- 1Department of Child and Adolescent Psychiatry, King's College London (KCL), Institute of Psychiatry, Psychology and Neuroscience (IoPPN), London, United Kingdom
- 2Department of Child and Adolescent Psychiatry and Psychology, Hospital Clínic de Barcelona, Institute of Neuroscience, Barcelona, Spain
- 3Child and Adolescent Psychiatry and Psychology, August Pi i Sunyer Biomedical Research Institute (IDIBAPS), Barcelona, Spain
- 4Department of Medicine, Universitat de Barcelona, Barcelona, Spain
- 5Department of Psychiatry and Psychotherapy, Medical Faculty Mannheim, Central Institute of Mental Health, Heidelberg University, Mannheim, Germany
- 6Centre for Neuroimaging Sciences, King's College London, London, United Kingdom
- 7Behavioural and Developmental Psychiatry Clinical Academic Group, Behavioural Genetics Clinic, Adult Autism and Attention Deficit Hyperactivity Disorder Service, South London and Maudsley NHS Foundation Trust, London, United Kingdom
- 8Department of Forensic and Neurodevelopmental Sciences, Institute of Psychiatry, Psychology and Neuroscience, King's College London, London, United Kingdom
Theory of mind (ToM) or mentalizing difficulties is reported in attention-deficit/hyperactivity disorder (ADHD) and autism spectrum disorder (ASD), but the mechanism underpinning these apparently shared deficits is relatively unknown. Eighty-three young adult males, 19 with ASD alone, 21 with ADHD alone, 18 with dual diagnosis of ASD and ADHD, and 25 typically developing (TD) controls completed the functional magnetic resonance imaging version of the Frith-Happé animated-triangle ToM task. We compared neural function during ToM with two non-ToM conditions, random and goal directed motions, using whole-brain and region-of-interest analysis of brain activation and functional connectivity analyses. The groups showed comparable ToM task performance. All three clinical groups lacked local connectivity increase shown by TD controls during ToM in the right temporoparietal cortex, a key mentalizing region, with a differentially increased activation pattern in both ASD and comorbid groups relative to ADHD. Both ASD groups also showed reduced connectivity between right inferior lateral prefrontal and posterior cingulate cortices that could reflect an atypical information transmission to the mentalizing network. In contrast, with mentalizing both ADHD groups showed decreasing connectivity between the medial prefrontal and left temporoparietal cortices when compared to TD controls. Therefore, despite the complex pattern of atypical brain function underpinning ToM across the three disorders, some neurofunctional abnormalities during ToM are associated with ASD and appeared differentiable from those associated with ADHD, with the comorbid group displaying combined abnormalities found in each condition.
Introduction
Autism spectrum disorder (ASD) is characterized by social communication difficulties and stereotypical/repetitive behaviors, while attention-deficit/hyperactivity disorder (ADHD) is characterized by age-inappropriate symptoms of inattention, hyperactivity, and impulsivity (1). Despite the distinct presentations, up to 55% of children and adolescents with the disorders meet both diagnostic criteria (2, 3). Furthermore, reports of a co-occurrence of both disorders in adulthood may indicate its persistence (4–6). Reviews and meta-analyses suggest overlapping cognitive impairments in both disorders including the difficulties in theory of mind (ToM), i.e., the inference of others' mental states or “mentalizing” (7–9), even though ToM deficits have been thought to be ASD-specific [e.g., (10–13)].
In ASD, mentalizing problems are conceptualized as a core symptom (11) or a consequence of diminished social motivation (14). The deficits in ASD are commonly associated with underactivation of temporo- and frontolimbic social brain networks, in medial and ventrolateral prefrontal, temporo-parietal cortices, and amygdala in children [e.g., (15, 16)] and adults [e.g., (17–19)], leading to the “hypo-intentionality” hypothesis of autism (20). However, overactivation of these regions has also been reported in children and adults with ASD (21–24). Reduced activation in the right temporoparietal junction (TPJ) and medial prefrontal cortex (mPFC), particularly, has been shown to correlate with increased autism severity scores (15, 17, 25).
During mentalizing, children and adults with ASD also showed reduced functional connectivity (FC) (17, 20, 21, 26, 27) in the social brain network that is typically increased in TD controls (17, 28, 29). Such FC reduction in ASD has been reported between frontal, especially mPFC, and temporo-parietal regions, particularly on the right hemisphere (20, 26, 30), ventral premotor and sensorimotor areas (25), and among widespread seed regions in the network (21, 27). These findings, together with observation of reduced FC across other cognitive domains (31–33), have led to the theory of domain-general frontal-posterior underconnectivity in ASD (34).
In ADHD, social cognition, and mentalizing deficits are increasingly reported, mostly in children (35–39) but also in adults (40–43), albeit not as consistently as in ASD (8). For the adult age group particularly, recent studies have reported intact mentalizing in ADHD, who are instead impaired in empathy and in their ability for generating solutions for social problems (44, 45). In line with these findings, a review has suggested that social cognition deficits in ADHD are less severe and more heterogeneous than in ASD (8).
In the context of neuroimaging of ADHD, altered orbitofrontal and lateral fronto-striatal activation, which is typicaly linked to executive function deficits (46–48), has been cited as possible mediator for the social cognition deficits in ADHD (49, 50), although, to our knowledge, no fMRI studies have explored neural correlates of ToM in ADHD. Importantly, it is unclear if social cognition and mentalizing deficits are intrinsic to ADHD (41) or reflect co-occuring ASD traits in the population (40).
Comparative fMRI studies involving people with ADHD alone, ASD alone, and/or combined ASD+ADHD could tease apart disorder-differentiating impairments in social brain regions. To date, these studies have been conducted exclusively in children and adolescents with the disorders (51–55). One functional magnetic resonance imaging (fMRI) study has found ASD-specific inferior frontal gyrus underactivation relative to TD and ADHD during emotion identification (51). Furthermore, using resting-state fMRI, ASD-differentiating intrinsic FC impairments were observed in regions typically involved in mentalizing, with increased local (i.e., “degree centrality”) temporo-limbic FC in the ASD and ASD+ADHD groups relative to TD and ADHD, and shared precuneus overconnectivity across all clinical groups (55). Electroencephalography (EEG) biomarkers for face and eye-gaze processings such as N170 have also been found to be ASD-differentiating relative to ADHD (52–54). These findings suggest that the neural abnormalities underlying social cognition, based on a number of neural and electrophysiological correlates, might be specific to ASD and not ADHD. However, the hypothesis has not been tested during mentalizing specifically.
Therefore, we directly compare hemodynamic response and FC in brain regions during ToM relative to non-ToM conditions across individuals with ADHD, ASD, ASD+ADHD, and typical development. To address the lack of investigation of brain function correlates of comorbidity in the adult population, this study focused on young adults using the Frith-Happé animated-triangle fMRI task that is frequently used for investigating ToM in ASD (21, 24, 27), which has also been shown to detect ToM deficits in ADHD, albeit in children (35). We aimed to investigate if ToM performance deficits are similar or different and are associated with similar or different neural correlates across the three clinical groups. Based on previous findings of neuroimaging (51), FC (55), and EEG (52–54), we hypothesized that abnormalities in the social brain network, e.g., under-activation in right TPJ and mPFC, and reduced fronto-posterior FC during ToM (21, 27, 34) would distinguish the ASD groups, with and without ADHD, from the group with ADHD alone, who would show no neurofunctional impairments.
Materials and Methods
Participants
A total of 107 young adult males aged 20–27 years with ASD, ADHD, ASD+ADHD, and TD and full-scale intelligent quotient (FSIQ) ≥70, estimated using the Wechsler Abbreviated Scale of Intelligence (56), took part in the study. Only males were included to increase group homogeneity for these predominantly male-prevalent disorders (57, 58). Handedness was assessed using the Edinburgh Handedness Inventory (59) and did not differ between groups. Excluded were participants with epilepsy, personality disorder, current substance abuse/dependence, lifetime history of bipolar disorder, schizophrenia or head injury, or contraindication for fMRI. The participants completed a larger study involving several fMRI tasks and a neurocognitive task battery (60). After excluding 24 subjects (four with incomplete fMRI data, seven with missing performance data due to technical or compliance issues [these participants answered ≤3 out of 12 questions], 12 with excessive movement (>3 mm) and one with an incidental MRI finding), 83 subjects remained (19 ASD; 21 ADHD; 18 ASD+ADHD; 25 TD). The groups differed in FSIQ, but not age (See Table 1 for descriptive statistics).
The clinical groups were recruited through specialist adult ASD and ADHD clinics, support organizations, social media, and from a well-characterized population-based cohort of young adults with ASD, the Special Needs and Autism Project (SNAP), who were followed up since 10–12 years and are now young adults (61). Psychostimulant medications, which were withdrawn 48 h before testing, and selective serotonin reuptake inhibitors (SSRIs) prescription, were not exclusion criteria for the clinical groups. Participants with non-stimulant ADHD medications were excluded due to their relatively longer withdrawal periods.
The ASD group consisted of 14 participants with clinical diagnoses (six autism, eight Asperger's syndrome) and five participants with research diagnoses from SNAP [two autism, two atypical autism, and one pervasive developmental disorder (PDD) unspecified], based on the International Classification of Diseases criteria (ICD-10) (62). All but one diagnoses were accompanied by gold-standard research instruments, the Autism Diagnostic Observation Schedule (ADOS) (63) or parent interviews on the Autism Diagnostic Interview-Revised (ADI-R) (64). Where available, current ADOS scores were collected from the examiners. One participant received childhood ASD diagnosis from a specialist neurodevelopmental clinic, supported by an assessment on the Diagnostic Interview for Social and Communication Disorders (DISCO) (65), but had no current scores. None were prescribed medication.
In the ASD+ADHD group, 14 subjects had clinical diagnoses (five autism, six Asperger's syndrome, three atypical autism) and four had research diagnoses of ASD from SNAP (two atypical autism, two PDD unspecified) based on the ICD-10. All but one ASD diagnoses were accompanied by the ADOS or the ADI-R (ADOS score on Table 1). One participant's childhood ASD diagnosis from a specialist neurodevelopmental clinic was supported by the DISCO (no current scores). Furthermore, thirteen participants met the criteria for combined and five for inattentive DSM-5 ADHD subtype. Nine had current clinical DSM-5 ADHD diagnoses supported by current assessments on the Diagnostic Interview for Adult ADHD (DIVA 2.0) (66) or the Conners' Adult ADHD Diagnostic Interview for DSM-IV (CAADID) (67). Nine other participants had a significant history of ADHD symptoms assessed through SNAP and met the current ADHD DSM-5 criteria on the Young Adult Psychiatric Assessment (68). Two were prescribed psychostimulants alone [methylphenidate (MPH), dexamphetamine], one SSRIs alone (escitalopram) and one both (MPH, sertraline).
All participants in the ADHD group met the DSM-5 diagnostic criteria: 12 with combined, eight inattentive, and one hyperactive subtype, diagnosed by consultant psychiatrists in specialist adult ADHD clinics. Eighteen diagnoses were supported by the DIVA 2.0 and three by the CAADID. Four were prescribed psychostimulants alone (MPH and lisdexamphetamine), one with SSRIs alone (sertraline) and one with both (MPH and sertraline).
The TD participants were from local communities, were non-medicated, and scored below clinical cut-off on the Conners' Adult ADHD Rating Scale (CAARS) (69) and the Social Responsiveness Scale-2 (SRS-2) (70) for ADHD and ASD traits, respectively. This study was in accordance of the Declaration of Helsinki and had ethical approval from a local National Health Service Research Ethics Committee (NHS REC 13/LO/0373). Each participant gave written informed consent and was given £50 and travel reimbursement.
Clinical Measures
The CAARS ADHD index and the hyperactivity/inattention domain of the Strengths and Difficulties Questionnaires (SDQ) for adults (http://www.sdqinfo.com) indexed ADHD traits, while total algorithm score of SRS-2 indexed ASD traits. The participants completed self-report measures, corroborated by informant (e.g., parents/partner/siblings) for those in the clinical groups.
The Frith-Happé Animated Triangles Task
The block-design fMRI version of the Frith-Happé task was selected as it evokes large effect size of brain activation, which is associated with increased statistical power. The task was also selected based on its frequent use in ToM investigation in ASD children and adult populations (21, 24, 27). A recent study furthermore reported ToM deficits in ADHD children using the same task (35). The task consists of twelve 26–48 s cartoons involving two triangles whose movements express: (1) ToM, e.g., persuading (length = 39.0±2.2s), (2) Goal-directed (GD) interaction, e.g., following (length = 39.5 ± 9.5 s), and (3) Random (RD) purposeless motions, e.g., floating (length = 39.8 ± 1.5 s) (71). Each condition is depicted by four different clips, shown in the same pseudo-randomized block order across participants. Each block consists of 1-s fixation cross (jittered between 0.3–1.9 s), one clip, a 3-s fixation and a visual multi-choice question prompting the participants to identify the movement type shown with a maximum duration of 5 s. The chosen answer is highlighted for 1 s (Supplementary Figure 1) before the start of the next block. Response accuracy and response time (RT) were collected during the task.
Outside the fMRI scanning, a subset of the clips (four ToM and four GD) was shown to the participants, followed by the question “What do you think is happening during the clip?” Neutral prompts (e.g. “uh-hum”) were given by the examiner when the answer was not forthcoming. Responses were transcribed and rated by the first author DI, blinded to the participants' diagnoses, yielding scores for intention attributions (0–5), their appropriateness (0–3), the primary measures; and response length (i.e., number of clauses) and number of prompts, our secondary measure of ToM ability (72). Forty-eight randomly selected transcriptions (12 per group) were independently rated by the second author SL, achieving good interrater agreement for ratings of intention attribution (weighted κ =.71; weighted agreement = 92.8%), appropriateness (weighted κ =.69; weighted agreement = 88.6%), response length [intra-class correlation coefficient (ICC) = 0.97], and number of prompts (ICC = 0.99). Behavioral data were analyzed in STATA 14.0 (73) using (Group × Condition) repeated measures ANOVA, with post-hoc analyses corrected using the Tukey–Kramer method, accounting for unequal sample sizes across groups.
Neuroimaging Data Acquisition
Data were acquired using a General Electric (GE) MR750 3T scanner (General Electric, Boston, MA, United States) at the Center for Neuroimaging Sciences, King's College London. The scanner's body coil was used for RF transmission, while an eight-channel head coil was used for signal reception. An echo planar image (EPI) gradient-echo pulse sequence (TR/TE = 2,000/30 ms, flip angle = 80°, FOV = 19.2 × 19.2 cm, 64 × 64 matrix, in-plane resolution = 3 mm, slice thickness/gap = 3/0.3 mm) was used to acquire 40 slices of T2*-weighted MR images angled at 20° up from inter-commissural plane, prescribed consecutively top-to-bottom, covering the entire brain. The 10-min, 14-s task produced 307 volumes in time series. A whole-brain high resolution structural T1-weighted scan (Sagittal ADNI-GO/2 ACC MPRAGE), co-registered with individual activation maps during pre-processing, was acquired in the inter-commissural plane with TE = 3.016 s, TR = 7.312 s, 196 slices, FOV = 27 cm × 27 cm, 256 × 256 matrix, and slice thickness of 1.2 mm.
Neuroimaging Analyses
fMRI data were corrected for slice timing, realigned, co-registered to the individual T1-weighted scan, segmented, normalized to the Montreal Neurological Institute EPI template, and smoothed using an 8-mm Gaussian kernel. Statistical analyses were completed in two steps on the Statistical Parametric Mapping (SPM8). At the subject-level analyses, BOLD response was predicted using a vector of onsets and durations convolved with the canonical hemodynamic response function (HRF). Six nuisance motion regressors [x-, y-, z-translations, rotations, and additional regressors for each motion spike (>1 mm)] controlled for volume-to-volume head motion and abrupt movements. A high-pass filter was applied at the cut-off of 128 s and a first-order autoregressive model corrected for time series correlation. Investigations were carried out in the orthogonal contrast ToM > RD, typical of past studies (21, 27, 74–76), and separately in ToM > GD, a higher-level contrast investigated in a recent study (72).
Both contrasts were entered to second-level analyses. Within-group activations were analyzed with a cluster extent threshold of p <.05, family-wise error corrected (FWEcor), and a cluster-forming voxel threshold of p < 0.001 (Figure 2). Between-group activation was modeled with group as predictor, covarying for total frame-wise head displacement to control for residual motion variation. The group differences were analyzed, first, using an exploratory whole-brain analysis (voxel threshold p < 0.05, FWEcor) and, second, using a hypothesis-driven region-of-interest (ROI) analyses, by means of small-volume correction, using 10-mm radius spherical ROIs in the bilateral inferior frontal gyrus (IFG), bilateral medial prefrontal cortex (mPFC), bilateral posterior cingulate cortex (PCC), bilateral angular gyrus (ANG), and bilateral temporo-parietal junction/superior-temporal sulcus (TPJ/STS). The ROIs were centered on independently derived coordinates from a whole-brain activation map of ToM > RD [http://neurovault.org/images/3180 (77)], following Kana et al. (27). Mean beta weights of BOLD data were extracted for post-hoc pairwise group comparisons, applying Tukey–Kramer correction, and for correlational analyses with primary trait and task performance measures, applying false-discovery rate [FDR] correction. Logarithmic transformations were applied to normalize skewed data distribution as appropriate.
To compare FC between task conditions, we investigated the synchronization of BOLD activation time series across regions to facilitate comparison with previous studies [e.g., (21, 26, 27, 78)]. Activation time-series were first extracted from the spherical ROIs for everyone. The activation time series in ipsilateral TPJ and STS were combined by averaging due to regional overlap, yielding eight seed regions for the subsequent FC analyses. To control for artifacts, six orthogonal head motion parameters, head movement spikes, white matter, and cerebrospinal fluid signal plus their derivatives were regressed out. Artifact-corrected time-series were segmented pertaining to each video clip and those representing the same movement condition were concatenated into a condition-specific time series (27). These time series were correlated pairwise between ROIs, yielding altogether 28 unique correlation coefficients per movement condition (i.e., ToM, GD, and RD), which were then Fisher's z-transformed using an inverse hyperbolic tangent function to produce a FC strength index. They were then analyzed using multilevel mixed-effects linear regression models with group, condition, and group × condition as fixed effects and individual factor as random effects. Model fit was first examined for the overall averaged FC, calculated by averaging z-scores from the 28 pairwise correlations between ROIs. Further examination of model fit then took place in each pairwise FC. Group × condition interaction effects and main effects of group and condition were explored in models with significant fixed-effect model fit. Then, significant post-hoc simple effect testing was performed and corrected for multiple testing using the FDR method. Analyses were repeated, covarying for FSIQ and medications in each model to assess their influence in each model. Finally, Pearson's correlations assessed the relation between FC strength, primary measures of clinical traits, and task performance during ToM, corrected for multiple testing with the FDR method.
Results
Task Performance Results
In the performance data from the out-of-scanner task, there were no significant group × condition interactions (Supplementary Table 1). No group differences were found in ratings of intention attributions [F(3, 77) = 2.09, p = 0.1] and their appropriateness [F(3, 77) = 0.88, p = 0.5]. A group effect was found on the length of description [F(3, 77) = 2.73, p < 0.05] and prompts required [F(3, 77) = 3.48, p = 0.02; Figure 1A, Supplementary Table 1]. Post-hoc t-tests showed that the ASD group gave the shortest ToM descriptions (ps < 0.01), despite receiving more prompts than the ADHD (p < 0.01) and TD groups (p < 0.05). An effect of condition was found on the intentionality scores [F(1, 77) = 239.0, p < 0.0001], appropriateness scores [F(1, 77) = 33.3, p < 0.0001], length of description [F(1, 77) = 117.3, p < 0.0001] and prompts [F(1, 77) = 184.3, p < 0.0001]. Post-hoc t-tests suggested that intentionality scores and length of description were higher in ToM than GD across all groups (p < 0.001); appropriateness scores were lower in ToM than GD in the TD, ASD, and ADHD groups (p < 0.001); and more prompts were needed during ToM than the GD condition (p < 0.0001).
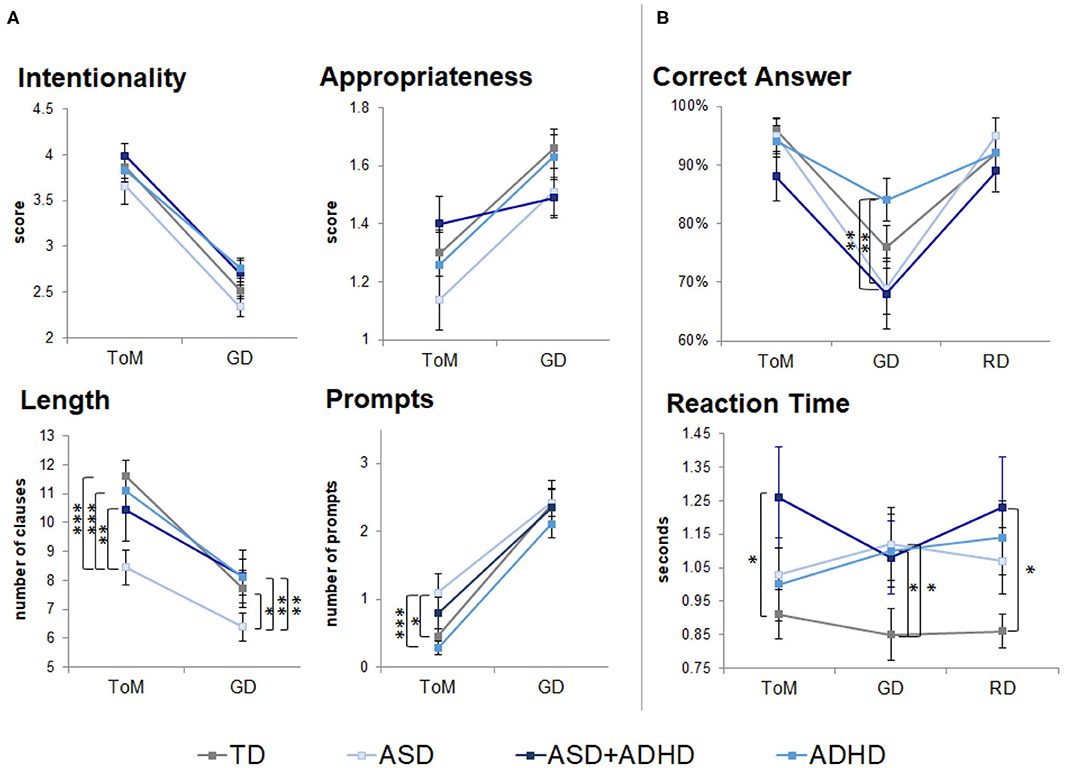
Figure 1. Behavioral performance outside (neuropsychological task) and during fMRI scanning (fMRI task). TD, typical development; ASD, autism spectrum disorder; ADHD, attention-deficit/hyperactivity disorder; GD, goal directed; RD, random movement; ToM, theory of mind. Only pairwise group differences are highlighted although an effect of condition was found in scores for intentionality, appropriateness, length of descriptions and number of prompts during neuropsychological task. An effect of condition was also found for correct identification of motion (correct answers) during the fMRI task. *p < 0.05, **p < 0.01, ***p < 0.001, with Tukey–Kramer multiple comparison correction. Error bars represent standard error of the mean. (A) Neuropsychological task behavioral results. (B) fMRI task behavioral results.
During the fMRI task, a participant with ADHD answered eight out of 12 questions (others answered ≥11 questions), which could reflect ToM difficulties. The participant was included in all analysis. There were no significant group × condition interactions (Supplementary Table 1). A trend-level group effect [F(3, 79) = 2.49, p = 0.07] and a significant effect of condition [F(2, 158) = 42.3, p < 0.0001] were found for motion type identification (Figure 1B, Supplementary Table 1). Post-hoc t-tests showed GD motion were identified more accurately by the ADHD than the ASD and ASD+ADHD groups (p < 0.01), and that RD and ToM motions were identified more accurately than GD motions (p < 0.001) by the TD, ASD and ASD+ADHD groups. RT comparisons showed a trend effect of group [F(3, 79) = 2.60, p = 0.06], with the clinical groups being slower than the TD group, although this was only statistically significant in RD and ToM conditions for the ASD+ADHD group (p < 0.05) and in GD for the ASD and ADHD groups (p < 0.05) when tested post-hoc.
Motion
Total volume-to-volume head movement in the x, y, and z rotation and translation did not differ across groups [F(3, 79) = 0.74, p = 0.53].
Within-Group Brain Activation
For the ToM > RD contrast, TD controls showed increased activation in ToM relative to RD condition in a region extending from inferior/middle occipital gyri (IOG/MOG) to posterior inferior temporal gyri (ITG) (BA19/37). In ASD, increased activation for ToM > RD was observed in bilateral IOG/MOG/ITG, in precuneus/posterior cingulate (BA7/23), and in bilateral supramarginal/ANG/posterior superior temporal gyrus (STG), extending to right middle temporal gyrus (MTG)/temporal pole (BA 22/21/38). In ADHD, increased activation for ToM > RD was found in right occipital pole, while in ASD+ADHD, the increased activation extended from bilateral IOG/MOG/ITG to right fusiform and lingual gyri, and from bilateral posterior MTG to right posterior STG/supramarginal gyrus/ANG (BA42/39/21/22) (Figure 2).
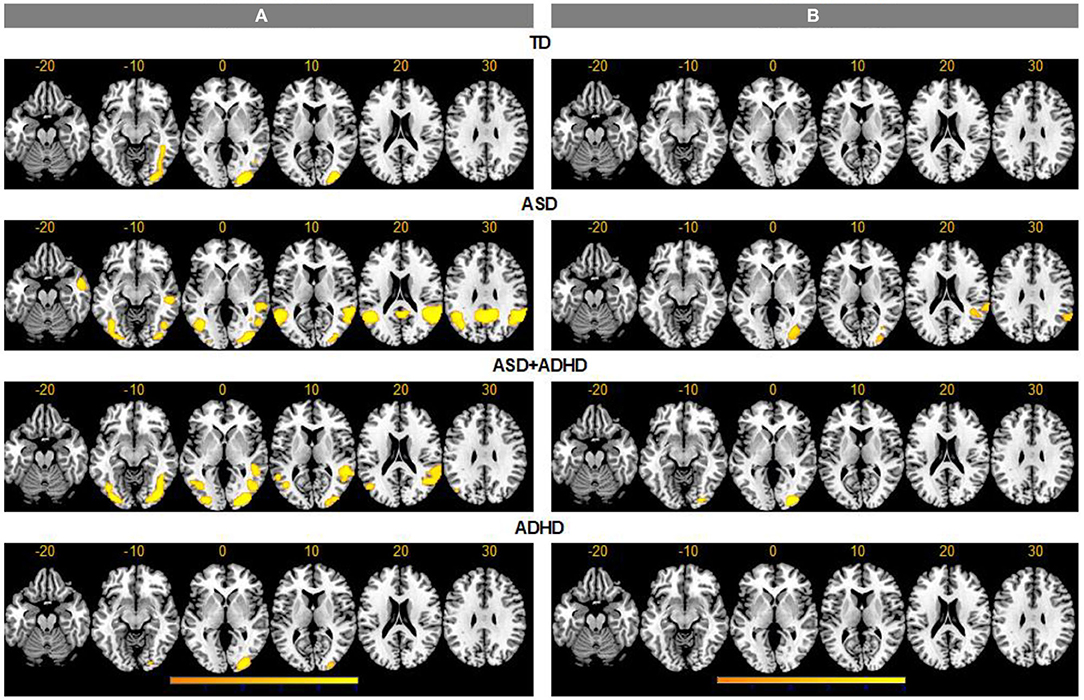
Figure 2. Activation clusters for theory of mind (ToM) over random (RD) and goal-directed (GD) motion experimental conditions. TD, typical development; ASD, autism spectrum disorder; ADHD, attention-deficit/hyperactivity disorder. (A) ToM > RD, (B) ToM > GD.
For the ToM > GD contrast, the TD and ADHD groups showed no clusters of increased activation at the chosen threshold. In ASD, increased activation was observed in right supramarginal/ANG/posterior STG (BA 42/41/40/22/48) and right IOG/MOG reaching into fusiform gyrus. In ASD+ADHD, increased activation was found in right IOG/MOG/lingual gyri (Figure 2).
Between-Group Brain Activation
Whole-brain analysis revealed no group effect for the contrast of ToM > GD. A group effect was observed for ToM > RD in the rANG [p =.005, F = 12.5, (x = 60, y = −54, z = 34), cluster size = 40 voxels]. Post-hoc pairwise comparisons revealed lower right ANG activation in the ADHD than the ASD (p < 0.001) and ASD+ADHD (p = 0.009) groups, and a trend-level increase in ASD relative to TD (p = 0.058; Figure 3A, Table 2).
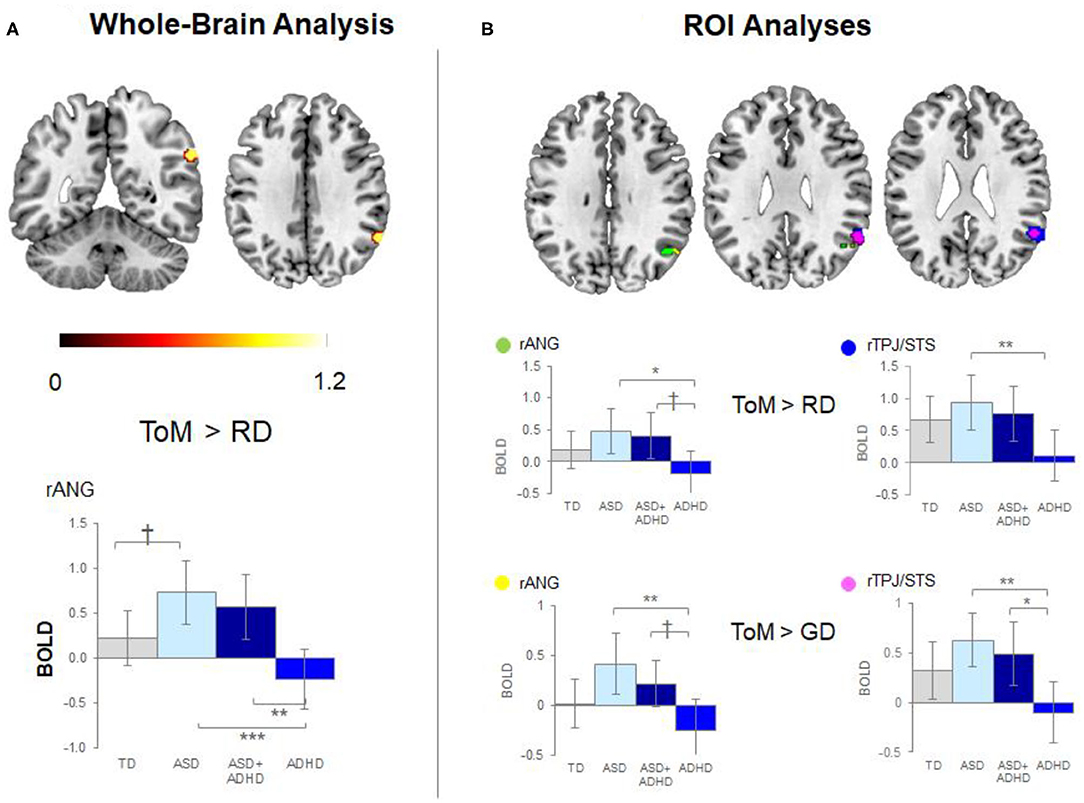
Figure 3. Significant peak activation by clusters and bar graphs showing within pairwise comparisons. (A) Whole-brain analysis and (B) Region of interest (ROI) analyses. TD, typical development; ASD, autism spectrum disorder; ADHD, attention-deficit/hyperactivity disorder; BOLD, blood-oxygen-level dependent; GD, goal directed; RD, random movement; ToM, theory of mind; rANG, right angular gyrus; rTPJ/STS, right temporoparietal junction/superior temporal sulcus *p < 0.05, **p < 0.01, ***p < 0.001, †p < 0.1 with Tukey–Kramer multiple comparison correction.
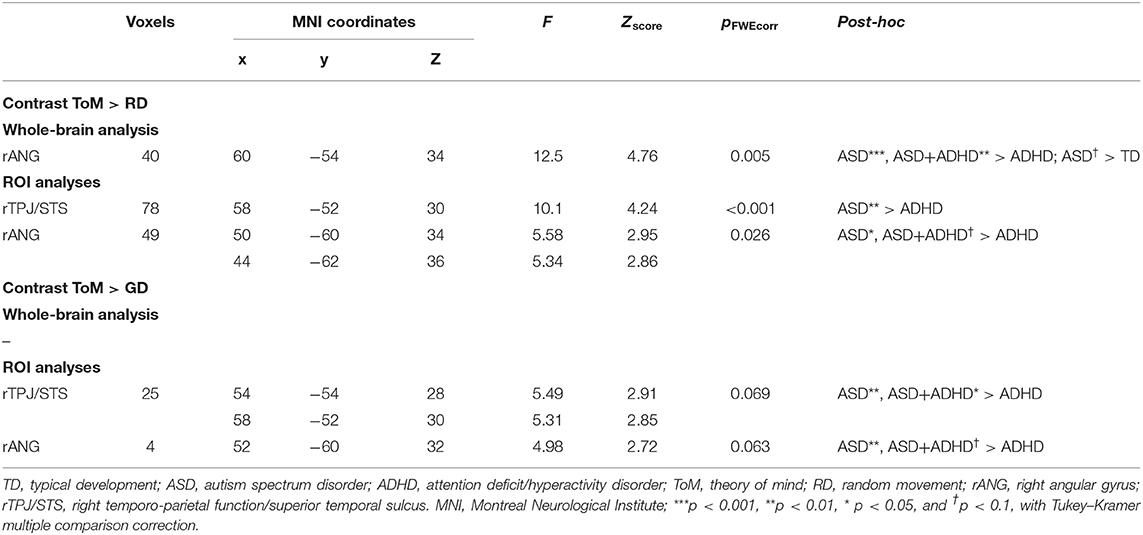
Table 2. Significant peak activation by cluster for the contrasts theory of mind (ToM) over random (RD) and goal-directed (GD) motion in the whole-brain and region-of-interest (ROI) analyses.
Between-group ROI analyses of ToM > RD revealed a group effect in rTPJ/STS [p < 0.001, F = 10.1, (x = 58, y = −52, z = 30), k = 78 voxels] and rANG [p =.026, F = 5.6, (x = 50, y = −60, z = 34), k = 49 voxels]. Post-hoc t-tests revealed significant higher activation in ASD than ADHD (ps ≤ 0.016) in both regions and a trend-level increase in rANG in ASD+ADHD relative to ADHD (p = 0.070). Corresponding analyses of ToM > GD contrast showed a trend-level group effect in rTPJ/STS [p = 0.069, F = 5.5, (x = 54, y = −54, z = 28), k = 25 voxels] and rANG [p = 0.063, F = 5.0, (x = 52, y = −60, z = 32), k = 4 voxels], with higher activation in ASD than ADHD (ps ≤ 0.004) for both regions, and a higher increase in ASD+ADHD than ADHD in rTPJ/STS (p = 0.025) and a trend-level increase rANG (p = 0.067; Figure 3B, Table 2).
In all analyses, the effects of FSIQ (ps ≥ 0.34), prescription of SSRI alone (ps ≥ 0.38), psychostimulant alone (ps ≥ 0.49), and all medication together (ps ≥ 0.17) were non-significant.
In the TD group, RT to ToM clips showed significant positive correlation with ROI-based activation, for the ToM > RD and ToM > GD contrasts, respectively, in rANG (r = 0.59, p = 0.008, r = 0.46, p = 0.021) and rTPJ/STS (r = 0.55, p = 0.014, r = 0.52, p = 0.016) and in the whole-brain analysis based rANG cluster for ToM > RD (r = 0.66, p = 0.002). In the ASD group, the number of prompts during ToM was significantly positively correlated with activation in rANG (r = 0.58, p = 0.022), and at trend-level, in the whole-brain rANG cluster (r = 0.44, p = 0.071) in the ToM > RD contrast; and with ROI activation in rANG (r = 0.63, p = 0.020) and, at a trend-level, in rTPJ (r = 0.45, p = 0.093) for the ToM > GD contrast.
None of the activation clusters correlated with the severity of ASD or ADHD traits (ps ≥ 0.26).
Functional Connectivity
Analyses of overall averaged FC revealed significant effects of condition [χ2(2) = 7.79, p = 0.02] and of group × condition interaction [χ2 (6) = 16.3, p = 0.012] but not group. Post-hoc t-test showed increased overall FC during ToM relative to RD or GD in TD controls only (ps ≤ 0.001), although the pattern of findings subtly differed for the individual FC pairs.
Among the ROI pairs, a significant mixed-effect model fit was found between rIFG and PCC, [χ2(11) = 46.6, p < 0.0001); and trend-level significance between mPFC and lTPJ [χ2(11) = 27.7, p = 0.051]; and between rANG with lTPJ [χ2(11) = 24.9, p = 0.076] and rTPJ [χ2(11) = 24.5, p = 0.076] (see Figure 4). Between rIFG and PCC, there was a significant effect of condition [χ2(2) = 36.4, p < 0.0001], qualified by increased FC during ToM relative to RD or GD in the TD (ps ≤ 0.0004) and the ADHD groups (ps ≤ 0.04). Between mPFC and lTPJ, a significant group × condition interaction [χ2(6) = 14.5, p = 0.024) and a significant group effect [χ2(3) = 9.3, p = 0.025) were found. Post-hoc analyses for the interaction showed FC increase in TD controls and decrease in ASD+ADHD and ADHD (ps ≤ 0.007) during ToM relative to RD; and comparable FC during ToM and GD in TD controls, and FC decrease during ToM relative to GD (ps ≤ 0.045) in ASD+ADHD and ADHD. Post-hoc analyses for the group effect showed increased FC in ADHD relative to TD controls during RD condition (p = 0.024), and relative to ASD during the GD condition (p = 0.024). Between rANG and bilateral TPJ, there was a significant effect of condition [rANG-lTPJ: χ2(2) = 10.2, p = 0.006; rANG-rTPJ χ2(2) = 15.8, p = 0.0004] due to increased FC in TD controls during ToM relative to RD or GD (ps ≤ 0.025) between rANG and rTPJ; and during ToM relative to GD (p = 0.002) between rANG and lTPJ. No other effects or interactions were significant.
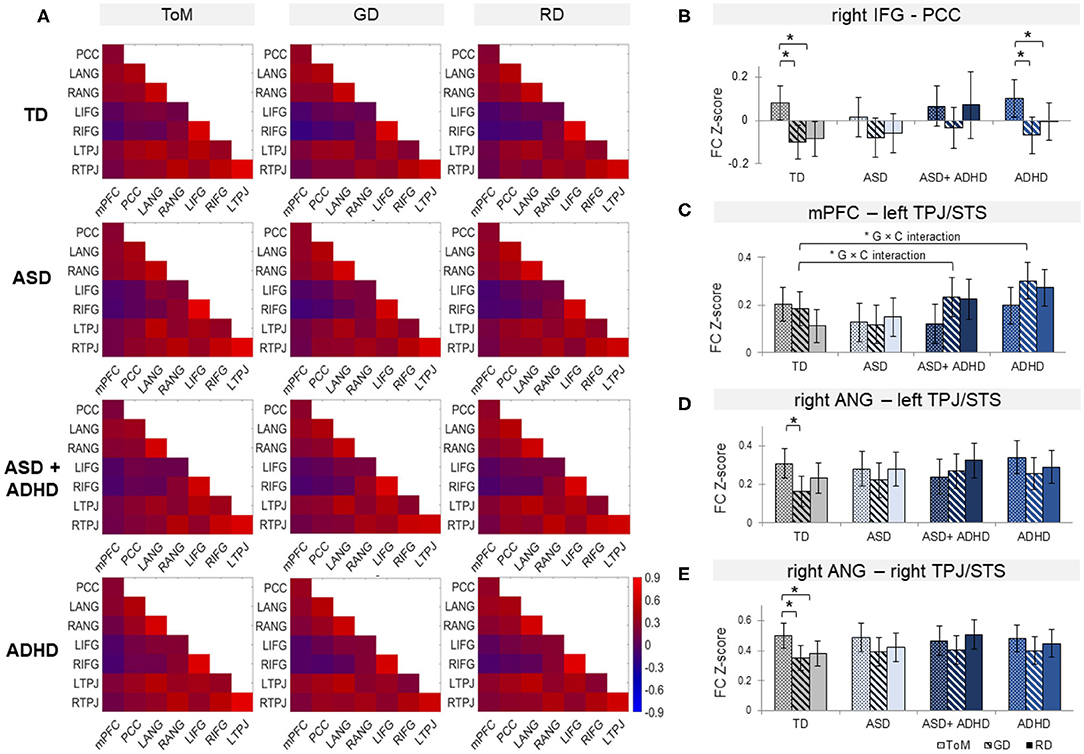
Figure 4. (A) Functional connectivity (FC) matrices within each group for theory of mind (ToM), goal-directed (GD), and random (RD) movement among medial Prefrontal Cortex (mPFC), posterior cingulate cortex (PCC), bilateral angular gyrus (ANG), bilateral inferior frontal gyrus (IFG), and bilateral temporoparietal junction (TPJ), combined with superior temporal sulcus (STS). Bar graphs of FC for right IFG-PCC (B), mPFC-left TPJ/STS (C), right ANG with left (D), and right TPJ/STS (E) by group and condition. *G × C represents significant group by condition interaction. Error bars represent 95% confidence interval. TD, typically developing controls; ASD, autism spectrum disorder; ADHD, attention-deficit/hyperactivity disorder. *p < 0.05 with false-discovery rate multiple comparison correction.
The FSIQ (ps ≥ 0.10), SSRIs alone (ps ≥ 0.72), psychostimulants alone (ps ≥ 0.42), and all medications together (ps ≥ 0.38) exerted non-significant effects in the models.
The FC strength neither correlated with the severity of ASD and ADHD traits (ps >0.39) nor task performance measures (ps > 0.11).
Discussion
To our knowledge, this is the first study to investigate brain activation and FC associated with ToM in young adult males with ASD, ADHD, ASD+ADHD, and TD. Despite comparable task performance, ASD adults with and without ADHD had increased activation relative to ADHD alone during mentalizing in a key temporo-parietal ToM region, which was trend-wise increased relative to TD in the ASD group without ADHD. In FC, during mentalizing, there were mixed patterns of findings with all clinical groups sharing the lack of increased average FC over all connectivity pairs when compared to TD, which was particularly significant when considering the individual FC pairs in posterior temporo-parietal regions. Furthermore, underconnectivity between right inferior frontal and posterior cingulate cortices was found in the ASD groups, with and without ADHD, while the decreasing FC in medial frontal cortex and left temporo-parietal junction with mentalizing was found only in the ADHD groups, with and without ASD.
Increased temporoparietal activation in rANG during mentalizing appears to differentiate adults with ASD, with or without ADHD, from those with ADHD alone, and at a trend-level from TD controls. Therefore, we show for the first time that this overactivation is ASD-specific relative to the ADHD. This finding was significant in whole-brain analyses of ToM > RD contrast, and either significant or at trend-level in ROI analyses of both mentalizing contrasts. Previous studies have shown reduced rANG activation in children and adults with ASD relative to controls (15, 17–19). However, some studies have also found overactivation in the social brain regions during mentalizing in children and adults with ASD relative to TD (21–24), typically concomitant with unimpaired mentalizing task performance. The present findings could reflect increased processing effort in ASD to perform mentalizing as well as the TD controls and ADHD group [e.g., (23, 24)]. Supporting this interpretation was the positive correlation between rANG activation and the increased prompts for describing ToM movement in ASD, and between rANG activation and RT for classifying ToM clips in TD controls.
Discrepancies of findings were observed across contrasts (ToM > GD vs. ToM > RD) and analysis types (e.g., whole brain vs. ROI). Specifically, the ToM > GD contrast, relative to ToM > RD in whole-brain and ROI analyses, reduced some brain activation differences to non-significance. Thus, GD motion seems to evoke temporoparietal activation at intermediate level between RD and ToM, which could express parametric modulation of right temporoparietal activation when observing interaction based on goal pursuit alone compared to interaction involving the attribution of mental states in others.
Overall, the clinical groups showed a lack of increased FC during ToM compared to TD controls. The underconnectivity between rANG and bilateral TPJ/STS in particular seemed in line with the findings of anterior and posterior TPJ dysconnectivity between ASD and ADHD during resting state (79). The right-lateralized local rANG-rTPJ/STS underconnectivity during ToM is interesting, given the strong evidence of a positive association between BOLD activation and local FC density during mentalizing (80). Specifically, the rANG activation in both ASD groups, with and without ADHD, exhibited opposite effect to what is expected from a reduced local temporoparietal connectivity between rANG and rTPJ. This supports the atypical brain hypothesis [e.g., (21–24, 27)], instead of the hypo-intentionality hypothesis of ASD (20), which now extends to the comorbid group.
The lack of increased connectivity in rIFG-PCC during ToM, was found in ASD and ASD+ADHD but not in ADHD or TD controls. As well as implicated during mentalizing, the rIFG is part of the mirroring networks and is implicated in action processing and social attention. The rIFG is hypothesized as a gateway into the mentalizing networks (81, 82), which include the PCC. Thus, the rIFG-PCC underconnectivity may reflect information transmission failures across the two networks (26, 30), which is ASD-differentiating.
Relative to TD controls, ADHD and ASD+ADHD showed decreasing FC between mPFC and lTPJ with increasing ToM. Functional coupling between mPFC and the temporal cortices occurs bilaterally during mentalizing [e.g., (28, 75, 82, 83)]. While it has not been discussed as much as the rTPJ in the context of mentalizing, the lTPJ is implicated in explicit ToM in meta-analytic connectivity modeling (84), and lesions in the region impaired spontaneous ToM in adults (85, 86). Those specific subdomains of ToM difficulties could perhaps be investigated further in ADHD.
The findings of the study should be viewed with its strengths and limitations. Firstly, the sample size was relatively small, which may have reduced statistical power to detect small effects and increased probability of false positives. The inclusion of young adult males only enhanced the group homogeneity at the expense of the finding's generalizability to other population groups, including female, children, and older adults with the conditions. The absence of correlations between primary ToM measures and brain activation and connectivity also constrained the interpretation of findings. There was limited variance of task performance across all participant groups, which could have reflected reduced symptom severity in non-clinically referred participants (87) or a lack of sensitivity of the simplistic animated-triangle fMRI task for detecting differences between groups. Finally, it is also possible that ToM impairments are present in ADHD in a subtle form that was undetectable by the animated triangle task. Future studies could investigate clinically referred participants, using more complex and naturalistic mentalizing tasks [e.g., (18, 26)].
To summarize, despite evidence of reduced connectivity in all three clinical groups relative to TD controls during ToM, a differentially increased activation pattern was found in both ASD and comorbid groups relative to ADHD in right temporoparietal cortex, which is a key mentalizing region. Both ASD and comorbid groups also showed reduced right inferior frontal and posterior cingulate coupling, which may reflect an atypical information transmission to the mentalizing network. In contrast, both ADHD and comorbid groups showed decreasing connectivity between medial prefrontal and left temporoparietal cortices with increasing ToM when compared to TD controls. These findings denote a complex pattern of atypical brain function underpinning mentalizing in these three conditions in young adult males, with some evidence of ASD- and ADHD-differentiating features and a combined neurofunctional atypicality in the comorbid group.
Data Availability Statement
The datasets presented in this study can be found in online repositories. The names of the repository/repositories and accession number(s) can be found at: https://osf.io/nrj8g/.
Ethics Statement
The studies involving human participants were reviewed and approved by National Health Service Research Ethics Committee (NHS REC 13/LO/0373). The patients/participants provided their written informed consent to participate in this study.
Author Contributions
SL, KR, and ES conceptualized the study. CMM, KA, and VS contributed to recruitment. SL conducted the recruitment and data collection. SL, DI, OO'D, and CM contributed to the analysis of the data. SL and DI drafted the manuscript. DL, CMM, CM, KA, VS, KR, and ES contributed to the manuscript preparation. All authors contributed to the article and approved the submitted version.
Funding
DI has held a Río Hortega grant from Instituto de Salud Carlos III, Spanish Ministry of Science, Innovation and Universities and a Fellowship in Child and Adolescent Psychiatry and Psychology from Fundación Alicia Koplowitz. SL was supported by the UK Medical Research Council (MRC) and IoPPN PhD Excellence awards. SL is supported by an MRC Grant MR/P012647/1 to KR. KR also receives research funding from Shire/Takeda and Action Medication Research. ES and KR receive research support from the National Institute of Health Research (NIHR) Biomedical Research Center at South London and Maudsley Foundation Trust (IS-BRC-1215-20018). ES furthermore is supported by the NIHR through a program grant (RP-PG-1211-20016) and Senior Investigator Award (NF-SI-0514-10073), the European Union Innovative Medicines Initiative (EU-IMI 115300), Autistica (7237) Medical Research Council (MR/R000832/1, MR/P019293/1), the Economic and Social Research Council (ESRC 003041/1), and Guy's and St Thomas' Charitable Foundation (GSTT EF1150502) and the Maudsley Charity. This study is supported by funding from the UK Department of Health via the National Institute for Health Research (NIHR) Biomedical Research Center (BRC) for Mental Health at South London and the Maudsley National Health Service (NHS) Foundation Trust and the IoPPN, King's College London, which also support both senior authors, ES and KR. The views expressed are those of the authors and not necessarily those of the NHS, the NIHR or the Department of Health.
Conflict of Interest
DI has received honoraria and travel support from Otsuka-Lundbeck and Janssen. KR has received a grant from Shire/Takeda for another study.
The remaining authors declare that the research was conducted in the absence of any commercial or financial relationships that could be construed as a potential conflict of interest.
Acknowledgments
We thank the young adults and families who took part in this study and the Adult ADHD and ASD Service at South London and Maudsley Hospital (SLaM) NHS Foundation Trust for their support in this study. With thanks to Siobhan Higgins, Teresa Schenk, and Mavis Nyakunengwa for their help with participant recruitment, to Allison Cooper and the neuroimaging team at the Center for Neuroimaging Sciences at King's College London for their support with the imaging data acquisition. We thank Dr Sarah White at the Institute of Cognitive Neuroscience, University College London for providing us with the stimuli for the task. The fMRI task in this study is aligned to that used by the European Autism Interventions—A Multicentre Study for Developing New Medications (EU-AIMS) study.
Supplementary Material
The Supplementary Material for this article can be found online at: https://www.frontiersin.org/articles/10.3389/fpsyt.2020.544482/full#supplementary-material
Abbreviations
ADHD, attention-deficit/hyperactivity disorder; ASD, autism spectrum disorder; FC, functional connectivity; GD, goal directed movement; fMRI, functional Magnetic Resonance Imaging; TD, typically developing controls; mPFC, medial prefrontal cortex; ToM, Theory of mind; TPJ, temporoparietal junction; rANG, right angular gyrus; RD, random movement; ROI, region-of-interest.
References
1. American Psychiatric Association (APA). Diagnostic and Statistical Manual of Mental Disorders: DSM-5, APA. Washington, DC: American Psychiatric Association Publishing (2013).
2. Lai MC, Kassee C, Besney R, Bonato S, Hull L, Mandy W, et al. Prevalence of co-occurring mental health diagnoses in the autism population: a systematic review and meta-analysis. Lancet Psychiatry. (2019) 6:819–29. doi: 10.1016/S2215-0366(19)30289-5
3. Hollingdale J, Woodhouse E, Young S, Fridman A, Mandy W. Autistic spectrum disorder symptoms in children and adolescents with attention-deficit/hyperactivity disorder: a meta-analytical review. Psychol Med. (2020) 50:2240–53. doi: 10.1017/S0033291719002368
4. Joshi G, Wozniak J, Petty C, Martelon MK, Fried R, Bolfek A, et al. Psychiatric comorbidity and functioning in a clinically referred population of adults with autism spectrum disorders: a comparative study. J Autism Dev Disord. (2013) 43:1314–25. doi: 10.1007/s10803-012-1679-5
5. Houghton R, Ong RC, Bolognani F. Psychiatric comorbidities and use of psychotropic medications in people with autism spectrum disorder in the United States. Autism Res. (2017) 10:2037–47. doi: 10.1002/aur.1848
6. Solberg BS, Zayats T, Posserud MB, Halmoy A, Engeland A, Haavik J, et al. Patterns of psychiatric comorbidity and genetic correlations provide new insights into differences between attention-deficit/hyperactivity disorder and autism spectrum disorder. Biol Psychiatry. (2019) 86:587–98. doi: 10.1016/j.biopsych.2019.04.021
7. Craig F, Margari F, Legrottaglie AR, Palumbi R, de Giambattista C, Margari L. A review of executive function deficits in autism spectrum disorder and attention-deficit/hyperactivity disorder. Neuropsychiatr Dis Treat. (2016) 12:1191–202. doi: 10.2147/NDT.S104620
8. Bora E, Pantelis C. Meta-analysis of social cognition in attention-deficit/hyperactivity disorder (ADHD): comparison with healthy controls and autistic spectrum disorder. Psychol Med. (2016) 46:699–716. doi: 10.1017/S0033291715002573
9. Miranda A, Berenguer C, Rosello B, Baixauli I, Colomer C. Social cognition in children with high-functioning autism spectrum disorder and attention-deficit/hyperactivity disorder. Associations with executive functions. Front Psychol. (2017) 8:1035. doi: 10.3389/fpsyg.2017.01035
10. Bal E, Yerys BE, Sokoloff JL, Celano MJ, Kenworthy L, Giedd JN, et al. Do social attribution skills improve with age in children with high functioning autism spectrum disorders? Res Autism Spectr Disord. (2013) 7:9–16. doi: 10.1016/j.rasd.2012.07.004
11. Baron-Cohen S, Leslie AM, Frith U. Does the autistic child have a “theory of mind”? Cognition. (1985) 21:37–46. doi: 10.1016/0010-0277(85)90022-8
12. Happé FG. An advanced test of theory of mind: understanding of story characters' thoughts and feelings by able autistic, mentally handicapped, and normal children and adults. J Autism Dev Disord. (1994) 24:129–54. doi: 10.1007/BF02172093
13. White SJ, Coniston D, Rogers R, Frith U. Developing the frith-happé animations: a quick and objective test of theory of mind for adults with autism. Autism Res. (2011) 4:149–54. doi: 10.1002/aur.174
14. Chevallier C, Kohls G, Troiani V, Brodkin ES, Schultz RT. The social motivation theory of autism. Trends Cogn Sci. (2012) 16:231–39. doi: 10.1016/j.tics.2012.02.007
15. O'Nions E, Sebastian CL, McCrory E, Chantiluke K, Happe F, Viding E. Neural bases of theory of mind in children with autism spectrum disorders and children with conduct problems and callous-unemotional traits. Dev Sci. (2014) 17:786–96. doi: 10.1111/desc.12167
16. Sugranyes G, Kyriakopoulos M, Corrigall R, Taylor E, Frangou S. Autism spectrum disorders and schizophrenia: meta-analysis of the neural correlates of social cognition. PLoS ONE. (2011) 6:e25322. doi: 10.1371/journal.pone.0025322
17. Lombardo MV, Chakrabarti B, Bullmore ET, Baron-Cohen S. Specialization of right temporo-parietal junction for mentalizing and its relation to social impairments in autism. Neuroimage. (2011) 56:1832–38. doi: 10.1016/j.neuroimage.2011.02.067
18. Pantelis PC, Byrge L, Tyszka JM, Adolphs R, Kennedy DP. A specific hypoactivation of right temporo-parietal junction/posterior superior temporal sulcus in response to socially awkward situations in autism. Soc Cogn Affect Neurosci. (2015) 10:1348–56. doi: 10.1093/scan/nsv021
19. Nijhof AD, Bardi L, Brass M, Wiersema JR. Brain activity for spontaneous and explicit mentalizing in adults with autism spectrum disorder: an fMRI study. Neuroimage Clin. (2018) 18:475–84. doi: 10.1016/j.nicl.2018.02.016
20. Ciaramidaro A, Bolte S, Schlitt S, Hainz D, Poustka F, Weber B, et al. Schizophrenia and autism as contrasting minds: neural evidence for the hypo-hyper-intentionality hypothesis. Schizophr Bull. (2015) 41:171–79. doi: 10.1093/schbul/sbu124
21. Kana RK, Keller TA, Cherkassky VL, Minshew NJ, Just MA. Atypical frontal-posterior synchronization of theory of mind regions in autism during mental state attribution. Soc Neurosci. (2009) 4:135–52. doi: 10.1080/17470910802198510
22. Mason RA, Williams DL, Kana RK, Minshew N, Just MA. Theory of mind disruption and recruitment of the right hemisphere during narrative comprehension in autism. Neuropsychologia. (2008) 46:269–80. doi: 10.1016/j.neuropsychologia.2007.07.018
23. Kim E, Kyeong S, Cheon KA, Park B, Oh MK, Chun JW, et al. Neural responses to affective and cognitive theory of mind in children and adolescents with autism spectrum disorder. Neurosci Lett. (2016) 621:117–25. doi: 10.1016/j.neulet.2016.04.026
24. White SJ, Frith U, Rellecke J, Al-Noor Z, Gilbert SJ. Autistic adolescents show atypical activation of the brain's mentalizing system even without a prior history of mentalizing problems. Neuropsychologia. (2014) 56:17–25. doi: 10.1016/j.neuropsychologia.2013.12.013
25. Lombardo MV, Chakrabarti B, Bullmore ET, Sadek SA, Pasco G, Wheelwright SJ, et al. Atypical neural self-representation in autism. Brain. (2010) 133:611–24. doi: 10.1093/brain/awp306
26. Cole EJ, Barraclough NE, Andrews TJ. Reduced connectivity between mentalizing and mirror systems in autism spectrum condition. Neuropsychologia. (2019) 122:88–97. doi: 10.1016/j.neuropsychologia.2018.11.008
27. Kana RK, Maximo JO, Williams DL, Keller TA, Schipul SE, Cherkassky VL, et al. Aberrant functioning of the theory-of-mind network in children and adolescents with autism. Mol Autism. (2015) 6:59. doi: 10.1186/s13229-015-0052-x
28. Burnett S, Blakemore SJ. Functional connectivity during a social emotion task in adolescents and in adults. Eur J Neurosci. (2009) 29:1294–301. doi: 10.1111/j.1460-9568.2009.06674.x
29. Klapwijk ET, Goddings AL, Burnett Heyes S, Bird G, Viner RM, Blakemore SJ. Increased functional connectivity with puberty in the mentalising network involved in social emotion processing. Horm Behav. (2013) 64:314–22. doi: 10.1016/j.yhbeh.2013.03.012
30. Libero LE, Maximo JO, Deshpande HD, Klinger LG, Klinger MR, Kana RK. The role of mirroring and mentalizing networks in mediating action intentions in autism. Mol Autism. (2014) 5:50. doi: 10.1186/2040-2392-5-50
31. Just MA, Keller TA, Malave VL, Kana RK, Varma S. Autism as a neural systems disorder: a theory of frontal-posterior underconnectivity. Neurosci Biobehav Rev. (2012) 36:1292–313. doi: 10.1016/j.neubiorev.2012.02.007
32. Koshino H, Kana RK, Keller TA, Cherkassky VL, Minshew NJ, Just MA. fMRI investigation of working memory for faces in autism: visual coding and underconnectivity with frontal areas. Cereb Cortex. (2008) 18:289–300. doi: 10.1093/cercor/bhm054
33. Kana RK, Keller TA, Minshew NJ, Just MA. Inhibitory control in high-functioning autism: decreased activation and underconnectivity in inhibition networks. Biol Psychiatry. (2007) 62:198–206. doi: 10.1016/j.biopsych.2006.08.004
34. Just MA, Keller TA, Kana RK. A theory of autism based on frontal-posterior underconnectivity. In: Just MA, Pelphrey KA, editors. Development and Brain Systems in Autism. New York, NY: Psychology Press (2013).
35. Mohammadzadeh A, Tehrani-Doost M, Khorrami A, Noorian N. Understanding intentionality in children with attention-deficit/hyperactivity disorder. Atten Defic Hyperact Disord. (2016) 8:73–8. doi: 10.1007/s12402-015-0187-9
36. Maoz H, Gvirts HZ, Sheffer M, Bloch Y. Theory of mind and empathy in children with ADHD. J Atten Disord. (2019) 23:1331–38. doi: 10.1177/1087054717710766
37. Mary A, Slama H, Mousty P, Massat I, Capiau T, Drabs V, et al. Executive and attentional contributions to theory of mind deficit in attention deficit/hyperactivity disorder (ADHD). Child Neuropsychol. (2016) 22:345–65. doi: 10.1080/09297049.2015.1012491
38. Caillies S, Bertot V, Motte J, Raynaud C, Abely M. Social cognition in ADHD: irony understanding and recursive theory of mind. Res Dev Disabil. (2014) 35:3191–8. doi: 10.1016/j.ridd.2014.08.002
39. Buitelaar JK, van der Wees M, Swaab-Barneveld H, van der Gaag RJ. Theory of mind and emotion-recognition functioning in autistic spectrum disorders and in psychiatric control and normal children. Dev Psychopathol. (1999) 11:39–58. doi: 10.1017/S0954579499001947
40. Ibáñez A, Aguado J, Baez S, Huepe D, Lopez V, Ortega R, et al. From neural signatures of emotional modulation to social cognition: individual differences in healthy volunteers and psychiatric participants. Soc Cogn Affect Neurosci. (2014) 9:939–50. doi: 10.1093/scan/nst067
41. Nilsen ES, Mewhort Buist TA, Gillis R, Fugelsang J. Communicative perspective-taking performance of adults with ADHD symptoms. J Atten Disord. (2013) 17:589–97. doi: 10.1177/1087054711428947
42. Perroud N, Badoud D, Weibel S, Nicastro R, Hasler R, Kung AL, et al. Mentalization in adults with attention deficit hyperactivity disorder: comparison with controls and patients with borderline personality disorder. Psychiatry Res. (2017) 256:334–41. doi: 10.1016/j.psychres.2017.06.087
43. Tatar ZB, Cansiz A. Executive function deficits contribute to poor theory of mind abilities in adults with ADHD. Appl Neuropsychol Adult. (2020). doi: 10.1080/23279095.2020.1736074. [Epub ahead of print].
44. Abdel-Hamid M, Niklewski F, Hessmann P, Guberina N, Kownatka M, Kraemer M, et al. Impaired empathy but no theory of mind deficits in adult attention deficit hyperactivity disorder. Brain Behav. (2019) 9:e01401. doi: 10.1002/brb3.1401
45. Thoma P, Sonnenburg S, Marcinkowski N, Juckel G, Edel MA, Suchan B. Social problem solving in adult patients with attention deficit hyperactivity disorder. Psychiatry Res. (2020) 285:112721. doi: 10.1016/j.psychres.2019.112721
46. Norman LJ, Carlisi C, Lukito S, Hart H, Mataix-Cols D, Radua J, et al. Structural and functional brain abnormalities in attention-deficit/hyperactivity disorder and obsessive-compulsive disorder: a comparative meta-analysis. JAMA Psychiatry. (2016) 73:815–25. doi: 10.1001/jamapsychiatry.2016.0700
47. Rubia K. Cognitive neuroscience of attention deficit hyperactivity disorder (ADHD) and its clinical translation. Front Hum Neurosci. (2018) 12:100. doi: 10.3389/fnhum.2018.00100
48. Lukito S, Norman L, Carlisi C, Radua J, Hart H, Simonoff E, et al. Comparative meta-analyses of brain structural and functional abnormalities during cognitive control in attention-deficit/hyperactivity disorder and autism spectrum disorder. Psychol Med. (2020) 50:894–919. doi: 10.1017/S0033291720000574
49. Nijmeijer JS, Minderaa RB, Buitelaar JK, Mulligan A, Hartman CA, Hoekstra PJ. Attention-deficit/hyperactivity disorder and social dysfunctioning. Clin Psychol Rev. (2008) 28:692–708. doi: 10.1016/j.cpr.2007.10.003
50. Uekermann J, Kraemer M, Abdel-Hamid M, Schimmelmann BG, Hebebrand J, Daum I, et al. Social cognition in attention-deficit hyperactivity disorder (adhd). Neurosci Biobehav Rev. (2010) 34:734–43. doi: 10.1016/j.neubiorev.2009.10.009
51. Malisza KL, Clancy C, Shiloff D, Holden J, Jones C, Paulson K, et al. Functional magnetic resonance imaging of facial information processing in children with autistic disorder, attention deficit hyperactivity disorder and typically developing controls. Int J Adolesc Med Health. (2011) 23:269–77. doi: 10.1515/ijamh.2011.055
52. Tye C, Battaglia M, Bertoletti E, Ashwood KL, Azadi B, Asherson P, et al. Altered neurophysiological responses to emotional faces discriminate children with ASD, ADHD and ASD+ADHD. Biol Psychol. (2014) 103:125–34. doi: 10.1016/j.biopsycho.2014.08.013
53. Tye C, Mercure E, Ashwood KL, Azadi B, Asherson P, Johnson MH, et al. Neurophysiological responses to faces and gaze direction differentiate children with ASD, ADHD and ASD+ADHD. Dev Cogn Neurosci. (2013) 5:71–85. doi: 10.1016/j.dcn.2013.01.001
54. Groom MJ, Kochhar P, Hamilton A, Liddle EB, Simeou M, Hollis C. Atypical processing of gaze cues and faces explains comorbidity between autism spectrum disorder (ASD) and attention deficit/hyperactivity disorder (ADHD). J Autism Dev Disord. (2017) 47:1496–509. doi: 10.1007/s10803-017-3078-4
55. Di Martino A, Zuo X-N, Kelly C, Grzadzinski R, Mennes M, Schvarcz A, et al. Shared and distinct intrinsic functional network centrality in autism and attention-deficit/hyperactivity disorder. Biol Psychiatry. (2013) 74:623–32. doi: 10.1016/j.biopsych.2013.02.011
56. Wechsler D. Wechsler Abbreviated Scale of Intelligence - 2nd Edition (WASI-II). San Antonio: Pearson (2011).
57. Fayyad J, De Graaf R, Kessler R, Alonso J, Angermeyer M, Demyttenaere K, et al. Cross-national prevalence and correlates of adult attention-deficit hyperactivity disorder. Br J Psychiatry. (2007) 190:402–9. doi: 10.1192/bjp.bp.106.034389
58. Fombonne E. Epidemiology of autistic disorder and other pervasive developmental disorders. J Clin Psychiatry. (2005) 66 (Suppl. 10):3–8.
59. Oldfield RC. The assessment and analysis of handedness: the edinburgh inventory. Neuropsychologia. (1971) 9:97–113. doi: 10.1016/0028-3932(71)90067-4
60. Lukito SD, O'Daly OG, Lythgoe DJ, Whitwell S, Debnam A, Murphy CM, et al. Neural correlates of duration discrimination in young adults with autism spectrum disorder, attention-deficit/hyperactivity disorder and their comorbid presentation. Front Psychiatry. (2018) 9:569. doi: 10.3389/fpsyt.2018.00569
61. Baird G, Simonoff E, Pickles A, Chandler S, Loucas T, Meldrum D, et al. Prevalence of disorders of the autism spectrum in a population cohort of children in South Thames: the special needs and Autism project (SNAP). Lancet. (2006) 368:210–5. doi: 10.1016/S0140-6736(06)69041-7
62. World Health Organization. ICD-10: International Statistical Classification of Diseases and Related Health Problems Tenth Revision. Geneva: World Health Organization (2004).
63. Lord C, Risi S, Lambrecht L, Cook EH Jr, Leventhal BL, et al. The autism diagnostic observation schedule-generic: a standard measure of social and communication deficits associated with the spectrum of autism. J Autism Dev Disord. (2000) 30:205–23. doi: 10.1023/A:1005592401947
64. Lord C, Rutter M, Le Couteur A. Autism diagnostic interview-revised: a revised version of a diagnostic interview for caregivers of individuals with possible pervasive developmental disorders. J Autism Dev Disord. (1994) 24:659–85. doi: 10.1007/BF02172145
65. Wing L, Leekam SR, Libby SJ, Gould J, Larcombe M. The diagnostic interview for social and communication disorders: background, inter-rater reliability and clinical use. J Child Psychol Psychiatry. (2002) 43:307–25. doi: 10.1111/1469-7610.00023
67. Epstein JN, Johnson DE, Conners CK. Conners' Adult ADHD Diagnostic Interview for DSM-IV. North Tonawanda, NY: Multi-Health Systems Inc (2001).
68. Angold A, Cox A, Prendergast M, Rutter M, Simonoff E. The Young Adult Psychiatric Assessment (YAPA) Version 2.0.3. Durham: Duke University (2009).
69. Conners CK, Erhardt D, Sparrow E. Conners' Adult ADHD Rating Scales (CAARS): Technical Manual. New York, Toronto: Multi-Health Systems Inc (1999).
70. Constantino JN, Gruber C. Social Responsiveness Scale™, Second Edition (SRS™-2). Torrance: Western Psychological Services (2012).
71. Moessnang C, Schafer A, Bilek E, Roux P, Otto K, Baumeister S, et al. Specificity, reliability and sensitivity of social brain responses during spontaneous mentalizing. Soc Cogn Affect Neurosci. (2016) 11:1687–97. doi: 10.1093/scan/nsw098
72. Abell F, Happe F, Frith U. Do triangles play tricks? Attribution of mental states to animated shapes in normal and abnormal development. Cogn Dev. (2000) 15:1–16. doi: 10.1016/S0885-2014(00)00014-9
74. Das P, Lagopoulos J, Coulston CM, Henderson AF, Malhi GS. Mentalizing impairment in schizophrenia: a functional MRI study. Schizophr Res. (2012) 134:158–64. doi: 10.1016/j.schres.2011.08.019
75. Das P, Calhoun V, Malhi GS. Mentalizing in male schizophrenia patients is compromised by virtue of dysfunctional connectivity between task-positive and task-negative networks. Schizophr Res. (2012) 140:51–8. doi: 10.1016/j.schres.2012.06.023
76. Gobbini MI, Koralek AC, Bryan RE, Montgomery KJ, Haxby JV. Two takes on the social brain: a comparison of theory of mind tasks. J Cogn Neurosci. (2007) 19:1803–14. doi: 10.1162/jocn.2007.19.11.1803
77. Gorgolewski KJ, Varoquaux G, Rivera G, Schwarz Y, Ghosh SS, Maumet C, et al. Neurovault.Org: a web-based repository for collecting and sharing unthresholded statistical maps of the human brain. Front Neuroinform. (2015) 9:8. doi: 10.3389/fninf.2015.00008
78. Fishman I, Keown CL, Lincoln AJ, Pineda JA, Muller RA. Atypical cross talk between mentalizing and mirror neuron networks in autism spectrum disorder. JAMA Psychiatry. (2014) 71:751–60. doi: 10.1001/jamapsychiatry.2014.83
79. Kernbach JM, Satterthwaite TD, Bassett DS, Smallwood J, Margulies D, Krall S, et al. Shared endo-phenotypes of default mode dysfunction in attention deficit/hyperactivity disorder and autism spectrum disorder. Transl Psychiatry. (2018) 8:133. doi: 10.1038/s41398-018-0179-6
80. Tomasi D, Volkow ND. Association between brain activation and functional connectivity. Cereb Cortex. (2019) 29:1984–96. doi: 10.1093/cercor/bhy077
81. Enrici I, Adenzato M, Cappa S, Bara BG, Tettamanti M. Intention processing in communication: a common brain network for language and gestures. J Cogn Neurosci. (2011) 23:2415–31. doi: 10.1162/jocn.2010.21594
82. Tettamanti M, Vaghi MM, Bara BG, Cappa SF, Enrici I, Adenzato M. Effective connectivity gateways to the theory of mind network in processing communicative intention. Neuroimage. (2017) 155:169–76. doi: 10.1016/j.neuroimage.2017.04.050
83. Atique B, Erb M, Gharabaghi A, Grodd W, Anders S. Task-specific activity and connectivity within the mentalizing network during emotion and intention mentalizing. Neuroimage. (2011) 55:1899–911. doi: 10.1016/j.neuroimage.2010.12.036
84. Molenberghs P, Johnson H, Henry JD, Mattingley JB. Understanding the minds of others: a neuroimaging meta-analysis. Neurosci Biobehav Rev. (2016) 65:276–91. doi: 10.1016/j.neubiorev.2016.03.020
85. Biervoye A, Dricot L, Ivanoiu A, Samson D. Impaired spontaneous belief inference following acquired damage to the left posterior temporoparietal junction. Soc Cogn Affect Neurosci. (2016) 11:1513–20. doi: 10.1093/scan/nsw076
86. Samson D, Apperly IA, Chiavarino C, Humphreys GW. Left temporoparietal junction is necessary for representing someone else's belief. Nat Neurosci. (2004) 7:499–500. doi: 10.1038/nn1223
Keywords: theory of mind (ToM), neurodevelopmental disorder, attention deficit and hyperactivity disorder (ADHD), autism spectrum disoder (ASD), functional magnetic resonance imaging (fMRI)
Citation: Ilzarbe D, Lukito S, Moessnang C, O'Daly OG, Lythgoe DJ, Murphy CM, Ashwood K, Stoencheva V, Rubia K and Simonoff E (2020) Neural Correlates of Theory of Mind in Autism Spectrum Disorder, Attention-Deficit/Hyperactivity Disorder, and the Comorbid Condition. Front. Psychiatry 11:544482. doi: 10.3389/fpsyt.2020.544482
Received: 20 March 2020; Accepted: 25 September 2020;
Published: 06 November 2020.
Edited by:
Sara Calderoni, Fondazione Stella Maris (IRCCS), ItalyReviewed by:
Inna Fishman, San Diego State University, United StatesTakashi Itahashi, Showa University, Japan
Ryu-Ichiro Hashimoto, Showa University, Japan
Copyright © 2020 Ilzarbe, Lukito, Moessnang, O'Daly, Lythgoe, Murphy, Ashwood, Stoencheva, Rubia and Simonoff. This is an open-access article distributed under the terms of the Creative Commons Attribution License (CC BY). The use, distribution or reproduction in other forums is permitted, provided the original author(s) and the copyright owner(s) are credited and that the original publication in this journal is cited, in accordance with accepted academic practice. No use, distribution or reproduction is permitted which does not comply with these terms.
*Correspondence: Steve Lukito, steve.lukito@kcl.ac.uk
†These authors share first authorship
‡These authors share senior authorship