- 1Department of Psychiatry, The Second Xiangya Hospital, Central South University, Changsha, China
- 2Critical Care Laboratory, Critical Care Directorate, Manchester Royal Infirmary, Manchester University NHS Foundation Trust, Manchester, United Kingdom
- 3School of Healthcare Science, Faculty of Science and Engineering, Manchester Metropolitan University, Manchester, United Kingdom
- 4School of Medicine, Medical Sciences and Nutrition, Institute of Medical Sciences, University of Aberdeen, Aberdeen, United Kingdom
Though much progress has been made in recent years towards understanding the function and physiology of primary cilia, they remain a somewhat elusive organelle. Some studies have explored the role of primary cilia in the developing nervous system, and their dysfunction has been linked with several neurosensory deficits. Yet, very little has been written on their potential role in psychiatric disorders. This article provides an overview of some of the functions of primary cilia in signalling pathways, and demonstrates that they are a worthy candidate in psychiatric research. The links between primary cilia and major mental illness have been demonstrated to exist at several levels, spanning genetics, signalling pathways, and pharmacology as well as cell division and migration. The primary focus of this review is on the sensory role of the primary cilium and the neurodevelopmental hypothesis of psychiatric disease. As such, the primary cilium is demonstrated to be a key link between the cellular environment and cell behaviour, and hence of key importance in the considerations of the nature and nurture debate in psychiatric research.
Introduction
Recently Muñoz-Estrada et al. (1) published results from experiments on olfactory neuronal precursor cells obtained from human sufferers of schizophrenia (SCZ) and bipolar disorder (BD), linking primary cilia (PC) with major mental illness (MMI). Their work showed a general decrease in the percentage of cells with PC in subjects suffering from MMI. Furthermore, in vitro supplementation with lithium (a common pharmacotherapy for BD, mania and depression (2), and previously shown (3) to cause in vivo and in vitro PC elongation in mouse neuronal cells) was shown to have a positive effect on PC length. While their study (1) was conducted on samples obtained from a very limited number of patients suffering from a variety of MMI and on different treatment regimes, it highlights an area of psychiatric research that has been largely ignored.
PC are cellular protrusions originating from the centrosome's mother centriole, and are present on most mammalian cells (4, 5). Since they are linked with the centrosome, they need to be disassembled or retracted whenever the centrosome needs to perform its microtubule organizing centre functions, such as during cell division and migration (4, 6–9), making the exact role of the PC in these processes somewhat unclear. PC are largely regarded as cellular sensory antennae and signalling hubs, facilitating key developmental pathways such as Sonic Hedgehog (SHH) and WNT signalling (10–12). More recently, proposals have been made that PC have an extracellular signalling role, as thanks to its biochemical autonomy from the rest of the cell membrane the cilium can express distinct proteins on its membrane as well as have a different concentration of various factors in its cytoplasm (13, 14). As such ciliary vesicles, can form distinct exosomic parcels, but their role, especially in mammals, is not yet clear, and arguments have been made that their primary function is to dispose of redundant ciliary content (13, 14). This article reviews various levels of evidence for the role of PC in MMI, focusing on the well-established developmental hypothesis of MMI (15, 16). PC play an important role in the development of the central nervous system (CNS) and in a wide variety of roles in the adult brain (10, 17). PC have been even called “neurons little helpers” in the context of neurodevelopment (18), and have recently been discussed in the context of the neuronal migration hypothesis of dyslexia (19). Nevertheless, as discussed later, PC retract during some cellular events, and might not be present on all types of neurons during early development (8, 20). The following sections will show why this elusive organelle should be considered an attractive target for psychiatric research.
Genetics
Genomic and bioinformatics research has revealed that some PC genes are linked to MMI. Of course, the mere fact that a gene might be linked to both PC and MMI does not guarantee the involvement of PC in MMI, as proteins might function in different cellular compartments and in different cellular processes. Similarly, a different severity of mutation might be required to cause an effect on the PC or to precipitate MMI. Nevertheless, the fact that data from an RNA interference study looking at 41 MMI genes found that 23 affect cilia length (21) should at least prompt one to look at the correlation between PC and MMI genes. Here, we present evidence for two candidate genes which may potentially connect PC with MMI.
Previously (22), researchers described a region on chromosome 4p linked to MMI. One gene found in that region was CC2D2A (23), also known as MKS6 and JBTS9. It is involved in ciliogenesis (24), and in vesicles trafficking in the PC's transition zone (the region of the cilium that regulates the trafficking of proteins between the cilium and the rest of the cell, allowing the cilium to retain its distinct protein composition), and has been implicated in neural tube development and Sonic Hedgehog signalling (25–27). CC2D2A has been linked to a range of CNS developmental conditions linked to PC: Joubert syndrome (28–30), Meckel syndrome (31) and mental retardation (32, 33); there is also a potential link with Bardet-Biedl syndrome (BBS) (29). CC2D2A's link to MMI has not yet been thoroughly investigated (34), but MMI problems have been observed in individuals with Joubert syndrome, and AHI, also associated with Joubert syndrome, has been proposed as a marker for SCZ (35).
Disc1, a gene involved in the formation and regulation of cilia (36), has also been associated with MMI (37, 38). DISC1 associates with a variety of centrosomal components (39, 40), recruiting BBS proteins to the centrosome (41, 42), and acting as a switch between the processes of neuronal migration and proliferation. DISC1 also interacts with the dynein complex (43), which, together with the Intraflagellar Transport (IFT) complex A, is vital for retrograde transport within the PC (12). Moreover, one reported zebrafish DISC1 aberration caused a decrease in β-catenin levels (44) correlating to a decrease in canonical WNT activity.
As such, DISC1 is perhaps one of the strongest links between PC and MMI.
Neurodevelopment
PC associated CNS defects range from cerebellar hypoplasia through mental retardation to encephalocele and enlarged ventricles (45–47). Moreover, various neurodevelopmental defects have been associated with MMI, for which there is evidence of PC involvement. This is unsurprising, as PC are present from the earliest stages of CNS development through to the mature brain (17, 48, 49). The centrosome, with which PC are closely interlinked, is also a key player in CNS development (50, 51).
Defective neuronal migration has been reported in several studies relating to MMI, and is likely to contribute to reductions of grey matter in patients affected by MMI (15, 52–56). Additionally, BBS has been associated with cortical volume reductions in both a human and mouse study (57, 58). Molecular links between psychiatric pathways and PC, such as the interaction of DISC1, WNT signalling, the BBS complex and the centrosome exist in the context of cell migration and proliferation (41–43, 59, 60). For example, PC have been shown to be involved in several aspects of neuronal migration like radial glial scaffold formation and interneuron migration (8, 61) as well as galvanotaxic migration (62–64).
PC factors also influence important migratory processes of microtubule (65–67) and actin (68, 69) organisation. Further, CDC42, a molecule important for ciliary initiation (70–72) promotes actin skeleton remodelling (73) and cell polarity (74, 75) through non-canonical WNT signalling (51). Furthermore, CDC42 and actin skeleton remodelling have been associated with deficits in dendritic spine formation frequently reported in SCZ and BD (76–78). Issues relating to neuronal network health, such as synaptic connectivity and neurite number have also been highlighted in both mental illness and PC dysfunction (53, 54, 79–85).
Problems with neuronal differentiation have been associated with SCZ, and can result from DISC1 related changes in WNT signalling (86–88). Asymmetric PC membrane inheritance occurs during neocortical development, and is linked with the inheritance of the centrosome, which is important for proper neurogenesis (89–91), suggesting that PC function might be important for cell division and fate specification, further contributing to the aforementioned changes in cortical volume (57, 58).
Moreover, PC are known to be involved in other developmental aspects that could contribute to defective neurogenesis and CNS cell migration, often involving PC's close association with the centrosome and the Golgi apparatus. These involve SHH (92–94) and Platelet Derived Growth Factor (95–98) signalling, and governance of cell migration (4, 8, 9, 50, 63, 99–102) and cell division (95–97, 103–110) through sensing extracellular cues. This last point is exemplified by the fact that serum withdrawal during cell culture is a ciliogenic condition (97, 103, 105, 109), indicating that cells may use their cilia to ensure that the extracellular conditions are right for mitosis initiation.
WNT Signalling
The WNT pathway is one of the best studied MMI signalling pathways (111–114). Those affected by BD and SCZ have been found to express mRNA levels suggestive of attenuated canonical WNT signalling and enhanced non-canonical signalling, particularly the WNT/Ca2+ pathway (111); although a recent human cerebral organoid study showed an increase in canonical WNT signalling in the early developmental stages of brains with disrupted DISC1, suggesting that WNT changes might be context/age dependent (114). The changes in mRNA expression levels is a noteworthy finding since PC are known to facilitate the switch from canonical to non-canonical WNT signalling via Ca2+ signalling (103). PC modulates WNT signalling via the degradation of Dishevelled by Inversin at the basal body (51, 115, 116), repressing the canonical signalling pathway (117, 118) and promoting the planar cell polarity pathway (95). Curiously, different ciliary gene mutations perturb WNT signalling in different ways, with mutations in some genes being able to both increase and decrease β-catenin levels (119).
Moreover, WNT signalling affects motile cilia, and might affect PC by influencing basal body positioning on the apical membrane (51, 120–122). The importance of such an overlap and interaction between motile and primary cilia has been highlighted in hydrocephalus (96), where defects in both motile and primary cilia are known to be present (96, 123). Hydrocephalus-like changes have similarly been reported in SCZ (124). Motile cilia generate fluid flow, to which PC respond (125), which is crucial for establishing body asymmetry (126), and is detected by the polycystin receptors PC1 and PC2 (127–129), which facilitate Ca2+ entry (127). This flow-induced calcium signalling not only facilitates the switch from canonical to non-canonical WNT signalling (103) but also regulates the cell cycle (95), although recent experiments have started questioning whether flow sensing happens via Ca2+ signalling (130).
Nevertheless, there is some evidence disputing PC's role in WNT signalling (11, 119). There is evidence from both zebrafish and mice showing that disrupting PC does not affect WNT signalling (131–133). The role of PC in WNT signalling is further complicated by the fact that WNT signalling has a regulatory role in ciliogenesis (120, 134, 135). As such, the exact role of PC in WNT signalling, particularly in canonical WNT (11), requires further investigation, though as argued in this section, such an investigation might bring fruitful results if carried out in the MMI context.
Fibroblast Growth Factor
The importance of the Fibroblast Growth Factor (FGF) signalling system has been highlighted in SCZ research (136–138). This system can regulate neuronal differentiation via the Stat1 pathway, and neuronal proliferation and function via the ERK pathway (139). Moreover, FGF function has a positive effect on dopamine neuron survival and neurite outgrowth (139). Recently, bioinformatic and stem cell experiments investigated the role of the FGF receptor 1 (FGFR1) (136, 137). FGFR1 dysregulation can upregulate developmental pathways involved in neurogenesis and downregulate those involved in oligodendrogenesis (136), and data suggests that it can also lead to cortical maldevelopment (137), though the dysregulation of this pathway still awaits confirmation in a larger patient sample.
PC themselves do not seem to mediate FGF signalling, yet both motile and tethering cilia (a type of kinocilium, located on hair cells in the ear, with a microtubule structure similar to motile cilia (5, 140, 141)) length is affected by FGF (142–144). While it remains to be seen how ciliogenesis and PC length can be controlled by FGF in mammals, zebrafish and Xenopus studies suggest that FGF modulate the expression of Ift88 via FGFR1 (144). The IFT machinery is responsible for trafficking anterograde and retrograde cargo along the PC (145, 146) and IFT dysregulation can result in underdevelopment of certain organs, including the brain (145, 147). While the role of IFT in MMI requires further study, IFT27 [which together with IFT88 and IFT172 belongs to the IFT complex B (148)] has in one study been associated with BD (149), however the authors of that study note that this conclusion should be taken with caution due to the amount of variation present throughout the study. Since IFT172 has been identified as also being BBS20 (150) it is worthy to highlight that BBS is associated with such traits as reductions in hippocampal, white and grey matter volumes (57), traits often associated with MMI (151–154) and depression (155) belongs to the IFT complex B.
Therefore, if FGFR1 is proven to be implicated in a larger cohort of individuals with schizophrenia and in the regulation of human PC length, then there would be a mechanistic correlation between defective PC and SCZ. However, it would remain to be seen whether it was the PC dysfunction that contributed to SCZ or whether they were independent consequences of aberrant FGF signalling belongs to the IFT complex B.
Primary Cilia and Dopamine
The dopamine hypothesis is prominent in SCZ research (156, 157), and various dopamine receptors localise to PC (36) in a manner dependent on IFT and BBS components (158, 159). Type 1 and 2 receptors have been shown to localise to PC in neurons in regions such as the striatum, amygdala, and pituitary gland (36, 158, 160, 161). Type 5 receptors, mediating both chemical and mechanical signalling in the PC, were shown on mouse endothelial cell (162), and type 4 receptors have also been shown on non-neuronal cells (36).
While the relationship of dopamine signalling, PC and MMI has not been explored, there might be an overlap between these during brain development. A possible explanation involves the dysregulation of WNT signalling important for the appropriate differentiation of dopaminergic neurons (163). The WNT pathway also regulates dopaminergic neural progenitor cell migration during electrotaxis (62); the health of PC has been shown to affect electrotaxis in fibroblasts (63), though studies in neurons are lacking. Moreover, dopamine signalling has been found to affect PC length in striatal neurons (160). As such, the implications of this interplay between PC and dopamine on MMI remain to be explored.
Cilia–Nature and Nurture
The theme of this research topic compilation concerns neuropsychiatric disorders within the nature and nurture debate, and as such it is fitting to discuss how PC might fit within this debate. It is therefore valuable to reassess some of the aforementioned points within the context of some of the hypotheses of MMI.
The watershed hypothesis of MMI (164) suggests that the diseases might manifest themselves as the cumulative effects of smaller (potentially benign on their own) changes in physiological processes. PC dysfunction might contribute to small changes in several neurally important signalling pathways, not all of which have been mentioned here (165). These changes do not need to originate from serious mutations affecting a single gene (e.g., Disc1), but in themselves might be the result of several less severe changes in PC genes. Nevertheless, it must be remembered that some ciliary proteins might perform the majority of their work outside of the PC (166).
More importantly, genetic changes might, in themselves, not result in a pathological phenotype, but an environmental insult (or several) might be required to trigger the pathological process. This is known as the Two-Hit Hypothesis (167), and is of particular interest here due to the sensory role of the PC. There is a correlation between famine and SCZ (168–170), and there is experimental evidence that environmental stressors, such as maternal ethanol consumption, methylmercury exposure, and pentylenetetrazole (PTZ)-induced maternal seizures can cause neural damage to developing embryos, even at relatively low doses (171). This neural damage is associated with Heat Shock Factor expression level variability, which might be caused by oxidative stress damage (171). PC are involved in stress regulating pathways, such as ERK, but are also affected by the ERK response to oxidative stress and ischaemia (172, 173). Heat shock itself was found to cause ciliary absorption mediated via a reduced association of heat shock protein 90 with HDAC6, and was hypothesised to decrease PC mediated signalling during times of extracellular stress (174). Therefore, PC might provide a molecular link bridging the genetic and environmental components of MMI pathology.
Future Directions
As noted, this manuscript explored several possible links between PC and MMI. Nevertheless, there is little literature directly exploring this topic. As such, we hope that this manuscript will encourage more research in this area. This section highlights some avenues that might be taken in this exploration.
PC length and frequency could be explored in histological specimens from animal models of MMI, and from human MMI patients. With the advances in microscopy and image analysis techniques [we have ourselves proposed such an analysis algorithm (175) for PC length], this is becoming a viable experimental strategy. Perhaps the biggest obstacle might be obtaining human post-mortem samples that would be of good enough quality to visualise PC.
This obstacle could be partly eliminated through the use of induced pluripotent stem cell technology, where human neurons (or other CNS cells) could be generated from tissues samples of MMI patients that could be ethically obtained during their lifetime. These cells could be subjected through a battery of tests, such as the study of their migration responses to a variety of cues. Such experiments would help to overcome several limitations highlighted in the text, e.g., the study of MMI and PC deficient neurons in electric fields. Moreover, using genetic editing technologies the effect of specific MMI associated mutations can also be investigated. These systems could also be used to evaluate the effects of environmental stressors on PC in MMI neurons, a link hypothesised in the previous section. The development of methods for growing cerebral organoids (114, 137), while perhaps raising ethical considerations, will allow for even more complex PC functions to be evaluated in a CNS-like environment.
Finally, while this paper has shown the involvement of PC in a range of signalling pathways. Yet, the evidence might not yet be strong enough to call the PC a signalling hub crucial for MMI. More research should be done to elucidate the role of PC in such key signalling processes for MMI as the dopamine and serotonin pathways (176) [5-HT6 receptors are predominantly expressed on PC (177, 178)], or to look at PC facilitation of pathways involved in the neurodevelopmental defects exhibited by those affected by MMI.
Summary
This review has outlined why PC should be considered as an interesting area for MMI research (see summary in Table 1). It has demonstrated the involvement of PC in a wide variety of cellular processes, such as cell migration and proliferation, and as a signalling hub for various intracellular pathways related to MMI. PC have a unique ability to integrate information necessary for various developmental processes, and as such might be the missing link between the genetic and environmental causes of MMI.
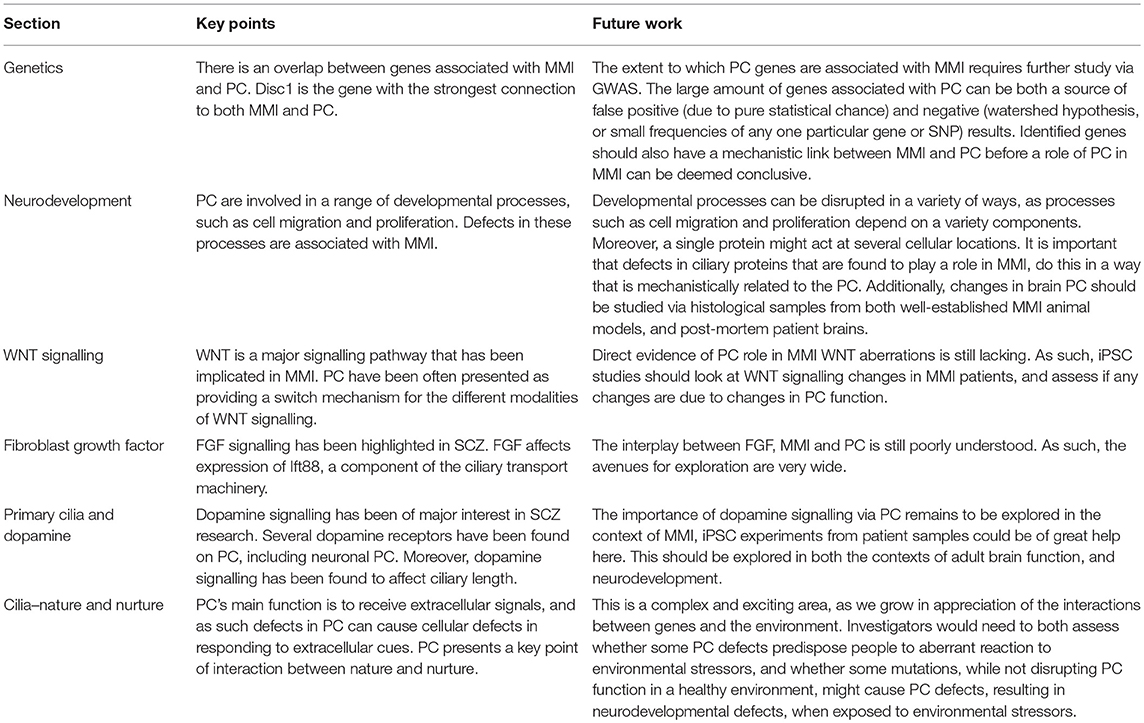
Table 1. Summary of the key points from each section, and avenues for future research related to each section.
PC and neuropsychiatric disorders are interesting fields for research, and much remains to be uncovered. While the arguments presented here show a correlation between PC and several different levels of biological processes associated with psychiatric disease as well as treatment, much yet remains to be experimentally proven. It is up to basic and clinical scientists to determine whether these are just correlations or if there is, indeed, a causative relationship between PC and MMI.
Author Contributions
BL conceived the idea. MP wrote the manuscript with the support of BL.
Funding
MP would like to thank the Scottish Life Science Alliance, the British Council and China Scholarship Council for funding he received during his PhD studies where most of the ideas contained in this manuscript originated. Central South University provided funding to cover the costs of the manuscript.
Conflict of Interest Statement
MP has been a student of two of the special issue editors (BL and YD), and as such collaborated with them on several projects in the last 5 years. BL is an editor of this special issue. All steps were taken to ensure impartial peer-review, and BL did not participate in making the decision about final acceptance of the manuscript for publication.
Acknowledgments
The authors wish to acknowledge the help of Ann Rajnicek and Colin McCaig from the University of Aberdeen as well as Yu-Qiang Ding from Tongji University for comments on several concepts and fragments included in this work. The authors would also want to acknowledge Emma Padfield and Lucia Chaplin for proofreading.
References
1. Muñoz-Estrada J, Lora-Castellanos A, Meza I, Alarcón Elizalde S, Benítez-King G. Primary cilia formation is diminished in schizophrenia and bipolar disorder: a possible marker for these psychiatric diseases. Schizophr Res. (2017) 195:412–20. doi: 10.1016/j.schres.2017.08.055
2. British Medical Association Royal Pharmaceutical Society. British National Formulary. UK (2019). Available online at: https://www.bnf.org/
3. Miyoshi K, Kasahara K, Miyazaki I, Asanuma M. Lithium treatment elongates primary cilia in the mouse brain and in cultured cells. Biochem Biophys Res Commun. (2009) 388:757–62. doi: 10.1016/j.bbrc.2009.08.099
4. Baudoin J-P, Viou L, Launay P-S, Luccardini C, Espeso Gil S, Kiyasova V, et al. Tangentially migrating neurons assemble a primary cilium that promotes their reorientation to the cortical plate. Neuron. (2012) 76:1108–22. doi: 10.1016/j.neuron.2012.10.027
5. Gerdes JM, Davis EE, Katsanis N. The vertebrate primary cilium in development, homeostasis, and disease. Cell. (2009) 137:32–45. doi: 10.1016/j.cell.2009.03.023
6. Goto H, Inoko A, Inagaki M. Cell cycle progression by the repression of primary cilia formation in proliferating cells. Cell Mol Life Sci. (2013) 70:3893–905. doi: 10.1007/s00018-013-1302-8
7. Rieder CL, Jensen CG, Jensen LCW. The resorption of primary cilia during mitosis in a vertebrate (PtK1) cell line. J Ultrastruct Res. (1979) 68:173–85. doi: 10.1016/S0022-5320(79)90152-7
8. Higginbotham H, Eom T-Y, Mariani LE, Bachleda A, Hirt J, Gukassyan V, et al. Arl13b in primary cilia regulates the migration and placement of interneurons in the developing cerebral cortex. Dev Cell. (2012) 23:925–38. doi: 10.1016/j.devcel.2012.09.019
9. Veland IR, Lindbæk L, Christensen ST. Linking the primary cilium to cell migration in tissue repair and brain development. BioScience. (2014) 64:1115–25. doi: 10.1093/biosci/biu179
10. Kirschen G, Xiong Q. Primary cilia as a novel horizon between neuron and environment. Neural Regen Res. (2017) 12:1225. doi: 10.4103/1673-5374.213535
11. Wheway G, Nazlamova L, Hancock JT. Signaling through the primary cilium. Front Cell Dev Biol. (2018) 6:8. doi: 10.3389/fcell.2018.00008
12. Elliott KH, Brugmann SA. Sending mixed signals: cilia-dependent signaling during development and disease. Dev Biol. (2018) 447:28–41. doi: 10.1016/j.ydbio.2018.03.007
13. Wood CR, Rosenbaum JL. Ciliary ectosomes: transmissions from the cell's antenna. Trends Cell Biol. (2015) 25:276–85. doi: 10.1016/j.tcb.2014.12.008
14. Phua SC, Nihongaki Y, Inoue T. Autonomy declared by primary cilia through compartmentalization of membrane phosphoinositides. Curr Opin Cell Biol. (2018) 50:72–8. doi: 10.1016/j.ceb.2018.01.008
15. Muraki K, Tanigaki K. Neuronal migration abnormalities and its possible implications for schizophrenia. Front Neurosci. (2015) 9:74. doi: 10.3389/fnins.2015.00074
16. Yoon K-J, Nguyen HN, Ursini G, Zhang F, Kim N-S, Wen Z, et al. Modeling a genetic risk for schizophrenia in iPSCs and mice reveals neural stem cell deficits associated with adherens junctions and polarity. Cell Stem Cell. (2014) 15:79–91. doi: 10.1016/j.stem.2014.05.003
17. Louvi A, Grove EA. Cilia in the CNS: the quiet organelle claims center stage. Neuron. (2011) 69:1046–60. doi: 10.1016/j.neuron.2011.03.002
18. Lepanto P, Badano JL, Zolessi FR. Neuron's little helper: the role of primary cilia in neurogenesis. Neurogenesis. (2016) 3:e1253363. doi: 10.1080/23262133.2016.1253363
19. Guidi LG, Velayos-Baeza A, Martinez-Garay I, Monaco AP, Paracchini S, Bishop DVM, et al. The neuronal migration hypothesis of dyslexia: a critical evaluation 30 years on. Eur J Neurosci. (2018) 48:3212–33. doi: 10.1111/ejn.14149
20. Arellano JI, Guadiana SM, Breunig JJ, Rakic P, Sarkisian MR. Development and distribution of neuronal cilia in mouse neocortex. J Comp Neurol. (2012) 520:848–73. doi: 10.1002/cne.22793
21. Marley A, von Zastrow M. A simple cell-based assay reveals that diverse neuropsychiatric risk genes converge on primary cilia. PLoS ONE. (2012) 7:e46647. doi: 10.1371/journal.pone.0046647
22. Blackwood DH, He L, Morris SW, McLean A, Whitton C, Thomson M, et al. A locus for bipolar affective disorder on chromosome 4p. Nat Genet. (1996) 12:427–30. doi: 10.1038/ng0496-427
23. Christoforou A, McGhee KA, Morris SW, Thomson PA, Anderson S, McLean A, et al. Convergence of linkage, association and GWAS findings for a candidate region for bipolar disorder and schizophrenia on chromosome 4p. Mol Psychiatry. (2011) 16:240–2. doi: 10.1038/mp.2010.25
24. Veleri S, Manjunath SH, Fariss RN, May-Simera H, Brooks M, Foskett TA, et al. Ciliopathy-associated gene Cc2d2a promotes assembly of subdistal appendages on the mother centriole during cilia biogenesis. Nat Commun. (2014) 5:4207. doi: 10.1038/ncomms5207
25. Szymanska K, Johnson CA. The transition zone: an essential functional compartment of cilia. Cilia. (2012) 1:10. doi: 10.1186/2046-2530-1-10
26. Sang L, Miller JJ, Corbit KC, Giles RH, Brauer MJ, Otto EA, et al. Mapping the nephronophthisis-joubert-meckel-gruber protein network reveals ciliopathy disease genes and pathways. Cell. (2011) 145:513–28. doi: 10.1016/j.cell.2011.04.019
27. Garcia-Gonzalo FR, Corbit KC, Sirerol-Piquer MS, Ramaswami G, Otto EA, Noriega TR, et al. A transition zone complex regulates mammalian ciliogenesis and ciliary membrane composition. Nat Genet. (2011) 43:776–84. doi: 10.1038/ng.891
28. Bachmann-Gagescu R, Ishak GE, Dempsey JC, Adkins J, O'Day D, Phelps IG, et al. Genotype-phenotype correlation in CC2D2A-related Joubert syndrome reveals an association with ventriculomegaly and seizures. J Med Genet. (2012) 49:126–37. doi: 10.1136/jmedgenet-2011-100552
29. Gorden NT, Arts HH, Parisi MA, Coene KLM, Letteboer SJF, van Beersum SEC, et al. CC2D2A is mutated in joubert syndrome and interacts with the ciliopathy-associated basal body protein CEP290. Am J Hum Genet. (2008) 83:559–71. doi: 10.1016/j.ajhg.2008.10.002
30. Sattar S, Gleeson JG. The ciliopathies in neuronal development: a clinical approach to investigation of joubert syndrome and joubert syndrome-related disorders: review. Dev Med Child Neurol. (2011) 53:793–8. doi: 10.1111/j.1469-8749.2011.04021.x
31. Tallila J, Jakkula E, Peltonen L, Salonen R, Kestilä M. Identification of CC2D2A as a meckel syndrome gene adds an important piece to the ciliopathy puzzle. Am J Hum Genet. (2008) 82:1361–7. doi: 10.1016/j.ajhg.2008.05.004
32. Noor A, Windpassinger C, Patel M, Stachowiak B, Mikhailov A, Azam M, et al. CC2D2A, encoding A coiled-coil and C2 domain protein, causes autosomal-recessive mental retardation with retinitis pigmentosa. Am J Hum Genet. (2008) 82:1011–8. doi: 10.1016/j.ajhg.2008.01.021
33. Shi Z-Y, Li Y-J, Zhang K-J, Gao X-C, Zheng Z-J, Han N, et al. Positive association of CC2D1A and CC2D2A gene haplotypes with mental retardation in a Han Chinese population. DNA Cell Biol. (2012) 31:80–7. doi: 10.1089/dna.2011.1253
34. Guipponi M, Santoni FA, Setola V, Gehrig C, Rotharmel M, Cuenca M, et al. Exome Sequencing in 53 sporadic cases of schizophrenia identifies 18 putative candidate genes. PLoS ONE. (2014) 9:112745. doi: 10.1371/journal.pone.0112745
35. Ingason A, Giegling I, Cichon S, Hansen T, Rasmussen HB, Nielsen J, et al. A large replication study and meta-analysis in European samples provides further support for association of AHI1 markers with schizophrenia. Hum Mol Genet. (2010) 19:1379–86. doi: 10.1093/hmg/ddq009
36. Marley A, von Zastrow M. DISC1 regulates primary cilia that display specific dopamine receptors. PLoS ONE. (2010) 5:e10902. doi: 10.1371/journal.pone.0010902
37. Blackwood DH, Fordyce A, Walker MT, St Clair DM, Porteous DJ, Muir WJ. Schizophrenia and affective disorders–cosegregation with a translocation at chromosome 1q42 that directly disrupts brain-expressed genes: clinical and P300 findings in a family. Am J Hum Genet. (2001) 69:428–33. doi: 10.1086/321969
38. Shen S, Lang B, Nakamoto C, Zhang F, Pu J, Kuan S-L, et al. Schizophrenia-related neural and behavioral phenotypes in transgenic mice expressing truncated disc1. J Neurosci. (2008) 28:10893–904. doi: 10.1523/JNEUROSCI.3299-08.2008
39. Soares DC, Carlyle BC, Bradshaw NJ, Porteous DJ. DISC1: structure, function, and therapeutic potential for major mental illness. ACS Chem Neurosci. (2011) 2:609–32. doi: 10.1021/cn200062k
40. Miyoshi K, Asanuma M, Miyazaki I, Diaz-Corrales FJ, Katayama T, Tohyama M, et al. DISC1 localizes to the centrosome by binding to kendrin. Biochem Biophys Res Commun. (2004) 317:1195–9. doi: 10.1016/j.bbrc.2004.03.163
41. Kamiya A, Tan PL, Kubo K, Engelhard C, Ishizuka K, Kubo A T, et al. PCM1 is recruited to the centrosome by the cooperative action of DISC1 and BBS4 and is a candidate for psychiatric illness. Arch Gen Psychiatry. (2008) 65:996–1006. doi: 10.1001/archpsyc.65.9.996
42. Ishizuka K, Kamiya A, Oh EC, Kanki H, Seshadri S, Robinson JF, et al. DISC1-dependent switch from progenitor proliferation to migration in the developing cortex. Nature. (2011) 473:92–6. doi: 10.1038/nature09859
43. Kamiya A, Kubo K, Tomoda T, Takaki M, Youn R, Ozeki Y, et al. A schizophrenia-associated mutation of DISC1 perturbs cerebral cortex development. Nat Cell Biol. (2005) 7:1167–78. doi: 10.1038/ncb1328
44. De Rienzo G, Bishop JA, Mao Y, Pan L, Ma TP, Moens CB, et al. Disc1 regulates both -catenin-mediated and non-canonical Wnt signaling during vertebrate embryogenesis. FASEB J. (2011) 25:4184–97. doi: 10.1096/fj.11-186239
45. Davis EE, Katsanis N. The ciliopathies: a transitional model into systems biology of human genetic disease. Curr Opin Genet Dev. (2012) 22:290–303. doi: 10.1016/j.gde.2012.04.006
46. Valente EM, Rosti RO, Gibbs E, Gleeson JG. Primary cilia in neurodevelopmental disorders. Nat Rev Neurol. (2014) 10:27–36. doi: 10.1038/nrneurol.2013.247
47. Brancati F, Dallapiccola B, Valente EM. Joubert syndrome and related disorders. Orphanet J Rare Dis. (2010) 5:20. doi: 10.1186/1750-1172-5-20
48. Guemez-Gamboa A, Coufal NG, Gleeson JG. Primary Cilia in the developing and mature brain. Neuron. (2014) 82:511–21. doi: 10.1016/j.neuron.2014.04.024
49. Willaredt MA, Tasouri E, Tucker KL. Primary cilia and forebrain development. Mech Dev. (2013) 130:373–80. doi: 10.1016/j.mod.2012.10.003
50. Hong E, Jayachandran P, Brewster R. The polarity protein Pard3 is required for centrosome positioning during neurulation. Dev Biol. (2010) 341:335–45. doi: 10.1016/j.ydbio.2010.01.034
51. May-Simera HL, Kelley MW. Cilia, Wnt signaling, and the cytoskeleton. Cilia. (2012) 1:7. doi: 10.1186/2046-2530-1-7
52. Brennand K, Savas JN, Kim Y, Tran N, Simone A, Hashimoto-Torii K, et al. Phenotypic differences in hiPSC NPCs derived from patients with schizophrenia. Mol Psychiatry. (2015) 20:361–8. doi: 10.1038/mp.2014.22
53. Lang B, Pu J, Hunter I, Liu M, Martin-Granados C, Reilly TJ, et al. Recurrent deletions of ULK4 in schizophrenia: a gene crucial for neuritogenesis and neuronal motility. J Cell Sci. (2014) 127:630–40. doi: 10.1242/jcs.137604
54. Lang B, Zhang L, Jiang G, Hu L, Lan W, Zhao L, et al. Control of cortex development by ULK4, a rare risk gene for mental disorders including schizophrenia. Sci Rep. (2016) 6:31126. doi: 10.1038/srep31126
55. Fukuda T, Yanagi S. Psychiatric behaviors associated with cytoskeletal defects in radial neuronal migration. Cell Mol Life Sci. (2017) 74:3533–52. doi: 10.1007/s00018-017-2539-4
56. Cannon TD, Hennah W, Erp TGM, Thompson PM, Lonnqvist J, Huttunen M, et al. Association of DISC1/TRAX haplotypes with schizophrenia, reduced prefrontal gray matter, and impaired short- and long-term memory. Arch Gen Psychiatry. (2005) 62:1205–13. doi: 10.1001/archpsyc.62.11.1205
57. Baker K, Northam GB, Chong WK, Banks T, Beales P, Baldeweg T. Neocortical and hippocampal volume loss in a human ciliopathy: a quantitative MRI study in Bardet–Biedl syndrome. Am J Med Genet A. (2011) 155:1–8. doi: 10.1002/ajmg.a.33773
58. Davis RE, Swiderski RE, Rahmouni K, Nishimura DY, Mullins RF, Agassandian K, et al. A knockin mouse model of the Bardet Biedl syndrome 1 M390R mutation has cilia defects, ventriculomegaly, retinopathy, and obesity. Proc Natl Acad Sci. USA. (2007) 104:19422–7. doi: 10.1073/pnas.0708571104
59. Burdick KE, Kamiya A, Hodgkinson CA, Lencz T, DeRosse P, Ishizuka K, et al. Elucidating the relationship between DISC1, NDEL1 and NDE1 and the risk for schizophrenia: Evidence of epistasis and competitive binding. Hum Mol Genet. (2008) 17:2462–73. doi: 10.1093/hmg/ddn146
60. Gurling HM, Critchley H, Datta SR, McQuillin A, Blaveri E, Thirumalai S, et al. Genetic association and brain morphology studies and the chromosome 8p22 pericentriolar material 1 (PCM1) gene in susceptibility to schizophrenia. Arch Gen Psychiatry. (2006) 63:844–54. doi: 10.1001/archpsyc.63.8.844
61. Higginbotham H, Guo J, Yokota Y, Umberger NL, Su C-Y, Li J, et al. Arl13b-regulated cilia activities are essential for polarized radial glial scaffold formation. Nat Neurosci. (2013) 16:1000–7. doi: 10.1038/nn.3451
62. Liu J, Zhu B, Zhang G, Wang J, Tian W, Ju G, et al. Electric signals regulate directional migration of ventral midbrain derived dopaminergic neural progenitor cells via Wnt/GSK3β signaling. Exp Neurol. (2015) 263:113–21. doi: 10.1016/j.expneurol.2014.09.014
63. Pruski M, Rajnicek A, Yang Z, Clancy H, Ding Y-Q, McCaig CD, et al. The ciliary GTPase Arl13b regulates cell migration and cell cycle progression. Cell Adhes Migr. (2016) 10:393–405. doi: 10.1080/19336918.2016.1159380
64. Cao L, Wei D, Reid B, Zhao S, Pu J, Pan T, et al. Endogenous electric currents might guide rostral migration of neuroblasts. EMBO Rep. (2013) 14:184–90. doi: 10.1038/embor.2012.215
65. Humbert MC, Weihbrecht K, Searby CC, Li Y, Pope RM, Sheffield VC, et al. ARL13B, PDE6D, and CEP164 form a functional network for INPP5E ciliary targeting. Proc Natl Acad Sci. USA. (2012) 109:19691–6. doi: 10.1073/pnas.1210916109
66. Plotnikova OV, Seo S, Cottle DL, Conduit S, Hakim S, Dyson JM, et al. INPP5E interacts with AURKA, linking phosphoinositide signaling to primary cilium stability. J Cell Sci. (2015) 128:364–72. doi: 10.1242/jcs.161323
67. Yamada M, Hirotsune S, Wynshaw-Boris A. The essential role of LIS1, NDEL1 and Aurora-A in polarity formation and microtubule organization during neurogensis. Cell Adhes Migr. (2010) 4:180–4. doi: 10.4161/cam.4.2.10715
68. Barral DC, Garg S, Casalou C, Watts GFM, Sandoval JL, Ramalho JS, et al. Arl13b regulates endocytic recycling traffic. Proc Natl Acad Sci USA. (2012) 109:21354–9. doi: 10.1073/pnas.1218272110
69. Casalou C, Seixas C, Portelinha A, Pintado P, Barros M, Ramalho JS, et al. Arl13b and the non-muscle myosin heavy chain IIA are required for circular dorsal ruffle formation and cell migration. J Cell Sci. (2014) 127:2709–22. doi: 10.1242/jcs.143446
70. Choi SY, Chacon-Heszele MF, Huang L, McKenna S, Wilson FP, Zuo X, et al. Cdc42 deficiency causes ciliary abnormalities and cystic kidneys. J Am Soc Nephrol. (2013) 24:1435–50. doi: 10.1681/ASN.2012121236
71. Ruppersburg CC, Hartzell HC. The Ca2+-activated Cl- channel ANO1/TMEM16A regulates primary ciliogenesis. Mol Biol Cell. (2014) 25:1793–807. doi: 10.1091/mbc.E13-10-0599
72. Zuo X, Fogelgren B, Lipschutz JH. The small GTPase Cdc42 is necessary for primary ciliogenesis in renal tubular epithelial cells. J Biol Chem. (2011) 286:22469–77. doi: 10.1074/jbc.M111.238469
73. Rajnicek AM, Foubister LE, McCaig CD. Temporally and spatially coordinated roles for Rho, Rac, Cdc42 and their effectors in growth cone guidance by a physiological electric field. J Cell Sci. (2006) 119:1723–35. doi: 10.1242/jcs.02896
74. Schlessinger K, Hall A, Tolwinski N. Wnt signaling pathways meet Rho GTPases. Genes Dev. (2009) 23:265–77. doi: 10.1101/gad.1760809
75. Schlessinger K, McManus EJ, Hall A. Cdc42 and non-canonical Wnt signal transduction pathways cooperate to promote cell polarity. J Cell Biol. (2007) 178:355–61. doi: 10.1083/jcb.200701083
76. Ide M, Lewis DA. Altered cortical CDC42 signaling pathways in schizophrenia: implications for dendritic spine deficits. Biol Psychiatry. (2010) 68:25–32. doi: 10.1016/j.biopsych.2010.02.016
77. Datta D, Arion D, Corradi JP, Lewis DA. Altered expression of CDC42 signaling pathway components in cortical layer 3 pyramidal cells in schizophrenia. Biol Psychiatry. (2015) 78:775–85. doi: 10.1016/j.biopsych.2015.03.030
78. Zhao Z, Xu J, Chen J, Kim S, Reimers M, Bacanu S-A, et al. Transcriptome sequencing and genome-wide association analyses reveal lysosomal function and actin cytoskeleton remodeling in schizophrenia and bipolar disorder. Mol Psychiatry. (2015) 20:563–72. doi: 10.1038/mp.2014.82
79. Guo J, Otis JM, Higginbotham H, Monckton C, Cheng J, Asokan A, et al. Primary cilia signaling shapes the development of interneuronal connectivity. Dev Cell. (2017) 42:286–300.e4. doi: 10.1016/j.devcel.2017.07.010
80. Guadiana SM, Semple-Rowland S, Daroszewski D, Madorsky I, Breunig JJ, Mykytyn K, et al. Arborization of dendrites by developing neocortical neurons is dependent on primary cilia and type 3 adenylyl cyclase. J Neurosci. (2013) 33:2626–38. doi: 10.1523/JNEUROSCI.2906-12.2013
81. Kumamoto N, Gu Y, Wang J, Janoschka S, Takemaru K-I, Levine J, et al. A role for primary cilia in glutamatergic synaptic integration of adult-born neurons. Nat Neurosci. (2012) 15:399–405. doi: 10.1038/nn.3042
82. Brennand KJ, Simone A, Jou J, Gelboin-Burkhart C, Tran N, Sangar S, et al. Modelling schizophrenia using human induced pluripotent stem cells. Nature. (2011) 473:221–5. doi: 10.1038/nature09915
83. Wen Z, Nguyen HN, Guo Z, Lalli MA, Wang X, Su Y, et al. Synaptic dysregulation in a human iPS cell model of mental disorders. Nature. (2014) 515:414–8. doi: 10.1038/nature13716
84. Whalley HC, Simonotto E, Marshall I, Owens DGC, Goddard NH, Johnstone EC, et al. Functional disconnectivity in subjects at high genetic risk of schizophrenia. Brain. (2005) 128:2097–108. doi: 10.1093/brain/awh556
85. Brown SM, Clapcote SJ, Millar JK, Torrance HS, Anderson SM, Walker R, et al. Synaptic modulators Nrxn1 and Nrxn3 are disregulated in a Disc1 mouse model of schizophrenia. Mol Psychiatry. (2011) 16:585–7. doi: 10.1038/mp.2010.134
86. Srikanth P, Han K, Callahan DG, Makovkina E, Muratore CR, Lalli MA, et al. Genomic DISC1 disruption in hiPSCs alters Wnt signaling and neural cell fate. Cell Rep. (2015) 12:1414–29. doi: 10.1016/j.celrep.2015.07.061
87. Robicsek O, Karry R, Petit I, Salman-Kesner N, Müller F-J, Klein E, et al. Abnormal neuronal differentiation and mitochondrial dysfunction in hair follicle-derived induced pluripotent stem cells of schizophrenia patients. Mol Psychiatry. (2013) 18:1067–76. doi: 10.1038/mp.2013.67
88. Yu DX, Di Giorgio FP, Yao J, Marchetto MC, Brennand K, Wright R, et al. Modeling hippocampal neurogenesis using human pluripotent stem cells. Stem Cell Rep. (2014) 2:295–310. doi: 10.1016/j.stemcr.2014.01.009
89. Paridaen JTML, Wilsch-Bräuninger M, Huttner WB. Asymmetric inheritance of centrosome-associated primary cilium membrane directs ciliogenesis after cell division. Cell. (2013) 155:333–44. doi: 10.1016/j.cell.2013.08.060
90. Bakircioglu M, Carvalho OP, Khurshid M, Cox JJ, Tuysuz B, Barak T, et al. The essential role of centrosomal NDE1 in human cerebral cortex neurogenesis. Am J Hum Genet. (2011) 88:523–35. doi: 10.1016/j.ajhg.2011.03.019
91. Gabriel E, Wason A, Ramani A, Gooi LM, Keller P, Pozniakovsky A, et al. CPAP promotes timely cilium disassembly to maintain neural progenitor pool. EMBO J. (2016) 35:803–19. doi: 10.15252/embj.201593679
92. Corbit KC, Aanstad P, Singla V, Norman AR, Stainier DYR, Reiter JF. Vertebrate smoothened functions at the primary cilium. Nature. (2005) 437:1018–21. doi: 10.1038/nature04117
93. Huangfu D, Anderson KV. Cilia and hedgehog responsiveness in the mouse. Proc Natl Acad Sci USA. (2005) 102:11325–30. doi: 10.1073/pnas.0505328102
94. Larkins CE, Aviles GDG, East MP, Kahn RA, Caspary T. Arl13b regulates ciliogenesis and the dynamic localization of Shh signaling proteins. Mol Biol Cell. (2011) 22:4694–703. doi: 10.1091/mbc.E10-12-0994
95. D'Angelo A, Franco B. The dynamic cilium in human diseases. PathoGenetics. (2009) 2:3. doi: 10.1186/1755-8417-2-3
96. Sotak BN, Gleeson JG. Can't get there from here: cilia and hydrocephalus. Nat Med. (2012) 18:1742–3. doi: 10.1038/nm.3011
97. Schneider L, Clement CA, Teilmann SC, Pazour GJ, Hoffmann EK, Satir P, Christensen ST. PDGFRαα signaling is regulated through the primary cilium in fibroblasts. Curr Biol. (2005) 15:1861–6. doi: 10.1016/j.cub.2005.09.012
98. Schneider L, Cammer M, Lehman J, Nielsen SK, Guerra CF, Veland IR, et al. Directional cell migration and chemotaxis in wound healing response to PDGF-AA are coordinated by the primary cilium in fibroblasts. Cell Physiol Biochem. (2010) 25:279–92. doi: 10.1159/000276562
99. Bisel B, Wang Y, Wei J-H, Xiang Y, Tang D, Miron-Mendoza M, et al. ERK regulates Golgi and centrosome orientation towards the leading edge through GRASP65. J Cell Biol. (2008) 182:837–43. doi: 10.1083/jcb.200805045
100. Chabin-Brion K, Marceiller J, Perez F, Settegrana C, Drechou A, Durand G, et al. The golgi complex is a microtubule-organizing organelle. Mol Biol Cell. (2001) 12:2047–60. doi: 10.1091/mbc.12.7.2047
101. Rao S, Ge S, Shelly M. Centrosome positioning and primary cilia assembly orchestrate neuronal development. Front Biol. (2012) 7:412–27. doi: 10.1007/s11515-012-1231-1
102. Yanagida M, Miyoshi R, Toyokuni R, Zhu Y, Murakami F. Dynamics of the leading process, nucleus, and Golgi apparatus of migrating cortical interneurons in living mouse embryos. Proc Natl Acad Sci USA. (2012) 109:16737–42. doi: 10.1073/pnas.1209166109
103. Basten SG, Giles RH. Functional aspects of primary cilia in signaling, cell cycle and tumorigenesis. Cilia. (2013) 2:6. doi: 10.1186/2046-2530-2-6
104. Adolphe C, Hetherington R, Ellis T, Wainwright B. Patched1 functions as a gatekeeper by promoting cell cycle progression. Cancer Res. (2006) 66:2081–8. doi: 10.1158/0008-5472.CAN-05-2146
105. Plotnikova OV, Pugacheva EN, Golemis EA. Primary cilia and the cell cycle. Methods Cell Biol. (2009) 94:137–160. doi: 10.1016/S0091-679X(08)94007-3
106. Plotnikova OV, Golemis EA, Pugacheva EN. Cell cycle-dependent ciliogenesis and cancer. Cancer Res. (2008) 68:2058–61. doi: 10.1158/0008-5472.CAN-07-5838
107. Crane R, Gadea B, Littlepage L, Wu H, Ruderman JV. Aurora A, meiosis and mitosis. Biol Cell. (2004) 96:215–29. doi: 10.1016/j.biolcel.2003.09.008
108. Liu Q, Ruderman JV. Aurora A, mitotic entry, and spindle bipolarity. Proc Natl Acad Sci USA. (2006) 103:5811–6. doi: 10.1073/pnas.0601425103
109. Mühlhans J, Brandstätter JH, Gießl A. The centrosomal protein pericentrin identified at the basal body complex of the connecting cilium in mouse photoreceptors. PLoS ONE. (2011) 6:e26496. doi: 10.1371/journal.pone.0026496
110. Carter CS, Vogel TW, Zhang Q, Seo S, Swiderski RE, Moninger TO, et al. Abnormal development of NG2+PDGFR-α+ neural progenitor cells leads to neonatal hydrocephalus in a ciliopathy mouse model. Nat Med. (2012) 18:1797–804. doi: 10.1038/nm.2996
111. Hoseth EZ, Krull F, Dieset I, Mørch RH, Hope S, Gardsjord ES, et al. Exploring the Wnt signaling pathway in schizophrenia and bipolar disorder. Transl Psychiatry. (2018) 8:55. doi: 10.1038/s41398-018-0102-1
112. Muneer A. Wnt and GSK3 signaling pathways in bipolar disorder: clinical and therapeutic implications. Clin Psychopharmacol Neurosci. (2017) 15:100–14. doi: 10.9758/cpn.2017.15.2.100
113. Topol A, Zhu S, Tran N, Simone A, Fang G, Brennand KJ. Altered WNT signaling in hiPSC NPCs derived from four schizophrenia patients. Biol Psychiatry. (2015) 78:e29–34. doi: 10.1016/j.biopsych.2014.12.028
114. Srikanth P, Lagomarsino VN, Muratore CR, Ryu SC, He A, Taylor WM, et al. Shared effects of DISC1 disruption and elevated WNT signaling in human cerebral organoids. Transl Psychiatry. (2018) 8:77. doi: 10.1038/s41398-018-0122-x
115. Gerdes JM, Liu Y, Zaghloul NA, Leitch CC, Lawson SS, Kato M, et al. Disruption of the basal body compromises proteasomal function and perturbs intracellular Wnt response. Nat Genet. (2007) 39:1350–60. doi: 10.1038/ng.2007.12
116. Schneider-Maunoury S, Mahuzier A, Gaudé H, Anselme I, Silbermann F, Leroux-Berger M, et al. Dishevelled stablisation at the cilium by RPGRIP1L is essential for planar cell polarity. Cilia. (2012) 1:O21. doi: 10.1186/2046-2530-1-S1-O21
117. Corbit KC, Shyer AE, Dowdle WE, Gaulden J, Singla V, Chen M-H, et al. Kif3a constrains beta-catenin-dependent Wnt signalling through dual ciliary and non-ciliary mechanisms. Nat Cell Biol. (2008) 10:70–6. doi: 10.1038/ncb1670
118. Lancaster MA, Schroth J, Gleeson JG. Subcellular spatial regulation of canonical Wnt signalling at the primary cilium. Nat Cell Biol. (2011) 13:702–9. doi: 10.1038/ncb2259
119. Oh EC, Katsanis N. Context-dependent regulation of Wnt signaling through the primary cilium. J Am Soc Nephrol. (2013) 24:10–8. doi: 10.1681/ASN.2012050526
120. Caron A, Xu X, Lin X. Wnt/β-catenin signaling directly regulates Foxj1 expression and ciliogenesis in zebrafish Kupffer's vesicle. Dev Camb Engl. (2012) 139:514–24. doi: 10.1242/dev.071746
121. Park TJ, Mitchell BJ, Abitua PB, Kintner C, Wallingford JB. Dishevelled controls apical docking and planar polarization of basal bodies in ciliated epithelial cells. Nat Genet. (2008) 40:871–9. doi: 10.1038/ng.104
122. Pitaval A, Tseng Q, Bornens M, Thery M. Cell shape and contractility regulate ciliogenesis in cell cycle-arrested cells. J Cell Biol. (2010) 191:303–12. doi: 10.1083/jcb.201004003
123. Lang B. Expression of the human PAC1 receptor leads to dose-dependent hydrocephalus-related abnormalities in mice. J Clin Invest. (2006) 116:1924–34. doi: 10.1172/JCI27597
124. Malaspina D. Looking schizophrenia in the eye. Am J Psychiatry. (2013) 170:1382–4. doi: 10.1176/appi.ajp.2013.13081136
125. Yoshiba S, Shiratori H, Kuo IY, Kawasumi A, Shinohara K, Nonaka S, et al. Cilia at the Node of mouse embryos sense fluid flow for left-right determination via Pkd2. Science. (2012) 338:226–31. doi: 10.1126/science.1222538
126. Davis EE, Brueckner M, Katsanis N. The emerging complexity of the vertebrate cilium: new functional roles for an ancient organelle. Dev Cell. (2006) 11:9–19. doi: 10.1016/j.devcel.2006.06.009
127. Hanaoka K, Qian F, Boletta A, Bhunia AK, Piontek K, Tsiokas L, et al. Co-assembly of polycystin-1 and-2 produces unique cation-permeable currents. Nature. (2000) 408:990–4. doi: 10.1038/35050128
128. Hoffmeister H, Babinger K, Gurster S, Cedzich A, Meese C, Schadendorf K, et al. Polycystin-2 takes different routes to the somatic and ciliary plasma membrane. J Cell Biol. (2011) 192:631–45. doi: 10.1083/jcb.201007050
129. Majhi R, Sardar P, Goswami L, Goswami C. Right time–right location–right move: TRPs find motors for common functions. Channels. (2011) 5:375–81. doi: 10.4161/chan.5.4.16969
130. Delling M, Indzhykulian AA, Liu X, Li Y, Xie T, Corey DP, et al. Primary cilia are not calcium-responsive mechanosensors. Nature. (2016) 531:656–60. doi: 10.1038/nature17426
131. Stottmann R, Tran P, Turbe-Doan A, Beier D. Ttc21b is required to restrict sonic hedgehog activity in the developing mouse forebrain. Dev Biol. (2009) 335:166–78. doi: 10.1016/j.ydbio.2009.08.023
132. Huang P, Schier AF. Dampened hedgehog signaling but normal Wnt signaling in zebrafish without cilia. Dev Camb Engl. (2009) 136:3089–98. doi: 10.1242/dev.041343
133. Ocbina PJR, Tuson M, Anderson KV. Primary Cilia are not required for normal canonical Wnt signaling in the mouse embryo. PLoS ONE. (2009) 4:e6839. doi: 10.1371/journal.pone.0006839
134. Nakaya M, Biris K, Tsukiyama T, Jaime S, Rawls JA, Yamaguchi TP. Wnt3a links left-right determination with segmentation and anterior-posterior axis elongation. Dev Camb Engl. (2005) 132:5425–36. doi: 10.1242/dev.02149
135. Ganner A, Lienkamp S, Schäfer T, Romaker D, Wegierski T, Park TJ, et al. Regulation of ciliary polarity by the APC/C. Proc Natl Acad Sci USA. (2009) 106:17799–804. doi: 10.1073/pnas.0909465106
136. Narla ST, Lee Y-W, Benson CA, Sarder P, Brennand KJ, Stachowiak EK, et al. Common developmental genome deprogramming in schizophrenia — Role of integrative nuclear FGFR1 signaling (INFS). Schizophr Res. (2017) 185:17–32. doi: 10.1016/j.schres.2016.12.012
137. Stachowiak EK, Benson CA, Narla ST, Dimitri A, Chuye LEB, Dhiman S, et al. Cerebral organoids reveal early cortical maldevelopment in schizophrenia—computational anatomy and genomics, role of FGFR1. Transl Psychiatry. (2017) 7:6. doi: 10.1038/s41398-017-0054-x
138. van Scheltinga AFT, Bakker SC, Kahn RS. Fibroblast growth factors in schizophrenia. Schizophr Bull. (2010) 36:1157–66. doi: 10.1093/schbul/sbp033
139. Grothe C, Timmer M. The physiological and pharmacological role of basic fibroblast growth factor in the dopaminergic nigrostriatal system. Brain Res Rev. (2007) 54:80–91. doi: 10.1016/j.brainresrev.2006.12.001
140. Baxendale S, Whitfield TT. Zebrafish inner ear development and function. In: Romand R, Varela-Nieto I, editors. Development of Auditory and Vestibular Systems. Oxford: Elsevier (2014). p. 63–105. doi: 10.1016/B978-0-12-408088-1.00003-8
141. Spoon C, Grant W. Biomechanics of hair cell kinocilia: experimental measurement of kinocilium shaft stiffness and base rotational stiffness with Euler-Bernoulli and Timoshenko beam analysis. J Exp Biol. (2011) 214:862–70. doi: 10.1242/jeb.051151
142. Basu B, Brueckner M. Fibroblast “Cilia Growth” factor in the development of left-right asymmetry. Dev Cell. (2009) 16:489–90. doi: 10.1016/j.devcel.2009.04.004
143. Lodh S, O'Hare EA, Zaghloul NA. Primary cilia in pancreatic development and disease. Birth Defects Res Part C Embryo Today Rev. (2014) 102:139–58. doi: 10.1002/bdrc.21063
144. Neugebauer JM, Amack JD, Peterson AG, Bisgrove BW, Yost HJ. FGF signaling during embryo development regulates cilia length in diverse epithelia. Nature. (2009) 458:651–4. doi: 10.1038/nature07753
145. Haycraft CJ, Banizs B, Aydin-Son Y, Zhang Q, Michaud EJ, Yoder BK. Gli2 and Gli3 localize to cilia and require the intraflagellar transport protein polaris for processing and function. PLoS Genet. (2005) 1:e53. doi: 10.1371/journal.pgen.0010053
146. Ocbina PJR, Anderson KV. Intraflagellar transport, cilia, and mammalian Hedgehog signaling: analysis in mouse embryonic fibroblasts. Dev Dyn. (2008) 237:2030–8. doi: 10.1002/dvdy.21551
147. Murdoch JN, Copp AJ. The relationship between Sonic hedgehog signalling, cilia and neural tube defects. Birt Defects Res A Clin Mol Teratol. (2010) 88:633–52. doi: 10.1002/bdra.20686
148. Follit JA, Xu F, Keady B, Pazour GJ. Characterization of mouse IFT complex B. Cell Motil Cytoskeleton. (2009) 66:457–68. doi: 10.1002/cm.20346
149. Nissen S, Liang S, Shehktman T, Kelsoe JR, The Bipolar Genome Study (BiGS). Evidence for association of bipolar disorder to haplotypes in the 22q12.3 region near the genes stargazin, ift27 and parvalbumin. Am J Med Genet B Neuropsychiatr Genet. (2012) 159B:941–50. doi: 10.1002/ajmg.b.32099
150. Schaefer E, Stoetzel C, Scheidecker S, Geoffroy V, Prasad MK, Redin C, et al. Identification of a novel mutation confirms the implication of IFT172 (BBS20) in Bardet–Biedl syndrome. J Hum Genet. (2016) 61:447–50. doi: 10.1038/jhg.2015.162
151. Heckers S. Neuroimaging studies of the hippocampus in schizophrenia. Hippocampus. (2001) 11:520–8. doi: 10.1002/hipo.1068
152. Selvaraj S, Arnone D, Job D, Stanfield A, Farrow TF, Nugent AC, et al. Grey matter differences in bipolar disorder: a meta-analysis of voxel-based morphometry studies. Bipolar Disord. (2012) 14:135–45. doi: 10.1111/j.1399-5618.2012.01000.x
153. McIntosh AM, Maniega SM, Lymer GKS, McKirdy J, Hall J, Sussmann JED, et al. White matter tractography in bipolar disorder and schizophrenia. Biol Psychiatry. (2008) 64:1088–92. doi: 10.1016/j.biopsych.2008.07.026
154. Douaud G, Smith S, Jenkinson M, Behrens T, Johansen-Berg H, Vickers J, et al. Anatomically related grey and white matter abnormalities in adolescent-onset schizophrenia. Brain. (2007) 130:2375–86. doi: 10.1093/brain/awm184
155. Schmaal L, Yücel M, Ellis R, Vijayakumar N, Simmons JG, Allen NB, et al. Brain structural signatures of adolescent depressive symptom trajectories: a longitudinal magnetic resonance imaging study. J Am Acad Child Adolesc Psychiatry. (2017) 56:593–601.e9. doi: 10.1016/j.jaac.2017.05.008
156. Hains AB, Arnsten AFT. Molecular mechanisms of stress-induced prefrontal cortical impairment: implications for mental illness. Learn Mem Cold Spring Harb N. (2008) 15:551–64. doi: 10.1101/lm.921708
157. Eyles D, Feldon J, Meyer U. Schizophrenia: do all roads lead to dopamine or is this where they start? Evidence from two epidemiologically informed developmental rodent models. Transl Psychiatry. (2012) 2:e81. doi: 10.1038/tp.2012.6
158. Domire JS, Green JA, Lee KG, Johnson AD, Askwith CC, Mykytyn K. Dopamine receptor 1 localizes to neuronal cilia in a dynamic process that requires the bardet-biedl syndrome proteins. Cell Mol Life Sci. (2011) 68:2951–60. doi: 10.1007/s00018-010-0603-4
159. Leaf A, Von Zastrow M. Dopamine receptors reveal an essential role of IFT-B, KIF17, and Rab23 in delivering specific receptors to primary cilia. eLife. 4:6996. doi: 10.7554/eLife.06996
160. Miyoshi K, Kasahara K, Murakami S, Takeshima M, Kumamoto N, Sato A, et al. Lack of Dopaminergic inputs elongates the primary cilia of striatal neurons. PLos ONE. (2014) 9:e97918. doi: 10.1371/journal.pone.0097918
161. Iwanaga T, Hozumi Y, Takahashi-Iwanaga H. Immunohistochemical demonstration of dopamine receptor D2R in the primary cilia of the mouse pituitary gland. Biomed Res. (2011) 32:225–35. doi: 10.2220/biomedres.32.225
162. Abdul-Majeed S, Nauli SM. Dopamine receptor type-5 in the primary cilia has a dual chemo- and mechano-sensory role. Hypertension. (2011) 58:325–31. doi: 10.1161/HYPERTENSIONAHA.111.172080
163. Cajanek L, Ganji RS, Henriques-Oliveira C, Theofilopoulos S, Konik P, Bryja V, et al. Tiam1 regulates the Wnt/Dvl/Rac1 signaling pathway and the differentiation of midbrain dopaminergic neurons. Mol Cell Biol. (2013) 33:59–70. doi: 10.1128/MCB.00745-12
164. Cannon TD, Keller MC. Endophenotypes in the genetic analyses of mental disorders. Annu Rev Clin Psychol. (2006) 2:267–90. doi: 10.1146/annurev.clinpsy.2.022305.095232
165. Green JA, Mykytyn K. Neuronal primary cilia: an underappreciated signaling and sensory organelle in the brain. Neuropsychopharmacology. (2014) 39:244–5. doi: 10.1038/npp.2013.203
166. Yuan S, Sun Z. Expanding horizons: ciliary proteins reach beyond cilia. Annu Rev Genet. (2013) 47:353–76. doi: 10.1146/annurev-genet-111212-133243
167. Maynard TM, Sikich L, Lieberman JA, LaMantia A-S. Neural development, cell-cell signaling, and the “Two-Hit” hypothesis of schizophrenia. Schizophr Bull. (2001) 27:457–76. doi: 10.1093/oxfordjournals.schbul.a006887
168. Hoek HW, Brown AS, Susser E. The Dutch famine and schizophrenia spectrum disorders. Soc Psychiatry Psychiatr Epidemiol. (1998) 33:373–9.
169. St Clair D, Xu M, Wang P, Yu Y, Fang Y, Zhang F, et al. Rates of adult schizophrenia following prenatal exposure to the Chinese famine of 1959-1961. JAMA. (2005) 294:557–62. doi: 10.1001/jama.294.5.557
170. Xu M-Q, Sun W-S, Liu B-X, Feng G-Y, Yu L, Yang L, et al. Prenatal Malnutrition and adult schizophrenia: further evidence from the 1959-1961 Chinese famine. Schizophr Bull. (2009) 35:568–76. doi: 10.1093/schbul/sbn168
171. Hashimoto-Torii K, Torii M, Fujimoto M, Nakai A, El Fatimy R, Mezger V, et al. Roles of heat shock factor 1 in neuronal response to fetal environmental risks and its relevance to brain disorders. Neuron. (2014) 82:560–72. doi: 10.1016/j.neuron.2014.03.002
172. Kim JI, Kim J, Jang H-S, Noh MR, Lipschutz JH, Park KM. Reduction of oxidative stress during recovery accelerates normalization of primary cilia length that is altered after ischemic injury in murine kidneys. AJP Ren Physiol. (2013) 304:F1283–94. doi: 10.1152/ajprenal.00427.2012
173. Incani A, Deiana M, Corona G, Vafeiadou K, Vauzour D, Dessì MA, et al. Involvement of ERK, Akt and JNK signalling in H2O2-induced cell injury and protection by hydroxytyrosol and its metabolite homovanillic alcohol. Mol Nutr Food Res. (2010) 54:788–96. doi: 10.1002/mnfr.200900098
174. Prodromou NV, Thompson CL, Osborn DPS, Cogger KF, Ashworth R, Knight MM, et al. Heat shock induces rapid resorption of primary cilia. J Cell Sci. (2012) 125:4297–305. doi: 10.1242/jcs.100545
175. Szulc M, Muellerschoen L, Penny L, Pruski M, Lang B. 3D measurement of large quantities of cilia in ImageJ/FIJI [V1; not peer reviewed]. In: F1000Research, 604(poster) Edinburgh. doi: 10.7490/f1000research.1111669.1
176. Beaulieu J-M. A role for Akt and glycogen synthase kinase-3 as integrators of dopamine and serotonin neurotransmission in mental health. J Psychiatry Neurosci JPN. (2012) 37:7–16. doi: 10.1503/jpn.110011
177. Hu L, Wang B, Zhang Y. Serotonin 5-HT6 receptors affect cognition in a mouse model of Alzheimer's disease by regulating cilia function. Alzheimers Res Ther. (2017) 9:76. doi: 10.1186/s13195-017-0304-4
Keywords: primary cilia, schizophrenia, bipolar disorder, neurodevelopment, brain
Citation: Pruski M and Lang B (2019) Primary Cilia–An Underexplored Topic in Major Mental Illness. Front. Psychiatry 10:104. doi: 10.3389/fpsyt.2019.00104
Received: 17 September 2018; Accepted: 12 February 2019;
Published: 04 March 2019.
Edited by:
Dimitrios Avramopoulos, Johns Hopkins University, United StatesReviewed by:
Pippa Ann Thomson, Medical Research Council Institute of Genetics and Molecular Medicine (MRC), United KingdomKirk Mykytyn, The Ohio State University, United States
Copyright © 2019 Pruski and Lang. This is an open-access article distributed under the terms of the Creative Commons Attribution License (CC BY). The use, distribution or reproduction in other forums is permitted, provided the original author(s) and the copyright owner(s) are credited and that the original publication in this journal is cited, in accordance with accepted academic practice. No use, distribution or reproduction is permitted which does not comply with these terms.
*Correspondence: Bing Lang, bing.lang@csu.edu.cn
Michal Pruski, 17104596@stu.mmu.ac.uk
orcid.org0000-0001-7582-1418