- 1Université de Bordeaux, Institut de Neurosciences Cognitives et Intégratives d’Aquitaine, Pessac, France
- 2CNRS UMR 5287, Institut de Neurosciences Cognitives et Intégratives d’Aquitaine, Pessac, France
Persistent regional glucocorticoid (GC) dysregulation in alcohol-withdrawn subjects emerges as a key factor responsible for protracted molecular and neural alterations associated with long-term cognitive dysfunction. Regional brain concentrations of corticosterone vary independently from plasma concentrations in alcohol-withdrawn subjects, which may account for the treatment of alcohol withdrawal–induced persistent pathology. Thus, from a pharmacological point of view, a main issue remains to determine the relative efficacy of compounds targeting the GC receptors to attenuate or suppress the long-lasting persistence of brain regional GC dysfunctions in abstinent alcoholics, as well as persistent changes of neural plasticity. Data from animal research show that acting directly on GC receptors during the withdrawal period, via selective antagonists, can significantly counteract the development and persistence of cognitive and neural plasticity disorders during protracted abstinence. A critical remaining issue is to better assess the relative long-term efficacy of GC antagonists and other compounds targeting the corticotropic axis activity such as gamma-aminobutyric acid A (GABAA) and GABAB agonists. Indeed, benzodiazepines (acting indirectly on GABAA receptors) and baclofen (agonist of the GABAB receptor) are the compounds most widely used to reduce alcohol dependence. Clinical and preclinical data suggest that baclofen exerts an effective and more powerful counteracting action on such persistent cognitive and endocrine dysfunctions as compared to diazepam, even though its potential negative effects on memory processes, particularly at high doses, should be better taken into account.
Introduction
Alcoholism is characterized by periods of sustained alcohol consumption, in part due to changes in neural circuits mediating anxiety and stress disorders, notably the prefrontal cortex (PFC) and structures such as the hippocampus (HPC) and the amygdala (AMG) (1, 2). Indeed, the PFC–HPC–AMG circuit plays key roles in modulating neuroadaptive responses to stress and anxiety and is markedly and consistently altered in most of neuropsychiatric disorders (3, 4).
Alterations of hypothalamic–pituitary–adrenal (HPA) axis activity is a prime mechanism contributing to protracted alcoholism (5) and the release of glucocorticoids (GCs; cortisol in humans and primates, corticosterone in rodents) from the adrenal glands. Clinical and experimental data in both humans (6–8) and rodents (5, 9, 10) have shown that both acute and chronic alcohol consumption, as well as alcohol withdrawal, enhanced plasma GCs and decreased GC receptor (GR) availability (11). In addition, even though the relationships between HPA axis activity, craving, and alcohol intake during early abstinence have been particularly well documented (4, 12), little is known on such a relationships during protracted abstinence. Moreover, whereas most of the measures of the HPA axis activity are peripheral, some brain regions playing a critical role in either memory or reward processes have been shown to exhibit sustained local GC dysfunction in contrast to a transient increase in circulating GC level, a phenomenon that is as yet insufficiently taken into account to understand alcohol relapse in abstinent subjects (13, 14).
In the first part, this review thus provides updated clinical and experimental evidence for the persistence of brain regional GCs over protracted alcohol abstinence and how sustained GC-related neurocognitive dysfunction might possibly lead to relapse. In the second part, this paper focuses on the efficacy of pharmacological compounds modulating, directly or indirectly, GC receptors to suppress or attenuate these long-lasting neurocognitive alterations in alcohol-withdrawn subjects. Even though alcohol withdrawal affects numerous brain structures and networks (15–17), in this review, we focused on PFC-related studies. Indeed, on the one hand, it has been shown that neurons of the PFC are dramatically vulnerable to the oxidative stress mediated by chronic alcohol exposure, leading to important neuronal cell death (18), and on the other hand, our own studies have shown that alcohol withdrawal induced protracted GC alterations in the PFC that were responsible for working memory (WM) impairments in mice (13, 19, 20).
Persistent Brain Regional Glucocorticoid Alterations After Protracted Alcohol Abstinence
Alcohol withdrawal induced protracted alterations of corticosteroid-releasing factor (CRF) and plasma corticosterone in the HPC, the PFC, and the hypothalamus, far beyond the detoxification step (10). Interestingly, the long-lasting neuroadaptive changes of GCs caused by prolonged alcohol withdrawal within neural circuits involved in learning, memory, and emotions are only scarcely known.
The initial phase of alcohol withdrawal is characterized by increases of both plasma and brain GC concentrations (13, 14, 19, 21). Little and colleagues (14) were the first to show in rodents that during the initial phase of withdrawal after 8 months of chronic alcohol consumption (CAC), rats and mice showed exaggerated corticosterone levels in the PFC and the HPC. The excessive corticosterone level in the PFC of alcohol-withdrawn rodents persisted for up to 2 months, whereas circulating corticosterone level already returned to basal concentrations. Other studies also reported that protracted high levels of local corticosterone concentration are important factors for the maintenance of cognitive impairments after prolonged cessation of alcohol intake in rodents (19, 22, 23) and in abstinent patients (11, 24). The persistence of altered regional GC responses to long-term alcohol withdrawal could be a clue to understand how the local neuroadaptive changes to withdrawal generate sustained downstream molecular and neurofunctional activity disorders, notably in the PFC–HPC–AMG circuit, and could promote relapse to alcohol-seeking behavior (see Figure 1).
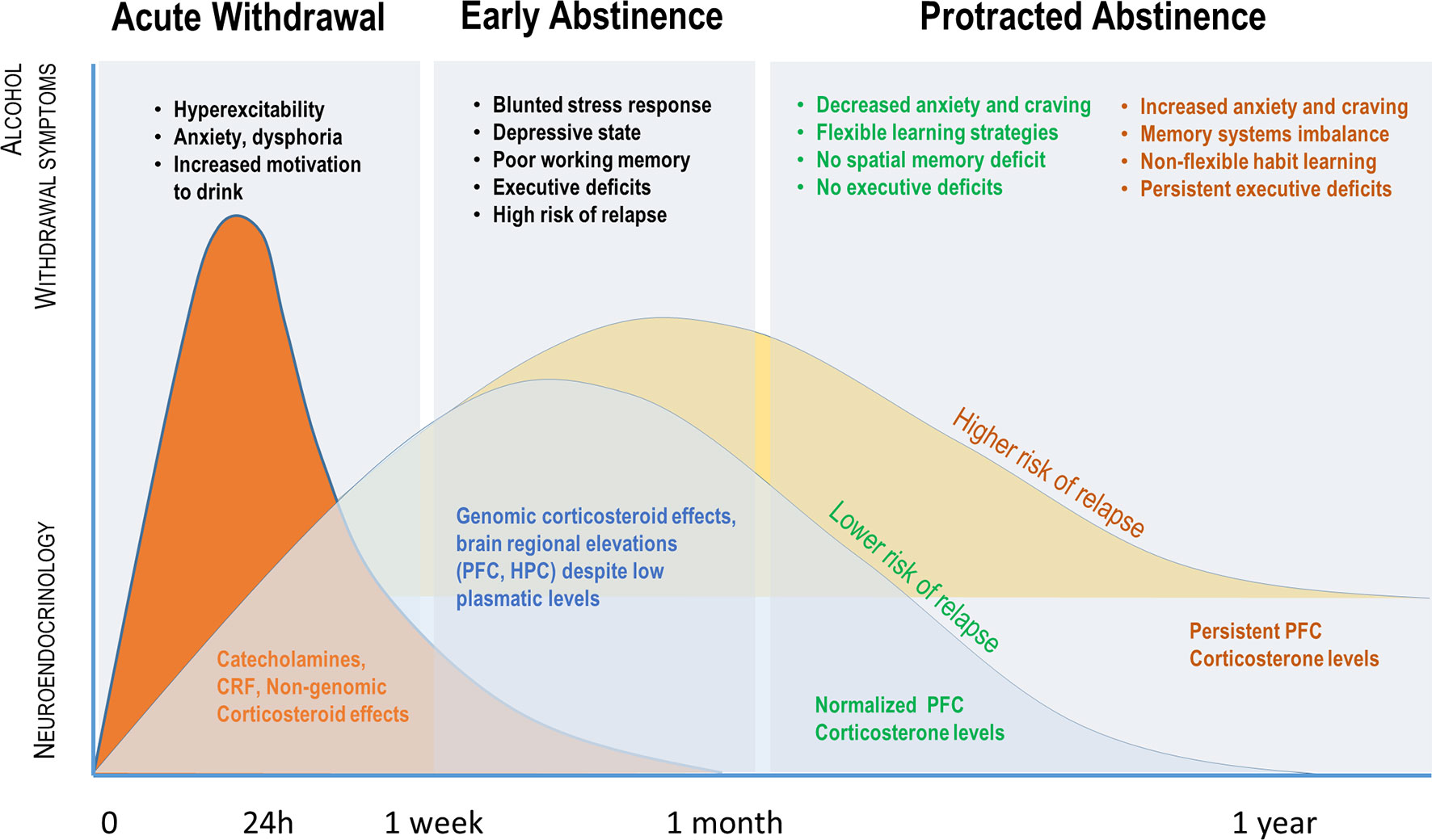
Figure 1 Parallel evolution of alcohol withdrawal–induced symptoms and plasmatic or brain regional glucocorticoid (GC) levels over time. Acute withdrawal is associated with a release of catecholamines, CRF, and high plasmatic GCs, which mediate physiological and behavioral symptoms initially through non-genomic effects. The early abstinence period is associated with a decrease in plasmatic GC concentration as opposed to a brain regional GC increase, particularly in the PFC, likely involving genomic effects of GC. Although brain GC concentration and affective/cognitive symptoms will be normalized in many dependent subjects, persistence of elevated brain GC levels and cognitive impairments in others is predictive of high risk of relapse (see text for details).
In our own studies, in vivo intracerebral microdialysis was used to evaluate the evolution of corticosterone concentration over time in the medial PFC and the dorsal HPC during and after completion of a WM task in mice previously submitted to a 6-month CAC period followed by either a short (1 week) or prolonged (6 weeks) withdrawal period (13, 19). This WM task was based on spontaneous alternation behavior, which involves intact interconnections between the PFC and the HPC for successful performance (25, 26). We observed that behavioral testing produced an exaggerated corticosterone rise in the medial PFC regardless of withdrawal duration, in spite of normal circulating GC levels. In addition, a late deficit in the inhibitory feedback response on HPA axis activity in both the PFC and the dorsal hippocampus (dHPC) was also observed in withdrawn mice, even though unrelated with the WM deficit (19). Interestingly, the severity of the memory deficit correlated positively with high levels of PFC corticosterone concentration, showing that there is a functional link between exaggerated corticosteroid responses and PFC-related cognitive dysfunction (27–29). The persistent elevation in PFC corticosterone levels in withdrawn mice could be due to the presence of local GC production, proximity to target cells, and possibly, tissue-specific control mechanisms (30). Our findings agree with many neuroimaging studies showing structural and functional deficits in PFC regulatory regions (31) or blood flow alterations in the medial frontal lobe (32). Thus, a functional disconnection between the PFC and the AMG emerges as an early index of neuroadaptation in alcohol dependence that predicts PFC-dependent cognitive impairments during abstinence (29, 33, 34). Endogenous GCs are critically implicated in maintaining PFC-dependent cognitive functions (35). Consistently, an increase in cortisol predicts frontal cortex–related cognitive deficit as shown either after a hydrocortisone administration or in pathological condition such as in Cushing’s disease (33, 36–39).
Regional GC Alterations and Neural Plasticity
GCs influence brain function through two types of GRs, the high-affinity mineralocorticoid receptor (MR) or the low-affinity GR (40). GR acts as a nuclear transcription factor to regulate expression of various target genes (41–43). They also play an important role in the addiction to alcohol. For instance, GR-mediated plasticity increased voluntary alcohol intake (44), whereas GR antagonist reduced alcohol intake (45) in rats. Thus, alterations of GCs in withdrawn subjects could impair neural plasticity within the PFC–HPC–AMG circuitry implicated in stress and higher cognitive function, such as declarative memory and WM (19, 23, 29, 46, 47).
Several lines of research have shown that GC alterations disrupt memory processes through either changes in activated/phosphorylated cAMP response element-binding protein (pCREB) due to GR dysfunction (48–50) or the MR-mediated effects on the cAMP–protein kinase A (PKA) cascade (51, 52). A recent study in our lab reported that early and protracted withdrawal periods after prolonged alcohol consumption produced WM deficits in a sequential alternation task, which were associated with reduced pCREB levels, more specifically in the PFC, whereas none of these impairments were observed in mice still under alcohol condition (13). Results further indicated that local injection of the PKA activator (Sp-cAMPS) into the PFC significantly improves or impairs, respectively, WM performance in withdrawn and water animals (13). These findings strongly support the view that dysregulation of the cAMP–PKA–CREB signaling pathway, particularly in PFC, is a key molecular signature of the cognitive dysfunction during alcohol withdrawal (53–56). The impact of GCs on PFC function is thought to be driven mainly, although not exclusively (57), via complex local interactions between dopaminergic and glutamatergic receptors (58, 59).
Rescuing Long-Lasting Withdrawal-Induced Cognitive and Glucocorticoid Dysfunction by Targeting GC Receptors
The highest densities of MR are expressed in the HPC (60–62). In contrast, the GRs are widely distributed throughout the brain (40, 63, 64), mainly in areas involved in learning and memory. These areas are particularly sensitive to the effects of stress, namely the PFC–HPC–AMC.
AMG circuitry (65–67). More specifically, as shown in human studies of Cushing’s syndrome, sustained cortisol elevation over the years alters the integrity of the HPC–PFC circuitry and accordingly influences the severity of various cognitive dysfunctions (37, 38, 68–70). Indeed, GC impairment of WM critically depends on influences within the PFC (27–29), and selective impairments of frontal cortical functions during withdrawal in detoxified alcoholics have been reported (16, 34, 71–74). These findings are in agreement with several studies indicating that exaggerated concentrations of GCs produced PFC dysfunction, as also reported in depression or Cushing’s syndrome (16, 36, 37, 68, 75–78).
Several types of pharmacological compounds acting on GC release or the GC receptors have been used to restore memory function after alcohol withdrawal. Thus, mifepristone (a GR antagonist) or the dihydropyridine calcium channel nimodipine, delivered prior to withdrawal from chronic alcohol exposure, reduced both the protracted rises in brain corticosterone and sustained cognitive or motivational deficits in mice (22) or rats (79). Recently, we studied whether the regional GC blockade in the medial PFC suppressed WM deficits in alcohol-withdrawn mice. To that aim, withdrawn mice were given intraperitoneal administration of metyrapone (a corticosterone synthesis inhibitor) prior to testing. We found that the withdrawal-induced WM impairments were totally alleviated, confirming the key role of persistent enhanced GC levels in withdrawal-associated cognitive impairments. Similarly, acute intra-PFC infusion of spironolactone that diminished MR activation, and to a lesser extent, of mifepristone that diminished GRs activation, fully restored WM function in withdrawn mice. In contrast, neither spironolactone nor mifepristone had any effect when infused into the dorsal HPC (19). These data are congruent with findings reporting that high GC levels via either corticosterone administration or local infusion of the GR agonist RU 28362 into the medial PFC shortly before testing similarly impair WM (29), while the GR antagonist RU 38486 infused into the PFC can restore stress-induced deficits in executive function (59). All together, these findings suggest that long-term adaptive behavioral effects of withdrawal after a long alcohol exposure are mediated in large part through sustained GC dysregulation within the PFC circuitry, while circulating corticosterone levels are already normalized.
Recovery of PFC Functions and Successful Protracted Abstinence
There is now extensive evidence showing that recovery of PFC cognitive function is related to long-lasting abstinence in alcoholics (16, 80, 81). This raises two critical issues that should be addressed by future clinical and animal research. Firstly: is recovery of executive functions relying on restoration of normal PFC activity, or is it the result of compensatory activity in other cortical or hippocampal regions as previously suggested (82)? For instance, it remains unclear whether some of the withdrawal-induced cognitive impairments are due to the PFC itself or the HPC (16, 73, 74, 83, 84). Since many confounding factors may limit the relevance of clinical studies in that matter, it is an essential task of preclinical models of alcohol dependence to better understand regional cellular substrates of these cognitive deficits. Secondly, GC release corresponds to a physiological mechanism (negative feedback control), which is preparing the organism to cope and eventually to recover from various environmental threats (85). The medial PFC is a critical target area for the negative-feedback effects of GCs on HPA activity after stress (86). There is increasing evidence showing that PFC-dependent cognitive impairments in many alcohol-dependent subjects are no longer observed after 1 year of abstinence (81, 82, 87). Therefore, a fundamental issue will be to better evaluate the long-term benefits of targeting GC activity, in order to determine what GC-related treatments are effective in reducing transient withdrawal-induced cognitive deficits without compromising normalization of the stress system reactivity and cognitive function.
Targeting GC Activity During Withdrawal via GABAergic Agonists
Another way to rescue the protracted regional GC dysregulation in alcohol-withdrawn subjects and rodents is to act directly or indirectly on the GABAergic neurotransmission during the withdrawal period. Indeed, the GABAergic system modulates the HPA axis response to stress (88–91) mainly through its inhibitory action on corticotropin-releasing hormone (CRH) cells of the paraventricular nucleus of the hypothalamus, which regulates GC release by the adrenal gland (92). Experimental data have already shown benefic effects of GABAA agonist (muscimol) on alcohol tolerance and dependence in rats (93). However, among benzodiazepines (such as lorazepam, chlordiazepoxide, and oxazepam) acting on the GABAA receptor, diazepam is the most commonly used, mainly given its prolonged half-life (94). Given that, diazepam has been widely used to reduce the negative side effects of alcohol withdrawal and transiently delivered in alcoholics mainly with the aims of reducing anxiety and decreasing neural excitability in the early phase of the cessation of alcohol intake (95–100).
However, given the high variability of patients’ reactions to diazepam, its use may also be causal of strong deleterious neurocognitive and affective disorders (101). For example, diazepam induces deleterious effects on cognitive functions (mainly amnesia) in humans (102–104) and rodents (89, 105, 106) that resemble those induced by chronic alcohol consumption and withdrawal (105). In addition, it is well established that addiction to benzodiazepines can develop over time in treated alcoholics or in people with a history of a substance use disorder (100, 107). Furthermore, chronic diazepam treatment potentiates the addictive properties of psychostimulants such as cocaine (108). In a rodent model of chronic intermittent access to alcohol leading to escalation of alcohol intake, George et al. (109) showed that recruitment of GABAergic and CRH cells in the medial PFC during withdrawal and disruption of the PFC–central AMG pathway are causal factors for impairments of executive control over motivated behavior, suggesting that alterations of medial PFC interneurons may be a prime signature of neuroadaptation in dependence on alcohol. Interestingly, functional inactivation of the orbitofrontal cortex by agonists of the GABAA (muscimol) and GABAB (baclofen) receptors disrupts the context-induced relapse to alcohol and executive control in rats (110). Overall, in spite of motivational and cognitive disorders potentially linked to the use of benzodiazepines, they remain the most common pharmacological compounds used to reduce the negative side effects of alcohol withdrawal in humans. Indeed, other compounds such as anticonvulsant drugs (carbamazepine, valproic acid, or gabapentin, for instance) and barbiturates such as phenobarbitone also attenuate alcohol withdrawal symptoms, but their use is often limited by negative side effects or insufficient benefic effects as compared to benzodiazepines [for a comprehensive review, see Ref. (111)].
Initially used for its myorelaxant effect through its agonist action on GABAB receptors (112), baclofen was found to modulate HPA axis activity (113) and to reduce HPA axis activity in withdrawn alcoholics (114). Baclofen has been used only recently in the treatment of alcohol dependence (97, 115, 116). Although the initial case report put an emphasis on its anticraving properties (117), increasing evidence suggests that different mechanisms could account for the effects of baclofen on motivational and physical symptoms of alcohol withdrawal (115–119). Clinical and experimental data have mentioned an attenuation of alcohol dependence in both humans and animals, even though adverse events have been also reported, mainly with high baclofen doses (110, 120–123). Whereas beneficial effects of both diazepam and baclofen after a short period of alcohol withdrawal are well documented, a critical issue that remains under question is to determine the relative efficacy of these compounds at rescue from the persistent cognitive and biological alterations resulting from long withdrawal periods. In humans, diazepam and baclofen induced comparable and similar physical symptoms to those of alcohol withdrawal, such as anxiety, sweating, and tremors over a 10-day withdrawal period (124). A recent survey study did not report different qualitative effects of baclofen as regards other benzodiazepines (diazepam, chlordiazepoxide) in the treatment of severe alcohol withdrawal syndrome (125). In contrast, another study showed a greater efficacy of chlordiazepoxide as compared to baclofen in reducing the physical symptoms of alcohol withdrawal (126). Low doses of baclofen associated with benzodiazepine administration lowered the dose of lorazepam used to counteract the increase in anxiety resulting from the cessation of alcohol intake (127). We recently confirmed the corrective effects of a 9-day diazepam administration on memory dysfunction, GC levels, and altered pCREB in the PFC after a short (1 week) withdrawal period in mice; however, these benefic effects were only transient since they were not observed after a longer (4 weeks) alcohol withdrawal period (128) (and see Figure 2). The lack of efficacy of subchronic diazepam injections to alleviate the protracted cognitive and biological alterations in 4-weeks-withdrawn mice may result from sustained alterations of GABAA receptors (99, 129, 130), increased downregulation of these receptors over repeated diazepam administration (131), or other neuroadaptations that may progressively emerge after withdrawal, such as alterations of epigenetic mechanisms (4, 20).
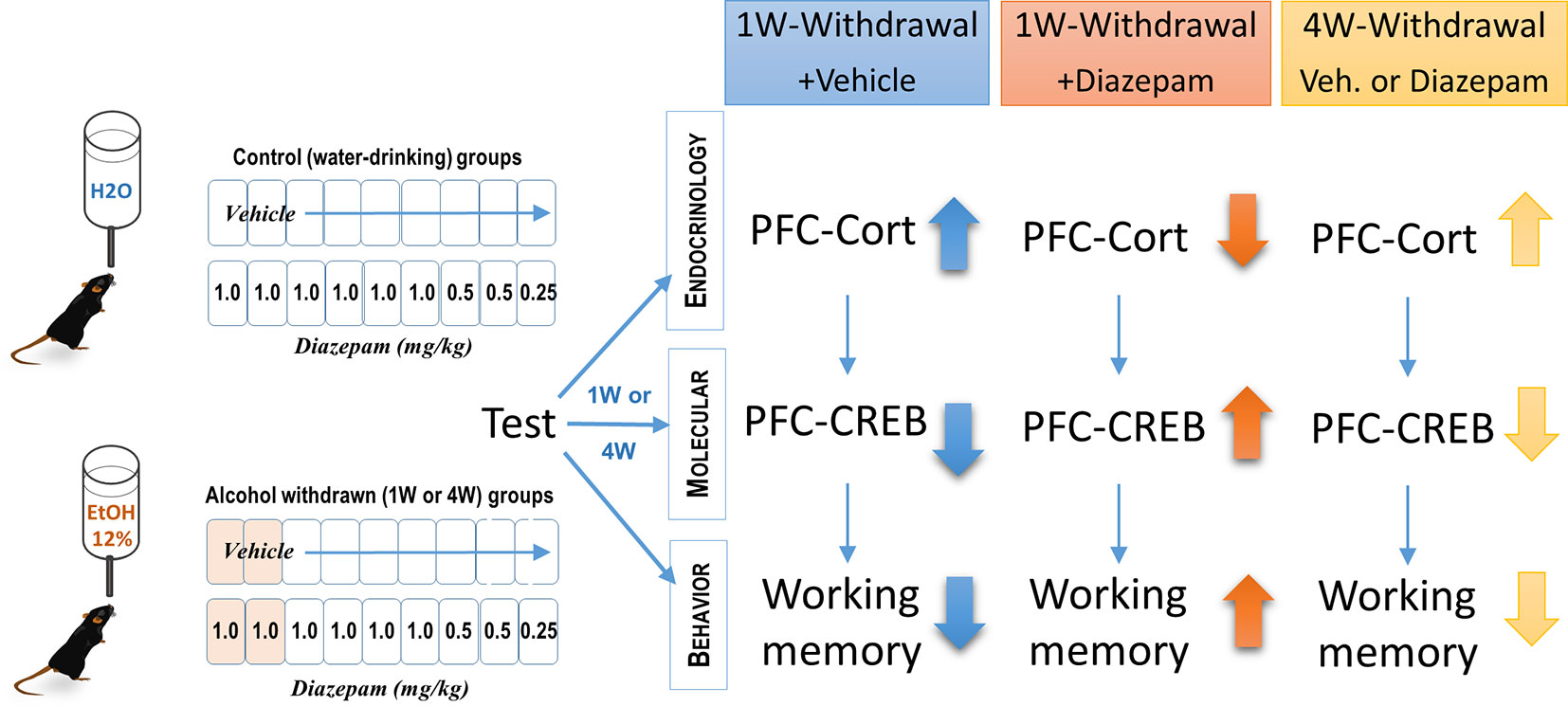
Figure 2 Effects of diazepam treatment on cognitive deficits, prefrontal cortical (PFC) GC levels, and pCREB expression in alcohol-withdrawn C57BL6/J mice. Chronic alcohol consumption lasted 6 months at 12% (v/v). This pharmacological study showed that 1-week-withdrawn mice receiving vehicle exhibited increased levels of corticosterone, reduced pCREB activity in the PFC, and working memory deficits as assessed with a sequential alternation task, 24 h after the last diazepam injection. Diazepam administered i.p. at decreasing doses ranging from 1.0 to 0.25 mg/kg every day during the 9 days of the withdrawal phase transiently (1 week but not 4 weeks) reversed both the endocrine and cognitive impairments observed in vehicle-treated animals (128).
In contrast to diazepam, other studies have reported beneficial effects of baclofen after protracted alcohol withdrawal. More specifically, Geisel et al. (114) evidenced in abstinent alcoholics sustained increased plasma GC levels, which decreased significantly in baclofen-treated patients, up to 14 weeks after treatment. Authors suggested that a decrease of CG levels during treatment with high-dose baclofen contributes to its preventive effects on alcohol relapse. In line with this hypothesis, we reported in recent experiments, as yet unpublished, a clear-cut dissociation between baclofen and diazepam in the protracted GCs and motivational dysfunction in alcohol-withdrawn mice. Using an odor place preference paradigm, we showed that alcohol-withdrawn animals receiving an acute stress (electric foot shocks) before the recognition session exhibited an abnormal rise of plasma corticosterone as compared to stressed controls, as well as a strong preference for an area impregnated with the odor of alcohol at the expense of a zone impregnated with water. Interestingly, repeated administration of baclofen administered during the withdrawal period normalized the stress-induced plasma corticosterone rise and concomitantly suppressed the stress-induced alcohol place preference, up to 4 weeks after the cessation of alcohol intake, whereas diazepam had only a short transitory (1 week) beneficial effect (132).
In spite of its promising effect in the treatment of protracted alcohol-related neurocognitive and motivational disorders, one might note that a limitation of the use of baclofen is associated with the determination of the relevant dose to induce beneficial effects without negative side disorders (116) such as cognitive and emotional disorders, which have been reported with high doses (133). Its use in humans might require closet medical surveillance given the pathological alterations associated with self-misuse or high doses of this compound.
Concluding Remarks
From a functional point of view, persistent regional GC dysregulation in alcohol-withdrawn subjects emerges as a key factor responsible for protracted molecular and neural alterations associated with long-term cognitive dysfunction. The demonstration that regional brain concentrations of GCs can change in alcohol-withdrawn subjects independently from circulating concentrations has important implications for the treatment of alcohol withdrawal–induced persistent pathology. Thus, from a pharmacological point of view, a main issue remaining to be resolved concerns the relative efficacy of compounds targeting the GC receptors to attenuate or suppress the long-lasting persistence of brain regional GC dysfunctions in abstinent alcoholics, as well as other persistent changes of neural plasticity. Data from animal experimentation show that acting directly on GRs during the withdrawal period, via selective antagonists, can significantly counteract the development and persistence of cognitive and neural plasticity disorders during protracted abstinence. A critical remaining issue is to better assess the relative long-term efficacy of GABAA and GABAB agonists in counteracting the protracted brain regional GCs and neurocognitive dysfunctions resulting from alcohol withdrawal. Clinical and preclinical data suggest that the agonist of the GABAB receptor baclofen exerts an effective counteracting action on such persistent dysfunctions. However, there is still a need for a better evaluation of its potential negative side effects, particularly when using high doses over a long period of time.
Author Contributions
DB and VD wrote the paper. NM edited it.
Funding
This work was supported by the Centre National de la Recherche Scientifique (CNRS), The University of Bordeaux, and the Regional Council of the Aquitaine Region (DB, VD).
Conflict of Interest Statement
The authors declare that the research was conducted in the absence of any commercial or financial relationships that could be construed as a potential conflict of interest.
References
1. Heilig M, Koob GF. A key role for corticotropin-releasing factor in alcohol dependence. Trends Neurosci (2007) 30(8):399–406. doi: 10.1016/j.tins.2007.06.006
2. Breese GR, Sinha R, Heilig M. Chronic alcohol neuroadaptation and stress contribute to susceptibility for alcohol craving and relapse. Pharmacol Ther (2011) 129(2):149–71. doi: 10.1016/j.pharmthera.2010.09.007
3. Godsil BP, Kiss JP, Spedding M, Jay TM. The hippocampal–prefrontal pathway: the weak link in psychiatric disorders? Eur Neuropsychopharmacol (2013) 23(10):1165–81. doi: 10.1016/j.euroneuro.2012.10.018
4. Palmisano M, Pandey SC. Epigenetic mechanisms of alcoholism and stress-related disorders. Alcohol (2017) 60:7–18. doi: 10.1016/j.alcohol.2017.01.001
5. Richardson HN, Lee SY, O'Dell LE, Koob GF, Rivier CL. Alcohol self-administration acutely stimulates the hypothalamic–pituitary–adrenal axis, but alcohol dependence leads to a dampened neuroendocrine state. Eur J Neurosci (2008) 28(8):1641–53. doi: 10.1111/j.1460-9568.2008.06455.x
6. Lovallo WR, Dickensheets SL, Myers DA, Thomas TL, Nixon SJ. Blunted stress cortisol response in abstinent alcoholic and polysubstance-abusing men. Alcohol Clin Exp Res (2000) 24(5):651–8. doi: 10.1111/j.1530-0277.2000.tb02036.x
7. Adinoff B, Ruether K, Krebaum S, Iranmanesh A, Williams MJ. Increased salivary cortisol concentrations during chronic alcohol intoxication in a naturalistic clinical sample of men. Alcohol Clin Exp Res (2003) 27(9):1420–7. doi: 10.1097/01.ALC.0000087581.13912.64
8. Sinha R, Fox HC, Hong KI, Hansen J, Tuit K, Kreek MJ. Effects of adrenal sensitivity, stress- and cue-induced craving, and anxiety on subsequent alcohol relapse and treatment outcomes. Arch Gen Psychiatry (2011) 68(9):942–52. doi: 10.1001/archgenpsychiatry.2011.49
9. Rasmussen DD, Boldt BM, Bryant CA, Mitton DR, Larsen SA, Wilkinson CW, et al. Chronic daily ethanol and withdrawal: 1. Long-term changes in the hypothalamo–pituitary–adrenal axis. Alcohol Clin Exp Res (2000) 24(12):1836–49. doi: 10.1111/j.1530-0277.2000.tb01988.x
10. Zorrilla EP, Valdez GR, Weiss F. Changes in levels of regional CRF-like immunoreactivity and plasma corticosterone during protracted drug withdrawal in dependent rats. Psychopharmacology (Berl) (2001) 158(4):374–81. doi: 10.1007/s002130100773
11. Errico AL, King AC, Lovallo WR, Parsons OA. Cortisol dysregulation and cognitive impairment in abstinent male alcoholics. Alcohol Clin Exp Res (2002) 26(8):1198–204. doi: 10.1097/01.ALC.0000025885.23192.FF
12. Stephens MA, Wand G. Stress and the HPA axis: role of glucocorticoids in alcohol dependence. Alcohol Res (2012) 34(4):468–83.
13. Dominguez G, Dagnas M, Decorte L, Vandesquille M, Belzung C, Béracochéa D, et al. Rescuing prefrontal cAMP–CREB pathway reverses working memory deficits during withdrawal from prolonged alcohol exposure. Brain Struct Funct (2016) 221(2):865–77. doi: 10.1007/s00429-014-0941-3
14. Little HJ, Croft AP, O'Callaghan MJ, Brooks SP, Wang G, Shaw SG. Selective increases in regional brain glucocorticoid: a novel effect of chronic alcohol. Neuroscience (2008) 156(4):1017–27. doi: 10.1016/j.neuroscience.2008.08.029
15. Primeaux SD, Wilson SP, Bray GA, York DA, Wilson MA. Overexpression of neuropeptide Y in the central nucleus of the amygdala decreases ethanol self-administration in “anxious” rats. Alcohol Clin Exp Res (2006) 30(5):791–801. doi: 10.1111/j.1530-0277.2006.00092.x
16. Staples MC, Mandyam CD. Thinking after drinking: impaired hippocampal-dependent cognition in human alcoholics and animal models of alcohol dependence. Front Psychiatry (2016) 7:162. doi: 10.3389/fpsyt.2016.00162
17. Stevenson JR, Schroeder JP, Nixon K, Besheer J, Crews FT, Hodge CW. Abstinence following alcohol drinking produces depression-like behavior and reduced hippocampal neurogenesis in mice. Neuropsychopharmacology (2009) 34(5):1209–22. doi: 10.1038/npp.2008.90
18. Fowler AK, Thompson J, Chen L, Dagda M, Dertien J, Dossou KS, et al. Differential sensitivity of prefrontal cortex and hippocampus to alcohol-induced toxicity. PLoS One (2014) 9(9):e106945. doi: 10.1371/journal.pone.0106945
19. Dominguez G, Belzung C, Pierard C, David V, Henkous N, Decorte L, et al. Alcohol withdrawal induces long-lasting spatial working memory impairments: relationship with changes in corticosterone response in the prefrontal cortex. Addict Biol (2017) 22(4):898–910. doi: 10.1111/adb.12371
20. Mons N, Beracochea D. Behavioral neuroadaptation to alcohol: from glucocorticoids to histone acetylation. Front Psychiatry (2016) 7:165. doi: 10.3389/fpsyt.2016.00165
21. Rose AK, Shaw SG, Prendergast MA, Little HJ. The importance of glucocorticoids in alcohol dependence and neurotoxicity. Alcohol Clin Exp Res (2010) 34(12):2011–8. doi: 10.1111/j.1530-0277.2010.01298.x
22. Jacquot C, Croft AP, Prendergast MA, Mulholland P, Shaw SG, Little HJ. Effects of the glucocorticoid antagonist, mifepristone, on the consequences of withdrawal from long term alcohol consumption. Alcohol Clin Exp Res (2008) 32(12):2107–16. doi: 10.1111/j.1530-0277.2008.00799.x
23. Kesner RP, Churchwell JC. An analysis of rat prefrontal cortex in mediating executive function. Neurobiol Learn Mem (2011) 96(3):417–31. doi: 10.1016/j.nlm.2011.07.002
24. Keedwell PA, Kumari V, Poon L, Marshall EJ, Checkley SA. Information processing deficits in withdrawing alcoholics. Addict Biol (2001) 6(3):239–45. doi: 10.1080/13556210120056571
25. Lee I, Kesner RP. Differential roles of dorsal hippocampal subregions in spatial working memory with short versus intermediate delay. Behav Neurosci (2003) 117(5):1044–53. doi: 10.1037/0735-7044.117.5.1044
26. Spellman T, Rigotti M, Ahmari SE, Fusi S, Gogos JA, Gordon JA. Hippocampal–prefrontal input supports spatial encoding in working memory. Nature (2015) 522(7556):309–14. doi: 10.1038/nature14445
27. Dalley JW, Cardinal RN, Robbins TW. Prefrontal executive and cognitive functions in rodents: neural and neurochemical substrates. Neurosci Biobehav Rev (2004) 28(7):771–84. doi: 10.1016/j.neubiorev.2004.09.006
28. Runyan JD, Dash PK. Distinct prefrontal molecular mechanisms for information storage lasting seconds versus minutes. Learn Mem (2005) 12(3):232–8. doi: 10.1101/lm.92405
29. Roozendaal B, McReynolds JR, McGaugh JL. The basolateral amygdala interacts with the medial prefrontal cortex in regulating glucocorticoid effects on working memory impairment. J Neurosci (2004) 24(6):1385–92. doi: 10.1523/JNEUROSCI.4664-03.2004
30. Davies E, MacKenzie SM. Extra-adrenal production of corticosteroids. Clin Exp Pharmacol Physiol (2003) 30(7):437–45. doi: 10.1046/j.1440-1681.2003.03867.x
31. Buhler M, Mann K. Alcohol and the human brain: a systematic review of different neuroimaging methods. Alcohol Clin Exp Res (2011) 35(10):1771–93. doi: 10.1111/j.1530-0277.2011.01540.x
32. Nöel X, Sferrazza R, Van Der Linden M, Paternot J, Verhas M, Hanak C, et al. Contribution of frontal cerebral blood flow measured by (99m)Tc-Bicisate spect and executive function deficits to predicting treatment outcome in alcohol-dependent patients. Alcohol Alcohol (2002) 37(4):347–54. doi: 10.1093/alcalc/37.4.347
33. Cerqueira JJ, Pêgo JM, Taipa R, Bessa JM, Almeida OF, Sousa N, et al. Morphological correlates of corticosteroid-induced changes in prefrontal cortex–dependent behaviors. J Neurosci (2005) 25(34):7792–800. doi: 10.1523/JNEUROSCI.1598-05.2005
34. Moselhy HF, Georgiou G, Kahn A. Frontal lobe changes in alcoholism: a review of the literature. Alcohol Alcohol (2001) 36(5):357–68. doi: 10.1093/alcalc/36.5.357
35. Mizoguchi K, Ishige A, Takeda S, Aburada M, Tabira T. Endogenous glucocorticoids are essential for maintaining prefrontal cortical cognitive function. J Neurosci (2004) 24(24):5492–9. doi: 10.1523/JNEUROSCI.0086-04.2004
36. León-Carrión J, Atutxa AM, Mangas MA, Soto-Moreno A, Pumar A, Leon-Justel A, et al. A clinical profile of memory impairment in humans due to endogenous glucocorticoid excess. Clin Endocrinol (Oxf) (2009) 70(2):192–200. doi: 10.1111/j.1365-2265.2008.03355.x
37. Starkman MN, Giordani B, Berent S, Schork MA, Schteingart DE. Elevated cortisol levels in Cushing’s disease are associated with cognitive decrements. Psychosom Med (2001) 63(6):985–93. doi: 10.1097/00006842-200111000-00018
38. Terfehr K, Wolf OT, Schlosser N, Fernando SC, Otte C, Muhtz C, et al. Hydrocortisone impairs working memory in healthy humans, but not in patients with major depressive disorder. Psychopharmacology (Berl) (2011) 215(1):71–9. doi: 10.1007/s00213-010-2117-z
39. Vaz LJ, Pradella-Hallinan M, Bueno OF, Pompéia S. Acute glucocorticoid effects on the multicomponent model of working memory. Hum Psychopharmacol (2011) 26(7):477–87. doi: 10.1002/hup.1230
40. Reul JM, de Kloet ER. Two receptor systems for corticosterone in rat brain: microdistribution and differential occupation. Endocrinology. (1985) 117 (6):2505–11. doi: 10.1210/endo-117-6-2505
41. Greenwood M, Greenwood MP, Mecawi AS, Loh SY, Rodrigues JA, Paton JFR, et al. Transcription factor CREB3L1 mediates cAMP and glucocorticoid regulation of arginine vasopressin gene transcription in the rat hypothalamus. Mol Brain (2015) 8(1):68. doi: 10.1186/s13041-015-0159-1
42. Luca F, Maranville JC, Richards AL, Witonsky DB, Stephens M, Di Rienzo A, et al. Genetic, functional and molecular features of glucocorticoid receptor binding. PLoS One (2013) 8(4):e61654. doi: 10.1371/journal.pone.0061654
43. Meijsing SH. Mechanisms of glucocorticoid-regulated gene transcription. Adv Exp Med Biol (2015) 872:59–81. doi: 10.1007/978-1-4939-2895-8_3
44. Vendruscolo LF, Barbier E, Schlosburg JE, Misra KK, Whitfield TW Jr, Logrip ML, et al. Corticosteroid-dependent plasticity mediates compulsive alcohol drinking in rats. J Neurosci (2012) 32(22):7563–71. doi: 10.1523/JNEUROSCI.0069-12.2012
45. Koenig HN, Olive MF. The glucocorticoid receptor antagonist mifepristone reduces ethanol intake in rats under limited access conditions. Psychoneuroendocrinology (2004) 29(8):999–1003. doi: 10.1016/j.psyneuen.2003.09.004
46. Lee I, Kesner RP. Time-dependent relationship between the dorsal hippocampus and the prefrontal cortex in spatial memory. J Neurosci (2003) 23(4):1517–23. doi: 10.1523/JNEUROSCI.23-04-01517.2003
47. Wan RQ, Pang K, Olton DS. Hippocampal and amygdaloid involvement in nonspatial and spatial working memory in rats: effects of delay and interference. Behav Neurosci (1994) 108(5):866–82. doi: 10.1037//0735-7044.108.5.866
48. Barsegyan A, Mackenzie SM, Kurose BD, McGaugh JL, Roozendaal B. Glucocorticoids in the prefrontal cortex enhance memory consolidation and impair working memory by a common neural mechanism. Proc Natl Acad Sci U S A (2010) 107(38):16655–60. doi: 10.1073/pnas.1011975107
49. Roozendaal B, Quirarte GL, McGaugh JL. Glucocorticoids interact with the basolateral amygdala beta-adrenoceptor—cAMP/cAMP/PKA system in influencing memory consolidation. Eur J Neurosci (2002) 15(3):553–60. doi: 10.1046/j.0953-816x.2001.01876.x
50. Shansky RM, Bender G, Arnsten AF. Estrogen prevents norepinephrine alpha-2a receptor reversal of stress-induced working memory impairment. Stress (2009) 12(5):457–63. doi: 10.1080/10253890802520988
51. Grossman C, Ruhs S, Seiferth A, Gekle M. Interaction between mineralocorticoid receptor and cAMP/CREB signaling. Steroids (2010) 75(8-9):539–43. doi: 10.1016/j.steroids.2009.10.006
52. Grossmann C, Wuttke M, Ruhs S, Seiferth A, Mildenberger S, Rabe S, et al. Mineralocorticoid receptor inhibits CREB signaling by calcineurin activation. FASEB J (2010) 24(6):2010–9. doi: 10.1016/j.steroids.2009.10.006
53. Pandey SC. Anxiety and alcohol abuse disorders: a common role for CREB and its target, the neuropeptide Y gene. Trends Pharmacol Sci (2003) 24(9):456–60. doi: 10.1016/S0165-6147(03)00226-8
54. Pandey SC, Chartoff EH, Carlezon WA Jr, Zou J, Zhang H, Kreibich AS, et al. CREB gene transcription factors: role in molecular mechanisms of alcohol and drug addiction. Alcohol Clin Exp Res (2005) 29(2):176–84. doi: 10.1097/01.ALC.0000153550.31168.1D
55. Pandey SC, Mittal N, Lumeng L, Li TK. Involvement of the cyclic AMP–responsive element binding protein gene transcription factor in genetic preference for alcohol drinking behavior. Alcohol Clin Exp Res (1999) 23(9):1425–34. doi: 10.1111/j.1530-0277.1999.tb04663.x
56. Pandey SC, Roy A, Mittal N. Effects of chronic ethanol intake and its withdrawal on the expression and phosphorylation of the creb gene transcription factor in rat cortex. J Pharmacol Exp Ther (2001) 296(3):857–68. doi: 10.1046/j.1471-4159.2001.00309.x
57. Bazov I, Sarkisyan D, Kononenko O, Watanabe H, Karpyak VM, Yakovleva T, et al. Downregulation of the neuronal opioid gene expression concomitantly with neuronal decline in dorsolateral prefrontal cortex of human alcoholics. Transl Psychiatry (2018) 8(1):122. doi: 10.1038/s41398-017-0075-5
58. Yuen EY, Liu W, Karatsoreos IN, Feng J, McEwen BS, Yan Z. Acute stress enhances glutamatergic transmission in prefrontal cortex and facilitates working memory. Proc Natl Acad Sci U S A (2009) 106(33):14075–9. doi: 10.1073/pnas.0906791106
59. Butts KA, Weinberg J, Young AH, Phillips AG. Glucocorticoid receptors in the prefrontal cortex regulate stress-evoked dopamine efflux and aspects of executive function. Proc Natl Acad Sci U S A (2011) 108(45):18459–64. doi: 10.1073/pnas.1111746108
60. Arriza JL, Weinberger C, Cerelli G, Glaser TM, Handelin BL, Housman DE, et al. Cloning of human mineralocorticoid receptor complementary DNA: structural and functional kinship with the glucocorticoid receptor. Science (1987) 237(4812):268–75. doi: 10.1126/science.3037703
61. Arriza JL, Simerly RB, Swanson LW, Evans RM. The neuronal mineralocorticoid receptor as a mediator of glucocorticoid response. Neuron (1988) 1(9):887–900. doi: 10.1016/0896-6273(88)90136-5
62. Reul JM, van den Bosch FR, de Kloet ER. Relative occupation of type-I and type-II corticosteroid receptors in rat brain following stress and dexamethasone treatment: functional implications. J Endocrinol (1987) 115(3):459–67. doi: 10.1677/joe.0.1150459
63. Seckl JR, Dickson KL, Yates C, Fink G. Distribution of glucocorticoid and mineralocorticoid receptor messenger RNA expression in human postmortem hippocampus. Brain Res (1991) 561(2):332–7. doi: 10.1016/0006-8993(91)91612-5
64. Joels M, De Kloet ER. Coordinative mineralocorticoid and glucocorticoid receptor–mediated control of responses to serotonin in rat hippocampus. Neuroendocrinology (1992) 55(3):344–50. doi: 10.1159/000126135
65. Fuxe K, Cintra A, Härfstrand A, Agnati LF, Kalia M, Zoli M, et al. Central glucocorticoid receptor immunoreactive neurons: new insights into the endocrine regulation of the brain. Ann N Y Acad Sci (1987) 512:362–93. doi: 10.1111/j.1749-6632.1987.tb24974.x
66. Cintra A, Bhatnagar M, Chadi G, Tinner B, Lindberg J, Gustafsson JA, et al. Glial and neuronal glucocorticoid receptor immunoreactive cell populations in developing, adult, and aging brain. Ann N Y Acad Sci (1994) 746:42–61. doi: 10.1111/j.1749-6632.1994.tb39210.x
67. Patel PD, Lopez JF, Lyons DM, Burke S, Wallace M, Schatzberg AF. Glucocorticoid and mineralocorticoid receptor mRNA expression in squirrel monkey brain. J Psychiatr Res (2000) 34(6):383–92. doi: 10.1016/S0022-3956(00)00035-2
68. Starkman MN. Neuropsychiatric findings in Cushing syndrome and exogenous glucocorticoid administration. Endocrinol Metab Clin North Am (2013) 42(3):47788. doi: 10.1016/j.ecl.2013.05.010
69. Lupien SJ, de Leon M, de Santi S, Convit A, Tarshish C, Nair NP, et al. Cortisol levels during human aging predict hippocampal atrophy and memory deficits. Nat Neurosci (1998) 1(1):69–73. doi: 10.1038/271
70. Patil CG, Prevedello DM, Lad SP, Vance ML, Thorner MO, Katznelson L, et al. Late recurrences of Cushing’s disease after initial successful transsphenoidal surgery. J Clin Endocrinol Metab (2008) 93(2):358–62.
71. O’Daly OG, Trick L, Scaife J, Marshall J, Ball D, Phillips ML, et al. Withdrawal-associated increases and decreases in functional neural connectivity associated with altered emotional regulation in alcoholism. Neuropsychopharmacology (2012) 37(10):2267–76. doi: 10.1038/npp.2012.77
72. Pfefferbaum A, Rosenbloom M, Deshmukh A, Sullivan E. Sex differences in the effects of alcohol on brain structure. Am J Psychiatry (2001) 158(2):188–97. doi: 10.1176/appi.ajp.158.2.188
73. Le Berre AP, Fama R, Sullivan EV. Executive functions, memory, and social cognitive deficits and recovery in chronic alcoholism: a critical review to inform future research. Alcohol Clin Exp Res (2017) 41(8):1432–43. doi: 10.1111/acer.13431
74. Pitel AL, Rivier J, Beaunieux H, Vabret F, Desgranges B, Eustache F. Changes in the episodic memory and executive functions of abstinent and relapsed alcoholics over a 6-month period. Alcohol Clin Exp Res (2009) 33(3):490–8. doi: 10.1111/j.1530-0277.2008.00859.x
75. Bazov I, Sarkisyan D, Kononenko O, Watanabe H, Yakovleva T, Hansson AC, et al. Dynorphin and kappa-opioid receptor dysregulation in the dopaminergic reward system of human alcoholics. Mol Neurobiol (2018) 55(8):7049–61. doi: 10.1007/s12035-017-0844-4
76. Blum K, Oscar-Berman M, Badgaiyan R, Braverman ER, Gold MS. Hypothesizing darkness induced alcohol intake linked to dopaminergic regulation of brain function. Psychology (Irvine) (2014) 5(4):282288.
77. Patil CG, Lad SP, Katznelson L, Laws ER Jr. Brain atrophy and cognitive deficits in Cushing’s disease. Neurosurg Focus (2007) 23(3):E11. doi: 10.3171/FOC-07/09/E11
78. Somkuwar SS, Vendruscolo LF, Fannon MJ, Schmeichel BE, Nguyen TB, Guevara J, et al. Abstinence from prolonged ethanol exposure affects plasma corticosterone, glucocorticoid receptor signaling and stress-related behaviors. Psychoneuroendocrinology (2017) 84:17–31. doi: 10.1016/j.psyneuen.2017.06.006
79. Brooks SP, Croft AP, Norman G, Shaw SG, Little HJ. Nimodipine prior to alcohol withdrawal prevents memory deficits during the abstinence phase. Neuroscience (2008) 157(2):376–84. doi: 10.1016/j.neuroscience.2008.09.010
80. Schacht JP, Randall PK, Waid LR, Baros AM, Latham PK, Wright TM, et al. Neurocognitive performance, alcohol withdrawal, and effects of a combination of flumazenil and gabapentin in alcohol dependence. Alcohol Clin Exp Res (2011) 35(11):2030–8. doi: 10.1111/j.1530-0277.2011.01554.x
81. Stavro K, Pelletier J, Potvin S. Widespread and sustained cognitive deficits in alcoholism: a meta-analysis. Addict Biol (2013) 18(2):203–13. doi: 10.1111/j.1369-1600.2011.00418.x
82. Oscar-Berman M, Valmas MM, Sawyer KS, Ruiz SM, Luhar RB, Gravitz ZR. Profiles of impaired, spared, and recovered neuropsychologic processes in alcoholism. Handb Clin Neurol (2014) 125:183–210. doi: 10.1016/B978-0-444-62619-6.00012-4
83. Noel X, Van der Linden M, Brevers D, Campanella S, Hanak C, Kornreich C, et al. The contribution of executive functions deficits to impaired episodic memory in individuals with alcoholism. Psychiatry Res (2012) 198(1):116–22. doi: 10.1016/j.psychres.2011.10.007
84. Pitel AL, Beaunieux H, Witkowski T, Vabret F, Guillery-Girard B, Quinette P, et al. Genuine episodic memory deficits and executive dysfunctions in alcoholic subjects early in abstinence. Alcohol Clin Exp Res (2007) 31(7):1169–78. doi: 10.1111/j.1530-0277.2007.00418.x
85. McEwen BS. The brain on stress: toward an integrative approach to brain, body, and behavior. Perspect Psychol Sci (2013) 8(6):673–5. doi: 10.1177/1745691613506907
86. Diorio D, Viau V, Meaney MJ. The role of the medial prefrontal cortex (cingulate gyrus) in the regulation of hypothalamic–pituitary–adrenal responses to stress. J Neurosci (1993) 13(9):3839–47. doi: 10.1523/JNEUROSCI.13-09-03839.1993
87. Loeber S, Duka T, Welzel Márquez H, Nakovics H, Heinz A, Mann K, et al. Effects of repeated withdrawal from alcohol on recovery of cognitive impairment under abstinence and rate of relapse. Alcohol Alcohol (2010) 45(6):541–7. doi: 10.1093/alcalc/agq065
88. Arvat E, Giordano R, Grottoli S, Ghigo E. Benzodiazepines and anterior pituitary function. J Endocrinol Invest (2002) 25(8):735–47. doi: 10.1007/BF03345110
89. Béracochéa D, Tronche C, Coutan M, Dorey R, Chauveau F, Piérard C. Interaction between diazepam and hippocampal corticosterone after acute stress: impact on memory in middle-aged mice. Front Behav Neurosci (2011) 5:14. doi: 10.3389/fnbeh.2011.00014
90. Calogero AE, Gallucci WT, Chrousos GP, Gold PW. Interaction between GABAergic neurotransmission and rat hypothalamic corticotropin-releasing hormone secretion in vitro. Brain Res (1988) 463(1):28–36. doi: 10.1016/0006-8993(88)90523-9
91. Cullinan WE, Ziegler DR, Herman JP. Functional role of local GABAergic influences on the HPA axis. Brain Struct Funct (2008) 213(1–2):63–72. doi: 10.1007/s00429-008-0192-2
92. Levy BH, Tasker JG. Synaptic regulation of the hypothalamic–pituitary–adrenal axis and its modulation by glucocorticoids and stress. Front Cell Neurosci (2012) 6:24. doi: 10.3389/fncel.2012.00024
93. Rouhani S, Dall’Ava-Santucci J, Bajenaru O, Emmanouilidis E, Tran G, Manicom R, et al. Effects of muscimol or homotaurine on sleep–wake states in alcohol-dependent rats during withdrawal. Pharmacol Biochem Behav (1998) 59(4):955–60. doi: 10.1016/S0091-3057(97)00521-2
94. Weintraub SJ. Diazepam in the treatment of moderate to severe alcohol withdrawal. CNS Drugs (2017) 31(2):87–95. doi: 10.1007/s40263-016-0403-y
95. Addolorato G, Armuzzi A, Gasbarrini G, De Lorenzi G, Ancona C, Abenavoli L, et al. Pharmacological approaches to the management of alcohol addiction. Eur Rev Med Pharmacol Sci (2002) 6(5):89–97.
96. Adinoff B. Double-blind study of alprazolam, diazepam, clonidine, and placebo in the alcohol withdrawal syndrome: preliminary findings. Alcohol Clin Exp Res (1994) 18(4):873–8. doi: 10.1111/j.1530-0277.1994.tb00053.x
97. Agabio R, Sinclair JM, Addolorato G, Aubin HJ, Beraha EM, Caputo F, et al. Baclofen for the treatment of alcohol use disorder: the Cagliari Statement. Lancet Psychiatry (2018) 5(12):957–60. doi: 10.1016/S2215-0366(18)30433-4
98. Lejoyeux M, Solomon J, Ades J. Benzodiazepine treatment for alcohol-dependent patients. Alcohol Alcohol (1998) 33(6):563–75. doi: 10.1093/alcalc/33.6.563
99. Liang J, Olsen RW. Alcohol use disorders and current pharmacological therapies: the role of GABA(A) receptors. Acta Pharmacol Sin (2014) 35(8):981–93. doi: 10.1038/aps.2014.50
100. Ross HE. Benzodiazepine use and anxiolytic abuse and dependence in treated alcoholics. Addiction (1993) 88(2):209–18. doi: 10.1111/j.1360-0443.1993.tb00804.x
101. Nelson LS. Alcohol withdrawal and flumazenil: not for the faint of heart. J Med Toxicol (2014) 10(2):123–5. doi: 10.1007/s13181-014-0392-5
102. Coull JT, Frith CD, Dolan RJ. Dissociating neuromodulatory effects of diazepam on episodic memory encoding and executive function. Psychopharmacology (Berl) (1999) 145(2):213–22. doi: 10.1007/s002130051051
103. Coull JT, Middleton HC, Robbins TW, Sahakian BJ. Contrasting effects of clonidine and diazepam on tests of working memory and planning. Psychopharmacology (Berl) (1995) 120(3):311–21. doi: 10.1007/BF02311179
104. Lister RG. The amnesic action of benzodiazepines in man. Neurosci Biobehav Rev (1985) 9(1):87–94. doi: 10.1016/0149-7634(85)90034-X
105. Borde N, Jaffard R, Beracochea D. Effects of chronic alcohol consumption or diazepam administration on item recognition and temporal ordering in a spatial working memory task in mice. Eur J Neurosci (1998) 10(7):2380–7. doi: 10.1046/j.1460-9568.1998.00261.x
106. Grimm VE, Hershkowitz M. The effect of chronic diazepam treatment on discrimination performance and 3H-flunitrazepam binding in the brains of shocked and nonshocked rats. Psychopharmacology (Berl) (1981) 74(2):132–6. doi: 10.1007/BF00432679
107. Schmitz HP. The global health network on alcohol control: successes and limits of evidence-based advocacy. Health Policy Plan (2016) 31 Suppl 1:i87–97. doi: 10.1093/heapol/czu064
108. David V, Gold LH, Koob GF, Cazala P. Anxiogenic-like effects limit rewarding effects of cocaine in balb/cbyj mice. Neuropsychopharmacology (2001) 24(3):300–18. doi: 10.1016/S0893-133X(00)00205-0
109. George O, Sanders C, Freiling J, Grigoryan E, Vu S, Allen CD, et al. Recruitment of medial prefrontal cortex neurons during alcohol withdrawal predicts cognitive impairment and excessive alcohol drinking. Proc Natl Acad Sci U S A (2012) 109(44):18156–61. doi: 10.1073/pnas.1116523109
110. Bianchi PC, Carneiro de Oliveira PE, Palombo P, Leão RM, Cogo-Moreira H, Planeta CDS, et al. Functional inactivation of the orbitofrontal cortex disrupts context-induced reinstatement of alcohol seeking in rats. Drug Alcohol Depend (2018) 186:102–12. doi: 10.1016/j.drugalcdep.2017.12.045
111. Sachdeva A, Choudhary M, Chandra M. Alcohol withdrawal syndrome: benzodiazepines and beyond. J Clin Diagn Res (2015) 9(9):VE01–7. doi: 10.7860/JCDR/2015/13407.6538
112. Hudgson P, Weightman D. Baclofen in the treatment of spasticity. Br Med J (1971) 4(5778):15–7. doi: 10.1136/bmj.4.5778.15
113. Hausler A, Monnet G, Peter O. Involvement of GABAB receptors in the regulation of the hypothalamo–pituitary–adrenocortical (HPA) axis in rats. J Steroid Biochem Mol Biol (1993) 46(6):767–71. doi: 10.1016/0960-0760(93)90317-P
114. Geisel O, Schlemm L, Hellweg R, Wiedemann K, Müller CA. Hypothalamic–pituitary–adrenocortical axis activity in alcohol-dependent patients during treatment with high-dose baclofen. Pharmacopsychiatry (2019) 52(1):32–7. doi: 10.1055/s-0043-124189
115. Agabio R, Colombo G. GABAB receptor ligands for the treatment of alcohol use disorder: preclinical and clinical evidence. Front Neurosci (2014) 8:140. doi: 10.3389/fnins.2014.00140
116. de Beaurepaire R. The use of very high-doses of baclofen for the treatment of alcohol-dependence: a case series. Front Psychiatry (2014) 5:143. doi: 10.3389/fpsyt.2014.00143
117. Ameisen O. Complete and prolonged suppression of symptoms and consequences of alcohol-dependence using high-dose baclofen: a self-case report of a physician. Alcohol Alcohol (2005) 40(2):147–50. doi: 10.1093/alcalc/agh130
118. Colombo G, Serra S, Brunetti G, Atzori G, Pani M, Vacca G. The GABA(B) receptor agonists baclofen and CGP 44532 prevent acquisition of alcohol drinking behaviour in alcohol-preferring rats. Alcohol Alcohol (2002) 37(5):499–503. doi: 10.1093/alcalc/37.5.499
119. Farokhnia M, Deschaine SL, Sadighi A, Farinelli LA, Lee MR, Akhlaghi F, et al. A deeper insight into how GABA-B receptor agonism via baclofen may affect alcohol seeking and consumption: lessons learned from a human laboratory investigation. Mol Psychiatry (2018). doi: 10.1038/s41380-018-0287-y
120. Maccioni P, Colombo G. Reduction of alcohol’s reinforcing and motivational properties by the positive allosteric modulator of the GABA(B) receptor, BHF177, in alcohol-preferring rats. Alcohol Clin Exp Res (2009) 33(10):1749–56. doi: 10.1111/j.1530-0277.2009.01012.x
121. Maccioni P, Colombo G. Role of the GABA(B) receptor in alcohol-seeking and drinking behavior. Alcohol (2009) 43(7):555–8. doi: 10.1016/j.alcohol.2009.09.030
122. Maccioni P, Lorrai I, Contini A, Leite-Morris K, Colombo G. Microinjection of baclofen and CGP7930 into the ventral tegmental area suppresses alcohol self-administration in alcohol-preferring rats. Neuropharmacology (2018) 136(Pt A):146–58. doi: 10.1016/j.neuropharm.2017.10.012
123. Thompson A, Owens L, Richardson P, Pirmohamed M. Systematic review: baclofen dosing protocols for alcohol use disorders used in observational studies. Eur Neuropsychopharmacol (2017) 27(11):1077–89. doi: 10.1016/j.euroneuro.2017.08.434
124. Addolorato G, Leggio L, Abenavoli L, Agabio R, Caputo F, Capristo E, et al. Baclofen in the treatment of alcohol withdrawal syndrome: a comparative study vs diazepam. Am J Med (2006) 119(3):276 e13–8. doi: 10.1016/j.amjmed.2005.08.042
125. Liu J, Wang LN. Baclofen for alcohol withdrawal. Cochrane Database Syst Rev (2017) 8:CD008502. doi: 10.1002/14651858.CD008502.pub5
126. Girish K, Vikram Reddy K, Pandit LV, Pundarikaksha HP, Vijendra R, Vasundara K, et al. A randomized, open-label, standard controlled, parallel group study of efficacy and safety of baclofen, and chlordiazepoxide in uncomplicated alcohol withdrawal syndrome. Biomed J (2016) 39(1):72–80. doi: 10.1016/j.bj.2015.09.002
127. Lyon JE, Khan RA, Gessert CE, Larson PM, Renier CM. Treating alcohol withdrawal with oral baclofen: a randomized, double-blind, placebo-controlled trial. J Hosp Med (2011) 6(8):469–74. doi: 10.1002/jhm.928
128. Dominguez G, Henkous N, Pierard C, Belzung C, Mons N, Beracochea D. Repeated diazepam administration reversed working memory impairments and glucocorticoid alterations in the prefrontal cortex after short but not long alcohol-withdrawal periods. Cogn Affect Behav Neurosci (2018) 18(4):665679. doi: 10.3758/s13415-018-0595-3
129. Cagetti E, Liang J, Spigelman I, Olsen RW. Withdrawal from chronic intermittent ethanol treatment changes subunit composition, reduces synaptic function, and decreases behavioral responses to positive allosteric modulators of GABAA receptors. Mol Pharmacol (2003) 63(1):53–64. doi: 10.1124/mol.63.1.53
130. Devaud LL, Fritschy JM, Sieghart W, Morrow AL. Bidirectional alterations of GABA(A) receptor subunit peptide levels in rat cortex during chronic ethanol consumption and withdrawal. J Neurochem (1997) 69(1):126–30. doi: 10.1046/j.1471-4159.1997.69010126.x
131. Auta J, Gatta E, Davis JM, Pandey SC, Guidotti A. Potential role for histone deacetylation in chronic diazepam-induced downregulation of alpha1-GABAA receptor subunit expression. Pharmacol Res Perspect (2018) 6(4):e00416. doi: 10.1002/prp2.416
132. Rabat Y, Henkous N, Corio M, Nogues X, Beracochea D. Baclofen but not diazepam alleviates alcohol-seeking behavior and hypothalamic–pituitary–adrenal axis dysfunction in stressed withdrawn mice. Front Psychiatry (2019) 10:238. doi: 10.3389/fpsyt.2019.00238
Keywords: alcohol withdrawal and relapse, benzodiazepines, baclofen, corticosterone, gaba receptors, glucocorticoids, prefrontal cortex, working memory
Citation: Béracochéa D, Mons N and David V (2019) Targeting the Glucocorticoid Receptors During Alcohol Withdrawal to Reduce Protracted Neurocognitive Disorders. Front. Psychiatry 10:580. doi: 10.3389/fpsyt.2019.00580
Received: 21 December 2018; Accepted: 23 July 2019;
Published: 18 September 2019.
Edited by:
Renaud de Beaurepaire, Groupe hospitalier Paul Guiraud (GHPG), FranceReviewed by:
Verica Milivojevic, Yale University, United StatesGabriel Rubio, University Hospital October 12, Spain
Copyright © 2019 Béracochéa, Mons and David. This is an open-access article distributed under the terms of the Creative Commons Attribution License (CC BY). The use, distribution or reproduction in other forums is permitted, provided the original author(s) and the copyright owner(s) are credited and that the original publication in this journal is cited, in accordance with accepted academic practice. No use, distribution or reproduction is permitted which does not comply with these terms.
*Correspondence: Daniel Béracochéa, ZGFuaWVsLmJlcmFjb2NoZWFAdS1ib3JkZWF1eC5mcg==; Vincent David, dmluY2VudC5kYXZpZEB1LWJvcmRlYXV4LmZy