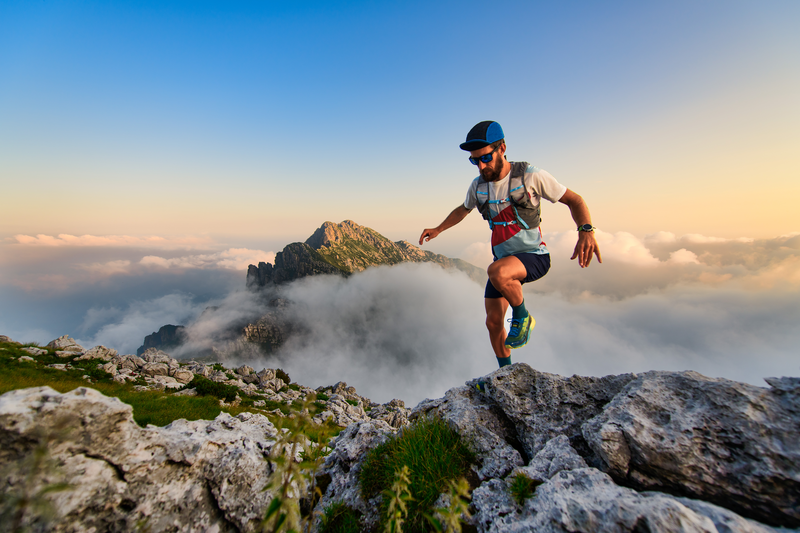
95% of researchers rate our articles as excellent or good
Learn more about the work of our research integrity team to safeguard the quality of each article we publish.
Find out more
ORIGINAL RESEARCH article
Front. Psychiatry , 23 October 2020
Sec. Molecular Psychiatry
Volume 11 - 2020 | https://doi.org/10.3389/fpsyt.2020.553269
This article is part of the Research Topic Linking Treatment Target Identification to Biological Mechanisms Underlying Mood Disorders View all 24 articles
The glutamatergic system has previously been shown to be involved in the pathophysiology of schizophrenia and the mechanisms of action of antipsychotic treatment. The present study aimed to investigate the relationship between the levels of glutamate (Glu) or Glu/total creatine (Glu/Cr+PCr) in the anterior cingulate cortex (ACC) and psychiatric symptoms as well as the response to antipsychotic treatment. We performed proton magnetic resonance spectroscopy (1H–MRS) to measure Glu and Glu/Cr+PCr in the ACC of 35 drug-naïve first-episode schizophrenia (FES) patients and 40 well-matched healthy controls (HCs). After scanning, we treated the patients with risperidone for eight weeks. Remission status was based on the Positive and Negative Syndrome Scale (PANSS) scores at week 8. At baseline, there were no significant differences in the levels of Glu or Glu/Cr+PCr in the ACC between drug-naïve FES patients and HCs. Lower baseline levels of Glu/Cr+PCr but not Glu in the ACC were associated with more severe negative symptoms of schizophrenia. Compared to the remission group (RM), the non-remission group (NRM) had lower baseline ACC Glu levels (P < 0.05). Our results suggest that ACC Glu levels may be related to the severity of symptoms in the early stages of schizophrenia and therefore may be a marker with which to evaluate the treatment effect of antipsychotics in schizophrenia patients.
Schizophrenia is a complex and severe mental disorder with a lifetime prevalence of 1% worldwide (1). Although it has been over 60 years since the first antipsychotic, chlorpromazine, was initially developed, a third of patients with schizophrenia have a suboptimal response to first-line antipsychotic treatment, most of which mainly target dopamine D2 receptors (2, 3). The dopaminergic hypothesis can partially explain the psychopathology of positive symptoms in schizophrenia. However, dopaminergic antipsychotics have only a limited effect on negative symptoms and cognitive deficit, which are the best predictive factors of persistent disability and a poor response to antipsychotics (4, 5).
Converging lines of evidence from pharmacological and neuropharmacological studies suggest that glutamatergic dysfunction also contributes to deficits in schizophrenia (6–8). Studies have shown that administration of phencyclidine (PCP), dizocilpine (MK801), and ketamine, antagonists of the N-methyl-d-aspartate glutamate receptor (NMDAR), to healthy volunteers or rodents can induce schizophrenia-like symptoms (9–11). In pharmacological animal models of schizophrenia, compared with other drugs, such as amphetamine, NMDAR antagonists can cause positive and negative symptoms that more closely mimic those of schizophrenia (12). Genetic animal models also provide a wealth of data showing that decreased NMDAR activity can lead to changes in the brain and behavior, similar to those observed in schizophrenia (13). First-episode schizophrenia studies have shown that approximately one-quarter of first-episode schizophrenia (FES) patients have persistent psychosis symptoms despite adequate initial treatment (14), and this finding, together with the glutamatergic hypothesis, may suggest that dopaminergic drugs are ineffective throughout illness in the subgroup of schizophrenia patients, who have an inadequate response to initial treatment with dopaminergic drugs.
Proton magnetic resonance spectroscopy (1H-MRS) is an efficient and non-invasive method for detecting the concentration of Glu in the human brain. Many studies have found differences in glutamate levels in target areas of the brain between schizophrenia patients and healthy controls (15–18), and glutamate levels have been associated with the severity of symptoms and cognitive function (19–21). These studies have aroused great interest in whether the levels of glutamate in the brain can predict the response to antipsychotics and the outcome of schizophrenia. The current hypothesis is that inadequate response to conventional dopaminergic antipsychotics is more likely to be associated with the glutamatergic system (22). In previous 1H-MRS studies, the results of measurements of glutamate levels in the brains of schizophrenia patients were mixed (23), and the findings of the relationship between glutamate levels and the severity of symptoms of schizophrenia were also inconsistent (24). The discrepancy of the above results may be due to the effect of age, stage of the disease, and treatment with antipsychotics (25, 26).
In this study, we implemented a longitudinal design to explore the relationship between glutamate levels and symptom severity as well as the response to antipsychotics. To reduce the effect of the confounding factors mentioned above, we selected drug naïve first-episode psychiatric patients as the experimental cohort. In previous studies, the ACC has been shown to play a critical role in cognitive impairment and psychotic symptoms in schizophrenia patients (27, 28). Therefore, we selected the ACC as the target region. At baseline, 1H-MRS scans were performed on patients and healthy controls who met the study criteria. We assessed the symptom severity of the patients using the PANSS scale at both baseline and week 8 after risperidone treatment. We chose risperidone as the administered antipsychotic because it is widely prescribed and very inexpensive in China. We expected to determine whether baseline levels of Glu or Glu/Cr+PCr in the ACC are associated with the severity of psychiatric symptoms and with the response to initial antipsychotic administration in drug naïve FES.
The demographic characteristics and metabolite levels are summarized in Table 1. Thirty-five FES patients met the inclusion criteria, were first diagnosed with schizophrenia, and had never been treated with antipsychotics. There was no significant difference in age, gender, education, or tobacco or alcohol use between drug-naïve FES patients and HCs. As shown in Table 1, no significant differences were observed in Glu and Glu/Cr+PCr levels in the ACC between HCs and FES patients at baseline. There were no significant differences in the Glu and Glu/Cr+PCr levels of the males and females between the patient group and the control group. Both p-values were higher than 0.05 (Supplementary Table 1).
The mean ± SD of the FWHM from the LC Model in the ACC was 0.069 ± 0.017 ppm for the FES patients and 0.065 ± 0.021 ppm for the HCs. The mean ± SD of the S/N in the ACC was 23.97 ± 2.70 for FES patients and 24.73 ± 2.91 for HCs. There were no significant differences in the FWHM and S/N in the target voxels between FES patients and HCs (FWHM, T73 = 0.849 p = 0.399; S/N, T73 = −1.156, p = 0.251). The CRLB% values of Glu, Cr, and PCr in the ACC for both the FES patients and HCs were <20%. Data on the quality of the 1H-MRS spectra are summarized in Table 1.
At baseline, lower levels of Glu and Glu/Cr+PCr in ACC were associated with more severe negative symptoms (Figure 1) (Glu and PANSS-n: r = 0.360, p = 0.034; Glu/Cr+PCr and PANSS-n: r = −0.432, p = 0.010). After controlling for age, the relationship between the Glu level in the ACC and negative symptoms was not significant (r = −0.323, p = 0.062), but lower Glu/Cr+PCr levels remained associated with a higher PANSS-n score (r = −0.446 p = 0.008).
Figure 1. Relationship between levels of glutamate (Glu and Glu/Cr+PCr) in anterior cingulate cortex (ACC) and PANSS negative symptom scores. (A) ACC-Glu and PANSS negative symptom scores at baseline (n = 35, r = −0.360, p = 0.034). (B) ACC-Glu/Cr+PCr and PANSS negative symptom scores at baseline (n = 35, r = −0.432, p = 0.010).
According to the standards proposed by the Remission in Schizophrenia Working Group (29), 25 patients (71%) met the remission criteria, and 10 patients (29%) were in non-remission status. Non-remission patients were younger, had more severe symptoms, and were treated with a higher dose of risperidone during the study (Table 2).
Compared with the remission group, non-remission patients had a significantly lower Glu level in the ACC (T33 = −2.634 P = 0.013). However, the difference in Glu/Cr+PCr levels in the ACC between the remission and non-remission groups was not significant (1.67 ± 0.26 vs. 1.53 ± 0.28, T33 = −1.612, p = 0.117) (Figure 2, Table 2).
Figure 2. Baseline Glu and Glu/Cr+PCr in ACC of remission and non-remission groups. (A) At baseline, the remission group had a significantly higher Glu level than the non-remission group (mean values, 9.22 ± 1.05 vs. 8.17 ± 1.08; T33 = −2.634, P = 0.013). (B) At baseline, the remission group had a higher Glu/Cr+PCr than the non-remission group, but there was no statistically significant difference between the two groups (mean values, 1.67 ± 0.26 vs. 1.53 ± 0.28; T33 = −1.612, p = 0.117). *p < 0.05.
To distinguish between remission and non-remission patients, we implemented a binary logistic regression model. Baseline Glu or Glu/Cr+PCr in the ACC was associated with the likelihood of remission in FES patients after treatment with risperidone. In the Glu model (Nagelkerke R2 = 0.256, B = −1.048, Wald = 4.945, p = 0.026, EXP[B] = 0.351), 77.1% of FES patients (96% remission patients and 30% non-remission patients) were correctly segregated. In the Glu/Cr+PCr model (Nagelkerke R2 = 0.110, B = −2.602, Wald = 2.367, p = 0.124, EXP[B] = 0.074), 74.3% of FES patients (96% remission patients and 20% non-remission patients) were correctly segregated. When age and PANSS-total score were included in the two models, the accuracy increased to 85.7% (92% remission patients and 70% non-remission patients, Nagelkerke R2 = 0.674) in the model with baseline Glu and 82.9% (88% remission patients and 70% non-remission patients, Nagelkerke R2 = 0.655) in the model with baseline Glu/Cr+PCr (Table 3).
Glutamate is the most important excitatory neurotransmitter in the nervous system (30), and NMDAR is widely distributed throughout the human brain. The glutamate measurement provided by 1H-MRS reflects the amount of glutamate in the target region in the brain and is also considered an indicator of motor cortical excitability (31, 32). Previous studies have observed that phencyclidine (PCP) and ketamine, which are antagonists of NMDAR, can transiently induce positive psychotic symptoms, such as delusions, hallucinations, agitation, and catatonic behavior, and negative psychotic symptoms including blunted affect, anhedonia, avolition, and inattention (6, 33). Evidence from MRS and microdialysis studies has shown that PCP or ketamine and other antagonists of NMDAR can increase glutamate release (34–37). To verify that glutamatergic system dysfunction is involved in the psychopathology of schizophrenia, a large number of studies on glutamate levels in the brain were conducted through MRS, and according to the ketamine or PCP model, glutamate concentrations in the brain should increase in first-episode schizophrenia patients. Supporting the ketamine model, recent studies based on samples of first-episode schizophrenia have observed glutamate concentrations in the frontal, prefrontal, and anterior cingulate cortexes (38), and the striatum (26, 39). Contrary to this hypothesis, some studies found reductions in glutamate concentrations in the ACC (16) and the medial frontal cortex of first-episode schizophrenia patients (40). In our study, there were no significant differences in glutamate levels in the ACC between drug-naïve first-episode schizophrenia patients and healthy controls. The inconsistency in these studies may suggest that the ketamine model does not accurately reflect the mechanisms underlying schizophrenia.
Accumulating evidence has shown that glutamatergic dysfunction may be involved in the pathogenesis of schizophrenia, and glutamate levels may be associated with the outcome of schizophrenia and the severity of psychiatric symptoms (41, 42). In our study, we found lower Glu/Cr+PCr was correlated with more severe negative symptoms as assessed by the PANSS at baseline. Some studies with samples of chronic schizophrenia patients report that the severity of negative symptoms was associated with lower levels of Glu levels in the brain (41, 43, 44). However, in contrast to our results, several studies found that more severe negative symptoms were associated with higher levels of Glu in first-episode psychosis (16, 45, 46). The inconsistencies were most likely due to the effects of age, antipsychotic administration, and disease stage on glutamate concentration. Natsubori et al. (47) found that the Glx level of chronic and medicated schizophrenia patients in the mPFC and ACC was lower than that in the healthy control group, patients at ultrahigh risk for psychosis, and patients with first-episode schizophrenia. One study found that Glu levels in the ACC were significantly lower than those at baseline in patients with first-episode schizophrenia after 4 weeks of amisulpride administration (46). Therefore, demographic characteristics of different samples can influence the Glu level, which affects the determination of the correlation between Glu levels and symptoms. In the present study, our subjects were younger than those in many studies and had never taken antipsychotic drugs. Recently, a study (48) with samples that had a similar mean age with ours (22.3 ± 4.4 years) found that the Glu level positively correlated with the cognitive function of the patients. According to our results, a lower level of Glu/Cr+PCr indicates more severe negative symptoms in patients with schizophrenia in the early stage before treatment. ACC glutamate levels were associated only with negative symptoms, suggesting that lower glutamate levels are linked to poor clinical outcomes.
Longitudinal studies on the relationship between antipsychotic response and Glu levels before treatment are rare. Recently, a multi-center 1H-MRS study found that first-episode schizophrenia patients with a worse response to amisulpride had higher ACC glutamate levels at baseline before treatment (46). The advantage of this study was that amisulpride has high selectivity to dopamine D2 and D3 receptors (49, 50); thus, in schizophrenia patients, Glu levels could be linked to the dopamine-blocking effect, rather than to the effect of blocking other receptors. However, this study did not require that the patients taking part in the study had never taken the antipsychotics, and they were also examined without a withdrawal period before the experiment. Additionally, a large proportion of the patients in this study were users of other substances. These factors make it impossible to eliminate the possible effect of pre-study drug therapy and substance use on the patients' Glu levels and the outcome of amisulpride administration (51). In our study, we selected individuals with schizophrenia who had never been treated by antipsychotics and excluded substance users. We found that patients with a worse response to risperidone had lower Glu levels than patients with a better response. Binary logistic regression models showed that the overall discrimination accuracy of remission by ACC Glu and Glu/Cr+PCr was 77.1 and 74.3%, respectively. When the age and PANSS-total scores were introduced into the regression models, the discrimination accuracy of remission reached 85.7 and 82.9%, respectively, which also showed clinical significance.
Ketamine has been shown to induce schizophrenia-like symptoms (6, 33, 52). The advantage of the ketamine model is that the relative proportions of positive and negative symptoms induced by the drug are more similar to schizophrenia than the respective proportions of symptoms induced by amphetamine or LSD (33, 53). According to the hypothesis of the ketamine model, schizophrenia patients should have higher levels of glutamate, which is associated with more severe psychiatric symptoms. However, this popular model is not without its limitations. First, some studies have found that ketamine also has a high affinity for D2 receptors (54, 55), while a recent study found that ketamine has no affinity for D2 receptors (56). Other studies have found that ketamine can change the level of glutamate in the brain of humans or animals (57), so the psychotomimetic effects of ketamine may be related to the dopaminergic system. Second, schizophrenia is considered a neurodevelopmental disorder in which the nervous system undergoes a long process to reach the disease state (58). Therefore, it is not reasonable to study the pathological process of schizophrenia using the more acute ketamine model. Third, many results based on MRS, including ours, are inconsistent with or even wholly contrary to the hypothesis of the ketamine model (16, 44, 48). Therefore, future studies on glutamate levels based on MRS should not only rely on the hypothesis of the ketamine model but should also be considered from multiple perspectives.
Our study also had some limitations. First, we did not perform 1H-MRS scans on patients at week 8, so we could not evaluate the changes in glutamate concentration caused by risperidone, which was not considered in our initial design. Second, we assessed only the psychiatric symptoms by the PANSS but did not evaluate the cognitive and social functions, so we could not fully judge the overall functional status and outcome of the drug-naïve FES patients. Third, because 1H-MRS scans require individuals to be relatively calm, we excluded patients with underlying impulsivity, agitation, and excitement symptoms, so our sample was not representative of all first-episode schizophrenia patients. Fourth, our sample size is small, and the lower limit of the age of the inclusion criteria was relaxed to 16 years old, so the influence of age on Glu concentration cannot be excluded entirely. In addition, non-remission patients were younger, had more severe symptoms, and were consequently treated with higher doses of risperidone. The difference in risperidone dosage will affect the final outcome of the patient. Fifth, we asked only the patient himself/herself and his/her guardians about the patient's use of tobacco, alcohol, and substances. We did not perform urine or blood tests on each subject to determine whether they used tobacco, alcohol, or other substances. Thus, we cannot guarantee the authenticity of the above reported data.
In conclusion, we observed that there were no significant differences in baseline Glu or Glu/Cr+PCr in the ACC between drug-naïve FES patients and HCs. However, we found that lower Glu/Cr+PCr levels were highly associated with serve negative symptoms and that the patients with worse outcomes had lower baseline Glu levels. Our results suggested that baseline glutamate levels in the ACC may be used as a marker to evaluate the treatment effect of antipsychotics in schizophrenia patients.
We recruited 39 drug-naïve FES subjects from outpatients in the Second Xiangya Hospital, Central South University, China, and 42 HC subjects through advertisements posted at the same time. All FES patients were first diagnosed with schizophrenia using the DSM-IV criteria with a Mini-International Neuropsychiatric Interview (59) by two experienced senior psychiatrists. The inclusion criteria of all subjects were as follows: (1) right-handed Han Chinese individuals aged 16–30 years; (2) education≧6 years; (3) no prior exposure to antipsychotics; (4) no history of substance use; and (5) no major medical or neurological illness. None of the HC subjects met the diagnostic criteria for any mental illness in the DSM-IV or had a history of mental illness or a family history of mental disorders. Data from four patients (two lost to follow-up and two with MR contraindications) and two healthy controls (one with MR contraindications and one with an anatomical abnormality on structural scan) were excluded. Data from 35 FES patients and 40 HCs were included in the final analyses. The study was approved by the Second Xiangya Hospital Ethics Committee (No. S008,2012). All participants were aware of the detailed procedures of our study and signed informed consent forms.
All subjects were scanned in a 3T scanner (Siemens, Verio, Germany) with a 16-channel head coil at the Magnetic Imaging Centre of Hunan Children's Hospital, China. Scans of patients were performed before treatment with antipsychotics, while healthy controls had no time limit on undergoing the scan. Patients with acute psychotic symptoms underwent an 1H-MRS scan within a few hours and took risperidone immediately after the scan was completed. Patients with predominantly negative symptoms had an 1H-MRS scan later in the day, taking risperidone in the evening or morning the next day. Participants wore specially made foam pads to reduce head motion and scanning noise. T1-weighted anatomical MRI images were acquired with 3-dimensional magnetization-prepared fast gradient echo sequences (TR/TE = 2530 ms/2.33 ms; FOV = 256 × 256 mm; slice thickness = 1.0 mm; gap = 0 mm; NEX = 1; and 192 sagittal slices). Target voxels were placed in the ACC (10 × 20 × 20 mm), and the 1H-MRS data were acquired using a point-resolved spectroscopy sequence (PRESS) (svs_se; TR = 3000 ms; TE = 30 ms; NEX 80) (Figure 3).
Figure 3. Spectroscopic voxel placement and proton magnetic resonance (1H-MRS) spectra. Voxel placement in the anterior cingulate cortex (ACC) and Sample 1H-MRS spectra acquired from ACC by LCModel.
1H-MRS spectra were analyzed with a linear combination model (LCModel version 6.3–1B) (Figure 3) at the Second Affiliated Hospital, Shantou, China. The cerebrospinal fluid in the target voxels was used as the internal reference to calculate the absolute concentration of Glu and Cr+PCr. The FWHM and SNR were checked to guarantee the quality of the MRS data, and only those spectra with an FWHM <0.1 ppm and an SNR> 10 were retained. Furthermore, data with a Cramer–Rao minimum variance ≥ 20% were discarded.
All patients were taking antipsychotics for the first time and were treated with risperidone monotherapy for eight weeks following the scanning. It is contraindicated for co-use with mood stabilizers and antidepressants. None of the patients dropped out of the study because of severe side effects.
We assessed the severity of illness at both baseline and week 8 using the 30-item Positive and Negative Syndrome Scale (PANSS) (60). The assessment was carried out by two psychiatrists (interrater reliability score > 0.8). Remission status was defined by the criteria proposed by the Remission in Schizophrenia Working Group (29), but it did not include the six-month observation period. This standard requires that the score of the following items of the PANSS be less than 3: PANSS-Positive (P1, P2, P3), PANSS-Negative (N1, N4), PANSS-General (G5, G9).
We performed all statistical analyses in SPSS (version 20 IBM Inc., New York, USA), with statistical significance indicated by two-tailed p-values < 0.05. Group differences in demographical characteristics, levels of Glu and Glu/Cr+PCr, and PANSS scores were assessed using independent-samples t-tests or chi-square tests. Relationships between metabolite levels and baseline symptom scores of the PANSS were described with Pearson's correlation analysis. The association between baseline metabolite levels of Glu and Glu/Cr+PCr and patient outcome (remission and non-remission) at week 8 was analyzed with binary logistic regression.
The raw data supporting the conclusions of this article will be made available by the authors, without undue reservation.
The studies involving human participants were reviewed and approved by The Second Xiangya Hospital Ethics Committee. Written informed consent to participate in this study was provided by the participants' legal guardian/next of kin.
JL, JT, HH, and XC designed the study. JL, ZL, HR, DW, XM, and LY collected the samples and clinical information. CL carried out the brain scanning. JL, HR, ZL, HH, JZ, and JT analyzed and discussed the experimental result. JL and HR wrote the first draft of the manuscript. All authors contributed to and have approved the final manuscript.
The authors declare that the research was conducted in the absence of any commercial or financial relationships that could be construed as a potential conflict of interest.
We would like to express our sincere thanks to all participants in this work. Our study was supported by the National Natural Science Foundation of China (No.81871057 to JT and No.81871056 to XC) and by the Fundamental Research Funds for the Central Universities of The Central South University (CX20190251).
The Supplementary Material for this article can be found online at: https://www.frontiersin.org/articles/10.3389/fpsyt.2020.553269/full#supplementary-material
1H–MRS, proton magnetic resonance spectroscopy; ACC, anterior cingulate cortex; Glu, glutamate; Cr, creatine; PCr, phosphocreatine; CRLBs, Cramér Rao lower Bounds; FWHM, full width at half maximum; SNR, signal-noise ratio; HC, healthy control; FES, first-episode schizophrenia; PANSS, Positive and Negative Syndrome Scale; NMDAR, N-methyl-D-aspartate receptor; RM, remission; NRM, non-remission.
1. McGrath J, Saha S, Chant D, Welham J. Schizophrenia: a concise overview of incidence, prevalence, and mortality. Epidemiol Rev. (2008) 30:67–76. doi: 10.1093/epirev/mxn001
2. Howes OD, Kambeitz J, Kim E, Stahl D, Slifstein M, Abi-Dargham A, et al. The nature of dopamine dysfunction in schizophrenia and what this means for treatment. Arch Gen Psychiatry. (2012) 69:776–86. doi: 10.1001/archgenpsychiatry.2012.169
3. Kapur S, Zipursky R, Jones C, Remington G, Houle S. Relationship between dopamine D(2) occupancy, clinical response, and side effects: a double-blind PET study of first-episode schizophrenia. Am J Psychiatry. (2000) 157:514–20. doi: 10.1176/appi.ajp.157.4.514
4. Lim J, Lee SA, Lam M, Rapisarda A, Kraus M, Keefe RS, et al. The relationship between negative symptom subdomains and cognition. Psychol Med. (2016) 46:2169–77. doi: 10.1017/S0033291716000726
5. Ventura J, Hellemann GS, Thames AD, Koellner V, Nuechterlein KH. Symptoms as mediators of the relationship between neurocognition and functional outcome in schizophrenia: a meta-analysis. Schizophr Res. (2009) 113:189–99. doi: 10.1016/j.schres.2009.03.035
6. Javitt DC. Glutamate and schizophrenia: phencyclidine, N-methyl-D-aspartate receptors, and dopamine-glutamate interactions. Int Rev Neurobiol. (2007) 78:69–108. doi: 10.1016/S0074-7742(06)78003-5
7. Stone JM, Morrison PD, Pilowsky LS. Glutamate and dopamine dysregulation in schizophrenia–a synthesis and selective review. J Psychopharmacol. (2007) 21:440–52. doi: 10.1177/0269881106073126
8. Thomas EHX, Bozaoglu K, Rossell SL, Gurvich C. The influence of the glutamatergic system on cognition in schizophrenia: a systematic review. Neurosci Biobehav Rev. (2017) 77:369–87. doi: 10.1016/j.neubiorev.2017.04.005
9. Newcomer JW, Farber NB, Jevtovic-Todorovic V, Selke G, Melson AK, Hershey T, et al. Ketamine-induced NMDA receptor hypofunction as a model of memory impairment and psychosis. Neuropsychopharmacology. (1999) 20:106–18. doi: 10.1016/S0893-133X(98)00067-0
10. Neill JC, Barnes S, Cook S, Grayson B, Idris NF, McLean SL, et al. Animal models of cognitive dysfunction and negative symptoms of schizophrenia: focus on NMDA receptor antagonism. Pharmacol Ther. (2010) 128:419–32. doi: 10.1016/j.pharmthera.2010.07.004
11. Adler CM, Goldberg TE, Malhotra AK, Pickar D, Breier A. Effects of ketamine on thought disorder, working memory, and semantic memory in healthy volunteers. Biol Psychiatry. (1998) 43:811–6. doi: 10.1016/S0006-3223(97)00556-8
12. Jones CA, Watson DJG, Fone KCF. Animal models of schizophrenia. Br J Pharmacol. (2011) 164:1162–94. doi: 10.1111/j.1476-5381.2011.01386.x
13. Balu DT. The NMDA receptor and schizophrenia: from pathophysiology to treatment. Adv Pharmacol. (2016) 76:351–82. doi: 10.1016/bs.apha.2016.01.006
14. Schennach R, Riedel M, Musil R, Moller HJ. Treatment response in first-episode schizophrenia. Clin Psychopharmacol Neurosci. (2012) 10:78–87. doi: 10.9758/cpn.2012.10.2.78
15. Aoyama N, Theberge J, Drost DJ, Manchanda R, Northcott S, Neufeld RW, et al. Grey matter and social functioning correlates of glutamatergic metabolite loss in schizophrenia. Br J Psychiatry. (2011) 198:448–56. doi: 10.1192/bjp.bp.110.079608
16. Reid MA, Salibi N, White DM, Gawne TJ, Denney TS, Lahti AC. 7T proton magnetic resonance spectroscopy of the anterior cingulate cortex in first-episode schizophrenia. Schizophr Bull. (2019) 45:180–9. doi: 10.1093/schbul/sbx190
17. Tayoshi S, Sumitani S, Taniguchi K, Shibuya-Tayoshi S, Numata S, Iga J, et al. Metabolite changes and gender differences in schizophrenia using 3-Tesla proton magnetic resonance spectroscopy (1H-MRS). Schizophr Res. (2009) 108:69–77. doi: 10.1016/j.schres.2008.11.014
18. Theberge J, Bartha R, Drost DJ, Menon RS, Malla A, Takhar J, et al. Glutamate and glutamine measured with 4.0 T proton MRS in never-treated patients with schizophrenia and healthy volunteers. Am J Psychiatry. (2002) 159:1944–6. doi: 10.1176/appi.ajp.159.11.1944
19. Olbrich HM, Valerius G, Rusch N, Buchert M, Thiel T, Hennig J, et al. Frontolimbic glutamate alterations in first episode schizophrenia: evidence from a magnetic resonance spectroscopy study. World J Biol Psychiatry. (2008) 9:59–63. doi: 10.1080/15622970701227811
20. Shirayama Y, Obata T, Matsuzawa D, Nonaka H, Kanazawa Y, Yoshitome E, et al. Specific metabolites in the medial prefrontal cortex are associated with the neurocognitive deficits in schizophrenia: a preliminary study. NeuroImage. (2010) 49:2783–90. doi: 10.1016/j.neuroimage.2009.10.031
21. Szulc A, Konarzewska B, Galinska-Skok B, Lazarczyk J, Waszkiewicz N, Tarasow E, et al. Proton magnetic resonance spectroscopy measures related to short-term symptomatic outcome in chronic schizophrenia. Neurosci Lett. (2013) 547:37–41. doi: 10.1016/j.neulet.2013.04.051
22. Mouchlianitis E, Bloomfield MA, Law V, Beck K, Selvaraj S, Rasquinha N, et al. Treatment-resistant schizophrenia patients show elevated anterior cingulate cortex glutamate compared to treatment-responsive. Schiz Bull. (2016) 42:744–52. doi: 10.1093/schbul/sbv151
23. Merritt K, Egerton A, Kempton MJ, Taylor MJ, McGuire PK. Nature of glutamate alterations in schizophrenia: a meta-analysis of proton magnetic resonance spectroscopy studies. JAMA Psychiatry. (2016) 73:665–74. doi: 10.1001/jamapsychiatry.2016.0442
24. Merritt K, McGuire P, Egerton A. Relationship between glutamate dysfunction and symptoms and cognitive function in psychosis. Front Psychiatry. (2013) 4:151. doi: 10.3389/fpsyt.2013.00151
25. Bustillo JR, Chen H, Jones T, Lemke N, Abbott C, Qualls C, et al. Increased glutamine in patients undergoing long-term treatment for schizophrenia: a proton magnetic resonance spectroscopy study at 3 T. JAMA Psychiatry. (2014) 71:265–72. doi: 10.1001/jamapsychiatry.2013.3939
26. de la Fuente-Sandoval C, Leon-Ortiz P, Azcarraga M, Stephano S, Favila R, Diaz-Galvis L, et al. Glutamate levels in the associative striatum before and after 4 weeks of antipsychotic treatment in first-episode psychosis: a longitudinal proton magnetic resonance spectroscopy study. JAMA Psychiatry. (2013) 70:1057–66. doi: 10.1001/jamapsychiatry.2013.289
27. Bersani FS, Minichino A, Fojanesi M, Gallo M, Maglio G, Valeriani G, et al. Cingulate Cortex in Schizophrenia: its relation with negative symptoms and psychotic onset. A review study. Eur Rev Med Pharmacol Sci. (2014) 18:3354–67. Available online at: https://www.europeanreview.org/article/8074
28. Goghari VM, Sponheim SR, MacDonald AW, 3rd. The functional neuroanatomy of symptom dimensions in schizophrenia: a qualitative and quantitative review of a persistent question. Neurosci Biobehav Rev. (2010) 34:468–86. doi: 10.1016/j.neubiorev.2009.09.004
29. Andreasen NC, Carpenter WT, Jr., Kane JM, Lasser RA, Marder SR, et al. Remission in schizophrenia: proposed criteria and rationale for consensus. Am J Psychiatry. (2005) 162:441–9. doi: 10.1176/appi.ajp.162.3.441
30. Farber NB, Newcomer JW, Olney JW. The glutamate synapse in neuropsychiatric disorders. Focus on schizophrenia and Alzheimer's disease. Prog Brain Res. (1998) 116:421–37. doi: 10.1016/S0079-6123(08)60453-7
31. Stagg CJ, Bestmann S, Constantinescu AO, Moreno LM, Allman C, Mekle R, et al. Relationship between physiological measures of excitability and levels of glutamate and GABA in the human motor cortex. J Physiol. (2011) 589(Pt 23):5845–55. doi: 10.1113/jphysiol.2011.216978
32. Stagg CJ, Wylezinska M, Matthews PM, Johansen-Berg H, Jezzard P, Rothwell JC, et al. Neurochemical effects of theta burst stimulation as assessed by magnetic resonance spectroscopy. J Neurophysiol. (2009) 101:2872–7. doi: 10.1152/jn.91060.2008
33. Krystal JH, Bennett A, Abi-Saab D, Belger A, Karper LP, D'Souza DC, et al. Dissociation of ketamine effects on rule acquisition and rule implementation: possible relevance to NMDA receptor contributions to executive cognitive functions. Biol Psychiatry. (2000) 47:137–43. doi: 10.1016/S0006-3223(99)00097-9
34. Moghaddam B, Adams B, Verma A, Daly D. Activation of glutamatergic neurotransmission by ketamine: a novel step in the pathway from NMDA receptor blockade to dopaminergic and cognitive disruptions associated with the prefrontal cortex. J Neurosci. (1997) 17:2921–7. doi: 10.1523/JNEUROSCI.17-08-02921.1997
35. Kraguljac NV, Frolich MA, Tran S, White DM, Nichols N, Barton-McArdle A, et al. Ketamine modulates hippocampal neurochemistry and functional connectivity: a combined magnetic resonance spectroscopy and resting-state fMRI study in healthy volunteers. Mol Psychiatry. (2017) 22:562–9. doi: 10.1038/mp.2016.122
36. Rowland LM, Bustillo JR, Mullins PG, Jung RE, Lenroot R, Landgraf E, et al. Effects of ketamine on anterior cingulate glutamate metabolism in healthy humans: a 4-T proton MRS study. Am J Psychiatry. (2005) 162:394–6. doi: 10.1176/appi.ajp.162.2.394
37. Kim SY, Lee H, Kim HJ, Bang E, Lee SH, Lee DW, et al. In vivo and ex vivo evidence for ketamine-induced hyperglutamatergic activity in the cerebral cortex of the rat: potential relevance to schizophrenia. NMR Biomed. (2011) 24:1235–42. doi: 10.1002/nbm.1681
38. Smesny S, Gussew A, Biesel NJ, Schack S, Walther M, Rzanny R, et al. Glutamatergic dysfunction linked to energy and membrane lipid metabolism in frontal and anterior cingulate cortices of never treated first-episode schizophrenia patients. Schizophr Res. (2015) 168:322–9. doi: 10.1016/j.schres.2015.07.013
39. de la Fuente-Sandoval C, Leon-Ortiz P, Favila R, Stephano S, Mamo D, Ramirez-Bermudez J, et al. Higher levels of glutamate in the associative-striatum of subjects with prodromal symptoms of schizophrenia and patients with first-episode psychosis. Neuropsychopharmacology. (2011) 36:1781–91. doi: 10.1038/npp.2011.65
40. Wang J, Tang Y, Zhang T, Cui H, Xu L, Zeng B, et al. Reduced gamma-aminobutyric acid and glutamate+glutamine levels in drug-naive patients with first-episode schizophrenia but not in those at ultrahigh risk. Neural Plasti. (2016) 2016:3915703. doi: 10.1155/2016/3915703
41. Ohrmann P, Kugel H, Bauer J, Siegmund A, Kolkebeck K, Suslow T, et al. Learning potential on the WCST in schizophrenia is related to the neuronal integrity of the anterior cingulate cortex as measured by proton magnetic resonance spectroscopy. Schizophr Res. (2008) 106:156–63. doi: 10.1016/j.schres.2008.08.005
42. Rusch N, Tebartz van Elst L, Valerius G, Buchert M, Thiel T, Ebert D, et al. Neurochemical and structural correlates of executive dysfunction in schizophrenia. Schizophr Res. (2008) 99:155–63. doi: 10.1016/j.schres.2007.05.024
43. Reid MA, Stoeckel LE, White DM, Avsar KB, Bolding MS, Akella NS, et al. Assessments of function and biochemistry of the anterior cingulate cortex in schizophrenia. Biol Psychiatry. (2010) 68:625–33. doi: 10.1016/j.biopsych.2010.04.013
44. Bustillo JR, Chen H, Gasparovic C, Mullins P, Caprihan A, Qualls C, et al. Glutamate as a marker of cognitive function in schizophrenia: a proton spectroscopic imaging study at 4 Tesla. Biol Psychiatry. (2011) 69:19–27. doi: 10.1016/j.biopsych.2010.08.024
45. Egerton A, Brugger S, Raffin M, Barker GJ, Lythgoe DJ, McGuire PK, et al. Anterior cingulate glutamate levels related to clinical status following treatment in first-episode schizophrenia. Neuropsychopharmacology. (2012) 37:2515–21. doi: 10.1038/npp.2012.113
46. Egerton A, Broberg BV, Van Haren N, Merritt K, Barker GJ, Lythgoe DJ, et al. Response to initial antipsychotic treatment in first episode psychosis is related to anterior cingulate glutamate levels: a multicentre (1)H-MRS study (OPTiMiSE). Mol Psychiatry. (2018) 23:2145–55. doi: 10.1038/s41380-018-0082-9
47. Natsubori T, Inoue H, Abe O, Takano Y, Iwashiro N, Aoki Y, et al. Reduced frontal glutamate + glutamine and N-acetylaspartate levels in patients with chronic schizophrenia but not in those at clinical high risk for psychosis or with first-episode schizophrenia. Schizophr Bull. (2014) 40:1128–39. doi: 10.1093/schbul/sbt124
48. Wang AM, Pradhan S, Coughlin JM, Trivedi A, DuBois SL, Crawford JL, et al. Assessing brain metabolism with 7-t proton magnetic resonance spectroscopy in patients with first-episode psychosis. JAMA Psychiatry. (2019) 76:314–23. doi: 10.1001/jamapsychiatry.2018.3637
49. Schoemaker H, Claustre Y, Fage D, Rouquier L, Chergui K, Curet O, et al. Neurochemical characteristics of amisulpride, an atypical dopamine D2/D3 receptor antagonist with both presynaptic and limbic selectivity. J Pharmacol Exp Ther. (1997) 280:83–97.
50. Chivers JK, Gommeren W, Leysen JE, Jenner P, Marsden CD. Comparison of the in-vitro receptor selectivity of substituted benzamide drugs for brain neurotransmitter receptors. J Pharm Pharm. (1988) 40:415–21. doi: 10.1111/j.2042-7158.1988.tb06306.x
51. Hellem T, Shi X, Latendresse G, Renshaw PF. The utility of magnetic resonance spectroscopy for understanding substance use disorders: a systematic review of the literature. J Am Psychiatr Nurs Assoc. (2015) 21:244–75. doi: 10.1177/1078390315598606
52. Lahti AC, Koffel B, LaPorte D, Tamminga CA. Subanesthetic doses of ketamine stimulate psychosis in schizophrenia. Neuropsychopharmacology. (1995) 13:9–19. doi: 10.1016/0893-133X(94)00131-I
53. Gouzoulis-Mayfrank E, Heekeren K, Neukirch A, Stoll M, Stock C, Obradovic M, et al. Psychological effects of (S)-ketamine and N,N-dimethyltryptamine (DMT): a double-blind, cross-over study in healthy volunteers. Pharmacopsychiatry. (2005) 38:301–11. doi: 10.1055/s-2005-916185
54. Seeman P, Ko F, Tallerico T. Dopamine receptor contribution to the action of PCP, LSD and ketamine psychotomimetics. Mol Psychiatry. (2005) 10:877–83. doi: 10.1038/sj.mp.4001682
55. Kapur S, Seeman P. NMDA receptor antagonists ketamine and PCP have direct effects on the dopamine D(2) and serotonin 5-HT(2)receptors-implications for models of schizophrenia. Molecular Psychiatry. (2002) 7:837–44. doi: 10.1038/sj.mp.4001093
56. Can A, Zanos P, Moaddel R, Kang HJ, Dossou KSS, Wainer IW, et al. Effects of ketamine and ketamine metabolites on evoked striatal dopamine release, dopamine receptors, and monoamine transporters. J Pharmacol Exp Ther. (2016) 359:159–70. doi: 10.1124/jpet.116.235838
57. Kokkinou M, Ashok AH, Howes OD. The effects of ketamine on dopaminergic function: meta-analysis and review of the implications for neuropsychiatric disorders. Mol Psychiatry. (2018) 23:59–69. doi: 10.1038/mp.2017.190
58. Murray RM, Lewis SW. Is schizophrenia a neurodevelopmental disorder? Br Med J. (1988) 296:63. doi: 10.1136/bmj.296.6614.63
59. Sheehan DV, Lecrubier Y, Sheehan KH, Amorim P, Janavs J, Weiller E, et al. The Mini-International Neuropsychiatric Interview (M.I.N.I.): the development and validation of a structured diagnostic psychiatric interview for DSM-IV and ICD-10. J Clin Psychiatry. (1998) 59 Suppl 20:22–33;quiz 4–57.
Keywords: glutamate, magnetic resonance spectroscopy, ACC, anterior cingulate cortex, Cr, creatine, FES, first-episode schizophrenia
Citation: Li J, Ren H, He Y, Li Z, Ma X, Yuan L, Ouyang L, Zhou J, Wang D, Li C, Chen X, Han H and Tang J (2020) Anterior Cingulate Cortex Glutamate Levels Are Related to Response to Initial Antipsychotic Treatment in Drug-Naive First-Episode Schizophrenia Patients. Front. Psychiatry 11:553269. doi: 10.3389/fpsyt.2020.553269
Received: 18 April 2020; Accepted: 22 September 2020;
Published: 23 October 2020.
Edited by:
J. John Mann, Columbia University, United StatesReviewed by:
Marisa Moller, North-West University, South AfricaCopyright © 2020 Li, Ren, He, Li, Ma, Yuan, Ouyang, Zhou, Wang, Li, Chen, Han and Tang. This is an open-access article distributed under the terms of the Creative Commons Attribution License (CC BY). The use, distribution or reproduction in other forums is permitted, provided the original author(s) and the copyright owner(s) are credited and that the original publication in this journal is cited, in accordance with accepted academic practice. No use, distribution or reproduction is permitted which does not comply with these terms.
*Correspondence: Jinsong Tang, dGFuZ2ppbnNvbmdAemp1LmVkdS5jbg==; Xiaogang Chen, Y2hlbnhpYW9nYW5nQGNzdS5lZHUuY24=; Hongying Han, aGFuaHkzQG1haWwuc3lzdS5lZHUuY24=
†These authors have contributed equally to this work
Disclaimer: All claims expressed in this article are solely those of the authors and do not necessarily represent those of their affiliated organizations, or those of the publisher, the editors and the reviewers. Any product that may be evaluated in this article or claim that may be made by its manufacturer is not guaranteed or endorsed by the publisher.
Research integrity at Frontiers
Learn more about the work of our research integrity team to safeguard the quality of each article we publish.