- 1The National Clinical Research Center for Mental Disorders and Beijing Key Laboratory of Mental Disorders and Beijing Institute for Brain Disorders Center of Schizophrenia, Beijing Anding Hospital, Capital Medical University, Beijing, China
- 2Advanced Innovation Center for Human Brain Protection, Capital Medical University, Beijing, China
- 3Department of Psychiatry, University of North Carolina at Chapel Hill, Chapel Hill, NC, United States
Dopamine-β-hydroxylase (DβH) is an enzyme converting dopamine to norepinephrine, a key neurotransmitter in mood disorders, such as major depressive disorder (MDD) and bipolar disorder (BD). Due to overlapping symptomology of unipolar and bipolar depression, the present study attempted to explorer if the plasma DβH activity could discriminate the depressive episodes of BD from MDD. The aim of this study was to compare the plasma DβH activity among MDD patients (n = 104), BD patients (n = 101), and healthy controls (n = 160). Clinical characteristics and cognitive function were assessed using the Young Mania Rating Scale (YMRS), Hamilton Depression Scale (HAM-D), Hamilton Anxiety Scale (HAM-A), Patient Health Questionnaire-9 (PHQ-9), and Repeatable Battery for the Assessment of Neuropsychological Status (RBANS). Our data showed a lower plasma DβH activity in patients with BD, not MDD, than that in controls. For the BD patients, the plasma DβH activities were negatively correlated with HAM-D scores and HAM-A scores. However, there was no significant correlation between plasma DβH activity and severity of depressive symptoms in MDD patients. No significant correlation between DβH activities and cognitive assessments neither in BD nor in MDD patients. The present study provides evidence that BD is associated with decreased circulating DβH activity.
Introduction
Bipolar disorder (BD) is a complex and chronic psychiatric disease, with a prevalence to range from 0.5 to 5% in community-based samples (1, 2). BD is a disabling disease due to its early onset, severity and chronic nature although relatively rare (3). The important character of BD is the alternative episodes of mania and depression. However, major depressive disorder (MDD) and BD at depressive phase are similar in clinical manifestations, their overlapping symptomology makes difficult to differentiate BD at depressive phase from MDD (4). More importantly, the use of antidepressants in BD patients have limited efficacy even might increase the possible switch to manic episodes (5). Therefore, it is important to find biomarker to differentiate BD at depressive phase from MDD, thereby to improve the therapeutic effect on mood disorders.
Unfortunately, the underlying mechanisms of BD and MDD have not yet been fully elucidated, while the monoaminergic theory has been regarded as the main cause of both MDD and BD (6–8). According to the monoamine hypothesis, monoamine neurotransmitters, including serotonin (5-HT), dopamine (DA), and norepinephrine (NE), have always been the key aspects in mood disorders. Although previous genetic, biological and pharmacological studies confirmed the important role of 5-HT in MDD (7, 9), accumulating evidence suggested the contribution of dysregulated DA and NE transmission on both MDD and BD (10–12). DA and NE play an important role not only in cognitive function, but also in emotion regulation (13, 14). In general, depression was associated with reduced activity in the dopaminergic signaling system (15), whereas mania was associated with increased dopaminergic signaling (16). For example, depressive symptoms have been linked with hypodopaminergic transmission in the reward system (17), and dopaminergic stimulant agents augment the efficacy of antidepressants (18). On the other hand, hyperdopaminergic mice showed mania-like behaviors (19), and changed levels of 3-methoxy-4-hydroxyphenylglycol (MHPG, metabolites of NE) and homovanillic acid (HVA, metabolites of DA) were found in BD patients compared to controls (10, 20). Thus, the different changes of DA and NE transmission may contribute to explore the mechanism of MDD and BD.
Dopamine β-hydroxylase (DβH) is the key regulatory enzyme required to synthesize NE from DA (21), and it is also important to maintain the brain DA/NE balance. DβH is located in both central (catecholamine vesicles) and peripheral systems (sympathetic nerves and adrenal medulla) (22, 23). Since DβH can be released from catecholamine vesicles, the protein can be determined in the plasma or serum (21). Previous studies with mice deficient in DβH demonstrated the role of DβH in mood disorders. The birth rate of DBH gene knockout mice was much lower than wild-type mice, and the surviving mice almost died in the first week of life (24), suggesting the important role of DβH in development and survival. Considering the important effect of NE and DA in cognition, several evidences indicated that DβH might play a role in the cognitive defects in mental disorders (25–29), although DβH-deficient patients failed to display neurocognitive impairment (30, 31).
Abundant evidence has shown that low serum/plasma DβH activity might be a risk factor for mental illness (21, 25, 27, 32). Furthermore, the genetic, biological and pharmacological studies indicated the important role of DβH in BD and MDD. The DBH gene variants were proved to join in predicting individual differences in social and affective processing (33). Ates et al. indicated the mutated DBH gene increased the risk of BD (34). In addition, the serum DβH activity was significant decreased in BD patients in depressive state compared with MDD patients (35), while it was lower in MDD patients than that in healthy controls (36–40). Interestingly, compared with drug-naive BD patients, serum DβH activity was higher in lithium-treated BD patients (41). However, other studies showed the contrary results (42, 43). Animal studies indicated stress increased expression of DβH mRNA and protein in mood-related areas in the brain (44, 45). The contrary studies suggested the complex role of DβH in patients with mood disorders, even between MDD patients and BD patients in depressive state.
Due to overlapping symptomology of unipolar and bipolar depression, the present study attempted to explorer if the plasma DβH activity could discriminate the depressive episodes of BD from MDD. The aim of this study was to investigate whether plasma DβH activity differs between: (i) BD patients and healthy controls (HCs), (ii) MDD patients and HCs, (iii) BD patients and MDD patients. The plasma DβH activity was tested in individuals with three groups: BD patients, MDD patients and HCs. Furthermore, the associations of DβH activity and phenotypes of patients were also assessed in this study.
Materials and Methods
Subjects
Subjects were recruited from Beijing Anding Hospital (China) from September 2014 to September 2016. The ethics committee of Beijing Anding Hospital approved the research. All of the individuals provided written informed consent to participate in the present study after fully explaining the purpose and procedure. Male and female patients aged 16 to 60 years who met the Diagnostic and Statistical Manual of Mental Disorders, Fourth Edition (DSM-IV) criteria for MDD, or BD were recruited. All patients were screened using the Structured Clinical Interview for DSM-IV Axis I disorders-Patient Edition (SCID) by experienced psychiatrists. In the present study, all the patients with MDD were undergone depressive episodes, while the BD patients also with a current depressive episode were recruited.
The inclusion criteria of all patients were as follows: (1) aged 16 to 60 years; (2) formal education ≥9 years; (3) total scores of Young Mania Rating Scale (YMRS) ≤ 6. The exclusion criteria of all patients were as follows: (1) comorbidity with other psychiatric disorders, such as schizophrenia; (2) electric convulsive therapy in recent 3 months; (3) were or had a history of substance dependence; (4) severe suicidal tendencies; (5) severe physical diseases, such as neurological diseases, cardiovascular disease, hepatic or renal diseases; (6) current pregnancy or breastfeeding.
The present study recruited gender-matched HC individuals with no history of psychosis or cognitive impairment. HCs underwent a psychiatric interview by experienced clinicians using the SCID to exclude psychiatric disorders. HCs were excluded when encounter the following situations: (1) had any lifetime DSM-IV psychiatric disorder; (2) had severe physical diseases, such as neurological diseases, cardiovascular disease, hepatic or renal diseases; (3) had a family history of psychiatric diseases; (4) were or had a history of substance dependence; (5) were current pregnancy or breastfeeding.
Clinical Assessments
Clinical assessments were administrated by experienced psychiatrists, who were blinded to participants. The patients were assessed with several clinical scales, including the Young Mania Rating Scale (YMRS) (46), Hamilton Depression Scale (HAM-D) (47), Hamilton Anxiety Scale (HAM-A) (47), and Patient Health Questionnaire-9 (PHQ-9) (48). In addition, the cognitive function of each participant was assessed with Wechsler Adult Intelligence Scale (brief form), Stroop's color-word test (49), and Repeatable Battery for the Assessment of Neuropsychological Status (RBANS) (50). Five aspects of the RBANS were evaluated, including attention, speech, visual span, immediate memory, and delayed memory.
Plasma DβH Activity Assay
Peripheral blood from each individual was collected in this study. Plasma was harvested and stored at −80 °C until they were used for the detection of DβH activity. The DβH activity was detected with the method reported from our laboratory (25), which was based on the enzymatic conversion of tyramine (substrate) to octopamine by DβH. Briefly, the reaction mixture (100 μL) was composed of 1 M CH3COONa buffer (pH = 5.0), 0.2 M sodium fumarate, 0.2 M ascorbic acid, 1,500 U catalase, 0.2 M tyramine hydrochloride, 0.02 M pargyline, 0.2 M N-ethylmaleimide, pure water and plasma (5 μL). After being incubated at 37°C for 1 h, the reaction was stopped by adding 20% HClO4 (20 μL). The supernatant was transferred to high performance liquid chromatography (HPLC) system after centrifugation (2,000 rpm) for 10 min. Octopamine concentration was measured by column-switching, reverse phase HPLC system (U3000, Thermo Fisher Scientific, Waltham, MA, USA), with electrochemical detection. Electrodes 1 and 2 of the cell were set at +700 and −320 mV, respectively. Synephrine was used as internal standard. Octopamine and synephrine were separated by a 5 μm particle size reversed-phase SB-C18 analytical column (2.1 × 150 mm) obtained from Agilent Technologies (Santa Clara, CA, USA). The mobile phase was composed of 50.9 mM potassium acetate, 14.3 mM citric acid, 1.38 mM 1-octanesulfonic acid, and 9.5% methanol (v/v). All chemicals were purchased from Sigma-Aldrich (St. Louis, MO, USA).
Statistical Analysis
The data were analyzed using SPSS with version 20.0 (SPSS Inc., Chicago, IL, USA). Demographic and clinical characteristics were compared among three groups using χ2-tests or one-way analysis of variance (ANOVA). Consideration of the significant difference in age, the covariance analysis was used to compare the continuous variables, including plasma DβH activities among groups with age as a covariate. On the other hand, the multivariate logistic regression analysis was used to evaluate the associations between discontinuous variable, including sex, family history, current state of illness, and mood disorders susceptibility with adjustment for age. Two-way ANOVA was used to evaluate the interaction effects between groups and gender, or compare the plasma DβH activity in patients with different episodes. The comparison of plasma DβH activity in patients with different kinds of drugs was also used with two-way ANOVA. In order to minimize the bias of demographic data on the analysis, the partial correlation was used to analyze the association of DβH activity and clinical and cognitive assessments, with age, education, duration of illness and first onset age as covariates. The level of significance was set at p < 0.05. However, the corrected p-value was set at 0.0167 considering the multiple testing of cognitive functions of the patients in the partial correlation analysis.
Results
Demographic and Clinical Characteristics
A total of 389 individuals were initially screened for this study, and 24 (14 in MDD group, six in BD group, and four in control group) were excluded due to the declining to participate, not drawing blood or not meeting the inclusion criteria. Ultimately, the study population comprised of 104 MDD patients, 101 BD patients and 160 HCs. Although more than 90% patients completed all the clinical and cognitive assessments, only 116 HCs completed all the clinical assessments. The comparison of demographics and clinical characters among these three groups is shown in Table 1. Notably, there was a significant difference in age among groups (F = 6.795, p = 0.001). Significant differences in the YMRS, HAM-D, and HAM-A total scores were found among three groups. More concretely, the HAM-D, HAM-A, and PHQ-9 scores were significantly increased in MDD or BD patients compared to HCs. However, the YMRS scores were higher in BD patients than MDD patients or HCs. Furthermore, these differences still exist after covariance analysis controlling for age (Table 1). In addition, according to the assessments of intelligence quotient (IQ), RBANS, and Stroop's tests, the MDD or BD patients showed significant deficits in cognitive function.
Plasma DβH Activity
The average plasma DβH activity in each group was shown in Figure 1 (the value was 17.31 ± 11.85 in HCs, 15.77 ± 11.19 in MDD patients, 13.49 ± 7.56 in BD patients). Covariance analysis (controlling for age) showed a significant decrease in plasma DβH activity in BD patients compared to HCs (p = 0.005). However, there was no significant difference in DβH activity between MDD patients and HCs (p = 0.634). It should be noted that no significant difference in plasma DβH activity was found between BD and MDD patients (p = 0.245).
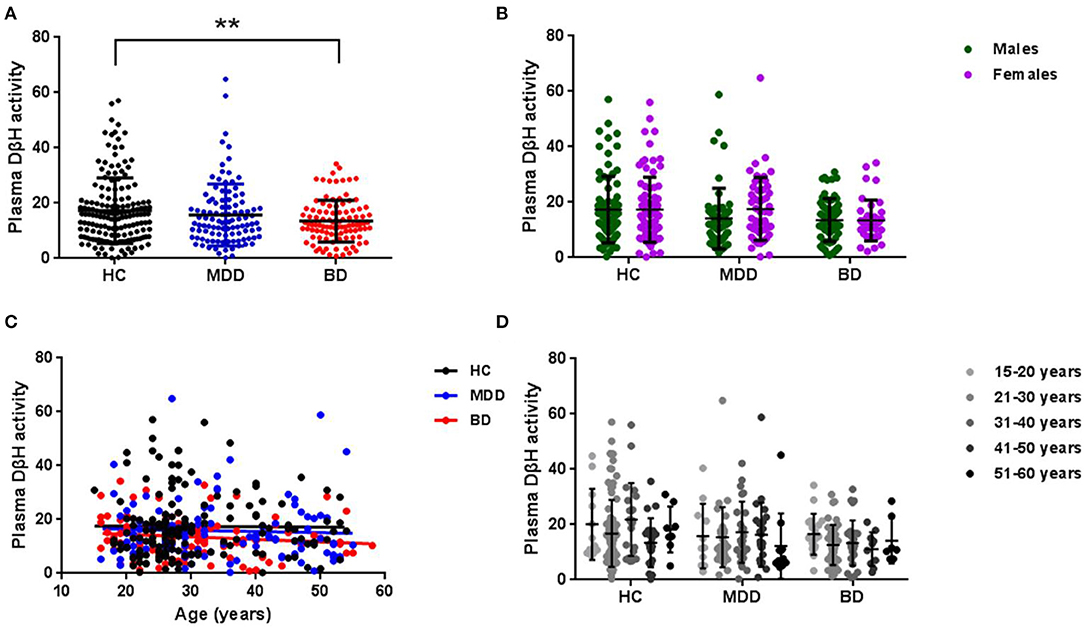
Figure 1. Plasma DβH activity in patients with MDD or BD, and healthy controls (HC). (A) Compared the plasma DβH activity in HC (n = 160), MDD patients (n = 104), and BD patients (n = 101). (B) Showed the sex difference of DβH activity among HC (n = 80 in males; n = 80 in females), MDD patients (n = 54 in males; n = 50 in females), and BD patients (n = 62 in males; n = 39 in females). (C,D) Showed the age-dependent changes of DβH activity in each group. Data was presented as mean ± S.D. **p < 0.01.
In addition, we also analyzed the sex difference in plasma DβH activity among three groups. No significant difference in plasma DβH activity was found between males and females in these three groups (Figure 1B, F = 0.830, p = 0.363). Figures 1C,D showed there was no significant association in plasma DβH activities with age in each group (all p > 0.05).
In order to exclude confounding factors, the patients were divided into first-episode and multi-episode patients (Figure 2). Only 10% patients (n = 10) were in first-episode in BD, while this ratio was 38% (n = 40) in MDD. Though there was no significant difference in plasma DβH activity between first-episode and multi-episode patients, the multi-episode patients showed a decrease trend compared with first-episode patients in both MDD (14.45 ± 8.96 and 17.87 ± 13.91, respectively) and BD (13.32 ± 7.35 and 15.10 ± 9.66, respectively).
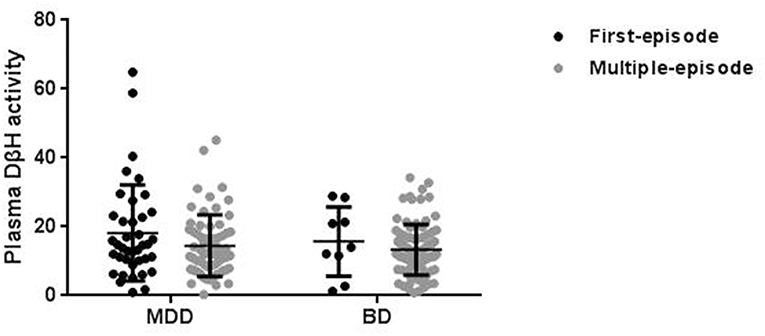
Figure 2. Plasma DβH activity in patients with different episodes, including first-episode (FE) and multi-episode (ME) in MDD and BD. There was no significant difference in DβH activity in patients with different episodes in neither MDD (n = 40 in FE; n = 64 in ME) nor BD (n = 10 in FE; n = 91 in ME) groups. Data was presented as mean ± S.D.
Associations of Plasma DβH Activity and Clinical Variables
No significant associations were found between DβH activities and clinical assessments or cognitive function in MDD patients (Table 2, all p > 0.05). However, significant negative correlations were found between DβH activities and HAM-D scores (r = −0.234, p = 0.021), or HAM-A scores (r = −0.201, p = 0.041) in BD patients (Table 3, Figure 3). Nevertheless, plasma DβH activities showed no significant correlation with cognitive assessments in BD patients (all p > 0.0167).
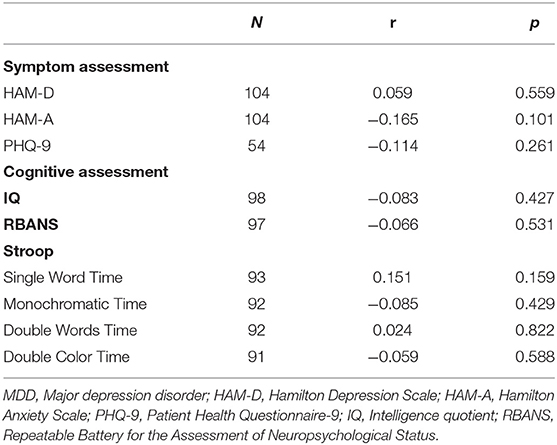
Table 2. Associations between plasma DβH activity and clinical or cognitive assessments in patients with MDD.
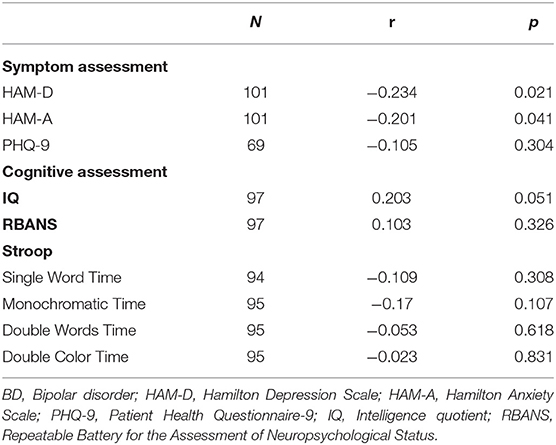
Table 3. Associations between plasma DβH activity and clinical or cognitive assessments in patients with BD.
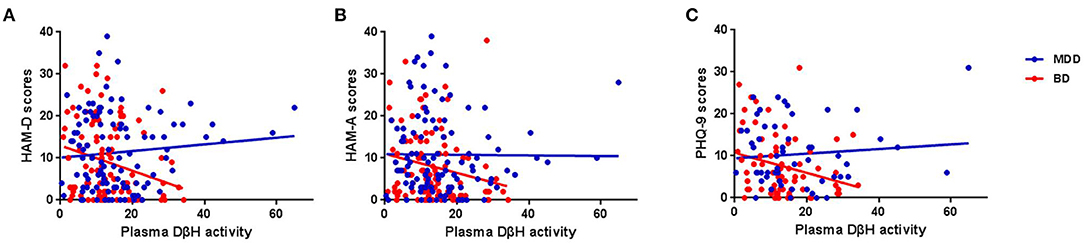
Figure 3. Associations between plasma DβH activity and clinical assessments in MDD and BD patients. (A–C) Showed the associations between plasma DβH activity and Hamilton Depression Scale (HAM-D) scores, Hamilton Anxiety Scale (HAM-A) scores, and Patient Health Questionnaire-9 (PHQ-9) scores, respectively. Significant negative correlations were found between DβH activities and HAM-D scores (r = −0.234, p = 0.021), or HAM-A scores (r = −0.201, p = 0.041) in BD patients. However, no significant correlation was found between plasma DβH activity and clinical assessments in MDD patients (all p > 0.05).
Discussion
In this study, we enrolled 365 subjects, including 104 MDD patients, 101 BD patients, and 160 HCs, to complete the plasma DβH activity assay and clinical assessments. Five findings were obtained in our study: (1) Patients with BD, not MDD, showed a significant decrease in plasma DβH activities compared with HCs; (2) No significant differences in plasma DβH activities were found between the BD and MDD patients; (3) Significant negative correlations were found between DβH activity and mood-related assessments in BD patients; (4) There was no correlation between DβH activity and cognitive function in BD; (5) In contrast to BD patients, no correlations were found between DβH activity and clinical symptoms or cognition in MDD patients.
In the present study, we found that the plasma DβH activity was significantly lower in BD patients; this was in line with the previous studies in BD (37, 41). It is interesting to note that we also found no significant difference in plasma DβH activity between BD and MDD patients. This may be due to the similarity of biological mechanisms and phenotypes between BD and MDD (30, 33). In addition, the DβH product, NE also showed similar changes in BD and MDD (20, 51–53). For example, NE was used as a stress factor to induced depression in several studies (54, 55), while stress was also a major risk factor of BD (56). Interestingly, there is report showed CSF NE concentration increased while DA levels decreased in rats after stress (57). The decrease of DβH activity may be one of the reasons for the alteration in NE/DA imbalance. The studies of animal models of depression or mania proved the changes in NE system (1, 3, 58–61). Furthermore, the NE metabolism disruption also might be caused by the reduced DβH in BD. The metabolite of NE, MHPG in the circulating system was found higher in BD patients than controls (4, 35), but contrary results were also reported in several studies (10, 20). Interestingly, neuromodulation treatment on mood disorders was also associated with up-regulation of NE system or DβH expression (45, 62, 63). The inconstant results in plasma DβH activity and NE levels in MDD or BD indicated the complex role of DβH in mood disorders. One of the possible reasons for the contrary results might be the difference in detected tissues, such as the blood or the CSF; another reason might be different episodes with the BD patients, such as the manic or the remission state. In addition, the antidepressants, especially NE reuptake inhibitors improved the depressive symptoms directly through regulation of NE levels (64, 65). Together, the present study showed a decrease of plasma DβH activity in BD patients, which may result in the reduced levels of NE to induced the symptoms of patients. Regrettably, plasma levels of NE were not detected in our study.
The main factor affecting DβH activity is heredity. Numerous studies indicate that DBH gene is a major quantitative trait locus that regulates blood and CSF DβH activity (25, 66). Previous studies have reported several single nucleotide polymorphisms (SNPs) which correlate with plasma DβH activity (21, 66–68). For example, a previous study reported −1021C>T (rs1611115) accounted for 35–52% of the variation in DβH activity in African American, European American and Japanese (69); while our previous study showed the ratio was 12.6% in Chinese (25). However, few studies explored the association between DBH gene polymorphisms and mood disorders. For example, one study showed that the 1603C>T polymorphism of the DBH gene is associated with susceptibility to BD in a Turkish population (34). Zhou et al. reported that DBH 5′- Ins/Del polymorphism might be associated with susceptibility to MDD in a Chinese population (70). These results indicated the regulated role of DBH gene mutation in DβH activity and its association with mood disorders (71).
Though evidence suggested low NE concentration was associated with MDD and BD, several studies showed that NE levels were different in patients between MDD and BD. It is thought that high levels of NE resulted in mania, while low levels of NE led to depression (72). Wiste et al. compared the tyrosine hydroxylase (TH, the key enzyme of DA synthesis) immunoreactive cells in locus coeruleus (LC) among different subjects (73), and found that the TH immunoreactive cells in LC in BD patients were about half of those in controls or MDD patients, suggesting the lower NE transmission in BD. In addition, neuronal damage in LC also emerged in BD, not MDD (74). However, no significant difference in plasma DβH activity was found in our study. This result should be further confirmed due to several confusion factors in the present study. First, only 10% patients (n = 10) were in first-episode in BD, while this ratio was 38% (n = 40) in MDD in our study. Compared with first-episode patients, multi-episode patients showed decrease trend in both BD and MDD, suggesting the difference in DβH activity might relate to the duration of disease. Second, several studies indicated the influence of antidepressants and mood stabilizers on DβH activity (41, 75–77). In our study, the patients were treated with different psychotropics, including antidepressants (escitalopram, duloxetine), antipsychotics (olanzapine, aripiprazole and quetiapine), and mood stabilizers (lithium and valproic acid). For example, MDD patients were treated with different drugs (76% antidepressants, 19% antipsychotics and 3% mood stabilizers), meanwhile, BD patients were also treated with different kinds of drugs (70% mood stabilizers, 62% antipsychotics, and 27% antidepressants). However, our study did not show the significant difference in patients treated with different kinds of psychotropics (Supplementary Figure 1, p > 0.05). Previous studies indicated that the antidepressive effect of mood stabilizers and antidepressants might be partly mediated by DβH and NE system (75, 78). Moreover, the DβH activity was normalized during antidepressant therapy or mood stabilizers treatment (40, 41, 79). However, our present study showed similar plasma DβH activities in patients with different drug treatment groups, suggesting the similar regulation effect of different kinds of drugs. On the other hand, this inconsistency might also relate to the relative small sample size of patients and different medication duration (2–85 months).
To our knowledge, we firstly reported that plasma DβH activity was associated with anxiety and depressive symptoms in BD. Previous studies showed low DβH activity in CSF and serum in patients with mood disorders (37), and lower plasma DβH activity in untreated patients with BD was found than that in controls and lithium-treated patients (41). However, no reports have shown a clear correlation between plasma/serum DβH activity and severity of mood disorders. We found significant negative correlations between plasma DβH activity and anxious and depressive symptoms in BD. In other words, lower DβH activity is associated with more severe anxious or depressive symptoms in BD. This confirmed the important role of plasma DβH activity and monoamine neurotransmitter system in BD. However, no significant correlations were found between plasma DβH activity and mania severity (YMRS scores) in our study. This might relate to the current state of the BD patients when recruited in the present study. About 57% BD patients recruited in our study were in the remission stage, and the others were in the depressive stage (Table 1). It was a great pity that there was no BD patients in the manic state, while the YMRS scores were 3.27 (SD = 6.02). Further study should be conducted in the BD patients with manic state.
However, several limitations should be noted in this study. First, this study focused on the DβH activity in MDD and BD. However, other factors might also affect the dopaminergic transmission, such as polymorphisms of dopaminergic related genes as an endophenotype of MDD. Second, the three study groups, including patients with MDD or BD and HCs, were not fully age-matched. These variables were corrected in the analysis. Third, a majority of patients were receiving antipsychotics and antidepressants, which have confounding effect to explore the role of DβH in mood disorders. Finally, only 10% patients were in first-episode in BD, while the ratio was 38% in MDD.
Taken together, this is a pilot study and shows a reduction of plasma DβH activity as well as hypoactivity of the noradrenergic system in patients with BD. The plasma DβH activity is here proposed as a measure to evaluate the severity of BD.
Data Availability Statement
The raw data supporting the conclusions of this article will be made available by the authors, without undue reservation.
Ethics Statement
The studies involving human participants were reviewed and approved by The ethics committee of Beijing Anding Hospital approved the research. Written informed consent to participate in this study was provided by the participants' legal guardian/next of kin.
Author Contributions
CW, ZS, and QB obtained funding for this study. ZS and QB designed the research. ZS, QB, ZM, FL, WL, FH, and XM performed the experiments and statistical analysis. ZS, QB, YH, and CP wrote the manuscript. All authors contributed to the article and approved the submitted version.
Funding
This work was supported by Beijing Municipal Administration of Hospitals Clinical Medicine Development of Special Funding Support (ZYLX201807), National Natural Science Foundation of China (81801339), Beijing Science and Technology Commission (D121100005012002), Beijing Municipal Organization Department talents project (2016000021469G192), and Beijing Biobank of Clinical Resources-Mental Disorders (D131100005313011). No investigator benefited from participating in the study.
Conflict of Interest
The authors declare that the research was conducted in the absence of any commercial or financial relationships that could be construed as a potential conflict of interest.
Acknowledgments
The authors are grateful to all study participants in this study.
Supplementary Material
The Supplementary Material for this article can be found online at: https://www.frontiersin.org/articles/10.3389/fpsyt.2021.566091/full#supplementary-material
Supplementary Figure 1. Differences of plasma DβH activity in patients treated with different kinds of psychotropics. Data was presented as mean ± S.D.
References
1. Charlson F, van Ommeren M, Flaxman A, Cornett J, Whiteford H, Saxena S. New WHO prevalence estimates of mental disorders in conflict settings: a systematic review and meta-analysis. Lancet. (2019) 394:240–8. doi: 10.1016/S0140-6736(19)30934-1
2. Merikangas KR, Jin R, He JP, Kessler RC, Lee S, Sampson NA, et al. Prevalence and correlates of bipolar spectrum disorder in the world mental health survey initiative. Arch Gen Psychiatry. (2011) 68:241–51. doi: 10.1001/archgenpsychiatry.2011.12
3. Ferrari AJ, Stockings E, Khoo JP, Erskine HE, Degenhardt L, Vos T, et al. The prevalence and burden of bipolar disorder: findings from the Global Burden of Disease Study 2013. Bipolar Disord. (2016) 18:440–50. doi: 10.1111/bdi.12423
4. Grande I, Berk M, Birmaher B, Vieta E. Bipolar disorder. Lancet. (2016) 387:1561–72. doi: 10.1016/S0140-6736(15)00241-X
5. Fornaro M, Anastasia A, Novello S, Fusco A, Solmi M, Monaco F, et al. Incidence, prevalence and clinical correlates of antidepressant-emergent mania in bipolar depression: a systematic review and meta-analysis. Bipolar Disord. (2018) 20:195–227. doi: 10.1111/bdi.12612
6. Schildkraut JJ. The catecholamine hypothesis of affective disorders: a review of supporting evidence. Am J Psychiatry. (1965) 122:509–22. doi: 10.1176/ajp.122.5.509
7. Perez-Caballero L, Torres-Sanchez S, Romero-Lopez-Alberca C, Gonzalez-Saiz F, Mico JA, Berrocoso E. Monoaminergic system and depression. Cell Tissue Res. (2019) 377:107–13. doi: 10.1007/s00441-018-2978-8
8. Ashok AH, Marques TR, Jauhar S, Nour MM, Goodwin GM, Young AH, et al. The dopamine hypothesis of bipolar affective disorder: the state of the art and implications for treatment. Mol Psychiatry. (2017) 22:666–79. doi: 10.1038/mp.2017.16
9. Kraus C, Castren E, Kasper S, Lanzenberger R. Serotonin and neuroplasticity - links between molecular, functional and structural pathophysiology in depression. Neurosci Biobehav Rev. (2017) 77:317–26. doi: 10.1016/j.neubiorev.2017.03.007
10. Kurita M, Nishino S, Numata Y, Okubo Y, Sato T. The noradrenaline metabolite MHPG is a candidate biomarker from the manic to the remission state in bipolar disorder I: a clinical naturalistic study. PLoS ONE. (2014) 9:e100634. doi: 10.1371/journal.pone.0100634
11. Belujon P, Grace AA. Dopamine system dysregulation in major depressive disorders. Int J Neuropsychopharmacol. (2017) 20:1036–46. doi: 10.1093/ijnp/pyx056
12. Nikolaus S, Mamlins E, Hautzel H, Muller HW. Acute anxiety disorder, major depressive disorder, bipolar disorder and schizophrenia are related to different patterns of nigrostriatal and mesolimbic dopamine dysfunction. Rev Neurosci. (2019) 30:381–426. doi: 10.1515/revneuro-2018-0037
13. Schwarz LA, Luo L. Organization of the locus coeruleus-norepinephrine system. Curr Biol. (2015) 25:R1051–6. doi: 10.1016/j.cub.2015.09.039
14. Cools R, Frobose M, Aarts E, Hofmans L. Dopamine and the motivation of cognitive control. Handb Clin Neurol. (2019) 163:123–43. doi: 10.1016/B978-0-12-804281-6.00007-0
15. Palucha-Poniewiera A, Podkowa K, Lenda T, Pilc A. The involvement of monoaminergic neurotransmission in the antidepressant-like action of scopolamine in the tail suspension test. Prog Neuropsychopharmacol Biol Psychiatry. (2017) 79(Pt B):155–61. doi: 10.1016/j.pnpbp.2017.06.022
16. Milienne-Petiot M, Geyer MA, Arnt J, Young JW. Brexpiprazole reduces hyperactivity, impulsivity, and risk-preference behavior in mice with dopamine transporter knockdown-a model of mania. Psychopharmacology (Berl). (2017) 234:1017–28. doi: 10.1007/s00213-017-4543-7
17. Clery-Melin ML, Jollant F, Gorwood P. Reward systems and cognitions in Major Depressive Disorder. CNS Spectr. (2019) 24:64–77. doi: 10.1017/S1092852918001335
18. Yan T, Greene M, Chang E, Houle CR, Tarbox MH, Broder MS. Impact of atypical antipsychotics as adjunctive therapy on psychiatric cost and utilization in patients with major depressive disorder. Clinicoecon Outcomes Res. (2020) 12:81–9. doi: 10.2147/CEOR.S231824
19. Kwiatkowski MA, Hellemann G, Sugar CA, Cope ZA, Minassian A, Perry W, et al. Dopamine transporter knockdown mice in the behavioral pattern monitor: a robust, reproducible model for mania-relevant behaviors. Pharmacol Biochem Behav. (2019) 178:42–50. doi: 10.1016/j.pbb.2017.12.007
20. Palsson E, Sellgren C, Ryden E, Kizza R, Pelanis A, Zetterberg H, et al. Cerebrospinal fluid monoamine metabolite profiles in bipolar disorder, ADHD, and controls. J Neural Transm (Vienna). (2017) 124:1135–43. doi: 10.1007/s00702-017-1746-3
21. Gonzalez-Lopez E, Vrana KE. Dopamine beta-hydroxylase and its genetic variants in human health and disease. J Neurochem. (2020) 152:157–81. doi: 10.1111/jnc.14893
22. Catelas DN, Serrao MP, Soares-Da-Silva P. Effects of nepicastat upon dopamine-beta-hydroxylase activity and dopamine and norepinephrine levels in the rat left ventricle, kidney, and adrenal gland. Clin Exp Hypertens. (2020) 42:118–25. doi: 10.1080/10641963.2019.1583245
23. Schmidt K, Bari B, Ralle M, Washington-Hughes C, Muchenditsi A, Maxey E, et al. Localization of the locus coeruleus in the mouse brain. J Vis Exp. (2019) 145. doi: 10.3791/58652
24. Thomas SA, Matsumoto AM, Palmiter RD. Noradrenaline is essential for mouse fetal development. Nature. (1995) 374:643–6. doi: 10.1038/374643a0
25. Sun Z, Ma Y, Li W, He J, Li J, Yang X, et al. Associations between the DBH gene, plasma dopamine beta-hydroxylase activity and cognitive measures in Han Chinese patients with schizophrenia. Schizophr Res. (2018) 193:58–63. doi: 10.1016/j.schres.2017.06.028
26. Shalev N, Vangkilde S, Neville MJ, Tunbridge EM, Nobre AC, Chechlacz M. Dissociable catecholaminergic modulation of visual attention: differential effects of catechol-O-methyltransferase and dopamine beta-hydroxylase genes on visual attention. Neuroscience. (2019) 412:175–89. doi: 10.1016/j.neuroscience.2019.05.068
27. Ji N, Shuai L, Chen Y, Liu L, Li HM, Li ZH, et al. Dopamine beta-hydroxylase gene associates with stroop color-word task performance in Han Chinese children with attention deficit/hyperactivity disorder. Am J Med Genet B Neuropsychiatr Genet. (2011) 156B:730–6. doi: 10.1002/ajmg.b.31215
28. Bensmann W, Zink N, Arning L, Beste C, Stock AK. The presynaptic regulation of dopamine and norepinephrine synthesis has dissociable effects on different kinds of cognitive conflicts. Mol Neurobiol. (2019) 56:8087–100. doi: 10.1007/s12035-019-01664-z
29. Punchaichira TJ, Mukhopadhyay A, Kukshal P, Bhatia T, Deshpande SN, Thelma BK. Association of regulatory variants of dopamine beta-hydroxylase with cognition and tardive dyskinesia in schizophrenia subjects. J Psychopharmacol. (2020) 34:358–69. doi: 10.1177/0269881119895539
30. Jepma M, Deinum J, Asplund CL, Rombouts SA, Tamsma JT, Tjeerdema N, et al. Neurocognitive function in dopamine-beta-hydroxylase deficiency. Neuropsychopharmacology. (2011) 36:1608–19. doi: 10.1038/npp.2011.42
31. Wassenberg T, Deinum J, van Ittersum FJ, Kamsteeg EJ, Pennings M, Verbeek MM, et al. Clinical presentation and long-term follow-up of dopamine beta hydroxylase deficiency. J Inherit Metab Dis. (2020). doi: 10.1002/jimd.12321. [Epub ahead of print].
32. Cubells JF, Sun X, Li W, Bonsall RW, McGrath JA, Avramopoulos D, et al. Linkage analysis of plasma dopamine beta-hydroxylase activity in families of patients with schizophrenia. Hum Genet. (2011) 130:635–43. doi: 10.1007/s00439-011-0989-6
33. Gong P, Liu J, Li S, Zhou X. Dopamine beta-hydroxylase gene modulates individuals' empathic ability. Soc Cogn Affect Neurosci. (2014) 9:1341–5. doi: 10.1093/scan/nst122
34. Ates O, Celikel FC, Taycan SE, Sezer S, Karakus N. Association between 1603C>T polymorphism of DBH gene and bipolar disorder in a Turkish population. Gene. (2013) 519:356–9. doi: 10.1016/j.gene.2013.01.031
35. Strandman E, Wetterberg L, Perris C, Ross SB. Serum dopamine-beta-hydroxylase in affective disorders. Neuropsychobiology. (1978) 4:248–55. doi: 10.1159/000117637
36. Sapru MK, Rao BS, Channabasavanna SM. Serum dopamine-beta-hydroxylase activity in clinical subtypes of depression. Acta Psychiatr Scand. (1989) 80:474–8. doi: 10.1111/j.1600-0447.1989.tb03008.x
37. Meltzer HY, Tong C, Luchins DJ. Serum dopamine-beta-hydroxylase activity and lateral ventricular size in affective disorders and schizophrenia. Biol Psychiatry. (1984) 19:1395–402.
38. Meyers BS, Alexopoulos GS, Kakuma T, Tirumalasetti F, Gabriele M, Alpert S, et al. Decreased dopamine beta-hydroxylase activity in unipolar geriatric delusional depression. Biol Psychiatry. (1999) 45:448–52. doi: 10.1016/S0006-3223(98)00085-7
39. Cubells JF, Price LH, Meyers BS, Anderson GM, Zabetian CP, Alexopoulos GS, et al. Genotype-controlled analysis of plasma dopamine beta-hydroxylase activity in psychotic unipolar major depression. Biol Psychiatry. (2002) 51:358–64. doi: 10.1016/S0006-3223(01)01349-X
40. Paclt I, Koudelova J, Pacltova D, Kopeckova M. Dopamine beta hydroxylase (DBH) plasma activity in childhood mental disorders. Neuro Endocrinol Lett. (2009) 30:604–9.
41. Sofuoglu S, Dogan P, Kose K, Esel E, Basturk M, Oguz H, et al. Changes in platelet monoamine oxidase and plasma dopamine-beta-hydroxylase activities in lithium-treated bipolar patients. Psychiatry Res. (1995) 59:165–70. doi: 10.1016/0165-1781(95)02631-2
42. Matuzas W, Meltzer HY, Uhlenhuth EH, Glass RM, Tong C. Plasma dopamine-beta-hydroxylase in depressed patients. Biol Psychiatry. (1982) 17:1415–24.
43. Markianos ES, Nystrom I, Reichel H, Matussek N. Serum dopamine-beta-hydroxylase in psychiatric patients and normals. Effect of d-amphetamine and haloperidol. Psychopharmacology. (1976) 50:259–67. doi: 10.1007/BF00426842
44. Fan Y, Chen P, Li Y, Cui K, Noel DM, Cummins ED, et al. Corticosterone administration up-regulated expression of norepinephrine transporter and dopamine beta-hydroxylase in rat locus coeruleus and its terminal regions. J Neurochem. (2014) 128:445–58. doi: 10.1111/jnc.12459
45. Stefanovic B, Spasojevic N, Jovanovic P, Dronjak S. Melatonin treatment affects changes in adrenal gene expression of catecholamine biosynthesizing enzymes and norepinephrine transporter in the rat model of chronic stress-induced depression. Can J Physiol Pharmacol. (2019) 97:685–90. doi: 10.1139/cjpp-2018-0612
46. Xiao L, Gao Y, Zhang L, Chen P, Sun X. The relationship between cognitive function and quality of life in euthymic Chinese patients with bipolar disorder. Psychiatry Res. (2016) 246:427–31. doi: 10.1016/j.psychres.2016.10.026
47. Meng G, Ma X, Li L, Tan Y, Liu X, Liu X, et al. Predictors of early-onset post-ischemic stroke depression: a cross-sectional study. BMC Neurol. (2017) 17:199. doi: 10.1186/s12883-017-0980-5
48. Hu A, Xue Z, Mwansisya TE, Zhou A, Pu W, Chen X, et al. Major depressive disorder in hemodialysis patients in China. Asia Pac Psychiatry. (2015) 7:78–84. doi: 10.1111/appy.12110
49. Chung YS, Kang DH, Shin NY, Yoo SY, Kwon JS. Deficit of theory of mind in individuals at ultra-high-risk for schizophrenia. Schizophr Res. (2008) 99:111–8. doi: 10.1016/j.schres.2007.11.012
50. Phillips R, Cheung YB, Collinson SL, Lim ML, Ling A, Feng L, et al. The equivalence and difference between the english and chinese language versions of the repeatable battery for the assessment of neuropsychological status. Clin Neuropsychol. (2015) 29(Suppl. 1):1–18. doi: 10.1080/13854046.2015.1034182
51. Yatham LN, Sossi V, Ding YS, Vafai N, Arumugham SS, Dhanoa T, et al. A positron emission tomography study of norepinephrine transporter occupancy and its correlation with symptom response in depressed patients treated with quetiapine XR. Int J Neuropsychopharmacol. (2018) 21:108–13. doi: 10.1093/ijnp/pyx066
52. Borodovitsyna O, Joshi N, Chandler D. Persistent stress-induced neuroplastic changes in the locus coeruleus/norepinephrine system. Neural Plast. (2018) 2018:1892570. doi: 10.1155/2018/1892570
53. Wang B, Wang Y, Wu Q, Huang HP, Li S. Effects of alpha2A adrenoceptors on norepinephrine secretion from the locus coeruleus during chronic stress-induced depression. Front Neurosci. (2017) 11:243. doi: 10.3389/fnins.2017.00243
54. Gao J, Xi B, Chen K, Song R, Qin T, Xie J, et al. The stress hormone norepinephrine increases the growth and virulence of Aeromonas hydrophila. Microbiologyopen. (2018) 8:e00664. doi: 10.1002/mbo3.664
55. Feng Y, Feng Q, Yin S, Xu X, Song X, Qu H, et al. Stress adaptation disorders play a role in rat gestational diabetes with oxidative stress and glucose transporter-4 expression. Gynecol Endocrinol. (2020) 36:786–90. doi: 10.1080/09513590.2019.1707797
56. Umeoka EHL, van Leeuwen JMC, Vinkers CH, Joels M. The role of stress in bipolar disorder. Curr Top Behav Neurosci. (2020) 48:21–39. doi: 10.1007/7854_2020_151
57. Gu S, Jing L, Li Y, Huang JH, Wang F. Stress induced hormone and neuromodulator changes in menopausal depressive rats. Front Psychiatry. (2018) 9:253. doi: 10.3389/fpsyt.2018.00253
58. Soares-Cunha C, Coimbra B, Borges S, Domingues AV, Silva D, Sousa N, et al. Mild prenatal stress causes emotional and brain structural modifications in rats of both sexes. Front Behav Neurosci. (2018) 12:129. doi: 10.3389/fnbeh.2018.00129
59. Siddique SA, Tamilselvan T, Vishnupriya M, Balamurugan E. Evaluation of neurotransmitter alterations in four distinct brain regions after Rapid Eye Movement Sleep Deprivation (REMSD) induced mania-like behaviour in swiss albino mice. Neurochem Res. (2018) 43:1171–81. doi: 10.1007/s11064-018-2533-8
60. Morilak DA, Barrera G, Echevarria DJ, Garcia AS, Hernandez A, Ma S, et al. Role of brain norepinephrine in the behavioral response to stress. Prog Neuropsychopharmacol Biol Psychiatry. (2005) 29:1214–24. doi: 10.1016/j.pnpbp.2005.08.007
61. Meng L, Bai X, Zheng Y, Chen D, Zheng Y. Altered expression of norepinephrine transporter participate in hypertension and depression through regulated TNF-alpha and IL-6. Clin Exp Hypertens. (2019) 42:181–9. doi: 10.1080/10641963.2019.1601205
62. Ventura-Bort C, Wirkner J, Genheimer H, Wendt J, Hamm AO, Weymar M. Effects of Transcutaneous Vagus Nerve Stimulation (tVNS) on the P300 and alpha-amylase level: a pilot study. Front Hum Neurosci. (2018) 12:202. doi: 10.3389/fnhum.2018.00202
63. Torres-Sanchez S, Perez-Caballero L, Mico JA, Celada P, Berrocoso E. Effect of deep brain stimulation of the ventromedial prefrontal cortex on the noradrenergic system in rats. Brain Stimul. (2018) 11:222–30. doi: 10.1016/j.brs.2017.10.003
64. Ahmadimanesh M, Balarastaghi S, Rashedinia M, Yazdian-Robati R. A systematic review on the genotoxic effect of serotonin and norepinephrine reuptake inhibitors (SNRIs) antidepressants. Psychopharmacology. (2020) 237:1909–15. doi: 10.1007/s00213-020-05550-8
65. Dremencov E, el Mansari M, Blier P. Brain norepinephrine system as a target for antidepressant and mood stabilizing medications. Curr Drug Targets. (2009) 10:1061–8. doi: 10.2174/138945009789735165
66. Tang YL, Epstein MP, Anderson GM, Zabetian CP, Cubells JF. Genotypic and haplotypic associations of the DBH gene with plasma dopamine beta-hydroxylase activity in African Americans. Eur J Hum Genet. (2007) 15:878–83. doi: 10.1038/sj.ejhg.5201838
67. Tang Y, Anderson GM, Zabetian CP, Kohnke MD, Cubells JF. Haplotype-controlled analysis of the association of a non-synonymous single nucleotide polymorphism at DBH (+ 1603C –>T) with plasma dopamine beta-hydroxylase activity. Am J Med Genet B Neuropsychiatr Genet. (2005) 139B:88–90. doi: 10.1002/ajmg.b.30220
68. Gonzalez-Lopez E, Kawasawa-Imamura Y, Zhang L, Huang X, Koltun WA, Coates MD, et al. A single nucleotide polymorphism in dopamine beta hydroxylase (rs6271(C>T)) is over-represented in inflammatory bowel disease patients and reduces circulating enzyme. PLoS ONE. (2019) 14:e0210175. doi: 10.1371/journal.pone.0210175
69. Zabetian CP, Anderson GM, Buxbaum SG, Elston RC, Ichinose H, Nagatsu T, et al. A quantitative-trait analysis of human plasma-dopamine beta-hydroxylase activity: evidence for a major functional polymorphism at the DBH locus. Am J Hum Genet. (2001) 68:515–22. doi: 10.1086/318198
70. Zhou Y, Wang J, He Y, Zhou J, Xi Q, Song X, et al. Association between dopamine beta-hydroxylase 19-bp insertion/deletion polymorphism and major depressive disorder. J Mol Neurosci. (2015) 55:367–71. doi: 10.1007/s12031-014-0339-y
71. Azadmarzabadi E, Haghighatfard A, Mohammadi A. Low resilience to stress is associated with candidate gene expression alterations in the dopaminergic signalling pathway. Psychogeriatrics. (2018) 18:190–201. doi: 10.1111/psyg.12312
72. Maas JW, Huang Y. Noradrenergic function and depression, too much or too little? Can J Neurol Sci. (1980) 7:267–8. doi: 10.1017/S0317167100023337
73. Wiste AK, Arango V, Ellis SP, Mann JJ, Underwood MD. Norepinephrine and serotonin imbalance in the locus coeruleus in bipolar disorder. Bipolar Disord. (2008) 10:349–59. doi: 10.1111/j.1399-5618.2007.00528.x
74. Bielau H, Brisch R, Bernard-Mittelstaedt J, Dobrowolny H, Gos T, Baumann B, et al. Immunohistochemical evidence for impaired nitric oxide signaling of the locus coeruleus in bipolar disorder. Brain Res. (2012) 1459:91–9. doi: 10.1016/j.brainres.2012.04.022
75. Schank JR, Liles LC, Weinshenker D. Reduced anticonvulsant efficacy of valproic acid in dopamine beta-hydroxylase knockout mice. Epilepsy Res. (2005) 65:23–31. doi: 10.1016/j.eplepsyres.2005.03.010
76. Perlis RH, Adams DH, Fijal B, Sutton VK, Farmen M, Breier A, et al. Genetic association study of treatment response with olanzapine/fluoxetine combination or lamotrigine in bipolar I depression. J Clin Psychiatry. (2010) 71:599–605. doi: 10.4088/JCP.08m04632gre
77. Nciri R, Bourogaa E, Jbahi S, Allagui MS, Elfeki A, Vincent C, et al. Chronic neuroprotective effects of low concentration lithium on SH-SY5Y cells: possible involvement of stress proteins and gene expression. Neural Regen Res. (2014) 9:735–40. doi: 10.4103/1673-5374.131578
78. Cryan JF, Dalvi A, Jin SH, Hirsch BR, Lucki I, Thomas SA. Use of dopamine-beta-hydroxylase-deficient mice to determine the role of norepinephrine in the mechanism of action of antidepressant drugs. J Pharmacol Exp Ther. (2001) 298:651–7.
Keywords: dopamine-β-hydroxylase, bipolar disorder, major depressive disorder, mood disorder, cognitive function
Citation: Sun Z, Bo Q, Mao Z, Li F, He F, Pao C, Li W, He Y, Ma X and Wang C (2021) Reduced Plasma Dopamine-β-Hydroxylase Activity Is Associated With the Severity of Bipolar Disorder: A Pilot Study. Front. Psychiatry 12:566091. doi: 10.3389/fpsyt.2021.566091
Received: 27 May 2020; Accepted: 29 March 2021;
Published: 28 April 2021.
Edited by:
Shaohua Hu, Zhejiang University, ChinaReviewed by:
Zhifen Liu, First Hospital of Shanxi Medical University, ChinaRafael Silva, Pontifical Catholic University of Rio de Janeiro, Brazil
Copyright © 2021 Sun, Bo, Mao, Li, He, Pao, Li, He, Ma and Wang. This is an open-access article distributed under the terms of the Creative Commons Attribution License (CC BY). The use, distribution or reproduction in other forums is permitted, provided the original author(s) and the copyright owner(s) are credited and that the original publication in this journal is cited, in accordance with accepted academic practice. No use, distribution or reproduction is permitted which does not comply with these terms.
*Correspondence: Chuanyue Wang, d2N5YWR5eUAxNjMuY29t
†These authors have contributed equally to this work