- 1NEUROex: Research Group in Physical Activity, Cognition and Behavior, Center of Health Sciences, Federal University of Rio Grande do Norte, Natal, Brazil
- 2Department of Physiology, Federal University of Juiz de Fora, Juiz de Fora, Brazil
Drug addiction is a worldwide public health problem, resulting from multiple phenomena, including those both social and biological. Chronic use of psychoactive substances has been shown to induce structural and functional changes in the brain that impair cognitive control and favor compulsive seeking behavior. Physical exercise has been proven to improve brain function and cognition in both healthy and clinical populations. While some studies have demonstrated the potential benefits of physical exercise in treating and preventing addictive behaviors, few studies have investigated its cognitive and neurobiological contributions to drug-addicted brains. Here, we review studies in humans using cognitive behavioral responses and neuroimaging techniques, which reveal that exercise can be an effective auxiliary treatment for drug addictive disorders. Moreover, we describe the neurobiological mechanisms by which exercise-induced neuroplasticity in the prefrontal cortex improves executive functions and may decrease compulsive behaviors in individuals prone to substance use disorders. Finally, we propose an integrative cognitive-psychobiological model of exercise for use in future research in drug addiction and practical guidance in clinical settings.
Introduction
Addiction to psychoactive substances (e.g., nicotine, cocaine, marijuana, alcohol, heroin, inhalants, LSD, and ecstasy) is a public health problem of the modern world (1). The Diagnostic and Statistical Manual of Mental Disorders of the American Psychiatric Association (DSM-V 2013) classifies drug addiction as a substance use disorder (SUD) when an individual meets two or more of the following criteria regarding the use of psychoactive substances: tolerance, craving, repeated attempts to stop use, or social, personal, physical, or psychological problems related to drug use (2). In addition to the influences of biological, cultural, social, economic, and psychological factors on individuals with SUD (3), studies in animal models and humans have shown that psychoactive substance use induces epigenetic, molecular, structural, and functional changes to the brain (4). Thus, the neurobiological model of drug addiction has proposed a complex interaction between biological and environmental factors and created new integrative perspectives for prevention, treatment, and pharmacological targets (5).
SUD is traditionally related to abnormal dopamine release and sensitivity in the brain reward system. This neural network is composed of several interconnected brain areas, including the ventral tegmental area, nucleus accumbens, amygdala, striatum, hippocampus, and prefrontal cortex (PFC) (6). The PFC is an integrated neural system in humans required for normal executive functioning, including decision-making and inhibitory control, and beneficial socio-emotional functioning (7). Studies using positron emission tomography (PET) and functional magnetic resonance imaging (fMRI) have demonstrated that individuals with SUD present decreased activityin the PFC (8). This condition seems to be related to a reduced number of dopamine receptors and an abnormal firing rate of dopaminergic neurons (9). These changes in the dopamine system and PFC activity may favor compulsive substance intake and seeking behaviors, as well as loss of control over drug consumption (8). Similarly, incomplete prefrontal cortex development and the resulting decrease in ability to control impulsive decisions has been suggested as an explanation for adolescents’ particular vulnerability to drug abuse (10), highlighting the importance of preventing the use of addictive psychoactive drugs during this period of brain development. Hence, contemporary rehabilitation programs have emphasized the importance of interdisciplinary treatment approaches that target the reestablishment of normal PFC functioning while combining the use of medication, social care, and behavioral therapy supported by psychiatrists, psychologists, social workers, and family (5).
Physical exercise has been proposed as a complementary therapy for individuals with SUD undergoing treatment at different stages of addiction rehabilitation (11–13). Preclinical animal research has shown evidence of neurobiological mechanisms induced by physical exercise that support its potential use as a therapeutic strategy to treat drug addiction. Examples are the following: normalizing dopaminergic and glutaminergic transmissions, promoting epigenetic interactions mediated by BDNF (brain-derived neurotrophic factor), and modifying dopaminergic signaling in the basal ganglia (11, 14). However, identifying similar molecular interactions between exercise and the human brain presents significant methodological challenges that need to be overcome in order totranslate these findings from animal models to humans.
The benefits of physical exercise for cognitive functioning and brain structure in humans are, on the other hand, well documented in literature (15). For instance, aerobic exercise is linked to improvements in executive functions and increased gray matter volume and activity in PFC regions (16, 17). Furthermore, children and adults with higher cardiorespiratory fitness (i.e., VO2 max) show improved cognitive performance and neuronal activity in the PFC and anterior cingulate cortex (ACC) (18). The results of preclinical animal studies show that these brain adaptations seem to be related to the release of exercise-induced molecules, such as BDNF (19) and IGF-1 (insulin-like growth factor 1) (20). Both molecules act as neurotrophic factors and create new synapses, neurons, and neural networks (18). These adaptations are facilitated by an increase in cerebral blood flow during exercise (21) and a release of a vascular endothelial growth factor (VEGF) (22), which promotes mitotic activity in vascular endothelial cells, thereby promoting angiogenesis and enhancing the oxygen and nutrient supply to neurons (18). Additionally, exercise is also related to the integrity of the brain-blood barrier (23). However, despite the wide range of benefits of the exercising brain, its effects on individuals with SUD who have impaired PFCs and cognitive functions need to be further investigated.
In this mini review, we present the results of a review of the current literature on exercise and SUD. We limited our search to studies that investigated the effect of acute or chronic aerobic exercise on cognitive and/or neurobiological markers in humans with SUD. The search terms used to select the articles were “tobacco cigarettes,” “nicotine,” “alcohol,” “methamphetamine,” “crack,” “cocaine and marijuana,” “physical activity,” “endurance exercise,” “aerobic exercise,” “addiction,”:substance use disorder,” “executive functions,” “prefrontal cortex,” “cognition,” and “brain.” Two authors selected the published and peer-reviewed articles identified on electronic databases (Pubmed Central, Medline, Scopus, and Web of Science) in February 2019, while a third author resolved differences in opinion. Only articles published in English were considered. Finally, we propose an integrative cognitive-psychobiological model of exercise to support future research on the subject and provide methodological guidance for its application in clinical settings as a therapeutic tool for the treatment of SUD.
The Effect of Aerobic Exercise on Brain and Cognitive Function in Individuals With SUD
Aerobic exercise is typically performed at submaximal intensity for a long duration with most of the energy consumption coming from mitochondrial oxygen-dependent production of ATP. Organic adaptations of the cardiorespiratory system as a result of aerobic training are mainly reflected by higher values of VO2 max, which has been associated with improvements in several health parameters, as well as brain and cognitive functioning (18, 24). Examples of aerobic exercise include running, swimming, and cycling among summer sports and cross-country skiing or speed skating among winter sports (25). Table 1 describes studies that investigated the effect of aerobic exercise on the brain and cognitive functions in individuals with SUD. Acute effects of aerobic exercise (i.e., immediately after exercise cessation) have been shown to include increases in PFC oxygenation associated with greater inhibitory control (26) and improved memory, attention, and speed processing in polysubstance users (27). Similarly, methamphetamine users who exercised on a stationary cycling ergometer exhibited improvements afterward, such as better drug-specific inhibitory control, reduced craving levels, and enhanced brain activity in the ACC, the area involved in conflict monitoring and inhibition (28). Wang et al. (29) and Wang, Zhou, and Chang (30) also studied methamphetamine users and showed that exercise performed at moderate intensity (i.e., 65–75% of maximum heart rate) elicits a decrease in craving levels, improves performance on a go/no-go task, and increases N2 amplitude during no-go conditions when the individuals have to inhibit the impulse to press the bottom of the computer screen after a visual cue. Notably, the N2 is an event-related potential, monitored using non-invasive electroencephalography (EEG), that originates from the fronto-parietal cortex and is directly associated with inhibitory control (31).
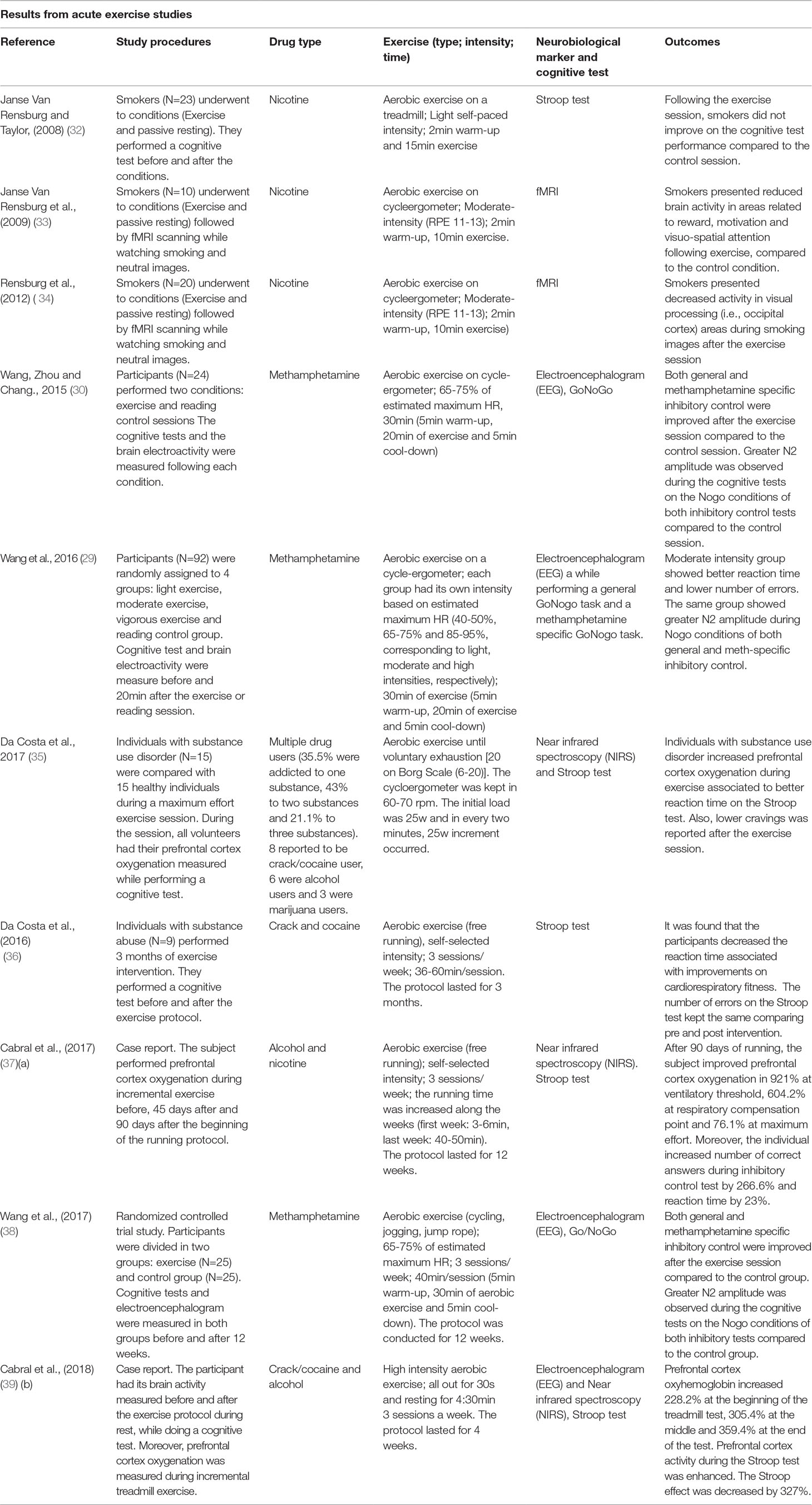
Table 1 Studies investigating the effects of physical exercise on the brain and cognitive functions in individuals with substance use disorders.
In nicotine users, a meta-analysis (40) and a systematic review (41) show little or no effect of exercise in smoking cessation. However, those reviews did not include studies using cognitive or neurobiological markers as outcomes. On the other hand, Rensburg et al. (32–34) conducted a series of important experiments that suggest potential benefits of aerobic exercise to the brain and cognitive functions of nicotine users. The first study showed that 15 min of light-intensity treadmill exercise reduced craving levels compared to a control condition (passive resting) but did not find improvements in inhibitory control. However, performance on the inhibitory control task was only measured by reaction time and not by the number of errors, which might limit our interpretation of the results (32). In the second experiment, 10 min of moderate-intensity cycling exercise elicited decreases in craving levels compared to a control condition (passive sitting for 10 min). After each condition, participants underwent fMRI scanning while viewing neutral pictures and pictures related to smoking. While viewing smoking images participants demonstrated reduced activation in brain areas related to reward (i.e., caudate nucleus), motivation (i.e., orbitofrontal cortex), and visuo-spatial attention (i.e., parietal lobe and parahippocampal gyrus) after exercise (33). Another study replicated the same experimental design with a larger sample of smokers. The results showed that 10 min of moderate-intensity exercise also reduced craving levels, and the fMRI analyses revealed decreased activity in visual processing (i.e., occipital cortex) areas during smoking images for the exercise condition but not for the control condition (passive sitting) (34). Thus, these results show the potential effects of aerobic exercise in modulating craving and correlated brain areas in nicotine users.
Therefore, despite the limited amount of studies available in the literature so far, it is apparent that acute sessions of aerobic exercise decrease craving levels and seem to benefit cognitive and brain functions in these individuals. However, it could also be important to understand if regularly performed exercise (i.e., chronic effects) may potentialize the acute benefits to the brain and cognition of individuals with SUD throughout weeks and months of exercise training. To date, only two studies have investigated the chronic effects of aerobic exercise in individuals with SUD using neurobiological and cognitive markers (Table 1). In one study, methamphetamine users showed improved inhibitory control and greater activation of the ACC during an inhibition task after performing 3 months of moderate-intensity exercise for 30 min three times a week (38). Curiously, this pioneering work by Wang et al. (38) did not report changes in cardiorespiratory fitness, which limited the association between the cardiorespiratory adaptations induced by exercise and improvements in brain and cognitive functioning. However, the results of a different pilot longitudinal study with polysubstance users showed that 3 months of aerobic exercise improved inhibitory control and was correlated with cardiorespiratory fitness improvements (36).
Because of the lack of longitudinal studies in the literature, we have conducted two case reports, in which we tested two different exercise interventions. The first one was a 3-month running program (three times a week), based on self-selected moderate-intensity exercise. The study was conducted with a chronic alcohol user receiving treatment in a public psychiatric hospital. Measures of PFC oxygenation, inhibitory control, and the need for medical intervention were assessed before and after the exercise program. At the end of the 3-month period, the participant demonstrated improved PFC oxygenation, decreased reaction time in the inhibitory control task, and reduced need for medical intervention (37). The second case report involved a crack/cocaine and alcohol user receiving treatment. They engaged in 4 weeks of high-intensity exercise (three times a week), and we measured PFC oxygenation, brain activity through electroencephalography, and inhibitory control before and after the intervention. The participant showed increased PFC activity during the inhibitory control test and increased PFC oxygenation during exercise (39). Taken together, the relationship between cognitive abilities and brain function and regular exercise suggests a promising role of physical exercise in promoting greater executive control on the compulsive behavior of individuals with SUD.
Psychobiology of Self-Selected Exercise Intensity: Practical Tool for Clinical Settings And Research
From an evolutionary perspective, humans have adapted to withstanding prolonged aerobic exercise through the search for food and persistence hunting of prey (supposedly pursued until physical exhaustion) (42). Aerobic self-selected exercise along with the cognitive appraisal of environmental cues for the acquisition of food and survival have been postulated to be key features in the development of the human brain (43). However, modern society has removed the need for humans to run/walk for food or shelter. As a result there is an increasing rate of hypokinetic behavior and related diseases such as diabetes, obesity, and hypertension (44, 45). Rational declarative decision-making concerning the volume, intensity, and frequency of exercise has not been sufficient to change sedentary behavior. Therefore, methods are being proposed to promote greater adherence to physical activity regiments, and a psychobiological integrative perspective appears to be a promising approach to achieve this goal (46, 47).
Cognitive and affective regulation of exercise intensity have been suggested to play a key role in both tolerance and adherence to exercise programs. For instance, homeostatic disturbances caused by high-intensity exercise have been associated with negative affective states and lower pleasure during exercise in sedentary individuals (45), leading to lower rates of adherence (48). Conversely, self-selected exercise intensity has been associated with positive affective states and higher levels of pleasure during exercise (45). Self-selected exercise intensity emphasizes the brain as the central governor of exercise intensity fluctuations (46), whereas the decision-making to increase and decrease velocity or tolerate or terminate the exercise session is controlled by the PFC through a bi-directional mind/body integration (49). Within this framework, top-down mechanisms are those initiated via declarative or non-declarative mental processing at the PFC level, which regulates muscle recruitment and alters physiological and behavioral responses. On the other hand, bottom-up mechanisms are initiated by sensitizing the ubiquitous somato-, viscero-, chemo-, and mechanical sensory receptors that influence central neural processing from the periphery to the brainstem, limbic system, and cerebral cortex (50). While performing any physical activity with self-selected intensity, the cognitive interpretation of the physiological state may be constantly working to preserve body homeostasis in order to reach the established goal (46, 51). In other words, fluctuations in pace while running are a behavioral outcome monitored by the brain (52). This behavioral modification results from integrating the task cognitive appraisal with afferent information related to biochemical and biophysical changes, such as temperature, heart and respiratory rate, blood pressure, blood concentrations of metabolites (e.g., PO2, PCO2, H+, HCO3−, and lactate), intramuscular H+, and energy substrate availability during the exercise (53).
Furthermore, feelings of fatigue and self-defeating thoughts demand inhibitory control mediated by the PFC in order to maintain physical activity (54). In this context, decision-making might be based on feelings such as perceived exertion (i.e., how hard the exercise is), affect (i.e., generic valence for good and bad feelings), and internal conversations such as “I cannot do it,” “I will give up,” or “it is very difficult” (53, 55). Therefore, self-selected exercise intensity emphasizes cognitive control (top-down) under the physiological changes (bottom-up) during physical effort (Figure 1), and it can be used as a strategy to develop self-monitoring and self-control abilities during the treatment of individuals with SUD. For instance, when setting a goal during an exercise session, such as running for a specific time or distance (i.e., time trial exercise), individuals need to regulate their pace to successfully complete that task. Thus, during the exercise, the decision to regulate the pace (running velocity) will be influenced by several environmental stimuli (i.e. weather, terrain, competitors, verbal instructions, and time or distance feedbacks) combined with the physiological state.
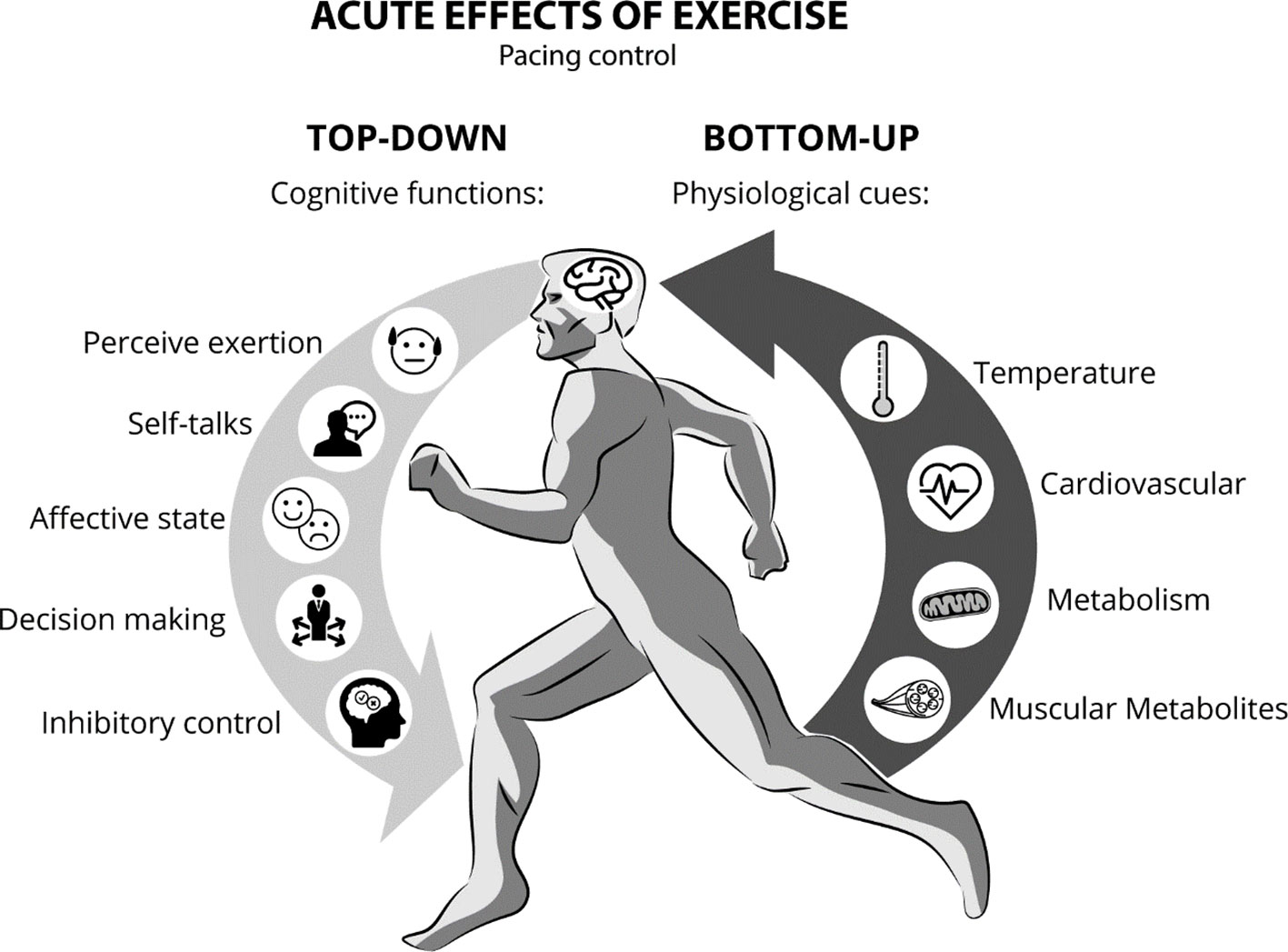
Figure 1 Pace control during continuous exercise while integrating top-down (cognitive functions) and bottom-up processing factors (physiological responses).
Several therapies focusing on this mind-body interaction through the top-down and bottom-up bi-directional mechanism have been suggested as promising rehabilitation tools in regulating stress and the immune system (56, 57). Therefore, we hypothesize that self-selected exercise intensity employs the bi-directional mechanism enabling improvements in self-control abilities associated with brain exercise-induced neuroplasticity. This cognitive regulation can be tested in humans while investigating perceptual responses, exercise-induced effects, and PFC function using neuroimaging methods (e.g., fMRI, PET scan, and fNIRS) and/or electroencephalogram. In addition, the brain responses can be associated with tests that evaluate the executive constructs of SUD-specific decision-making and inhibitory control, such as cue-reactivity go/no-go tests in which individuals have to inhibit their responses to salient stimuli relating to drug-related cues (e.g., drug behavior pictures). This cue-reactivity response has been shown to activate areas of the PFCand to predict relapses in different substances disorders (58, 59). Thus, we suggest that randomized clinical trials could follow the neuroscience paradigm and cognitive methodologies to test this hypothesis. In addition, the implementation of a control group would play a key role in these experimental designs in order to compare the self-selected intensity of exercise with other types of exercise intensity regulation to demonstrate its efficacy.
Conclusion
Despite the need for further prospective studies and clinical trials to test the efficacy of the psychobiological model of exercise as an intervention and treatment for SUD, physical exercise has been shown to be an effective and promising additional therapeutic tool for individuals with SUD. Here, we have described the brain areas affected by chronic substance use in patients with SUD as well as those improved by aerobic exercise. Some of these areas are primarily related to executive functions, which refer to a set of self-regulatory processes associated with the control of thoughts and behavior, including inhibitory control and decision-making. Therefore, in the same way that physical exercise is advised for treating other diseases, the neuroplasticity promoted by aerobic exercise may indicate its usefulness as a potential additional treatment for individuals with SUD. Specifically, these benefits may be seen in brain areas related to executive control, such as those areas involved in inhibition of drug-seeking behavior and impulsivity, as well as in decision-making regarding drug consumption. Furthermore, individuals with SUD who improve their fitness levels may enhance PFC function and cognition. These benefits should improve an individual’s ability to inhibit drug consumption behavior when exposed to environmental cues and, consequently, their ability to maintain abstinence. However, this is still a hypothesis, and further studies are necessary to provide evidence of the effectiveness of exercise on maintaining drug abstinence, specifically exercise of self-regulated intensity. Thus, we propose an integrative cognitive-psychobiological model of exercise for future research and provide practical guidance to optimize its potential benefits during rehabilitation programs.
Author Contributions
KC and EF conceived the idea, draft, figure and final revision. DC reviewed literature for table, described the results and final revision. RH reviewed manuscript and added theoretical framework, practical application and final revision.
Conflict of Interest Statement
The authors declare that the research was conducted in the absence of any commercial or financial relationships that could be construed as a potential conflict of interest.
References
1. Ali SF, Onaivi ES, Dodd PR, Cadet JL, Schenk S, Kuhar MJ, et al. Understanding the global problem of drug addiction is a challenge for IDARS scientists. Curr Neuropharmacol (2011) 9(1):2–7. doi: 10.2174/157015911795017245
2. Hasin DS, O’Brien CP, Auriacombe M, Borges G, Bucholz K, Budney A, et al. DSM-5 criteria for substance use disorders: recommendations and rationale. Am J Psychiatry (2013) 170(8):834–51. doi: 10.1176/appi.ajp.2013.12060782
3. Farisco M, Evers K, Changeux J-P. Drug addiction: from neuroscience to ethics. Front Psychiatry (2018) 9:595. doi: 10.3389/fpsyt.2018.00595
4. Volkow Nora D, Koob GF, McLellan AT. Neurobiologic advances from the brain disease model of addiction. N Eng J Med (2016) 374(4):363–71. doi: 10.1056/NEJMra1511480
5. Volkow Nora D, Boyle M. Neuroscience of addiction: relevance to prevention and treatment. Am J Psychiatry (2018) 175(8):729–40. doi: 10.1176/appi.ajp.2018.17101174
6. Leshner AI. Addiction is a brain disease, and it matters. Science (1997) 278(5335):45–7. doi: 10.1126/science.278.5335.45
7. Damasio AR. The somatic marker hypothesis and the possible functions of the prefrontal cortex. Philos Trans R Soc Lond B Biol Sci (1996) 351(1346):1413–20. doi: 10.1098/rstb.1996.0125
8. Goldstein RZ, Volkow ND. Dysfunction of the prefrontal cortex in addiction: neuroimaging findings and clinical implications. Nat Rev Neurosci (2011) 12(11):652–69. doi: 10.1038/nrn3119
9. Volkow ND, Fowler JS, Wang GJ, Baler R, Telang F. Imaging dopamine’s role in drug abuse and addiction. Neuropharmacology (2009) 56 Suppl 1:3–8. doi: 10.1016/j.neuropharm.2008.05.022
10. Winters KC, Arria A. Adolescent brain development and drugs. Prev Res (2011) 18(2):21–4. doi: 10.1037/e552592011-006
11. Lynch WJ, Peterson AB, Sanchez V, Abel J, Smith MA. Exercise as a novel treatment for drug addiction: a neurobiological and stage-dependent hypothesis. Neurosci Biobehav Rev (2013) 37(8):1622–44. doi: 10.1016/j.neubiorev.2013.06.011
12. Smith MA, Lynch WJ. Exercise as a potential treatment for drug abuse: evidence from preclinical studies. Front Psychiatry (2011) 2:82. doi: 10.3389/fpsyt.2011.00082
13. Wang D, Wang Y, Wang Y, Li R, Zhou C. Impact of physical exercise on substance use disorders: a meta-analysis. PLoS ONE (2014) 9(10):e110728. doi: 10.1371/journal.pone.0110728
14. Robison LS, Swenson S, Hamilton J, Thanos PK. Exercise reduces dopamine D1R and increases D2R in rats: implications for addiction. Med Sci Sports Exerc (2018) 50(8):1596–602. doi: 10.1249/MSS.0000000000001627
15. Baek S-S. Role of exercise on the brain. J Exerc Rehabil (2016) 12(5):380–5. doi: 10.12965/jer.1632808.404
16. Colcombe S, Kramer AF. Fitness effects on the cognitive function of older adults: a meta-analytic study. Psychol Sci (2003) 14(2):125–30. doi: 10.1111/1467-9280.t01-1-01430
17. Erickson KI, Kramer AF. Aerobic exercise effects on cognitive and neural plasticity in older adults. Br J Sports Med (2009) 43(1):22–4. doi: 10.1136/bjsm.2008.052498
18. Hillman CH, Erickson KI, Kramer AF. Be smart, exercise your heart: exercise effects on brain and cognition. Nat Rev Neurosci (2008) 9(1):58–65. doi: 10.1038/nrn2298
19. Griffin ÉW, Mullally S, Foley C, Warmington SA, O’Mara SM, Kelly AM. Aerobic exercise improves hippocampal function and increases BDNF in the serum of young adult males. Physiol Behav (2011) 104(5):934–41. doi: 10.1016/j.physbeh.2011.06.005
20. Trejo JL, Llorens-Martín MV, Torres-Alemán I. The effects of exercise on spatial learning and anxiety-like behavior are mediated by an IGF-I-dependent mechanism related to hippocampal neurogenesis. Mol Cell Neurosci (2008) 37(2):402–11. doi: 10.1016/j.mcn.2007.10.016
21. Ogoh S, Ainslie PN. Cerebral blood flow during exercise: mechanisms of regulation. J Appl Physiol (1985) (2009) 107(5):1370–80. doi: 10.1152/japplphysiol.00573.2009
22. During MJ, Cao L. VEGF, a mediator of the effect of experience on hippocampal neurogenesis. Curr Alzheimer Res (2006) 3(1):29–33. doi: 10.2174/156720506775697133
23. Buttler L, Jordão MT, Fragas MG, Ruggeri A, Ceroni A, Michelini LC. Maintenance of blood-brain barrier integrity in hypertension: a novel benefit of exercise training for autonomic control. Front Physiol (2017) 8:1048. doi: 10.3389/fphys.2017.01048
24. Ruegsegger GN, Booth FW. Health benefits of exercise. Cold Spring Harb Perspect Med (2018) 8(7). doi: 10.1101/cshperspect.a029694
25. Morici G, Gruttad’Auria CI, Baiamonte P, Mazzuca E, Castrogiovanni A, Bonsignore MR. Endurance training: is it bad for you? Breathe (2016) 12(2):140–7. doi: 10.1183/20734735.007016
26. Grandjean da Costa K, Soares Rachetti V, Quirino Alves da Silva W, Aranha Rego Cabral D, Gomes da Silva Machado D, Caldas Costa E, et al. Drug abusers have impaired cerebral oxygenation and cognition during exercise. PLoS ONE (2017) 12(11):e0188030. doi: 10.1371/journal.pone.0188030
27. Ferreira SE, dos Santos AK, de M, Okano AH, Gonçalves B, da SB, et al. Efeitos agudos do exercício físico no tratamento da dependência química. Revista Bras Ciênc Do Esporte (2017) 39(2):123–31. doi: 10.1016/j.rbce.2016.01.016
28. Leland DS, Arce E, Miller DA, Paulus MP. Anterior cingulate cortex and benefit of predictive cueing on response inhibition in stimulant dependent individuals. Biol Psychiatry (2008) 63(2):184–90. doi: 10.1016/j.biopsych.2007.04.031
29. Wang D, Zhou C, Zhao M, Wu X, Chang YK. Dose–response relationships between exercise intensity, cravings, and inhibitory control in methamphetamine dependence: an ERPs study. Drug Alcohol Depend (2016) 161:331–9.
30. Wang D., Zhou C., Chang Y. K. Acute exercise ameliorates craving and inhibitory deficits in methamphetamine: an ERP study. Physiol Behav (2015) 147:38–46.
31. Folstein JR, Van Petten C. Influence of cognitive control and mismatch on the N2 component of the ERP: a review. Psychophysiology (2008) 45(1):152–70. doi: 10.1111/j.1469-8986.2007.00602.x
32. Janse Van Rensburg K, Taylor AH. The effects of acute exercise on cognitive functioning and cigarette cravings during temporary abstinence from smoking. Hum Psychopharmacol (2008) 23(3):193–9. doi: 10.1002/hup.925
33. Janse Van Rensburg K, Taylor A, Hodgson T, Benattayallah A. Acute exercise modulates cigarette cravings and brain activation in response to smoking-related images: an fMRI study. Psychopharmacology (2009) 203(3):589–98. doi: 10.1007/s00213-008-1405-3
34. Janse Van Rensburg K, Taylor A, Benattayallah A, Hodgson T. The effects of exercise on cigarette cravings and brain activation in response to smoking-related images. Psychopharmacology (2012) 221(4):659–66. doi: 10.1007/s00213-011-2610-z
35. Da Costa KG, Rachetti VS, Da Silva WQA, Cabral DAR, da Silva Machado DG, Costa E. C., et al. (2017) Drug abusers have impaired cerebral oxygenation and cognition during exercise. PLoS One (2017) 12(11):e0188030.
36. da Costa KG, Barbieri JF, Hohl R, Costa EC, Fontes EB. Exercise training improves cardiorespiratory fitness and cognitive function in individuals with substance use disorders: a pilot study. Sport Sci Health (2016), 1–5. doi: 10.1007/s11332-016-0338-1
37. Cabral DA, da Costa KG, Okano AH, Elsangedy HM, Rachetti VP, Fontes EB. Improving cerebral oxygenation, cognition and autonomic nervous system control of a chronic alcohol abuser through a three-month running program. Addict Behav Rep (2017) 6(Supplement C):83–9. doi: 10.1016/j.abrep.2017.08.004
38. Wang D, Zhu T, Zhou C, Chang Y-K. Aerobic exercise training ameliorates craving and inhibitory control in methamphetamine dependencies: a randomized controlled trial and event-related potential study. Psychol Sport Exerc (2017) 30:82–90. doi: 10.1016/j.psychsport.2017.02.001
39. Cabral D, Tavares V, Costa K, Nascimento P, Faro H, Elsangedy H, et al. The benefits of high intensity exercise on the brain of a drug abuser. Global J Health Sci (2018) 10(6):123. doi: 10.5539/gjhs.v10n6p123
40. Klinsophon T, Thaveeratitham P, Sitthipornvorakul E, Janwantanakul P. Effect of exercise type on smoking cessation: a meta-analysis of randomized controlled trials. BMC Res Notes (2017) 10(1):442. doi: 10.1186/s13104-017-2762-y
41. Colledge F, Gerber M, Pühse U, Ludyga S. Anaerobic exercise training in the therapy of substance use disorders: a systematic review. Front Psychiatry (2018) 9:644. doi: 10.3389/fpsyt.2018.00644
42. Liebenberg L. The relevance of persistence hunting to human evolution. J Hum Evol (2008) 55(6):1156–9. doi: 10.1016/j.jhevol.2008.07.004
43. Lieberman Daniel E. The story of the human body: evolution, health, and disease. Vintage Books (2014).
44. Blair SN. Physical inactivity: the biggest public health problem of the 21st century. Br J Sports Med (2009) 43(1):1–2.
45. Ekkekakis P, Parfitt G, Petruzzello SJ. The pleasure and displeasure people feel when they exercise at different intensities: decennial update and progress towards a tripartite rationale for exercise intensity prescription. Sports Med (2011) 41(8):641–71. doi: 10.2165/11590680-000000000-00000
46. Ekkekakis P. Let them roam free? Physiological and psychological evidence for the potential of self-selected exercise intensity in public health. Sports Med (2009) 39(10):857–88. doi: 10.2165/11315210-000000000-00000
47. Parfitt G, Rose EA, Burgess WM. The psychological and physiological responses of sedentary individuals to prescribed and preferred intensity exercise. Br J Health Psychol 11(Pt (2006) 1:39–53. doi: 10.1348/135910705X43606
48. Mama SK, McNeill LH, McCurdy SA, Evans AE, Diamond PM, Adamus-Leach HJ, et al. Psychosocial factors and theory in physical activity studies in minorities. Am J Health Behav (2015) 39(1):68–76. doi: 10.5993/AJHB.39.1.8
49. Robertson CV, Marino FE. A role for the prefrontal cortex in exercise tolerance and termination. J Appl Physiol (1985) (2016) 120(4):464–6. doi: 10.1152/japplphysiol.00363.2015
50. Damasio A, Carvalho GB. The nature of feelings: evolutionary and neurobiological origins. Nat Rev Neurosci (2013) 14(2):143–52. doi: 10.1038/nrn3403
51. Noakes T, St C, Lambert E. From catastrophe to complexity: a novel model of integrative central neural regulation of effort and fatigue during exercise in humans. Br J Sports Med (2004) 38(4):511–4. doi: 10.1136/bjsm.2003.009860
52. Tucker R, Lambert MI, Noakes TD. An analysis of pacing strategies during men’s world-record performances in track athletics. Int J Sports Physiol Perform (2006) 1(3):233–45. doi: 10.1123/ijspp.1.3.233
53. St Clair Gibson A, Lambert EV, Rauch LHG, Tucker R, Baden DA, Foster C, et al. The role of information processing between the brain and peripheral physiological systems in pacing and perception of effort. Sports Med (2006) 36(8):705–22. doi: 10.2165/00007256-200636080-00006
54. Martin K, Staiano W, Menaspà P, Hennessey T, Marcora S, Keegan R, et al. Superior inhibitory control and resistance to mental fatigue in professional road cyclists. PLoS ONE (2016) 11(7). doi: 10.1371/journal.pone.0159907
55. Hardy J, Hall CR, Alexander MR. Exploring self-talk and affective states in sport. J Sports Sci (2001) 19(7):469–75. doi: 10.1080/026404101750238926
56. Buchanan TW, Tranel D. Central and peripheral nervous system interactions: from mind to brain to body. Int J Psychophysiol (2009) 72(1):1–4. doi: 10.1016/j.ijpsycho.2008.09.002
57. Taylor AG, Goehler LE, Galper DI, Innes KE, Bourguignon C. Top-down and bottom-up mechanisms in mind-body medicine: development of an integrative framework for psychophysiological research. Explore (NY) (2010) 6(1):29–41. doi: 10.1016/j.explore.2009.10.004
58. Hanlon CA, Dowdle LT, Gibson NB, Li X, Hamilton S, Canterberry M, et al. Cortical substrates of cue-reactivity in multiple substance dependent populations: transdiagnostic relevance of the medial prefrontal cortex. Transl Psychiatry (2018) 8. doi: 10.1038/s41398-018-0220-9
Keywords: aerobic exercise, neuralplasticity, substance use disorder, addiction, alcohol abuse
Citation: Costa KG, Cabral DA, Hohl R and Fontes EB (2019) Rewiring the Addicted Brain Through a Psychobiological Model of Physical Exercise. Front. Psychiatry 10:600. doi: 10.3389/fpsyt.2019.00600
Received: 12 February 2019; Accepted: 29 July 2019;
Published: 27 August 2019.
Edited by:
Alexander Mario Baldacchino, University of St Andrews, United KingdomReviewed by:
Ruben David Baler, National Institutes of Health (NIH), United StatesFlora Colledge, University of Basel, Switzerland
Copyright © 2019 Costa, Cabral, Hohl and Fontes. This is an open-access article distributed under the terms of the Creative Commons Attribution License (CC BY). The use, distribution or reproduction in other forums is permitted, provided the original author(s) and the copyright owner(s) are credited and that the original publication in this journal is cited, in accordance with accepted academic practice. No use, distribution or reproduction is permitted which does not comply with these terms.
*Correspondence: Eduardo Bodnariuc Fontes, ZWR1YXJkb2Jmb250ZXNAZ21haWwuY29t