whole brain (see Supplementary Material for details).
Functional Connectivity and Statistical Analysis
The left SFG was selected as seed point and DPARSF software was used to perform functional connectivity analysis. First, the time series of the left SFG were extracted, and correlation analyses were conducted with the average time series of other voxels in the whole brain to acquire a correlation coefficient (r-value). Then, the left SFG functional connection network map of each individual was obtained. Next, Fisher Z transformation was used to convert r-value into z-value to improve normal distribution, thus getting the zFC map of the whole brain for all participants. Finally, zFC-values were used for statistical analysis.
Two-sample t-test was performed using DPABI software (http://rfmri.org/dpabi) to find the FC abnormal brain areas between the youth-onset drug-naïve schizophrenia group and HC group. Age, gender, years of education, and frame-wise displacement (FD) were used as covariates, and threshold-free cluster enhancement (TFCE) method was used for multiple comparison correction, and the significance difference level was p < 0.05 and the cluster size >14 voxels.
Statistical Package for the Social Sciences version 25.0 (SPSS 25.0) was used for statistical analysis. The two-sample t-test was used to compare the difference of demographics and IQ between youth-onset schizophrenia and HCs. Chi-squared test was used for gender. The analysis of covariance was used to analyze the neuropsychological date after taking IQ as a covariate control. Furthermore, Pearson's correlation analysis was performed to examine whether our FC findings were related to cognition and clinical symptoms.
Results
Demographic and Clinical Characteristics
The demographic and clinical characteristics are displayed in Table 1 and Figure 1. There were significant differences in age (p = 0.002), education (p = 0.01), and IQ (p < 0.001) between the two groups (youth-onset schizophrenia group and HC group) and no significant difference in gender (p = 0.11). Considering the cognitive level can be influenced by IQ, the effects of IQ were added to analyze model as a covariate to compare the differences in cognitive domains between the two groups. Compared with HCs, the youth-onset schizophrenia group demonstrated lower scores in each cognitive domain than HCs, especially when processing speed was more obvious.
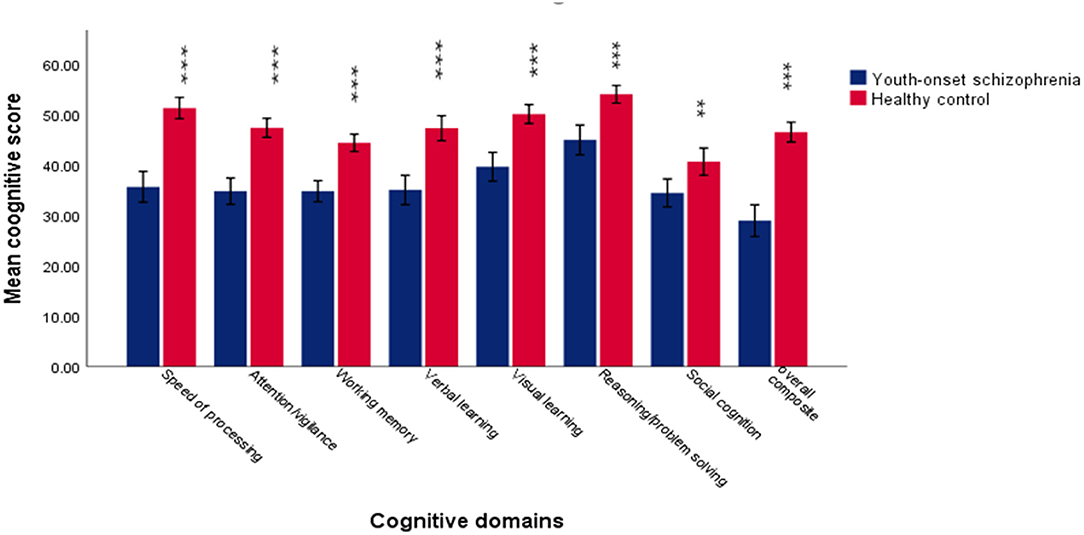
Figure 1. The difference in each cognitive domain between youth-onset schizophrenia and healthy control. The results suggested that patients exhibited significantly lower scores on all the cognitive domains of MCCB. ***Represent p < 0.001. **Represent p < 0.05. Error bars represent standard deviation.
Functional Connectivity Network
The left SFG functional connectivity outcome is shown in Table 2 and Figure 2. Compared with HCs, results indicated that the youth-onset schizophrenia group showed significantly decreased FC between the left SFG and right hippocampus, right parahippocampal gyrus, left thalamus, insula, left caudate, bilateral precuneus, and right superior parietal lobule (SPL), whereas an increased FC-value in the left MFG was shown (p < 0.05, TFCE corrected). Moreover, from Pearson correlation analysis, the insula's FC-value was negatively correlated with processing speed in the youth-onset drug-naïve schizophrenia group (r = −0.313, p = 0.011, uncorrected, Figure 3). No significant correlations were observed between FC-value and PANSS score.
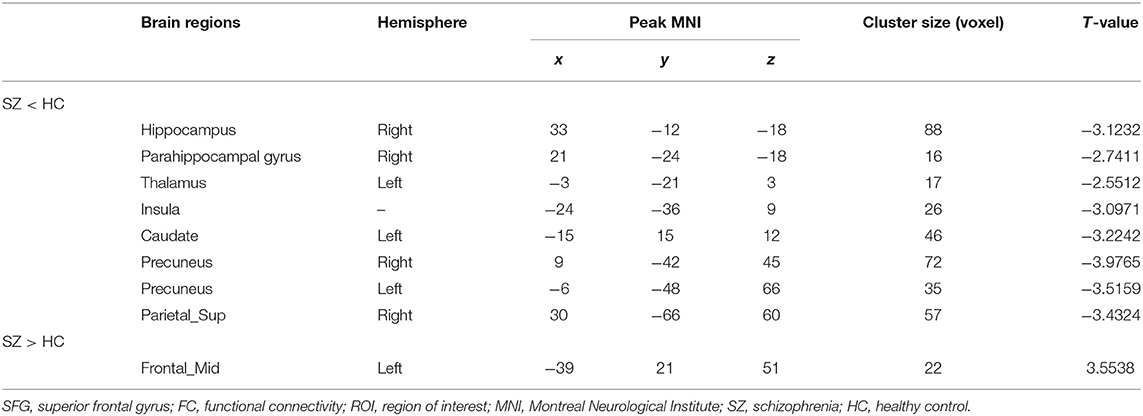
Table 2. Altered regions of left SFG FC based on the ROI analysis between youth-onset schizophrenia and HC.
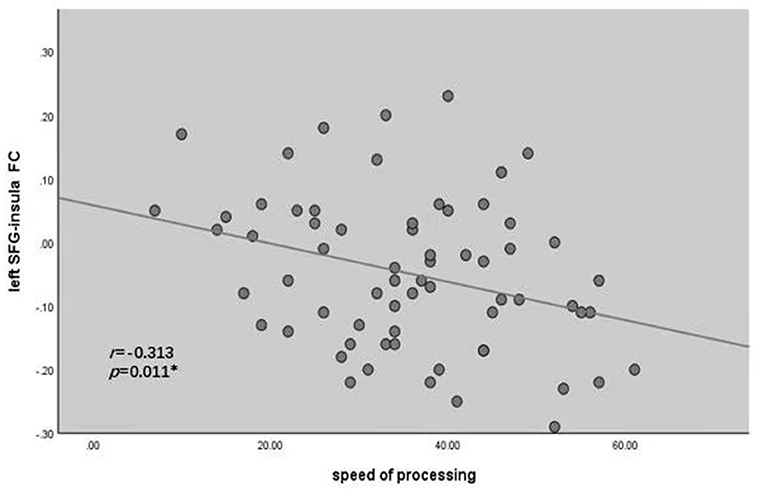
Figure 2. Correlations between the FC of altered regions and cognitive assessments in youth-onset schizophrenia. Pearson's correlation analysis in the patients showed that the decreased FC between left SFG and insula was negatively correlated with processing speed (r = −0.313, *P < 0.05). SFG, Superior Frontal Gyrus; FC, functional connectivity.
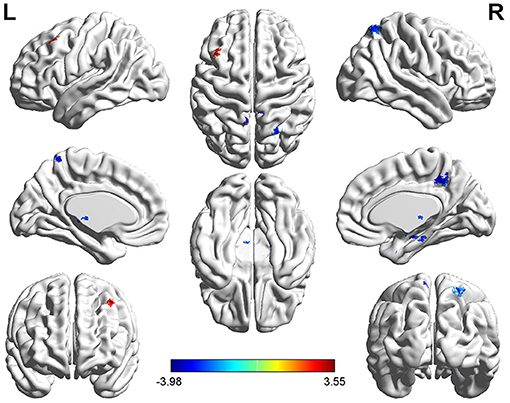
Figure 3. Functional connectivity analysis based on the seed of left SFG between youth-onset schizophrenia and healthy control. The color bar represents T-scores. Warm color represents regions where youth-onset schizophrenia had higher FC, and cold color represents lower FC than HCs. The statistical threshold was set with a combination of voxel-level p < 0.05 and cluster size >14 mm3 voxels for left SFG FC analysis (youth-onset drug naïve schizophrenia vs. HCs), TFCE corrected after controlling age, gender, education, and frame-wise displacement. L, left; R, Right.
Discussion
Our study is the first systematic study to explore the relationships between abnormal brain networks of the left SFG and cognitive impairment using the ROI method and MCCB cognitive tool in drug-naïve young schizophrenia. Notably, we used highly homogeneous individuals who were youth-onset, first episode, and without any antipsychotic drugs, which effectively avoid the influence of age at onset and other confounding factors, thus giving us a new perspective to understand schizophrenia. As expected, compared with HCs, the youth-onset drug-naïve schizophrenia group showed significant differences in several neural loops and networks, including the fronto-limbic pathway, fronto-striatal-thalamic-cortical loops, FPN, DMN, CEN, and SN. Each cognitive domain in patients with drug-naïve youth-onset schizophrenia showed poor performance, consistent with a previous study (16). In addition, we identified significant correlations between decreased FC and cognitive domain scores. Our research found that the insula was negatively correlated with the speed of processing. Therefore, the abnormal FC between the left SFG and insula may be the underlying neural basis of cognitive disorder. Regrettably, we found no correlation between abnormal left SFG functional connectivity and clinical symptoms in the patient's group. Future studies with larger sample sizes need to be used for further exploration.
In the present study, we observed decreased connectivity in the bilateral precuneus (PCUN), right hippocampus, and right parahippocampal gyrus associated with left SFG in youth-onset schizophrenia. These regions are the important node of DMN (30), indicating that FC between the SFG and DMN are destroyed in the early stage of schizophrenia. In the resting state, DMN has a strong spontaneous activity closely related to the human brain's monitoring of the external environment, awareness maintenance, emotion processing, self-introspection, and episodic memory extraction (30). Numerous structural and functional imaging studies have confirmed abnormal DMN (31). A disconnectivity functional survey revealed that some brain regions of the DMN in schizophrenia patients, such as the PCUN and part of the frontal lobe, have abnormal functional connectivity (11). The PCUN plays a vital role in regulating the self-consciousness of DMN, a key point of information transmission (30). Evidence from the cognitive model of schizophrenia suggests that the normal interaction disorder between these regions may be the basis of many related symptoms and cognitive deficits (32). Recent studies on the first-degree relatives of patients with schizophrenia found decreased DMN activity in unaffected siblings of schizophrenic patients (33, 34). Moreover, the hippocampus and parahippocampal gyrus also belong to the limbic system. Recent studies have found that the functional connectivity between the medial prefrontal cortex and hippocampus/parahippocampal gyrus was weakened. The parahippocampal gyrus serves as the primary cortical input to the hippocampus, critically involved in cognition and emotion. The hippocampus is considered an emotional and memory hub. Dysfunctional connectivity between the PFC and hippocampus/parahippocampal gyrus in schizophrenia patients leads to dysregulation in working memory (10, 35). Boyer et al. (36) believed that hippocampal dysfunction might cause abnormal long-term memory in patients with schizophrenia, supported by Zhu et al. (37). A meta-analysis of neural correlates of functional outcome in schizophrenia included 37 structural and 16 functional brain imaging studies. A total of 1,631 schizophrenia patients were examined for neural correlation. They found an abnormal alteration in the fronto-limbic system no matter the functional and structural neuroimaging. They suggested that reduced gray matter in the frontal limbic area impairs functional outcomes and leads to extensive cognitive impairment (38). Another study found that compared to HCs, the internal connections between the hippocampus and prefrontal cortex were damaged in first-episode schizophrenia (FES) patients and an at-risk mental state (ARMS) in delayed matching to sample (DMTS) task (39). As described above, this suggested that left SFG integration disorder appeared before the onset of schizophrenia and maybe a potential endophenotype, which provides a basis for early monitoring and early intervention.
Recently, studies about the insula and cognitive impairment are given attention, particularly in the collaborative management of cognitive impairments with the frontal cortex. Salience network anatomy areas include the insula and anterior cingulate cortex (ACC). The insula is an information hub, mainly involved in processing emotion and cognition (40, 41). A postmortem study showed a general decrease in myelin and glial density, especially in the white matter region that connects the frontal lobe with the rest of the brain (42, 43). Extensive white matter abnormalities in DTI are associated with the decreased speed of processing (44). Another resting-state FC study found significant correlations between several networks and working memory and processing speed, especially in SN, attention network (AN), and DMN in the schizophrenia group (45). Peter et al. found that the DLPFC-insular connection pathway was related to emotion and cognition; the higher the abnormal degree of DLPFC-insular connection, the more severe the emotional and cognitive impairment (46). In addition, some studies have found that the insula plays a regulatory role in the activation vs. inactivation states of the CEN and the DMN. Therefore, structural and functional changes in the insula may have participated in the onset and progression of schizophrenia (47). In the present study, our main observations were decreased FC between the left SFG and insula. Moreover, a negative correlation between the abnormal FC of the left SFG-insula and processing speed was found. Our findings indicate that lower SFG-insula functional connectivity was related to better cognitive function. These findings suggest that SFG functional connectivity abnormalities may have a negative regulatory mechanism on cognitive function in early schizophrenia. In view of the exploratory nature of the analysis, it should be interpreted with caution.
Compared with HCs, we found decreased connectivity between the left SFG and left caudate/thalamus in youth-onset drug-naive schizophrenia. The caudate nucleus is an important part of the striatum. The lesions of dopaminergic neurons in the striatum may lead to many psychiatric symptoms and cognitive disorders. A multimodal study suggests that dysfunction of the prefrontal cortex in schizophrenia was the cause of dopamine metabolism disorder. Meanwhile, the abnormal relationships between dopamine and the prefrontal cortex may be among the leading causes of schizophrenia (48). The connection between the PFC and thalamus/striatum may control the integration of perception, information representation, and the way people think abstract. Based on an ROI study using DLPFC as seed point to analyze the difference between 17 FES patients and 17 healthy subjects, they found decreased functional connectivity between bilateral DLPFC and the parietal lobe, PCC, thalamus, and striatum in patients with FES, which supported our outcome (11). These results suggest that the intrinsic neuronal activity of the fronto-striatal-thalamic-cortical loops was abnormal in young schizophrenia patients, which may be the pathological mechanism of young schizophrenia. However, some authors failed to replicate this result fully and even found increased FC-value in the thalamus and striatum (8). These reasons may have contributed to the heterogeneity in schizophrenia patients. Age at onset may be an important contributing factor, and another factor may be related to the drug and the disease course. In the future, research on patients of different age groups could help in better understanding schizophrenia and help develop personalized service and management.
Moreover, we observed decreased FC between the SFG and SPL, where increased FC was seen between SFG and MFG. These regions are the essential components of the FPN (49), which is related to memory, language attention, spatial attention, and visual processing (50). A previous study confirmed that schizophrenia patients with abnormal FPN, including functional and structural connectivity, are highly correlated with schizophrenia symptom scores and cognitive function (51). Middle frontal gyrus is a significant part of the PFC involving cognition and emotion management (52). Our previous study confirmed that the MFG was related to attention/vigilance and social cognition (16). The SPL is one of the nodes in FPN, which is related to attention. However, in this study, we did not find any relationship between FPN and cognition, where sample size, sample population, and drug might be the main reasons for the controversy of our findings.
Limitation
Although our study tries to keep the sample homogeneity and reduce the influence of confounding factors, there are still some limitations. Firstly, this study is cross-sectional, and the causal relationship between abnormal network changes and cognitive function is still unclear. Further longitudinal follow-up studies could answer these questions. Moreover, age and education were not matched between schizophrenia patients and HCs. However, we have corrected age, sex, and education to analyze crude cognitive scores and imaging data analysis. Lastly, the follow-up study will further expand the sample size and apply more rigorous statistical correction methods to explore the relationship between abnormal functional connectivity and cognition in schizophrenia.
Conclusion
In conclusion, our findings suggest widespread FC network abnormalities in the left SFG and cognitive impairments in the early stages of schizophrenia. The left SFG network contributes significantly to impaired cognition, which may underlie the neuropathological mechanism of schizophrenia, thus further promoting our understanding of frontal cortical dysfunction as a biomarker of cognitive impairments and a neuro-intervention target.
Data Availability Statement
The original contributions presented in the study are included in the article/supplementary material, further inquiries can be directed to the corresponding author/s.
Ethics Statement
The studies involving human participants were reviewed and approved by the review committee of the Affiliated Brain Hospital of Nanjing Medical University. Written informed consent to participate in this study was provided by the participants' legal guardian/next of kin.
Author Contributions
SX and RZ designed the study and revised the manuscript. MZ, WY, JD, and AZ collected the data. XQ and SL analyzed the data and wrote the manuscript. All authors contributed to the article and approved the submitted version.
Funding
This study was partially supported by the Ministry of Science and Technology of China, National Key R&D Program of China (2016YFC1306805), the Key Project of Nanjing Municipal Bureau of Health Commission (ZKX15033), the General Program of Jiangsu commission of health (H2017051), and the Foundation of Nanjing Medical University (NMUB2020222). These institutions had no role in the design of the study; collection, analysis, and interpretation of data; and writing of the manuscript.
Conflict of Interest
The authors declare that the research was conducted in the absence of any commercial or financial relationships that could be construed as a potential conflict of interest.
Publisher's Note
All claims expressed in this article are solely those of the authors and do not necessarily represent those of their affiliated organizations, or those of the publisher, the editors and the reviewers. Any product that may be evaluated in this article, or claim that may be made by its manufacturer, is not guaranteed or endorsed by the publisher.
Acknowledgments
The authors thank the generous contributions of the research participants and they are also grateful to their families for their support.
Supplementary Material
The Supplementary Material for this article can be found online at: https://www.frontiersin.org/articles/10.3389/fpsyt.2021.679642/full#supplementary-material
References
1. Braff DL, Ryan J, Rissling AJ, Carpenter WT. Lack of use in the literature from the last 20 years supports dropping traditional schizophrenia subtypes from DSM-5 and ICD-11. Schizophr Bull. (2013) 39:751–3. doi: 10.1093/schbul/sbt068
2. Castle DJ, Murray RM. The epidemiology of late-onset schizophrenia. Schizophr Bull. (1993) 19:691–700. doi: 10.1093/schbul/19.4.691
3. Kaleda VG. Youth-onset schizophrenia: psychopathology, clinical presentation and therapy. Zhur Nevr Psikhiat SS Korsak. (2015) 115:26. doi: 10.17116/jnevro201511511226-33
4. Jones PB. Adult mental health disorders and their age at onset. Br J Psychiatry. (2013) 54:s5–10. doi: 10.1192/bjp.bp.112.119164
5. Kessler RC, Amminger GP, Aguilar-Gaxiola S, Alonso J, Lee S, Üstün TB. Age of onset of mental disorders: a review of recent literature. Curr Opin Psychiatry. (2007) 20:359–64. doi: 10.1097/YCO.0b013e32816ebc8c
6. Chen L, Selvendra A, Stewart A, Castle D. Risk factors in early and late onset schizophrenia. Compr Psychiatry. (2018) 80:155–62. doi: 10.1016/j.comppsych.2017.09.009
7. Malaspina D, Harkavy-Friedman J, Corcoran C, Mujica-Parodi L, Printz D, Gorman JM, et al. Resting neural activity distinguishes subgroups of schizophrenia patients. Biol Psychiatry. (2004) 56:931–7. doi: 10.1016/j.biopsych.2004.09.013
8. He Y, Wu S, Chen C, Fan L, Li K, Wang G, et al. Organized resting-state functional dysconnectivity of the prefrontal cortex in patients with schizophrenia. Neuroscience. (2020) 446:14–27. doi: 10.1016/j.neuroscience.2020.08.021
9. Zhou Y, Fan L, Qiu C, Jiang T. Prefrontal cortex and the dysconnectivity hypothesis of schizophrenia. Neurosci Bull. (2015) 31:207–19. doi: 10.1007/s12264-014-1502-8
10. Meyer-Lindenberg AS, Olsen RK, Kohn PD, Brown T, Egan MF, Weinberger DR, et al. Regionally specific disturbance of dorsolateral prefrontal-hippocampal functional connectivity in schizophrenia. Arch Gen Psychiatry. (2005) 62:379–86. doi: 10.1001/archpsyc.62.4.379
11. Zhou Y, Liang M, Jiang T, Tian L, Liu Y, Liu Z, et al. Functional dysconnectivity of the dorsolateral prefrontal cortex in first-episode schizophrenia using resting-state fMRI. Neurosci Lett. (2007) 417:297–302. doi: 10.1016/j.neulet.2007.02.081
12. Szczepanski SM, Knight RT. Insights into human behavior from lesions to the prefrontal cortex. Neuron. (2014) 83:1002–18. doi: 10.1016/j.neuron.2014.08.011
13. Repovs G, Csernansky JG, Barch DM. Brain network connectivity in individuals with schizophrenia and their siblings. Biol Psychiatry. (2011) 69:967–73. doi: 10.1016/j.biopsych.2010.11.009
14. Spence SA, Hirsch SR, Brooks DJ, Grasby PM. Prefrontal cortex activity in people with schizophrenia and control subjects. Evidence from positron emission tomography for remission of “hypofrontality” with recovery from acute schizophrenia. Br J Psychiatry. (1998) 172:316–23. doi: 10.1192/bjp.172.4.316
15. Smee C, Krabbendam L, O'Daly O, Prins AM, Nalesnik N, Morley L, et al. An fMRI study of prefrontal dysfunction and symptomatic recovery in schizophrenia. Acta Psychiatr Scand. (2011) 123:440–50. doi: 10.1111/j.1600-0447.2010.01632.x
16. Yan W, Zhang R, Zhou M, Lu S, Li W, Xie S, et al. Relationships between abnormal neural activities and cognitive impairments in patients with drug-naive first-episode schizophrenia. BMC Psychiatry. (2020) 20:283. doi: 10.1186/s12888-020-02692-z
17. Vogel T, Smieskova R, Schmidt A, Walter A, Harrisberger F, Eckert A, et al. Increased superior frontal gyrus activation during working memory processing in psychosis: significant relation to cumulative antipsychotic medication and to negative symptoms. Schizophr Res. (2016) 175:20–6. doi: 10.1016/j.schres.2016.03.033
18. Rodríguez-Sánchez JM, Crespo-Facorro B, González-Blanch C, Pérez-Iglesias R, Vázquez-Barquero JL. Cognitive dysfunction in first-episode psychosis: the processing speed hypothesis. Br J Psychiatry. (2007) 51:s107–10. doi: 10.1192/bjp.191.51.s107
19. Heinrichs RW, Zakzanis KK. Neurocognitive deficit in schizophrenia: a quantitative review of the evidence. Neuropsychology. (1998) 12:426–45. doi: 10.1037/0894-4105.12.3.426
20. Rajji TK, Mulsant BH. Nature and course of cognitive function in late-life schizophrenia: a systematic review. Schizophr Res. (2008) 102:122–40. doi: 10.1016/j.schres.2008.03.015
21. Kahn RS, Keefe RSE. Schizophrenia is a cognitive illness: time for a change in focus. JAMA Psychiatry. (2013) 70:1107–12. doi: 10.1001/jamapsychiatry.2013.155
22. Giraldo-Chica M, Rogers BP, Damon SM, Landman BA, Woodward ND. Prefrontal-thalamic anatomical connectivity and executive cognitive function in schizophrenia. Biol Psychiatry. (2018) 83:509–17. doi: 10.1016/j.biopsych.2017.09.022
23. Yoon JH, Minzenberg MJ, Ursu S, Walters R, Wendelken C, Ragland JD, et al. Association of dorsolateral prefrontal cortex dysfunction with disrupted coordinated brain activity in schizophrenia: relationship with impaired cognition, behavioral disorganization, and global function. Am J Psychiatry. (2008) 165:1006–14. doi: 10.1176/appi.ajp.2008.07060945
24. Messent P. DSM-5. Clin Child Psychol Psychiatry. (2013) 18:479–82. doi: 10.1177/1359104513502138
25. Nuechterlein KH, Green MF, Kern RS, Baade LE, Barch DM, Cohen JD, et al. The MATRICS consensus cognitive battery, part 1: test selection, reliability, and validity. Am J Psychiatry. (2008) 165:203–13. doi: 10.1176/appi.ajp.2007.07010042
26. Kern RS, Nuechterlein KH, Green MF, Baade LE, Fenton WS, Gold JM, et al. The MATRICS consensus cognitive battery, part 2: co-norming and standardization. Am J Psychiatry. (2008) 165:214–20. doi: 10.1176/appi.ajp.2007.07010043
27. Kay SR, Fiszbein A, Opler LA. The positive and negative syndrome scale (PANSS) for schizophrenia. Schizophr Bull. (1987) 13:261–76. doi: 10.1093/schbul/13.2.261
28. Yan CG, Wang X Di, Zuo XN, Zang YF, DPABI. Data processing and analysis for (resting-state) brain imaging. Neuroinformatics. (2016) 14:339–51. doi: 10.1007/s12021-016-9299-4
29. Jenkinson M, Bannister P, Brady M, Smith S. Improved optimization for the robust and accurate linear registration and motion correction of brain images. Neuroimage. (2002) 17:825–41. doi: 10.1016/S1053-8119(02)91132-8
30. Raichle ME. The brain's default mode network. Annu Rev Neurosci. (2015) 38:433–47. doi: 10.1146/annurev-neuro-071013-014030
31. Gao X, Zhang W, Yao L, Xiao Y, Liu L, Liu J, et al. Association between structural and functional brain alterations in drug-free patients with schizophrenia: a multimodal meta-analysis. J Psychiatry Neurosci. (2018) 43:131–42. doi: 10.1503/jpn.160219
32. Rotarska-Jagiela A, van de Ven V, Oertel-Knöchel V, Uhlhaas PJ, Vogeley K, Linden DEJ. Resting-state functional network correlates of psychotic symptoms in schizophrenia. Schizophr Res. (2010) 117:21–30. doi: 10.1016/j.schres.2010.01.001
33. Guo W, Liu F, Zhang J, Zhang Z, Yu L, Liu J, et al. Abnormal default-mode network homogeneity in first-episode, drug-naive major depressive disorder. PLoS ONE. (2014) 9:e91102. doi: 10.1371/journal.pone.0091102
34. Guo W, Su Q, Yao D, Jiang J, Zhang J, Zhang Z, et al. Decreased regional activity of default-mode network in unaffected siblings of schizophrenia patients at rest. Eur Neuropsychopharmacol. (2014) 24:545–52. doi: 10.1016/j.euroneuro.2014.01.004
35. Harrison PJ. The hippocampus in schizophrenia: a review of the neuropathological evidence and its pathophysiological implications. Psychopharmacology (Berl). (2004) 174:151–62. doi: 10.1007/s00213-003-1761-y
36. Boyer P, Phillips JL, Rousseau FL, Ilivitsky S. Hippocampal abnormalities and memory deficits: new evidence of a strong pathophysiological link in schizophrenia. Brain Res Rev. (2007) 54:92–112. doi: 10.1016/j.brainresrev.2006.12.008
37. Zhu F, Liu Y, Liu F, Yang R, Li H, Chen J, et al. Functional asymmetry of thalamocortical networks in subjects at ultra-high risk for psychosis and first-episode schizophrenia. Eur Neuropsychopharmacol. (2019) 29:519–28. doi: 10.1016/j.euroneuro.2019.02.006
38. Wojtalik JA, Smith MJ, Keshavan MS, Eack SM. A Systematic and meta-analytic review of neural correlates of functional outcome in schizophrenia. Schizophr Bull. (2017) 43:1329–47. doi: 10.1093/schbul/sbx008
39. Benetti S, Mechelli A, Picchioni M, Broome M, Williams S, McGuire P. Functional integration between the posterior hippocampus and prefrontal cortex is impaired in both first episode schizophrenia and the at risk mental state. Brain. (2009) 132(Pt 9):2426–36. doi: 10.1093/brain/awp098
40. White TP, Joseph V, Francis ST, Liddle PF. Aberrant salience network (bilateral insula and anterior cingulate cortex) connectivity during information processing in schizophrenia. Schizophr Res. (2010) 123:105–15. doi: 10.1016/j.schres.2010.07.020
41. Gasquoine PG. Contributions of the insula to cognition and emotion. Neuropsychol Rev. (2014) 24:77–87. doi: 10.1007/s11065-014-9246-9
42. Kochunov P, Coyle TR, Rowland LM, Jahanshad N, Thompson PM, Kelly S, et al. Association of white matter with core cognitive deficits in patients with schizophrenia. JAMA Psychiatry. (2017) 74:958–66. doi: 10.1001/jamapsychiatry.2017.2228
43. Kochunov P, Ganjgahi H, Winkler A, Kelly S, Shukla DK, Du X, et al. Heterochronicity of white matter development and aging explains regional patient control differences in schizophrenia. Hum Brain Mapp. (2016) 37:4673–88. doi: 10.1002/hbm.23336
44. Stotesbury H, Kirkham FJ, Kölbel M, Balfour P, Clayden JD, Sahota S, et al. White matter integrity and processing speed in sickle cell anemia. Neurology. (2018) 90:e2042–e50. doi: 10.1212/WNL.0000000000005644
45. Adhikari BM, Hong LE, Sampath H, Chiappelli J, Jahanshad N, Thompson PM, et al. Functional network connectivity impairments and core cognitive deficits in schizophrenia. Hum Brain Mapp. (2019) 40:4593–605. doi: 10.1002/hbm.24723
46. Peeters SCT, van Bronswijk S, van de Ven V, Gronenschild EHBM, Goebel R, van Os J, et al. Cognitive correlates of frontoparietal network connectivity “at rest” in individuals with differential risk for psychotic disorder. Eur Neuropsychopharmacol. (2015) 25:1922–32. doi: 10.1016/j.euroneuro.2015.08.007
47. Zhou C, Tang X, You W, Wang X, Zhang X, Zhang X, et al. Altered patterns of the fractional amplitude of low-frequency fluctuation and functional connectivity between deficit and non-deficit schizophrenia. Front Psychiatry. (2019) 10:680. doi: 10.3389/fpsyt.2019.00680
48. Meyer-Lindenberg A, Miletich RS, Kohn PD, Esposito G, Carson RE, Quarantelli M, et al. Reduced prefrontal activity predicts exaggerated striatal dopaminergic function in schizophrenia. Nat Neurosci. (2002) 5:267–71. doi: 10.1038/nn804
49. Seeley WW, Menon V, Schatzberg AF, Keller J, Glover GH, Kenna H, et al. Dissociable intrinsic connectivity networks for salience processing and executive control. J Neurosci. (2007) 27:2349–56. doi: 10.1523/JNEUROSCI.5587-06.2007
50. Tu PC, Lee YC, Chen YS Li CT, Su TP. Schizophrenia and the brain's control network: aberrant within- and between-network connectivity of the frontoparietal network in schizophrenia. Schizophr Res. (2013) 147:339–47. doi: 10.1016/j.schres.2013.04.011
51. Unschuld PG, Buchholz AS, Varvaris M, Van Zijl PCM, Ross CA, Pekar JJ, et al. Prefrontal brain network connectivity indicates degree of both schizophrenia risk and cognitive dysfunction. Schizophr Bull. (2014) 40:653–64. doi: 10.1093/schbul/sbt077
Keywords: youth-onset schizophrenia, ROI, functional connectivity, superior frontal gyrus, cognitive, MCCB
Citation: Qiu X, Lu S, Zhou M, Yan W, Du J, Zhang A, Xie S and Zhang R (2021) The Relationship Between Abnormal Resting-State Functional Connectivity of the Left Superior Frontal Gyrus and Cognitive Impairments in Youth-Onset Drug-Naïve Schizophrenia. Front. Psychiatry 12:679642. doi: 10.3389/fpsyt.2021.679642
Received: 12 March 2021; Accepted: 02 September 2021;
Published: 13 October 2021.
Edited by:
Jong-Hwan Lee, Korea University, South KoreaReviewed by:
Jiajia Zhu, First Affiliated Hospital of Anhui Medical University, ChinaJijun Wang, Shanghai Jiao Tong University, China
Copyright © 2021 Qiu, Lu, Zhou, Yan, Du, Zhang, Xie and Zhang. This is an open-access article distributed under the terms of the Creative Commons Attribution License (CC BY). The use, distribution or reproduction in other forums is permitted, provided the original author(s) and the copyright owner(s) are credited and that the original publication in this journal is cited, in accordance with accepted academic practice. No use, distribution or reproduction is permitted which does not comply with these terms.
*Correspondence: Shiping Xie, eGllc2hpcGluZzYzNjUmI3gwMDA0MDsxMjYuY29t; Rongrong Zhang, emhhbmdyb25nODc5OSYjeDAwMDQwOzE2My5jb20=
†These authors have contributed equally to this work and share first authorship