- The Clinical Hospital of Chengdu Brain Science Institute, MOE Key Laboratory for NeuroInformation, Center for Information in Medicine, University of Electronic Science and Technology of China, Chengdu, China
Autism spectrum disorder (ASD) is an early onset developmental disorder which persists throughout life and is increasing in prevalence over the last few decades. Given its early onset and variable cognitive and emotional functional impairments, it is generally challenging to assess ASD individuals using task-based behavioral and functional MRI paradigms. Consequently, resting state functional MRI (rs-fMRI) has become a key approach for examining ASD-associated neural alterations and revealed functional alterations in large-scale brain networks relative to typically developing (TD) individuals, particularly those involved in social-cognitive and affective processes. Recent progress suggests that alterations in inter-hemispheric resting state functional connectivity (rsFC) between regions in the 2 brain hemispheres, particularly homotopic ones, may be of great importance. Here we have reviewed neuroimaging studies examining inter-hemispheric rsFC abnormities in ASD and its associations with symptom severity. As an index of inter-hemispheric functional connectivity, we have additionally reviewed previous studies on corpus callosum (CC) volumetric and fiber changes in ASD. There are converging findings on reduced inter-hemispheric (including homotopic) rsFC in large-scale brain networks particularly in posterior hubs of the default mode network, reduced volumes in the anterior and posterior CC, and on decreased FA and increased MD or RD across CC subregions. Associations between the strength of inter-hemispheric rsFC and social impairments in ASD together with their classification performance in distinguishing ASD subjects from TD controls across ages suggest that the strength of inter-hemispheric rsFC may be a more promising biomarker for assisting in ASD diagnosis than abnormalities in either brain wide rsFC or brain structure.
Introduction
Autism spectrum disorder (ASD) is an early onset developmental disorder which persists throughout life and has an increasing prevalence rate over the last decades, with a general average of around 1% of the population being affected (1). Although symptoms of ASD are highly heterogeneous, the disorder is generally characterized by deficits mainly in social communication/interaction, restricted interests, and repetitive behaviors (2). Task-based behavioral and neuroimaging studies have further shown specific behavioral deficits and corresponding neural dysfunctions in ASD primarily in social-cognitive and affective domains, such as facial emotion recognition (3–5), eye contact avoidance (6–8), and social interest (9, 10).
However, given the early onset of the disorder and general cognitive deficits in ASD, assessment of ASD individuals, particularly young children, by means of task-based behavioral and fMRI paradigms is challenging. Consequently, resting state fMRI (rs-fMRI) has been proposed as a promising approach for examining ASD-associated alterations. Rs-fMRI assesses subjects in the absence of a specific task and thus allows comparisons across different studies and data acquisition sites. Previous rs-fMRI studies have reported functional abnormalities in large-scale brain networks in ASD relative to typically developing (TD) individuals (11–14), particularly in brain networks involved in social-cognitive and affective processes (15–17). Importantly, rs-fMRI measurement across different sites and research groups allows the collection of large sample sizes and data sharing initiatives, such as the Autism Brain Imaging Data Exchange (ABIDE) databases (ABIDE-I and -II), although it should be acknowledged that these span wide age ranges and are restricted to individuals with high-functioning ASD. As a result of increased statistical power using these large databases, previous studies have demonstrated more consistent and reproducible patterns of alterations in resting state functional connectivity (rsFC) in ASD populations (11, 18, 19).
It has been shown that functional connectivity alterations in psychiatric disorders are mainly contributed by counterpart regions in the opposite hemisphere (20). This is also the case in ASD where rsFC alterations are primarily contributed by inter-hemispheric connectivity between regions in the 2 hemispheres (11, 21), and homotopic ones (between identical regions in the 2 hemispheres) may be of particular importance (19, 22, 23). The aims of the present review are to detail findings from neuroimaging studies directly examining interhemispheric rsFC abnormalities in ASD (see Table 1), particularly the homotopic ones together with their associations with symptom severity, and studies investigating structural changes in the corpus callosum (CC) in ASD (see Table 2 for volumetric studies and Table 3 for diffusion-weighted imaging (DWI) studies), which is also an important index of interhemispheric functional connectivity. We also discussed their functional significance and whether they can be used as promising biomarkers for future clinical application.
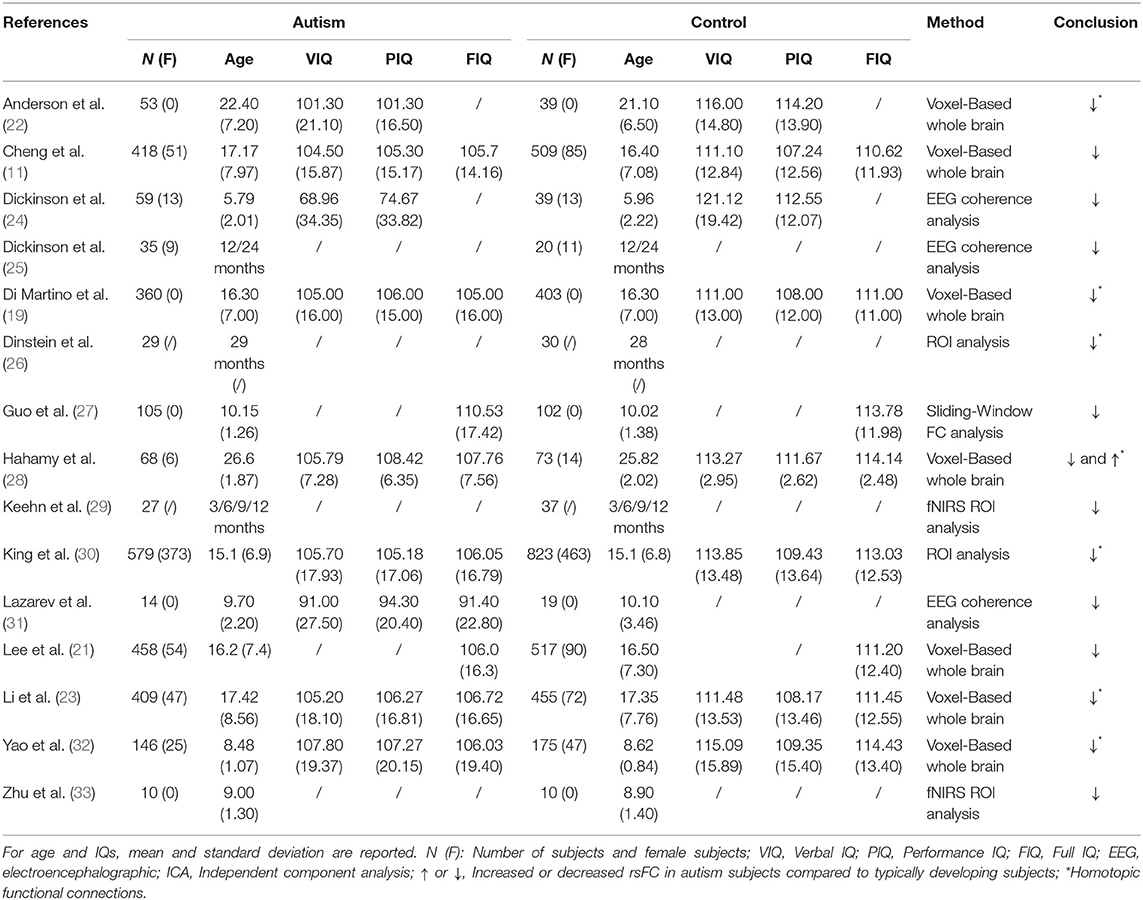
Table 1. Overview of the studies examining alterations in interhemispheric (homotopic) functional connectivity in ASD patients.
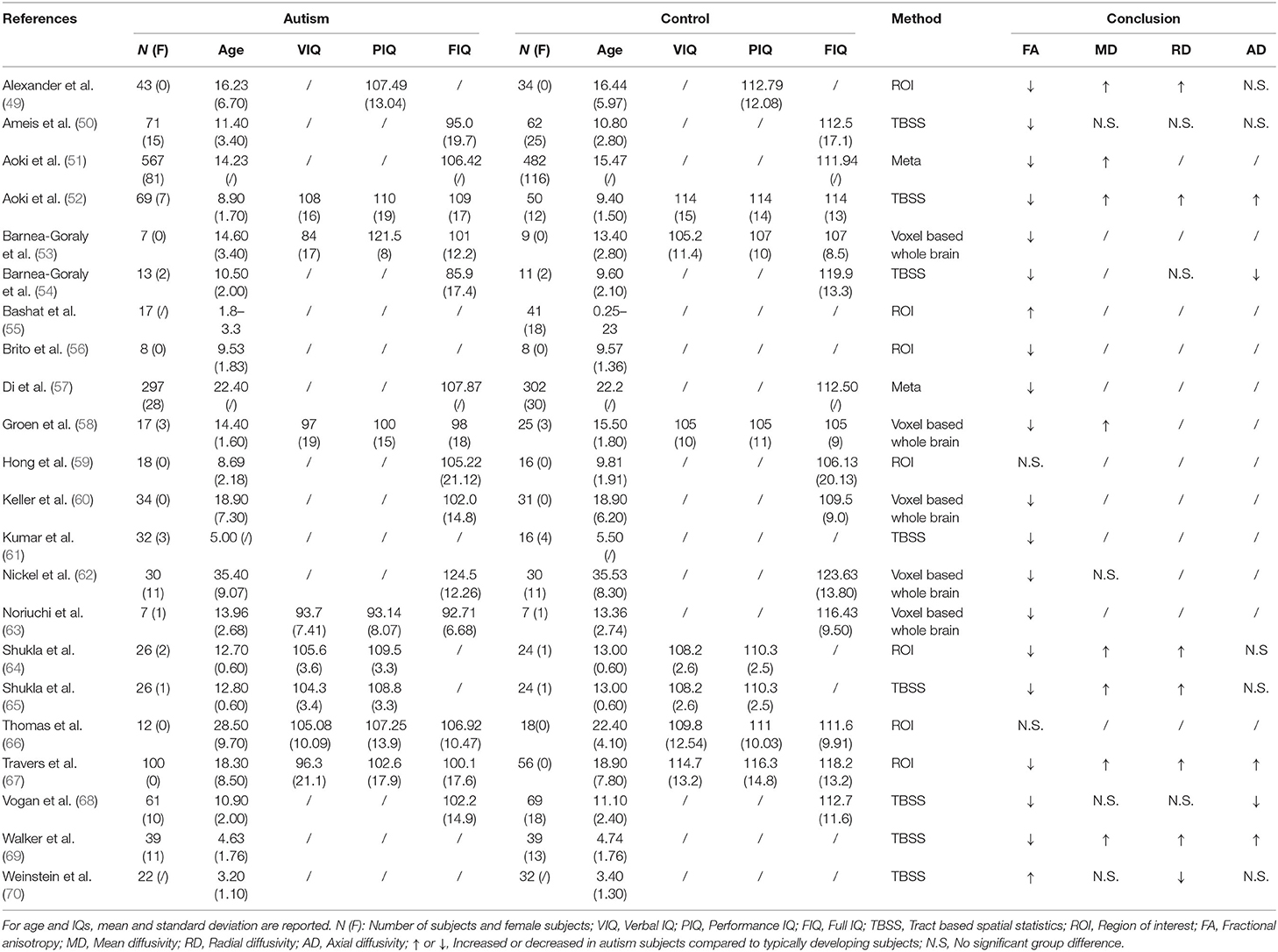
Table 3. Overview of the diffusion-weighted imaging studies examining corpus callosum abnormities in ASD patients.
Inter-hemispheric rsFC Abnormalities in ASD
Based on the cortical underconnectivity theory, aberrant rsFC patterns of ASD can be characterized by local over-connectivity but long-distance under-connectivity (13, 71, 72). As a typical long-distance connection, inter-hemispheric rsFC reflects the correlation of fMRI BOLD signal time series between brain regions belonging to the 2 brain hemispheres and can be used as an important index for delineating inter-hemispheric synchronization abnormalities in spontaneous neural activity in ASD. Support for the functional importance of inter-hemispheric connections comes from studies on agenesis or sections of CC. The CC is the major white-matter tract between the left and right hemispheres and plays a crucial role in the maintenance of interhemispheric functional communications (73). Individuals with congenital callosal agenesis have been reported to exhibit ASD-like symptoms such as deficits in language comprehension, theory of mind and social reasoning (74) and strongly elevated autistic traits as measured by the Autism Spectrum Quotient (75). Reduced inter-hemispheric rsFC in brain regions of the default mode network (DMN) and salience network have also been found in callosal dysgenesis subjects (76), although interestingly this was not found to influence the qualitative organization pattern of the rsFC (76). However, another study has reported an intact pattern of inter-hemispheric rsFC in callosal dysgenesis individuals using region of interest (ROI) and independent component analysis-based approaches (77). This inconsistency could be due to the occurrence of a remarkable plasticity compensating for the disconnection, as revealed by an animal callosotomy study reporting that a restoration of inter-hemispheric rsFC occurred from 7 to 28 days post-callosotomy but only when the callosotomy was partial and not when it was total (78). The human callosal dysgenesis studies reporting normal interhemispheric rsFC would similarly have been partial. Furthermore, another gene knockdown study in rodents has further emphasized the importance of inter-hemispheric connectivity in ASD symptomatology, such that permanent deficits in callosal projections and associated inter-hemispheric connections caused by downregulation of Wnt signaling induced ASD-like impairments, including social interaction deficits and repetitive motor behavior (79). Overall these studies both emphasize the importance of inter-hemispheric connections in the context of normal social functioning and autism and that under normal circumstances these functional connections can be restored to a large extent even when the CC does not develop fully or is partially disconnected.
Based on a voxel-based whole brain analysis, Cheng et al. (11) revealed that the majority of altered rsFC occur between regions from the opposite hemispheres using the ABIDE database, and more specifically that reduced rsFC is found mainly between brain regions implicated in face expression processing, theory of mind and self-other distinction. Also using the ABIDE database, another voxel-based study using a graph theory analysis approach specifically examined inter- and intra-hemispheric rsFC in ASD subjects and reported that they had reduced inter and intra-hemispheric functional connectivity strength in regions of the DMN and the visual system relative to TD subjects (21). Note that significant correlations were only observed between symptom severity, as measured by the restricted and repetitive behavior subscale of the Autism Diagnostic Observation Schedule (ADOS), and the density of inter- but not intra-hemispheric FC in the posterior cingulate cortex (PCC) and precuneus which both represent core nodes of the posterior DMN. Using only data from children included in the ABIDE database, a more recent study using a sliding-window analysis has shown increased inter- and intra-hemispheric dynamic FC variability in the medial pre-frontal cortex and the anterior cingulate cortex but decreased inter- and intra-hemispheric dynamic FC variability in the fusiform gyrus and the inferior temporal gyrus (ITG) in ASD relative to TD children (27). Furthermore, again only aberrant temporal variability of the inter- but not intra-hemispheric FC was associated with ADS symptom load, specifically social communication deficits as measured by the ADOS communication subscale. Overall, these findings tend to indicate that, although inter- and intra-hemispheric rsFC both show abnormities in ASD, the inter-hemispheric ones may be of greater clinical relevance.
Given that atypical developmental trajectories of functional and structural organization in these brain networks have also been demonstrated by longitudinal studies (72, 80, 81), attention should also be paid to the neurodevelopmental trajectories of inter-hemispheric functional connectivity, but current studies on this are very limited. Using functional near infrared spectroscopy (fNIRS), a longitudinal study on infants with a high risk of ASD in the first year of life has demonstrated that while they show an increase in both the inter- and intra-hemispheric connectivity in temporal and inferior frontal cortex at the age of 3 months, they exhibit a pattern of decreased functional connectivity at the age of 12 months (29). Another fNIRS study using similar regions of interest reported that this pattern of decreased inter-hemispheric functional connectivity was maintained in older children (mean age = 9.0 ± 1.3) (33), although no longitudinal study to date has specifically investigated the developmental trajectories of interhemispheric rsFC for children between the ages of 1 to 9 years of age. A recent study using electroencephalographic (EEG) measurement on alpha phase coherence has also revealed reduced inter-hemispheric connectivity in the temporal cortex in ASD children (mean age = 5.79 ± 2.01) (24). A similar pattern of reduced inter-hemispheric connection has also been found between temporal and parietal cortex in tuberous sclerosis complex infants who later developed ASD, which becomes to be most robust at 24 months (25), although the comorbidities of tuberous sclerosis complex and ASD have to be kept in mind when interpreting these findings. Finally, another EEG study with intermittent photic stimulation on an older sample size (mean age = 9.7 ± 2.2) revealed a more general decrease in inter-hemispheric coherent connections of the 20 highest connections at all the beta frequencies corresponding to photic stimulation (31).
Altered Homotopic Inter-hemispheric rsFC in ASD
Homotopic inter-hemispheric rsFC is a component part of overall interhemispheric rsFC and is an important measure reflecting activity synchronization between homotopic regions of the 2 hemispheres (82, 83). Homotopic FC can be measured using voxel-mirrored connectivity analysis on the correlation of fMRI BOLD signal time series between each voxel in 1 hemisphere and its mirrored counterpart in the other side (82). Homotopic inter-hemispheric rsFC abnormalities have been found in a number of psychiatric disorders including schizophrenia (84), major depression (85), substance dependence (86), and even Parkinson's disease (87).
In the first study examining homotopic inter-hemispheric rsFC changes in ASD, a moderate sample size (53 high-functioning ASD males vs. 39 TD controls) was used and found decreased homotopic rsFC between the bilateral sensorimotor cortex, anterior insula, and superior parietal lobule in ASD subjects compared to TD controls (22). A further ROI-based study has also revealed decreased homotopic inter-hemispheric rsFC between the superior temporal gyrus (STG) and inferior frontal gyrus (IFG) in naturally sleeping toddlers with autism relative to TD controls (29 ASD vs. 30 controls), with homotopic connectivity strengths of the IFG being negatively correlated with social and communication impairments, as measured by ADOS (26). However, while decreased homotopic connectivity was found in the lateral occipital cortex and the postcentral gyrus in ASD, Hahamy et al. (28) additionally demonstrated increased homotopic inter-hemispheric rsFC in the ITG and the middle frontal gyrus in ASD using a moderate sample size (68 ASD males vs. 73 TD controls) consisting of subjects from selective sites in ABIDE-I. This study has further revealed significantly greater individual topographical distortions in the homotopic rsFC patterns in ASD relative to TD controls (28). More robust findings have subsequently come from studies using larger numbers of subjects from the ABIDE database. In the first study using the ABIDE database, reduced homotopic inter-hemispheric rsFC has also been found in regions including the PCC, insula, and thalamus (19). In a further study which included gender as an additional covariate, Li et al. (23) carried out a voxel-based whole brain analysis on differences in homotopic inter-hemispheric rsFC in ASD subjects and further examined associations between homotopic rsFC and symptom severity. Results showed decreased homotopic rsFC of regions in large-scale brain networks including the DMN, salience network, mirror neuron/motor systems, and auditory and visual systems in ASD adolescents and adults (Figure 1), with homotopic rsFC in the PCC, insula and STG showing significant associations with social impairments as measured by ADOS. A more recent follow-up study using independent data from children in ABIDE I and ABIDE II databases and the same analysis approach has revealed consistent patterns of reduced homotopic inter-hemispheric rsFC in ASD children between the ages of 5 and 10 years old, suggesting that the homotopic alterations can occur at an early age and remain stable in contrast to other dysregulations which vary across developmental periods. Moreover, this study has also demonstrated significant associations between ASD symptom severity and homotopic inter-hemispheric rsFC changes in regions of the DMN (the PCC and the precuneus) and the visual processing networks (inferior and middle temporal gyri and inferior occipital gyrus) (32). Atypical developmental trajectories of homotopic rsFC and its association with symptom severity have also been reported in ASD (88). Note that although a recent study specifically examining reproducibility across sites has also demonstrated overall decreased homotopic inter-hemispheric rsFC in ASD using the whole ABIDE datasets, as consistently observed in most of the previous studies, the decreased homotopic rsFC pattern was not generally reproducible across sites (30), which could be due to decreased statistical power as a result of smaller sample sizes when individual sites were examined separately.
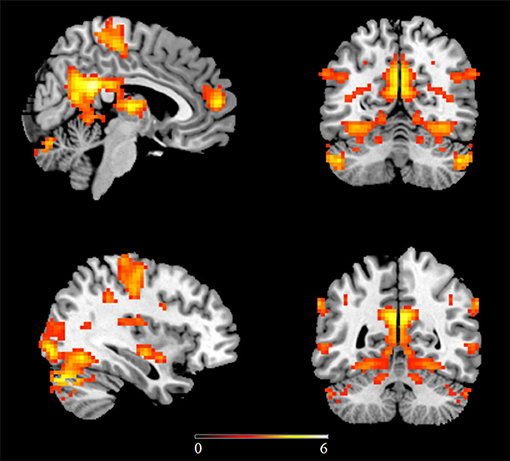
Figure 1. Regions showing decreased homotopic inter-hemispheric functional connectivity in ASD relative to matched typically developing controls using the ABIDE database. Statistic maps were displayed with a p < 0.05 FDR corrected threshold. Color bar indicates t values of the statistical map. This figure is adapted from Li et al. (23).
Interhemispheric rsFC—a More Promising Biomarker for ASD?
In both seed-based and voxel-based rsFC analyses, altered rsFC in the DMN, particularly the PCC, is one of the most replicated findings in ASD samples (12, 13, 19, 89–92). As we outlined above, this is also the case for interhemispheric rsFC, particularly the homotopic connections (19, 21, 23, 32). Interestingly, the PCC and precuneus of the DMN, the STG, and the insula which exhibited associations between their interhemispheric rsFC (including the homotopic ones) and symptom severity in ASD, are all regions strongly involved in social and affective processing (21, 23, 32). These associations have been demonstrated only for the inter-hemispheric (including the homotopic) rsFC alterations but not for the intra-hemispheric ones (21, 23, 27). Furthermore, based on inter-hemispheric, but not only the homotopic rsFC changes, pattern classification analysis achieved an average accuracy of 88.70% for distinguishing ASD subjects from TD controls across sites (23), which is slightly higher than a previous study (82.87%) using the same pattern classification approach based on whole brain-wide rsFC changes (namely more features) (11), although there is no direct statistical comparison. These findings together suggest that brain regions implicated in processing of social and affective domains maybe of greater clinical relevance. Note that although alterations in homotopic inter-hemispheric rsFC have also been found in other disorders such as schizophrenia (84), major depression (85), substance dependence (86), and Parkinson's disease (87), a consistent pattern of decreased homotopic interhemispheric rsFC has only been found in ASD in most of the previous studies across children, adolescents and adults. In other developmental disorders, increased rather than decreased homotopic rsFC has been observed in children with attention-deficit hyperactivity disorder (93) and both increased and decreased homotopic rsFC have been reported in children with Tourette's syndrome (94). Thus, although decreased inter-hemispheric rsFC may not be a biomarker specific for ASD, it may still be a promising biomarker for ASD diagnosis although probably in conjunction with other measures.
Corpus Callosum and Inter-hemispheric rsFC in ASD
As the major white-matter tract between the 2 brain hemispheres, CC serves as a bridge for interhemispheric functional communications. In ASD, CC volume has been proposed as an important index of interhemispheric functional connectivity (37, 46). Early findings on CC volume mainly utilize the traditional morphometric method based on the Witelson partition [Figure 2; (95)]. While these studies have consistently demonstrated an overall reduction of CC volume in ASD subjects with and without intellectual disability (35, 42, 43, 96), evidence on the main contributing subregions for such a global reduction effect has tended to be inconsistent with some studies showing significant decreased volume in the anterior subregions (40) and others reporting decreases in posterior part (35, 43) or the CC body (42), although subjects examined in these studies vary across young children, adolescents and adults (from 3 to 42 years old) and across high and low functioning ASD. Vidal et al. (46) further confirmed a global reduction in CC volume and the anterior part of the CC using traditional morphometric methods and found regional reduction in both the anterior (genu) and posterior (splenium) part of the CC using computational mapping methods, reflecting an impact of analysis methods on revealing which specific CC subregions exhibit alterations in ASD. Based on a voxel-based morphometric (VBM) whole brain analysis, a volume reduction has only been found in the posterior CC in ASD [splenium and isthmus; Waiter et al. (47)]. Another VBM study that specifically focused on the CC, has also revealed reduced volume in the posterior (splenium) and additionally in the anterior part (genu and rostrum) of the CC (34). Thus, the regionally restricted (ROI) approach in the latter study may be of greater sensitivity compared to analyses corrected at the whole brain used in the former one. A volume reduction in the anterior and posterior regions of the CC in ASD has also been confirmed by a VBM analysis in a more recent study using the ABIDE database, in which gender, age, IQ, and total brain volume were controlled as potential confounding variables (23). However, using a surface-based approach, Haar et al. (39) did not observe any significant changes in global CC volume in ASD using the ABIDE database, whereas they did find decreased volume in the central part of CC with a small effect size after segmenting the CC into 5 equal parts. Morphometric CC changes in the middle (midbody) and posterior (isthmus and splenium) part have also been confirmed by a diffusional kurtosis imaging study examining axonal density and caliber (45). Finally, another study using the ABIDE database, found no significant CC volume changes based on a more complex model-based analysis method but that CC scaled non-linearly with brain volume, as reflected by large brains having a proportionally smaller CC (41). Meta-analysis studies can provide us with more comprehensive and robust insight on ASD-associated CC changes. In one meta-analytic study ASD individuals were reported to exhibit a general CC volume reduction with the reduction magnitude decreasing from the anterior to posterior part (37). A subsequent meta-analysis has also suggested a group difference in CC volume between the ASD and the TD control groups, but this effect was highly underpowered due to the small sample sizes used in the included studies (41).
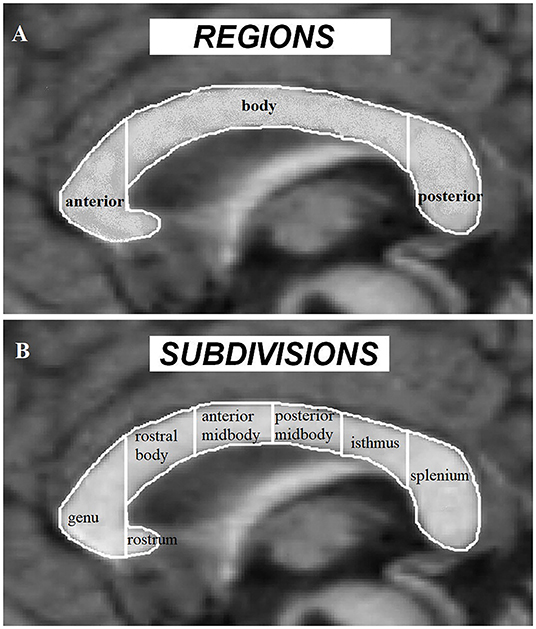
Figure 2. Corpus callosum regions (A) and Witelson subdivisions (B) adapted from Frazier and Hardan (37).
In addition, diffusion-weighted imaging (DWI) studies have also revealed CC abnormalities in ASD, as reflected by a general pattern of reduced fractional anisotropy (FA) and concomitantly increased mean diffusivity (MD) or radial diffusivity (RD) in ASD compared to TD controls. This pattern has been found not only in the total CC or across all subregions including the anterior (genu), body, and posterior (splenium) part of CC (49, 52, 58, 64, 65, 67–69), but also in specific CC subregions such as in the genu and body (49, 53, 62), the genu/rostral body (56, 63), the posterior midbody and isthmus (60), and the splenium of CC (50, 57) in high/low-functioning ASD individuals across different age ranges, including children, adolescents, and adults. This has also been confirmed by a diffusion tensor imaging meta-analysis study (51). While having confirmed this pattern of FA changes in ASD children, Barnea-Goraly et al. (54) further revealed that this pattern was shared between ASD children and their unaffected siblings, indicating that FA changes may represent a neural marker reflecting an increased genetic risk or generally increased vulnerability for ASD. Of note very young children with ASD exhibit a different pattern of alterations, such that increased FA in genu and splenium of CC have been found in ASD children aging from 1.8 to 3.3 (55) and an increase in FA but a decrease in RD in the genu and midbody of CC have been found in a slightly older sample (mean age 3.2 ± 1.1) with no changes in MD (70). Moreover, another study did not detect FA changes in adults with ASD (66). Thus, apart from these latter findings the majority of studies converge on a decrease in FA but an increase in MD and RD of CC in ASD. A longitudinal study has further revealed an atypical developmental trajectory of FA of the genu and body of CC before the age of 10 in ASD (67). In contrast, examination of axial diffusivity (AD) revealed somewhat inconsistent results in ASD, with some studies demonstrating significantly higher AD (52) or lower AD either across all 3 subregions (68) or specifically in the body or genu of CC (54, 64) in ASD and others reporting no significant AD differences (49, 70). With respect to associations between diffusion tensor imaging alterations and ASD symptom severity, FA in CC has been found to be negatively correlated with social impairment in ASD as measured by the Social Responsiveness Scale completed by Parents (SRS-P), while alterations in MD and RD have been found to be positively correlated with SRS-P scores (52). In contrast, no significant correlations between FA and diffusivity indices and symptom severity as measured by ADOS have been observed (49, 54, 68). In addition to lower FA and higher diffusivity (as measured by apparent diffusion coefficient), longer fiber length, higher fiber density but a lower fiber number of CC have been reported in ASD children (59, 61), with the fiber number in the anterior CC being negatively correlated with scores on the Childhood Autism Rating Scale (59).
Given that both interhemispheric rsFC and CC volumes exhibit a decreased pattern in ASD individuals, there are also a few studies which have investigated possible relationships between these 2 measures. An absence of significant correlations between the homotopic interhemispheric rsFC and CC volumes has been reported both in a study based on a moderate sample size (53 ASD vs. 39 TDs) (22) and in another larger one using the ABIDE database (23). However, no studies to date have explored the associations between overall interhemispheric rsFC (i.e., not only the homotopic ones) and either CC volumes or DWI indices. Pattern classification analyses based on anatomical abnormalities including variations in CC volumes have reported relatively low classification accuracies of <60% using the ABIDE database (39), which is much lower than pattern classification accuracy based on interhemispheric rsFC changes (23), indicating that anatomical abnormalities measured by conventional MRI protocols are probably too coarse to be a sensitive and robust diagnostic biomarker.
Taken together, although there are some studies which have found no significant CC volume or DWI indices changes in ASD patients (36, 38, 44, 66, 70), the majority of studies have provided converging evidence for decreased CC volumes and FA but increased MD or RD in ASD, particularly those studies based on the traditional morphometric, VBM and DWI methods, although findings on the involvement of specific CC subregions have been less consistent. The heterogeneity of findings on CC volume and DWI indices could be due to differences in sample size, age range, gender, and subject phenotypes [e.g., with and without intellectual disability/high vs. low functioning; cf., (36, 38, 44, 51, 57)]. Differences in analytical approaches and whether potential confounding covariates (e.g., age, gender, IQ, and total brain volume) are well-controlled may also have contributed to these inconsistencies [cf., (34, 40, 43)]. Furthermore, in a longitudinal study of infants (6–24 months), in which subjects were much younger than in all of the studies reviewed above, increased CC area and thickness, particularly robust in the anterior CC, have been found for ASD infants at 6 and 12 months and associated with repetitive behaviors shown at the age of 24 months (48). Similarly, different patterns of FA and RD of CC have been found in very young children with ASD as reviewed above (55, 70). Thus, the developmental trajectory of CC, similar to the aberrant developmental trajectory of the homotopic rsFC (88), should be taken into consideration when interpreting these findings.
Conclusion and Future Directions
In summary, we have reviewed the literature on inter-hemispheric rsFC and structural CC changes in ASD. While evidence on these differences are heterogeneous, due undoubtedly to some extent to variations in sample size, age range, gender, subject phenotypes and even whether potential confounding covariates are well-controlled, there are nevertheless converging findings on reduced interhemispheric (including homotopic) rsFC in large-scale brain networks particularly in the posterior hubs in the DMN, on reduced volumes in the anterior and posterior CC, and on decreased FA and increased MD or RD across CC subregions. Correlations between the inter-hemispheric rsFC changes and symptom severity in social impairments and the superior classification performance in distinguishing ASD subjects from TD controls based on the interhemispheric rsFC changes together suggest that inter-hemispheric rsFC changes, a typical form of long-distance connection, may be a more promising biomarker for ASD diagnosis than whole brain wide rsFC and structural abnormalities. Future studies will also need to focus on investigating whether these biomarkers can be applied to other novel samples with a high generalizability if we are to progress toward the ultimate goal of such biomarkers having utility at an individual as opposed to a group level. Additionally, the specificity of interhemispheric rsFC changes in ASD compared with other childhood disorders needs to be more fully established.
More future studies are also required using different multimodal brain imaging and behavioral approaches to better establish the functional consequences of dysfunctional inter-hemispheric connectivity in ASD. Furthermore, given evidence in both humans and animal models for considerably potential for functional recovery in interhemispheric rsFC following CC damage or impaired development it will be important to establish if this could be exploited therapeutically. In addition, based on previous finding that the development or microstructure of CC can be modulated by factors such as sex hormones (97, 98), age (99), prenatal inflammation (100) and mutation of specific genes (101, 102), future studies should aim at disentangling the potential contributions of these factors to CC alterations in ASD.
There has also been considerable interest in identifying sub-types in ASD, as in other disorders, and moving forward it will clearly be important to do this either based on brain- or behavioral-based subtyping. Previous research on brain-based biomarkers has also been focused almost exclusively on high functioning ASD and on adolescents and adults and therefore there is an urgent need for more studies and hopefully databases which include low-functioning individuals and particularly young children where early diagnosis and therapeutic interventions are potentially of greatest importance. Finally, to make findings across studies and databases more comparable and reproducible, it is also important for future studies follow standardized data processing procedures with potential confounding covariates being well-controlled [cf., (103, 104)].
Author Contributions
All authors contributed to the information and ideas presented in the review and writing of the manuscript.
Funding
This study was supported by the National Natural Science Foundation of China (NSFC) grants (Grant No. 31700998) and by the Department of Science and Technology of Guangdong Province (Grant No. 2018B030335001).
Conflict of Interest
The authors declare that the research was conducted in the absence of any commercial or financial relationships that could be construed as a potential conflict of interest.
References
1. Fombonne E. The rising prevalence of autism. J Child Psychol Psychiatry. (2018) 59:717–20. doi: 10.1111/jcpp.12941
2. American Psychiatric Association. Diagnostic and Statistical Manual of Mental Disorders: DSM-5. Washington, DC: APA (2013). doi: 10.1176/appi.books.9780890425596
3. Fedor J, Lynn A, Foran W, DiCicco-Bloom J, Luna B, O'Hearn K. Patterns of fixation during face recognition: differences in autism across age. Autism. (2018) 22:866–80. doi: 10.1177/1362361317714989
4. Joseph RM, Tanaka J. Holistic and part-based face recognition in children with autism. J Child Psychol Psychiatry. (2003) 44:529–42. doi: 10.1111/1469-7610.00142
5. Wang AT, Dapretto M, Hariri AR, Sigman M, Bookheimer SY. Neural correlates of facial affect processing in children and adolescents with autism spectrum disorder. J Am Acad Child Adolesc Psychiatry. (2004) 43:481–90. doi: 10.1097/00004583-200404000-00015
6. Madipakkam AR, Rothkirch M, Dziobek I, Sterzer P. Unconscious avoidance of eye contact in autism spectrum disorder. Sci Rep. (2017) 7:13378. doi: 10.1038/s41598-017-13945-5
7. Senju A, Johnson MH. Atypical eye contact in autism: models, mechanisms and development. Neurosci Biobehav Rev. (2009) 33:1204–14. doi: 10.1016/j.neubiorev.2009.06.001
8. von dem Hagen EA, Stoyanova RS, Rowe JB, Baron-Cohen S, Calder AJ. Direct gaze elicits atypical activation of the theory-of-mind network in autism spectrum conditions. Cereb Cortex. (2014) 24:1485–92. doi: 10.1093/cercor/bht003
9. Chevallier C, Grèzes J, Molesworth C, Berthoz S, Happé F. Brief report: selective social anhedonia in high functioning autism. J Autism Dev Disord. (2012) 42:1504–9. doi: 10.1007/s10803-011-1364-0
10. Morrison KE, Pinkham AE, Penn DL, Kelsven S, Ludwig K, Sasson NJ. Distinct profiles of social skill in adults with autism spectrum disorder and schizophrenia. Autism Res. (2017) 10:878–87. doi: 10.1002/aur.1734
11. Cheng W, Rolls ET, Gu G, Zhang J, Feng J. Autism: reduced connectivity between cortical areas involved in face expression, theory of mind and the sense of self. Brain. (2015) 138:1382–93. doi: 10.1093/brain/awv051
12. Hull JV, Jacokes ZJ, Torgerson CM, Irimia A, van Horn JD. Resting-state functional connectivity in autism spectrum disorders: a review. Front Psychiatry. (2017) 7:205. doi: 10.3389/fpsyt.2016.00205
13. Lau W, Leung MK, Lau B. Resting-state abnormalities in autism spectrum disorders: a meta-analysis. Sci Rep. (2019) 9:3892. doi: 10.1038/s41598-019-40427-7
14. Rane P, Cochran D, Hodge SM, Haselgrove C, Kennedy DN, Frazier JA. Connectivity in autism: a review of MRI connectivity studies. Harv Rev Psychiatry. (2015) 23:223–44. doi: 10.1097/HRP.0000000000000072
15. Fishman I, Keown CL, Lincoln AJ, Pineda JA, Müller RA. Atypical cross talk between mentalizing and mirror neuron networks in autism spectrum disorder. JAMA Psychiatry. (2014) 71:751–60. doi: 10.1001/jamapsychiatry.2014.83
16. Funakoshi Y, Harada M, Otsuka H, Mori K, Ito H, Iwanaga T. Default mode network abnormalities in children with autism spectrum disorder detected by resting-state functional magnetic resonance imaging. J Med Invest. (2016) 63:204–8. doi: 10.2152/jmi.63.204
17. Monk CS, Peltier SJ, Wiggins JL, Weng SJ, Carrasco M, Risi S, et al. Abnormalities of intrinsic functional connectivity in autism spectrum disorders. Neuroimage. (2009) 47:764–72. doi: 10.1016/j.neuroimage.2009.04.069
18. Holiga Š, Hip JF, Chatham CH, Garces P, Spooren W, D'Ardhuy XL, et al. Patients with autism spectrum disorders display reproducible functional connectivity alterations. Sci Transl Med. (2019) 11:eaat9223. doi: 10.1126/scitranslmed.aat9223
19. Di Martino A, Yan CG, Li Q, Denio E, Castellanos FX, Alaerts K, et al. The autism brain imaging data exchange: towards large-scale evaluation of the intrinsic brain architecture in autism. Mol Psychiatry. (2014) 19:659–67. doi: 10.1038/mp.2013.78
20. Zhang J, Kendrick KM, Lu G, Feng J. The fault lies on the other side: altered brain functional connectivity in psychiatric disorders is mainly caused by counterpart regions in the opposite hemisphere. Cereb Cortex. (2015) 25:3475–86. doi: 10.1093/cercor/bhu173
21. Lee JM, Kyeong S, Kim E, Cheon KA. Abnormalities of inter- and intra-hemispheric functional connectivity in autism spectrum disorders: a study using the autism brain imaging data exchange database. Front Neurosci. (2016) 10:191. doi: 10.3389/fnins.2016.00191
22. Anderson JS, Druzgal TJ, Froehlich A, DuBray MB, Lange N, Alexander AL, et al. Decreased interhemispheric functional connectivity in autism. Cereb Cortex. (2011) 21:1134–46. doi: 10.1093/cercor/bhq190
23. Li Q, Becker B, Jiang X, Zhao Z, Zhang Q, Yao S, et al. Decreased interhemispheric functional connectivity rather than corpus callosum volume as a potential biomarker for autism spectrum disorder. Cortex. (2019) 119:258–66. doi: 10.1016/j.cortex.2019.05.003
24. Dickinson A, DiStefano C, Lin YY, Scheffler AW, Senturk D, Jeste SS. Interhemispheric alpha-band hypoconnectivity in children with autism spectrum disorder. Behav Brain Res. (2018) 348:227–34. doi: 10.1016/j.bbr.2018.04.026
25. Dickinson A, Varcin KJ, Sahin M, Nelson CA III, Jeste SS. Early patterns of functional brain development associated with autism spectrum disorder in tuberous sclerosis complex. Autism Res. (2019) 12:1758–73. doi: 10.1002/aur.2193
26. Dinstein I, Pierce K, Eyler L, Solso S, Malach R, Behrmann M, et al. Disrupted neural synchronization in toddlers with autism. Neuron. (2011) 70:1218–25. doi: 10.1016/j.neuron.2011.04.018
27. Guo X, Duan X, Chen H, He C, Xiao J, Han S, et al. Altered inter- and intrahemispheric functional connectivity dynamics in autistic children. Hum Brain Mapp. (2020) 41:419–28. doi: 10.1002/hbm.24812
28. Hahamy A, Behrmann M, Malach R. The idiosyncratic brain: distortion of spontaneous connectivity patterns in autism spectrum disorder. Nat Neurosci. (2015) 18:302. doi: 10.1038/nn.3919
29. Keehn B, Wagner J, Tager-Flusberg H, Nelson CA. Functional connectivity in the first year of life in infants at-risk for autism: a preliminary near-infrared spectroscopy study. Front Hum Neurosci. (2013) 7:444. doi: 10.3389/fnhum.2013.00444
30. King JB, Prigge M, King CK, Morgan J, Weathersby F, Fox JC, et al. Generalizability and reproducibility of functional connectivity in autism. Mol Autism. (2019) 10:27. doi: 10.1186/s13229-019-0273-5
31. Lazarev VV, Pontes A, Mitrofanov AA, deAzevedo LC. Reduced interhemispheric connectivity in childhood autism detected by electroencephalographic photic driving coherence. J Autism Dev Disord. (2013) 45:537–47. doi: 10.1007/s10803-013-1959-8
32. Yao S, Zhou M, Zhang Y, Zhang Q, Zhao Z, Jiang X, et al. Decreased homotopic interhemispheric functional connectivity in children with autism spectrum disorder. bioRxiv. (2020). doi: 10.1101/2020.11.08.373126
33. Zhu H, Fan Y, Guo H, Huang D, He S. Reduced interhemispheric functional connectivity of children with autism spectrum disorder: evidence from functional near infrared spectroscopy studies. Biomed Opt Express. (2014) 5:1262–74. doi: 10.1364/BOE.5.001262
34. Chung MK, Dalton KM, Alexander AL, Davidson RJ. Less white matter concentration in autism: 2D voxel-based morphometry. Neuroimage. (2004) 23:242–51. doi: 10.1016/j.neuroimage.2004.04.037
35. Egaas B, Courchesne E, Saitoh O. Reduced size of corpus callosum in autism. Arch Neurol. (1995) 52:794–801. doi: 10.1001/archneur.1995.00540320070014
36. Elia M, Ferri R, Musumeci SA, Panerai S, Bottitta M, Scuderi C. Clinical correlates of brain morphometric features of subjects with low-functioning autistic disorder. J Child Neurol. (2000) 15:504–8. doi: 10.1177/088307380001500802
37. Frazier TW, Hardan AY. A meta-analysis of the corpus callosum in autism. Biol psychiatry. (2009) 66:935–41. doi: 10.1016/j.biopsych.2009.07.022
38. Gaffney GR, Kuperman S, Tsai LY, Minchin S, Hassanein KM. Midsagittal magnetic resonance imaging of autism. Br J Psychiatry. (1987) 151:831–3. doi: 10.1192/bjp.151.6.831
39. Haar S, Berman S, Behrmann M, Dinstein I. Anatomical abnormalities in autism. Cereb Cortex. (2016) 26:1440–52. doi: 10.1093/cercor/bhu242
40. Hardan AY, Minshew NJ, Keshavan MS. Corpus callosum size in autism. Neurology. (2000) 55:1033–6. doi: 10.1212/WNL.55.7.1033
41. Lefebvre A, Beggiato A, Bourgeron T, Toro R. Neuroanatomical diversity of corpus callosum and brain volume in autism: meta-analysis, analysis of the autism brain imaging data exchange project, and simulation. Biol Psychiatry. (2015) 78:126–34. doi: 10.1016/j.biopsych.2015.02.010
42. Manes F, Piven J, Vrancic D, Nanclares V, Plebst C, Starkstein SE. An MRI study of the corpus callosum and cerebellum in mentally retarded autistic individuals. J Neuropsychiatry Clin Neurosci. (1999) 11:470–4. doi: 10.1176/jnp.11.4.470
43. Piven J, Bailey J, Ranson BJ, Arndt S. An MRI study of the corpus callosum in autism. Am J Psychiatry. (1997) 154:1051–6. doi: 10.1176/ajp.154.8.1051
44. Rice SA, Bigler ED, Cleavinger HB, Tate DF, Sayer J, McMahon W, et al. Macrocephaly, corpus callosum morphology, and autism. J Child Neurol. (2005) 20:34–41. doi: 10.1177/08830738050200010601
45. Sui YV, Donaldson J, Miles L, Babb JS, Castellanos FX, Lazar M. Diffusional kurtosis imaging of the corpus callosum in autism. Mol Autism. (2018) 9:62. doi: 10.1186/s13229-018-0245-1
46. Vidal CN, Nicolson R, DeVito TJ, Hayashi KM, Geaga JA, Drost DJ, et al. Mapping corpus callosum deficits in autism: an index of aberrant cortical connectivity. Biol Psychiatry. (2006) 60:218–25. doi: 10.1016/j.biopsych.2005.11.011
47. Waiter GD, Williams JH, Murray AD, Gilchrist A, Perrett DI, Whiten A. Structural white matter deficits in high-functioning individuals with autistic spectrum disorder: a voxel-based investigation. Neuroimage. (2005) 24:455–61. doi: 10.1016/j.neuroimage.2004.08.049
48. Wolff JJ, Gerig G, Lewis JD, Soda T, Styner MA, Vachet C, et al. Altered corpus callosum morphology associated with autism over the first 2 years of life. Brain. (2015) 138:2046–58. doi: 10.1093/brain/awv118
49. Alexander AL, Lee JE, Lazar M, Boudos R, DuBray MB, Oakes TR, et al. Diffusion tensor imaging of the corpus callosum in Autism. Neuroimage. (2007) 34:61–73. doi: 10.1016/j.neuroimage.2006.08.032
50. Ameis SH, Lerch JP, Taylor MJ, Lee W, Viviano JD, Pipitone J, et al. A diffusion tensor imaging study in children with ADHD, autism spectrum disorder, OCD, and matched controls: distinct and non-distinct white matter disruption and dimensional brain-behavior relationships. Am J Psychiatry. (2016) 173:1213–22. doi: 10.1176/appi.ajp.2016.15111435
51. Aoki Y, Abe O, Nippashi Y, Yamasue H. Comparison of white matter integrity between autism spectrum disorder subjects and typically developing individuals: a meta-analysis of diffusion tensor imaging tractography studies. Mol Autism. (2013) 4:25. doi: 10.1186/2040-2392-4-25
52. Aoki Y, Yoncheva YN, Chen B, Nath T, Sharp D, Lazar M, et al. Association of white matter structure with autism spectrum disorder and attention-deficit/hyperactivity disorder. JAMA Psychiatry. (2017) 74:1120–8. doi: 10.1001/jamapsychiatry.2017.2573
53. Barnea-Goraly N, Kwon H, Menon V, Eliez S, Lotspeich L, Reiss AL. White matter structure in autism: preliminary evidence from diffusion tensor imaging. Biol Psychiatry. (2004) 55:323–6. doi: 10.1016/j.biopsych.2003.10.022
54. Barnea-Goraly N, Lotspeich LJ, Reiss AL. Similar white matter aberrations in children with autism and their unaffected siblings: a diffusion tensor imaging study using tract-based spatial statistics. Arch Gen Psychiatry. (2010) 67:1052–60. doi: 10.1001/archgenpsychiatry.2010.123
55. Bashat DB, Kronfeld-Duenias V, Zachor DA, Ekstein PM, Hendler T, Tarrasch R, et al. Accelerated maturation of white matter in young children with autism: a high b value DWI study. Neuroimage. (2007) 37:40–7. doi: 10.1016/j.neuroimage.2007.04.060
56. Brito AR, Vasconcelos MM, Domingues RC, Hygino da Cruz LC Jr, Rodrigues LDS, Gasparetto EL, et al. Diffusion tensor imaging findings in school-aged autistic children. J Neuroimaging. (2009) 19:337–43. doi: 10.1111/j.1552-6569.2009.00366.x
57. Di X, Azeez A, Li X, Haque E, Biswal BB. Disrupted focal white matter integrity in autism spectrum disorder: A voxel-based meta-analysis of diffusion tensor imaging studies. Prog Neuropsychopharmacol Biol Psychiatry. (2018) 82:242–8. doi: 10.1016/j.pnpbp.2017.11.007
58. Groen WB, Buitelaar JK, Van Der Gaag RJ, Zwiers MP. Pervasive microstructural abnormalities in autism: a DTI study. J Psychiatry Neurosci. (2011) 36:32. doi: 10.1503/jpn.090100
59. Hong S, Ke X, Tang T, Hang Y, Chu K, Huang H, et al. Detecting abnormalities of corpus callosum connectivity in autism using magnetic resonance imaging and diffusion tensor tractography. Psychiatry Res. (2011) 194:333–9. doi: 10.1016/j.pscychresns.2011.03.009
60. Keller TA, Kana RK, Just MA. A developmental study of the structural integrity of white matter in autism. Neuroreport. (2007) 18:23–7. doi: 10.1097/01.wnr.0000239965.21685.99
61. Kumar A, Sundaram SK, Sivaswamy L, Behen ME, Makki MI, Ager J, et al. Alterations in frontal lobe tracts and corpus callosum in young children with autism spectrum disorder. Cereb Cortex. (2010) 20:2103–13. doi: 10.1093/cercor/bhp278
62. Nickel K, Tebartz van Elst L, Perlov E, Endres D, Müller GT, Riedel A, et al. Altered white matter integrity in adults with autism spectrum disorder and an IQ > 100: a diffusion tensor imaging study. Acta Psychiatr Scand. (2017) 135:573–83. doi: 10.1111/acps.12731
63. Noriuchi M, Kikuchi Y, Yoshiura T, Kira R, Shigeto H, Hara T, et al. Altered white matter fractional anisotropy and social impairment in children with autism spectrum disorder. Brain Res. (2010) 1362:141–9. doi: 10.1016/j.brainres.2010.09.051
64. Shukla DK, Keehn B, Lincoln AJ, Müller RA. White matter compromise of callosal and subcortical fiber tracts in children with autism spectrum disorder: a diffusion tensor imaging study. J Am Acad Child Adolesc Psychiatry. (2010) 49:1269–78. doi: 10.1016/j.jaac.2010.08.018
65. Shukla DK, Keehn B, Müller RA. Tract-specific analyses of diffusion tensor imaging show widespread white matter compromise in autism spectrum disorder. J Child Psychol Psychiatry. (2011) 52:286–95. doi: 10.1111/j.1469-7610.2010.02342.x
66. Thomas C, Humphreys K, Jung KJ, Minshew N, Behrmann M. The anatomy of the callosal and visual-association pathways in high-functioning autism: a DTI tractography study. Cortex. (2011) 47:863–73. doi: 10.1016/j.cortex.2010.07.006
67. Travers BG, Tromp DP, Adluru N, Lange N, Destiche D, Ennis C, et al. Atypical development of white matter microstructure of the corpus callosum in males with autism: a longitudinal investigation. Mol Autism. (2015) 6:15. 10.1186/s13229-015-0001-8. doi: 10.1186/s13229-015-0001-8
68. Vogan VM, Morgan BR, Leung RC, Anagnostou E, Doyle-Thomas K, Taylor MJ. Widespread white matter differences in children and adolescents with autism spectrum disorder. J Autism Dev Disord. (2016) 46:2138–47. doi: 10.1007/s10803-016-2744-2
69. Walker L, Gozzi M, Lenroot R, Thurm A, Behseta B, Swedo S, et al. Diffusion tensor imaging in young children with autism: biological effects and potential confounds. Biol Psychiatry. (2012) 72:1043–51. doi: 10.1016/j.biopsych.2012.08.001
70. Weinstein M, Ben-Sira L, Levy Y, Zachor DA, Itzhak EB, Artzi M, et al. Abnormal white matter integrity in young children with autism. Hum Brain Mapp. (2011) 32:534–43. doi: 10.1002/hbm.21042
71. Courchesne E, Pierce K. Why the frontal cortex in autism might be talking only to itself: local over-connectivity but long-distance disconnection. Curr Opin Neurobiol. (2005) 15:225–30. doi: 10.1016/j.conb.2005.03.001
72. Geschwind DH, Levitt P. Autism spectrum disorders: developmental disconnection syndromes. Curr Opin Neurobiol. (2007) 17:103–11. doi: 10.1016/j.conb.2007.01.009
73. Roland JL, Snyder AZ, Hacker CD, Mitra A, Shimony JS, Limbrick DD, et al. On the role of the corpus callosum in interhemispheric functional connectivity in humans. Proc Natl Acad Sci USA. (2017) 114:13278–83. doi: 10.1073/pnas.1707050114
74. Paul LK, Corsello C, Kennedy DP, Adolphs R. Agenesis of the corpus callosum and autism: a comprehensive comparison. Brain. (2014) 137:1813–29. doi: 10.1093/brain/awu070
75. Lau YC, Hinkley LB, Bukshpun P, Strominger ZA, Wakahiro ML, Baron-Cohen S, et al. Autism traits in individuals with agenesis of the corpus callosum. J Autism Dev Disord. (2013) 43:1106–18. doi: 10.1007/s10803-012-1653-2
76. Owen JP, Li YO, Yang FG, Shetty C, Bukshpun P, Vora S, et al. Resting-state networks and the functional connectome of the human brain in agenesis of the corpus callosum. Brain Connect. (2013) 3:547–62. doi: 10.1089/brain.2013.0175
77. Tyszka JM, Kennedy DP, Adolphs R, Paul LK. Intact bilateral resting-state networks in the absence of the corpus callosum. J Neurosci. (2011) 31:15154–62. doi: 10.1523/JNEUROSCI.1453-11.2011
78. Zhou IY, Liang YX, Chan RW, Gao PP, Cheng JS, Hu Y, et al. Brain resting-state functional MRI connectivity: morphological foundation and plasticity. Neuroimage. (2014) 84:1–10. doi: 10.1016/j.neuroimage.2013.08.037
79. Bocchi R, Egervari K, Carol-Perdiguer L, Viale B, Quairiaux C, De Roo M, et al. Perturbed Wnt signaling leads to neuronal migration delay, altered interhemispheric connections and impaired social behavior. Nat Commun. (2017) 8:1158. doi: 10.1038/s41467-017-01046-w
80. Ecker C, Bookheimer SY, Murphy DGM. Neuroimaging in autism spectrum disorder: brain structure and function across the lifespan. Lancet Neurol. (2015) 14:1121–34. doi: 10.1016/S1474-4422(15)00050-2
81. Piven J, Elison JT, Zylka MJ. Toward a conceptual framework for early brain and behavior development in autism. Mol Psychiatry. (2017) 22:1385–94. doi: 10.1038/mp.2017.131
82. Zuo XN, Kelly C, Di Martino A, Mennes M, Margulies DS, Bangaru S, et al. Growing together and growing apart: regional and sex differences in the lifespan developmental trajectories of functional homotopy. J Neurosci. (2010) 30:15034–43. doi: 10.1523/JNEUROSCI.2612-10.2010
83. Stark DE, Marguiles DS, Shehzad ZE, Reiss P, Clare Kelly AMC, Milham MP. Regional variation in interhemispheric coordination of intrinsic hemodynamic fluctuations. J Neurosci. (2008) 28:13754–64. doi: 10.1523/JNEUROSCI.4544-08.2008
84. Li HJ, Xu Y, Zhang KR, Hoptman MJ, Zuo XN. Homotopic connectivity in drug-naïve, first-episode, early-onset schizophrenia. J Child Psychol Psychiatry. (2015) 56:432–43. doi: 10.1111/jcpp.12307
85. Wang L, Li K, Zhang QE, Zeng YW, Jin Z, Dai WJ, et al. Interhemispheric functional connectivity and its relationships with clinical characteristics in major depressive disorder: a resting state fMRI study. PLoS ONE. (2013) 8:e60191. doi: 10.1371/journal.pone.0060191
86. Kelly C, Zuo XN, Gotimer K, Cox CL, Lynch L, Brock D, et al. Reduced interhemispheric resting state functional connectivity in cocaine addiction. Biol Psychiatry. (2011) 69:684–92. doi: 10.1016/j.biopsych.2010.11.022
87. Hu X, Zhang J, Jiang X, Zhou C, Wei L, Yin X, et al. Decreased interhemispheric functional connectivity in subtypes of Parkinson's disease. J Neurol. (2015) 262:760–7. doi: 10.1007/s00415-014-7627-x
88. Kozhemiako N, Vakorin V, Nunes AS, Iarocci G, Ribary U, Doesburg SM. Extreme male developmental trajectories of homotopic brain connectivity in autism. Hum Brain Mapp. (2019) 40:987–1000. doi: 10.1002/hbm.24427
89. Anderson JS, Nielsen JA, Froehlich AL, DuBray MB, Druzgal TJ, Cariello AN, et al. Functional connectivity magnetic resonance imaging classification of autism. Brain. (2011) 134:3742–54. doi: 10.1093/brain/awr263
90. Lau W, Leung MK, Zhang R. Hypofunctional connectivity between the posterior cingulate cortex and ventromedial prefrontal cortex in autism: Evidence from coordinate-based imaging meta-analysis. Prog Neuropsychopharmacol Biol Psychiatry. (2020) 103:109986. doi: 10.1016/j.pnpbp.2020.109986
91. Padmanabhan A, Lynch CJ, Schaer M, Menon V. The default mode in autism. Biol Psychiatry. (2017) 2:476–86. doi: 10.1016/j.bpsc.2017.04.004
92. Weng SJ, Wiggins JL, Peltier SJ, Carrasco M, Risi S, Lord C, et al. Alterations of resting state functional connectivity in the default network in adolescents with autism spectrum disorders. Brain Res. (2010) 1313:202–14. doi: 10.1016/j.brainres.2009.11.057
93. Jiang K, Yi Y, Li L, Li H, Shen H, Zhao F, et al. Functional network connectivity changes in children with attention-deficit hyperactivity disorder: a resting-state fMRI study. Int J Dev Neurosci. (2019) 78:1–6. doi: 10.1016/j.ijdevneu.2019.07.003
94. Liao W, Yu Y, Miao HH, Feng YX, Ji GJ, Feng JH. Inter-hemispheric intrinsic connectivity as a Neuromarker for the diagnosis of boys with Tourette syndrome. Mol Neurobiol. (2017) 54:2781–9. doi: 10.1007/s12035-016-9863-9
95. Witelson SF. Hand and sex differences in the isthmus and genu of the human corpus callosum: a postmortem morphological study. Brain. (1989) 112:799–835. doi: 10.1093/brain/112.3.799
96. Bauman ML, Kemper TL. The Neurobiology of Autism. Baltimore, MD: Johns Hopkins University Press (1994).
97. van Hemmen J, Saris IM, Cohen-Kettenis PT, Veltman DJ, Pouwels PJW, Bakker J. Sex differences in white matter microstructure in the human brain predominantly reflect differences in sex hormone exposure. Cereb Cortex. (2017) 27:2994–001. doi: 10.1093/cercor/bhw156
98. Patel R, Moore S, Crawford DK, Hannsun G, Sasidhar MV, Tan K, et al. Attenuation of corpus callosum axon myelination and remyelination in the absence of circulating sex hormones. Brain Pathol. (2013) 23:462–75. doi: 10.1111/bpa.12029
99. Vannucci RC, Barron TF, Vannucci SJ. Development of the corpus callosum: an MRI study. Dev Neurosci. (2017) 39:97–106. doi: 10.1159/000453031
100. Pont-Lezica L, Beumer W, Colasse S, Drexhage H, Versnel M, Bessis A. Microglia shape corpus callosum axon tract fasciculation: functional impact of prenatal inflammation. Eur J Neurosci. (2014) 39:1551–7. doi: 10.1111/ejn.12508
101. Howard HC, Mount DB, Rochefort D, Byun N, Dupré N, Lu J, et al. The K-Cl cotransporter KCC3 is mutant in a severe peripheral neuropathy associated with agenesis of the corpus callosum. Nat Genet. (2002) 32:384–92. doi: 10.1038/ng1002
102. Qiu M, Anderson S, Chen S, Meneses JJ, Hevner R, Kuwana E, et al. Mutation of the Emx-1 homeobox gene disrupts the corpus callosum. Dev Biol. (1996) 178:174–8. doi: 10.1006/dbio.1996.0207
103. Di Martino A, O'connor D, Chen B, Alaerts K, Anderson JS, Assaf M, et al. Enhancing studies of the connectome in autism using the autism brain imaging data exchange II. Sci Data. (2017) 4:1–15. doi: 10.1038/sdata.2017.10
Keywords: autism spectrum disorder, resting state, inter-hemispheric functional connectivity, homotopic connectivity, corpus callosum, social deficits, biomarker
Citation: Yao S, Becker B and Kendrick KM (2021) Reduced Inter-hemispheric Resting State Functional Connectivity and Its Association With Social Deficits in Autism. Front. Psychiatry 12:629870. doi: 10.3389/fpsyt.2021.629870
Received: 16 November 2020; Accepted: 11 February 2021;
Published: 04 March 2021.
Edited by:
Asma Bouden, Tunis El Manar University, TunisiaCopyright © 2021 Yao, Becker and Kendrick. This is an open-access article distributed under the terms of the Creative Commons Attribution License (CC BY). The use, distribution or reproduction in other forums is permitted, provided the original author(s) and the copyright owner(s) are credited and that the original publication in this journal is cited, in accordance with accepted academic practice. No use, distribution or reproduction is permitted which does not comply with these terms.
*Correspondence: Shuxia Yao, yaoshuxia@uestc.edu.cn; Keith M. Kendrick, k.kendrick.uestc@gmail.com