- 1Department of Physiology, College of Medicine, Kyung Hee University, Seoul, South Korea
- 2Department of Food Science and Biotechnology, Kyonggi University, Suwon, South Korea
Menopause is a risk factor of anxiety and depression. Also, psychoneurological symptoms are shown in almost all women in the perimenopausal period. The present study investigated if repeated stress modulates behavioral changes or the balance of pro- and anti-inflammatory cytokines in ovariectomized (OVX) rats. Albino SD female rats were randomly divided into four groups: the naïve normal group (NOR), a surgically ovariectomized group (OVX), the only stressed group (ST), and the OVX and stressed groups (OVX + ST). We performed a battery of tests such as the forced swimming test (FST), the sucrose intake, and social exploration. In the same animals, corticosterone (CORT) was assessed in the serum, and also, two representative cytokines (IL-1β and IL-4) were examined in different brain regions after all the behavior sessions for all the experimental groups. The OVX + ST group showed more immobility time in FST than the OVX group or the ST group. Also, the OVX + ST group tended to have a decreased active social exploration and sucrose solution intake compared to the OVX group or ST group. The serum concentration of CORT of the OVX + ST group was higher than the OVX group or ST group and also the level of CORT in OVX + ST was markedly increased compared to the NOR group. In the brain, the number of IL-1β immunoreactive neurons of the OVX + ST group was increased compared to the NOR group. The OVX + ST group tended to have an increase in IL-1β-positive neurons compared to the OVX or ST group. However, the number of IL-4 immunoreactive neurons of the OVX + ST group was markedly decreased compared with the NOR group. Also, the IL-4-positive neurons in the OVX + ST group was significantly decreased when compared to the ST group. These results indicate that ovariectomy and stress combine to increase the depressive-like behaviors and neuroinflammatory responses. Together, these data show neuroinflammation as a potential contributor to depressive-like symptoms during menopausal transition.
Introduction
Ovarian hormones play a pivotal role in regulating affective disorder (1). Depletion of ovarian hormone as menopausal implicated increased mood disorders and deficient learning and memory (2). Also, several studies proved that estrogen and testosterone regulate HPA axis responses (3, 4). The postmenopausal stage in women exacerbates mood disorder or insomnia (5). Some studies implicated that mood disorders are related to change of neuroimmune systems (6–9). For example, chronic restraint stress or foot shock stress elicits depressive behaviors (learned helplessness, anhedonia, anxiety, etc.) and also chronic stress alter neuroimmune systems (10, 11). Other studies reported that psychological stress is associated with pro-inflammatory cytokine release or depressive behavior (12, 13). In a recent study, infectious challenge with IL-1β to IL-4 KO mice resulted in dysregulation of pro-inflammatory cytokines (14). IL-4 KO is capable of causing susceptibility to immune responses.
A recent study proved that the postmenopausal stage altered the neuroendocrine–immune system (15). Menopausal women experience difficulty in coping with stressful situations. Stress levels are perceived to vary with menopause (16). This is related to pro-inflammatory cytokine levels in the serum (16). Also, the ovarian hormone exerts potent immunomodulatory effects in neuroinflammation animal models (17–19). Although estrogen is associated with menopause-related neuronal inflammation changes, the mechanisms behind its effects are unclear.
In this study, we aimed to investigate whether repeated stress in the OVX rat model could change depressive-like behaviors and changes of pro- and anti-inflammatory cytokines in the brain regions. Depressive behavior was tested via a forced swimming test (FST), social exploration, and sucrose intake; moreover, we further assessed the changes of IL-1β- and IL-4-reactive neurons in the PVN and MTN using immunohistochemistry.
Materials and Methods
Animals and Immobilization Stress
Albino SD female rats (over 3 months old, weighing 240–300 g) (Orient, Inc. Korea) underwent ovariectomy. All rats were housed in a plastic cage with stainless steel lips under controlled conditions (temperature: 22–24°C, 12-h light/dark cycle, and humidity 65 ± 5%). The rats had ad libitum access to food and water. All the experiments were approved by the Kyung Hee University Institutional Animal Care and Use Committee [approval no. KHUAP(SE)-13-041].
Albino SD female rats were randomly divided into four groups: the naïve normal group (NOR, n = 6), a surgically ovariectomized group (OVX, n = 6), the only stressed group (ST, n = 6), and the OVX and stressed group (OVX + ST, n = 6). Bilateral ovariectomy was performed under pentobarbital sodium (50 mg/kg, i.p.). The animals were monitored for 1 week post-surgery. Postoperative recuperation was monitored for 1 week.
After 1 week, ST groups were exposed to repeated immobilization stress using the disposable plastic cone (a disposable rodent restraint cone, Yusung, Korea) for 2 h (10:00–12:00 a.m.) for 14 days. The experimental schedule is shown in Figure 1.
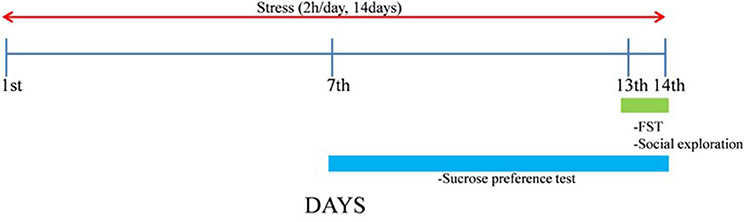
Figure 1. Experimental schedule. The naïve normal group (NOR), a surgically ovariectomized group (OVX), the only stressed group (ST), and the OVX and stressed group (OVX + ST). Each group contained six rats (n = 6).
Forced Swimming Test
Transparent Plexiglas cylinders (height: 50 cm × diameter: 20 cm) were used for FST. The room temperature water was filled to a 30-cm depth. Before test, all rats were taken pre-test for 15 min. After 24 h, rats were tested for 5 min. We used a video camera and analyzed. Total duration of immobility, climbing, and swimming were examined (20).
Social Exploration
On the first day, rats were introduced into their transparent home cage for 5 min. After 24 h, rats were recorded as doing “social exploration” for 5 min. We counted the behaviors (active behaviors: grooming of test rat, sniffing, and liking; inactive behaviors: boxing and kicking).
Sucrose Preference Test
All rats assessed adaptive training for 1 week. Training consisted of seven 12-h tests, and rats could freely choose between a bottle of water and a bottle containing a mild 1% [weight/volume (w/v)] sucrose solution. Two bottles were rotated daily. Each rat freely selected a pre-weighed tap water bottle or a 1% sucrose bottle. On the test day (13th day), the total consumption of each bottle was recorded after 12 h.
Corticosterone Measurement
The blood samples were collected and then centrifuged. The final supernatant was used for corticosterone analysis. Concentration of corticosterone was determined using ELISA kit (DuoSet ELISA development system, R&D Systems, Inc., Minneapolis, MN., USA) according to the manufacturer's protocols.
Immunohistochemistry
After all behavioral tests, rats were euthanized with an overdose of sodium pentobarbital (80 mg/kg, IP) and then they were transcardially perfused with phosphate buffered saline (PBS) into a protrusion of the left ventricle, with a steady flow of around 20 ml/min of saline solution. When all blood has been cleared from the body, we used a cold 4% paraformaldehyde solution at a flow rate of 7 ml/min (800 ml). The brains were removed and placed in a 4% paraformaldehyde solution for 24 h at 4°C. The brain was placed in O.C.T. compound at −20°C and cut into 30-μm serial coronal sections. Sections were rinsed with PBS containing Triton X-100. The primary antibody concentrations were at 1:100 and 1:1,000 for IL-1β (Santa Cruz Biotechnology, Delaware Avenue Santa Cruz, CA, USA) and IL-4 (Santa Cruz Biotechnology, Delaware Avenue Santa Cruz, CA, USA), respectively. The primary antibody was diluted with blocking serum in ABC kits (Vector Laboratories, Burlingame, CA) for 72 h at 4°C. Then, tissues were washed three times in PBS. For the secondary antibody use as the appropriate primary antibody (1:200) in PBST. Tissues were washed three times in PBS and stained using diaminobenzidine (DAB) chromogen with nickel intensification and coverslipped slides with mounting media. Light microscopy was used for acquiring photomicrographs. The stained cell was counted in paraventricular nucleus (PVN) and motor trigeminal nucleus (MTN) according to a brain atlas (21).
Statistical Analysis
SPSS 15.0 software (SPSS Inc., Chicago, IL) was used for statistical analysis. Differences among groups in the behavioral test and immunohistochemistry were analyzed using one-way ANOVA and LSD post hoc test. A P < 0.05 was considered statistically significant. GraphPad Prism 6.0 software was used for graph generation.
Results
Behavioral Tests
As shown in Figures 2A,B, the immobility time of the OVX groups in the FST significantly increased compared to the NOR group [F(3, 20) = 4.7, P < 0.05]. When tested in the FST, the OVX and OVX + ST groups tended to show decreased active behavior (swimming and climbing) compared to the NOR group. Figure 2C shows that the sucrose preference test was significantly different when compared among the groups [F(3, 20) = 22.9, P < 0.001]. Also, the OVX + ST group showed more immobility time compared to the OVX group or the ST group. Furthermore, the OVX + ST group showed a decrease in consumption of sucrose solution by 30% compared with the NOR group (P < 0.001). Also, the OVX + ST group tended to decrease sucrose solution intake compared to the OVX group or the ST group. Figure 2D shows that the social exploration tests were markedly different among the groups [F(3, 20) = 8.3, P < 0.01]. The active behavior of the juvenile was decreased in the OVX + ST group compared with the NOR group (P < 0.05). Also, the OVX + ST group tended to have a decreased active social exploration when compared to the OVX group or the ST group.
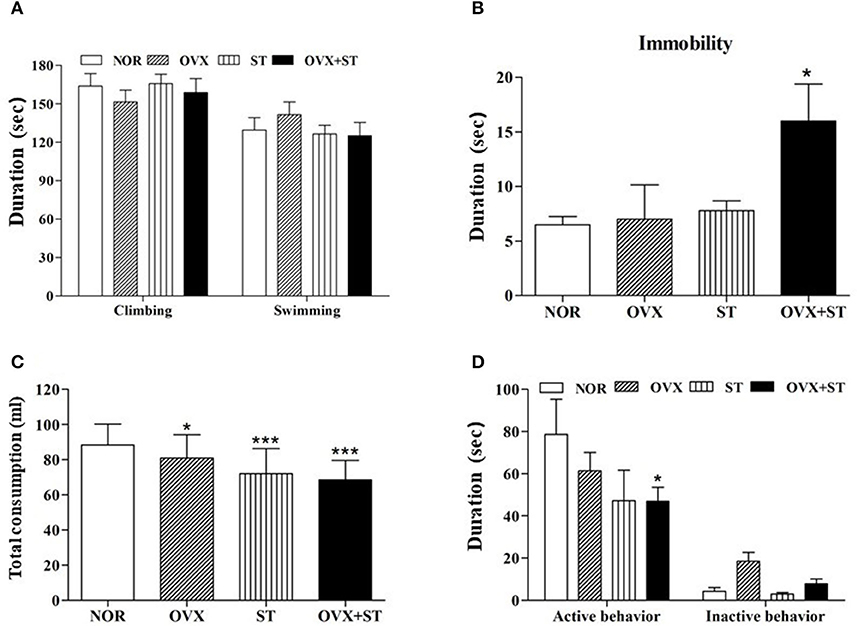
Figure 2. The effects of repeated immobilization stress on the forced swimming test in the rats. The data represent the means ± SEM of the duration of climbing and swimming (A) and immobility (B) during the 5-min test session. Also, the effects of repeated immobilization stress on the sucrose intake (C) and social exploration (D). The data represent the duration of active behavior during the 5-min test session. The naïve normal group (NOR), a surgically ovariectomized group (OVX), the only stressed group (ST), and the OVX and stressed group (OVX + ST). Each group contained six rats (n = 6). The results of behaviors were analyzed by performing separate one-way ANOVA among the groups. *p < 0.05, ***p < 0.001 compared to the normal group.
Corticosterone
As shown in Figure 3, the serum concentration of CORT was significantly different among the groups [F(3, 20) = 5.8, P < 0.01]. Statistical results indicated the markedly increased serum concentration of CORT in the OVX + ST group compared with the NOR group (P < 0.01), and the level of CORT in OVX +ST was markedly increased compared to the NOR group.
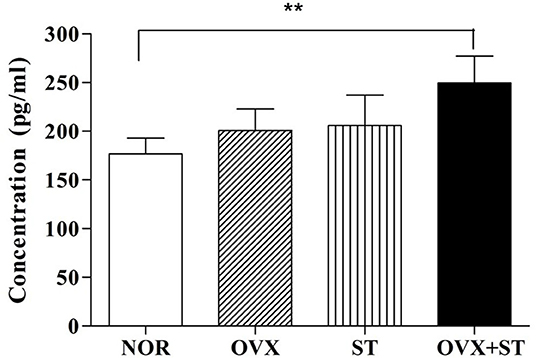
Figure 3. The effects of the corticosterone level in the serum. The naïve normal group (NOR), a surgically ovariectomized group (OVX), the only stressed group (ST), and the OVX and stressed group (OVX + ST). Each group contained six rats (n = 6). The results of ELISA were analyzed by performing separate one-way ANOVA among the groups. Each value represents the mean ± S.E.M. **p < 0.01 compared to the normal group.
IL-1β Immunohistochemistry
As shown in Figures 4A–H, the number of IL-1β-positive neurons in the PVN [F(3, 14) = 23.7, P < 0.001] and MTN (F(3, 14) = 9.0, P < 0.01) was significantly different among the groups. The expression of the IL-1β-positive neurons in the PVN of the OVX + ST group increased threefold compared with the NOR group (P < 0.001). Also, the expression of the IL-1β positive neurons in the MTN was significantly increased in the OVX + ST group compared with the NOR group (P < 0.01). The OVX + ST group tended to have an increase in IL-1β positive neurons compared to the OVX or ST group in the MTN and PVN.
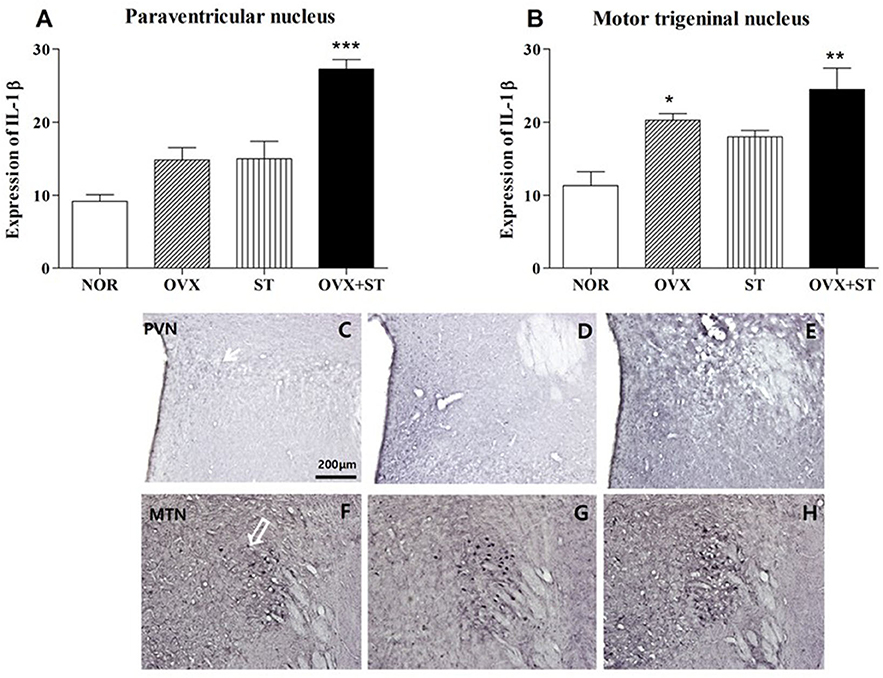
Figure 4. The number of IL-1β immunostained nuclei in the paraventricular nucleus (A) and motor trigeminal nucleus regions (B). The naïve normal group (NOR), a surgically ovariectomized group (OVX), the only stressed group (ST), and the OVX and stressed group (OVX + ST). Each group contained six rats (n = 6). The results of IL-1β-reactivity were analyzed by performing separate one-way ANOVA on the number of the IL-1β immunostained neurons among the groups. Each value represents the mean ± S.E.M. *p < 0.05, **p < 0.01, ***p < 0.001 compared to the normal group. Photographs showing the distribution of IL-1β immunoreactive cells in the brain of NOR (C,F), OVX (D,G), and OVX + ST (E,H). Sections were cut coronally at 30 μm and the scale bar represents 200 μm. PVN, paraventricular nucleus; MTN, motor trigeminal nucleus.
IL-4 Immunohistochemistry
As shown in Figures 5A–H, the number of IL-1β-positive neurons in the PVN [F(3, 13) = 7.2, p < 0.05] and MTN [F(3, 15) = 11.0, p < 0.001] was significantly different among the groups. The number of IL-4 neurons in the PVN area of the OVX + ST group was decreased compared to that in the normal group. Also, the expression of the IL-4-positive neurons in the PVN was significantly decreased in the OVX + ST group compared with the NOR group (P < 0.05). In the MTN, the expression of the IL-4-positive neurons was markedly decreased in the OVX +ST group compared with the NOR group (P < 0.05). Also, the IL-4-positive neurons in the OVX + ST group was significantly decreased when compared to the ST group.
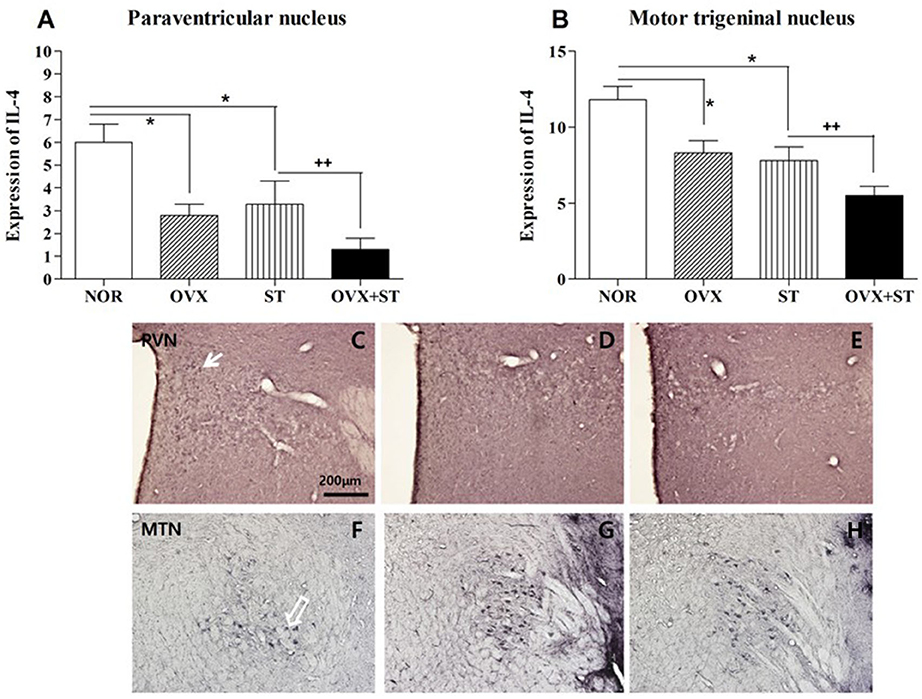
Figure 5. The number of IL-1β immunostained nuclei in the paraventricular nucleus (A) and motor trigeminal nucleus regions (B). The naïve normal group (NOR), a surgically ovariectomized group (OVX), the only stressed group (ST), and the OVX and stressed group (OVX + ST). Each group contained six rats (n = 6). The results of IL-4 reactivity were analyzed by performing separate one-way ANOVA on the number of the IL-4 immunostained neurons among the groups. Each value represents the mean ± S.E.M. *p < 0.05 compared to the normal group. ++p < 0.01 compared to the ST group. Photographs showing the distribution of IL-4 immunoreactive cells in the brain of normal group (C,F), OVX (D,G), and OVX + ST (E,H). Sections were cut coronally at 30 μm and the scale bar represents 200 μm. PVN, paraventricular nucleus; MTN, motor trigeminal nucleus.
The Relationships Between IL-1β and IL-4 Expression
The Spearman's correlation test revealed significant relationships between IL-1β and IL-4. In the experiment groups, biochemical and neuro-immunological characteristics were changed. In particular, the mean levels of IL-1β increased significantly after OVX and repeated stress, whereas IL-4 decreased significantly. In the PVN (r = 0.7, P < 0.05) and MTN (r = 0.7, P < 0.01), the change in IL-1β and IL-4 correlates significantly after OVX and repeated stress.
Discussion
The present results proved that repeated immobilization stress in the OVX rats poses an immune challenge that is capable of inducing depressive like behaviors, promoting exaggerated corticosterone responses and changing the cytokine expression in the brain. The present results showed that the interaction between the anti- and pro-inflammatory mediators ultimately determines both the severity of the manifestation of depressive-like behavior and neuro-immune dysfunction.
Ovarian hormones have played a role in the regulation of cognitive and mood functions (22). They have been proposed as a contributor to depressive behavior and the synthesis, release, and reuptake of many receptors for neurotransmitters (23). This study also showed an increase of depression-like behaviors including reduction of preferences for sucrose solution, inhibition of locomotor activities, and induction of learned helplessness behavior. Also, the effects of female sexual hormones on both neuroinflammatory responses and depressive behaviors have also been well described by Azizi-Malekabadi et al. (22). 17β-estradiol (E2) replacement exhibits anti-inflammatory properties in the central nervous system (18). Overproduction of pro-inflammatory cytokine could be related to the inflammatory response system, which is upregulated during depression, and this is related to the shift of the pro-/anti-inflammatory cytokine balance. A previous study proved that psychological stress is associated with pro-inflammatory cytokines in the brain such as IL-6, interleukin-1β (IL-1β), and tumor necrosis factor α (TNF-α) (24). You et al. proved that chronic mild stress induced inflammatory responses in the spleen and brain region, as well as increased depressive-like behaviors in rats (25). The present study also observed that the expression of IL-1β immunoreactive cells was increased in the PVN and MTN after OVX and repeated immobilization stress. These results indicated that life events and depressive symptoms are related to the rise of central pro-inflammatory cytokines such as IL-1β in major mood disorder patients (26–29) and stress-treated animals (30).
On the other hand, Myint et al. and Pavon et al. reported that depressed patients showed lower IL-4 levels in the serum (28, 31). Also, recent findings in laboratory studies have highlighted the role of interleukin-4, which is released from glia (32), in regulating the neuroinflammatory changes that occur in brain regions. We also observed that the IL-4 immunoreactivity was downregulated in the brain after repeated immobilization stress. The data in this current study indicate that there is an imbalance between anti-inflammatory and pro-inflammatory cytokines (IL-4 and IL-1β) in depressed female rats. The shift between anti-inflammatory and pro-inflammatory cytokines in different psychiatric disorders has been previously reported, and the dominance of macrophage cytokines in major depression has also been described (28). The present study showed that the increased IL-1β/IL-4 ratio may be associated with the activation of macrophage-induced inflammatory response. The present study also found that stimulated production of IL-1β and IL-4 displayed an opposite inflammatory pattern. These changes could be part of normal immune modulation. According to present results, we found an imbalance between anti-inflammatory and pro-inflammatory cytokines in depressed female rats that is associated with life events.
Data Availability Statement
The datasets generated for this study are available on request to the corresponding author.
Ethics Statement
All the experiments were approved by the Kyung Hee University Institutional Animal Care (Approval No. KHUAP(SE)-13-041) and in accordance with the US National Institutes of Health Guide for the Care and Use of Laboratory Animals (NIH Publication number 80-23, revised 1996).
Author Contributions
IS, HS, and HP designed the study. HP and HS acquired and analyzed the data. HP, HS, and IS wrote the article, which all other authors reviewed. All authors contributed to the article and approved the submitted version.
Funding
This research was supported by the National Research Foundation of Korea (NRF) grant funded by the Korean government (NRF-2016M3C7A1905384 and NRF 2018R1D1A1B07048597).
Conflict of Interest
The authors declare that the research was conducted in the absence of any commercial or financial relationships that could be construed as a potential conflict of interest.
References
1. Ceccarelli I, Fiorenzani P, Massafra C, Aloisi AM. Long-term ovariectomy changes formalin-induced licking in female rats: the role of estrogens. Reprod Biol Endocrinol. (2003) 1:24. doi: 10.1186/1477-7827-1-24
2. Shors TJ, Leuner B. Estrogen-mediated effects on depression and memory formation in females. J Affect Disord. (2003) 74:85–96. doi: 10.1016/S0165-0327(02)00428-7
3. Toufexis D, Rivarola MA, Lara H, Viau V. Stress and the reproductive axis. J Neuroendocrinol. (2014) 26:573–86. doi: 10.1111/jne.12179
4. Joseph DN, Whirledge S. Stress and the HPA axis: balancing homeostasis and fertility. Int J Mol Sci. (2017) 18:2224. doi: 10.3390/ijms18102224
5. Hantsoo L, Epperson CN. Anxiety disorders among women: a female lifespan approach. Focus. (2017) 15:162–72. doi: 10.1176/appi.focus.20160042
6. Cai YJ, Wang F, Chen ZX, Li L, Fan H, Wu ZB, et al. Hashimoto's thyroiditis induces neuroinflammation and emotional alterations in euthyroid mice. J Neuroinflamm. (2018) 15:299. doi: 10.1186/s12974-018-1341-z
7. Colpo GD, Leboyer M, Dantzer R, Trivedi MH, Teixeira AL. Immune-based strategies for mood disorders: facts and challenges. Expert Rev Neurotherap. (2018) 18:139–52. doi: 10.1080/14737175.2018.1407242
8. Kim YK, Na KS, Myint AM, Leonard BE. The role of pro-inflammatory cytokines in neuroinflammation, neurogenesis and the neuroendocrine system in major depression. Progress Neuro-Psychopharmacol Biol Psychiatry. (2016) 64:277–84. doi: 10.1016/j.pnpbp.2015.06.008
9. Slowik A, Lammerding L, Hoffmann S, Beyer C. Brain inflammasomes in stroke and depressive disorders: regulation by oestrogen. J Neuroendocrinol. (2018) 30:610–21. doi: 10.1111/jne.12482
10. Calcia MA, Bonsall DR, Bloomfield PS, Selvaraj S, Barichello T, Howes OD. Stress and neuroinflammation: a systematic review of the effects of stress on microglia and the implications for mental illness. Psychopharmacology. (2016) 233:1637–50. doi: 10.1007/s00213-016-4218-9
11. Zhu Y, Klomparens EA, Guo S, Geng X. Neuroinflammation caused by mental stress: the effect of chronic restraint stress and acute repeated social defeat stress in mice. Neurol Res. (2019) 41:762–9. doi: 10.1080/01616412.2019.1615670
12. Brebner K, Hayley S, Zacharko R, Merali Z, Anisman H. Synergistic effects of interleukin-1beta, interleukin-6, and tumor necrosis factor-alpha: central monoamine, corticosterone, and behavioral variations. Neuropsychopharmacology. (2000) 22:566–80. doi: 10.1016/S0893-133X(99)00166-9
13. Anisman H, Merali Z. Anhedonic and anxiogenic effects of cytokine exposure. Adv Exp Med Biol. (1999) 461:199–233. doi: 10.1007/978-0-585-37970-8_12
14. Newton C, McHugh S, Widen R, Nakachi N, Klein T, Friedman H. Induction of interleukin-4 (IL-4) by legionella pneumophila infection in BALB/c mice and regulation of tumor necrosis factor alpha, IL-6, and IL-1beta. Infect Immunity. (2000) 68:5234–40. doi: 10.1128/IAI.68.9.5234-5240.2000
15. Wang Y, Mishra A, Brinton RD. Transitions in metabolic and immune systems from pre-menopause to post-menopause: implications for age-associated neurodegenerative diseases. F1000Research. (2020) 9. doi: 10.12688/f1000research.21599.1. [Epub ahead of print].
16. Callan NGL, Mitchell ES, Heitkemper MM, Woods NF. Constipation and diarrhea during the menopause transition and early postmenopause: observations from the Seattle Midlife Women's Health Study. Menopause. (2018) 25:615–24. doi: 10.1097/GME.0000000000001057
17. Sárvári M, Hrabovszky E, Kalló I, Solymosi N, Likó I, Berchtold N, et al. Menopause leads to elevated expression of macrophage-associated genes in the aging frontal cortex: rat and human studies identify strikingly similar changes. J Neuroinfl. (2012) 9:264. doi: 10.1186/1742-2094-9-264
18. Brown CM, Mulcahey TA, Filipek NC, Wise PM. Production of proinflammatory cytokines and chemokines during neuroinflammation: novel roles for estrogen receptors alpha and beta. Endocrinology. (2010) 151:4916–25. doi: 10.1210/en.2010-0371
19. Lewis DK, Johnson AB, Stohlgren S, Harms A, Sohrabji F. Effects of estrogen receptor agonists on regulation of the inflammatory response in astrocytes from young adult and middle-aged female rats. J Neuroimmunol. (2008) 195:47–59. doi: 10.1016/j.jneuroim.2008.01.006
20. Park HJ, Shim HS, Lee S, Hahm DH, Lee H, Oh CT, et al. Anti-stress effects of human placenta extract: possible involvement of the oxidative stress system in rats. BMC Compl Alternat Med. (2018) 18:149. doi: 10.1186/s12906-018-2193-x
21. Paxinos G, Watson C, Pennisi M, Topple A. Bregma, lambda and the interaural midpoint in stereotaxic surgery with rats of different sex, strain and weight. J Neurosci Methods. (1985) 13:139–3. doi: 10.1016/0165-0270(85)90026-3
22. Azizi-Malekabadi H, Hosseini M, Pourganji M, Zabihi H, Saeedjalali M, Anaeigoudari A. Deletion of ovarian hormones induces a sickness behavior in rats comparable to the effect of lipopolysaccharide. Neurol Res Int. (2015) 2015:627642. doi: 10.1155/2015/627642
23. Shepherd JE. Effects of estrogen on congnition mood, and degenerative brain diseases. J Am Pharm Assoc. (2001) 41:221–8. doi: 10.1016/S1086-5802(16)31233-5
24. Buchanan JB, Sparkman NL, Chen J, Johnson RW. Cognitive and neuroinflammatory consequences of mild repeated stress are exacerbated in aged mice. Psychoneuroendocrinology. (2008) 33:755–65. doi: 10.1016/j.psyneuen.2008.02.013
25. You Z, Luo C, Zhang W, Chen Y, He J, Zhao Q, et al. Pro- and anti-inflammatory cytokines expression in rat's brain and spleen exposed to chronic mild stress: involvement in depression. Behav Brain Res. (2011) 225:135–141. doi: 10.1016/j.bbr.2011.07.006
26. Carpenter LL, Gawuga CE, Tyrka AR, Lee JK, Anderson GM, Price LH. Association between plasma IL-6 response to acute stress and early-life adversity in healthy adults. Neuropsychopharmacology. (2010) 35:2617–23. doi: 10.1038/npp.2010.159
27. Miller DB, O'Callaghan JP. Depression, cytokines, and glial function. Metabolism. (2005) 54 (5 Suppl. 1):33–8. doi: 10.1016/j.metabol.2005.01.011
28. Myint AM, Leonard BE, Steinbusch HW, Kim YK. Th1, Th2, and Th3 cytokine alterations in major depression. J Affect Disord. (2005) 88:167–73. doi: 10.1016/j.jad.2005.07.008
29. Piser TM. Linking the cytokine and neurocircuitry hypotheses of depression: a translational framework for discovery and development of novel anti-depressants. Brain Behav Immun. (2010) 24:515–24. doi: 10.1016/j.bbi.2010.02.006
30. Savignac HM, Hyland NP, Dinan TG, Cryan JF. The effects of repeated social interaction stress on behavioural and physiological parameters in a stress-sensitive mouse strain. Behav Brain Res. (2011) 216:576–84. doi: 10.1016/j.bbr.2010.08.049
31. Pavon L, Sandoval-Lopez G, Eugenia Hernandez M, Loria F, Estrada I, Perez M, et al. Th2 cytokine response in Major Depressive Disorder patients before treatment. J Neuroimmunol. (2006) 172:156–65. doi: 10.1016/j.jneuroim.2005.08.014
Keywords: cytokines, depression, motor trigeminal nucleus (MTN), para ventricular nucleus (PVN), ovariectomy
Citation: Park HJ, Shim HS and Shim I (2020) The Differential Role of Cytokines on Stress Responses in a Menopause Rat Model. Front. Psychiatry 11:577561. doi: 10.3389/fpsyt.2020.577561
Received: 27 July 2020; Accepted: 15 October 2020;
Published: 19 November 2020.
Edited by:
Jason H. Huang, Baylor Scott and White Health, United StatesReviewed by:
Hongyu Xu, Virginia Commonwealth University, United StatesJulia Fedotova, Russian Academy of Sciences, Russia
Copyright © 2020 Park, Shim and Shim. This is an open-access article distributed under the terms of the Creative Commons Attribution License (CC BY). The use, distribution or reproduction in other forums is permitted, provided the original author(s) and the copyright owner(s) are credited and that the original publication in this journal is cited, in accordance with accepted academic practice. No use, distribution or reproduction is permitted which does not comply with these terms.
*Correspondence: Insop Shim, ishim@khu.ac.kr