- 1Department of Psychological Medicine, Yong Loo Lin School of Medicine, National University of Singapore, Singapore, Singapore
- 2Department of Psychology, Faculty of Arts and Social Sciences, National University of Singapore, Singapore, Singapore
- 3Institute of Health Innovation and Technology (iHealthtech), National University of Singapore, Singapore, Singapore
Introduction: Major depressive disorder (MDD) is a global psychiatric disorder with no established biomarker. There is growing evidence that functional near-infrared spectroscopy (fNIRS) has the ability to aid in the diagnosis and prediction of the treatment response of MDD. The aim of this review was to systematically review, and gather the evidence from existing studies that used fNIRS signals in the diagnosis of MDD, correlations with depression symptomatology, and the monitoring of treatment response.
Methods: PubMed, EMBASE, ScienceDirect, and Cochrane Library databases were searched for published English articles from 1980 to June 2019 that focused on the application of fNIRS for (i) differentiating depressed versus nondepressed individuals, (ii) correlating with depression symptomatology, and in turn (iii) monitoring treatment responses in depression. Studies were included if they utilized fNIRS to evaluate cerebral hemodynamic variations in patients with MDD of any age group. The quality of the evidence was assessed using the Newcastle–Ottawa quality assessment scale.
Results: A total of 64 studies were included in this review, with 12 studies being longitudinal, while the rest were cross-sectional. More than two-thirds of the studies (n = 49) had acceptable quality. fNIRS consistently demonstrated attenuated cerebral hemodynamic changes in depressed compared to healthy individuals. fNIRS signals have also shown promise in correlating with individual symptoms of depression and monitoring various treatment responses.
Conclusions: This review provides comprehensive updated evidence of the diagnostic and predictive applications of fNIRS in patients with MDD. Future studies involving larger sample sizes, standardized methodology, examination of more brain regions in an integrative approach, and longitudinal follow-ups are needed.
Introduction
Major depressive disorder (MDD) is a global mental illness which is increasingly prevalent in modern societies. As per the World Health Organization, MDD affected approximately 322 million people of all ages globally. The total number of people likely to have depression increased by 18.4% between 2005 and 2015, and this number is expected to increase exponentially over time (1). Symptoms of depression include low mood, decreased energy, poor attention, memory problems, disturbed appetite and sleep, anhedonia, feelings of guilt, and worthlessness (2). In severe cases, depression may present with psychotic symptoms, suicidal thoughts and increase the possibility of unnatural death (3). MDD can also cause significant disability. Depression is one of the leading cause of world disability in the year 2020, and in 10 years' time, it is anticipated to be the biggest cause of global disease burden overtaking cardiovascular diseases (1). Despite its gaining prevalence, MDD is still considerably undertreated and under diagnosed, especially in primary care settings (4).
Depressive disorders have been correlated with problems in the limbic, thalamic and cortical areas (5). MDD has also been correlated with neuropsychological deficiencies in numerous cognitive areas, involving attention, language, memory, and executive function (6). To diagnose patients with MDD, clinicians conventionally refer to the International Statistical Classification of Diseases and Related Health Problems—10th revision (ICD-10) or the Diagnostic and Statistical Manual of Mental Disorders, 5th Edition (DSM-5) classifications as guides to confirm the diagnosis. However, the accuracy of reaching a diagnosis of MDD relying on history taking remains debated. Diagnosis is often based on the subjective assessment and clinical experience of the clinicians. Some patients may also not be forthcoming about their symptoms, especially suicidal ideation. Furthermore, many of the psychiatric symptoms are polymorphous and may overlap in various psychiatric disorders, thereby making diagnosis all the more challenging.
MDD is a multifaceted and varied illness in which up to two thirds of patients could experience treatment resistance that protracts and worsens the episodes (7). Only approximately 33% of patients with MDD attain remission, despite being treated with optimal medications based on measurement-based care and consensus guideline. Furthermore, the probability of treatment response seems to decrease with each new treatment option (8). Treatment-resistant depression (TRD) is related to increased morbidity and mortality, with recurring and chronic periods in the long run (9). Hence, it would be useful if there are ways to ascertain improvements in treatment at any stage of the illness, as it would offer wider benefits for global management of depression.
Biomarkers offer a conceivable target for assisting in the diagnosis and identifying predictors of response to various interventions (10). Biomarkers may come in various forms, such as inflammatory markers, endogenously produced hormones and brain imaging. In recent years, brain-imaging techniques such as electroencephalography (EEG), functional magnetic resonance imaging (fMRI), positron emission tomography (PET), and magnetoencephalography (MEG) existed to be used as adjuncts to help clinicians diagnose MDD. According to some neuroimaging findings using PET and fMRI to investigate brain function in patients with depression (11–13), they found blood flow reduction in the prefrontal cortex to be associated with a decline in activity within the cingulate cortex. There had been reports using neuroimaging techniques recording hemodynamic response relating to brain activity during cognitive stimulation on depressed patients. One such study using fMRI performed by Okada et al. revealed decreased left prefrontal activation and reduced task performance in depressed patients utilizing the verbal fluency task (VFT) (11). However, these tests are expensive to conduct. Additionally, the patients were required to place themselves in an awkward posture, i.e., lying supine in a narrow space with the head fixed during the investigation (14).
In 2009, functional near-infrared spectroscopy (fNIRS) was sanctioned in Japan. It was classified to be an advanced medical technology for differentiation of psychiatric illness (15). Additionally, in 2013, fNIRS obtained medical insurance coverage for being an adjunct diagnostic tool. fNIRS examinations have since been utilized for psychophysiological assessment of cognitive function.
fNIRS is a form of spectroscopy that utilizes light sources between a spectral window of 650 to 1000 nm which penetrates organic tissues. Oxygenated hemoglobin (oxy-Hb) variations are then computed using the variance in absorbance using the modified Beer–Lambert law. This is a noninvasive technique that can detect cortical oxygenation levels of hemoglobin (16), and low cortical oxygenation levels have been correlated with depressive illness. The advantages of using fNIRS are that it is a relatively inexpensive procedure, portable, and easy to set up, and it does not involve nonionizing radiation. Hence, it may be repeated multiple times on an as-needed and when-needed basis for patients. In fact, fNIRS has been applied in many other areas of the medical field, such as cognition and preoperative functional assessment (17). With regard to psychiatric illness, in addition to MDD, there are studies pertaining to its use for patients with schizophrenia and bipolar disorder among other disorders (18) (Table 1). Many fNIRS studies involve tasks that help activate brain activity in the subject, such as VFT or passively viewing photographs to trigger an emotional response.
To date, most of the studies on the diagnostic and predictive applications of fNIRS for MDD have been conducted in Japan. Furthermore, most studies have been conducted with a relatively small number of participants. To date, there is only one meta-analysis, published in 2015, looking at fNIRS for differentiating patients with depression from healthy subjects (23). Since then, more studies have been conducted on the matter as the use of fNIRS on depressed individuals to help in diagnosis and monitoring treatment response has gained acceptance over the last few years. Through a meta-analysis, Zhang et al. found that MDD patients had considerably decreased prefrontal cortex activation when undertaking cognitive tasks relative to controls. Patients with MDD, as opposed to controls, were associated with reduced rise in oxy-Hb in prefrontal regions during cognitive stimulation. This distinctive pattern of blood oxygen variations in the prefrontal cortex in MDD patients may be used as an objective diagnostic instrument for MDD. However, with such a low quantity of studies in the first meta-analysis, we decided to evaluate the use of fNIRS as a diagnostic biomarker for the diagnosis of MDD and its distinction between different stages of the illness, and to discuss its usefulness as a monitoring biomarker for treatment response in MDD patients, as based on a systematic review of the latest available literature. This paper aims to cover (i) the use of fNIRS to differentiate depressed from healthy individuals, (ii) correlation of fNIRS signals with depression symptomatology, and (iii) how it can be applied to monitor treatment response.
Methods
Data Sources and Search Strategy
This study was conducted on the basis of the Preferred Reporting Items for Systematic Reviews and Meta-Analysis (PRISMA). A systematic review was completed with published English-language literature from 1980 to June 2019, focusing on the utility of fNIRS for (i) differentiating depressed versus nondepressed individuals, (ii) correlating with depression symptomatology, and (iii) monitoring treatment responses in depression. The four electronic databases searched were PubMed, EMBASE, ScienceDirect, and Cochrane Library. The search terms used were “Spectroscopy” or “Near-infrared” or “near-infrared spectroscopy” or “fNIRS” or “optical topography” and “Depression” or “depressed” or “depressive disorder” or “mood disorder” or “affective disorder.” Terms were searched as both text words and subject headings. The team scanned for related publications, conference proceedings and bibliographies of papers gathered manually. This systematic review was funded by the National University of Singapore iHeathtech Other Operating Expenses (R-722-000-004-731).
Eligibility Criteria and Data Collection
Studies were short-listed if the authors utilized fNIRS to measure cerebral hemodynamic variations in patients with MDD of any age group. All titles and abstracts retrieved from the databases were independently reviewed by two reviewers (LL and NC). Where appropriate, full-text papers were extracted for further inspection. Studies which were selected by either reviewer and fulfilled the inclusion criteria proceeded on to full-text review, whereby the study characteristics and results were extracted. Any discrepancies in the study selection were brought to the attention of the third reviewer (CH) and resolved by discussion. Studies were divided into the three categories that were most appropriate for the three questions set out in the review. The data review form consisted of the subsequent data: authors and publication year, country, sample characteristics, diagnostic criteria used, type of NIRS device, paradigm used, and main findings of the study. As much data as possible were obtained from the articles, and efforts were made to contact the authors if supplementary data were needed.
Quality Assessment of the Articles
The articles included in the full-text review were evaluated by utilizing the Newcastle–Ottawa quality assessment scale (NOS) (24), with the subsequent generation of a table with star scores for each study. There is no overall score that determines whether a study is “good” or “bad,” but a star is awarded for meeting each criterion involving selection and outcome, with the exception of compatibility where two stars can be awarded. Two reviewers (LL and NC) independently assessed the papers, and discrepancies were brought to the third reviewer (CH) to resolve by discussion.
Results
Study Selection
A total of 4868 citations were identified from our database search, with 342 from PubMed, 1798 from EMBASE, 1625 from ScienceDirect, and 1103 from Cochrane Library. After reviewing the titles, abstracts, and removing duplicated publications, 128 articles were selected. Of these, 64 studies met the inclusion criteria and were encompassed in this analysis. The selection process is displayed in Figure 1, constructed according to the PRISMA statement. Forty-seven studies were from Japan, eight studies from China, six studies from Germany, one study from the UK, one study from the USA, and one study from Uzbekistan. These studies were further divided into categories based on which of the three questions they addressed: (i) differentiation of depressed from healthy individuals, (ii) correlation with depression symptomatology, and (iii) assessment of treatment response. Fifty-one studies addressed the first question, 31 studies addressed the second question, and 16 studies addressed the third question. Twelve studies were longitudinal, while the rest were cross-sectional studies. Seven studies were extracted from conference proceedings, while the remaining were full-text articles.
Use of fNIRS to Differentiate Depressed From Healthy Individuals
We identified 51 papers that used fNIRS to distinguish depressed patients from healthy controls (HCs) (Table 2). The bulk of the studies were conducted in Japan (n = 38), followed by China (n = 7), Germany (n = 4), and the United Kingdom (n = 1). The pooled sample across the studies comprised a total of 2094 unipolar depressed patients and 2457 HCs. All the depressed patients in the respective studies fulfilled the ICD-10 or DSM-IV diagnostic criteria for MDD, with the exception of two studies that used the DSM-5 and one study that used the DSM-III as the diagnostic criteria instrument. The psychopathology measure used for the studies was mostly the Hamilton Depression Rating Scale (HAMD). With the exception of six studies that did not include an indication of the medications that were consumed by the depressed patients, three studies that included medication-naive patients and two studies that included antidepressant-naive patients, the depressed patients generally received antidepressants of different types and dosages with or without other types of medication. The majority of studies utilized the VFT as the active paradigm, while others adopted the verbal repetition task, hyperventilation and paper-bag breathing, visuospatial task, Stroop task, Sternberg's task, Tower of Hanoi, CO2 inhalation task, working memory (WM) task, word generation tasks (WGT), emotional Stroop task, facial emotion recognition task, image-recall task, mirror drawing task (MDT), trail-making test (TMT), or a combination of tasks [e.g., word fluency task (WFT) with right-finger-tapping task]. The fNIRS instruments that were used and the number of channels utilized, with a large proportion using the 52-channel ETG-4000, varied across studies. Most of the studies measured the concentration of oxy-Hb and deoxygenated hemoglobin (deoxy-Hb) during various tasks for distinguishing the depressed from the healthy. The probe is normally positioned at the frontal (usually prefrontal) and/or temporal areas, with the exception of two papers that measured the parietal area as well and one paper that measured only the left and right hemispheres in general. For the papers that included the assessment of the parietal brain areas, one of them included subjects suffering from Alzheimer's disease, and the parietal dysfunctions were linked to the initial phase of dementia (30). Other studies such as Rosenbaum et al. (72) examined the frontoparietal networks of patients with early- and late-life depression (LLD), which is associated with cognitive control. The study discovered that the frontoparietal networks appeared to be essential in LLD as patients suffering from LLD and memory impairment are shown to be at elevated risk for having dementia (75).
The overall results showed that the depressed patients showed a smaller oxy-Hb increase than the controls, with a smaller increase in the frontal (especially the prefrontal) and temporal activation (appreciably lower activation in the depressed compared to HCs) while performing the WM task, VFT, WGT, and TMT. Kito et al. (30) measured both the frontal and parietal brain areas, and the results showed decreased cortical activation throughout the VFT in patients with depression and the HCs. With the same brain areas being measured, the depressed patients and HCs showed noteworthy dissimilarities in functional connectivity (FC) both in the resting state and during TMT performance, with depressed patients showing a decrease, and HCs showing a surge, in FC from the resting state to TMT performance (72). When subjects were asked to hyperventilate, there was a significant decrease in oxy-Hb levels, and deoxy-Hb was considerably elevated in the depressed and HCs, with the depressed patients showing a lesser reduction in oxy-Hb than healthy individuals (26, 31). During paper-bag breathing, Matsuo et al. (31) revealed that oxy-Hb significantly increased in both groups, whereas deoxy-Hb significantly decreased in the depressed juxtaposed to the healthy. Matsuo et al. (26) established no noteworthy alterations in oxy-Hb and deoxy-Hb in depressed patients or in HCs. Using the emotional Stroop task, Matsubara et al. found that the HCs compared to the depressed patients showed notably increased oxy-Hb during the happy-word trials, but the decreases in oxy-Hb during the threat-word trials were comparable in both depressed patients and HCs (32). However, Matsubara et al. and Nishizawa et al. concluded that the depressed when comparing with HCs showed notable increased in oxy-Hb within the left middle frontal region while performing the threat task, whereas depressed patients compared to HCs showed no noteworthy variation in oxy-Hb while performing the happy task (42, 60). For the CO2 inhalation task, the vasomotor reactivity was significantly decreased in the depressed patients than controls (35). When subjects were performing right-finger-tapping tasks, the increase in oxy-Hb was higher in the depressed patients when comparing with HCs (18). In the image-recall task, the change in oxy-Hb in the HCs was considerably more than in the depressed while experiencing unpleasant conditions (53).
Almost all of the studies did not indicate the specificity and sensitivity of differentiating between depressed patients and HCs, with the exception of one that indicated an 80% sensitivity but with its specificity being unspecified (29). One study had indicated a sensitivity of 71.5% and a specificity of 70% for differentiating depressed patients from those with Alzheimer's disease (30), and another study mentioned a sensitivity of 0.71 and a specificity of 0.46 to distinguish between the euthymic, unipolar and bipolar depressive patients (37). Last, there was one study that indicated different sensitivities and specificities, which were dependent on the integral values of the two regions of interest (region 1 consisted of frontopolar and dorsolateral prefrontal cortical regions, while region 2 consisted of the middle and superior temporal cortical regions and the ventrolateral prefrontal cortex) (39).
Correlation of fNIRS Signals With Depression Symptomatology
We identified 31 eligible papers reporting on fNIRS studies in which cerebral hemodynamic changes were correlated with depression symptomatology in a total of 1424 patients (Table 3). These studies were generally in those with MDD, but some were inclusive of patients with other mental health illness, for example: bipolar disorder, affective disorder, and schizophrenia. The majority of the studies adopted the VFT, except for 12 studies that utilized paradigms such as WFT, RPS task, image-recall task, resting state measurements, TMT, stop-signal task, WM task, MDT, conversation task, emotional Stroop task, and the use of self-report measures such as the rumination response scale (RRS) and visual analog scales (VAS). The NIRS instruments utilized markedly differed from study to study.
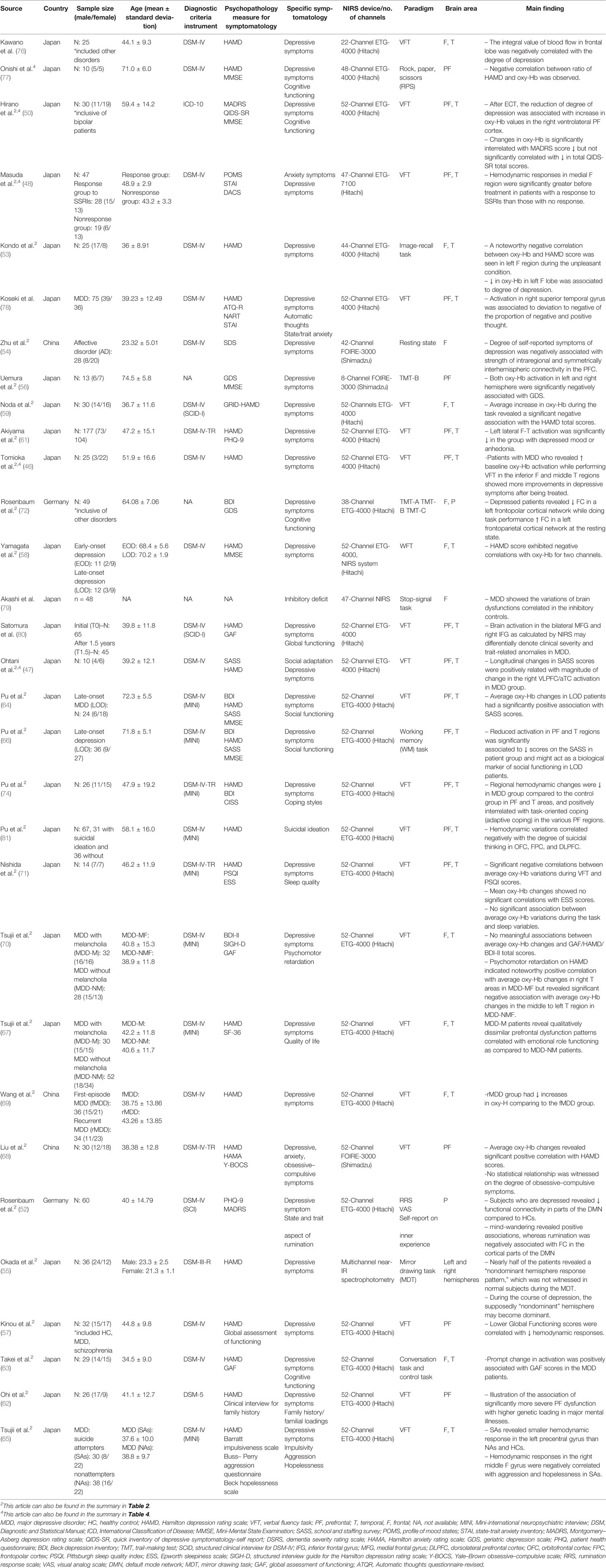
Table 3 Summary of fNIRS studies correlating cerebral hemodynamic changes with depression symptomatology.
According to our assessment of the authors' conclusions, almost every study discovered that oxy-Hb concentration values were negatively correlated with the degree of total depressive symptoms [HAMD, geriatric depression scale (GDS), Montgomery–Asberg depression rating scale (MADRS), quick inventory of depressive symptomatology-self rated (QIDS-SR), etc.] and Uemura et al. even demonstrated that such a significant negative correlation was still present after correcting for confounding factors such as gender, age, and educational history (56). Such a negative correlation was further supported by Wang et al. who illustrated that the recurrent MDD group had notably diminished increases in oxy-Hb compared to first-episode MDD patients (69). Additionally, Nishizawa et al. utilized the emotional Stroop task to evaluate brain regions associated with the severity of depression. They found that hyperactivated oxy-Hb was seen in the left frontal cortex upon contact to adverse stimuli, but no meaningful differences were established between the depressed compared with the healthy upon exposure to favorable stimuli (60). Additionally, the degree of depression was contrariwise associated with an evoked wave in the left upper frontal cortex following exposure to favorable stimuli. Akiyama et al. also found that there was significant decrease in left lateral frontotemporal activation in active depressed patients as compared to those who still had residual depressive symptoms despite MDD remission (61), further suggesting the possibility of NIRS's ability to differentiate depression of varying severity.
While such a noteworthy negative correlation was established in many papers, there were some studies that observed no significant correlations (70) and even a significant positive association (68) between HAMD scores and oxy-Hb concentrations. Such results could have been due to methodological limitations that were faced by these studies. For example, in the study by Tsujii et al. (70), the sample size was small. Thus, such slight difference in the hemodynamic response in the left temporal region among the two groups was not statistically different. A type II error might be a possibility if the size of the sample was enlarged, and the dissimilarity between the healthy and depressed in the left channels might be meaningful. Additionally, Liu et al. (68) argued that such inconsistency in results could have been due to the dynamic process of psychiatric disease development; therefore, there are some findings that may be momentary markers and not persistent markers.
Apart from the general negative association between the oxy-Hb concentration values and the degree of total depressive symptoms, many of the studies looked into a variety of individual depressive symptoms, such as suicidal thoughts, sleep quality, psychomotor retardation, obsessive–compulsive symptoms, cognitive functioning [assessed by the Mini-Mental State Examination (MMSE), Consortium to Establish a Registry for Alzheimer's Disease (CERAD) test battery, etc.], and many others.
Suicidality is often observed in MDD patients. Suicidal ideation usually precedes a suicide attempt. Pu et al. investigated individuals prior to any attempt (73), while Tsujii et al. explored responses after an attempt (65). Pu et al. (73) found that the hemodynamic variations in the OFC, FPC, and DLPFC negatively correlated to the degree of suicidal thinking in depressed individuals; Tsujii et al. (65) revealed that the suicide attempters (SAs) revealed a reduced hemodynamic response in the left precentral gyrus compared to the nonattempters (NAs) and HCs, and the hemodynamic responses in the right middle frontal gyrus were negatively correlated with aggression and hopelessness in the SAs but not in the HCs and NAs.
Nishida et al. examined the relationship between sleep quality and oxy-Hb concentrations (71). They detected a negative relationship between average oxy-Hb concentration variations while performing the task and Pittsburgh sleep quality index (PSQI) scores. This illustrated that self-rated sleep disturbances were related to a reduced left prefrontal reactivity when performing VFT in MDD patients, thus indicating that the reactivity of the prefrontal region was vulnerable to sleep disorders.
Psychomotor retardation is another classic symptom of MDD. In Tsujii et al., psychomotor retardation on the HAMD showed noteworthy affirmative association with average oxy-Hb changes in the right temporal region in the MDD patients with melancholic features (MDD-MF) but displayed a suggestive negative association with average oxy-Hb changes in the middle to left temporal region in the MDD patients with non-melancholic features (MDD-NMF) (70). These findings were consistent with earlier functional neuroimaging studies that determined that MDD with psychomotor retardation is related to reduced blood flow in patients with MDD-NMF, unlike those suffering from MDD-MF. Thus, this suggested that the pathophysiology of non-melancholic MDD is distinctive from melancholia. Specifically, the pathophysiology of melancholia could be linked to the right temporal region. MDD patients often showed impaired inhibitory control. In the study by Akashi et al. fluctuations in oxy-Hb concentrations in MDD patients in the superior frontal probes were associated with impairments in performance in inhibitory controls (79).
With regard to patients' functioning, while no statistical significance was established with the degree of obsessive–compulsive symptoms (68), average oxy-Hb concentrations were significantly positively interrelated with the global assessment of functioning (GAF) scores (57, 63) and SASS scores (47, 64, 66). For SASS in particular, observational changes in bilateral ventrolateral prefrontal cortex activity and the anterior portion of the temporal cortex (VLPFC/aTC) were associated with gains in social adaptability in MDD patients, although the MDD groups revealed less activation than the HCs in the VLPFC/aTC. Additionally, when Tsujii et al. assessed quality of life (QOL) using the Medical Outcomes Study 36-item short-form health survey (SF-36), hemodynamic responses in the prefrontal region were positively associated with the role emotional domain scores for the MDD-MF patients. Comparatively, MDD-NMF patients showed no noteworthy correlations (67). This indicated that while lower functioning was associated with lower oxy-Hb concentrations, patients with MDD-MF had qualitatively distinctive prefrontal impairment features associated with emotional role functioning unlike patients with MDD-NMF.
Pu et al. assessed coping styles with the coping inventory for stressful situations (CISS) and discovered that MDD patients were more possibly using an emotion-oriented coping style as compared to using task-orientated and avoidance-orientated coping. Emotion-oriented coping style was also positively associated with subjective assessments of the degree of depression (74). Task-orientated coping was positive correlated with regional hemodynamic changes in various prefrontal regions. The findings suggested that the hemodynamic responses in the various prefrontal regions when performing VFT imply patients who suffer from MDD utilize task-orientated coping when depressed.
Apart from the use of oxy-Hb concentration measures, three papers also assessed FC (52, 54, 72). First, in patients with affective disorders, Zhu et al. (54) showed altered patterns of FC, representing far greater disorganized patterns of correlation maps, by outlining and examining four FC types: the intraregional connectivity (SC-I), the intrahemispheric connectivity (SC-II), the symmetrically interhemispheric connectivity (LC-I), and the asymmetrical interhemispheric connectivity (LC-II). This showed that patients suffering from affective disorders indicated considerably weaker intraregional connectivity and symmetrical interhemispheric connectivity in the IFG as compared to HCs and that such disruptions in the right IFG are correlated with problems in emotional reactions and reducing negative thinking in affective disorder patients. Second, similar to previous results found by many others, Rosenbaum et al. (72) also found that the subjects who were depressed revealed increased FC in a left frontoparietal cortical network at the resting state and decreased FC in a left frontopolar cortical network while doing a performance task. However, when these relations were calculated on behalf of the separate diagnostic groups, these correlations were congruent only in the non-depressed group and varied in the depressed group, thus implying that these results might be more related to depression status than to the degree of symptoms per se. In comparison to controls, the depressed individuals indicated an exceedingly connected network with a left hemispheric focus during the resting-state condition, and this result was inferred to be related to rumination. This relationship was further examined by Rosenbaum et al. (52). Though ruminative thoughts were negatively associated with FC in the cortical areas of the default mode network (DMN), mind-wandering displayed positive correlations with FC.
Moreover, Okada et al. illustrated hemodynamic changes using brain response patterns (55). The response patterns were cerebral responses to the MDT. During the MDT, the overall response areas were analyzed by using a planimeter as the areas demarcated by the total Hb response curves and the baseline. The area deviation ratio was calculated by dividing the difference between the blood volume response areas of the right and left hemispheres by the hemisphere blood volume response area showing a lower response. When the ratios were higher (range 4–44), response patterns were classified as dominant hemispheric response patterns in normal subjects or non-dominant hemispheric response patterns in MDD patients. They demonstrated that about 50% of patients (12 out of 24 males and 4 out of 12 females) showed a “non-dominant hemisphere response pattern,” which was not seen in healthy subjects, and this “non-dominant” hemisphere could become dominant during depression. Furthermore, significant negative associations were found between the oxy-Hb and the age of onset in six channels but there were no noteworthy associations between age and oxy-Hb at the period of assessment.
Use of fNIRS to Monitor Treatment Response
All 16 studies included in Table 4 were case–control studies. Eleven studies were performed in Japan, one study in China, one study in Uzbekistan, one study in United Kingdom, one study in the United States, and one study in Germany. The combined sample throughout the 16 studies comprised of 282 MDD patients. Every patient met the MDD criteria of DSM-IV, DSM-5 or ICD-10. The majority of the studies examined the correlation of cerebral hemodynamic changes with improvements in the depressive symptoms of patients, while five studies (47, 48, 50, 77, 81) looked at either social and/or cognitive functioning of the patients as the treatment outcome.
Almost all patients responded favorably to treatment, as the articles reported significant improvement in social functioning or a decrease in depressive symptoms. However, the patients in the study by Takamiya et al. showed no meaningful differences in depressive scores following treatment in both the high- and low-dose groups (84). In group comparisons, there were significant outcomes on NIRS signals for high doses of antidepressants compared with low doses. This highlighted that the dose-dependent influence of antidepressants on the NIRS signal ought to be considered while understanding NIRS data. The most commonly used paradigm to stimulate cognitive activity was the VFT. One study (77) used a rock, paper, scissors (RPS) task. Two studies (87, 89) used mental arithmetic tasks, and another study (88) utilized a lateral visual field simulation. The prefrontal brain regions were examined in all of these studies.
Measuring NIRS signals before starting treatment has been shown to predict treatment progress in medication-naive patients suffering from MDD (46). In the paper by Tomioka et al. (46), the average oxy-Hb concentration values in the prefrontal and temporal regions during the VFT were significantly correlated with improvements in HAMD scores after treatment with antidepressants. The group comparisons revealed that the mean oxy-Hb concentration variations in the temporal and prefrontal cortices while performing VFT were considerably reduced in the depression group compared to HC group. This result concurred among other studies (18) that explored the uses of NIRS for the treatment of MDD.
Similar to the study by Tomioka et al. (46), Yamagata et al. also conducted a study on the application of NIRS in medication-naive participants with MDD (82). The latter study highlighted the noninvasive nature of NIRS as they conducted repeated measurements at short intervals (three follow-ups at 4-week intervals). According to their findings, they found a substantial adverse association between mean oxy-Hb concentration values in the significant cluster (at week 4) and differences in HAMD scores from weeks 4 to 8 (r = −.73) and from weeks 8 to 12 (r = −.63). As such, they also concluded that NIRS could be used in predicting MDD patients' response to antidepressant treatment.
Ohtani et al. looked at social adaptation in their study, and in their 6-month follow-up, they found that NIRS signals could be a predictor for the longitudinal assessment of social adaptation (47). Longitudinal increases in temporal and prefrontal regions were shown to be correlated with improvements in social adaptation for patients, although there was no significant change in the severity of their clinical symptoms.
On the other hand, Pu et al. investigated patients' social functioning in their study (81). The pretreatment NIRS activation in the prefrontal region was found to be positively associated with pretreatment social adaptation self-evaluation scale (SASS) scores and negatively correlated with increase in SASS scores after 8 weeks. Their discoveries suggested that NIRS signals would be useful in evaluating social functioning in patients afflicted by late-onset depression (prior to treatment) and predicting the improvement in their social functioning after treatment. Furthermore, fNIRS can be used to predict the treatment response of not only antidepressants but also other novel treatments, such as neurostimulation (50, 85, 89), music therapy (49), and animal-assisted therapy (83).
Quality Assessment of the Included Studies
The NOS was used to assess the risk of bias in the included studies, and the results are shown in Table 5. No study had a complete score of eight stars. More than two-thirds of the studies had acceptable quality, of which 49 studies were awarded four or more stars, with 11 studies given seven stars, 14 studies given six stars, 19 studies given five stars, and 5 studies given four stars. Fifteen studies fared more poorly in quality with less than four stars, of which 3 studies were given three stars, 11 studies were given two stars, and 1 study was given one star.
Discussion
Currently, there is no specific test or biomarker for diagnosing and monitoring the progression of depression. MRI studies have shown reduced size in the medial and lateral prefrontal cortex, amygdala, hippocampus, and striatum; increased functional activity in the medial prefrontal cortex, amygdala, and hippocampus; and decreased activity in the lateral prefrontal cortex and striatum in depressed subjects compared with HCs (90). Nevertheless, the applicability and utility of MRI in clinical practice is limited due to the high cost, need to stay immobile, presence of noise while scanning, long scanning duration, and risk of inducing claustrophobia. These considerations may be especially relevant for depressed patients who may be emotionally sensitive and thus find it harder to tolerate being confined to a tight space with recurrent noise for a prolonged period. Compared to fMRI, fNIRS is less expensive, fast to operate, quiet, and more portable and, thus, able to perform the scan in the clinic or ward directly and allows body movement in a naturalistic environment (91). However, some of its inherent limitations may influence the accuracy and eventual applicability in clinical practice. For instance, its spatial resolution and penetration depth are limited, which reduces access to the subcortical regions that have a pertinent role in psychiatric disorders. As fNIRS is established on the principles of neurovascular coupling, it is affected by blood pressure, hemoglobin level, blood circulation, vasculature, and carbon dioxide concentration (92, 93). Nevertheless, a meticulous study design, enhanced fNIRS techniques, and statistical processing could potentially mitigate these shortfalls.
This is the first systematic review on the application of fNIRS to depression that intended to provide an overview of the up-to-date information of 3 pertinent clinical questions: (i) the usefulness of fNIRS in differentiating depressed from healthy individuals, (ii) the correlation of fNIRS signals with depression symptomatology, and in turn (iii) monitoring treatment response. Answers to these questions are essential in ascertaining the applicability and utility of fNIRS as a tool to assist the diagnosing of depression, predicting clinical symptoms of patients, monitoring the treatment response and disease progression, and for prognosticating the disease. Based on the combined findings of the reviewed papers, most of the studies were conducted in Japan, although there has been an increasing trend for studies being conducted in China, Germany and other countries. The number of fNIRS studies has also exponentially increased year on year, suggesting an increased recognition of this technology in mainstream science. The most commonly used active paradigm is the VFT. This is not surprising as the VFT is quite a common bedside neuropsychological test that has been extensively utilized to ascertain executive function and language content (94) and is easy to perform within a short period, though it is imperative to realize that the performance on the VFT can be influenced by culture. Participants are obliged to state as many unique words that begin with a particular letter or are from a semantic category in a restricted time (up to a minute usually). Cognitive dysfunction occurs in patients who are depressed, of which impairments in executive ability is one of the core domains, in addition to learning/memory, attention/concentration, and processing speed (95). As a validated test to ascertain executive function, the VFT is able to elicit distinct differences in performance and neuroimaging responses between depressed and healthy people (23).
Based on the findings of this systematic review, the fNIRS signals show promise as an ideal biomarker in addressing our review's three fundamental questions: do fNIRS signals differentiate depressed from nondepressed individuals, correlate with specific depressive symptomatology, and in turn aid the monitoring of treatment responses (regardless of whether the treatment is a medication or another treatment modality)? The findings of our systematic review demonstrated a consistent pattern of attenuated pre-frontal stimulation in patients with depression while conducting cognitive tasks using the NIRS method, and cerebro-hemodynamic changes are associated with particular symptoms encountered by patients and treatment responses. However, most studies did not include sensitivity and specificity data that compared depressed patients from HCs, which would be important to elucidate the validity of fNIRS as a diagnostic tool. Thus, future studies may need to consider standardizing their analysis such that comparisons across studies can be made. The correlation of brain signals with clinical symptoms such as suicidal ideation, sleep quality, and psychomotor retardation may facilitate more in-depth personalized profiling of the individual's depression and may be able to better identify risk issues in management (e.g., self-harm, suicide, neglect). Symptom identification can be especially helpful when patients are not forthcoming about their symptoms, which makes it difficult to ascertain their risks. However, the majority of the selected studies had small sample populations, thereby reducing the power of the study, and there were variations in study methodologies, including the device and paradigm used, which limited the ability to effectively combine or compare results. One important aspect of fNIRS as a biomarker would be its potential to prognosticate the disease, i.e., whether the patient will improve or worsen with time. Nevertheless, the existing studies were either cross-sectional or had short longitudinal follow-ups, which made it difficult to address this issue. Longer longitudinal studies of at least 6 months to a year would be beneficial, considering that most depressive episodes last for at least a few months. Furthermore, longitudinal studies can help to elucidate whether the hemodynamic responses recorded by NIRS are a state- or trait-dependent marker of depression, as the current evidence is conflicting and confounded by several reasons.
Most studies scanned their subjects 2 weeks to 1 month after starting antidepressants, and then scans were performed monthly. Having more frequent fNIRS measurements in longitudinal studies (e.g., weekly) can provide us with a better understanding of brain dynamics and minimize the influence of confounding factors. The majority of studies to date have examined only the temporal and frontal regions. It may thus be worthwhile to expand the assessment to other brain regions, such as the occipital and parietal regions, especially because these two regions have also been implicated in depression (96, 97). Furthermore, most studies examined hemodynamic changes in discrete brain regions, with only a few studies investigating FC aberrations and even fewer ascertaining effective connectivity across brain regions (97). Connectivity studies allow a more holistic approach to how various brain areas interactively function and may represent another means of examining the disease state. Most of the studies involved patients on medication, and those with medication-naive patients had small sample sizes. Thus, we are unable to exclude the possibility that antidepressants interfered with the signal results. Studies have highlighted that antidepressants can affect the NIRS signals (84). Therefore, it may be worthwhile to have more studies involving scans of medication-naive patients, though this can be practically challenging at the ground level.
An encouraging application is combining different neuroimaging modalities to allow a more accurate and comprehensive surveillance of the neurophysiological changes. This can include combining fNIRS with EEG, fMRI, PET, or diffusion tensor imaging (DTI) to complement fNIRS. This can in turn improve the spatial and temporal resolution and thereby increase sensitivity and specificity. Nevertheless, the applicability of combining modalities such as fMRI and PET is limited due to the immobile and bulky apparatus, and although EEG is portable, use of EEG increases the setup time, and the increased noise-signal ratio with artifacts may reduce its accuracy. On the other hand, integrative approaches that combine various modalities of biomarkers such as genetic, neuroimaging, and neuropsychological data that are analyzed using high-dimensional multivariate statistical methods are gaining prominence and may more comprehensively facilitate disease diagnostics and prediction (98).
Our review has a number of limitations. First, we selected observational studies that had small sample sizes, thereby increasing the risk of selective and confounding biases and reduced power due to small effect sizes. However, based on our search, there were no randomized controlled trials of patients with depression using fNIRS. Second, we only included studies in English and searched in four databases. We recognize that there are an increasing number of fNIRS studies conducted in China and other countries that may not be published in English and indexed in these four databases. Thus, this potentially led to publication bias. Third, we also included conference proceedings, and several details of these datasets were unavailable from the source. However, it was essential to include them to reflect the spectrum of studies being performed in the current fNIRS research landscape. Regardless, this review has strengths in being the first to combine three pertinent questions pertaining to fNIRS as a biomarker, and there has not been an updated systematic review of fNIRS in depression since 2015. This study is a timely update of the current landscape of using fNIRS on depressed patients, given the increasing availability of this technology worldwide.
In conclusion, there is a good amount of evidence in the current literature to suggest fNIRS as a diagnostic and predictive tool for MDD, and it has shown consistent hemodynamic patterns in depressed patients compared to healthy individuals. There is also an increasing amount of studies on fNIRS in depression, indicating the increased recognition of this technique in the study of depression. Future studies involving larger sample sizes, standardized methodology, examination of more brain regions in an integrative approach, and longitudinal follow-ups are needed to advance the use of fNIRS in psychiatric clinical practice and research.
Author Contributions
Conceptualization: CH. Data extraction and review: LL, AL, NC, RT, SL. Writing: CH. Review and manuscript amendment: LL, AL, NC, RT, SL, RH.
Conflict of Interest
The authors declare that the research was conducted in the absence of any commercial or financial relationships that could be construed as a potential conflict of interest.
References
1. World Health Organization. (2017). Depression and Other Common Mental Disorders: Global Health Estimates. World Health Organization [cited 2019 Oct 13]; Available from: https://apps.who.int/iris/bitstream/handle/10665/254610/WHO-MSD-MER-2017.2-eng.pdf?sequence=1.
2. Lim GY, Tam WW, Lu Y, Ho CS, Zhang MW, Ho RC. Prevalence of Depression in the Community from 30 Countries between 1994 and 2014. Sci Rep (2018) 128(1):2861. doi: 10.1038/s41598-018-21243-x
3. Choo CC, Harris KM, Ho RC. Prediction of Lethality in Suicide Attempts: Gender Matters. Omega. (2019) 80(1):87–103. doi: 10.1177/0030222817725182
4. Sheehan DV. Depression: underdiagnosed, undertreated, underappreciated. Manag Care Langhorne Pa (2004) 13(6 Suppl Depression):6–8.
5. Mayberg HS. Modulating dysfunctional limbic-cortical circuits in depression: towards development of brain-based algorithms for diagnosis and optimised treatment. Br Med Bull (2003) 65:193–207. doi: 10.1093/bmb/65.1.193
6. Ottowitz WE, Dougherty DD, Savage CR. The neural network basis for abnormalities of attention and executive function in major depressive disorder: implications for application of the medical disease model to psychiatric disorders. Harv Rev Psychiatry (2002) 10(2):86–99. doi: 10.1080/10673220216210
7. Fava M. Diagnosis and definition of treatment-resistant depression. Biol Psychiatry (2003) 53(8):649–59. doi: 10.1016/S0006-3223(03)00231-2
8. Gaynes BN, Warden D, Trivedi MH, Wisniewski SR, Fava M, Rush AJ. What did STAR*D teach us? Results from a large-scale, practical, clinical trial for patients with depression. Psychiatr Serv Wash DC. (2009) 60(11):1439–45. doi: 10.1176/ps.2009.60.11.1439
9. Olchanski N, McInnis Myers M, Halseth M, Cyr PL, Bockstedt L, Goss TF, et al. The economic burden of treatment-resistant depression. Clin Ther (2013) 35(4):512–22. doi: 10.1016/j.clinthera.2012.09.001
10. Strawbridge R, Young AH, Cleare AJ. Biomarkers for depression: recent insights, current challenges and future prospects. Neuropsychiatr Dis Treat (2017) 13:1245–62. doi: 10.2147/NDT.S114542
11. Okada G, Okamoto Y, Yamashita H, Ueda K, Takami H, Yamawaki S. Attenuated prefrontal activation during a verbal fluency task in remitted major depression. Psychiatry Clin Neurosci (2009) 63(3):423–5. doi: 10.1111/j.1440-1819.2009.01952.x
12. Meyer JH, Houle S, Sagrati S, Carella A, Hussey DF, Ginovart N, et al. Brain serotonin transporter binding potential measured with carbon 11-labeled DASB positron emission tomography: effects of major depressive episodes and severity of dysfunctional attitudes. Arch Gen Psychiatry (2004) 61(12):1271–9. doi: 10.1001/archpsyc.61.12.1271
13. Drevets WC. Neuroimaging studies of mood disorders. Biol Psychiatry (2000) 48(8):813–29. doi: 10.1016/S0006-3223(00)01020-9
14. Lai CYY, Ho CSH, Lim CR, Ho RCM. Functional near-infrared spectroscopy in psychiatry. BJPsych Adv (2017) 23(5):324–30. doi: 10.1192/apt.bp.115.015610
15. Fukuda M. Near-infrared spectroscopy in psychiatry. Brain Nerve Shinkei Kenkyu No Shinpo. (2012) 64(2):175–83.
16. Scholkmann F, Kleiser S, Metz AJ, Zimmermann R, Mata Pavia J, Wolf U, et al. A review on continuous wave functional near-infrared spectroscopy and imaging instrumentation and methodology. NeuroImage (2014) 85 (Pt 1):6–27. doi: 10.1016/j.neuroimage.2013.05.004
17. Boas DA, Elwell CE, Ferrari M, Taga G. Twenty years of functional near-infrared spectroscopy: introduction for the special issue. NeuroImage (2014) 85 Pt 1:1–5. doi: 10.1016/j.neuroimage.2013.11.033
18. Suto T, Fukuda M, Ito M, Uehara T, Mikuni M. Multichannel near-infrared spectroscopy in depression and schizophrenia: cognitive brain activation study. Biol Psychiatry (2004) 55(5):501–11. doi: 10.1016/j.biopsych.2003.09.008
19. Noda T, Nakagome K, Setoyama S, Matsushima E. Working memory and prefrontal/temporal hemodynamic responses during post-task period in patients with schizophrenia: A multi-channel near-infrared spectroscopy study. J Psychiatr Res (2017) 95:288–98. doi: 10.1016/j.jpsychires.2017.09.001
20. Hirose T, Tsujii N, Mikawa W, Shirakawa O. Delayed hemodynamic responses associated with a history of suicide attempts in bipolar disorder: a multichannel near-infrared spectroscopy study. Psychiatry Res Neuroimaging. (2018) 280:15–21. doi: 10.1016/j.pscychresns.2018.08.003
21. Katzorke A, Zeller JBM, Müller LD, Lauer M, Polak T, Deckert J, et al. Decreased hemodynamic response in inferior frontotemporal regions in elderly with mild cognitive impairment. Psychiatry Res Neuroimaging. (2018) 274:11–8. doi: 10.1016/j.pscychresns.2018.02.003
22. Ueda S, Ota T, Iida J, Yamamuro K, Yoshino H, Kishimoto N, et al. Reduced prefrontal hemodynamic response in adult attention-deficit hyperactivity disorder as measured by near-infrared spectroscopy. Psychiatry Clin Neurosci (2018) 72(6):380–90. doi: 10.1111/pcn.12643
23. Zhang H, Dong W, Dang W, Quan W, Tian J, Chen R, et al. Near-infrared spectroscopy for examination of prefrontal activation during cognitive tasks in patients with major depressive disorder: a meta-analysis of observational studies. Psychiatry Clin Neurosci (2015) 69(1):22–33. doi: 10.1111/pcn.12209
24. Wells GA, Shea B, O'Connell D, Peterson J, Welch V, Losos M. (2000). The Newcastle– Ottawa Scale (NOS) for assessing the quality of nonrandomized studies in meta-analyses. [Internet]. [cited 2019 Oct 13]. Available from: http://www.ohri.ca/programs/clinical_epidemiology/oxford.asp.
25. Pu S, Yamada T, Yokoyama K, Matsumura H, Kobayashi H, Sasaki N, et al. A multi-channel near-infrared spectroscopy study of prefrontal cortex activation during working memory task in major depressive disorder. Neurosci Res (2011) 70(1):91–7. doi: 10.1016/j.neures.2011.01.001
26. Matsuo K, Kato T, Fukuda M, Kato N. Alteration of Hemoglobin Oxygenation in the Frontal Region in Elderly Depressed Patients as Measured by Near-infrared Spectroscopy. J Neuropsychiatry Clin Neurosci (2000) 12(4):465–71. doi: 10.1176/jnp.12.4.465
27. Herrmann MJ, Ehlis A-C, Fallgatter AJ. Bilaterally Reduced Frontal Activation During a Verbal Fluency Task in Depressed Patients as Measured by Near-Infrared Spectroscopy. J Neuropsychiatry Clin Neurosci (2004) 16(2):170–5. doi: 10.1176/jnp.16.2.170
28. Shoji Y, Morita K, Yanagimoto H, Fujiki R, Ishii Y, Uchimura N. Characteristics of the single event related [Oxy-Hb] changes in patients with depressive disorder.
29. Kinoshita S, Kanazawa T, Kikuyama H, Yoneda H. Clinical application of DEX/CRH test and multi-channel NIRS in patients with depression. Behav Brain Funct BBF. (2016) 12(1):25. doi: 10.1186/s12993-016-0108-x
30. Kito H, Ryokawa A, Kinoshita Y, Sasayama D, Sugiyama N, Ogihara T, et al. Comparison of alterations in cerebral hemoglobin oxygenation in late life depression and Alzheimer's disease as assessed by near-infrared spectroscopy. Behav Brain Funct BBF (2014) 10:8. doi: 10.1186/1744-9081-10-8
31. Matsuo K, Kato N, Kato T. Decreased cerebral haemodynamic response to cognitive and physiological tasks in mood disorders as shown by near-infrared spectroscopy. Psychol Med (2002) 32(6):1029–37. doi: 10.1017/S0033291702005974
32. Matsubara T, Matsuo K, Harada K, Nakashima M, Nakano M, Hirotsu M, et al. Different Fronto-Temporal Activation During an Emotional Word Task in Patients with Unipolar and Bipolar Depression: A Functional Near-Infrared Spectroscopy Study (2015). Biol Psychiatry (2015) 77:1S–444S.
33. Koike S, Sakakibara E, Satomura Y, Sakurada H, Yamagishi M, Matsuoka J, et al. Differentiation between Schizophrenia, Bipolar Disorder, and Major Depression Using the Prefrontal Brain Activity and the Evaluation of Ultra-high Risk for Psychosis: A Large-Sample Functional Near-infrared Spectroscopy Study. Early Interv Psychiatry (2018) 12(Suppl. 1):104–232. doi: 10.1111/eip.12724
34. Azechi M, Iwase M, Ishii R, Ikezawa K, Canuet L, Kurimoto R, et al. P27-5 Frontal lobe dysfunction and regional hemodynamic changes in major depression: A near infrared spectroscopy study. Clin Neurophysiol (2010) 121:S264. doi: 10.1016/S1388-2457(10)61079-6
35. Matsuo K, Onodera Y, Hamamoto T, Muraki K, Kato N, Kato T. Hypofrontality and microvascular dysregulation in remitted late-onset depression assessed by functional near-infrared spectroscopy. NeuroImage. (2005) 26(1):234–42. doi: 10.1016/j.neuroimage.2005.01.024
36. Ohta H, Yamagata B, Tomioka H, Takahashi T, Yano M, Nakagome K, et al. Hypofrontality in panic disorder and major depressive disorder assessed by multi-channel near-infrared spectroscopy. Depress Anxiety (2008) 25(12):1053–9. doi: 10.1002/da.20463
37. Shimodera S, Imai Y, Kamimura N, Morokuma I, Fujita H, Inoue S, et al. Near-infrared spectroscopy of bipolar disorder may be distinct from that of unipolar depression and of healthy controls: NIRS of bipolar vs unipolar disorder. Asia-Pac Psychiatry (2012) 4(4):258–65. doi: 10.1111/j.1758-5872.2012.00218.x
38. Ma X-Y, Wang Y-J, Xu B, Feng K, Sun G-X, Zhang X-Q, et al. Near-Infrared Spectroscopy Reveals Abnormal Hemodynamics in the Left Dorsolateral Prefrontal Cortex of Menopausal Depression Patients. Dis Markers (2017) 2017:1695930. doi: 10.1155/2017/1695930
39. Takizawa R, Fukuda M, Kawasaki S, Kasai K, Mimura M, Pu S, et al. Neuroimaging-aided differential diagnosis of the depressive state. NeuroImage (2014) 85 (Pt 1):498–507. doi: 10.1016/j.neuroimage.2013.05.126
40. Liske BCJ, Ackermann PH, Münch D, Florian H, Ehlis A-C, Stevens A, et al. New electrophysiological findings in the detection of malingering attention deficits in subjects with an episode of depression compared to healthy subjects. Clin Neurophysiology (2015) 126:e63–e170. doi: 10.1016/j.clinph.2015.04.178
41. Zhu Y, Quan W, Wang H, Ma Y, Yan J, Zhang H, et al. Prefrontal activation during a working memory task differs between patients with unipolar and bipolar depression: A preliminary exploratory study. J Affect Disord (2018) 225:64–70. doi: 10.1016/j.jad.2017.07.031
42. Matsubara T, Matsuo K, Nakashima M, Nakano M, Harada K, Watanuki T, et al. Prefrontal activation in response to emotional words in patients with bipolar disorder and major depressive disorder. NeuroImage (2014) 85 (Pt 1):489–97. doi: 10.1016/j.neuroimage.2013.04.098
43. Akashi H, Tsujii N, Mikawa W, Adachi T, Kirime E, Shirakawa O. Prefrontal cortex activation is associated with a discrepancy between self- and observer-rated depression severities of major depressive disorder: a multichannel near-infrared spectroscopy study. J Affect Disord (2015) 174:165–72. doi: 10.1016/j.jad.2014.11.020
44. Gao L, Cai Y, Wang H, Wang G, Zhang Q, Yan X. Probing prefrontal cortex hemodynamic alterations during facial emotion recognition for major depression disorder through functional near-infrared spectroscopy. J Neural Eng. (2019) 16(2):026026. doi: 10.1088/1741-2552/ab0093
45. Schecklmann M, Dresler T, Beck S, Jay JT, Febres R, Haeusler J, et al. Reduced prefrontal oxygenation during object and spatial visual working memory in unpolar and bipolar depression. Psychiatry Res (2011) 194(3):378–84. doi: 10.1016/j.pscychresns.2011.01.016
46. Tomioka H, Yamagata B, Kawasaki S, Pu S, Iwanami A, Hirano J, et al. A Longitudinal Functional Neuroimaging Study in Medication-Naïve Depression after Antidepressant Treatment. PloS One (2015) 10(3):e0120828. doi: 10.1371/journal.pone.0120828
47. Ohtani T, Nishimura Y, Takahashi K, Ikeda-Sugita R, Okada N, Okazaki Y. Association between longitudinal changes in prefrontal hemodynamic responses and social adaptation in patients with bipolar disorder and major depressive disorder. J Affect Disord (2015) 176:78–86. doi: 10.1016/j.jad.2015.01.042
48. Masuda K, Nakanishi M, Okamoto K, Kawashima C, Oshita H, Inoue A, et al. Different functioning of prefrontal cortex predicts treatment response after a selective serotonin reuptake inhibitor treatment in patients with major depression. J Affect Disord (2017) 214:44–52. doi: 10.1016/j.jad.2017.02.034
49. Feng K, Shen C-Y, Ma X-Y, Chen G-F, Zhang M-L, Xu B, et al. Effects of music therapy on major depressive disorder: A study of prefrontal hemodynamic functions using fNIRS. Psychiatry Res (2019) 275:86–93. doi: 10.1016/j.psychres.2019.03.015
50. Hirano J, Takamiya A, Yamagata B, Hotta S, Miyasaka Y, Pu S, et al. Frontal and temporal cortical functional recovery after electroconvulsive therapy for depression: A longitudinal functional near-infrared spectroscopy study. J Psychiatr Res (2017) 91:26–35. doi: 10.1016/j.jpsychires.2017.02.018
51. Downey D, Sabrina B, Liam T, , Rebecca E, Elwell C, Richard M-W, et al. Prefrontal cortex haemodynamic responses in severe major depression and the effects of ECT: an fNIRS study. Eur Neuropsychopharmacol (2016), S304.
52. Rosenbaum D, Haipt A, Fuhr K, Haeussinger FB, Metzger FG, Nuerk H-C, et al. Aberrant functional connectivity in depression as an index of state and trait rumination. Sci Rep (2017) 7(1):2174. doi: 10.1038/s41598-017-02277-z
53. Kondo A, Shoji Y, Morita K, Sato M, Ishii Y, Yanagimoto H, et al. Characteristics of oxygenated hemoglobin concentration change during pleasant and unpleasant image-recall tasks in patients with depression: Comparison with healthy subjects. Psychiatry Clin Neurosci (2018) 72(8):611–22. doi: 10.1111/pcn.12684
54. Zhu H, Xu J, Li J, Peng H, Cai T, Li X, et al. Decreased functional connectivity and disrupted neural network in the prefrontal cortex of affective disorders: A resting-state fNIRS study. J Affect Disord (2017) 221:132–44. doi: 10.1016/j.jad.2017.06.024
55. Okada F, Takahashi N, Tokumitsu Y. Dominance of the “nondominant” hemisphere in depression. J Affect Disord (1996) 37(1):13–21. doi: 10.1016/0165-0327(95)00040-2
56. Uemura K, Shimada H, Doi T, Makizako H, Park H, Suzuki T. Depressive symptoms in older adults are associated with decreased cerebral oxygenation of the prefrontal cortex during a trail-making test. Arch Gerontol Geriatr. (2014) 59(2):422–8. doi: 10.1016/j.archger.2014.07.003
57. Kinou M, Takizawa R, Marumo K, Kawasaki S, Kawakubo Y, Fukuda M, et al. Differential spatiotemporal characteristics of the prefrontal hemodynamic response and their association with functional impairment in schizophrenia and major depression. Schizophr Res (2013) 150(2–3):459–67. doi: 10.1016/j.schres.2013.08.026
58. Yamagata B, Tomioka H, Takahashi T, Isomura AJ, Kobayashi H, Mimura M. Differentiating early and late-onset depression with multichannel near-infrared spectroscopy. Psychogeriatrics. (2008) 8(2):79–87. doi: 10.1111/j.1479-8301.2008.00232.x
59. Noda T, Yoshida S, Matsuda T, Okamoto N, Sakamoto K, Koseki S, et al. Frontal and right temporal activations correlate negatively with depression severity during verbal fluency task: a multi-channel near-infrared spectroscopy study. J Psychiatr Res (2012) 46(7):905–12. doi: 10.1016/j.jpsychires.2012.04.001
60. Nishizawa Y, Kanazawa T, Kawabata Y, Matsubara T, Maruyama S, Kawano M, et al. fNIRS Assessment during an Emotional Stroop Task among Patients with Depression: Replication and Extension. Psychiatry Investig (2019) 16(1):80–6. doi: 10.30773/pi.2018.11.12.2
61. Akiyama T, Koeda M, Okubo Y, Kimura M. Hypofunction of left dorsolateral prefrontal cortex in depression during verbal fluency task: A multi-channel near-infrared spectroscopy study. J Affect Disord (2018) 231:83–90. doi: 10.1016/j.jad.2018.01.010
62. Ohi K, Shimada T, Kihara H, Yasuyama T, Sawai K, Matsuda Y, et al. Impact of Familial Loading on Prefrontal Activation in Major Psychiatric Disorders: A Near-Infrared Spectroscopy (NIRS) Study. Sci Rep (2017) 7:44268. doi: 10.1038/srep44268
63. Takei Y, Suda M, Aoyama Y, Sakurai N, Tagawa M, Motegi T, et al. Near-infrared spectroscopic study of frontopolar activation during face-to-face conversation in major depressive disorder and bipolar disorder. J Psychiatr Res (2014) 57:74–83. doi: 10.1016/j.jpsychires.2014.06.009
64. Pu S, Matsumura H, Yamada T, Ikezawa S, Mitani H, Adachi A, et al. Reduced frontopolar activation during verbal fluency task associated with poor social functioning in late-onset major depression: Multi-channel near-infrared spectroscopy study. Psychiatry Clin Neurosci (2008) 62(6):728–37. doi: 10.1111/j.1440-1819.2008.01882.x
65. Tsujii N, Mikawa W, Tsujimoto E, Adachi T, Niwa A, Ono H, et al. Reduced left precentral regional responses in patients with major depressive disorder and history of suicide attempts. PloS One (2017) 12(4):e0175249. doi: 10.1371/journal.pone.0175249
66. Pu S, Yamada T, Yokoyama K, Matsumura H, Mitani H, Adachi A, et al. Reduced prefrontal cortex activation during the working memory task associated with poor social functioning in late-onset depression: multi-channel near-infrared spectroscopy study. Psychiatry Res (2012) 203(2–3):222–8. doi: 10.1016/j.pscychresns.2012.01.007
67. Tsujii N, Mikawa W, Tsujimoto E, Akashi H, Adachi T, Kirime E, et al. Relationship between prefrontal hemodynamic responses and quality of life differs between melancholia and non-melancholic depression. Psychiatry Res Neuroimaging. (2016) 253:26–35. doi: 10.1016/j.pscychresns.2016.04.015
68. Liu X, Sun G, Zhang X, Xu B, Shen C, Shi L, et al. Relationship between the prefrontal function and the severity of the emotional symptoms during a verbal fluency task in patients with major depressive disorder: a multi-channel NIRS study. Prog Neuropsychopharmacol Biol Psychiatry (2014) 54:114–21. doi: 10.1016/j.pnpbp.2014.05.005
69. Wang J, Lv B, Quan W, Wydell TN, Tian J, Wang P, et al. Right fronto-temporal activation differs between Chinese first-episode and recurrent Major Depression Disorders during a verbal fluency task: A near-infrared spectroscopy study. Psychiatry Res Neuroimaging. (2017) 264:68–75. doi: 10.1016/j.pscychresns.2017.03.013
70. Tsujii N, Mikawa W, Akashi H, Tsujimoto E, Adachi T, Kirime E, et al. Right temporal activation differs between melancholia and nonmelancholic depression: a multichannel near-infrared spectroscopy study. J Psychiatr Res (2014) 55:1–7. doi: 10.1016/j.jpsychires.2014.04.003
71. Nishida M, Kikuchi S, Matsumoto K, Yamauchi Y, Saito H, Suda S. Sleep complaints are associated with reduced left prefrontal activation during a verbal fluency task in patients with major depression: A multi-channel near-infrared spectroscopy study. J Affect Disord (2017) 207:102–9. doi: 10.1016/j.jad.2016.09.028
72. Rosenbaum D, Hagen K, Deppermann S, Kroczek AM, Haeussinger FB, Heinzel S, et al. State-dependent altered connectivity in late-life depression: a functional near-infrared spectroscopy study. Neurobiol Aging. (2016) 39:57–68. doi: 10.1016/j.neurobiolaging.2015.11.022
73. Pu S, Nakagome K, Yamada T, Yokoyama K, Matsumura H, Yamada S, et al. Suicidal ideation is associated with reduced prefrontal activation during a verbal fluency task in patients with major depressive disorder. J Affect Disord (2015) 181:9–17. doi: 10.1016/j.jad.2015.04.010
74. Pu S, Nakagome K, Yamada T, Yokoyama K, Matsumura H, Mitani H, et al. The relationship between the prefrontal activation during a verbal fluency task and stress-coping style in major depressive disorder: a near-infrared spectroscopy study. J Psychiatr Res (2012) 46(11):1427–34. doi: 10.1016/j.jpsychires.2012.08.001
75. Alexopoulos GS. Depression in the elderly. Lancet Lond Engl (2005) 365(9475):1961–70. doi: 10.1016/S0140-6736(05)66665-2
76. Kawano M, Kanazawa T, Kikuyama H, Tsutsumi A, Kinoshita S, Kawabata Y, et al. Correlation between frontal lobe oxy-hemoglobin and severity of depression assessed using near-infrared spectroscopy. J Affect Disord (2016) 205:154–8. doi: 10.1016/j.jad.2016.07.013
77. Onishi Y, Kikuchi S, Watanabe E, Kato S. Alterations in prefrontal cortical activity in the course of treatment for late-life depression as assessed on near-infrared spectroscopy. Psychiatry Clin Neurosci (2008) 62(2):177–84. doi: 10.1111/j.1440-1819.2008.01752.x
78. Koseki S, Noda T, Yokoyama S, Kunisato Y, Ito D, Suyama H, et al. The relationship between positive and negative automatic thought and activity in the prefrontal and temporal cortices: a multi-channel near-infrared spectroscopy (NIRS) study. J Affect Disord (2013) 151(1):352–9. doi: 10.1016/j.jad.2013.05.067
79. Akashi H, Noa T, Sakai S, Mikawa W, Kirime E, Shirakawa O. Inhibitory controls in bipolar and major depressive disorder: a NIRS study. Bipolar Disord (2012) 14:52.
80. Satomura Y, Sakakibara E, Takizawa R, Koike S, Nishimura Y, Sakurada H, et al. Severity-dependent and -independent brain regions of major depressive disorder: A long-term longitudinal near-infrared spectroscopy study. J Affect Disord (2019) 243:249–54. doi: 10.1016/j.jad.2018.09.029
81. Pu S, Nakagome K, Yamada T, Yokoyama K, Matsumura H, Nagata I, et al. Prefrontal activation predicts social functioning improvement after initial treatment in late-onset depression. J Psychiatr Res (2015) 62:62–70. doi: 10.1016/j.jpsychires.2015.01.009
82. Yamagata B, Yamanaka K, Takei Y, Hotta S, Hirano J, Tabuchi H, et al. Brain functional alterations observed 4-weekly in major depressive disorder following antidepressant treatment. J Affect Disord (2019) 252:25–31. doi: 10.1016/j.jad.2019.04.001
83. Aoki J, Iwahashi K, Ishigooka J, Fukamauchi F, Numajiri M, Ohtani N, et al. Evaluation of cerebral activity in the prefrontal cortex in mood [affective] disorders during animal-assisted therapy (AAT) by near-infrared spectroscopy (NIRS): A pilot study. Int J Psychiatry Clin Pract (2012) 16(3):205–13. doi: 10.3109/13651501.2011.644565
84. Takamiya A, Hirano J, Ebuchi Y, Ogino S, Shimegi K, Emura H, et al. High-dose antidepressants affect near-infrared spectroscopy signals: A retrospective study. NeuroImage Clin (2017) 14:648–55. doi: 10.1016/j.nicl.2017.02.008
85. Shinba T, Kariya N, Matsuda S, Matsuda H, Obara Y. Increase of frontal cerebral blood volume during transcranial magnetic stimulation in depression is related to treatment effectiveness: A pilot study with near-infrared spectroscopy. Psychiatry Clin Neurosci (2018) 72(8):602–10. doi: 10.1111/pcn.12680
86. Usami M, Iwadare Y, Kodaira M, Watanabe K, Saito K. Near Infrared Spectroscopy Study of the Frontopolar Hemodynamic Response and Depressive Mood in Children with Major Depressive Disorder: A Pilot Study. Yoshikawa T, editor. PloS One (2014) 9(1):e86290. doi: 10.1371/journal.pone.0086290
87. Payzieva S, Maxmudova D. NIRS Study of the Effects of Computerized Brain Training Games for Cognitive Rehabilitation of Major Depressive Disorder Patients in Remission: A Pilot Study. Stud Health Technol Inform. (2014) 199:163–7.
88. Schiffer F, Johnston AL, Ravichandran C, Polcari A, Teicher MH, Webb RH, et al. Psychological benefits 2 and 4 weeks after a single treatment with near infrared light to the forehead: a pilot study of 10 patients with major depression and anxiety. Behav Brain Funct BBF. (2009) 5:46. doi: 10.1186/1744-9081-5-46
89. Eschweiler GW, Wegerer C, Schlotter W, Spandl C, Stevens A, Bartels M, et al. Left prefrontal activation predicts therapeutic effects of repetitive transcranial magnetic stimulation (rTMS) in major depression. Psychiatry Res (2000) 99(3):161–72. doi: 10.1016/S0925-4927(00)00062-7
90. Arnone D, McIntosh AM, Ebmeier KP, Munafò MR, Anderson IM. Magnetic resonance imaging studies in unipolar depression: systematic review and meta-regression analyses. Eur Neuropsychopharmacol J Eur Coll Neuropsychopharmacol (2012) 22(1):1–16. doi: 10.1016/j.euroneuro.2011.05.003
91. Ho CSH, Zhang MWB, Ho RCM. Optical Topography in Psychiatry: A Chip Off the Old Block or a New Look Beyond the Mind-Brain Frontiers? Front Psychiatry (2016) 7:74. doi: 10.3389/fpsyt.2016.00074
92. Kirilina E, Jelzow A, Heine A, Niessing M, Wabnitz H, Brühl R, et al. The physiological origin of task-evoked systemic artefacts in functional near infrared spectroscopy. NeuroImage. (2012) 61(1):70–81. doi: 10.1016/j.neuroimage.2012.02.074
93. Caldwell M, Scholkmann F, Wolf U, Wolf M, Elwell C, Tachtsidis I. Modelling confounding effects from extracerebral contamination and systemic factors on functional near-infrared spectroscopy. NeuroImage. (2016) 143:91–105. doi: 10.1016/j.neuroimage.2016.08.058
94. Hanlly JR, Dewick HC, Davies ADM, Playeer J, Turnbull C. Verbal fluency in parkinson's disease. Neuropsychologia. (1990) 28(7):737–41. doi: 10.1016/0028-3932(90)90129-C
95. Zuckerman H, Pan Z, Park C, Brietzke E, Musial N, Shariq AS, et al. Recognition and Treatment of Cognitive Dysfunction in Major Depressive Disorder. Front Psychiatry (2018) 9:655. doi: 10.3389/fpsyt.2018.00655
96. Freedman M. Frontal and parietal lobe dysfunction in depression: delayed alternation and tactile learning deficits. Neuropsychologia (1994) 32(8):1015–25. doi: 10.1016/0028-3932(94)90050-7
97. Li J, Xu C, Cao X, Gao Q, Wang Y, Wang Y, et al. Abnormal activation of the occipital lobes during emotion picture processing in major depressive disorder patients. Neural Regener Res (2013) 8(18):1693–701.
Keywords: diagnostic, prediction, functional near-infrared spectroscopy, major depressive disorder, systematic review
Citation: Ho CSH, Lim LJH, Lim AQ, Chan NHC, Tan RS, Lee SH and Ho RCM (2020) Diagnostic and Predictive Applications of Functional Near-Infrared Spectroscopy for Major Depressive Disorder: A Systematic Review. Front. Psychiatry 11:378. doi: 10.3389/fpsyt.2020.00378
Received: 01 December 2019; Accepted: 15 April 2020;
Published: 06 May 2020.
Edited by:
Francisco Navarrete Rueda, Universidad Miguel Hernández de Elche, SpainReviewed by:
Noa Tsujii, Kindai University, JapanAni Gasparyan, Institute of Neurosciences of Alicante (IN), Spain
Copyright © 2020 Ho, Lim, Lim, Chan, Tan, Lee and Ho. This is an open-access article distributed under the terms of the Creative Commons Attribution License (CC BY). The use, distribution or reproduction in other forums is permitted, provided the original author(s) and the copyright owner(s) are credited and that the original publication in this journal is cited, in accordance with accepted academic practice. No use, distribution or reproduction is permitted which does not comply with these terms.
*Correspondence: Cyrus S. H. Ho, c3VfaHVpX2hvQG51aHMuZWR1LnNn