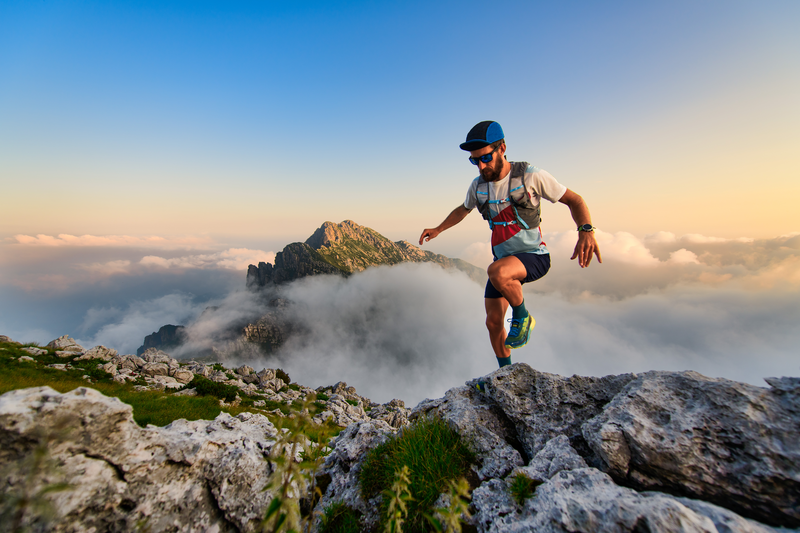
94% of researchers rate our articles as excellent or good
Learn more about the work of our research integrity team to safeguard the quality of each article we publish.
Find out more
PERSPECTIVE article
Front. Psychiatry , 16 July 2021
Sec. Public Mental Health
Volume 12 - 2021 | https://doi.org/10.3389/fpsyt.2021.565633
This article is part of the Research Topic Coronavirus Disease (COVID-19): Psychological, Behavioral, Interpersonal Effects, and Clinical Implications for Health Systems View all 204 articles
Studies investigating the psychosomatic effects of social isolation in animals have shown that one of the physiologic system that gets disrupted by this environment-affective change is the Endocannabinoid System. As the levels of endocannabinoids change in limbic areas and prefrontal cortex during stressful times, so is the subject more prone to fearful and negative thoughts and aggressive behavior. The interplay of social isolation on the hypothalamic-pituitary-adrenal axis and cannabinoid tone triggers a vicious cycle which further impairs the natural body's homeostatic neuroendocrine levels and provokes a series of risk factors for developing health complications. In this paper, we explore the psychosomatic impact of prolonged quarantine in healthy individuals, and propose management and coping strategies that may improve endocannabinoid tone, such as integration of probiotics, cannabidiol, meditation, and physical exercise interventions with the aim of supporting interpersonal, individual, and professional adherence with COVID-19 emergency public measures whilst minimizing their psycho-physical impact.
The current emergency public measures applied to prevent COVID-19 spread have restricted fully or partially people in lockdown cities, with half of humanity directly affected by these changes. (1) Billions of lives have been significantly altered, and a global, multilevel, and demanding stress-coping-adjustment process is ongoing.
Historically, social isolation has been used extensively as a research model for psychosis, suicide, anxiety and depression: all conditions in which the endocannabinoid system is implicated through different pathways, including the modulation of hypothalamic–pituitary–adrenal axis (HPA) function (2–4). Social isolation has been directly linked to increased incidence of suicide (5) and is considered a crucial factor contributing to suicide in humans (6–8). It induces abnormal forms of behavior including an increase in anxiety-and depressive-like activities—such as increase in immobility time, decrease in grooming activity (3), exacerbation of aggressive behavior, and impaired fear extinction (9–11)—effects which directly affect both glucocorticoid and endocannabinoid responses (12).
Although lockdowns are used for social distancing and not to “socially isolate” people, the psychopathologist Baek has introduced the concept of “socially withdrawn” to define those individuals who are isolated because of their extreme involvement in the cyber space and the lack of relationship in real life (13). One may reckon that “real life” and “cyber life” are separated by a fine line when all interpersonal and professional relationships are experienced remotely, as it is currently happening to billions of people worldwide.
Prolonged social distancing has inevitably decreased the physical expressions of affection and social bonding and altered the neuroendocrine balance of neuropeptides (e.g., oxytocin), endocannabinoids (e.g., anandamide), and corticosteroids (e.g., cortisol), key physiologic systems mutually involved not only in maintaining emotional wellbeing but also in the regulation of the immune response, making the implementation of homeostatic strategies targeting those neural pathways even more pivotal during a viral global pandemic (14).
Although the endocannabinoid (eCB) system is expressed ubiquitously in the brain, the highest CB1 receptor density is distributed in key areas for the regulation of stress and emotions: prefrontal cortex (PFC), hippocampus, and amygdala (15).
Previous studies have shown that antagonism or genetic removal of CB1 induces anxiety (16, 17); on the other hand, stress, fear, and negative emotions—as many people are prone to experience since COVID-19 diffusion—may alter the expression of CB1R in the amygdala, nucleus accumbens (reward system), and PFC (18–20). As the PFC is linked to the negative modulation of aggression in animals (21, 22), social isolation may induce a PFC-specific neuronal loss, which is associated with increased levels of aggressive behavior (23).
Social isolation also impairs the hypothalamic eCB system, a brain area also involved in the onset and manifestation of aggressive and fear-related behavior in mammals (24). Studies have demonstrated that the changes in the eCB system may contribute to the display of the stress response, yielding HPA axis stimulation and increased anxiety behavior (25). Indeed, rats that are housed as isolates show an alteration of the basal HPA axis activity and impairments in glucocorticoid-mediated negative feedback (26). These neuroendocrine unbalances are paralleled in human social isolation, with studies showing that cortisol levels are higher in chronically isolated individuals (27–29).
The endocannabinoids anandamide (AEA) and 2-arachidonoyl-glycerol (2-AG) are partial and full agonists at CB1 and CB2 receptors, respectively (30); increasing their levels via deletion or pharmacological inhibition of their metabolic enzyme fatty acid amide hydrolase (FAAH) was shown to reduce anxiety-like behavior (31, 32). An innate decreased anxiety-like conduct and better stress-coping behavior is associated, both in animals and in humans, with a common polymorphism in the FAAH gene and enhanced fronto-amygdala connectivity (33). Conversely, eCB levels are altered following several stressors. AEA is usually decreased, whereas 2-AG is most often increased under stressful conditions (25, 32, 34, 35). Chronic stress engenders a reduction of AEA concentration in the amygdala–hippocampal–cortico-striatal circuit, a feature commonly found in depression and posttraumatic stress disorder (PTSD) (2, 36). In these conditions, low AEA levels are associated with low cortisol levels and an upregulation of CB1 in the brain, effects which seem to be more pronounced in females than in males (37, 38). Gender differences on eCBs support the knowledge that women have a higher risk for anxiety, depression, and PTSD than men (39, 40). In addition, as reports have been showing consistently, during global health crises like COVID-19, women have been reporting higher rates of anxiety and depression than men, which relate with external stressors prevalently targeting women (increase of workload in the healthcare sector, increase of domestic burden, impoverishment, and increase in domestic violence) (41–45).
In short, endogenous cannabinoid signaling is essential for stress adaptation, while chronic stress (e.g., repeated restraint) reduces AEA levels throughout the corticolimbic stress circuit (31, 32).
These data were confirmed by studies examining the effects of social isolation on genes linked with eCB signaling, showing that this stressful condition alters several brain regions implicated in the pathophysiology of schizophrenia and PTSD, both in animals and in humans. CB1 is involved in stress regulation and result altered in several psychiatric disorders—such as anxiety, depression, bipolar disorder, PTSD, schizophrenia, attention deficit hyperactivity disorder, and eating disorders (46, 47). While prefrontal CB2 decreases after repeated stress both in males and in females, social isolation may induce upregulation of CB1R mRNA transcripts in cortical regions and downregulation in the amygdala in a gender-specific pattern due to the interaction between eCBs and sex steroids (48, 49). Females (both adolescent and adults) show higher baseline of CB1 and CB2 mRNA expression levels than males do, which is consistent among animal and human studies, as well as heighted eCB tone disruption to both acute and chronic models of stress (50–52).
Changes also occur in the regulation of eCB mRNA expression in amygdaloid regions, especially AEA, a fact associated with increased anxiety in rats reared in isolation (48, 53, 54). The COVID-19 pandemic has led to a worldwide stressful time with a dual impact: lockdown and fear of disease and death; thus, resilience and appropriate coping strategies become factors of crucial importance to reduce its impact and prevent stress switching into chronic ailments. Neurobiological observations have identified increased firing at the basolateral amygdala (BLA) and prelimbic PFC (plPFC) circuit as one of the key events in the “switch” from distress to psychiatric disorders (55). Recent findings have shown that the neuronal hyper-firing occurring in the BLA-plPFC circuit is due to the stress-induced downregulation of the eCB tone in this region; this induces an increased glutamate release, which, in turn, switches stress exposure into anxious behavior and burn-down (56).
Taken together, these data suggest the need for strategies aimed at not only decreasing stress per se but also helping to restore the eCB homeostasis, which is tightly bound to individual reactions in stressful environments and events (56). See Figure 1 for a summary on the effects of isolation on neurocircuitry and related mental health conditions.
It was shown that the microbiota is tightly connected to emotional behaviors and stress-induced changes (57) and that a perturbation in the microbiota causes functional changes in the eCB tone and behavioral alterations that correlate with depressive states. Therefore, the administration of probiotics may potentially help improve both depressive-like behaviors as well as stimulate the eCB tone, increasing AEA levels (58).
Another approved dietary supplement, N-palmitoylethanolamide (PEA), a fatty acid amide belonging to the eCB system (found increased under stressful conditions), has also shown promising potential antidepressant properties alone or in combination with other classes of antidepressants (59). Preclinical studies have shown its efficacy in depression and depression associated with neuropathic pain and traumatic brain injury models, which could serve the post-COVID symptoms (60). In a translational perspective, a randomized, double-blind study in depressed patients indicated a fast-antidepressant action of PEA when associated with citalopram (59). Several foods may also help modify gut microbial metabolism and glucocorticoid productions (61). One study demonstrated that a daily consumption of 40 g of dark chocolate during a 2-week period was sufficient to reduce the stress hormone cortisol and stress-related impairments (62). Moreover, it was shown that Theobroma cacao contains N-acylethanolamines (N-linoleoylethanolamide and N-oleoylethanolamide) which act as FAAH inhibitors (63–65).
Other food compounds that boost the eCB tone are found in black pepper, both via CB2 direct agonism by one of its sesquiterpenoids, b-caryophyllene, and via AEA uptake inhibition by the pepper's alkaloid guineensine (66, 67). B-Caryophyllene is commonly found in spices and hence called “dietary cannabinoid” and was recently shown to also elicit antidepressant-like activity in isolated and stressed animals via CB2 interaction (68, 69).
Remaining in the “dark colored” food palette, Tuber melanosporum (black truffles) contain biosynthetic enzymes for AEA; the eCB concentration increases up to levels that have been found sufficient to activate CB1 and CB2 as the truffles' pigmentation augments (70).
Fruit and vegetable containing the flavonoid kaempferol are also recommended to balance the eCB tone as it is possible that a high dietary intake of this substance could boost serum AEA levels via FAAH inhibition (71). Kaempferol is commonly found in capers, Kaempferia galanga, saffron, arugula, blackberries, and many other edible plants (72).
Finally, although it is still debated in literature whether a dietary intake would be sufficient to produce CBR-mediated effects, curcumin, the main constituent of turmeric, was nonetheless found to decrease immobility in animal depression models (73, 74).
During lockdown in Canada and a few US States, cannabis was declared an essential service, allowing dispensaries to continue their activity and deliver cannabis products to customers (75). The authors agree that there is a rationale for the use of cannabis during these times. Studies have shown that the depressive-like behaviors induced by social distancing are mitigated by CB receptor activation (3). Moreover, isolation induces a reduction in PFC dendritic dopamine D2 receptors which can be rescued by CB1 stimulation (76). Where cannabis was more available, an increase of its use during lockdown measures was reported, perhaps highlighting an intuitive understanding by consumers for the need to increase the eCB tone (77–81). The increased cannabis use was self-administered, yet not associated with an increase in DSM-5-CCSM total, depression, anxiety, and sleep problem scores in these Countries (78). However, as we recommend strategies that may be applied to the population at large, we would encourage the use of compounds other than tetrahydrocannabinol (THC)-containing cannabis, hence devoid of intoxicating effects. As lockdown poses an increased risk for isolation-induced aggression, the use of cannabidiol (CBD), a cannabis compound with anxiolytic, antidepressant, and antipsychotic properties, could prove useful (82). CBD has been tested in isolated animals, confirming its beneficial effects in attenuating aggressive behavior through a mechanism associated with an increase in AEA levels (via FAAH inhibition) and activation of 5-HT1A and CB1 receptors (83).
Another non-psychotropic plant, Echinacea, can interact with cannabinoid receptors, both directly via alkamide interaction at CB2 and indirectly via AEA reuptake inhibition (84, 85). Echinacea preparations, often used as a home remedy for colds, have been showing to induce an anxiolytic effect in animal models (86).
Physical activity has shown to play an important role in maintaining mental health, decreasing anxiety and alleviating depressive symptoms, and has been hence already recommended as a regular practice during the pandemic to prevent metabolic and immunological dysfunctions (87–89). Interestingly, it also modulates the eCB system balance, a fact suggesting its relevance from both a neurobiological and psychosomatic perspective (90). Indeed, increased eCB concentrations were found following a simple jog, bike ride, hike, and other moderate intensity aerobic exercises, while the differences of FAAH hydrolytic activity in active and sedentary individuals result in different AEA plasma levels (91–94).
Meditation and hypnosis, despite their seemingly different history and conceptualization, may be regarded as two faces of the same coin, sharing several historical, procedural, and neuropsychological aspects (95, 96) as well as their link to the placebo effect (97). Placebo effect involves both endogenous opioids and the eCB system (98). As a whole, the available data on neuropsychological aspects of hypnosis and meditation highlight the relevance of a holistic approach encompassing the inseparable mind–brain–body–environment unit. A wealth of data has shown how hypnosis and meditation may enhance metacognitive control and engender intentional changes of activation/deactivation of unconscious brain areas and circuits leading to outstanding results, one for all hypnotic analgesia (99–102). Both hypnosis and meditation may help develop awareness, mindfulness, and metacognition, restructuring the patient's problem, enhance one's control over mind and body, and manage functional and psychosomatic disorders (95, 103). Therefore, one must establish a bidirectional mind–brain relationship, where brain changes yield mind ones and vice versa. As a result, both pharmacological and nutraceutical interventions may improve brain functions and help decrease symptoms, while behavioral techniques directly modulate the same brain function through cognition, a top-down mind–brain rearrangement.
A broad range of neurological and psychiatric disorders as well as deficits in self-referential processing, such as depersonalization, seem to be related to an altered balance between the default mode network, the salience network, and the central executive network (104). Their complex interplay and connection with other circuits as a dynamic whole is able to update according to demands (105), while their alterations may engender psychiatric disorders. Likewise, traumatic experiences may lead to increased activity of amygdala paralleled by a decreased capacity of anterior cingulate cortex to inhibit it (106).
On a neuropsychological standpoint, both hypnosis and meditation strongly affect the default mode network, a circuit involved in self-referential processing, and the anterior cingulate cortex, which, as mentioned above, may play a central role in dissociative identity disorders and PTSD (107). In doing so, they can help modulate the interrelationship of the abovementioned networks, which are the neuropsychological components of several psychological and psychiatric disorders and favor resilience.
When life adversities and distress are concerned, like those related to COVID-19, resilience plays a key role. Resilience is a complex construct endowed with profound philosophical implications extending to Eastern culture (103); initially coined in physics and engineering, later on it has extended to both biology and psychology. Resilience is the capacity to withstand adversities by keeping the homeostasis, by recovering the initial balance following perturbation or achieve a new balance through allostasis.
At a physiological level, maintenance of homeostasis is the primary function of the eCB system. During stressful times, the eCB tone changes in limbic areas and prefrontal cortex, which makes subjects more prone to a fearful state, negative thoughts, and aggressive behavior.
On a psychological standpoint, resilience is the capacity to withstand or quickly recover from difficult conditions, such as those related to family and social relationships, financial stressors, and workplace and health problems; it is a dynamic process of both biological and psychological adaptation, including emotional and intellectual aspects and their management in the sociocultural and environmental interaction that allows a better adaptation and coping by cognitive improvement and self-transformation, features reflected in the brain through their interplay with neurotransmitter regulation. This is the rationale for presenting different lifestyle interventions and holistic approaches that integrate phytotherapy and nutraceutical agents together with behavioral techniques—like hypnosis and meditation—which are presented with the aim of spreading preventive awareness on maintaining a healthy eCB system, especially during stressful situations like COVID-19. See Figure 2 for a summary of the interventions. A detailed analysis of each of the therapies is far beyond the limit of this article; instead, we discuss first the knowledge drawn from the neurocorrelates of psychological and psychiatric disorders, and then we outline a few essential aspects of their implication in relation to the current state of emergency. We aim to support governments worldwide in using evidence-based suggestions and indications to manage the socioeconomic problems generated by poor mental health.
The original contributions presented in the study are included in the article/supplementary material, further inquiries can be directed to the corresponding author/s.
VB conceived and designed the study, and wrote the first draft of the manuscript. VB and EF wrote sections of the manuscript. All authors contributed to manuscript revision, read, and approved the submitted version.
This study was supported by Dipartimento Neuroscienze, UNIPD VB grant number was 1973.
The authors declare that the research was conducted in the absence of any commercial or financial relationships that could be construed as a potential conflict of interest.
The authors would like to acknowledge the contribution of Andrea Cristofoletto for the image, courtesy of Cannabiscienza Srl.
1. The New York Times. Coronavirus News Update (2020). Available online at: https://www.nytimes.com/2020/04/03/world/coronavirus-news-updates.html#link-290c3c8 (accessed April 22, 2020).
2. Weidenfeld J, Feldman S, Mechoulam R. Effect of the brain constituent anandamide, a cannabinoid receptor agonist, on the hypothalamo-pituitary-adrenal axis in the rat. Neuroendocrinology. (1994) 59:110–2. doi: 10.1159/000126646
3. Haj-Mirzaian A, Amini-Khoei H, Haj-Mirzaian A, Amiri S, Ghesmati M, Zahir M, et al. Activation of cannabinoid receptors elicits antidepressant-like effects in a mouse model of social isolation stress. Brain Res Bull. (2017) 130:200–10. doi: 10.1016/j.brainresbull.2017.01.018
4. Hillard CJ, Weinlander KM, Stuhr KL. Contributions of endocannabinoid signaling to psychiatric disorders in humans: genetic and biochemical evidence. Neuroscience. (2012) 204:207–29. doi: 10.1016/j.neuroscience.2011.11.020
6. Zamora-Kapoor A, Nelson LA, Barbosa-Leiker C, Comtois KA, Walker LR, Buchwald DS. Suicidal ideation in American Indian/Alaska native and white adolescents: the role of social isolation, exposure to suicide, and overweight. Am Indian Alsk Native Ment Health Res. (2016) 23:86–100. doi: 10.5820/aian.2304.2016.86
7. Cornwell EY, Waite LJ. Social disconnectedness, perceived isolation, and health among older adults. J Health Soc Behav. (2009) 50:31–48. doi: 10.1177/002214650905000103
8. Dickens AP, Richards SH, Greaves CJ, Campbell JL. Interventions targeting social isolation in older people: a systematic review. BMC Public Health. (2011) 11:647. doi: 10.1186/1471-2458-11-647
9. Pibiri F, Nelson M, Guidotti A, Costa E, Pinna G. Decreased corticolimbic allopregnanolone expression during social isolation enhances contextual fear: a model relevant for posttraumatic stress disorder. Proc Natl Acad Sci U S A. (2008) 105:5567–72. doi: 10.1073/pnas.0801853105
10. Nelson M, Pinna G. S-norfluoxetine microinfused into the basolateral amygdala increases allopregnanolone levels and reduces aggression in socially isolated mice. Neuropharmacology. (2011) 60:1154–9. doi: 10.1016/j.neuropharm.2010.10.011
11. Pinna G. In a mouse model relevant for post-traumatic stress disorder, selective brain steroidogenic stimulants (SBSS) improve behavioral deficits by normalizing allopregnanolone biosynthesis. Behav Pharmacol. (2010) 21:438–50. doi: 10.1097/FBP.0b013e32833d8ba0
12. Locci A, Pinna G. Social isolation as a promising animal model of PTSD comorbid suicide: neurosteroids and cannabinoids as possible treatment options. Prog Neuropsychopharmacol Biol Psychiatry. (2019) 92:243–59. doi: 10.1016/j.pnpbp.2018.12.014
13. Baek SB. Psychopathology of social isolation. J Exerc Rehabil. (2014) 10:143–7. doi: 10.12965/jer.140132
14. Finn DP. Endocannabinoid-mediated modulation of stress responses: physiological and pathophysiological significance. Immunobiology. (2010) 215:629–46. doi: 10.1016/j.imbio.2009.05.011
15. McPartland JM, Glass M, Pertwee RG. Meta-analysis of cannabinoid ligand binding affinity and receptor distribution: interspecies differences. Br J Pharmacol. (2007) 152:583–93. doi: 10.1038/sj.bjp.0707399
16. Haller J, Bakos N, Szirmay M, Ledent C, Freund TF. The effects of genetic and pharmacological blockade of the CB1 cannabinoid receptor on anxiety. Eur J Neurosci. (2002) 16:1395–8. doi: 10.1046/j.1460-9568.2002.02192.x
17. Marco EM, García-Gutiérrez MS, Bermúdez-Silva FJ, Moreira FA, Guimarães F, Manzanares J, et al. Endocannabinoid system and psychiatry: in search of a neurobiological basis for detrimental and potential therapeutic effects. Front Behav Neurosci. (2011) 5:63. doi: 10.3389/fnbeh.2011.00063
18. Breivogel CS, Sim-Selley LJ. Basic neuroanatomy and neuropharmacology of cannabinoids. Int Rev Psychiatry. (2009) 21:113–21. doi: 10.1080/09540260902782760
19. Herkenham M, Lynn AB, Johnson MR, Melvin LS, de Costa BR, Rice KC. Characterization and localization of cannabinoid receptors in rat brain: a quantitative in vitro autoradiographic study. J Neurosci. (1991) 11:563–83. doi: 10.1523/JNEUROSCI.11-02-00563.1991
20. Pazos MR, Núñez E, Benito C, Tolón RM, Romero J. Functional neuroanatomy of the endocannabinoid system. Pharmacol Biochem Behav. (2005) 81:239–47. doi: 10.1016/j.pbb.2005.01.030
21. Kolb B, Nonneman AJ. Frontolimbic lesions and social behavior in the rat. Physiol Behav. (1974) 13:637–43. doi: 10.1016/0031-9384(74)90234-0
22. Siegel A, Edinger H, Koo A. Suppression of attack behavior in the cat by the prefrontal cortex: role of the mediodorsal thalamic nucleus. Brain Res. (1977) 127:185–90. doi: 10.1016/0006-8993(77)90392-4
23. Biro L, Toth M, Sipos E, Bruzsik B, Tulogdi A, Bendahan S, et al. Structural and functional alterations in the prefrontal cortex after post-weaning social isolation: relationship with species-typical and deviant aggression. Brain Struct Funct. (2017) 222:1861–75. doi: 10.1007/s00429-016-1312-z
24. Toth M, Fuzesi T, Halasz J, Tulogdi A, Haller J. Neural inputs of the hypothalamic “aggression area” in the rat Behav. Brain Res. (2010) 215:7–20. doi: 10.1016/j.bbr.2010.05.050
25. Morena M, Patel S, Bains JS, Hill MN. Neurobiological interactions between stress and the endocannabinoid system. Neuropsychopharmacology. (2016) 41:80–102. doi: 10.1038/npp.2015.166
26. Cacioppo JT, Cacioppo S, Capitanio JP, Cole SW. The neuroendocrinology of social isolation. Annu Rev Psychol. (2015) 66:733–67. doi: 10.1146/annurev-psych-010814-015240
27. Cacioppo JT, Ernst JM, Burleson MH, McClintock MK, Malarkey WB, Hawkley LC, et al. Lonely traits and concomitant physiological processes: the MacArthur social neuroscience studies. Int J Psychophysiol. (2000) 35:143–54. doi: 10.1016/S0167-8760(99)00049-5
28. Pressman SD, Cohen S, Miller GE, Barkin A, Rabin BS, Treanor J, et al. social network size, and immune response to influenza vaccination in college freshmen. Health Psychol. (2005) 24:297–306. doi: 10.1037/0278-6133.24.3.297
29. Steptoe A, Owen N, Kunz-Ebrecht SR, Brydon L. Loneliness and neuroendocrine, cardiovascular, and inflammatory stress responses in middle-aged men and women. Psychoneuroendocrinology. (2004) 29:593–611. doi: 10.1016/S0306-4530(03)00086-6
30. Stella N, Schweitzer P, Piomelli D. A second endogenous cannabinoid that modulates long-term potentiation. Nature. (1997) 388:773–8. doi: 10.1038/42015
31. Hill MN, Kumar SA, Filipski SB, Iverson M, Stuhr KL, Keith JM, et al. Disruption of fatty acid amide hydrolase activity prevents the effects of chronic stress on anxiety and amygdalar microstructure. Mol Psychiatry. (2013) 18:1125–35. doi: 10.1038/mp.2012.90
32. Hill MN, Miller GE, Carrier EJ, Gorzalka BB, Hillard CJ. Circulating endocannabinoids and N-acylethanolamines are differentially regulated in major depression and following exposure to social stress. Psychoneuroendocrinology. (2009) 34:1257–62. doi: 10.1016/j.psyneuen.2009.03.013
33. Dincheva I, Drysdale AT, Hartley CA, Johnson DC, Jing D, King EC, et al. FAAH genetic variation enhances fronto-amygdala function in mouse and human. Nat Commun. (2015) 6:6395. doi: 10.1038/ncomms7395
34. Patel S, Cravatt BF, Hillard CJ. Synergistic interactions between cannabinoids and environmental stress in the activation of the central amygdala. Neuropsychopharmacology. (2005) 30:497–507. doi: 10.1038/sj.npp.1300535
35. Patel S, Kingsley PJ, Mackie K, Marnett LJ, Winder DG. Repeated homotypic stress elevates 2-arachidonoylglycerol levels and enhances short-term endocannabinoid signaling at inhibitory synapses in basolateral amygdala. Neuropsychopharmacology. (2009) 34:2699–709. doi: 10.1038/npp.2009.101
36. Hill MN, Patel S, Carrier EJ, Rademacher DJ, Ormerod BK, Hillard CJ, et al. Downregulation of endocannabinoid signaling in the hippocampus following chronic unpredictable stress. Neuropsychopharmacology. (2005) 30:508–15. doi: 10.1038/sj.npp.1300601
37. Neumeister A, Normandin MD, Pietrzak RH, Piomelli D, Zheng MQ, Gujarro-Anton A, et al. Elevated brain cannabinoid CB1 receptor availability in post-traumatic stress disorder: a positron emission tomography study. Mol Psychiatry. (2013) 18:1034–40. doi: 10.1038/mp.2013.61
38. Hill MN, McLaughlin RJ, Bingham B, Shrestha L, Lee TT, Gray JM, et al. Endogenous cannabinoid signaling is essential for stress adaptation. Proc Natl Acad Sci U S A. (2010) 107:9406–11. doi: 10.1073/pnas.0914661107
39. Yu S. Uncovering the hidden impacts of inequality on mental health: a global study. Transl Psychiatry. (2018) 8:1–10. doi: 10.1038/s41398-018-0148-0
40. Jalnapurkar I, Allen P, igott M. T. Sex differences in anxiety disorders: a review. J Psychiatry Depress Anxiety. (2018) 4:3–16. doi: 10.24966/PDA-0150/100011
41. Thibaut F, van Wijngaarden-Cremers P.. Women's mental health in the time of Covid-19 pandemic. Front Global Women's Health. (2020) 1:17. doi: 10.3389/fgwh.2020.588372
42. UN Women World Health Organization. Violence Against Women and Girls. Data Collection During COVID-19 (2020). Available online at: https://www.unwomen.org/-/media/headquarters/attachments/sections/library/publications/2020/issue-brief-covid-19-and-ending-violence-against-women-and-girls-en.pdf?la=enandvs,=5006 (accessed April 28, 2021).
43. Asim SS, Ghani S, Ahmed M, Asim A, Qureshi AFK. Assessing mental health of women living in karachi during the Covid-19 pandemic. Front Global Womens Health. (2021) 1:24. doi: 10.3389/fgwh.2020.594970
44. Bigalke JA, Greenlund IM, Carter JR. Sex differences in self-report anxiety and sleep quality during COVID-19 stay-at-home orders. Biol Sex Differ. (2020) 11:1–11. doi: 10.1186/s13293-020-00333-4
45. Yan S, Xu R, Stratton TD, Kavcic V, Luo D, Hou F, et al. Sex differences and psychological stress: responses to the COVID-19 pandemic in China. BMC Public Health. (2021) 21:1–8. doi: 10.1186/s12889-020-10085-w
46. Navarrete F, Garcia-Gutiérrez MS, Jurado-barba R, Rubio G, Gasparyan A, Austrich-Olivares A, et al. Endocannabinoid system components as potential biomarkers in Psychiatry. Front Psychiatry. (2020) 11:315. doi: 10.3389/fpsyt.2020.00315
47. Sciolino NR, Bortolato M, Eisenstein SA, Fu J, Oveisi F, Hohmann AG, et al. Social isolation and chronic handling alter endocannabinoid signaling and behavioral reactivity to context in adult rats. Neuroscience. (2010) 168:371–86. doi: 10.1016/j.neuroscience.2010.04.007
48. Robinson SA, Loiacono RE, Christopoulos A, Sexton PM, Malone DT. The effect of social isolation on rat brain expression of genes associated with endocannabinoid signaling. Brain Res. (2010) 1343:153–67. doi: 10.1016/j.brainres.2010.04.031
49. González S, Bisogno T, Wenger T, Manzanares J, Milone A, Berrendero F, et al. Sex steroid influence on cannabinoid CB1 receptor mRNA and endocannabinoid levels in the anterior pituitary gland. Biochem Biophys Res Commun. (2000) 270:260–6. doi: 10.1006/bbrc.2000.2406
50. Onaivi ES, Chaudhuri G, Abaci AS, Parker M, Manier DH, Martin PR, et al. Expression of cannabinoid receptors and their gene transcripts in human blood cells. Prog Neuropsychopharmacol Biol Psychiatry. (1999) 23:1063–77. doi: 10.1016/S0278-5846(99)00052-4
51. Reich CG, Taylor ME, McCarthy MM. Differential effects of chronic unpredictable stress on hippocampal CB1 receptors in male and female rats. Behav Brain Res. (2009) 203:264–9. doi: 10.1016/j.bbr.2009.05.013
52. Bradshaw HB, Rimmerman N, Krey JF, Walker JM. Sex and hormonal cycle differences in rat brain levels of pain-related cannabimimetic lipid mediators. Am J Physiol Regul Integr Comp Physiol. (2006) 291:R349–R58. doi: 10.1152/ajpregu.00933.2005
53. Lukkes JL, Watt MJ, Lowry CA, Forster GL. Consequences of post-weaning social isolation on anxiety behavior and related neural circuits in rodents. Front Behav Neurosci. (2009) 3:18. doi: 10.3389/neuro.08.018.2009
54. Van Den Berg CL, Van Ree JM, Spruijt BM. Sequential analysis of juvenile isolation-induced decreased social behavior in the adult rat. Physiol Behav. (1999) 67:483–8. doi: 10.1016/S0031-9384(99)00062-1
55. Davidson RJ. Anxiety and affective style: role of prefrontal cortex and amygdala. Biol Psychiatry. (2002) 51:68–80. doi: 10.1016/S0006-3223(01)01328-2
56. Marcus DJ, Bedse G, Gaulden AD, Ryan JD, Kondev V, Winters ND, et al. Endocannabinoid signaling collapse mediates stress-induced amygdalo-cortical strengthening. Neuron. (2020) 105:1062–76.e6. doi: 10.1016/j.neuron.2019.12.024
57. Bravo JA, Julio Pieper M, Forsythe P, Kunze W, Dinan TG, Bienenstock J, et al. Communication between gastrointestinal bacteria and the nervous system. Curr Opin Pharmacol. (2012) 12:667–72. doi: 10.1016/j.coph.2012.09.010
58. Guida F, Turco F, Iannotta M, De Gregorio D, Palumbo I, Sarnelli G, et al. microbiota perturbation causes gut endocannabinoidome changes, hippocampal neuroglial reorganization and depression in mice. Brain Behav Immun. (2018) 67:230–45. doi: 10.1016/j.bbi.2017.09.001
59. De Gregorio D, Manchia M, Carpiniello B, Valtorta F, Nobile M, Gobbi G, et al. Role of palmitoylethanolamide (PEA) in depression: translational evidence: Special Section on “Translational and Neuroscience Studies in Affective Disorders”. Section Editor, Maria Nobile MD, PhD. This Section of JAD focuses on the relevance of translational and neuroscience studies in providing a better understanding of the neural basis of affective disorders. The main aim is to briefly summaries relevant research findings in clinical neuroscience with particular regards to specific innovative topics. J Affect Disord. (2019) 255:195–200. doi: 10.1016/j.jad.2018.10.117
60. Noce A, Albanese M, Marrone G, Di Lauro M, Pietroboni Zaitseva A, Palazzetti D, et al. Ultramicronized Palmitoylethanolamide (um-PEA): a New Possible Adjuvant Treatment in COVID-19 patients. Pharmaceuticals. (2021) 14:336. doi: 10.3390/ph14040336
61. Di Marzo V. Endocannabinoids: an appetite for fat. Proc Natl Acad Sci U S A. (2011) 108:12567–8. doi: 10.1073/pnas.1109567108
62. Martin FP, Rezzi S, Peré-Trepat E, Kamlage B, Collino S, Leibold E, et al. Metabolic effects of dark chocolate consumption on energy, gut microbiota, and stress-related metabolism in free-living subjects. J Proteome Res. (2009) 8:5568–79. doi: 10.1021/pr900607v
63. di Tomaso E, Beltramo M, Piomelli D. Brain cannabinoids in chocolate. Nature. (1996) 382:677–8. doi: 10.1038/382677a0
64. Maurelli S, Bisogno T, De Petrocellis L, Di Luccia A, Marino G, Di Marzo. V. Two novel classes of neuroactive fatty acid amides are substrates for mouse neuroblastoma 'anandamide amidohydrolase'. FEBS Lett. (1995) 377:82–6. doi: 10.1016/0014-5793(95)01311-3
65. Di Marzo V, Sepe N, De Petrocellis L, Berger A, Crozier G, Fride E, et al. Trick or treat from food endocannabinoids? Nature. (1998) 396:636. doi: 10.1038/25267
66. Gertsch J, Leonti M, Raduner S, Racz I, Chen JZ, Xie XQ, et al. Beta-caryophyllene is a dietary cannabinoid. Proc Natl Acad Sci U S A. (2008) 105:9099–104. doi: 10.1073/pnas.0803601105
67. Nicolussi S, Viveros-Paredes JM, Gachet MS, Rau M, Flores-Soto ME, Blunder M, et al. Guineensine is a novel inhibitor of endocannabinoid uptake showing cannabimimetic behavioral effects in BALB/c mice. Pharmacol Res. (2014) 80:52–65. doi: 10.1016/j.phrs.2013.12.010
68. Hu B, Doods H, Treede RD, Ceci A. Depression-like behavior in rats with mononeuropathy is reduced by the CB2-selective agonist GW405833. Pain. (2009) 143:206–12. doi: 10.1016/j.pain.2009.02.018
69. Hwang ES, Kim HB, Lee S, Kim MJ, Kim KJ, Han G, et al. Antidepressant-like effects of β-caryophyllene on restraint plus stress-induced depression. Behav Brain Res. (2020) 380:112439. doi: 10.1016/j.bbr.2019.112439
70. Pacioni G, Rapino C, Zarivi O, Falconi A, Leonardi M, Battista N, et al. Truffles contain endocannabinoid metabolic enzymes and anandamide. Phytochemistry. (2015) 110:104–10. doi: 10.1016/j.phytochem.2014.11.012
71. Thors L, Belghiti M, Fowler CJ. Inhibition of fatty acid amide hydrolase by kaempferol and related naturally occurring flavonoids. Br J Pharmacol. (2008) 155:244–52. doi: 10.1038/bjp.2008.237
72. USDA Database. USDA Database for the Flavonoid Content of Selected Foods. Release 3" U.S. Department of Agriculture (2011). Available online at: https://www.ars.usda.gov/ARSUserFiles/80400525/Data/Flav/Flav_R03-1.pdf (accessed May 1, 2020).
73. Gertsch J, Pertwee RG, Di Marzo. V. Phytocannabinoids beyond the Cannabis plant-do they exist? Br J Pharmacol. (2010) 160:523–9. doi: 10.1111/j.1476-5381.2010.00745.x
74. Witkin JM, Leucke S, Thompson LK, Lynch RA, Ding C, Heinz B, et al. Further evaluation of the neuropharmacological determinants of the antidepressant-like effects of curcumin. CNS Neurol Disord Drug Targets. (2013) 12:498–505. doi: 10.2174/1871527311312040008
75. The New York Times. Is Marijuana an 'Essential' Like Milk or Bread? Some States Say Yes. (2020). Available online at: https://www.nytimes.com/article/coronavirus-weed-marijuana.html (accessed April 29, 2020).
76. Fitzgerald ML, Mackie K, Pickel VM. The impact of adolescent social isolation on dopamine D2 and cannabinoid CB1 receptors in the adult rat prefrontal cortex. Neuroscience. (2013) 235:40–50. doi: 10.1016/j.neuroscience.2013.01.021
77. Vanderbruggen N, Matthys F, Van Laere S, Zeeuws D, Santermans L, Van den Ameele S, et al. Self-reported alcohol, tobacco, and Cannabis use during COVID-19 lockdown measures: results from a web-based survey. Eur Addict Res. (2020) 26:309–15. doi: 10.1159/000510822
78. Cousijn J, Kuhns L, Larsen H, Kroon E. For better or for worse? A pre-post exploration of the impact of the COVID-19 lockdown on cannabis users. Addiction. (2021). doi: 10.1111/add.15387. [Epub ahead of print].
79. Gili A, Bacci M, Aroni K, Nicoletti A, Gambelunghe A, Mercurio I, et al. Changes in Drug Use Patterns during the COVID-19 Pandemic in Italy: monitoring a vulnerable group by hair analysis. Int J Environ Res Public Health. (2021) 18:1967. doi: 10.3390/ijerph18041967
80. Groshkova T, Stoian T, Cunningham A, Griffiths P, Singleton N, et al. Will the current COVID-19 pandemic impact on long-term cannabis buying practices? J Addict Med. (2020) 14:e13–0. doi: 10.1097/ADM.0000000000000698
81. Boehnke KF, McAfee J, Ackerman JM, Kruger DJ. Medication and substance use increases among people using cannabis medically during the COVID-19 pandemic. Int J Drug Policy. (2020) 92:103053. doi: 10.1016/j.drugpo.2020.103053
82. Bisogno T, Hanus L, De Petrocellis L, Tchilibon S, Ponde DE, Brandi I, et al. Molecular targets for cannabidiol and its synthetic analogues: effect on vanilloid VR1 receptors and on the cellular uptake and enzymatic hydrolysis of anandamide. Br J Pharmacol. (2001) 134:845–52. doi: 10.1038/sj.bjp.0704327
83. Hartmann A, Lisboa SF, Sonego AB, Coutinho D, Gomes FV, Guimarães FS. Cannabidiol attenuates aggressive behavior induced by social isolation in mice: Involvement of 5-HT1A and CB1 receptors. Prog Neuropsychopharmacol Biol Psychiatry. (2019) 94:109637. doi: 10.1016/j.pnpbp.2019.109637
84. Raduner S, Majewska A, Chen JZ, Xie XQ, Hamon J, Faller B, et al. Alkylamides from Echinacea are a new class of cannabinomimetics. Cannabinoid type 2 receptor-dependent and -independent immunomodulatory effects. J Biol Chem. (2006) 281:14192–206. doi: 10.1074/jbc.M601074200
85. Chicca A, Raduner S, Pellati F, Strompen T, Altmann KH, Schoop R, et al. Synergistic immunomopharmacological effects of N-alkylamides in Echinacea purpurea herbal extracts. Int Immunopharmacol. (2009) 9:50–858. doi: 10.1016/j.intimp.2009.03.006
86. Haller J, Hohmann J, Freund TF. The effect of Echinacea preparations in three laboratory tests of anxiety: comparison with chlordiazepoxide. Phytother Res. (2010) 24:1605–13. doi: 10.1002/ptr.3181
87. Schuch FB, Vancampfort D, Richards J, Rosenbaum S, Ward PB, Stubbs B. Exercise as a treatment for depression: a meta-analysis adjusting for publication bias. J Psychiatr Res. (2016) 77:42–51. doi: 10.1016/j.jpsychires.2016.02.023
88. Stubbs B, Vancampfort D, Rosenbaum S, Firth J, Cosco T, Veronese N, et al. An examination of the anxiolytic effects of exercise for people with anxiety and stress-related disorders: a meta-analysis. Psychiatry Res. (2017) 249:102–8. doi: 10.1016/j.psychres.2016.12.020
89. Jakobsson J, Malm C, Furberg M, Ekelund U, Svensson M. Physical activity during the coronavirus (COVID-19) pandemic: prevention of a decline in metabolic and immunological functions. Front Sports Active Living. (2020) 2:57. doi: 10.3389/fspor.2020.00057
90. Maccarrone M. Missing pieces to the endocannabinoid puzzle. Trends Mol Med. (2020) 26:263–72. doi: 10.1016/j.molmed.2019.11.002
91. Tantimonaco M, Ceci R, Sabatini S, Catani MV, Rossi A, Gasperi V, et al. Physical activity and the endocannabinoid system: an overview. Cell Mol Life Sci. (2014) 71:2681–98. doi: 10.1007/s00018-014-1575-6
92. Sparling PB, Giuffrida A, Piomelli D, Rosskopf L, Dietrich A. Exercise activates the endocannabinoid system. Neuroreport. (2003) 14:2209–11. doi: 10.1097/00001756-200312020-00015
93. Feuerecker M, Hauer D, Toth R, Demetz F, Hölzl J, Thiel M, et al. Effects of exercise stress on the endocannabinoid system in humans under field conditions. Eur J Appl Physiol. (2012) 112:2777–81. doi: 10.1007/s00421-011-2237-0
94. You T, Disanzo BL, Wang X, Yang R, Gong D. Adipose tissue endocannabinoid system gene expression: depot differences and effects of diet and exercise. Lipids Health Dis. (2011) 10:194. doi: 10.1186/1476-511X-10-194
95. Facco E. Meditazione e Ipnosi tra Neuroscienze, Filosofia e Pregiudizio. Lungavilla, PV: Altravista (2014).
96. Facco E. Meditation and hypnosis: two sides of the same coin? Int J Clin Exp Hypn. (2017) 65:169–88. doi: 10.1080/00207144.2017.1276361
97. Frisardi E, Piedimonte A, Benedetti F. Placebo and nocebo effects: a complex interplay between psychological factors and neurochemical networks. Am J Clin Hypn. (2015) 57:267–84. doi: 10.1080/00029157.2014.976785
98. Benedetti F, Amanzio M, Rosato R, Blanchard C. Non-opioid placebo analgesia is mediated by CB1 cannabinoid receptors. Nat Med. (2011) 17:1228–30. doi: 10.1038/nm.2435
99. Casiglia E, Finatti F, Tikhonoff V, Stabile MR, Mitolo M, Albertini F, et al. Mechanisms of hypnotic analgesia explained by functional magnetic resonance (fMRI). Int J Clin Exp Hypn. (2020) 68:1–15. doi: 10.1080/00207144.2020.1685331
100. Derbyshire SW, Whalley MG, Stenger VA, Oakley DA. Cerebral activation during hypnotically induced and imagined pain. Neuroimage. (2004) 23:392–401. doi: 10.1016/j.neuroimage.2004.04.033
101. Faymonville ME, Laureys S, Degueldre C, DelFiore G, Luxen A, Franck G, et al. Neural mechanisms of antinociceptive effects of hypnosis. Anesthesiology. (2000) 92:1257–67. doi: 10.1097/00000542-200005000-00013
102. Rainville P, Hofbauer RK, Paus T, Duncan GH, Bushnell MC, Price DD. Cerebral mechanisms of hypnotic induction and suggestion. J Cogn Neurosci. (1999) 11:110–25. doi: 10.1162/089892999563175
103. Facco E. Hypnosis for resilience. BMC Complement Altern Med. (2020) 5:20. doi: 10.21926/obm.icm.2003032
104. Menon V. Large-scale brain networks and psychopathology: a unifying triple network model. Trends Cogn Sci. (2011) 15:483–506. doi: 10.1016/j.tics.2011.08.003
105. Cole MW, Repovš G, Anticevic A. The Frontoparietal Control System. Neuroscientist. (2014) 20:652–64. doi: 10.1177/1073858414525995
106. Yamasue H, Kasai K, Iwanami A, Ohtani T, Yamada H, Abe O, et al. Voxel-based analysis of MRI reveals anterior cingulate gray-matter volume reduction in posttraumatic stress disorder due to terrorism. Proc Natl Acad Sci U S A. (2003) 100:9039–43. doi: 10.1073/pnas.1530467100
107. Facco E, Mendozzi L, Bona A, Motta A, Garegnani M, Costantini I, et al. Dissociative identity as a continuum from healthy mind to psychiatric disorders: epistemological and neurophenomenological implications approached through hypnosis. Med Hypotheses. (2019) 130:109274. doi: 10.1016/j.mehy.2019.109274
Keywords: cannabinoid, stress, HPA, resilience, lockdown, CBD, COVID-19, meditation
Citation: Brugnatelli V, Facco E and Zanette G (2021) Lifestyle Interventions Improving Cannabinoid Tone During COVID-19 Lockdowns May Enhance Compliance With Preventive Regulations and Decrease Psychophysical Health Complications. Front. Psychiatry 12:565633. doi: 10.3389/fpsyt.2021.565633
Received: 25 May 2020; Accepted: 18 May 2021;
Published: 16 July 2021.
Edited by:
Gianluca Castelnuovo, Catholic University of the Sacred Heart, ItalyReviewed by:
Arpan Dutta, The University of Manchester, United KingdomCopyright © 2021 Brugnatelli, Facco and Zanette. This is an open-access article distributed under the terms of the Creative Commons Attribution License (CC BY). The use, distribution or reproduction in other forums is permitted, provided the original author(s) and the copyright owner(s) are credited and that the original publication in this journal is cited, in accordance with accepted academic practice. No use, distribution or reproduction is permitted which does not comply with these terms.
*Correspondence: Viola Brugnatelli, dmlvbGFAY2FubmFiaXNjaWVuemEuaXQ=
Disclaimer: All claims expressed in this article are solely those of the authors and do not necessarily represent those of their affiliated organizations, or those of the publisher, the editors and the reviewers. Any product that may be evaluated in this article or claim that may be made by its manufacturer is not guaranteed or endorsed by the publisher.
Research integrity at Frontiers
Learn more about the work of our research integrity team to safeguard the quality of each article we publish.