- The Affiliated Brain Hospital of Guangzhou Medical University, Guangzhou Huiai Hospital, Guangzhou, China
Background: Previous studies have examined the effects of long-term ketamine use on gray matter volume. But it is unclear whether chronic ketamine use alters cortical thickness and whether cortical thickness changes in chronic ketamine users are associated with cognitive deficits observed in chronic ketamine users.
Methods: Here, 28 chronic ketamine users and 30 healthy controls (HCs) were recruited. Cortical morphometry based on Computational Anatomy Toolbox (CAT12) was used to measure cortical thickness. Cognitive performance was measured by MATRICS Consensus Cognitive Battery (MCCB). Two-sample t-test was used to assess differences in cortical thickness and cognitive performance between the two groups. Partial correlation analysis was used for assessing correlations between cortical thickness changes and clinical characteristics, cognitive performance in chronic ketamine users.
Results: Chronic ketamine users exhibited significantly reduced cortical thickness in frontal, parietal, temporal, and occipital lobes compared to HC [false discovery rate (FDR) corrected at p < 0.05]. In chronic ketamine users, the average quantity (g) of ketamine use/day was negatively correlated with cortical thickness in the left superior frontal gyrus (SFG), right caudal middle frontal gyrus (MFG), and right paracentral lobule. The frequency of ketamine use (days per week) was negatively correlated with cortical thickness in the left isthmus cingulate cortex. Duration of ketamine use (month) was negatively correlated with cortical thickness in the left precentral gyrus. The chronic ketamine users showed significantly poorer cognitive performance on the working memory (P = 0.009), visual learning (P = 0.009), speed of processing (P < 0.000), and Matrics composite (P = 0.01). There was no correlation between scores of domains of MCCB and reduced cortical thickness.
Conclusion: The present study observed reduced cortical thickness in multiple brain areas, especially in the prefrontal cortex (PFC) in chronic ketamine users. Dose, frequency, and duration of ketamine use was negatively correlated with cortical thickness of some brain areas. Our results suggest that chronic ketamine use may lead to a decrease of cortical thickness. But the present study did not observe any correlation between reduced cortical thickness and decreased cognitive performance in chronic ketamine users.
Introduction
Ketamine, a derivative of phencyclidine, is a non-competitive antagonist of N-methyl-D-aspartate glutamate receptor (1). Over the past two decades, ketamine has become one of the most commonly abused drugs in mainland China (2–4). Concerns about the consequences of chronic ketamine use are increasing. Ketamine administration in rodents led to a dose- and time-dependent increase in neuronal cell death in the frontal cortex (5), hippocampus, and thalamus (5). It suggested that chronic ketamine use probably results in abnormal brain structure changes. A voxel-based morphometric (VBM) study found that the gray matter volume of the prefrontal cortex (PFC) decreased in chronic ketamine users, and these abnormal gray matter alterations were negatively correlated with chronic ketamine use (6). In addition, the study observed overall deficits on executive function in chronic ketamine users (6), but the author did not conduct a correlation analysis between gray matter volume changes and executive function. Another study found extensive atrophy in multiple brain regions in patients with 2–4 years of chronic ketamine use, especially in the frontal, parietal, and occipital cortices (7). These findings reflect the deleterious effects of chronic ketamine use on brain regions, such as the PFC (6, 7), which is a key brain region for cognitive functions (e.g., working memory) (8–10). Ketamine use impaired a range of cognitive function, including working memory (11, 12), episodic and semantic memory (11), executive functions (13), and mental and motor speed (13). A previous study found that recognition memory was especially impaired in frequent ketamine users (14). A longitudinal study showed that the impairments caused by ketamine use in spatial working memory and visual recognition were correlated with the level of ketamine use (15). A 3-year longitudinal investigation in ketamine users discovered long-lasting impairments on episodic memory and attentional functioning (12). A previous study found that ketamine use was negatively correlated with performance on spatial working memory and pattern recognition memory (16). But the abnormal brain structure changes that underlie the cognitive deficits in ketamine users have not been elucidated.
Neuroimaging provides an effective methodology to study the abnormal brain structure changes and helps to reveal the underlying neurosubstrate leading to cognitive deficits in ketamine users. Previous VBM study in chronic ketamine users evaluated gray matter volume changes (6), but this evaluation has its limitations. Gray matter volume is more strongly correlated with surface area than cortical thickness. Additionally, VBM cannot separately measure the cortical changes (17), such as cortical thickness. Previous neuroimaging study suggested that surface-based morphometry (SBM) was more sensitive than VBM in detecting gray matter changes (18). SBM independently measures the thickness of the cortex and provides more precise information than VBM on the neuroanatomy changes of brain disorders (17). Cortical thickness reflects the arrangement, size, and density of neurons, neuroglia, and nerve fibers (19). It is sensitive to pathological changes (20) and cognitive function (e.g., executive function) (21, 22). A longitudinal study of cocaine users observed reduced cortical thickness in the lateral frontal regions and the alterations in cortical thickness were related to cognitive performance (attention) changes and cocaine use (23). Nevertheless, to the best of our knowledge, no study has explored cortical thickness abnormalities and its relationship with cognitive function changes in chronic ketamine users. And it is unclear whether the cortical thickness changes in chronic ketamine users are associated with decreased cognitive performance.
In the present study, we use SBM to measure cortical thickness and MATRICS Consensus Cognitive Battery (MCCB) to assess cognitive functions in chronic ketamine users. The purposes of the present study were (1) to perform a comprehensive analysis of the cortical thickness in chronic ketamine users and matched healthy controls (HCs); (2) to investigate the relationship between cortical thickness changes and cognitive performance; (3) to investigate the relationship between cortical thickness changes and clinical and ketamine use characteristics in chronic ketamine users.
Materials and Methods
Participants
Chronic ketamine users were enrolled from Guangzhou Huayou Healthcare, Guangdong Provincial Bureau of Drug Rehabilitation, and Guangzhou Baiyun Voluntary Drug Rehabilitation Hospital. HCs were recruited by advertisements. The ethics committee of the Affiliated Brain Hospital of Guangzhou Medical University approved this study. All subjects were given written informed consent before participating in the study. All participants received a semistructured interview to evaluate substance use, sociodemographic characteristics, and psychopathological status.
Here, 28 chronic ketamine users were recruited, whose urine test was positive for ketamine. The inclusion criteria for chronic ketamine users included: (1) patients diagnosed as having ketamine dependence with Diagnostic and Statistical Manual of Mental Disorders, Fourth Edition, Text Revision (DSM-IV-TR); (2) no history of substance dependence (except for ketamine or tobacco); (3) no other substance use (except for tobacco or alcohol) for at least 12 months; and (4) age between 18 and 45 years. Exclusion criteria were: (1) a diagnosis of any substance use disorder (except for ketamine or tobacco) in the past; (2) current or previous psychiatric disorders, antipsychotic treatment, neurological diseases (e.g., serious head injury); (3) intellectual disability; (4) pregnancy; (5) serious medical conditions, such as heart failure; (6) family history of psychiatric disorders (first- or second-degree relatives); (7) major infectious disease (e.g., HIV); (8) positive urine test result for use of other substances, such as methamphetamine, marijuana, cocaine, opioids, and ecstasy.
Thirty HCs were recruited. The inclusion and exclusion criteria were the same as chronic ketamine users (except a history of drug use). The HCs and chronic ketamine users were carefully matched on age, sex, years of education, and race.
Before image scanning, a clinical assessment was performed by psychiatrists. Clinical symptoms were evaluated with the Positive and Negative Syndrome Scale (PANSS) (24, 25) administered by one trained psychiatrist with more than 5 years' clinical experience.
The cognitive function was evaluated by the MCCB (Chinese version) (26, 27), which was translated and revised by Shi and showed good reliability and validity (27). The MCCB consists of 10 tests that measure seven cognitive domains, including verbal learning, visual learning, social cognition, reasoning and problem solving, attention/vigilance, speed of processing, and working memory. The scores of these seven domains are combined to get a composite score (28). Previous studies using MCCB to assess cognitive impairments in schizophrenia (29) and mood disorders (30) have shown that MCCB could provide useful clinical information on the extent of cognitive impairment.
MRI Data Acquisition
Structural data were acquired using a 3.0-Tesla Philips scanner (Achieva X-series, Philips Medical Systems, the Netherlands) at the Affiliated Brain Hospital of Guangzhou Medical University. A sagittal three-dimensional gradient-echo T1-weighted sequence was performed. The acquisition parameters were repetition time (TR) = 8.2 ms, echo time (TE) = 3.7 ms, flip angle (FA) = 7°, inversion time (TI) = 1,100 ms, acquisition matrix = 256 × 256, field of view (FOV) = 256 mm2 × 256 mm2, axial slices = 188, slice thickness = 1 mm.
Data Analysis
Surface-based morphometry was used for the cortical analysis, performed with Computational Anatomy Toolbox (CAT12, http://dbm.neuro.uni-jena.de/cat/). It is an extension with the default pipeline of SPM12 (http://www.fil.ion.ucl.ac.uk/spm/software/spm12/). Cat12 provides a method for automatic measurement of cortical thickness and central surface of hemispheres based on the projection-based thickness (PBT) method (31). Previous study provided evidence that CAT12 makes accurate and robust cortical thickness estimates and can be considered a reliable and fast alternative to FreeSurfer (32). The fully automatic method of CAT12 can access the thickness of the cortex and reconstruct the central surface (31).
All MRI images carry out automatic segmentation of gray matter, white matter, and cerebrospinal fluid, affine registration of MNI template space, and non-linear deformation. A 15-mm FWHM Gaussian kernel was used to smooth resampled surface data of cortical thickness. By using the common second-level models and algorithms provided by the CAT12 software package, we conducted a two-sample t-test between the two groups. The cortical thickness of the each hemisphere was compared between groups. Subsequently, we performed region of interest (ROI)-based analysis. The Desikan–Killiany atlas was used to mark the anatomical regions emerging from the between-group comparisons and extracted the ROI-based surface value of the cortical thickness (33). False discovery rate [(FDR)-corrected threshold of P < 0.05)] was used to correct for multiple testing.
Statistical Analyses
Other data statistical analyses were conducted with Statistical Package for Social Sciences version 24 (SPSS, Chicago, USA). We used the Kolmogorov–Smirnov test to investigate the normality of data distribution. Except for age, education, attention/vigilance (AV), and working memory (WM) domains in MCCB, other variables were distributed normally. According to normality of data distribution, the two-sample t-test and Mann–Whitney U test were used to compare the differences between the two groups. The α level was set at 0.05. Two-tailed levels of significance (P < 0.05) were used. Bonferroni corrections were then used for multiple comparisons in MCCB.
Besides, we were specifically interested in the relationship between clinical data and cortical thickness measures in chronic ketamine users. Pearson correlation (partial correlation) analysis (smoking as covariant) was used to evaluate the correlation between reduced cortical thickness and clinical data, including age of starting ketamine use (year), duration of ketamine use (month), frequency of ketamine use (days per week), average quantity (g) of ketamine use/day, estimated total ketamine consumption, smoking status, and MCCB domains. Benjamini–Hochberg corrections were then applied for reducing the FDR.
Results
Demographic Data and Clinical Characteristics of the Participants
Demographic and clinical characteristics of the participants are shown in Table 1. A total of 58 participants (chronic ketamine users/28, HC/30, Chinese Han ethnicity) were recruited in this study. All the participants are male, right-handed. Mean age in the ketamine group was 29.4 years (SD = 5.15), with 11.93 (SD = 2.81) years of education. The chronic ketamine users and HCs were matched for age, educational level, sex, and race. The chronic ketamine users consumed ketamine by snorting the powder. The age of onset of ketamine use was 20.64 ± 4.37 years, and the duration of ketamine use was 107.04 ± 44.24 months. The frequency of ketamine use was 6.018 ± 1.60 (days/week), the average quantity of ketamine use/day was 2.27 ± 1.99 g, and the estimated total ketamine consumption was 3939.6 ± 3873.5 g. There was no frequent alcohol user in either group. Four ketamine users reported occasionally co-use of one or two other drugs, including methamphetamine, ecstasy, gamma-hydroxybutyrate (all polydrug use less than seven occasions). There were no obvious positive and negative symptoms in the ketamine group. Smoking status was available for 14 of the 30 control participants; five were smokers.
Comparison of Cognitive Performance Between Chronic Ketamine Users and Healthy Controls
Compared with the HCs, significant differences between chronic ketamine users and HCs were observed in multiple MCCB domains (Table 2). The chronic ketamine users showed significantly decreased cognitive performance on the working memory (P = 0.009), visual learning (P = 0.009), speed of processing (P < 0.000), and Matrics composite (P = 0.01). While there were no significant differences observed on verbal learning, reasoning and problem-solving, social cognition, attention/vigilance domains between the two groups (P > 0.05).
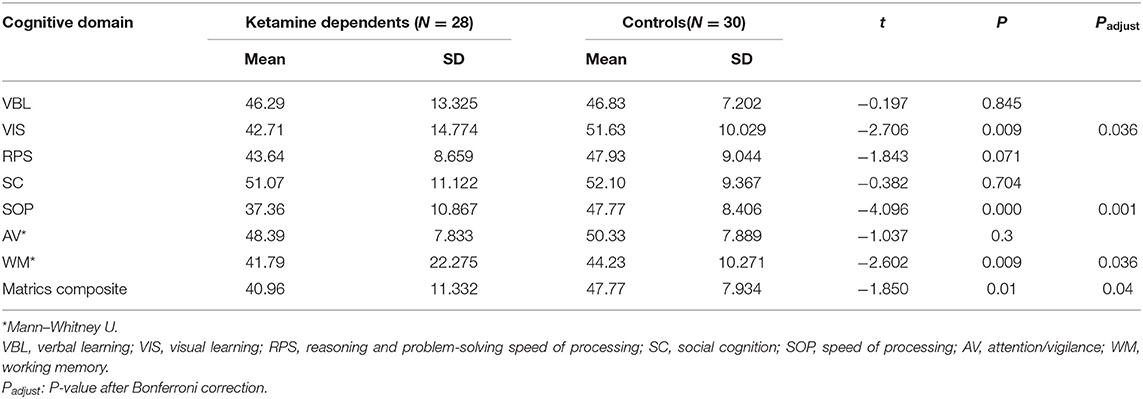
Table 2. Comparison of cognitive performance of MATRICS consensus cognitive battery (MCCB) between chronic ketamine users and healthy controls (HCs).
Group Differences in Regional Cortical Thickness
Compared with the HCs, chronic ketamine users exhibited reduced cortical thickness in the bilateral superior frontal gyrus, bilateral precentral gyrus, left isthmus cingulate cortex, right paracentral lobule, right superior parietal, right precuneus, right lateral orbitofrontal, right rostral middle frontal, pars orbitalis, middle temporal, caudal middle frontal, fusiform cortex, and lateral occipital (Figure 1, Table 3).
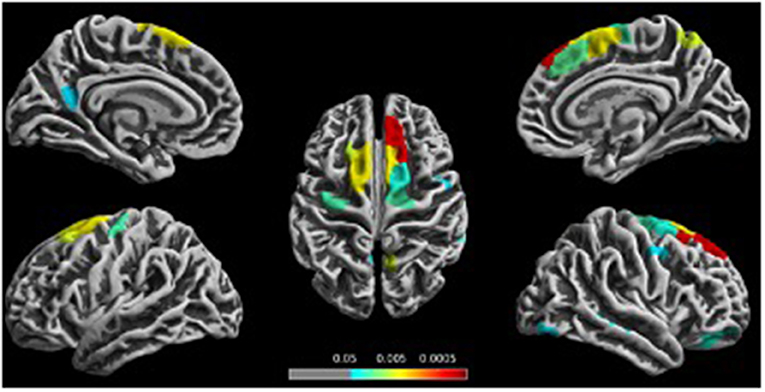
Figure 1. Reduced cortical thickness in the chronic ketamine users compared to the healthy controls (HCs). Figure shows localization of significantly reduced cortical thickness [P < 0.05, false discovery rate (FDR)-corrected] in the chronic ketamine users compared to the HC group.
Relationship Between Clinical Measures, Cognitive Performance, and Reduced Cortical Thickness
As shown in Table 4, the average quantity (g) of ketamine use/day was negatively correlated with cortical thickness in the left superior frontal gyrus (r = −0.404, P = 0.036), right caudal middle frontal gyrus (MFG) (r = −0.386, P = 0.047), and right paracentral lobule (r = −0.393, P = 0.043). The frequency of ketamine use (days per week) was negatively correlated with cortical thickness in the left isthmus cingulate cortex (r = −0.49, P = 0.01). Duration of ketamine use (month) was negatively correlated with cortical thickness in the left precentral gyrus (r = −0.437, P = 0.023). However, there was no correlation between any domain of MCCB and cortical thickness, which was significantly reduced in chronic ketamine users (P > 0.05). Additionally, we did not find any correlations between cortical thickness changes and age of starting ketamine use, estimated total ketamine consumption.
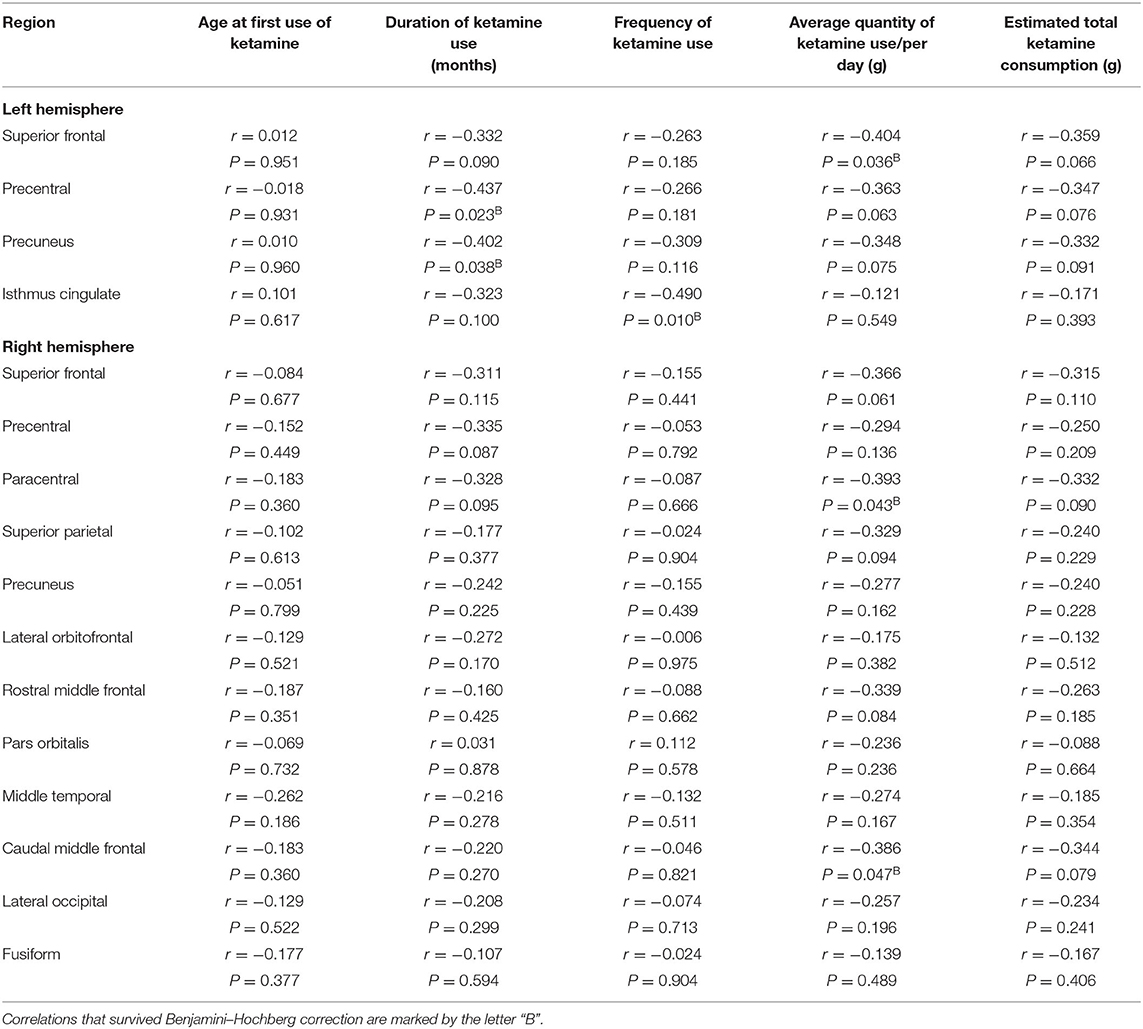
Table 4. Correlation between changes in cortical thickness and clinical associated characteristics in chronic ketamine users.
Discussion
In the present study, we found that cortical thickness was significantly reduced in the frontal, parietal, temporal, and occipital lobes in chronic ketamine users compared to HCs. We also observed negative correlations between the cortical thickness and dosage, duration, and frequency of ketamine use. But no correlation between cortical thickness and scores of domain of MCCB was found.
To the best of our knowledge, our study is the first to analyze the cortical thickness in chronic ketamine users. The present study found reduced cortical thickness in the left superior frontal gyrus, right caudal middle frontal gyrus (MFG). A previous neuroimaging study of chronic ketamine users observed a significant decrease in the gray matter volume of the left superior frontal gyrus and right middle frontal gyrus, and the reduced gray matter volume was correlated with the duration of ketamine use and estimated total ketamine consumption (6). In agreement with this study, we found reduced cortical thickness in similar brain regions in chronic ketamine users. Besides, the present study also found reduced cortical thickness in bilateral frontal cortices and parietal, temporal, and occipital lobes of chronic ketamine users. Our results indicated more abnormal gray matter changes in chronic ketamine users.
A previous study indicated that the neurotoxic effects of ketamine could cause neuronal apoptosis (34–36). Administration of ketamine 20 mg/kg resulted in a significant increase in neuronal death in rats (35). Ketamine infusion for a prolonged duration caused significant neuronal damage in the frontal cortex of rhesus monkeys (37, 38). The neurotoxic effects of ketamine depended on the frequency, dosage, and duration of ketamine administration (39). Consistent with a previous study, the present study found that the frequency, duration, and average quantity of ketamine used were correlated with abnormal cortical thickness changes, which was most prominent in the frontal lobes of chronic ketamine users (frontal cortices and paracentral lobule). These reduced cortical thicknesses are probably related to neuronal cell death due to neurotoxicity of ketamine. Therefore, it is crucial to interfere with the use of ketamine in the early stage to prevent the neurotoxic effect of ketamine on the brain.
The present study found reduced cortical thickness in the left superior frontal gyrus, MFG, and right paracentral lobule. These brain regions are related to cognitive control (40–43), which was highly involved in goal-directed behavior, decision-making, inhibition of impulses, and inhibition of conditioned behavior (41, 44, 45). The reduced cortical thickness of the PFC was correlated with the impaired cognitive control (46, 47). The deficits in cognitive control is a critical factor leading to continued drug use in individuals with addictive disorders (48–51). The present study observed multiple gray matter atrophy in the brain regions of cognitive control network. Therefore, as the neural structure supporting cognitive control deteriorated, the function of cognitive control was probably weakened, and the chronic ketamine users may have difficulty in controlling drug use. On the other hand, the reduced cortical thickness in bilateral PFC and paracentral lobule may lead to a higher dose of ketamine use due to decreased function of cognitive control. Meanwhile, the current study found reduced cortical thickness in the left isthmus cingulate cortex [part of the anterior cingulate (ACC)], and the cortical thickness in the left isthmus cingulate cortex (part of the ACC) was negatively correlated with the frequency of ketamine use in chronic ketamine users. The ACC was related to response inhibition (52). A previous study (53) suggested that the response inhibition is crucial to restrict drug cravings and drug-seeking behaviors. Also, response inhibition is a central construct for abstinence, and deficits in response inhibition characterize substance dependence. The cortical thickness in the ACC was associated with response control (47). The reduced cortical thickness in the left isthmus cingulate may reflect decreased response inhibition (54) and increased drug-seeking behaviors (55). Reduced cortical thickness in the left isthmus cingulate cortex may ultimately result in frequent use of ketamine in chronic ketamine users. On the other hand, due to the neurotoxicity of ketamine, the abnormal cortical changes found in the brain regions discussed above might be at least partially induced by chronic ketamine use.
We also found reduced cortical thickness in the left precentral gyrus, and a negative correlation between cortical thickness in the left precentral gyrus and duration of ketamine use was observed in chronic ketamine users. Previous studies of brain structure in methamphetamine (56, 57), marijuana (58), and alcohol users (59) have also reported reduced gray matter volume in the precentral gyrus. Duration of methamphetamine use was positively associated with a reduction in gray matter in the left precentral gyrus (56). Considering the neurotoxic effect of ketamine, we speculate that the reduced cortical thickness of the left precentral gyrus in chronic ketamine users may be a consequence of long-term drug consumption.
In summary, our study found abnormal cortical alterations in the prefrontal cortex (PFC), which was consistent with a previous study (6). But we found more abnormal cortical alterations in multiple brain regions, and the correlations between chronic ketamine use and abnormal gray matter changes are different from those of a previous study (6). These inconsistencies could be potentially attributed to the different methods of measuring gray matter changes. A previous study based on VBM used an ROI approach to analyze the gray matter volume changes in frontal cortices, while the current study based on SBM performed whole-brain vertex-level cortical thickness analysis. Evidence indicated that the SBM method is more sensitive than VBM in detecting gray matter changes, and cortical thickness analysis detects more changes in the gray matter layer compared with VBM (18). Besides, the assessment of gray matter volume by VBM reflects combined information that includes surface area, cortical folding, and cortical thickness (17, 60), therefore less specific. While the SBM method used in the current study reflected specific information of cortical thickness. The meaning of cortical thickness changes is not completely consistent with that of gray matter volume, which may lead to different correlational findings between abnormal gray matter changes and chronic ketamine use.
The present study also found reduced cortical thickness in orbitofrontal, precuneus, lateral occipital, parietal, and fusiform. These abnormal gray matter changes might relate to chronic ketamine use, but we did not observe any correlation between chronic ketamine use and cortical thickness in these brain regions. The consequences of these cortical changes and their relationship with ketamine use need to be further explored in future studies.
Consistent with previous researches (11, 13, 16, 61–63), the current study found deficits on cognitive domains of MCCB in chronic ketamine users, including working memory, visual learning, speed of processing, Matrics composite. Working memory, visual learning, and speed of processing were associated with the PFC (61, 64, 65). But the present study did not find correlations between cortical thickness changes and decreased cognitive performance. The domains of cognition, such as working memory (64) and speed of processing (66), are usually associated with a range of brain regions. It was possible that the functional impairments of specific brain regions could be compensated by itself or other brain regions (67). These neural compensatory mechanisms might postpone the onset of cognitive impairments. Besides, there may be individual differences in the mechanism of neural compensation. In view of this, the abnormal structure changes might not be plainly correlated with decreased cognitive performance. Also, our sample size is relatively small; there may not be adequate statistical power to detect the correlation between cortical thickness changes and cognitive performance in the current study. Further studies with larger sample sizes are needed to clarify the relationship between abnormal cortical thickness changes and cognitive performance in chronic ketamine users.
Also, ketamine is widely used as a pharmacological model of schizophrenia to mimic the psychotic symptoms and cognitive deficits (68). The psychotic symptom dimensions in chronic ketamine users were similar to those observed in schizophrenia patients (69). The schizophrenia patients showed broad reduced gray matter volume and cortical thickness in multiple brain regions (70–72), such as the superior frontal gyrus, middle temporal gyrus, and isthmus cingulate. Although acute ketamine administration cannot induce the abnormal gray matter changes similar to those observed in schizophrenia patients (73), chronic ketamine use could induce reduced gray matter volume or cortical thickness in bilateral frontal, parietal, temporal, and occipital lobes, which is very similar to those found in schizophrenia (70–72). Furthermore, a previous study in chronic ketamine users found decreased white matter fractional anisotropy in the medial frontal, which are remarkably in accordance with those shown in schizophrenia (74). In terms of chronic illness course, pattern of psychotic symptoms, cognitive deficits, and abnormalities in brain structure, chronic ketamine use may provide a better model of schizophrenia.
Limitation
Findings from the current study should be interpreted with several limitations in mind. First, the sample size was modest; nevertheless, our findings were primarily consistent with a previous study using different methods and analytic approaches (6), supporting the validity of our results. Second, as a cross-sectional study, the causal relationship between abnormal cortical alterations and chronic ketamine use could not be concluded. Preexisting abnormal gray matter changes may also predispose individuals to engage in substance use (75, 76). We are prone to consider that the abnormal cortical thickness changes in chronic ketamine users were at least partially induced by ketamine use. Longitudinal investigations will be important for a clear and definite understanding. Third, our study did not match tobacco use between the two groups, but we did not find any correlation between reduced cortical thickness and estimated total smoked cigarettes (P > 0.12). In line with the current study, previous neuroimaging study of chronic ketamine users did not find any correlation between reduced gray matter volume and estimated total smoked cigarettes (6). Although our analyses suggested that the smaller cortical thickness we observed is unlikely to reflect group differences in smoking, we cannot exclude the potential effect of smoking on cortical thickness, which should be taken into account in interpreting our results. Future studies should match groups on smoking status and history. Fourth, although we try to include only subjects who are rarely used in combination with other substances, exposure to other substances is almost inevitable for most drug addicts. In the present study, only four patients reported a history of co-use substance (all polydrug use of <7 lifetime occasions), and we can almost dismiss the influence of other substances. Finally, female ketamine users were not included in this study, so we cannot determine whether the present findings generalize to female ketamine users.
Conclusion
The present study showed reduced cortical thickness in the frontal, parietal, temporal, and occipital lobes in chronic ketamine users, especially in frontal cortices. Our results indicated that the dose, frequency, and duration of ketamine use were correlated to abnormal cortical thickness changes in chronic ketamine users. But the present study did not observe any correlation between reduced cortical thickness and decreased cognitive performance in chronic ketamine users. Longitudinal studies will be very important in the future. It can help us find out whether these abnormal changes in the gray matter of chronic ketamine users can be recovered or be used to predict prognosis.
Data Availability Statement
The raw data supporting the conclusions of this article will be made available by the authors, without undue reservation.
Ethics Statement
The studies involving human participants were reviewed and approved by The ethics committee of the Affiliated Brain Hospital of Guangzhou Medical University. The patients/participants provided their written informed consent to participate in this study.
Author Contributions
NF and HH designed the study and wrote the protocol. JZ and ZZ recruited the subjects and undertook the statistical analysis. NF and JZ wrote the manuscript. HW, PC, and JH contributed to data input. JZ and FW helped enrolling volunteers. All authors contributed to and have approved the final manuscript.
Funding
This work was supported by a grant to NF from the Guangdong Natural Science Funds (Grant No. 2020A1515011173), a grant to HH from Guangzhou Municipal Science And Technology Project (Grant No. 201804010056), and a grant to FW from Scientific Research Project of Traditional Chinese Medicine of Guangdong (Grant No. 20192070). The funding source had no role in the study design, analysis, or interpretation of data or in the preparation of the report or decision to publish.
Conflict of Interest
The authors declare that the research was conducted in the absence of any commercial or financial relationships that could be construed as a potential conflict of interest.
Acknowledgments
We would like to thank all the staff of Guangzhou Baiyun Voluntary Drug Rehabilitation Hospital, Guangzhou Huayou Healthcare, and Guangdong Provincial Bureau of Drug Rehabilitation for their invaluable assistance.
References
1. Jansen KL. A review of the nonmedical use of ketamine: use, users and consequences. J. Psychedelic Drugs. (2000) 32:419–33. doi: 10.1080/02791072.2000.10400244
2. Zhuo X, Cang Y, Yan H, Bu J, Shen B. The prevalence of drugs in motor vehicle accidents and traffic violations in Shanghai and neighboring cities. Accid Anal. Prev. (2010) 42:2179–84. doi: 10.1016/j.aap.2010.07.004
3. Ng SH, Tse ML, Ng HW, Lau FL. Emergency department presentation of ketamine abusers in Hong Kong: a review of 233 cases. Hong Kong Med. J. (2010) 16:6–11.
4. Hung C, Zhang S, Chen C, Duann J, Lin C, Lee TS, et al. Striatal functional connectivity in chronic ketamine users: a pilot study. Am. J. Drug Alcohol Abuse. (2019) 46:31–43. doi: 10.1080/00952990.2019.1624764
5. Zou X, Patterson TA, Sadovova N, Twaddle NC, Doerge DR, Zhang X, et al. Potential neurotoxicity of ketamine in the developing rat brain. Toxicol. Sci. (2009) 108:149–58. doi: 10.1093/toxsci/kfn270
6. Yanhui L, Jinsong T, Corlett PR, Xuyi W, Mei Y, Hongxian C, et al. Reduced dorsal prefrontal gray matter after chronic ketamine use. Biol. Psychiatry. (2011) 69:42–8. doi: 10.1016/j.biopsych.2010.08.030
7. Wang C, Zheng D, Xu J, Lam W, Yew DT. Brain damages in ketamine addicts as revealed by magnetic resonance imaging. Front. Neuroanat. (2013) 7:23. doi: 10.3389/fnana.2013.00023
8. Badre D, Wagner AD. Semantic retrieval, mnemonic control, and prefrontal cortex. Behav. Cogn. Neurosci. Rev. (2002) 1:206–18. doi: 10.1177/1534582302001003002
9. Erberk Ozen N, Rezaki M. Prefrontal cortex: implications for memory functions and dementia. Turk. Psikiyatri. Derg. (2007) 18:262–9. doi: 10.1055/s-2007-985391
10. Miller EK. The prefrontal cortex and cognitive control. Nat. Rev. Neurosci. (2000) 1:59–65. doi: 10.1038/35036228
11. Curran HV, Morgan. Cognitive, dissociative and psychotogenic effects of ketamine in recreational users on the night of drug use and 3 days later. Addiction. (2000) 95:575–90. doi: 10.1046/j.1360-0443.2000.9545759.x
12. Morgan CJ, Monaghan L, Curran HV. Beyond the K-hole: a 3-year longitudinal investigation of the cognitive and subjective effects of ketamine in recreational users who have substantially reduced their use of the drug. Addiction. (2004) 99:1450–61. doi: 10.1111/j.1360-0443.2004.00879.x
13. Tang WK, Liang HJ, Lau CG, Tang A, Ungvari GS. Relationship between cognitive impairment and depressive symptoms in current ketamine users. J. Stud. Alcohol. Drugs. (2013) 74:460–8. doi: 10.15288/jsad.2013.74.460
14. Morgan CJ, Muetzelfeldt L, Curran HV. Ketamine use, cognition and psychological wellbeing: a comparison of frequent, infrequent and ex-users with polydrug and non-using controls. Addiction. (2009) 104:77–87. doi: 10.1111/j.1360-0443.2008.02394.x
15. Morgan CJ, Curran HV. Ketamine use: a review. Addiction. (2011) 107:27–38. doi: 10.1111/j.1360-0443.2011.03576.x
16. Morgan CJ, Muetzelfeldt L, Curran HV. Consequences of chronic ketamine self-administration upon neurocognitive function and psychological wellbeing: a 1-year longitudinal study. Addiction. (2010) 105:121–33. doi: 10.1111/j.1360-0443.2009.02761.x
17. Kim JH, Suh SI, Lee HJ, Lee JH, Lee MS. Cortical and subcortical gray matter alterations in first-episode drug-naïve adolescents with major depressive disorder. Neuroreport. (2019) 30:1172–8. doi: 10.1097/WNR.0000000000001336
18. Hutton C, Draganski B, Ashburner J, Weiskopf N. A comparison between voxel-based cortical thickness and voxel-based morphometry in normal aging. Neuroimage. (2009) 48:371–80. doi: 10.1016/j.neuroimage.2009.06.043
19. Li Q, Zhao Y, Chen Z, Long J, Dai J, Huang X, et al. Meta-analysis of cortical thickness abnormalities in medication-free patients with major depressive disorder. Neuropsychopharmacology. (2020) 45:703–12. doi: 10.1038/s41386-019-0563-9
20. Di Biase MA, Cropley VL, Cocchi L, Fornito A, Calamante F, Ganella EP, et al. Linking cortical and connectional pathology in schizophrenia. Schizophr. Bull. (2019) 45:911–23. doi: 10.1093/schbul/sby121
21. Zhang L, Wang M, Sterling NW, Lee EY, Eslinger PJ, Wagner D, et al. Cortical thinning and cognitive impairment in parkinson's disease without dementia. IEEE/ACM Trans. Comput. Biol. Bioinform. (2018) 15:570–80. doi: 10.1109/TCBB.2015.2465951
22. Knöchel C, Reuter J, Reinke B, Stäblein M, Marbach K, Feddern R, et al. Cortical thinning in bipolar disorder and schizophrenia. Schizophr. Res. (2016) 172:78–85. doi: 10.1016/j.schres.2016.02.007
23. Hirsiger S, Hänggi J, Germann J, Vonmoos M, Preller KH, Engeli EJE, et al. Longitudinal changes in cocaine intake and cognition are linked to cortical thickness adaptations in cocaine users. Neuroimage Clin. (2019) 21:101652. doi: 10.1016/j.nicl.2019.101652
24. Kay SR, Fiszbein A, Opler LA. The positive and negative syndrome scale (PANSS) for schizophrenia. Schizophr. Bull. (1987) 13:261–76. doi: 10.1093/schbul/13.2.261
25. He YL, Zhang MZ. The Chinese norm and factor analysis of PANSS. Chin. J. Clin. Psychol. (2000) 8:65–9. doi: 10.3969/j.issn.1005-3611.2000.02.001
26. Green MF, Nuechterlein KH, Gold JM, Barch DM, Cohen J, Essock S, et al. Approaching a consensus cognitive battery for clinical trials in schizophrenia: the NIMH-MATRICS conference to select cognitive domains and test criteria. Biol. Psychiatry. (2004) 56:301–7. doi: 10.1016/j.biopsych.2004.06.023
27. Shi C, Kang L, Yao S, Ma Y, Li T, Liang Y, et al. The MATRICS consensus cognitive battery (MCCB): co-norming and standardization in China. Schizophr. Res. (2015) 169:109–15. doi: 10.1016/j.schres.2015.09.003
28. Nuechterlein KH, Green MF, Kern RS, Baade LE, Barch DM, Cohen JD, et al. The MATRICS consensus cognitive battery, part 1: test selection, reliability, and validity. Am. J. Psychiatry. (2008) 165:203–13. doi: 10.1176/appi.ajp.2007.07010042
29. Rodríguez-Jiménez R, Bagney A, Moreno-Ortega M, García-Navarro C, Aparicio AI, López-Antón R, et al. Cognitive deficit in schizophrenia: MATRICS consensus cognitive battery. Rev. Neurol. (2012) 55:549–55. doi: 10.33588/rn.5509.2012246
30. Bo Q, Mao Z, Li X, Wang Z, Wang C, Ma X. Use of the MATRICS consensus cognitive battery (MCCB) to evaluate cognitive deficits in bipolar disorder: a systematic review and meta-analysis. PLoS ONE. (2017) 12:e0176212. doi: 10.1371/journal.pone.0176212
31. Dahnke R, Yotter RA, Gaser C. Cortical thickness and central surface estimation. NeuroImage. (2013) 65:336–48. doi: 10.1016/j.neuroimage.2012.09.050
32. Seiger R, Ganger S, Kranz GS, Hahn A, Lanzenberger R. Cortical thickness estimations of freesurfer and the CAT12 toolbox in patients with Alzheimer's disease and healthy controls. J. Neuroimaging. (2018) 28:515–23. doi: 10.1111/jon.12521
33. Desikan RS, Ségonne F, Fischl B, Quinn BT, Dickerson BC, Blacker D, et al. An automated labeling system for subdividing the human cerebral cortex on MRI scans into gyral based regions of interest. Neuroimage. (2006) 31:968–80. doi: 10.1016/j.neuroimage.2006.01.021
34. Lee ST, Wu TT, Yu PY, Chen RM. Apoptotic insults to human HepG2 cells induced by S- (+)-ketamine occurs through activation of a Bax-mitochondria-caspase protease pathway. Br. J. Anaesth. (2009) 102:80–9. doi: 10.1093/bja/aen322
35. Liu F, Paule MG, Ali S, Wang C. Ketamine-induced neurotoxicity and changes in gene expression in the developing rat brain. Curr. Neuropharmacol. (2011) 9:256–61. doi: 10.2174/157015911795017155
36. Bosnjak ZJ, Yan Y, Canfield S, Muravyeva MY, Kikuchi C, Wells CW, et al. Ketamine induces toxicity in human neurons differentiated from embryonic stem cells via mitochondrial apoptosis pathway. Curr. Drug Saf. (2012) 7:106–19. doi: 10.2174/157488612802715663
37. Zou X, Patterson TA, Divine RL, Sadovova N, Zhang X, Hanig JP, et al. Prolonged exposure to ketamine increases neurodegeneration in the developing monkey brain. Int. J. Dev. Neurosci. (2009) 27:727–31. doi: 10.1016/j.ijdevneu.2009.06.010
38. Slikker W Jr, Zou X, Hotchkiss CE, Divine RL, Sadovova N, Twaddle NC, et al. Ketamine-induced neuronal cell death in the perinatal rhesus monkey. Toxicol. Sci. (2007) 98:145–58. doi: 10.1093/toxsci/kfm084
39. Cheung HM, Yew DTW. Effects of perinatal exposure to ketamine on the developing brain. Front. Neurosci. (2019) 13:138. doi: 10.3389/fnins.2019.00138
40. Ai H, Xin Y, Luo YJ, Gu R, Xu P. Volume of motor area predicts motor impulsivity. Eur. J. Neurosci. (2019) 49:1470–6. doi: 10.1111/ejn.14339
41. Li M, Hua K, Li S, Li C, Zhan W, Wen H, et al. Cortical morphology of chronic users of codeine-containing cough syrups: association with sulcal depth, gyrification, and cortical thickness. Eur. Radiol. (2019) 29:5901–9. doi: 10.1007/s00330-019-06165-0
42. Cisler JM, Elton A, Kennedy AP, Young J, Smitherman S, Andrew James G, et al. Altered functional connectivity of the insular cortex across prefrontal networks in cocaine addiction. Psychiatry Res. (2013) 213:39–46. doi: 10.1016/j.pscychresns.2013.02.007
43. Breukelaar IA, Antees C, Grieve SM, Foster SL, Gomes L, Williams LM, et al. Cognitive control network anatomy correlates with neurocognitive behavior: a longitudinal study. Hum. Brain Mapp. (2017) 38:631–43. doi: 10.1002/hbm.23401
44. Hanlon CA, Dowdle LT, Henderson JS. Modulating neural circuits with transcranial magnetic stimulation: implications for addiction treatment development. Pharmacol. Rev. (2018) 70:661–83. doi: 10.1124/pr.116.013649
45. Crews FT, Boettiger CA. Impulsivity, frontal lobes and risk for addiction. Pharmacol. Biochem. Behav. (2009) 93:237–47. doi: 10.1016/j.pbb.2009.04.018
46. Kim JS, Yang JJ, Lee DK, Lee JM, Youn J, Cho JW. Cognitive impairment and its structural correlates in the parkinsonian subtype of multiple system atrophy. Neurodegener. Dis. (2015) 15:294–300. doi: 10.1159/000430953
47. Sadeh N, Spielberg JM, Miller MW, Milberg WP, Salat DH, Amick MM, et al. Neurobiological indicators of disinhibition in posttraumatic stress disorder. Hum. Brain Mapp. (2015) 36:3076–86. doi: 10.1002/hbm.22829
48. de Wit H. Impulsivity as a determinant and consequence of drug use: a review of underlying processes. Addict. Biol. (2009) 14:22–31. doi: 10.1111/j.1369-1600.2008.00129.x
49. Garavan H, Hester R. The role of cognitive control in cocaine dependence. Neuropsychol. Rev. (2007) 17:337–45. doi: 10.1007/s11065-007-9034-x
50. Li CS, Sinha R. Inhibitory control and emotional stress regulation: neuroimaging evidence for frontal-limbic dysfunction in psycho-stimulant addiction. Neurosci. Biobehav. Rev. (2008) 32:581–97. doi: 10.1016/j.neubiorev.2007.10.003
51. Porrino LJ, Smith HR, Nader MA, Beveridge TJ. The effects of cocaine: a shifting target over the course of addiction. Prog. Neuro Psychopharmacol. Biol. Psychiatry. (2007) 31:1593–600. doi: 10.1016/j.pnpbp.2007.08.040
52. Zhang Y, Gong J, Xie C, Ye EM, Jin X, Song H, et al. Alterations in brain connectivity in three sub-regions of the anterior cingulate cortex in heroin-dependent individuals: evidence from resting state fMRI. Neuroscience. (2015) 284:998–1010. doi: 10.1016/j.neuroscience.2014.11.007
53. Spechler PA, Chaarani B, Hudson KE, Potter A, Foxe JJ, Garavan H. Response inhibition and addiction medicine: from use to abstinence. Prog. Brain Res. (2016) 223:143–64. doi: 10.1016/bs.pbr.2015.07.024
54. Migliorini R, Moore EM, Glass L, Infante MA, Tapert SF, Jones KL, et al. Anterior cingulate cortex surface area relates to behavioral inhibition in adolescents with and without heavy prenatal alcohol exposure. Behav. Brain Res. (2015) 292:26–35. doi: 10.1016/j.bbr.2015.05.037
55. Papachristou H, Nederkoorn C, Havermans R, Bongers P, Beunen S, Jansen A. Higher levels of trait impulsiveness and a less effective response inhibition are linked to more intense cue-elicited craving for alcohol in alcohol-dependent patients. Psychopharmacology. (2013) 228:641–9. doi: 10.1007/s00213-013-3063-3
56. Hall MG, Alhassoon OM, Stern MJ, Wollman SC, Kimmel CL, Perez-Figueroa A, et al. Gray matter abnormalities in cocaine versus methamphetamine-dependent patients: a neuroimaging meta-analysis. Am. J. Drug Alcohol Abuse. (2015) 41:290–9. doi: 10.3109/00952990.2015.1044607
57. Ruan X, Zhong N, Yang Z, Fan X, Zhuang W, Du J, et al. Gray matter volume showed dynamic alterations in methamphetamine users at 6 and 12 months abstinence: a longitudinal voxel-based morphometry study. Prog. Neuro Psychopharmacol. Biol. Psychiatry. (2018) 81:350–5. doi: 10.1016/j.pnpbp.2017.09.004
58. Lisdahl KM, Tamm L, Epstein JN, Jernigan T, Molina BS, Hinshaw SP, et al. The impact of ADHD persistence, recent cannabis use, and age of regular cannabis use onset on subcortical volume and cortical thickness in young adults. Drug Alcohol Depend. (2016) 161:135–46. doi: 10.1016/j.drugalcdep.2016.01.032
59. Fortier CB, Leritz EC, Salat DH, Venne JR, Maksimovskiy AL, Williams V, et al. Reduced cortical thickness in abstinent alcoholics and association with alcoholic behavior. Alcohol. Clin. Exp. Res. (2011) 35:2193–201. doi: 10.1111/j.1530-0277.2011.01576.x
60. Park HJ, Lee JD, Kim EY, Park B, Oh MK, Lee S, et al. Morphological alterations in the congenital blind based on the analysis of cortical thickness and surface area. Neuroimage. (2009) 47:98–106. doi: 10.1016/j.neuroimage.2009.03.076
61. Chan KW, Lee TM, Siu AM, Wong DP, Kam CM, Tsang SK, et al. Effects of chronic ketamine use on frontal and medial temporal cognition. Addict. Behav. (2013) 38:2128–32. doi: 10.1016/j.addbeh.2013.01.014
62. Ke X, Ding Y, Xu K, He H, Wang D, Deng X, et al. The profile of cognitive impairments in chronic ketamine users. Psychiatry Res. (2018) 266:124–31. doi: 10.1016/j.psychres.2018.05.050
63. Cheng WJ, Chen CH, Chen CK, Huang MC, Pietrzak RH, Krystal JH, et al. Similar psychotic and cognitive profile between ketamine dependence with persistent psychosis and schizophrenia. Schizophr. Res. (2018) 199:S0920996418301300. doi: 10.1016/j.schres.2018.02.049
64. Christophel TB, Klink PC, Spitzer B, Roelfsema PR, Haynes JD. The distributed nature of working memory. Trends Cogn. Sci. (2017) 21:111–24. doi: 10.1016/j.tics.2016.12.007
65. Pierrot-Deseilligny C, Müri RM, Rivaud-Pechoux S, Gaymard B, Ploner CJ. Cortical control of spatial memory in humans: the visuooculomotor model. Ann. Neurol. (2002) 52:10–9. doi: 10.1002/ana.10273
66. Righart R, Duering M, Gonik M, Jouvent E, Reyes S, Hervé D, et al. Impact of regional cortical and subcortical changes on processing speed in cerebral small vessel disease. Neuroimage Clin. (2013) 2:854–61. doi: 10.1016/j.nicl.2013.06.006
67. Ansado J, Monchi O, Ennabil N, Deslauriers J, Jubault T, Faure S, et al. Coping with task demand in aging using neural compensation and neural reserve triggers primarily intra-hemispheric-based neurofunctional reorganization. Neurosci. Res. (2013) 75:295–304. doi: 10.1016/j.neures.2013.01.012
68. Krystal JH, Karper LP, Seibyl JP, Freeman GK, Delaney R, Bremner JD, et al. Subanesthetic effects of the noncompetitive NMDA antagonist, ketamine, in humans. Psychotomimetic, perceptual, cognitive, and neuroendocrine responses. Arch. Gen. Psychiatry. (1994) 51:199–214. doi: 10.1001/archpsyc.1994.03950030035004
69. Xu K, Krystal JH, Ning Y, Chen DC, He H, Wang D, et al. Preliminary analysis of positive and negative syndrome scale in ketamine-associated psychosis in comparison with schizophrenia. J. Psychiatr. Res. (2015) 61:64–72. doi: 10.1016/j.jpsychires.2014.12.012
70. Madre M, Canales-Rodríguez EJ, Fuentes-Claramonte P, Alonso-Lana S, Salgado-Pineda P, Guerrero-Pedraza A, et al. Structural abnormality in schizophrenia versus bipolar disorder: a whole brain cortical thickness, surface area, volume and gyrification analyses. Neuroimage Clin. (2020) 25:102131. doi: 10.1016/j.nicl.2019.102131
71. Shahab S, Mulsant BH, Levesque ML, Calarco N, Nazeri A, Wheeler AL, et al. Brain structure, cognition, and brain age in schizophrenia, bipolar disorder, and healthy controls. Neuropsychopharmacology. (2019) 44:898–906. doi: 10.1038/s41386-018-0298-z
72. Yasuda Y, Okada N, Nemoto K, Fukunaga M, Yamamori H, Ohi K, et al. Brain morphological and functional features in cognitive subgroups of schizophrenia. Psychiatry Clin. Neurosci. (2020) 74:191–203. doi: 10.1111/pcn.12963
73. Höflich A, Ganger S, Tik M, Hahn A, Kranz GS, Vanicek T, et al. Imaging the neuroplastic effects of ketamine with VBM and the necessity of placebo control. Neuroimage. (2017) 147:198–203. doi: 10.1016/j.neuroimage.2016.12.032
74. Yanhui L, Jinsong T, Mingdong M, Zhimei W, Mei Y, Xuyi W, et al. Frontal white matter abnormalities following chronic ketamine use: a diffusion tensor imaging study. Brain A J. Neurol. (2010) 133:2115–22. doi: 10.1093/brain/awq131
75. Cheetham A, Allen NB, Whittle S, Simmons JG, Yücel M, Lubman DI. Orbitofrontal volumes in early adolescence predict initiation of cannabis use: a 4-year longitudinal and prospective study. Biol. Psychiatry. (2012) 71:684–92. doi: 10.1016/j.biopsych.2011.10.029
Keywords: ketamine, ketamine dependent, frontal lobes, gray matter, cortical thickness, surface-based morphometry
Citation: Zhong J, Wu H, Wu F, He H, Zhang Z, Huang J, Cao P and Fan N (2021) Cortical Thickness Changes in Chronic Ketamine Users. Front. Psychiatry 12:645471. doi: 10.3389/fpsyt.2021.645471
Received: 23 December 2020; Accepted: 09 February 2021;
Published: 25 March 2021.
Edited by:
Wei Hao, Central South University, ChinaReviewed by:
Ying Wang, First Affiliated Hospital of Jinan University, ChinaYanbin Jia, First Affiliated Hospital of Jinan University, China
Copyright © 2021 Zhong, Wu, Wu, He, Zhang, Huang, Cao and Fan. This is an open-access article distributed under the terms of the Creative Commons Attribution License (CC BY). The use, distribution or reproduction in other forums is permitted, provided the original author(s) and the copyright owner(s) are credited and that the original publication in this journal is cited, in accordance with accepted academic practice. No use, distribution or reproduction is permitted which does not comply with these terms.
*Correspondence: Ni Fan, fanni2005@126.com
†These authors have contributed equally to this work