- 1Laboratory of Psychophysiology and Cognitive Neuroscience, Department of Systems Medicine, University of Rome Tor Vergata, Rome, Italy
- 2IRCCS Fondazione Santa Lucia, Rome, Italy
- 3Child Neurology and Psychiatry Unit, Department of Systems Medicine, University of Rome Tor Vergata, Rome, Italy
- 4Psychiatry Unit, Campus Bio-Medico University of Rome, Rome, Italy
- 5Department of Biomedicine and Prevention, University of Rome Tor Vergata, Rome, Italy
- 6Department of Biotechnological and Applied Clinical Sciences, University of L'Aquila, L'Aquila, Italy
The present study investigates the differences in auditory mismatch negativity (MMN) parameters given in a sample of young subjects with autism spectrum disorder (ASD, n = 37) with or without co-occurrent attenuated psychosis syndrome (APS). Our results show that ASD individuals present an MMN decreased amplitude and prolonged latency, without being influenced by concurrent APS. Additionally, when correlating the MMN indexes to clinical features, in the ASD + APS group, we found a negative correlation between the severity of autistic symptoms and the MMN latency in both frequency (f-MMN r = −0.810; p < 0.0001) and duration (d-MMN r = −0.650; p = 0.006) deviants. Thus, our results may provide a more informative characterization of the ASD sub-phenotype when associated with APS, highlighting the need for further longitudinal investigations.
Introduction
Autism spectrum disorder (ASD) is a neurodevelopmental condition characterized by an early onset of social communication deficit associated with a restricted and repetitive pattern of behaviors (1). Since socio-communication impairment is known as a core symptom of ASD, several studies are investigating the role of language difficulties on the central auditory process that, in turn, may be linked to an atypical processing of auditory stimuli, an auditory working memory deficit, and a disrupted sensory acoustic discrimination (2–5).
To better understand the auditory processing in neurodevelopmental disorders, mismatch negativity (MMN) has been widely investigated by basic researches on neurocognitive processes and central auditory and attentional mechanisms (4, 6–9). Specifically, MMN is a negative wave deflection localized in the fronto-central region (10), resulting from the brain's response to infrequent auditory stimuli (deviants) in repetitive stimuli (standards) (6, 11). MMN originates in the auditory and frontal cortex by the central auditory system based on neuronal auditory memory (12) and reflects pre-attentive processes, involving executive functions such as set-shifting ability and working memory (13–15). Thus, researchers have proposed MMN deficit as a marker of auditory processing impairment and consequent disruption in higher cognitive domains (e.g., attentional control and shifting, working memory) (7). Even in the ASD population, MMN has been used to identify auditory processing deficits, with conflicting results (16–18): a recent meta-analysis (17) on the topic shows that most of the available data report an MMN deficit in ASD individuals, suggesting an altered central ability in auditory discrimination within this population; specifically, Chen et al. (17) report shorter MMN latencies in autistic individuals compared to increased MMN latencies in subjects with Asperger syndrome (AS).
Abnormally decreased MMN has also been reported in other psychiatric disorders such as schizophrenia (19–24), especially in the early stages of the illness (7, 25–27). MMN has been widely investigated as a promising biomarker of conversion to psychosis (7, 26) and of remission (28) in individuals at a clinical high risk (CHR) and with attenuated psychosis syndrome (APS). To date, the majority of the available data reports a reduced amplitude of MMN in the at-risk adult population (29). In this context, Bodatsch et al. (30), evaluating the MMN paradigm in a young adult sample of 62 CHR subjects compared to 33 individuals with first-episode schizophrenia and 67 healthy controls (HC), concluded that MMN amplitude was significantly reduced in at-risk subjects who later converted to first-episode psychosis compared to non-converter individuals and to HC.
Despite distinct clinical and electrophysiological features, several studies have focused on the overlap between autistic symptoms and psychotic experiences in both adult and adolescent population (31–33). Longitudinal studies report that 20–50% of individuals with childhood-onset schizophrenia met the criteria for premorbid ASD (34–37). Moreover, a recent meta-analysis confirms that people affected with schizophrenia spectrum disorders (SSDs) show higher autistic symptoms compared to healthy controls (38). Similarly, in ASD individuals, the comorbid rates of SSDs ranging from 0 to 34.8% are reported, with higher rates of transition to SSDs in specific clinical subgroups such as AS and pervasive developmental disorder—not otherwise specified (PDD-NOS), categorized based on the Diagnostic and Statistical Manual of Mental Disorders—Fourth Edition (DSM-IV) (32); this evidence could suggest a possible increased vulnerability in the autistic population (32).
Moreover, it is well-known that individuals with ASD and SSDs share some clinical features, such as social difficulties, language impairment, and common cognitive features (e.g., weak central coherence deficit and set-shifting difficulties) (33, 39, 40).
Thus, in the recent years, research aiming to detect possible cognitive and neurophysiological linkages between these disorders has grown considerably (33, 41–43).
However, despite the evidence of this strong association between ASD and SSDs, to our knowledge, no previous study using a clinical and neurophysiological approach has yet evaluated the co-occurrence of both conditions within a pediatric sample.
The present study aimed to characterize, from a clinical and neurophysiological point of view, a sample of ASD participants (age range: 9–18 years) with attenuated psychosis syndrome (ASD + APS) in comparison with ASD patients without APS (ASD) and with healthy control (HC) groups, pointing to better defining the ASD phenotype when associated with other psychiatric conditions such as APS.
Materials and Methods
Participants
A total of 40 ASD individuals (age range: 9–18 years; age median: 13.67; eight females and 32 males), according to Diagnostic and Statistical Manual of Mental Disorders—Fifth Edition (DSM-5) criteria (1), were recruited from the Children Psychiatry Unit of the University Hospital Tor Vergata of Rome between January 2018 and July 2019.
A healthy control (HC) group of 20 individuals (age range: 9–18 years; age median: 13.04; eight females and 12 males), voluntarily recruited from a sport club, was also included.
The present study was approved by the Ethical Committee of our University Hospital, Fondazione Policlinico Tor Vergata (Register number 126/18), and informed consent was obtained from all legal holders of custody of both ASD and HC groups.
The adopted exclusion criteria were the presence of syndromic autism, intellectual quotient (IQ) equal or below 70, non-fluent speech, epilepsy, and other concurrent psychiatric or neurodevelopmental conditions (e.g., obsessive–compulsive disorder, attention deficit and hyperactivity disorder).
All participants underwent a clinical evaluation and electroencephalogram (EEG) to evaluate the MMN paradigm.
From the initial sample of 40 ASD individuals, three did not complete the clinical assessment and dropped out of the study. The final sample of 57 participants (37 ASD + 20 HC) was divided in three groups: ASD (n = 21), ASD + APS (n = 16), and HC (n = 20).
Clinical Assessment
All ASD individuals underwent a cognitive evalution to assess IQ. Depending on the age and on each individual's ability to cooperate, the Leiter International Performance Scale—Revised (Leiter-R) (44), the Wechsler Intelligence Scale for Children—Fourth Edition (WISC-IV) (45), or the Wechsler Adult Intelligence Scale—Revised (WAIS-R) (46) was performed.
ASD diagnosis was based on the DSM-5 criteria (1). All participants underwent the Autism Diagnostic Observation Schedule—Second Edition (ADOS-2) test (47), performed by a licensed clinician. The ADOS-2, a semi-structured observational assessment of autistic symptoms, includes five modules based on expressive language level and age. The ADOS-2 algorithm is organized in social affect (SA), restricted and repetitive behaviors (RRB), and the total score. Modules 1, 2, and 3 provide the calibrated severity score (CSS), ranging from 1 to 10, indicating a measure of autism severity level. By contrast, even if a recent revision of the algorithm for module 4 is now available (48), this has not been applied yet to the Italian population. Thus, based on age, language, and adaptive abilities, all ASD subjects were administered module 3, being in line with the ADOS-2 manual (47) which allows clinicians and researchers to choose module 3 even for adolescents over 16 years of age in order to permit a comparison between CSS scores in the ASD sample (47, 49).
Finally, to evaluate the presence of a concurrent APS condition, the Structured Interview for Psychosis-Risk Syndromes (SIPS) was administered to all ASD individuals (50–52). In this context, the proposed “Attenuated Psychosis Syndrome (APS)” DSM-5 diagnosis refers to a condition characterized by the recent onset of sub-threshold psychotic symptoms associated to a higher risk of conversion to psychotic disorder within the next year (53–55).
According to a previous study (56), the presence of an APS condition was confirmed when a score of 3 or 4 of 5 was obtained at the SIPS positive symptoms scale (SIPS-P) (51, 55) (see Supplementary Figure 1 for SIPS-P score distributions in ASD and ASD + APS).
Moreover, the absence of a family history of schizophrenia was confirmed by the administration of a clinical interview.
Finally, the HC underwent a screening clinical evaluation of IQ [based on age, Raven Matrices colored (age < 11 years) or progressive (age > 11 years) (57)] and of behavioral problems (Child Behavior Checklist 6–18 years—CBCL 6–18 years) (58). Moreover, a clinical interview was performed by a child and adolescent psychiatrist in order to exclude the presence of autistic or psychotic symptoms in this population.
All the HC individuals presented normal cognitive function (IQ assessed with Raven Matrices above 25–50° centile for colored form and above 85 for the progressive form) and resulted negative for the presence of any behavioral problem or psychiatric condition.
Neurophysiological Evaluation
All subjects (ASD, ASD + APS, and HC) underwent a 64-channel EEG recorded while listening to a passive auditory paradigm and watching a silent video. The MMN waveforms to frequency-deviant tones (f-MMN) and duration-deviant tones (d-MMN) were obtained for the Fz channel. The MMN peak latency and peak amplitude were measured. MMN is, in fact, described by latency, which is the timing of the negative peak in different waveforms, and amplitude, which represents the average response on the negative peak, reflecting attentive cortical processes (4).
The EEG was recorded at 2,048 Hz with an EBNeuro 72-channel EEG system using sintered Ag/Ag-Cl electrodes in an electrode cap with 61 standard scalp sites and two single Ag/AgCl disk electrodes (1 mm in diameter) over the left and the right mastoids, with the reference electrode placed between AFz and Fz and the ground electrode between CPz and Pz; one polygraphic channel was also recorded to monitor vertical and horizontal eye movements, with the active electrode placed 1 cm lateral to the outer canthus of the right eye and the reference electrode placed on the lower eyelid. The electrode impedances were maintained below 5 kΩ.
Stimuli and Tasks
The auditory paradigm consisted of 2,000 standard tones (1,000 Hz, 50 ms), 200 frequency-deviant tones (1,200 Hz, 50-ms duration, 5-ms rise/fall), and 200 duration-deviant tones (1,000 Hz, 100 ms, 10-ms rise/fall), for a total of 2,400 tones. All tones were presented binaurally through Sennheiser HD25 SP II at 80 dB SPL with an interstimulus interval of 500 ms and in random order with the constraint that a deviant tone was preceded at minimum by three standard tones. During the auditory paradigm, the subjects were invited to watch a silent cartoon and to ignore the tones that they heard; at the end of the session, questions on the cartoon were asked to the participants in order to check the level of attention in watching the video.
MMN Analysis
The EEG data were processed offline using EEGLAB in MATLAB environment. The continuous EEG recordings were digitally filtered 1 to 20 Hz, re-referenced to the algebraic average of the left and the right mastoids, and segmented from −100 to 400 ms relative to the stimulus onset. Epochs with artifacts exceeding ±100 μV were excluded. Average ERPs were computed from the artifact-free epochs and baseline-corrected with a prestimulus baseline of −100 to 0 ms.
The frequency and duration MMNs were measured at Fz channel as an average amplitude under within 100 to 200 ms (frequency) and 150 to 250 ms (duration) post-stimulus onset in the different waveform (deviant–standard), respectively.
Statistical Analyses
Data were presented as means (SD) and frequencies (percentages). Differences in age and gender between HC, ASD, and ASD + APS were investigated, respectively, using one-way analysis of variance (ANOVA) and Pearson's chi-square test (χ2). Comparisons between ASD and ASD + APS in ADOS and SIPS measures were performed with Student's t-test.
Explorative data analysis with the Kolmogorov–Smirnov test showed that the MMN indices had a normal distribution (consistently, p > 0.20). ANOVA models were used to measure the difference between HC, ASD, and ASD + APS in d-MMN and f-MMN latencies and amplitudes. Post-hoc tests were performed with Bonferroni confidence interval adjustment for multiple comparisons to define which variables contributed to the major effects.
Explorative correlation analysis was performed in order to investigate the relation between MMN and clinical (ADOS and SIPS) indices. Statistical significance was set at p-values < 0.05.
Results
Demographic and Clinical Data
Age, sex, IQ, ADOS-2, and SIPS subscale scores are summarized in Table 1.
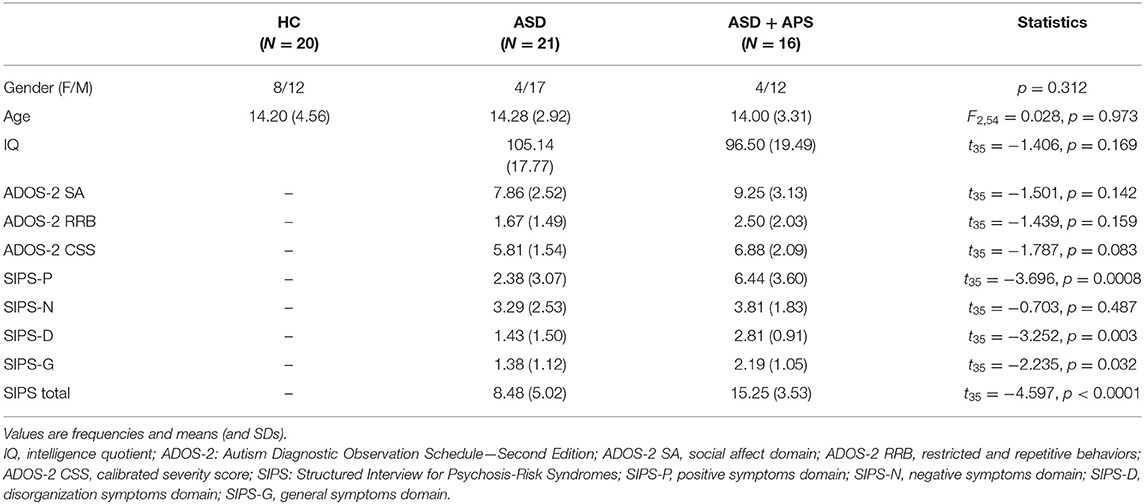
Table 1. Descriptive and univariate statistics of sociodemographic and clinical characteristics in healthy controls (HC) and autism spectrum disorder (ASD) and ASD + attenuated psychosis syndrome (ASD+APS) patients.
No significant differences emerged in terms of age [ASD vs. HC: 14.16 (3.05) vs. 14.20 (4.56); t55 = −0.040, p = 0.969] and sex ( = 2.172, p = 0.141). Moreover, within the ASD sample, no differences in terms of IQ came out (see Supplementary Figure 2).
At the clinical level, the ASD + APS group exhibits a higher level of psychotic symptoms as assessed by the SIPS interview compared to the ASD (SIPS total, p < 0.0001). Specifically, compared to the ASD, in the ASD + APS group, we observe a higher value of positive (SIPS-P, p = 0.001), disorganized (SIPS-D, p = 0.002), and general psychotic symptoms (SIPS-G, p = 0.031).
However, no relevant differences emerged between the two groups in terms of negative symptoms (SIPS-N, p = 0.468) and autistic features measured by the ADOS-2 test (ADOS-2 CSS, p = 0.098).
MMN Component Characteristics
Compared to HC, the whole ASD group showed significantly less negative values of d-MMN amplitude [−4.40 (2.02) vs. −6.72 (1.70); t55 = 4.355, p < 0.0001] as well as longer f-MMN latency [173.76 (29.50) vs. 153.80 (13.13); t55 = 2.867, p = 0.006] and less negative values of f-MMN amplitude [−3.43 (1.88) vs. −4.80 (1.33); t55 = 2.900, p = 0.005] (Table 2; Figure 1).
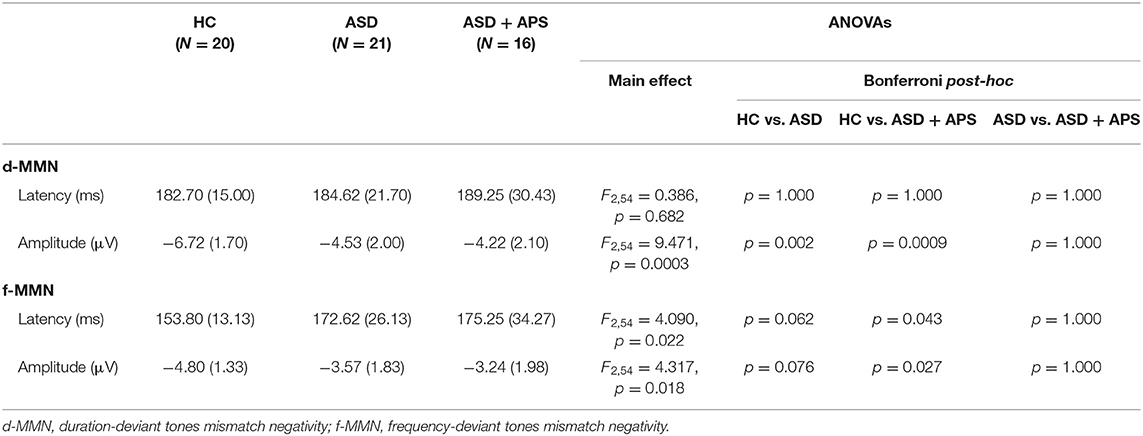
Table 2. Descriptive and univariate statistics of dMMN and fMMN latency and amplitude in healthy controls (HC) and autism spectrum disorder (ASD) and ASD + attenuated psychosis syndrome (ASD+APS) patients.
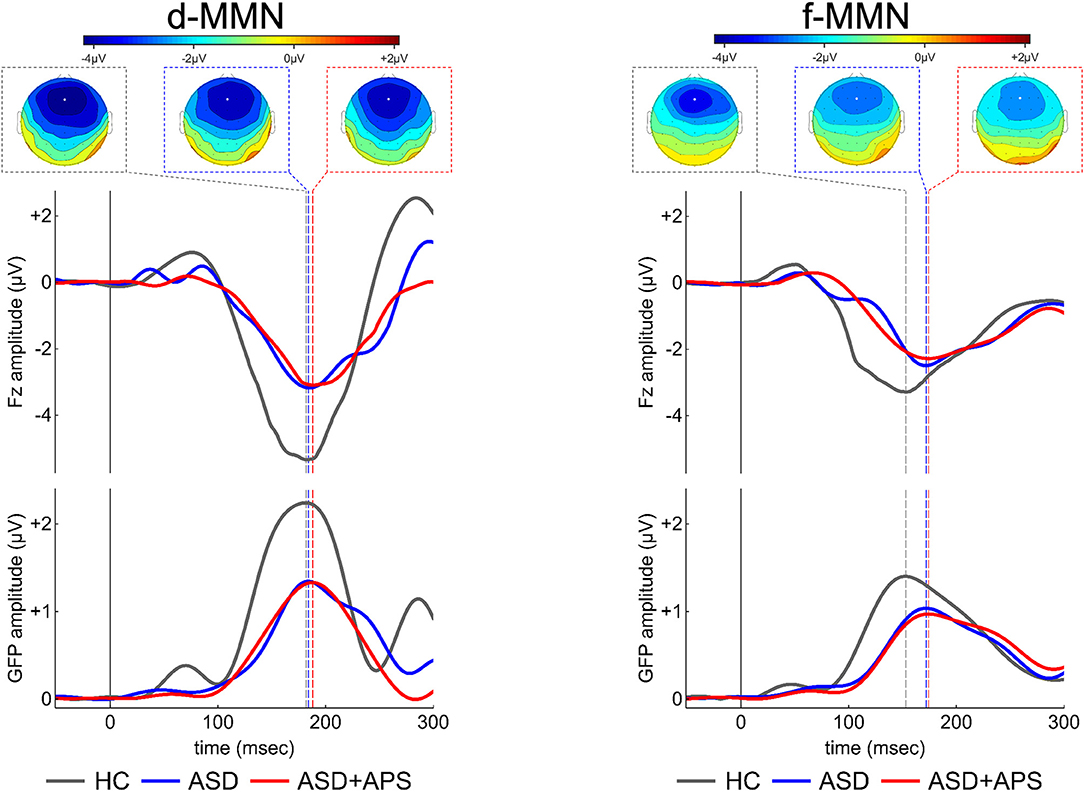
Figure 1. Electrical scalp map topographies (for each group, at the MMN grandaverage peak; upper panels portion), grandaverage waveforms at Fz channel (middle panels portion) and Global Field Power (GFP, a global measure of electrical field strength; middle panels portion) in the three groups (ASD, ASD+APS, and HC) for each condition, d-MMN (left panel) and f-MMN (right panel). As expected, MMN has a fronto-central distribution over the scalp midline, with strongest electrical field responses around Fz channel (indicated as a white circle in the scalp map images). ASD: Autism Spectrum disorder; ASD+APS: Autism Spectrum Disorder + Attenuated Psychosis Syndrome; HC: Healthy Controls.
No difference between ASD and HC in d-MMN latency [186.62 (25.55) vs. 182.70 (15.00); t55 = 0.629, p = 0.532] came out.
The ANOVA models showed that the three groups were different in d-MMN amplitudes and f-MMN latencies and amplitudes. Specifically, the post-hoc analysis showed that ASD and ASD + APS had higher values in latencies and lower values in amplitudes with respect to HC. However, in terms of MMN indexes, no significant differences came out between the ASD and the ASD + APS groups (Table 2). Moreover, including the IQ as a covariate, no significant differences emerged between the two groups (see Supplementary Tables 1, 2).
Correlation Between MMN Component Characteristics and Clinical Data
Correlation coefficients, with Bonferroni-adjusted P-values (p-value threshold / number of comparisons = 0.05/36 = 0.0013889 = 0.0014), were computed between d-MMN and f-MMN amplitude and latency indices and ADOS-2 and SIPS subscale scores separately in the ASD and the ASD + APS groups (Table 3).
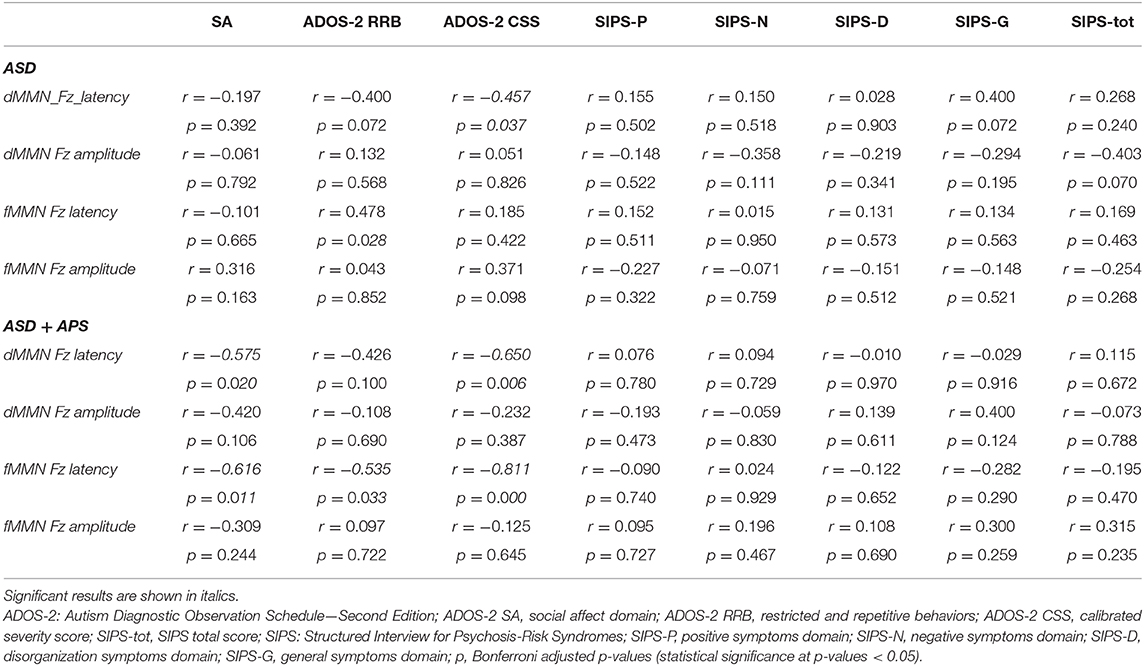
Table 3. Results of correlation analysis between mismatch negativity indices and clinical phenotype in autism spectrum disorder (ASD) and ASD + attenuated psychosis syndrome (ASD+APS) groups are reported.
Specifically, in the ASD group (Table 3), we found a negative correlation between d-MMN latency and ADOS-2 CSS score (r = −0.457; p = 0.037) and a positive correlation between f-MMN and ADOS-2 RRB (r = 0.478; p = 0.028).
In the ASD + APS group (Table 3), applying Bonferroni correction (p-value threshold / number of comparisons = 0.05/36 = 0.0013889 = 0.0014), a strong negative correlation emerged between f-MMN latency and ADOS-2 CSS (r = −0.811; p < 0.0001), RRB (r = −0.535; p = 0.033), and SA (r = −0.616; p = 0.011) and between d-MMN latency and ADOS-2 CSS (r = −0.650; p = 0.006) and ADOS-2 SA (r = −0.575; p = 0.020), demonstrating that a higher level of autistic symptoms was linked to reduced MMN latencies (Figure 2).
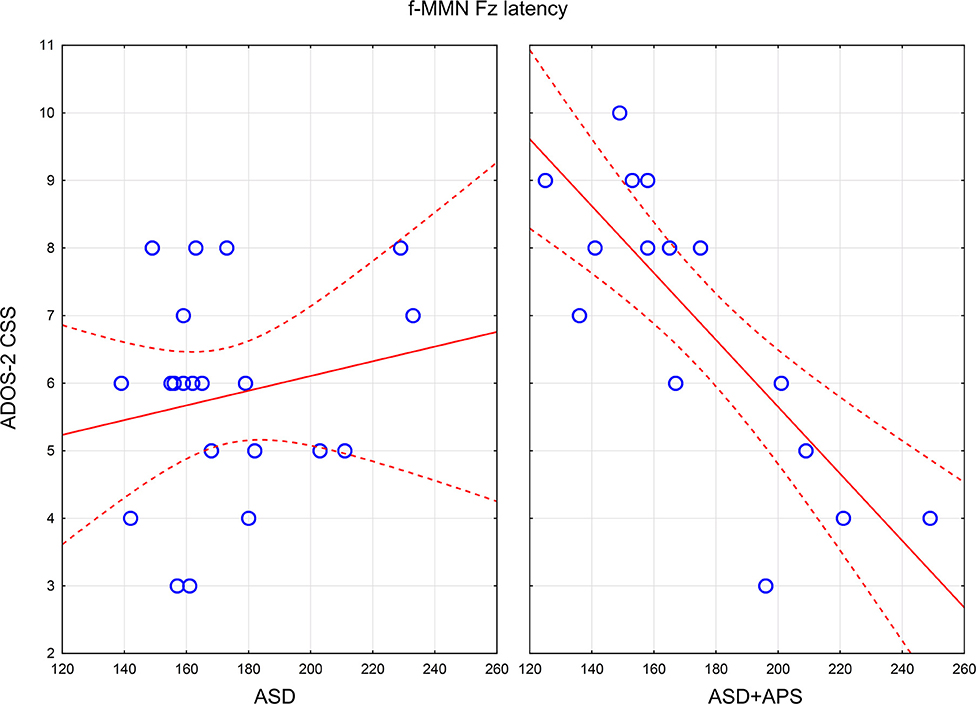
Figure 2. Scatterplots of relations between f-MMN latency and ADOS-2 CSS in ASD (left panel) and ASD+APS (right panel). In the panels, the continuous lines are the linear regression lines, the dashed lines indicate the 95% Confidence Intervals for mean, and the empty circles represent the value for each autistic subject. ADOS-2 CSS: Autism Diagnostic Observation ScheduleSecond Edition, calibrated severity score; ASD: Autism Spectrum disorder; ASD+APS: Autism Spectrum Disorder + Attenuated Psychosis Syndrome; f-MMN: frequency-deviant tones mismatch negativity.
No significant correlation emerged between MMN amplitude and latency parameters to SIPS scores in both groups (ASD and ASD + APS).
Discussion
MMN has been well-established as a good reliable marker for pre-attentional mechanism. However, during the past years, MMN has been separately studied in the autistic (16, 17) and in the at-risk psychotic population (20, 21, 59), and findings of the concurrent presence of both ASD and APS have not been reported so far. Thus, in the present study, we aimed to investigate differences in MMN features concerning both frequency and duration deviants in a pediatric sample of ASD participants with or without co-occurrent APS compared to a healthy control group.
Being in line with a recent meta-analysis on the topic by Chen et al. (17), showing a reduced amplitude in response to non-speech sound deviants in the ASD population, our results support the evidence that young ASD individuals present a different pattern of pre-attentional processes measured as MMN amplitude indexes compared to HC. By contrast, our data demonstrate a prolonged MMN latency in response to tone–frequency deviants in the whole ASD group, as reported in AS individuals. As is known, previous research also suggests a key role of the brain temporal regions in either processing language and social cognition abilities (60–62) or auditory memory processes in ASD individuals (63). Taking these results that refer to available studies together, our findings yield the knowledge that ASD individuals present a significant impairment in pre-attentive temporal auditory processing (64), showing a slower response in the sound duration identification task (65).
Additionally, we have evaluated the MMN paradigm not only in ASD participants but also in individuals with co-occurrent APS in order to investigate whether the presence of concurrent attenuated psychotic symptoms could influence the pre-attentive pattern in this “at-risk population.” Our results show that, when an APS condition is associated to ASD, no significant differences emerged in terms of MMN indexes, leading to the hypothesis that, in autistic individuals, the presence of a concurrent APS condition does not significantly impair the pre-attentive temporal auditory process measured at MMN.
Furthermore, to better investigate the differences between the two groups (ASD and ASD + APS) and the impact of the clinical phenotype, we analyzed the correlations between MMN indexes to both autistic and psychotic symptoms. Our results demonstrate a significant correlation between electrophysiological indexes, referred to MMN latencies, and autistic symptoms level. Specifically, in the ASD group, we found a negative correlation between d-MMN latency and ADOS-2 CSS score, with reduced latency in response to duration deviants linked to a higher autistic symptom level. In the ASD + APS group, we noticed an even stronger negative correlation between ADOS-2 CSS score and both d-MMN and f-MMN latency indexes, with reduced MMN latency associated with a higher level of autistic symptoms. No significant correlations to attenuated psychotic symptoms measured by the SIPS interview came out. Interestingly, it is known that a shorter MMN latency, generated by both frequency and duration tone deviants, is described in individuals affected with schizophrenia (66–68) and in those considered at risk for psychosis (59), without a significant correlation to the severity of the psychotic symptoms (68). Thus, from a neurobiological perspective, if the earlier peak of MMN could reflect temporal responsiveness in the auditory cortex, both in ASD and ASD + APS populations (69), from a clinical point of view, the presence of a strong negative correlation between the latency indexes in both deviants (d-MMN and f-MMN) to the severity of autistic symptoms in the ASD + APS group could describe a greater clinical impairment in this population, especially referring to autistic symptoms. Moreover, given that other studies (21, 70–72) reported that different MMN latency features, in both d-MMN and f-MMN, could be a possible biomarker in different stages of psychotic symptoms, it is crucial to further investigate whether the duration of the illness could impact on the correlation between MMN features and clinical phenotype. Thus, the next step of this research study will be the differentiation, in terms of MMN latency parameters, between ASD + APS subjects who would convert to psychosis and those who would not.
Although in ASD individuals it is still challenging to translate neurophysiological indexes into clinically prognostic features, understanding the role of MMN as a possible biomarker of psychotic symptom progression could help clinicians to better describe ASD individuals at risk for psychosis. In this context, our findings start to provide a more informative characterization of the ASD sub-phenotype by a dimensional approach and a better evaluation of illness progression within individuals considered “at-risk” for developing psychotic disorders. This knowledge might consequently lead to an optimal management of the therapeutic intervention in terms of choice, timing, and duration.
Thus, despite some strengths such as the clinically well-described ASD sample and the inclusion of young individuals, our study presents some limitations that should be taken into account when interpreting our data. Firstly, the sample size. Even if our study is in line with the majority of available studies aimed to assess MMN in ASD individuals (17), the sample size is relatively small if compared to other clinical studies [see Foss-Feig et al. (73)]. Secondly, the age range (9–18 years). Indeed even if including young individuals in the sample is a strength of the study, allowing to assess the presence of psychosis risk at a very early prodromal phase (74), the exclusion of individuals aged over 18 years old does not permit to explore the possible onset of psychotic symptoms in the ASD group at that point. Moreover, the evaluation of psychotic symptoms in young autistic individuals with cognitive borderline functioning could be challenging, even for trained clinicians. Besides, the lack of information about the age onset of psychotic symptoms in ASD individuals before our assessment is a limitation. Furthermore, the lack of an APS control group without ASD, in order to better evaluate the significance of our results in the ASD + APS group, is another limiting factor. Finally, it is important to highlight a possible recruitment bias in the fact that we included all participants with well-established compliance. This strongly highlights the need for further investigations, especially through longitudinal studies, in order to better evaluate the possible relevance and the prognostic meaning of the MMN preattentive patterns deficit in ASD individuals at risk for psychosis.
Data Availability Statement
The data that support the finding of this study are available on request from the corresponding authors, GDL and LM.
Ethics Statement
The studies involving human participants were reviewed and approved by Ethical Committee of our University Hospital, Fondazione Policlinico Tor Vergata, Rome (Register number 126/18). Written informed consent to participate in this study was provided by the participants' legal guardian/next of kin.
Author Contributions
LM, GDL, and MR conceived and designed the present study. AR and MS evaluated autism spectrum disorder and assessed the symptom severity. MR evaluated the psychotic symptoms. GDL performed the electrophysiological recordings and analyzed the data. GDL, AR, and MS wrote the manuscript, and LM and PC substantially revised the manuscript. All the authors contributed to the writing of the manuscript.
Conflict of Interest
The authors declare that the research was conducted in the absence of any commercial or financial relationships that could be construed as a potential conflict of interest.
Acknowledgments
We thank those people who generously gave their time to participate in this study.
Supplementary Material
The Supplementary Material for this article can be found online at: https://www.frontiersin.org/articles/10.3389/fpsyt.2020.555340/full#supplementary-material
References
1. American Psychiatric Association. Diagnostic and Statistical Manual of Mental Disorders. 5th ed. Washington, DC: American Psychiatric Association Publishing (2013). doi: 10.1176/appi.books.9780890425596
2. Anderson S, Kraus N. Sensory-cognitive interaction in the neural encoding of speech in noise: a review. J Am Acad Audiol. (2010) 21:575–85. doi: 10.3766/jaaa.21.9.3
3. Kwakye LD, Foss-Feig JH, Cascio CJ, Stone WL, Wallace MT. Altered auditory and multisensory temporal processing in autism spectrum disorders. Front Integr Neurosci. (2011) 4:129. doi: 10.3389/fnint.2010.00129
4. Naatanen R, Kujala T, Escera C, Baldeweg T, Kreegipuu K, Carlson S, et al. The mismatch negativity (MMN)–a unique window to disturbed central auditory processing in ageing and different clinical conditions. Clin Neurophysiol. (2012) 123:424–58. doi: 10.1016/j.clinph.2011.09.020
5. O'Connor K. Auditory processing in autism spectrum disorder: a review. Neurosci Biobehav Rev. (2012) 36:836–54. doi: 10.1016/j.neubiorev.2011.11.008
6. Naatanen R, Paavilainen P, Rinne T, Alho K. The mismatch negativity (MMN) in basic research of central auditory processing: a review. Clin Neurophysiol. (2007) 118:2544–90. doi: 10.1016/j.clinph.2007.04.026
7. Naatanen R, Sussman ES, Salisbury D, Shafer VL. Mismatch negativity (MMN) as an index of cognitive dysfunction. Brain Topogr. (2014) 27:451–66. doi: 10.1007/s10548-014-0374-6
8. Yamamuro K, Ota T, Iida J, Nakanishi Y, Kishimoto N, Kishimoto T. Associations between the mismatch-negativity component and symptom severity in children and adolescents with attention deficit/hyperactivity disorder. Neuropsych Dis Treat. (2016) 12:3183–90. doi: 10.2147/NDT.S120540
9. Rydkjaer J, Jepsen JRM, Pagsberg AK, Fagerlund B, Glenthoj BY, Oranje B. Mismatch negativity and P3a amplitude in young adolescents with first-episode psychosis: a comparison with ADHD. Psychol Med. (2017) 47:377–88. doi: 10.1017/S0033291716002518
10. Garrido MI, Kilner JM, Stephan KE, Friston KJ. The mismatch negativity: a review of underlying mechanisms. Clin Neurophysiol. (2009) 120:453–63. doi: 10.1016/j.clinph.2008.11.029
11. Kujala T, Tervaniemi M, Schroger E. The mismatch negativity in cognitive and clinical neuroscience: theoretical and methodological considerations. Biol Psychol. (2007) 74:1–19. doi: 10.1016/j.biopsycho.2006.06.001
12. Naatanen R, Astikainen P, Ruusuvirta T, Huotilainen M. Automatic auditory intelligence: an expression of the sensory-cognitive core of cognitive processes. Brain Res Rev. (2010) 64:123–36. doi: 10.1016/j.brainresrev.2010.03.001
13. Jacobsen T, Schroger E. Is there pre-attentive memory-based comparison of pitch? Psychophysiology. (2001) 38:723–7. doi: 10.1111/1469-8986.3840723
14. Naatanen R, Jacobsen T, Winkler I. Memory-based or afferent processes in mismatch negativity (MMN): a review of the evidence. Psychophysiology. (2005) 42:25–32. doi: 10.1111/j.1469-8986.2005.00256.x
15. Maess B, Jacobsen T, Schroger E, Friederici AD. Localizing pre-attentive auditory memory-based comparison: magnetic mismatch negativity to pitch change. Neuroimage. (2007) 37:561–71. doi: 10.1016/j.neuroimage.2007.05.040
16. Schwartz S, Shinn-Cunningham B, Tager-Flusberg H. Meta-analysis and systematic review of the literature characterizing auditory mismatch negativity in individuals with autism. Neurosci Biobehav R. (2018) 87:106–17. doi: 10.1016/j.neubiorev.2018.01.008
17. Chen TC, Hsieh MH, Lin YT, Chan PYS, Cheng CH. Mismatch negativity to different deviant changes in autism spectrum disorders: a meta-analysis. Clin Neurophysiol. (2020) 131:766–77. doi: 10.1016/j.clinph.2019.10.031
18. Ruiz-Martínez FJ, Rodríguez-Martínez EI, Wilson CE, Yau S, Saldaña D, Gómez CM. Impaired P1 habituation and mismatch negativity in children with autism spectrum disorder. J Autism Dev Disord. (2020) 50:603–16. doi: 10.1007/s10803-019-04299-0
19. Light GA, Braff DL. Mismatch negativity deficits are associated with poor functioning in schizophrenia patients. Arch Gen Psychiat. (2005) 62:127–36. doi: 10.1001/archpsyc.62.2.127
20. Umbricht D, Krljes S. Mismatch negativity in schizophrenia: a meta-analysis. Schizophr Res. (2005) 76:1–23. doi: 10.1016/j.schres.2004.12.002
21. Nagai T, Tada M, Kirihara K, Yahata N, Hashimoto R, Araki T, et al. Auditory mismatch negativity and P3a in response to duration and frequency changes in the early stages of psychosis. Schizophr Res. (2013) 150:547–54. doi: 10.1016/j.schres.2013.08.005
22. Baldeweg T, Hirsch SR. Mismatch negativity indexes illness-specific impairments of cortical plasticity in schizophrenia: a comparison with bipolar disorder and Alzheimer's disease. Int J Psychophysiol. (2015) 95:145–55. doi: 10.1016/j.ijpsycho.2014.03.008
23. Haigh SM, Coffman BA, Salisbury DF. Mismatch negativity in first-episode schizophrenia: a meta-analysis. Clin EEG Neurosci. (2017) 48:3–10. doi: 10.1177/1550059416645980
24. Fisher DJ, Rudolph ED, Ells EML, Knott VJ, Labelle A, Tibbo PG. Mismatch negativity-indexed auditory change detection of speech sounds in early and chronic schizophrenia. Psychiat Res-Neuroim. (2019) 287:1–9. doi: 10.1016/j.pscychresns.2019.03.010
25. Todd J, Michie PT, Schall U, Karayanidis F, Yabe H, Naatanen R. Deviant matters: duration, frequency, and intensity deviants reveal different patterns of mismatch negativity reduction in early and late schizophrenia. Biol Psychiat. (2008) 63:58–64. doi: 10.1016/j.biopsych.2007.02.016
26. Naatanen R, Todd J, Schall U. Mismatch negativity (MMN) as biomarker predicting psychosis in clinically at-risk individuals. Biol Psychol. (2016) 116:36–40. doi: 10.1016/j.biopsycho.2015.10.010
27. Kim M, Lee TH, Yoon YB, Lee TY, Kwon JS. Predicting remission in subjects at clinical high risk for psychosis using mismatch negativity. Schizophrenia Bull. (2018) 44:575–83. doi: 10.1093/schbul/sbx102
28. de Wit S, Schothorst PF, Oranje B, Ziermans TB, Durston S, Kahn RS. Adolescents at ultra-high risk for psychosis: long-term outcome of individuals who recover from their at-risk state. Eur Neuropsychopharm. (2014) 24:865–73. doi: 10.1016/j.euroneuro.2014.02.008
29. Naatanen R, Shiga T, Asano S, Yabe H. Mismatch negativity (MMN) deficiency: a break-through biomarker in predicting psychosis onset. Int J Psychophysiol. (2015) 95:338–44. doi: 10.1016/j.ijpsycho.2014.12.012
30. Bodatsch M, Ruhrmann S, Wagner M, Muller R, Schultze-Lutter F, Frommann I, et al. Prediction of psychosis by mismatch negativity. Biol Psychiat. (2011) 69:959–66. doi: 10.1016/j.biopsych.2010.09.057
31. Cochran DM, Dvir Y, Frazier JA. “Autism-plus” spectrum disorders intersection with psychosis and the schizophrenia spectrum. Child Adolesc Psychiatr Clin N Am. (2013) 22:609–27. doi: 10.1016/j.chc.2013.04.005
32. Chisholm K, Lin A, Abu-Akel A, Wood SJ. The association between autism and schizophrenia spectrum disorders: a review of eight alternate models of co-occurrence. Neurosci Biobehav R. (2015) 55:173–83. doi: 10.1016/j.neubiorev.2015.04.012
33. Kincaid DL, Doris M, Shannon C, Mulholland C. What is the prevalence of autism spectrum disorder and ASD traits in psychosis? A systematic review Psychiat Res. (2017) 250:99–105. doi: 10.1016/j.psychres.2017.01.017
34. Leyfer OT, Folstein SE, Bacalman S, Davis NO, Dinh E, Morgan J, et al. Comorbid psychiatric disorders in children with autism: interview development and rates of disorders. J Autism Dev Disord. (2006) 36:849–61. doi: 10.1007/s10803-006-0123-0
35. Rapoport J, Chavez A, Greenstein D, Addington A, Gogtay N. Autism spectrum disorders and childhood-onset schizophrenia: clinical and biological contributions to a relation revisited. J Am Acad Child Adolesc Psychiatry. (2009) 48:10–8. doi: 10.1097/CHI.0b013e31818b1c63
36. Jones RB, Thapar A, Lewis G, Zammit S. The association between early autistic traits and psychotic experiences in adolescence. Schizophr Res. (2012) 135:164–9. doi: 10.1016/j.schres.2011.11.037
37. Sullivan S, Rai D, Golding J, Zammit S, Steer C. The association between autism spectrum disorder and psychotic experiences in the avon longitudinal study of parents and children (ALSPAC) birth cohort. J Am Acad Child Adolesc Psychiatry. (2013) 52:806–14. doi: 10.1016/j.jaac.2013.05.010
38. De Crescenzo F, Postorino V, Siracusano M, Riccioni A, Armando M, Curatolo P, et al. (2019). Autistic symptoms in schizophrenia spectrum disorders: a systematic review and meta-analysis. Front Psychiatry. 10:78. doi: 10.3389/fpsyt.2019.00078
39. Larson FV, Wagner AP, Jones PB, Tantam D, Lai MC, Baron-Cohen S, et al. Psychosis in autism: comparison of the features of both conditions in a dually affected cohort. Brit J Psychiatry. (2017) 210:269–75. doi: 10.1192/bjp.bp.116.187682
40. Warrier V, Toro R, Chakrabarti B, Borglum AD, Grove J, Hinds DA, et al. Genome-wide analyses of self-reported empathy: correlations with autism, schizophrenia, and anorexia nervosa. Transl Psychiatry. (2018) 8:35. doi: 10.1038/s41398-017-0082-6
41. Gadow KD, DeVincent CJ. Comparison of children with autism spectrum disorder with and without schizophrenia spectrum traits: gender, season of birth, and mental health risk factors. J Autism Dev Disord. (2012) 42:2285–96. doi: 10.1007/s10803-012-1473-4
42. Gadow KD. Association of schizophrenia spectrum and autism spectrum disorder (ASD) symptoms in children with ASD and clinic controls. Res Dev Disabil. (2013) 34:1289–99. doi: 10.1016/j.ridd.2013.01.011
43. Zheng Z, Zheng P, Zou XB. Association between schizophrenia and autism spectrum disorder: a systematic review and meta-analysis. Autism Res. (2018) 11:1110–9. doi: 10.1002/aur.1977
44. Roid G, Miller L. Leiter International Performance Scale-Revised: Examiner's Manual. Wood Dale, IL: Stoelting (1997).
45. Wechsler D. Wechsler Intelligence Scale for Children. 4th ed. San Antonio, TX: The Psychological Corporation (2003). doi: 10.1037/t15174-000
46. Wechsler D. WAIS-R Manual: Wechsler Adult Intelligence Scale-Revised. New York, NY: Psychological Corporation (1981).
47. Lord C, Rutter M, DiLavore PC, Risi S, Luyster RJ, Gotham K, et al. Autism Diagnostic Observation Schedule, Second Edition (ADOS-2). Torrance, CA: Western Psychological Services (2012).
48. Hus V, Lord C. The autism diagnostic observation schedule, module 4: revised algorithm and standardized severity scores. J Autism Dev Disord. (2014) 44:1996–2012. doi: 10.1007/s10803-014-2080-3
49. Luyster R, Gotham K, Guthrie W, Coffing M, Petrak R, Pierce K, et al. The autism diagnostic observation schedule-toddler module: a new module of a standardized diagnostic measure for autism spectrum disorders. J Autism Dev Disord. (2009) 39:1305–20. doi: 10.1007/s10803-009-0746-z
50. Miller TJ, McGlashan TH, Rosen JL, Somjee L, Markovich PJ, Stein K, et al. Prospective diagnosis of the initial prodrome for schizophrenia based on the structured interview for prodromal syndromes: preliminary evidence of interrater reliability and predictive validity. Am J Psychiatry. (2002) 159:863–5. doi: 10.1176/appi.ajp.159.5.863
51. Miller TJ, McGlashan TH, Rosen JL, Cadenhead K, Cannon T, Ventura J, et al. Prodromal assessment with the structured interview for prodromal syndromes and the scale of prodromal symptoms: predictive validity, interrater reliability, and training to reliability. Schizophrenia Bull. (2004) 29:703–15. doi: 10.1093/oxfordjournals.schbul.a007040
52. McGlashan TWB, Woods S. The Psychosis-Risk Syndrome: Handbook for Diagnosis and Follow-up. Oxford: Oxford University (2010).
53. Woods SW, Walsh BC, Saksa JR, McGlashan TH. The case for including attenuated psychotic symptoms syndrome in DSM-5 as a psychosis risk syndrome. Schizophr Res. (2010) 123:199–207. doi: 10.1016/j.schres.2010.08.012
54. Tsuang MT, Van Os J, Tandon R, Barch DM, Bustillo J, Gaebel W, et al. Attenuated psychosis syndrome in DSM-5. Schizophr Res. (2013) 150:31–5. doi: 10.1016/j.schres.2013.05.004
55. de Pablo GS, Catalan A, Fusar-Poli P. Clinical validity of DSM-5 attenuated psychosis syndrome advances in diagnosis, prognosis, and treatment. Jama Psychiatry. (2020) 77:311–20. doi: 10.1001/jamapsychiatry.2019.3561
56. Ribolsi M, Lin A, Wardenaar KJ, Pontillo M, Mazzone L, Vicari S, et al. Clinical presentation of attenuated psychosis syndrome in children and adolescents: is there an age effect? Psychiat Res. (2017) 252:169–74. doi: 10.1016/j.psychres.2017.02.050
58. Achenbach TM, Rescorla LA. Manual for ASEBA Preschool Forms and Profiles. Burlington, VT: University of Vermont, Research Center for Children, Youth, and Families (2000).
59. Lepock JR, Mizrahi R, Korostil M, Bagby RM, Pang EW, Kiang M. Event-related potentials in the clinical high-risk (CHR) state for psychosis: a systematic review. Clin EEG Neurosci. (2018) 49:215–25. doi: 10.1177/1550059418755212
60. Bigler ED, Mortensen S, Neeley ES, Ozonoff S, Krasny L, Johnson M, et al. Superior temporal gyrus, language function, and autism. Dev Neuropsychol. (2007) 31:217–38. doi: 10.1080/87565640701190841
61. Hotier S, Leroy F, Boisgontier J, Laidi C, Mangin JF, Delorme R, et al. Social cognition in autism is associated with the neurodevelopment of the posterior superior temporal sulcus. Acta Psychiat Scand. (2017) 136:517–25. doi: 10.1111/acps.12814
62. d'Albis MA, Guevara P, Guevara M, Laidi C, Boisgontier J, Sarrazin S, et al. Local structural connectivity is associated with social cognition in autism spectrum disorder. Brain. (2018) 141:3472–81. doi: 10.1093/brain/awy275
63. Zilbovicius M, Boddaert N, Belin P, Poline JB, Remy P, Mangin JF, et al. Temporal lobe dysfunction in childhood autism: a PET study. Am J Psychiatry. (2000) 157:1988–93. doi: 10.1176/appi.ajp.157.12.1988
64. Lepisto T, Silokallio S, Nieminen-von Wendt T, Alku P, Naatanen R, Kujala T. Auditory perception and attention as reflected by the brain event-related potentials in children with asperger syndrome. Clin Neurophysiol. (2006) 117:2161–71. doi: 10.1016/j.clinph.2006.06.709
65. Maister L, Plaisted-Grant KC. Time perception and its relationship to memory in autism spectrum conditions. Dev Sci. (2011) 14:1311–22. doi: 10.1111/j.1467-7687.2011.01077.x
66. Horton J, Millar A, Labelle A, Knott VJ. MMN responsivity to manipulations of frequency and duration deviants in chronic, clozapine-treated schizophrenia patients. Schizophr Res. (2011) 126:202–11. doi: 10.1016/j.schres.2010.11.028
67. Domjan N, Csifcsak G, Drotos G, Janka Z, Szendi I. Different patterns of auditory information processing deficits in chronic schizophrenia and bipolar disorder with psychotic features. Schizophr Res. (2012) 139:253–9. doi: 10.1016/j.schres.2012.06.002
68. Kargel C, Sartory G, Kariofillis D, Wiltfang J, Muller BW. Mismatch negativity latency and cognitive function in schizophrenia. PLoS ONE. (2014) 9:e84536. doi: 10.1371/journal.pone.0084536
69. Sugranyes G, Kyriakopoulos M, Corrigall R, Taylor E, Frangou S. Autism spectrum disorders and schizophrenia: meta-analysis of the neural correlates of social cognition. PLoS ONE. (2011) 6:e25322. doi: 10.1371/journal.pone.0025322
70. Shinozaki N, Yabe H, Sato Y, Hiruma T, Sutoh T, Nashida T, et al. The difference in mismatch negativity between the acute and post-acute phase of schizophrenia. Biol Psychol. (2002) 59:105–19. doi: 10.1016/S0301-0511(01)00129-6
71. Oades RD, Wild-Wall N, Juran SA, Sachsse J, Oknina LB, Ropcke B. Auditory change detection in schizophrenia: sources of activity, related neuropsychological function and symptoms in patients with a first episode in adolescence, and patients 14 years after an adolescent illness-onset. Bmc Psychiatry. (2006) 6:7. doi: 10.1186/1471-244X-6-7
72. Atkinson RJ, Michie PT, Schall U. Duration mismatch negativity and P3a in first-episode psychosis and individuals at ultra-high risk of psychosis. Biol Psychiatry. (2012) 71:98–104. doi: 10.1016/j.biopsych.2011.08.023
73. Foss-Feig JH, Velthorst E, Smith L, Reichenberg A, Addington J, Cadenhead KS, et al. (2019). Clinical profiles and conversion rates among young individuals with autism spectrum disorder who present to clinical high risk for psychosis services. J Am Acad Child Adolesc Psychiatry. 58:582–8. doi: 10.1016/j.jaac.2018.09.446
Keywords: autism spectrum disorder, attenuated psychosis syndrome, mismatch negativity, EEG, pediatric
Citation: Di Lorenzo G, Riccioni A, Ribolsi M, Siracusano M, Curatolo P and Mazzone L (2020) Auditory Mismatch Negativity in Youth Affected by Autism Spectrum Disorder With and Without Attenuated Psychosis Syndrome. Front. Psychiatry 11:555340. doi: 10.3389/fpsyt.2020.555340
Received: 24 April 2020; Accepted: 20 October 2020;
Published: 24 November 2020.
Edited by:
Frauke Schultze-Lutter, University Hospital Düsseldorf, GermanyReviewed by:
Marie Gomot, Université de Tours, FranceLeonhard Schilbach, Ludwig Maximilian University of Munich, Germany
Copyright © 2020 Di Lorenzo, Riccioni, Ribolsi, Siracusano, Curatolo and Mazzone. This is an open-access article distributed under the terms of the Creative Commons Attribution License (CC BY). The use, distribution or reproduction in other forums is permitted, provided the original author(s) and the copyright owner(s) are credited and that the original publication in this journal is cited, in accordance with accepted academic practice. No use, distribution or reproduction is permitted which does not comply with these terms.
*Correspondence: Giorgio Di Lorenzo, di.lorenzo@med.uniroma2.it; Luigi Mazzone, luigi.mazzone@uniroma2.it
†These authors have contributed equally to this work