- 1Department of Psychiatry and Biobehavioral Sciences, Semel Institute for Neuroscience and Human Behavior, University of California, Los Angeles, Los Angeles, CA, California
- 2Graduate School of Professional Psychology, University of Denver, Denver, CO, United States
- 3Division of the Humanities and Social Sciences, California Institute of Technology, Pasadena, CA, United States
- 4Department of Psychology, University of California, Los Angeles, Los Angeles, CA, United States
Recent studies have demonstrated substantial phenotypic overlap, notably social impairment, between autism spectrum disorder (ASD) and schizophrenia. However, the neural mechanisms underlying the pathogenesis of social impairments across these distinct neuropsychiatric disorders has not yet been fully examined. Most neuroimaging studies to date have focused on adults with these disorders, with little known about the neural underpinnings of social impairments in younger populations. Here, we present a narrative review of the literature available through April 2020 on imaging studies of adolescents with either ASD or early-onset psychosis (EOP), to better understand the shared and unique neural mechanisms of social difficulties across diagnosis from a developmental framework. We specifically focus on functional connectivity studies of the default mode network (DMN), as the most extensively studied brain network relevant to social cognition across both groups. Our review included 29 studies of DMN connectivity in adolescents with ASD (Mean age range = 11.2–21.6 years), and 14 studies in adolescents with EOP (Mean age range = 14.2–24.3 years). Of these, 15 of 29 studies in ASD adolescents found predominant underconnectivity when examining DMN connectivity. In contrast, findings were mixed in adolescents with EOP, with five of 14 studies reporting DMN underconnectivity, and an additional six of 14 studies reporting both under- and over-connectivity of the DMN. Specifically, intra-DMN networks were more frequently underconnected in ASD, but overconnected in EOP. On the other hand, inter-DMN connectivity patterns were mixed (both under- and over-connected) for each group, especially DMN connectivity with frontal, sensorimotor, and temporoparietal regions in ASD, and with frontal, temporal, subcortical, and cerebellar regions in EOP. Finally, disrupted DMN connectivity appeared to be associated with social impairments in both groups, less so with other features distinct to each condition, such as repetitive behaviors/restricted interests in ASD and hallucinations/delusions in EOP. Further studies on demographically well-matched groups of adolescents with each of these conditions are needed to systematically explore additional contributing factors in DMN connectivity patterns such as clinical heterogeneity, pubertal development, and medication effects that would better inform treatment targets and facilitate prediction of outcomes in the context of these developmental neuropsychiatric conditions.
Introduction
Autism spectrum disorder (ASD) and schizophrenia are heterogeneous conditions that share several phenotypic and genomic features (1–4). For instance, deficits in social interaction, emotional reciprocity, pragmatic speech, and theory of mind (ToM) are postulated to be central to both disorders (5). While early detection and clinical diagnosis of both disorders has improved over the past decade, frequent challenges still arise in differential diagnosis (e.g., in the event of later diagnosis of ASD) especially if predominant symptoms for both involve social difficulties and unusual social thinking (2, 6). Recent behavioral studies of adults with ASD and schizophrenia highlighted not only the similarities but also some divergent patterns of social impairments in the two disorders—with ASD characterized by lower social motivation, poorer social reciprocity, and undermentalizing, and schizophrenia characterized by greater reciprocity but poor expressiveness (7, 8). Moreover, these social impairments are associated with difficulties in the work setting (9, 10), social relationships (11, 12), and overall reduced quality of life (13, 14) across both groups. While several studies have demonstrated genetic overlap between ASD and schizophrenia (2–4, 15), the neural mechanisms underlying the pathogenesis of the social impairments observed in these disorders are still not well understood. Given the public health significance of social disability and social isolation (16), it is crucial to explore the neurobiological mechanisms underlying social deficits across both groups, as well as to understand how they relate to real-world behaviors. Exploring the shared and distinct neural underpinnings in ASD and schizophrenia could advance our understanding of social cognitive deficits across these conditions, which will ultimately help better inform treatment. Although antipsychotics have been shown to be effective in reducing positive symptoms in schizophrenia, they are not effective in addressing the devastating social disability associated with the disorder which contributes to chronic functional impairment (17, 18). It is thus imperative to identify behavioral interventions for children and adolescents that have already shown promise in other clinical groups such as ASD. By enhancing our understanding of the neurobiological underpinnings of social impairments in ASD and how they compare to those observed in schizophrenia, we will be able to refine treatment targets and predict outcomes for each group. Hence, here we conduct a narrative review of the existing neuroimaging literature on functional connectivity of a key social brain network (default mode network; DMN) in ASD and schizophrenia, in order to elucidate the shared and unique neural mechanisms underlying social impairments across diagnosis from a developmental framework.
Adolescence or youth (ages 10–24; 19, 20) is a particularly critical window for social development and thus is an important time to investigate neural mechanisms implicated in social functioning. Adolescence is a developmental period classified by gaining independence and autonomy from caretakers (21), with marked changes in identity, self-consciousness, and cognitive flexibility (22–24). As a part of this process of developing as an independent individual, there is typically an increase in peer-directed social interactions (21, 23, 25). As a result of this increase in sociality, adolescence is a time when the social demands change most dramatically, requiring individuals with social deficits to work harder. Prior research has shown that social deficits become even more apparent during this period as social contexts increase in complexity and pose higher social expectations (24, 26). Consequences of poor social skills include peer rejection or victimization, poor friendship quality, lack of social support, experiences of loneliness, poor academic and vocational outcomes, and the development of anxiety, depression, or other psychopathologies (27–29). For individuals with ASD, adolescence may be a particularly difficult developmental period as they are also experiencing increased motivation to engage with peers, yet likely have a greater awareness of their social deficits (30). For individuals with psychotic disorder, negative symptoms including social withdrawal, reduced communication, and general apathy often precede positive symptoms and are linked more strongly to poor prognosis (31–34). The fact that social deficits often precede full-blown positive symptoms in schizophrenia implies that there are likely neural changes occurring during adolescence that precede manifestation of psychotic symptoms in early adulthood. While social impairment is a hallmark of both ASD and psychosis, these difficulties may have distinct origins: for example, the hypo-hyper-intentionality hypothesis (1, 35) postulates that individuals with ASD may under-attribute intentions to others or “undermentalize”, whereas those with schizophrenia may over-attribute intentions to others or “overmentalize”, parlaying into symptoms of suspiciousness and paranoia.
In addition to contextual changes in the social environment, adolescence is also a period marked by significant neural changes, particularly in the prefrontal cortex, a major hub in several brain networks associated with social functioning (23, 36–41). Evidence suggests that while sensory and motor brain regions are fully myelinated within the first several years of an infants’ life, neurons in the frontal cortex continue to be myelinated through adolescence (21, 23, 40). This increased myelination as well as white matter density is coupled with decreases in cortical thickness and gray matter in social brain hubs in frontal and parietal lobes (38, 40). Additionally, synaptic pruning—the process of eliminating unused neural connections, and the reorganization of strengthened pathways—is occurring actively in the prefrontal cortex during puberty (23, 25, 42–44). As a result, adolescents experience a net decrease in synaptic density during this time (23) along with increased long-distance and decreased short-distance functional connections in the brain, indexing better network integration and segregation during this period (45, 46). Increases in functional activation of prefrontal cortex are also observed in typical adolescents compared to adults in response to social tasks (25, 38). Increased functional connectivity between prefrontal cortex and temporal brain regions during adolescence is also related to increased social information processing during this age (39).
Although the social brain is not a specifically defined network, there is general consensus in the literature that the medial prefrontal regions, the temporoparietal junction, anterior and lateral temporal regions, anterior insula, and the posterior cingulate cortex/precuneus subserve several crucial social functions (25, 40, 47). Of note, the aforementioned brain regions are all highly represented within the DMN—a large-scale brain network with hubs in the medial prefrontal cortex (mPFC), posterior cingulate cortex/precuneus (PCC), inferior parietal lobe (IPL), and temporal lobe structures (48–50). The DMN is one of the most extensively studied functional networks, and it shows substantial overlap with several other “social brain” networks such as the mentalizing network and emotion recognition network (47, 51, 52). It has been proposed that the DMN is specifically involved in self-referential thinking (53–56), thoughts about self versus others and theory of mind (50, 52, 57, 58), and autobiographical memory (55, 56). Prior studies investigating DMN connectivity in health adolescents have suggested that there is a strengthening of connectivity in this network with age, particularly between anterior and posterior hubs from childhood to late adolescence, indicating increased integration in typical development (36, 37, 41, 45). Additionally, these same studies have suggested that DMN connectivity with other functional networks such as the central executive network becomes sparse from childhood to late adolescence, suggestive of increased autonomy and segregation of the DMN from task-related networks in typical development.
Disrupted DMN functional connectivity has been implicated in several psychiatric conditions with associated social difficulties (47, 59–61), including ASD (62) and schizophrenia (63). With such a rich literature, investigation of DMN function in adolescence offers a window into understanding how these social brain regions are functionally connected, how they are altered in disorders affecting social function, and their relationship to real-world social deficits. Much of this existing literature on the social brain and DMN connectivity has, however, focused on children (for ASD) and adults (for schizophrenia/psychosis), with fewer studies focusing on adolescents. While ASD may be diagnosed earlier in life, there is evidence to suggest that functional connectivity patterns in individuals with this condition undergo substantial changes from childhood to adulthood, likely influenced by factors such as puberty and/or access to treatment interventions over the years (64). In contrast, the age of onset for psychotic disorder peaks in adolescence, but more subtle cognitive and socio-emotional disturbances are present in early childhood (65). It is posited that overt symptom onset of psychosis during adolescence may be related to underlying changes in brain connectivity patterns affected by hormonal changes and increased stress response during this period (40). Due to the importance of this developmental period for brain development in general, as well as the relevant changes to social contexts, examining brain networks implicated with social cognition such as the DMN in adolescence requires substantial attention to further our understanding of the shared and distinct neural mechanisms underlying the social cognition deficits present in each group.
Hence, the current article aims to further explore cross-sectional studies on DMN connectivity in ASD and early-onset psychosis (EOP) during the adolescent years. For this purpose, we reviewed the literature available through April 2020 in PubMed, Google Scholar, and PsycINFO on DMN connectivity in adolescents with ASD and/or EOP, using search terms including “default mode network, functional connectivity,” combined with “adolescence, autism, ASD, Asperger’s” or “psychosis, adolescent-onset psychosis, adolescent-onset schizophrenia, first-episode psychosis, early-onset psychosis, early-onset schizophrenia”. The initial literature search revealed 160 relevant studies in ASD and 46 in EOP. Studies were subsequently included in the review based on age-range spanning adolescence and patient groups meeting diagnostic criteria for either ASD or EOP. All included studies were also required to have a control group of typically developing adolescents. Additionally, we focused only on empirical studies that included either: 1) static resting-state analysis or dynamic functional connectivity (DFC) analysis that examines temporal variations in connectivity patterns across the duration of the scan (66–68), and 2) provided information about the directionality of their findings. The methods used for these studies included:
1. Traditional seed-based analysis (SBA), wherein the time-series from a seed-region are correlated with all other voxels in the entire brain or a mask of the DMN (69, 70).
2. SBA that quantify the amplitude of low-frequency fluctuations (ALFF), i.e., the magnitude of signal intensity of spontaneous fluctuations for a given brain region. In ALFF analyses, the time-series from a given seed-region are transformed into a frequency domain from which the power spectrum are obtained (70, 71).
3. Independent component analyses (ICA), a data-driven method wherein whole-brain signal are decomposed to identify spatially and temporally independent components. Software templates of the DMN are then used to identify components that correspond to this network (70).
4. Support vector machines (SVM) are data-driven supervised machine-learning methods using pattern recognition algorithms to automatically classify neuroimaging data into typical or atypical categories (72).
5. Self-organizing map (SOM) algorithm, a clustering analysis technique wherein voxels are organized on a two-dimensional matrix with each node representing clusters of voxels that are highly correlated and nodes that are closer together on matrix representing neural networks (72, 73).
6. Regional Homogeneity (ReHo), a voxel-based approach to measuring brain connectivity wherein the similarity between the time-series of a given voxel and its nearest neighbors within a network is evaluated (74).
7. Granger Causality Analysis (GCA), a statistical method that allows for prediction of causality between functional connectivity of two seed-regions/nodes from time-series data (75).
8. Network Homogeneity (NH), a voxel-wise measurement of homogeneity and cohesiveness of each voxel within a functional network that provides an index of network integrity (76).
Our final review included 29 studies of DMN connectivity in adolescents with ASD (mean age range = 11.2–21.6 years; see Table 1 for demographic details), and 14 studies in adolescents with EOP (Mean age range = 14.2–24.3 years; see Table 2 for demographic details). Our goal is to synthesize the findings of altered DMN connectivity from the existing literature for each clinical population within a developmental framework and discuss how the potential commonalities or differences in underlying neural mechanisms may relate to characteristic symptomatology. We conclude by providing some insights into gaps within the extant literature and highlighting future directions for research.
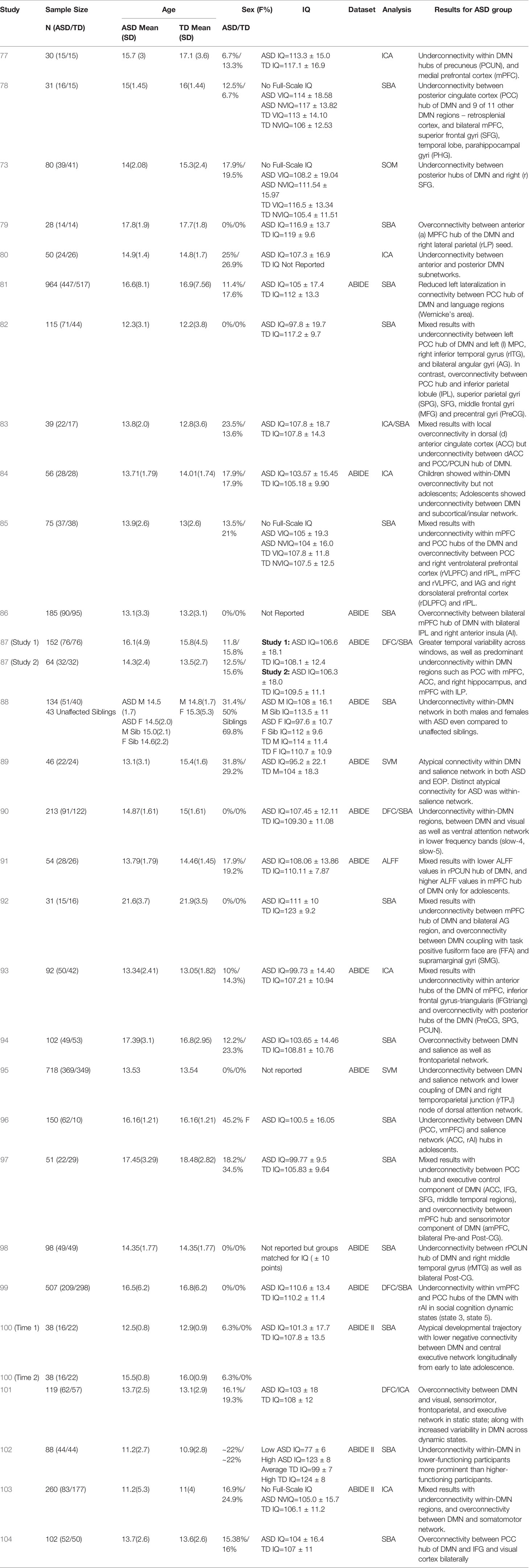
Table 1 Summary of demographic details and results for studies on DMN functional connectivity in adolescents with ASD.
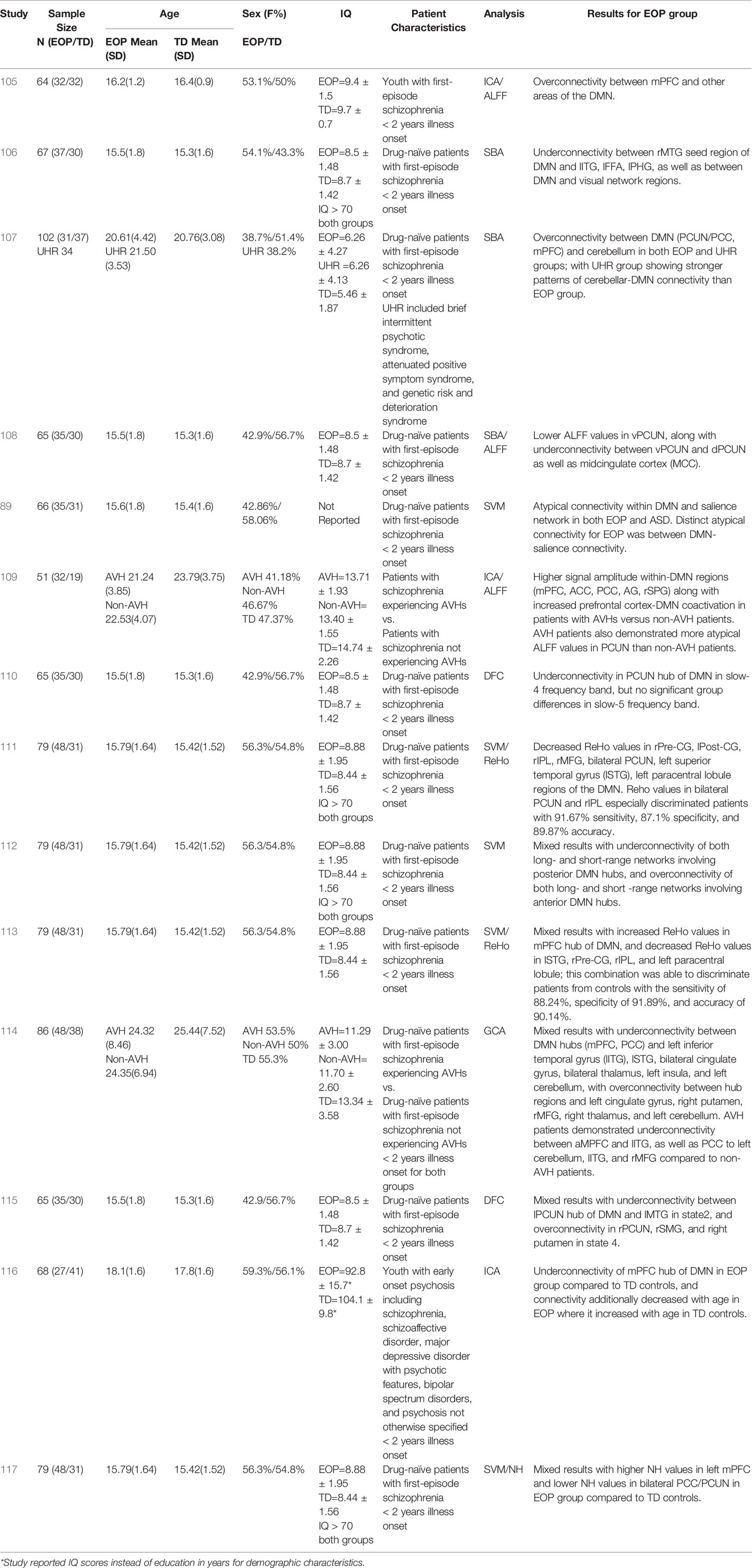
Table 2 Summary of demographic details and results for studies on DMN functional connectivity in adolescents with EOP.
DMN Connectivity in Adolescents With ASD
The past few years have witnessed a proliferation of resting-state connectivity studies in adolescents with ASD, facilitated in part by the availability of open-access multinational datasets such as the Autism Brain Imaging Data Exchange (ABIDE; 118). Approximately 52% of the studies in adolescents with ASD presented in our review (Table 1) have utilized the ABIDE dataset to investigate DMN connectivity (81, 84, 86–91, 93, 95, 98–100, 102, 103), using a combination of traditional SBA, SVM, ICA, ALFF, and DFC analytic techniques. These studies appear to be using a largely overlapping, although not exactly the same, set of ABIDE participants. About half of the studies (15 out of 29) in adolescents with ASD have found a global pattern of underconnectivity both within the DMN hubs (77, 78, 80, 87, 88, 90, 96, 98, 102), as well as between the DMN and other brain regions such as insula, subcortical regions, fronto-parietal regions, and visual cortex (73, 81, 84, 90, 95, 99), regardless of analytic methods used. Relatively fewer studies (five out of 29) have observed over-connectivity between the DMN and task-positive regions within the fronto-parietal, visual, and sensorimotor regions, as well as the salience network (79, 86, 94, 101, 104). Some studies (nine out of 29) have additionally found mixed patterns involving under- and over-connectivity of ASD youth relative to typically developing (TD) controls, largely highlighting a pattern of within-DMN underconnectivity, with overconnectivity between DMN and other networks such as task-positive or sensorimotor networks (82, 83, 85, 89, 91–93, 97, 100, 103). Of the studies using the ABIDE dataset, one reported predominant DMN overconnectivity with task-positive regions (86), while nine collectively reported DMN underconnectivity both within its hubs (87, 88, 90, 98, 102) as well as with other brain regions (81, 84, 90, 95, 99). Another five studies using the ABIDE dataset reported mixed DMN connectivity findings (89, 91, 93, 100, 103) using a range of analytic techniques (see Table 1 for main results from each study).
Additional perspectives on DMN connectivity in ASD have been offered by new and emerging studies investigating whole brain DFC. Functional connectivity of neural networks are not static (temporally nor spatially); hence, functional connectivity of a network can vary in terms of connectivity strength and directionality during different temporal “windows” or timepoints of a scan (67, 119), Additionally, DFC clustering analysis allows for the identification of recurring patterns of connectivity among networks that is consistent with those observed during tasks. This coupling of specific functional networks during various timepoints of a resting-state scan are often referred to as “states” or state-dependency” of neural activity (67, 120). While some of these studies have found broader temporal variability of DMN connectivity across states in adolescents with ASD (87, 101), others show predominant patterns of underconnectivity between the DMN and salience, attentional, and visual networks, which is state-dependent and may be related to social cognition states (90, 99). Since DFC is a relatively new realm of functional connectivity research, additional investigations of dynamic DMN connectivity as it relates to adolescents with ASD is warranted to further delineate such state-dependent patterns. In addition to static versus dynamic models, one study also examined lateralization of the DMN and its relationship to language networks in adolescents with ASD (81), and found that the ASD group had significantly less left lateralization of these networks compared to TD controls, suggesting that these language and social cognition networks may not be as functionally specialized in ASD. They additionally found that this reduced left-lateralization was associated with higher ASD symptom severity.
A few studies have also explored the maturational trajectory of DMN connectivity in individuals with ASD (73, 84, 100). Wiggins et al. (73) looked at age-related patterns of DMN connectivity cross-sectionally, and found that the ASD group did not demonstrate typical age-related increases in connectivity between the precuneus/PCC hub of the DMN and frontal regions. Nomi and Uddin (84) further looked at differences in DMN connectivity between children and adolescents with ASD cross-sectionally, and found that children with ASD showed a pattern of within-DMN overconnectivity and between-network DMN underconnectivity relative to controls. Comparatively, adolescents with ASD did not differ from age-matched controls in within-DMN connectivity but demonstrated underconnectivity between DMN and the salience network and subcortical regions. In a longitudinal study, Lawrence et al. (100) looked at changes in DMN connectivity between ASD and TD controls from early to late adolescence, and found that TD controls had an age-associated increase in negative functional connectivity between the DMN and the task-positive central executive network, not observed in adolescents with ASD. These findings support the theory of a crucial maturational shift in DMN connectivity patterns during adolescence which is likely significantly impacted in individuals with ASD such that the typically expected strengthening and honing of DMN connectivity is disrupted in this population during this age period. However, the mechanism underlying the shift in DMN connectivity patterns after the onset of puberty is not fully understood in ASD yet, and requires further exploration to elucidate differential trajectories and their impact on symptomatology.
So far, only one study has systematically examined sex differences in DMN connectivity in ASD (88). This study spanned a wide age range from childhood to adulthood, but in the adolescent subset female TD controls demonstrated stronger within-DMN connectivity relative to male TD controls; comparatively, ASD females and males showed similar within-DMN connectivity strength, that in turn was significantly lower than their TD counterparts. Notably, this DMN hypoconnectivity appeared to be an endophenotype, as it was also observed in the unaffected siblings of ASD cases, relative to TD controls. These findings suggest aberrant DMN connectivity may underlie a broader continuum of autism-relevant traits in the general population.
Intellectual functioning is another variable of interest relevant to DMN connectivity in adolescents with ASD, given the wide range of cognitive abilities in this population (121). Most of the studies included in this review focused on adolescents within the normative intellectual functioning range; however, one recent study (101) examined the differences in within-DMN connectivity between low (Mean IQ=77 ± 6) and high-IQ ASD participants (Mean IQ=123 ± 8) and found that the low cognitive functioning group demonstrated significant within-DMN underconnectivity compared to the high-functioning group, even after controlling for symptom severity.
Lastly, several of the studies (12 out of 29) included in our review have examined the relationship between aberrant DMN connectivity in adolescents with ASD and behavioral measures of symptom severity such as the Autism Diagnostic Observation Schedule (ADOS; 122, 123), the Autism Diagnostic Interview-Revised (ADI-R; 124), and the Social Responsiveness Scale (SRS; 125, 126). Studies looking at the association of within-DMN network connectivity with behavioral measures of symptom severity (N=6 studies) found mixed effects, with most (five out of six studies) reporting greater within-DMN network underconnectivity associated with higher social impairment scores on the ADOS (77, 90, 91), ADI-R (78, 103), and SRS (77); while one of the six found greater within-DMN overconnectivity to be associated with higher social impairment scores on the SRS (83), and one (using the ADOS) reporting a mixed pattern (91). Studies looking at the association of DMN between-network connectivity with behavioral measures of symptom severity (N=7) mostly found that greater overconnectivity between DMN and other brain regions (mostly in the frontal and temporal lobes; four out of seven studies) was associated with higher social impairments on the ADOS (89), ADI-R (trend level; 97), and SRS (85, 86). Interestingly, in one of the first studies to report DMN overconnectivity in adolescents with ASD, Redcay et al. (79) found that greater DMN overconnectivity with the right lateral parietal region was associated with less impairment on the social-communication domain of the ADOS, suggesting the possibility of an underlying compensatory mechanism in this particular brain network. Only two out of seven studies looking at the association of DMN between-network connectivity with behavioral measures of symptom severity found that greater underconnectivity between DMN hubs and other brain regions (salience, attention networks) in ASD was associated with higher symptom severity on the ADOS (90, 99). Additionally, Doyle-Thomas et al. (82) and Ypma et al. (88) found that anomalous DMN connectivity patterns in adolescents with ASD (mixed within-DMN connectivity results in the former study, and within-DMN underconnectivity in the latter study) were associated with poorer performance on the “Reading the Mind in the Eyes” Test (RMET; 127), a measure of affective theory of mind (ToM). Of the studies (all 12 reporting associations between DMN connectivity and behavioral measures of symptom severity) that looked at both the social interaction domain and the repetitive behaviors/restricted interests domain of the ADOS-2 and ADI-R (77–79, 83, 85, 86, 89–91, 97, 99, 103), only one study (78) reported a significant relationship for DMN within-network underconnectivity patterns and measures of repetitive behaviors/restricted interests (ADI-R) in ASD adolescents. Hence, it appears that aberrant DMN connectivity may play a larger role in the social functioning deficits experienced by this population rather than other features of ASD.
DMN Connectivity in Adolescents With EOP
Prior research on adults with schizophrenia spectrum disorders suggests that disrupted DMN connectivity may play an important role in the pathophysiology of schizophrenia (63). Specifically, findings in adults with schizophrenia frequently include within-DMN overconnectivity, as well as mixed findings of under- and over-connectivity between DMN and task-positive networks; in turn, these disruptions have been associated with positive symptoms, poor social functioning, as well as poor cognitive functioning in schizophrenia (63). Additionally, DMN connectivity has been found to become more “normative” in response to anti-psychotic treatment in adults with schizophrenia (128, 129). Some of the inconsistencies found in the literature on DMN connectivity patterns in schizophrenia, with both under- and over-connectivity involving this network being associated with symptom severity, as well as social and cognitive functioning, could be attributed to the heterogeneity of patient characteristics within schizophrenia spectrum disorders. For instance, studies thus far have included individuals with first-episode schizophrenia, chronic patients, drug-naïve patients, as well as patients treated with antipsychotic medications which may have impacted the results across these studies. Hence, how disease progression as well as treatment status impacts DMN connectivity and its relationship with behavioral outcomes in schizophrenia is not yet clear.
More recent studies of DMN connectivity in adolescents with EOP offer some insight into neural anomalies in the earlier stages of this disorder (Table 2). Several EOP studies (11 out of 14) have focused on drug-naïve adolescent patients with psychotic disorder with illness onset within two years (89, 106–108, 110–115, 117). Of these, the majority (eight out of 11) appear to report on the same (or at least, largely overlapping) cohort (106, 108, 110–113, 115, 117). Other EOP studies (three out of 14) have involved independent cohorts of adolescents with recent-onset psychotic disorder receiving anti-psychotic treatment (105, 109, 116). Collectively, results suggest a mixed pattern of under- and over-connectivity involving the DMN, similar to that observed in adults with schizophrenia (63) and regardless of analytic method or cohort used (see Table 2 for main results from each study).
One study comparing adolescents at clinical high risk for psychosis (CHR) to drug-naïve adolescents with a diagnosed psychotic disorder suggested that while both groups showed increased connectivity between DMN and cerebellum compared to TD control groups, the connectivity strength was attenuated in those with overt illness (107). On the other hand, some studies of drug-naïve adolescents with psychotic disorder have reported underconnectivity within the DMN (108, 110, 111) relative to healthy controls, and between DMN and other brain areas such as prefrontal cortex, temporal gyrus, parietal cortex, and limbic regions (106). However, six out of 14 studies investigating DMN connectivity in youth with EOP indicate a mixed pattern of connectivity, both within the DMN as well as between the DMN and other brain regions such as temporal lobe, subcortical regions, and cerebellum (89, 112–115, 117). One interesting perspective offered by Wang et al. (112) from their examination of short versus long-range DMN connectivity is that there is potentially a pattern of overconnectivity involving the anterior hubs of the DMN, compared to underconnectivity involving the posterior hubs of the DMN in drug-naïve adolescents with psychotic disorder. This perspective is further supported by recent findings of higher network homogeneity in anterior hubs of the DMN but lower in posterior hubs of the DMN in drug-naïve adolescents with psychotic disorder compared to controls (117). In the past few years, studies investigating whole-brain DFC in adolescents with EOP have also emerged (110, 115). These studies have been largely consistent with the mixed connectivity findings of the DMN for drug-naïve adolescents with a diagnosed psychotic disorder (110, 115) and suggested that over- or under-connectivity of the DMN could be state-dependent, with the precuneus hub of the DMN especially demonstrating differential state-dependent connectivity patterns with other brain regions.
Studies of youth with EOP receiving anti-psychotic medication mostly showed overconnectivity relative to healthy controls within the DMN (105, 109), as well as increased co-activation between DMN and prefrontal cognitive control regions (109) with only one study reporting underconnectivity within the DMN (116). It is therefore tempting to speculate that prior to the introduction of anti-psychotic medication the DMN tends to be underconnected or mixed in its connectivity patterns, with changes occurring in the pattern of connectivity after implementation of a medication regimen or as the course of the disease progresses.
Current symptom severity may also impact DMN connectivity patterns in adolescents with EOP. Ten out of 14 studies reviewed here examined the relationship between DMN connectivity and symptom severity on the Positive and Negative Syndrome Scale (PANSS), a widely used measure in schizophrenia (130). Out of these, four studies did not find any significant associations between DMN connectivity patterns and PANSS scores (89, 112, 115, 116). However, results from six out of 10 studies that found significant relationships between DMN connectivity and the PANSS revealed that aberrant within-DMN connectivity (105, 108, 110) as well as disrupted connectivity between DMN and other brain regions (106, 107, 114) in EOP tended to be more strongly associated with PANSS negative symptoms scores rather than positive symptom scores. Lastly, one recent study found that within-DMN underconnectivity accounted for ~16% of the variance in ToM performance measured by the RMET in adolescents with EOP treated with anti-psychotics (116). This suggests that the DMN may have a more crucial role in the social impairments observed in adolescents with EOP, rather than positive symptoms such as unusual thought content or perceptual disturbances.
Shared and Distinct DMN Connectivity Patterns in Adolescents With ASD and EOP
Figure 1 provides a schematic representation of findings from all the studies for each group, with yellow dots representing the DMN hub regions, blue (underconnected) or red (overconnected) dots representing connectivity with other brain regions, and thickness of lines connecting the dots representing frequency of findings across studies in each group. Here, we see that studies examining within-DMN connectivity (intra-DMN) found underconnectivity involving the posterior hub of the DMN or between the anterior and posterior hubs of the DMN more frequently in ASD (77, 78, 80, 82, 83, 85, 87, 88, 90, 91, 97, 102, 103), while overconnectivity involving the anterior hub or between the anterior and posterior hubs of the DMN was often found in EOP studies (105, 109, 112, 113, 117). Some studies reported intra-DMN underconnectivity in EOP involving the posterior hub of the DMN or between the medial and lateral hubs of the DMN (106, 108, 110, 117). However, it should be noted that all these studies reporting intra-DMN underconnectivity in EOP are based on the same or largely overlapping subjects. On the other hand, overconnectivity within the ASD group was most frequently seen between the anterior and lateral hubs of the DMN (79, 85, 86, 91, 97). For studies examining connectivity between DMN and other brain regions (inter-DMN), underconnectivity in ASD relative to TD controls mostly involved the posterior hub of the DMN and frontal regions as well as right anterior insula, a hub region of the salience network (73, 82, 84, 89, 93, 95–99). It should be noted that six out of 10 of these studies reporting inter-DMN underconnectivity involving the posterior hub in ASD are based on the same or largely overlapping ABIDE subjects (84, 89, 93, 95, 98, 99); as such, these cannot be considered independent findings. In contrast, inter-DMN underconnectivity for EOP relative to controls was seen most frequently between the anterior hub of the DMN and left temporal lobe (106, 111, 113–115). Overconnectivity for inter-DMN networks in the ASD group also involved the posterior hubs of the DMN, mostly with somatomotor and visual regions as well as anterior hubs of the DMN with the right anterior insula (82, 85, 86, 92–94, 97, 100, 101, 103, 104). In contrast, inter-DMN overconnectivity in EOP relative to controls was predominantly observed between DMN hubs (both anterior and posterior) and the subcortex and cerebellum (107, 114, 115). Hence, it appears that intra-DMN networks seem to be more frequently underconnected (between anterior and posterior hubs) in ASD adolescents, but mixed (i.e., underconnected for anterior hub, or between medial and lateral hubs, and overconnected for posterior hub or between anterior and posterior hubs) in EOP adolescents. On the other hand, inter-DMN connectivity patterns appear to be mixed for both groups, especially in its connectivity with frontal, sensorimotor, and temporoparietal regions in ASD, and with frontal, temporal, subcortical, and cerebellar regions in EOP.
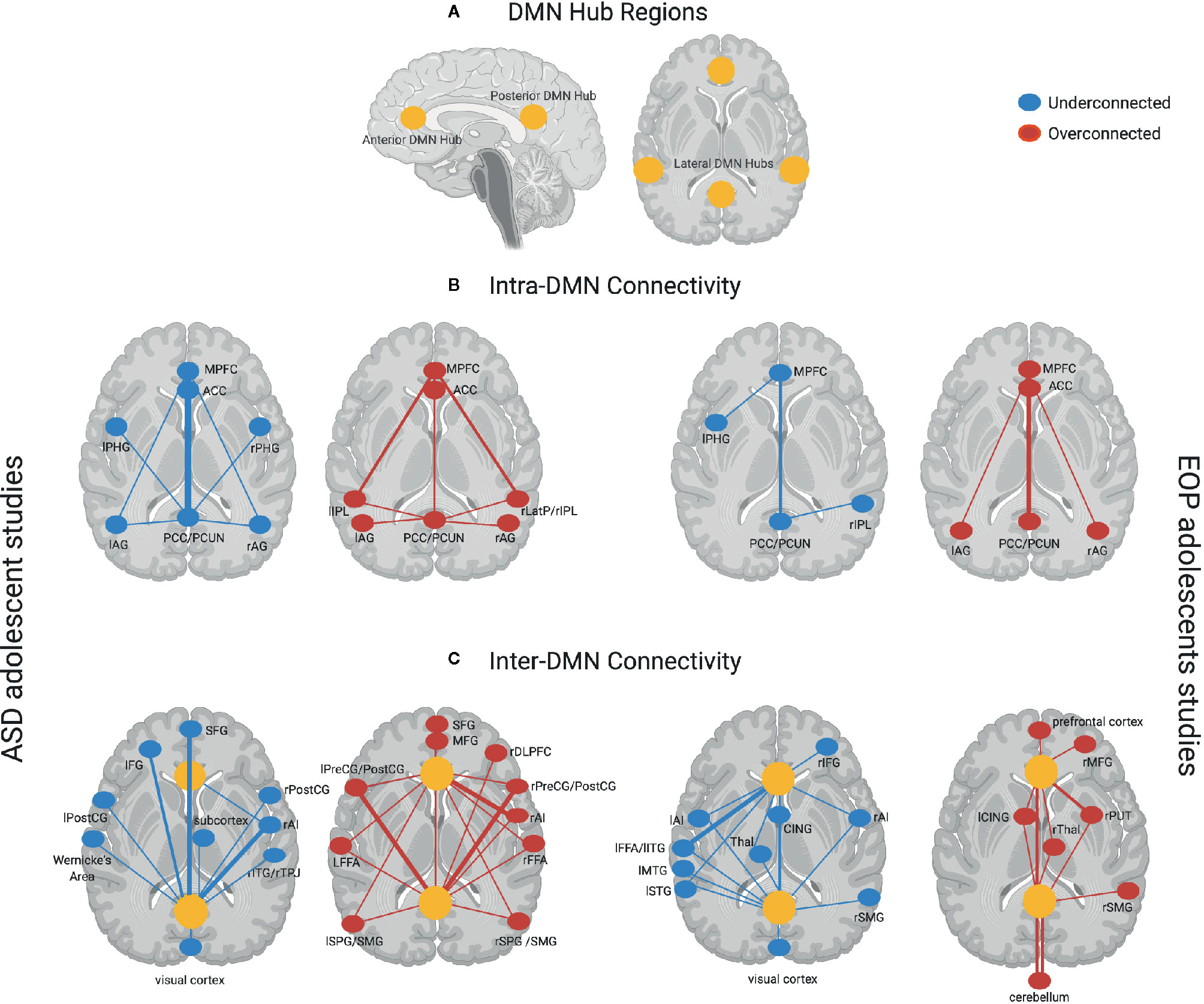
Figure 1 (A) DMN hub regions included in analyses across most studies in the present review denoted in yellow circles—anterior hubs include medial prefrontal regions (mPFC) and anterior cingulate cortex (ACC), posterior hubs include the posterior cingulate cortex (PCC) and precuneus (PCUN), lateral hubs include inferior parietal lobule (IPL), angular gyrus (AG), and medial temporal regions such as parahippocampal gyrus (PHG); (B) Intra-DMN connectivity findings across ASD adolescent studies (left panel)—Underconnectivity findings (denoted by blue dots) mostly involve the posterior hubs of the DMN. Thicker lines such as between the PCC/PCUN and the anterior hubs of the DMN (mPFC, ACC) denote overlapping findings across multiple studies, with thinner lines indicating underconnectivity within DMN regions found in fewer studies. Overconnectivity findings (denoted by red dots) for the ASD group involve the anterior hubs of the DMN slightly more prominently than the posterior hubs on the DMN. Thicker lines such as between the mPFC and left and right IPL denote overlapping findings across multiple studies, with thinner lines indicating overconnectivity within DMN regions found in fewer studies. Intra-DMN connectivity findings for EOP adolescent group are depicted in the right panel—Underconnectivity within the DMN regions (blue dots) was found by fewer studies for this group indicated by thinner lines, while overconnectivity (red dots) was mostly found within the anterior and posterior hubs of the DMN; (C) Inter-DMN connectivity findings across ASD adolescent studies (left panel)—Underconnectivity findings (denoted by blue dots) mostly involve the posterior hub (PCC/PCUN) of the DMN especially in its connectivity with prefrontal regions and the right anterior insula (rAI) hub of the salience network denoted by thicker lines with additional findings of underconnectivity between DMN and other brain regions denoted by thinner lines. Overconnectivity findings (denoted by red dots) for the ASD group also involve the posterior hubs of the DMN mostly with somatomotor regions as well as anterior hubs of the DMN with the salience network hub (rAI) denoted by thicker lines. Other regions demonstrating overconnectivity with the DMN for the ASD group are denoted by thinner lines. Inter-DMN connectivity findings for EOP adolescent group are depicted in the left panel—Underconnectivity between anterior DMN hubs and left temporal lobe was most prominently found across studies depicted by thicker lines, with additional findings of underconnectivity between both the anterior and posterior hubs of the DMN and other brain regions denoted by thinner lines. On the other hand, overconnectivity in the EOP group was predominant between DMN hubs (both anterior and posterior) and the subcortex and cerebellum highlighted by thicker lines, with additional overconnectivity between DMN hubs and other brain regions denoted by thinner lines. Additional abbreviations used in figure: PHG, parahippocampal gyrus; AG, angular gyrus; LatP, lateral parietal lobule; SFG, superior frontal gyrus; IFG, inferior frontal gyrus; PreCG, precentral gyrus; POSTCG, postcentral gyrus; ITG, inferior temporal gyrus; TPJ, temporoparietal junction; MFG, middle frontal gyrus; DLPFC, dorsolateral prefrontal gyrus; FFA, fusiform face area; SPG, superior parietal gyrus; SMG, supramarginal gyrus; CING, cingulate gyrus; Thal, thalamus; MTG, middle temporal gyrus; STG, superior temporal gyrus; PUT, putamen.
Taken together, the findings reviewed thus far highlight that ASD and EOP have both convergent, as well as divergent, patterns of dysregulation of DMN networks. Convergently, the mixed findings reported to date suggest poor functional segregation and integration of the DMN in both ASD and EOP during adolescence. The only currently published study that has directly compared whole-brain connectivity patterns in adolescents with ASD and EOP (89) found that ASD and EOP youth shared a common pattern of disrupted connectivity compared to TD controls, mainly involving the prefrontal nodes of the DMN and salience networks, which is also implicated in social functioning (131, 132). In contrast, they found that disrupted connectivity between DMN and salience network was more characteristic of EOP, whereas in ASD the atypical connections were primarily found within the salience network. Studies examining the maturational trajectory of resting state networks in ASD suggest that DMN connectivity appears to decrease from childhood to adolescence (73, 84, 100), similar to the DMN “network pruning” found in studies of typically developing adolescents (36, 37, 41, 45). However, unlike typically developing adolescents, there is a lack of both strengthening between anterior and posterior hubs of the DMN and segregation from other brain regions reported during this developmental period in the reviewed ASD studies. There were no available studies examining longitudinal trajectories of DMN connectivity in EOP adolescents to address age-associated changes or medication effects in this group. Thus, more longitudinal studies are warranted to understand DMN connectivity changes as a function of development and disease progression in both ASD and EOP groups.
Additionally, the studies reviewed here that reported brain-behavior associations have mostly used symptom severity measures (such as the ADOS, ADI-R, SRS, PANSS) rather than measures of social functioning or social cognition per se. One reason for the absence of specific social cognition measures across studies might be the use of shared datasets which provides researchers with large sample sizes, but a limited amount of common behavioral data collected across all contributing sites. Another reason could be the absence of a priori hypotheses about the association between DMN connectivity and social functioning. Only two ASD studies (82, 88) and one EOP study (116) used the RMET to measure social cognition in these clinical populations. Notably, all three studies found a significant inverse association between intra-DMN connectivity and RMET performance in both patient groups (i.e., less intra-DMN connectivity was associated with poorer task performance). This might suggest that the strengthening of connectivity between anterior and posterior DMN hubs during adolescence plays a crucial role in social functioning, and disruptions to this process in these neuropsychiatric conditions may be pertinent to social impairments. Other studies reporting brain-behavior findings additionally suggest that disrupted DMN connectivity appears to be associated with social impairments in both ASD (using the ADOS, ADI-R, or SRS) and EOP (using the PANSS), rather than other features distinct to each condition (e.g., repetitive behaviors and restricted interests in ASD vs. presence of hallucinations or delusions in EOP). Collectively, these may be considered preliminary findings for the shared role of DMN connectivity specifically underlying social functioning deficits characteristic of both ASD and EOP. However, more comprehensive assessments of social cognition abilities are needed in future studies to better elucidate the shared and distinct role of DMN connectivity disruptions in the developing brain and its relevance to social functioning.
Future Directions
In this review, we have summarized resting state functional MRI studies of DMN connectivity from empirical studies in two different clinical populations involving marked social impairment, autism spectrum disorder and early onset psychosis, during the crucial developmental window of adolescence. While the literature thus far has helped shed some light on both the common and unique patterns of DMN connectivity across these two groups, several gaps remain in our understanding of how DMN connectivity might contribute to the unique pathophysiology of both neuropsychiatric conditions. First, there have been far fewer studies on DMN connectivity in EOP than ASD adolescents. This may be due in part to difficulties in ascertaining adolescents with EOP compared to adults with psychotic disorder, given its relatively lower prevalence (133, 134). Another reason is the wider availability of large, open-access imaging datasets of adolescents with ASD such as ABIDE. Given the difficulties of collecting neuroimaging data in unique clinical populations at single sites, it is advantageous for more researchers to combine their imaging datasets using a systematic and open-source forum to allow large-scale statistical analyses cross-diagnostically. However, at present, the extent of overlap in subjects is unclear in ASD studies using ABIDE data, as well as the EOP studies that used a similar cohort of drug-naïve adolescent patients. Additionally, differences in methodologies implemented across ASD and EOP studies preclude direct comparisons of effect size for the reviewed findings. Hence, we encourage future studies on DMN connectivity in these patient populations to provide greater transparency and consistency in reporting of methods and results. Furthermore, both these conditions are characterized as spectrum disorders of varying severity, heterogeneous etiologies, and comorbidities. Since the DMN connectivity patterns observed for each of these neuropsychiatric disorders did not appear to map on well to the symptom severity measures used to assess social functioning (ADOS, ADI-R, SRS, PANSS), future studies might want to examine dimensional relationships between symptoms, social cognition performance, and their neural correlates, rather than the traditional case-control designs implemented in the majority of studies to date. Such a dimensional approach can include multiple neural features of DMN connectivity and more detailed clinical assessments of social cognition, but will require larger samples to provide meaningful findings. Finally, the impact of factors such as genetic risk, symptoms endorsed, pubertal development, and treatment history on DMN connectivity have yet to be explored within both groups. For instance, few studies have examined the contribution of medication on DMN connectivity, despite evidence that antipsychotic medication can impact brain connectivity patterns (63, 128, 135). Similarly, more work examining the impact of disease progression in EOP on DMN connectivity is needed to understand if abnormal DMN connectivity within this population remains relatively stable across the duration of illness or if further declines are associated with longer-term illness. In the ASD population, imaging studies have generally focused on high-functioning individuals, with only one study so far exploring the differences in DMN connectivity between high-and low-functioning ASD adolescents (101). It would be important to explore the influence of such contributing factors to DMN connectivity anomalies to interpret the divergent findings across studies and develop a potential mechanistic model of how genetics, neural wiring, and environmental factors may cascade into the phenotypic features we observe in these neuropsychiatric conditions.
Author Contributions
AN and CB took the lead role in reviewing papers and drafting the manuscript. MJ and SL assisted in reviewing papers and preparing tables. All authors read and approved the final manuscript. All authors contributed to the article and approved the submitted version.
Funding
This work was supported by the National Institute of Mental Health K99MH113820 (author AN).
Conflict of Interest
The authors declare that the research was conducted in the absence of any commercial or financial relationships that could be construed as a potential conflict of interest.
References
1. Crespi B, Badcock C. Psychosis and autism as diametrical disorders of the social brain. Behav Brain Sci (2008) 31(3):241–61; discussion 61-320. doi: 10.1017/S0140525X08004214
2. Rapoport J, Chavez A, Greenstein D, Addington A, Gogtay N. Autism spectrum disorders and childhood-onset schizophrenia: clinical and biological contributions to a relation revisited. J Am Acad Child Adolesc Psychiatry (2009) 48(1):10–8. doi: 10.1097/CHI.0b013e31818b1c63
3. Stone WS, Iguchi L. Do Apparent Overlaps between Schizophrenia and Autistic Spectrum Disorders Reflect Superficial Similarities or Etiological Commonalities? N Am J Med Sci (Boston) (2011) 4(3):124–33. doi: 10.7156/v4i3p124
4. Chisholm K, Lin A, Abu-Akel A, Wood SJ. The association between autism and schizophrenia spectrum disorders: A review of eight alternate models of co-occurrence. Neurosci Biobehav Rev (2015) 55:173–83. doi: 10.1016/j.neubiorev.2015.04.012
5. Chung YS, Barch D. Strube M. A meta-analysis of mentalizing impairments in adults with schizophrenia and autism spectrum disorder. Schizophr Bull (2014) 40(3):602–16. doi: 10.1093/schbul/sbt048
6. Dossetor DR. ‘All that glitters is not gold’: misdiagnosis of psychosis in pervasive developmental disorders–a case series. Clin Child Psychol Psychiatry (2007) 12(4):537–48. doi: 10.1177/1359104507078476
7. Morrison KE, Pinkham AE, Penn DL, Kelsven S, Ludwig K, Sasson NJ. Distinct profiles of social skill in adults with autism spectrum disorder and schizophrenia. Autism Res: Off J Int Soc Autism Res (2017) 10(5):878–87. doi: 10.1002/aur.1734
8. Pepper KL, Demetriou EA, Park SH, Song YC, Hickie IB, Cacciotti-Saija C, et al. Autism, early psychosis, and social anxiety disorder: understanding the role of social cognition and its relationship to disability in young adults with disorders characterized by social impairments. Transl Psychiatry (2018) 8(1):233. doi: 10.1038/s41398-018-0282-8
9. Marwaha S, Johnson S. Schizophrenia and employment - a review. Soc Psychiatry Psychiatr Epidemiol (2004) 39(5):337–49. doi: 10.1007/s00127-004-0762-4
10. Taylor JL, Henninger NA, Mailick MR. Longitudinal patterns of employment and postsecondary education for adults with autism and average-range IQ. Autism : Int J Res Practice (2015) 19(7):785–93. doi: 10.1177/1362361315585643
11. Howlin P, Goode S, Hutton J, Rutter M. Adult outcome for children with autism. J Child Psychol Psychiat Allied Discipl (2004) 45(2):212–29. doi: 10.1111/j.1469-7610.2004.00215.x
12. Horan WP, Subotnik KL, Snyder KS, Nuechterlein KH. Do recent-onset schizophrenia patients experience a “social network crisis”? Psychiatry (2006) 69(2):115–29. doi: 10.1521/psyc.2006.69.2.115
13. Eack SM, Newhill CE, Anderson CM, Rotondi AJ. Quality of life for persons living with schizophrenia: more than just symptoms. Psychiatr Rehabil J (2007) 30(3):219–22. doi: 10.2975/30.3.2007.219.222
14. Barneveld PS, Swaab H, Fagel S, van Engeland H, de Sonneville LM. Quality of life: a case-controlled long-term follow-up study, comparing young high-functioning adults with autism spectrum disorders with adults with other psychiatric disorders diagnosed in childhood. Compr Psychiatry (2014) 55(2):302–10. doi: 10.1016/j.comppsych.2013.08.001
15. O’Connell KS, McGregor NW, Lochner C, Emsley R, Warnich L. The genetic architecture of schizophrenia, bipolar disorder, obsessive-compulsive disorder and autism spectrum disorder. Mol Cell Neurosci (2018) 88:300–7. doi: 10.1016/j.mcn.2018.02.010
16. Green MF, Horan WP, Lee J, McCleery A, Mahdavi A, Reddy LF. Social Disconnection in Schizophrenia and the General Community. Schizophr Bull (2018) 44:242–9. doi: 10.1093/schbul/sbx082
17. Owen MJ, Sawa A, Mortensen PB. Schizophrenia. Lancet (2016) 388(10039):86–97. doi: 10.1016/S0140-6736(15)01121-6
18. Horan WP, Green MF. Treatment of social cognition in schizophrenia: Current status and future directions. Schizophr Res (2017) 203:3–11. doi: 10.1016/j.schres.2017.07.013
19. Sawyer SM, Azzopardi PS, Wickremarathne D, Patton GC. The age of adolescence. Lancet Child Adolesc Health (2018) 2(3):223–8. doi: 10.1016/S2352-4642(18)30022-1
20. WHO. World Health Organization. (n.d). Available from: https://www.who.int/southeastasia/health-topics/adolescent-health.
21. Casey B, Jones RM, Somerville LH. Braking and Accelerating of the Adolescent Brain. J Res Adolesc (2011) 21(1):21–33. doi: 10.1111/j.1532-7795.2010.00712.x
23. Blakemore SJ, Choudhury S. Development of the adolescent brain: implications for executive function and social cognition. J Child Psychol Psychiat Allied Discipl (2006) 47(3-4):296–312. doi: 10.1111/j.1469-7610.2006.01611.x
24. Happe F, Frith U. Annual research review: Towards a developmental neuroscience of atypical social cognition. J Child Psychol Psychiat Allied Discipl (2014) 55(6):553–7. doi: 10.1111/jcpp.12162
25. Blakemore SJ. Development of the social brain during adolescence. Q J Exp Psychol (Hove) (2008) 61(1):40–9. doi: 10.1080/17470210701508715
26. White SW, Keonig K, Scahill L. Social skills development in children with autism spectrum disorders: a review pf the intervention research. J Autism Dev Disord (2007) 10(37):1858–68. doi: 10.1007/s10803-006-0320-x
27. Bauminger N, Kasari C. Loneliness and friendship in high-functioning children with autism. Child Dev (2000) 71(2):447–56. doi: 10.1111/1467-8624.00156
28. Chamberlain B, Kasari C, Rotheram-Fuller E. Involvement or isolation? The social networks of children with autism in regular classrooms. J Autism Dev Disord (2007) 37(2):230–42. doi: 10.1007/s10803-006-0164-4
29. Rao PA, Beidel DC, Murray MJ. Social skills interventions for children with Asperger’s syndrome or high-functioning autism: a review and recommendations. J Autism Dev Disord (2008) 38(2):353–61. doi: 10.1007/s10803-007-0402-4
30. Tantam D. The challenge of adolescents and adults with Asperger syndrome. Child Adolesc Psychiatr Clin N Am (2003) 12(1):143–63. doi: 10.1016/s1056-4993(02)00053-6
31. Hooley JM. Social factors in schizophrenia. Curr Dir psychol Sci (2010) 19(4):238–42. doi: 10.1177/0963721410377597
32. Carrion RE, Demmin D, Auther AM, McLaughlin D, Olsen R, Lencz T, et al. Duration of attenuated positive and negative symptoms in individuals at clinical high risk: Associations with risk of conversion to psychosis and functional outcome. J Psychiatr Res (2016) 81:95–101. doi: 10.1016/j.jpsychires.2016.06.021
33. Addington J, Liu L, Perkins DO, Carrion RE, Keefe RS, Woods SW. The Role of Cognition and Social Functioning as Predictors in the Transition to Psychosis for Youth With Attenuated Psychotic Symptoms. Schizophr Bull (2017) 43(1):57–63. doi: 10.1093/schbul/sbw152
34. Kaneko K. Negative Symptoms and Cognitive Impairments in Schizophrenia: Two Key Symptoms Negatively Influencing Social Functioning. Yonago Acta Med (2018) 61(2):91–102. doi: 10.33160/yam.2018.06.001
35. Abu-Akel A, Bailey AL. The possibility of different forms of theory of mind impairment in psychiatric and developmental disorders. psychol Med (2000) 30(3):735–8. doi: 10.1017/s0033291799002123
36. Fair DA, Cohen AL, Dosenbach NU, Church JA, Miezin FM, Barch DM, et al. The maturing architecture of the brain’s default network. Proc Natl Acad Sci U States A (2008) 105(10):4028–32. doi: 10.1073/pnas.0800376105
37. Power JD, Fair DA, Schlaggar BL, Petersen SE. The development of human functional brain networks. Neuron (2010) 67(5):735–48. doi: 10.1016/j.neuron.2010.08.017
38. Sturman DA, Moghaddam B. The neurobiology of adolescence: changes in brain architecture, functional dynamics, and behavioral tendencies. Neurosci Biobehav Rev (2011) 35(8):1704–12. doi: 10.1016/j.neubiorev.2011.04.003
39. Klapwijk ET, Goddings AL, Burnett Heyes S, Bird G, Viner RM, Blakemore SJ. Increased functional connectivity with puberty in the mentalising network involved in social emotion processing. Horm Behav (2013) 64(2):314–22. doi: 10.1016/j.yhbeh.2013.03.012
40. Keshavan MS, Giedd J, Lau JY, Lewis DA, Paus T. Changes in the adolescent brain and the pathophysiology of psychotic disorders. Lancet Psychiatry (2014) 1(7):549–58. doi: 10.1016/S2215-0366(14)00081-9
41. Sole-Padulles C, Castro-Fornieles J, de la Serna E, Calvo R, Baeza I, Moya J, et al. Intrinsic connectivity networks from childhood to late adolescence: Effects of age and sex. Dev Cogn Neurosci (2016) 17:35–44. doi: 10.1016/j.dcn.2015.11.004
42. Bourgeois JP, Goldman-Rakic PS, Rakic P. Synaptogenesis in the prefrontal cortex of rhesus monkeys. Cereb Cortex (1994) 4(1):78–96. doi: 10.1093/cercor/4.1.78
43. Rakic P, Bourgeois JP, Goldman-Rakic PS. Synaptic development of the cerebral cortex: implications for learning, memory, and mental illness. Prog Brain Res (1994) 102:227–43. doi: 10.1016/S0079-6123(08)60543-9
44. Zecevic N, Rakic P. Development of layer I neurons in the primate cerebral cortex. J Neurosci : Off J Soc Neurosci (2001) 21(15):5607–19. doi: 10.1523/JNEUROSCI.21-15-05607.2001
45. Rubia K. Functional brain imaging across development. Eur Child Adolesc Psychiatry (2013) 22(12):719–31. doi: 10.1007/s00787-012-0291-8
46. Hulvershorn LA, Cullen KR, Francis M, Westlund M. Developmental Resting State Functional Connectivity for Clinicians. Curr Behav Neurosci Rep (2014) 1(3):161–9. doi: 10.1007/s40473-014-0020-3
47. Kennedy DP, Adolphs R. The social brain in psychiatric and neurological disorders. Trends Cogn Sci (2012) 16(11):559–72. doi: 10.1016/j.tics.2012.09.006
48. Raichle ME, MacLeod AM, Snyder AZ, Powers WJ, Gusnard DA, Shulman GL. A default mode of brain function. Proc Natl Acad Sci U States A (2001) 98(2):676–82. doi: 10.1073/pnas.98.2.676
49. Fox MD, Snyder AZ, Vincent JL, Corbetta M, Van Essen DC, Raichle ME. The human brain is intrinsically organized into dynamic, anticorrelated functional networks. Proc Natl Acad Sci U States A (2005) 102(27):9673–8. doi: 10.1073/pnas.0504136102
50. Buckner RL, Andrews-Hanna JR, Schacter DL. The brain’s default network: anatomy, function, and relevance to disease. Ann New Y Acad Sci (2008) 1124:1–38. doi: 10.1196/annals.1440.011
51. Mars RB, Neubert FX, Noonan MP, Sallet J, Toni I, Rushworth MF. On the relationship between the “default mode network” and the “social brain”. Front Hum Neurosci (2012) 6:189. doi: 10.3389/fnhum.2012.00189
52. Li W, Mai X, Liu C. The default mode network and social understanding of others: what do brain connectivity studies tell us. Front Hum Neurosci (2014) 8:74. doi: 10.3389/fnhum.2014.00074
53. Gusnard DA, Akbudak E, Shulman GL, Raichle ME. Medial prefrontal cortex and self-referential mental activity: relation to a default mode of brain function. Proc Natl Acad Sci U States A (2001a) 98(7):4259–64. doi: 10.1073/pnas.071043098
54. Gusnard DA, Raichle ME, Raichle ME. Searching for a baseline: functional imaging and the resting human brain. Nat Rev Neurosci (2001b) 2(10):685–94. doi: 10.1038/35094500
55. Andrews-Hanna JR, Reidler JS, Huang C, Buckner RL. Evidence for the default network’s role in spontaneous cognition. J Neurophysiol (2010) 104(1):322–35. doi: 10.1152/jn.00830.2009
56. Andrews-Hanna JR, Smallwood J, Spreng RN. The default network and self-generated thought: component processes, dynamic control, and clinical relevance. Ann New Y Acad Sci (2014) 1316:29–52. doi: 10.1111/nyas.12360
57. Schilbach L, Eickhoff SB, Rotarska-Jagiela A, Fink GR, Vogeley K. Minds at rest? Social cognition as the default mode of cognizing and its putative relationship to the “default system” of the brain. Conscious Cogn (2008) 17(2):457–67. doi: 10.1016/j.concog.2008.03.013
58. Lombardo MV, Chakrabarti B, Bullmore ET, Wheelwright SJ, Sadek SA, Suckling J, et al. Shared neural circuits for mentalizing about the self and others. J Cogn Neurosci (2010) 22(7):1623–35. doi: 10.1162/jocn.2009.21287
59. Menon V. Large-scale brain networks and psychopathology: a unifying triple network model. Trends Cogn Sci (2011) 15(10):483–506. doi: 10.1016/j.tics.2011.08.003
60. Whitfield-Gabrieli S, Ford JM. Default mode network activity and connectivity in psychopathology. Annu Rev Clin Psychol (2012) 8:49–76. doi: 10.1146/annurev-clinpsy-032511-143049
61. Philippi CL, Koenigs M. The neuropsychology of self-reflection in psychiatric illness. J Psychiatr Res (2014) 54:55–63. doi: 10.1016/j.jpsychires.2014.03.004
62. Padmanabhan A, Lynch CJ, Schaer M, Menon V. The Default Mode Network in Autism. Biol Psychiatry Cognit Neurosci Neuroimag (2017) 2(6):476–86. doi: 10.1016/j.bpsc.2017.04.004
63. Hu ML, Zong XF, Mann JJ, Zheng JJ, Liao YH, Li ZC, et al. A Review of the Functional and Anatomical Default Mode Network in Schizophrenia. Neurosci Bull (2017) 33(1):73–84. doi: 10.1007/s12264-016-0090-1
64. Uddin LQ, Supekar K, Menon V. Reconceptualizing functional brain connectivity in autism from a developmental perspective. Front Hum Neurosci (2013) 7:458. doi: 10.3389/fnhum.2013.00458
65. Cannon TD, van Erp TG, Bearden CE, Loewy R, Thompson P, Toga AW, et al. Early and late neurodevelopmental influences in the prodrome to schizophrenia: contributions of genes, environment, and their interactions. Schizophr Bull (2003) 29(4):653–69. doi: 10.1093/oxfordjournals.schbul.a007037
66. Chang C, Glover GH. Time-frequency dynamics of resting-state brain connectivity measured with fMRI. Neuroimage (2010) 50(1):81–98. doi: 10.1016/j.neuroimage.2009.12.011
67. Allen EA, Damaraju E, Plis SM, Erhardt EB, Eichele T, Calhoun VD. Tracking whole-brain connectivity dynamics in the resting state. Cereb Cortex (2014) 24(3):663–76. doi: 10.1093/cercor/bhs352
68. Hutchison RM, Everling S. Broad intrinsic functional connectivity boundaries of the macaque prefrontal cortex. Neuroimage (2014) 88:202–11. doi: 10.1016/j.neuroimage.2013.11.024
69. Biswal B, Yetkin FZ, Haughton VM, Hyde JS. Functional connectivity in the motor cortex of resting human brain using echo-planar MRI. Magnetic Resonance Med : Off J Soc Magnetic Resonance Med Soc Magnetic Resonance Med (1995) 34(4):537–41. doi: 10.10002/mrm.1910340409
70. Smitha KA, Akhil Raja K, Arun KM, Rajesh PG, Thomas B, Kapilamoorthy TR, et al. Resting state fMRI: A review on methods in resting state connectivity analysis and resting state networks. Neuroradiol J (2017) 30(4):305–17. doi: 10.1177/1971400917697342
71. Zang YF, He Y, Zhu CZ, Cao QJ, Sui MQ, Liang M, et al. Altered baseline brain activity in children with ADHD revealed by resting-state functional MRI. Brain Dev (2007) 29(2):83–91. doi: 10.1016/j.braindev.2006.07.002
72. Chaplot S, Patnaik LM, Jagannathan NR. Classification of magnetic resonance brain images using wavelets as input to support vector machine and neural network. Biomed Signal Process Control (2006) 1(1):86–92. doi: 10.1016/j.bspc.2006.05.002
73. Wiggins JL, Peltier SJ, Ashinoff S, Weng SJ, Carrasco M, Welsh RC, et al. Using a self-organizing map algorithm to detect age-related changes in functional connectivity during rest in autism spectrum disorders. Brain Res (2011) 1380:187–97. doi: 10.1016/j.brainres.2010.10.102
74. Zang Y, Jiang T, Lu Y, He Y, Tian L. Regional homogeneity approach to fMRI data analysis. Neuroimage (2004) 22(1):394–400. doi: 10.1016/j.neuroimage.2003.12.030
75. Seth AK, Barrett AB, Barnett L. Granger causality analysis in neuroscience and neuroimaging. J Neurosci Off J Soc Neurosci (2015) 35(8):3293–7. doi: 10.1523/JNEUROSCI.4399-14.2015
76. Uddin LQ, Kelly AM, Biswal BB, Margulies DS, Shehzad Z, Shaw D, et al. Network homogeneity reveals decreased integrity of default-mode network in ADHD. J Neurosci Methods (2008) 169(1):249–54. doi: 10.1016/j.jneumeth.2007.11.031
77. Assaf M, Jagannathan K, Calhoun VD, Miller L, Stevens MC, Sahl R, et al. Abnormal functional connectivity of default mode sub-networks in autism spectrum disorder patients. Neuroimage (2010) 53(1):247–56. doi: 10.1016/j.neuroimage.2010.05.067
78. Weng SJ, Wiggins JL, Peltier SJ, Carrasco M, Risi S, Lord C, et al. Alterations of resting state functional connectivity in the default network in adolescents with autism spectrum disorders. Brain Res (2010) 1313:202–14. doi: 10.1016/j.brainres.2009.11.057
79. Redcay E, Moran JM, Mavros PL, Tager-Flusberg H, Gabrieli JD, Whitfield-Gabrieli S. Intrinsic functional network organization in high-functioning adolescents with autism spectrum disorder. Front Hum Neurosci (2013) 7:573. doi: 10.3389/fnhum.2013.00573
80. Starck T, Nikkinen J, Rahko J, Remes J, Hurtig T, Haapsamo H, et al. Resting state fMRI reveals a default mode dissociation between retrosplenial and medial prefrontal subnetworks in ASD despite motion scrubbing. Front Hum Neurosci (2013) 7:802. doi: 10.3389/fnhum.2013.00802
81. Nielsen JA, Zielinski BA, Fletcher PT, Alexander AL, Lange N, Bigler ED, et al. Abnormal lateralization of functional connectivity between language and default mode regions in autism. Mol Autism (2014) 5(1):8. doi: 10.1186/2040-2392-5-8
82. Doyle-Thomas KA, Lee W, Foster NE, Tryfon A, Ouimet T, Hyde KL, et al. NeuroDevNet ASDIG. Atypical functional brain connectivity during rest in autism spectrum disorders. Ann Neurol (2015) 77(5):866–76. doi: 10.1002/ana.24391
83. Jann K, Hernandez LM, Beck-Pancer D, McCarron R, Smith RX, Dapretto M, et al. Altered resting perfusion and functional connectivity of default mode network in youth with autism spectrum disorder. Brain Behav (2015) 5(9):e00358. doi: 10.1002/brb3.358
84. Nomi JS, Uddin LQ. Developmental changes in large-scale network connectivity in autism. NeuroImage Clin (2015) 7:732–41. doi: 10.1016/j.nicl.2015.02.024
85. Abbott AE, Nair A, Keown CL, Datko M, Jahedi A, Fishman I, et al. Patterns of Atypical Functional Connectivity and Behavioral Links in Autism Differ Between Default, Salience, and Executive Networks. Cereb Cortex (2016) 26(10):4034–45. doi: 10.1093/cercor/bhv191
86. Elton A, Di Martino A, Hazlett HC, Gao W. Neural Connectivity Evidence for a Categorical-Dimensional Hybrid Model of Autism Spectrum Disorder. Biol Psychiatry (2016) 80(2):120–8. doi: 10.1016/j.biopsych.2015.10.020
87. Falahpour M, Thompson WK, Abbott AE, Jahedi A, Mulvey ME, Datko M, et al. Underconnected, But Not Broken? Dynamic Functional Connectivity MRI Shows Underconnectivity in Autism Is Linked to Increased Intra-Individual Variability Across Time. Brain Connectivity (2016) 6(5):403–14. doi: 10.1089/brain.2015.0389
88. Ypma RJ, Moseley RL, Holt RJ, Rughooputh N, Floris DL, Chura LR, et al. Default Mode Hypoconnectivity Underlies a Sex-Related Autism Spectrum. Biol Psychiatry Cognit Neurosci Neuroimag (2016) 1(4):364–71. doi: 10.1016/j.bpsc.2016.04.006
89. Chen H, Uddin LQ, Duan X, Zheng J, Long Z, Zhang Y, et al. Shared atypical default mode and salience network functional connectivity between autism and schizophrenia. Autism Res: Off J Int Soc Autism Res (2017) 10(11):1776–86. doi: 10.1002/aur.1834
90. Duan X, Chen H, He C, Long Z, Guo X, Zhou Y, et al. Resting-state functional under-connectivity within and between large-scale cortical networks across three low-frequency bands in adolescents with autism. Prog Neuropsychopharmacol Biol Psychiatry (2017) 79(Pt B):434–41. doi: 10.1016/j.pnpbp.2017.07.027
91. Guo X, Chen H, Long Z, Duan X, Zhang Y, Chen H. Atypical developmental trajectory of local spontaneous brain activity in autism spectrum disorder. Sci Rep (2017) 7:39822. doi: 10.1038/srep39822
92. Joshi G, Arnold Anteraper S, Patil KR, Semwal M, Goldin RL, Furtak SL, et al. Integration and Segregation of Default Mode Network Resting-State Functional Connectivity in Transition-Age Males with High-Functioning Autism Spectrum Disorder: A Proof-of-Concept Study. Brain Connectivity (2017) 7(9):558–73. doi: 10.1089/brain.2016.0483
93. Bi XA, Zhao J, Xu Q, Sun Q, Wang Z. Abnormal Functional Connectivity of Resting State Network Detection Based on Linear ICA Analysis in Autism Spectrum Disorder. Front Physiol (2018) 9:475. doi: 10.3389/fphys.2018.00475
94. Hogeveen J, Krug MK, Elliott MV, Solomon M. Insula-Retrosplenial Cortex Overconnectivity Increases Internalizing via Reduced Insight in Autism. Biol Psychiatry (2018) 84(4):287–94. doi: 10.1016/j.biopsych.2018.01.015
95. Kernbach JM, Satterthwaite TD, Bassett DS, Smallwood J, Margulies D, Krall S, et al. Shared endo-phenotypes of default mode dsfunction in attention deficit/hyperactivity disorder and autism spectrum disorder. Transl Psychiatry (2018) 8(1):133. doi: 10.1038/s41398-018-0179-6
96. Neufeld J, Kuja-Halkola R, Mevel K, Cauvet E, Fransson P, Bolte S. Alterations in resting state connectivity along the autism trait continuum: a twin study. Mol Psychiatry (2018) 23(7):1659–65. doi: 10.1038/mp.2017.160
97. Pereira AM, Campos BM, Coan AC, Pegoraro LF, de Rezende TJR, Obeso I, et al. Differences in Cortical Structure and Functional MRI Connectivity in High Functioning Autism. Front Neurol (2018) 9:539. doi: 10.3389/fneur.2018.00539
98. Borras-Ferris L, Perez-Ramirez U, Moratal D. Link-Level Functional Connectivity Neuroalterations in Autism Spectrum Disorder: A Developmental Resting-State fMRI Study. Diagn (Basel) (2019) 9(1):32. doi: 10.3390/diagnostics9010032
99. Guo X, Duan X, Suckling J, Chen H, Liao W, Cui Q, et al. Partially impaired functional connectivity states between right anterior insula and default mode network in autism spectrum disorder. Hum Brain Mapp (2019) 40(4):1264–75. doi: 10.1002/hbm.24447
100. Lawrence KE, Hernandez LM, Bookheimer SY, Dapretto M. Atypical longitudinal development of functional connectivity in adolescents with autism spectrum disorder. Autism Res : Off J Int Soc Autism Res (2019) 12(1):53–65. doi: 10.1002/aur.1971
101. Mash LE, Linke AC, Olson LA, Fishman I, Liu TT, Muller RA. Transient states of network connectivity are atypical in autism: A dynamic functional connectivity study. Hum Brain Mapp (2019) 40(8):2377–89. doi: 10.1002/hbm.24529
102. Reiter MA, Mash LE, Linke AC, Fong CH, Fishman I, Muller RA. Distinct Patterns of Atypical Functional Connectivity in Lower-Functioning Autism. Biol Psychiatry Cognit Neurosci Neuroimag (2019) 4(3):251–9. doi: 10.1016/j.bpsc.2018.08.009
103. Wang K, Xu M, Ji Y, Zhang L, Du X, Li J, et al. Altered social cognition and connectivity of default mode networks in the co-occurrence of autistic spectrum disorder and attention deficit hyperactivity disorder. Aust New Z J Psychiatry (2019a) 53(8):760–71. doi: 10.1177/0004867419836031
104. Gao Y, Linke A, Jao Keehn RJ, Punyamurthula S, Jahedi A, Gates K, et al. The language network in autism: Atypical functional connectivity with default mode and visual regions. Autism Res: Off J Int Soc Autism Res (2019) 12(9):1344–55. doi: 10.1002/aur.2171
105. Tang J, Liao Y, Song M, Gao JH, Zhou B, Tan C, et al. Aberrant default mode functional connectivity in early onset schizophrenia. PloS One (2013) 8(7):e71061. doi: 10.1371/journal.pone.0071061
106. Zhang Y, Zheng J, Fan X, Guo X, Guo W, Yang G, et al. Dysfunctional resting-state connectivities of brain regions with structural deficits in drug-naive first-episode schizophrenia adolescents. Schizophr Res (2015) 168(1-2):353–9. doi: 10.1016/j.schres.2015.07.031
107. Wang H, Guo W, Liu F, Wang G, Lyu H, Wu R, et al. Patients with first-episode, drug-naive schizophrenia and subjects at ultra-high risk of psychosis shared increased cerebellar-default mode network connectivity at rest. Sci Rep (2016) 6:26124. doi: 10.1038/srep26124
108. Zheng J, Zhang Y, Guo X, Duan X, Zhang J, Zhao J, et al. Disrupted amplitude of low-frequency fluctuations in antipsychotic-naive adolescents with early-onset schizophrenia. Psychiatry Res Neuroimag (2016) 249:20–6. doi: 10.1016/j.pscychresns.2015.11.006
109. Cui LB, Liu L, Guo F, Chen YC, Chen G, Xi M, et al. Disturbed Brain Activity in Resting-State Networks of Patients with First-Episode Schizophrenia with Auditory Verbal Hallucinations: A Cross-sectional Functional MR Imaging Study. Radiology (2017) 283(3):810–9. doi: 10.1148/radiol.2016160938
110. Wang X, Zhang Y, Long Z, Zheng J, Zhang Y, Han S, et al. Frequency-specific alteration of functional connectivity density in antipsychotic-naive adolescents with early-onset schizophrenia. J Psychiatr Res (2017a) 95:68–75. doi: 10.1016/j.jpsychires.2017.07.014
111. Liu Y, Zhang Y, Lv L, Wu R, Zhao J, Guo W. Abnormal neural activity as a potential biomarker for drug-naive first-episode adolescent-onset schizophrenia with coherence regional homogeneity and support vector machine analyses. Schizophr Res (2018) 192:408–15. doi: 10.1016/j.schres.2017.04.028
112. Wang S, Zhan Y, Zhang Y, Lyu L, Lyu H, Wang G, et al. Abnormal long- and short-range functional connectivity in adolescent-onset schizophrenia patients: A resting-state fMRI study. Prog Neuropsychopharmacol Biol Psychiatry (2018a) 81:445–51. doi: 10.1016/j.pnpbp.2017.08.012
113. Wang S, Zhang Y, Lv L, Wu R, Fan X, Zhao J, et al. Abnormal regional homogeneity as a potential imaging biomarker for adolescent-onset schizophrenia: A resting-state fMRI study and support vector machine analysis. Schizophr Res (2018b) 192:179–84. doi: 10.1016/j.schres.2017.05.038.
114. Zhao Z, Li X, Feng G, Shen Z, Li S, Xu Y, et al. Altered Effective Connectivity in the Default Network of the Brains of First-Episode, Drug-Naive Schizophrenia Patients With Auditory Verbal Hallucinations. Front Hum Neurosci (2018) 12:456. doi: 10.3389/fnhum.2018.00456
115. Wang X, Liao W, Han S, Li J, Zhang Y, Zhao J, et al. Altered dynamic global signal topography in antipsychotic-naive adolescents with early-onset schizophrenia. Schizophr Res (2019b) 208:308–16. doi: 10.1016/j.schres.2019.01.035
116. Ilzarbe D, de la Serna E, Baeza I, Rosa M, Puig O, Calvo A, et al. The relationship between performance in a theory of mind task and intrinsic functional connectivity in youth with early onset psychosis. Dev Cogn Neurosci (2019) 40:100726. doi: 10.1016/j.dcn.2019.100726
117. Zhang S, Yang G, Ou Y, Guo W, Peng Y, Hao K, et al. Abnormal default-mode network homogeneity and its correlations with neurocognitive deficits in drug-naive first-episode adolescent-onset schizophrenia. Schizophr Res (2020) 215:140–7. doi: 10.1016/j.schres.2019.10.056
118. Di Martino A, Yan CG, Li Q, Denio E, Castellanos FX, Alaerts K, et al. The autism brain imaging data exchange: towards a large-scale evaluation of the intrinsic brain architecture in autism. Mol Psychiatry (2014) 19(6):659–67. doi: 10.1038/mp.2013.78
119. Cribben I, Haraldsdottir R, Atlas LY, Wager TD, Lindquist MA. Dynamic connectivity regression: determining state-related changes in brain connectivity. Neuroimage (2012) 61(4):907–20. doi: 10.1016/j.neuroimage.2012.03.070
120. Calhoun VD, Miller R, Pearlson G, Adali T. The chronnectome: time-varying connectivity networks as the next frontier in fMRI data discovery. Neuron (2014) 84(2):262–74. doi: 10.1016/j.neuron.2014.10.015
121. DSM-V. Diagnostic and statistical manual of mental disorders. 5th ed. Washington, DC: Author: American Psychiatric Association (2013).
122. Lord C, Rutter M, DiLavore PC, Risi S. Autism Diagnotic Observation Schedule. Los Angeles, CA: Western Psychological Services (1999).
123. Lord C, Rutter M, DiLavore PC, Risi S. Autism Diagnostic Observation Schedule, Second Edition. Los Angeles, CA: Western Psychological Services (2012).
124. Rutter M, Le Couteur A, Lord C. Autism Diagnostic Interview - Revised. Los Angeles, CA: Western Psychological Services (2003).
125. Constantino JN, Gruber CP. Social Responsiveness Scale. Los Angeles, CA: Western Psychological Services (2005).
126. Constantino JN, Gruber CP. Social Responsiveness Scale. 2nd Edition. Los Angeles, CA: Western Psychological Services (2012).
127. Baron-Cohen S, Wheelwright S, Hill J, Raste Y, Plumb I. The “Reading the Mind in the Eyes” Test revised version: a study with normal adults, and adults with Asperger syndrome or high-functioning autism. J Child Psychol Psychiat Allied Discipl (2001) 42(2):241–51. doi: 10.1023/a:1015497629971
128. Sambataro F, Blasi G, Fazio L, Caforio G, Taurisano P, Romano R, et al. Treatment with olanzapine is associated with modulation of the default mode network in patients with Schizophrenia. Neuropsychopharmacol: Off Publ Am Coll Neuropsychopharmacol (2010) 35(4):904–12. doi: 10.1038/npp.2009.192
129. Surguladze SA, Chu EM, Marshall N, Evans A, Anilkumar AP, Timehin C, et al. Emotion processing in schizophrenia: fMRI study of patients treated with risperidone long-acting injections or conventional depot medication. J Psychopharmacol (2011) 25(6):722–33. doi: 10.1177/0269881110363316
130. Kay SR, Fiszbein A, Opler LA. The positive and negative syndrome scale (PANSS) for schizophrenia. Schizophr Bull (1987) 13(2):261–76. doi: 10.1093/schbul/13.2.261
131. Di Martino A, Ross K, Uddin LQ, Sklar AB, Castellanos FX, Milham MP. Functional brain correlates of social and nonsocial processes in autism spectrum disorders: an activation likelihood estimation meta-analysis. Biol Psychiatry (2009) 65(1):63–74. doi: 10.1016/j.biopsych.2008.09.022
132. Rosen ML, Sheridan MA, Sambrook KA, Dennison MJ, Jenness JL, Askren MK, et al. Salience network response to changes in emotional expressions of others is heightened during early adolescence: relevance for social functioning. Dev Sci (2018) 21(3):e12571. doi: 10.1111/desc.12571
133. Ballageer T, Malla A, Manchanda R, Takhar J, Haricharan R. Is adolescent-onset first-episode psychosis different from adult onset? J Am Acad Child Adolesc Psychiatry (2005) 44(8):782–9. doi: 10.1097/01.chi.0000164591.55942
134. Stevens JR, Prince JB, Prager LM, Stern TA. Psychotic disorders in children and adolescents: a primer on contemporary evaluation and management. Prim Care Companion CNS Disord (2014) 16(2):PCC.13f01514. doi: 10.4088/PCC.13f01514
Keywords: functional connectivity, default mode network, social cognition, autism spectrum disorder, early-onset psychosis
Citation: Nair A, Jolliffe M, Lograsso YSS and Bearden CE (2020) A Review of Default Mode Network Connectivity and Its Association With Social Cognition in Adolescents With Autism Spectrum Disorder and Early-Onset Psychosis. Front. Psychiatry 11:614. doi: 10.3389/fpsyt.2020.00614
Received: 04 April 2020; Accepted: 12 June 2020;
Published: 25 June 2020.
Edited by:
Noah James Sasson, The University of Texas at Dallas, United StatesReviewed by:
Dienke Johanna Bos, University Medical Center Utrecht, NetherlandsAhmad Abu-Akel, University of Lausanne, Switzerland
Copyright © 2020 Nair, Jolliffe, Lograsso and Bearden. This is an open-access article distributed under the terms of the Creative Commons Attribution License (CC BY). The use, distribution or reproduction in other forums is permitted, provided the original author(s) and the copyright owner(s) are credited and that the original publication in this journal is cited, in accordance with accepted academic practice. No use, distribution or reproduction is permitted which does not comply with these terms.
*Correspondence: Aarti Nair, YW5haXJAbWVkbmV0LnVjbGEuZWR1