- 1Laboratory for Molecular Neuropsychiatry, Division of Molecular Medicine, Rudjer Boskovic Institute, Zagreb, Croatia
- 2Department of Biological Psychiatry and Psychogeriatry, University Psychiatric Hospital Vrapce, Zagreb, Croatia
- 3Faculty of Medicine, Josip Juraj Strossmayer University of Osijek, Osijek, Croatia
- 4School of Medicine, University of Zagreb, Zagreb, Croatia
- 5Department of Psychiatry, University Hospital Centre Zagreb, Zagreb, Croatia
- 6Department of Psychopharmacology, Croatian Institute for Brain Research, School of Medicine, University of Zagreb, Zagreb, Croatia
Posttraumatic stress disorder (PTSD) is a trauma and stressor related disorder that may develop after exposure to an event that involved the actual or possible threat of death, violence or serious injury. Its molecular underpinning is still not clear. Brain-derived neurotrophic factor (BDNF) modulates neuronal processes such as the response to stress, but also weight control, energy and glucose homeostasis. Plasma BDNF levels and a functional BDNF Val66Met (rs6265) polymorphism were reported to be associated with PTSD, as well as with increased body mass index (BMI) and dyslipidaemia in healthy subjects and patients with cardio-metabolic diseases, but these results are controversial. The other frequently studied BDNF polymorphism, C270T (rs56164415), has been associated with the development of different neuropsychiatric symptoms/disorders. As far as we are aware, there are no data on the association of BDNF Val66Met and C270T polymorphisms with metabolic indices in PTSD. Due to high rates of obesity and dyslipidaemia in PTSD, the aim of this study was to elucidate the association of BDNF Val66Met and C270T polymorphisms with BMI and lipid levels in veterans with PTSD. We hypothesized that BDNF variants contribute to susceptibility to metabolic disturbances in PTSD. The study included 333 Caucasian males with combat related PTSD, diagnosed according to DSM-5 criteria. Genotyping of the BDNF Val66Met and C270T polymorphisms was performed using the real-time PCR method. Results were analyzed using hierarchical multiple linear regression and the Mann–Whitney test, with p-value corrected to 0.005. The results showed that BDNF Val66Met and BDNF C270T polymorphisms were not significantly associated with BMI, total cholesterol, LDL-cholesterol, HDL-cholesterol or triglycerides. Although the BDNF C270T polymorphism was nominally associated only with HDL-cholesterol in veterans with PTSD, this significance disappeared after controlling for the effect of age. Namely, slightly higher plasma HDL values in T allele carriers, compared to CC homozygotes, were associated with differences in age. Our results, controlled for the critical covariates, revealed that BDNF Val66Met and C270T were not significantly associated with metabolic indices in veterans with PTSD and that these genetic variants do not contribute to susceptibility to metabolic disturbances in PTSD.
Introduction
Posttraumatic Stress Disorder
Posttraumatic stress disorder (PTSD) is a trauma- and stressor-related disorder (1) that develops in some, but not all individuals exposed to death, threatened death, actual or threatened serious injury, or actual or threatened sexual violence. Its molecular underpinning is still not clear. Various potential stressful events or traumas happen to people during their lifetime, but different populations vary in their exposure to traumatic events (2, 3). PTSD commonly co-occurs with different somatic disorders (4), such as cardiovascular, metabolic, dermatological, musculoskeletal, and pulmonary diseases (5).
Metabolic Disturbances in PTSD
Metabolic complications and components of the metabolic syndrome are associated with increased body mass index (BMI) values or weight gain, and with other clusters of physiological, biochemical, clinical, and metabolic factors that strongly increase the risk of type 2 diabetes and different cardiovascular diseases (6). These metabolic abnormalities include central obesity measured as waist circumference, dyslipidaemia (reduced HDL levels and increased triglyceride levels), high blood pressure, insulin resistance, as well as raised fasting plasma glucose. People with a sedentary life style, who do not exercise, consume a high fat diet and develop these metabolic abnormalities, have increased risk for cardiovascular and metabolic disorders (6).
Metabolic syndrome is frequent in PTSD (7–14). Metabolic complications include insulin resistance (15), higher mean triglycerides, higher blood pressure and fasting glucose levels, and lower HDL values in individuals with PTSD than in subjects from the general population (8). Young US veterans with PTSD had BMI values in the overweight range, higher than controls (15). Similarly, Croatian veterans with combat related PTSD had more frequently comorbid cardiometabolic disorders than control subjects (5), and this population had a high prevalence of obesity (16). Therefore, there is an urgent need for establishing the potential biomarkers of metabolic disturbances in PTSD (17).
Brain-Derived Neurotrophic Factor
The most abundant neurotrophin in the central nervous system is brain-derived neurotrophic factor (BDNF) and it modulates neuronal differentiation, synapse formation, survival, support and function of neurons, brain neurotransmission, proliferation, long-term potentiation, and synaptic growth in the central nervous system (18). Due to its localization and expression in the limbic system, brain regions that are involved in the regulation of fear and stress responses, and its modulatory role in dopaminergic, serotonergic and glutamatergic synthesis, metabolism, neuronal activity and release (18, 19), it is not surprising that BDNF is involved in the development of different neuropsychiatric disorders, including PTSD (19–21).
BDNF Genetic Variants
In humans, BDNF is encoded by the BDNF gene which extends over 70 kb, located on chromosome 11, region p13–14 (22). There are hundreds of polymorphisms in the BDNF gene, however, the most frequently studied functional polymorphisms include the Val66Met single-nucleotide polymorphism (rs6265) in the coding exon (23), and the C270T single-nucleotide polymorphism (rs56164415), in the 5′-untranslated region (UTR) of the BDNF gene (24). The A (Met) allele, compared to the G (Val) allele, of the BDNF Val66Met is associated with disrupted cellular processing, trafficking and intracellular packaging of the pro-BDNF and reduced activity-dependent secretion of the mature BDNF (23, 25). The other polymorphism, BDNF C270T, in the BDNF 5'-non-coding region (26), may affect BDNF expression (27) and might lead to regionally specific quantitative BDNF disbalance in the brain (24). Consequently, both BDNF polymorphisms, BDNF Val66Met (19, 28) and BDNF C270T (24, 29) have been associated with different neuropsychiatric disorders.
BDNF and PTSD
In patients with PTSD, inconsistent findings were reported regarding peripheral BDNF levels: blood levels were reported to be decreased (30), unchanged (31, 32), or increased (15, 33, 34). However, a meta-analysis did not confirm a significant association between BDNF levels and PTSD (35), which is in line with no significant relationship between cerebrospinal fluid BDNF levels and PTSD (36). There are conflicting findings on the relationship between BDNF genetic variants and PTSD (21, 37–40). The association between BDNF Val66Met and PTSD was confirmed in only one study (40), while other studies failed to detect a significant association (41–45). The association between PTSD and the other C270T polymorphism in the BDNF gene was investigated in two individual case-control studies, producing opposite results: positive (46) as well as no (45) association.
BDNF and Metabolic Complications
The localization of BDNF in the hippocampus and the hypothalamus explains its moderating effects on energy metabolism, homeostasis, metabolic regulation (47, 48), weight control, fasting and feeding (49, 50). BDNF is located also in lungs, heart, spleen, gastrointestinal tract and liver, and it is involved in the development of cardiovascular and metabolic disorders, metabolic syndrome (51), and body weight gain (52, 53). In the blood, BDNF is stored mainly in platelets and released into plasma (54), but it is also synthesized and released from different cell types including cells from the cardiovascular system (55, 56), pancreatic beta cells, as well as cells from adipose tissue (57). BDNF exhibits an anorexigenic effect and suppresses food intake (48). A sedentary life style, overweight, obesity and lack of exercise are related to reduced BDNF signaling and increased activity of the sympathetic nervous system, decreased activity of the parasympathetic outflow, increased heart rate, blood pressure, elevated inflammation, and reduced gut motility (49).
There are conflicting data regarding an association of plasma or serum BDNF levels and metabolic indices. Reduced BDNF levels were detected in patients with type 2 diabetes (58), metabolic or coronary syndromes (57, 59, 60), non-obese and non-diabetic subjects with acute coronary syndrome (60), and in patients with angina pectoris (61). Increased BDNF levels were found in a population based study showing a positive association with increased risk for obesity, metabolic syndrome and coronary disease (51, 62). In contrast, in another study, subjects with or without metabolic syndrome had similar serum BDNF levels (63). A recent meta-analysis found lower BDNF levels associated with the presence of metabolic syndrome in healthy adult subjects (64). On the other hand, a longitudinal study including a large community-based cohort (65) detected higher serum BDNF levels significantly associated with lower risk of cardiovascular diseases and mortality, independent of markers of low-grade inflammation, BMI, physical activity, and depression.
BDNF gene variants were studied as risk factors for metabolic complications, such as BMI, dyslipidaemia, obesity, insulin resistance (66–68) and eating disorders (19). The A allele of the BDNF Val66Met polymorphism has been associated with higher BMI in adult women (69) and in young preschool children (70), but not in older healthy individuals (71). When these adult healthy groups were enlarged, a significant association was found between BMI categories and BDNF Val66Met (52, 72), since the A allele was more frequently found in the group with normal weight (52, 72–74). In conformation, obese subjects more frequently had the G allele of the BDNF Val66Met (73, 75). As far as we are aware, the reports on the association between the BDNF C270T polymorphism and BMI or plasma lipid levels in humans have not been studied.
In addition, to the best of our knowledge, there are no data on the association of BDNF Val66Met and BDNF C270T polymorphisms with metabolic indices in PTSD. Due to the high rates of both obesity and dyslipidaemia in PTSD, the aim of this study was to elucidate the association of BDNF Val66Met and BDNF C270T polymorphisms with BMI and lipid levels in veterans with PTSD. We hypothesized that BDNF variants might contribute to susceptibility to metabolic disturbances in PTSD and that these BDNF metabolic-risk variants might be more frequently present in patients with PTSD in comparison to healthy subjects or other diagnostic categories.
Methods
Participants
The study included 333 male veterans with combat related PTSD, with median Clinician Administered PTSD Scale (CAPS) scores of 86 (range 68–102). They were all unrelated Caucasian subjects of Croatian origin. The diagnosis of current and chronic PTSD was done using SCID based on DSM-5 criteria (1). Participants were sampled consecutively in the University Psychiatric Hospital Vrapce, Zagreb, from September 2015 to June 2017. They were exposed to similar potentially traumatic events during the Homeland war in Croatia. Inclusion criteria were in- and out-patients aged 38–77 years. Exclusion criteria were: drug abuse, alcohol dependence or pathophysiological changes in the liver, such as fibrosis, sclerosis, cirrhosis and malignant liver disease [alcoholic liver cirrhosis (K70.3), alcoholic liver fibrosis and sclerosis (K70.2) and hepatocellular carcinoma (C22.0), according to ICD-10], schizophrenia, bipolar disorder, adult ADHD, Alzheimer's disease (according to DSM-5 criteria), current or recent (previous 3 months) use of lipid-lowering agents, antihypertensive and antidiabetic medication. The study was approved by the Ethics Committee of the University Psychiatric Hospital Vrapce, Zagreb, Croatia, and was carried out in accordance with the Helsinki declaration (1975), as revised in 1983. All patients have signed informed consent prior to study procedures.
Anthropological Measures
Height of subjects wearing no shoes was measured with a meter to the nearest 0.5 cm; whereas body weight of subjects was measured with a digital scale to the nearest 0.1 kg. BMI was calculated as ratio of weight (kg) over height (m2).
Measurements of the Metabolic Indices
Blood samples were collected between 7:30 a.m. and 8:00 a.m. after overnight fasting. Total cholesterol (normal values < 5 mmol/l) was determined with cholesterol oxidase-phenol aminophenazone method and the absorbance read on a Siemens Dimension Xpand analyser. Triglycerides (normal values < 1.7 mmol/l), LDL (normal values < 3 mmol/l), and HDL (normal values >1.2 mmol/L) were determined using the enzymatic-colorimetric assay and were analyzed with Siemens Dimension Xpand analyser.
Genotyping
Genomic DNA was isolated from peripheral blood using a salting out method (76). BDNF Val66Met (rs6265) and C270T (rs56164415) were determined with TaqMan® Genotyping Assays (Applied Biosystems, Foster City, CA, USA) following the manufacturer's protocol on an Applied Biosystems® 7300 Real-Time PCR System apparatus. The 10 μL reaction volume contained around 20 ng of DNA. Assay IDs were C_11592758_10 for rs6265 and C_89097201_10 for rs56164415. Around 10% of randomly selected samples were genotyped again as a quality control for genotyping assays.
Minor allele frequency (MAF) for BDNF Val66Met in our sample was 19% (A allele), which is in accordance with the MAF of 20% (A allele) in the European population (77). In our European sample, MAF for BDNF C270T was 17% (T allele), while 1000 Genomes reports much smaller frequency of T allele (6%) in this population.
Since there were only 6 AA genotype carriers (of the BDNF Val66Met) and 3 TT genotype carriers (of the BDNF C270T) in the whole sample, we assessed only the dominant model for the BDNF Val66Met: A carriers (AA + AG) vs. GG homozygous genotype, and the dominant model for the BDNF C270T: T carriers (TT + TC) vs. CC homozygous genotype (78, 79).
Statistical Analysis
All data regarding lipid levels, BMI and age failed to reach normal distribution (Kolmogorov-Smirnov test). The data were expressed as median, 25th (Q1) and 75th (Q3) percentile after excluding outliers. Outliers were determined as values that lie below Q1–1.5 interquartile range (IQR) or above Q3+1.5 IQR. Hierarchical multiple linear regression was used to determine possible effect of age, smoking, BMI, BDNF Val66Met, and BDNF C270T on each metabolic parameter after excluding outliers. The data were evaluated with the non-parametric Mann-Whitney test for each metabolic parameter using Sigma Stat 3.5 (Jandell Scientific Corp. San Raphael, California, USA). For determination of the linkage disequilibrium (LD) between BDNF Val66Met and C270T loci, we used Haploview software v. 4.2 (80). Loci are considered to be in high LD if the D' coefficient is >0.80 and logarithm of odds (LOD) ≥ 2 (80, 81). In our study these two loci were not in high LD since the D′ coefficient was < 0.80 (D′ = 0.36; LOD = 0.32). Therefore, we did not perform haplotype analysis. Due to multiple (N = 10) comparisons (testing the association of 2 single nucleotide polymorphisms (SNPs) with 5 metabolic indices = 10), a Bonferroni correction was performed and p-value was set to p = 0.005. Before the study G*Power 3 Software (82) was used to determine the required sample size and actual statistical power. For hierarchical multiple linear regression, with p = 0.005; medium effect size = 0.25; and power (1 – β) = 0.800; number of predictors = 5; the required sample size was 142. For the Mann–Whitney test, with p = 0.005; medium effect size = 0.15; and power (1 – β) = 0.800; the required sample size was 217. Since the study included 333 participants in the beginning, and 294–311 participants after the removal of outliers, it had adequate sample size and statistical power to detect significant differences among the groups.
Results
Clinical Data
Demographic and clinical data, including age, levels of total, HDL and LDL cholesterol, triglyceride levels, and BMI, of veterans with PTSD are presented in Table 1. Initially, the study enrolled 333 subjects. However, after removal of the outliers for each metabolic parameter (values that were lower than Q1–1.5 IQR or higher Q3+1.5 IQR in our sample), there were N = 294 participants for HDL (removed HDL values ≤ 0.5 and HDL ≥ 2.0), N = 316 for BMI (removed BMI values ≤ 19); N = 315 for cholesterol (removed cholesterol values ≥8.4), N = 311 for LDL (removed LDL values ≥5.7 and LDL ≤ 0.2) and N = 303 for triglycerides (removed triglyceride values ≥3.8), respectively.
The Effect of Age, Smoking, BMI, and BDNF Polymorphisms on Metabolic Parameters
Hierarchical multiple linear regression was performed in order to assess the influence of various independent variables, such as age, smoking, BMI, and BDNF Val66Met and BDNF C270T on different metabolic parameters (dependent variables) (Table 2). It was designed in the following way: for all metabolic indices, except BMI, the first step included age, BMI, and smoking status as dependent variables, and the second step BDNF Val66Met genotype (dominant model) and BDNF C2790T genotype (dominant model). For BMI, age, and smoking were entered as dependent variables in the first step, while both genotypes were added in the second one. Hierarchical multiple regression revealed age as the only significant (p < 0.001) variable influencing HDL and triglycerides, while other variables were not significantly associated with metabolic indices due to Bonferroni corrected significance (p = 0.005).
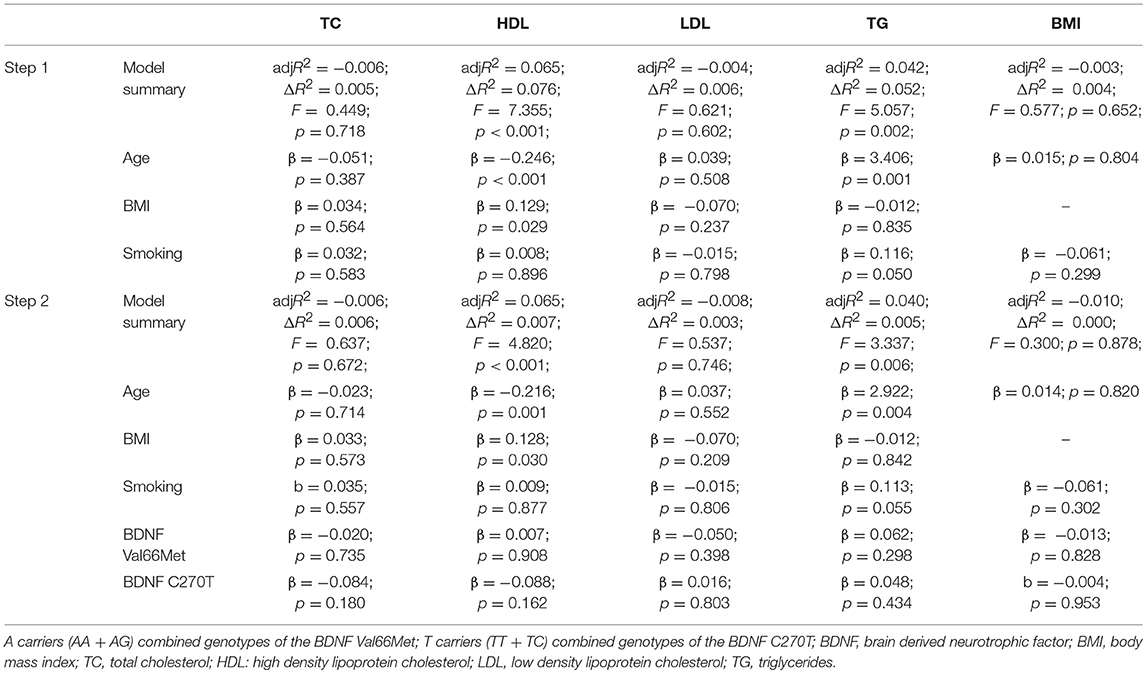
Table 2. Hierarchical multiple linear regression showing variables influencing different metabolic parameters.
BDNF Val66Met and BDNF C270T Polymorphisms and Metabolic Indices
Table 3 shows that metabolic parameters did not differ significantly between individuals with PTSD subdivided into A carriers (AA+AG) vs. homozygous GG genotype carriers of BDNF Val66Met, as well as between T carriers (TT+CT) vs. CC genotype carriers of the BDNF C270T. Namely, no significant differences between A carriers and GG homozygotes of the BDNF Val66Met polymorphism, were detected in the BMI values (p = 0.979), plasma concentrations of total (p = 0.933), HDL (p = 0.829), and LDL (p = 0.146) cholesterol, as well as triglycerides (p = 0.409). In the case of the BDNF C270T polymorphism, similar results were observed. The Mann Whitney test revealed that BMI (p = 0.822), plasma concentrations of total (p = 0.738) and LDL (p = 0.290) cholesterol, as well as triglyceride (p = 0.092) levels were similar in T carriers and CC homozygotes. However, HDL levels differed nominally (p = 0.006) between T carriers and CC homozygotes of the BDNF C270T polymorphism. Since this association was not confirmed using hierarchical multiple linear regression, the observed difference in HDL levels was presumably associated with the age differences between these groups.
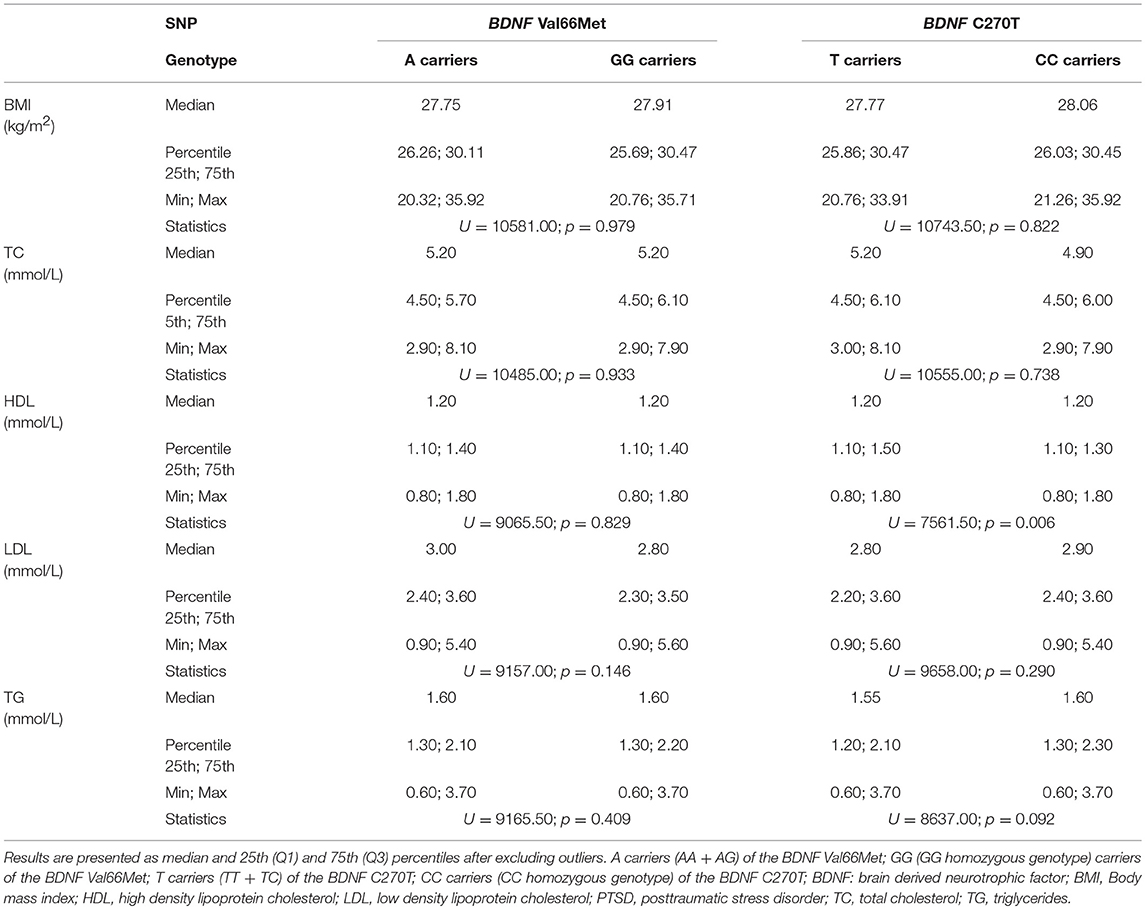
Table 3. The metabolic parameters of veterans with PTSD subdivided according to the BDNF Val66Met and BDNF C270T genetic variants.
Therefore, as age was a significant (p < 0.001) variable that affects HDL and triglycerides in the regression model, and HDL levels were nominally (p = 0.006) different between T carriers and CC homozygotes, to further evaluate this age-related, but also BDNF C270T -related influence, patients were additionally subdivided into 3 age groups. Since the age range of the patients was 38–77, we subdivided participants into approximately 13-year age groups, i.e., into individuals between the ages of 38 and 51 (N = 76 for HDL, and N = 74 for triglycerides after removing outliers), ages 52–65 (N = 162 for HDL, N = 171 for triglycerides), and ages 66–77 (N = 56 for HDL and N = 58 for triglycerides). The Mann-Whitney test demonstrated no significant (p > 0.005) differences between BDNF Val66Met A vs. GG genotype carriers, and between BDNF C270T T vs. CC genotype carriers in HDL and triglyceride levels (Table 4) in each age group. These results revealed that these two polymorphisms were not significantly associated with HDL and triglyceride levels in any of the age groups. Nevertheless, median HDL values (Table 4) showed a decline (as evidenced by the multiple linear regression analysis: β = −0.243) with increased age in both T and CC genotype carriers.
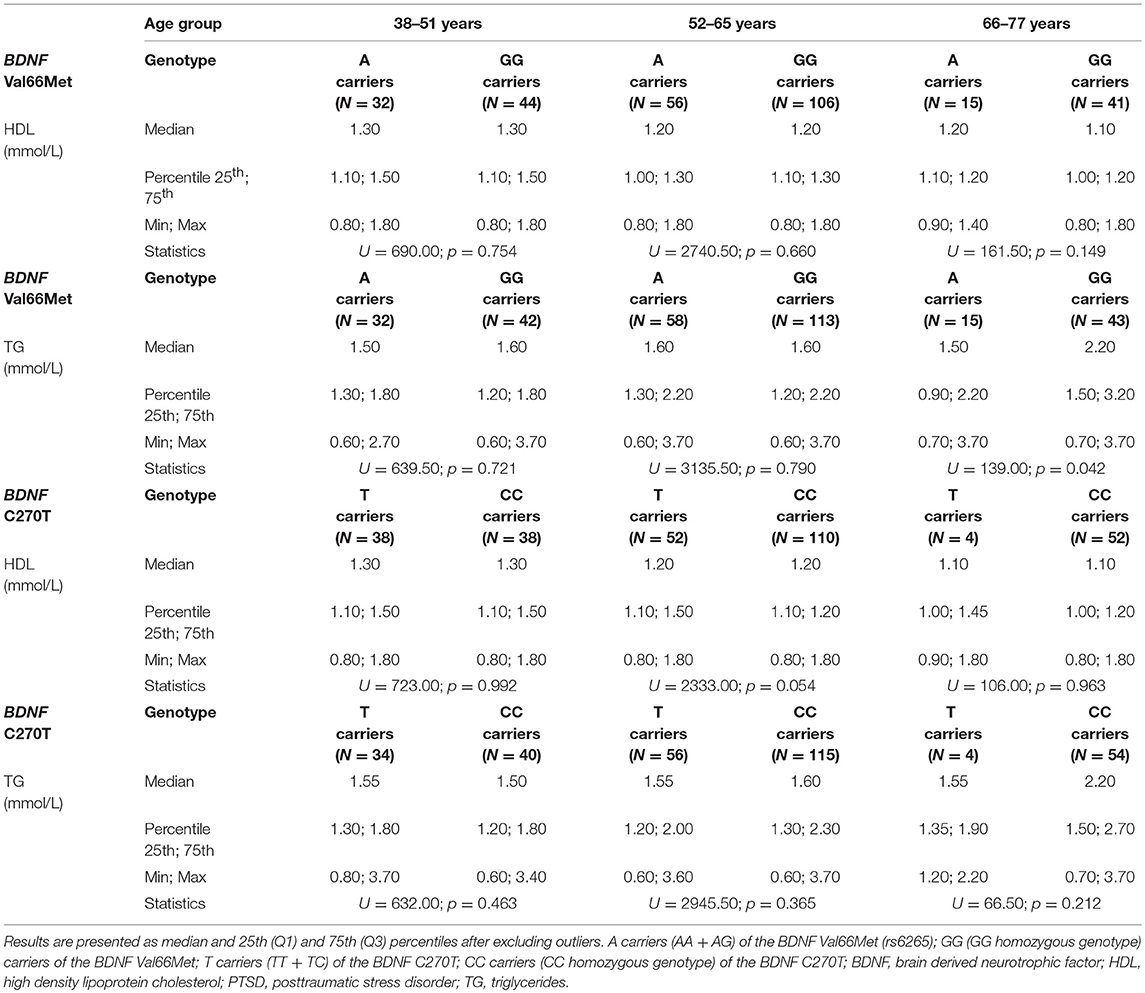
Table 4. The HDL and TG values in different age groups of veterans with PTSD subdivided according to the BDNF Val66Met and BDNF C270T genetic variants.
Discussion
The results of this study revealed that in a homogeneous sample of middle-aged Caucasian (Croatian origin) veterans with combat related PTSD: (1) BDNF Val66Met and BDNF C270T polymorphisms were not significantly associated with BMI or plasma lipid levels; (2) the presence of one or two T alleles of BDNF C270T polymorphism was related to slightly (nominally) higher HDL cholesterol values; but this association disappeared after controlling for the influence of age.
In the present study, veterans had chronic and current PTSD, and moderate PTSD symptoms, as revealed by their mean CAPS scores of 86 (range 68–102) (83). Their lipid levels were either slightly higher (total cholesterol and triglycerides) or within the normal laboratory range (i.e., for HDL and LDL cholesterol levels). Data from the literature regarding lipid levels in PTSD are inconsistent (84). Similar HDL and LDL cholesterol levels were found in different groups of Caucasian veterans with PTSD of the same origin (85), or in Japanese civilians with PTSD, who were victims of sarin poisoning (86). Further, HDL levels did not differ between civilian participants with and without PTSD (87, 88). Similarly, PTSD was associated with unchanged LDL cholesterol levels (89, 90). Other studies found dyslipidaemia, i.e., increased cholesterol, triglyceride, and LDL cholesterol levels in PTSD (88, 91–93). In contrast to our data, some studies have detected lower HDL cholesterol levels in PTSD (89, 90, 94), or similar triglyceride levels in large groups of community-living adults with or without PTSD (87). Further, cholesterol, LDL cholesterol and triglyceride levels did not differ between participants with PTSD compared to non-PTSD individuals (94). Dyslipidaemia and increased BMI are risk factors for various cardiovascular diseases and myocardial infarction in all subjects (6), especially individuals with PTSD (4, 8, 10, 13). Therefore, careful monitoring of these patients is warranted to prevent development of cardiometabolic disorders. The current investigation included patients with PTSD without cardiovascular diseases and the exclusion criteria included taking cardiovascular drugs or statins, but their mean BMI values of almost 28 suggest that the majority of participants were overweight. This is in line with data obtained with the different groups of Croatian veterans with PTSD (16) but also with data from a Croatian population sample (16), and with findings from young military US veterans with PTSD, who were all overweight (15). All these findings suggest that overweight, obesity and dyslipidaemia are becoming health risks in PTSD, but also global health problems in the general population. Various risk factors such as different diagnoses and sizes of the groups, civilian or military individuals, diet, food, sedentary life style and physical activity, age, exercise, alcohol dependence, and smoking might affect BMI and lipid levels. As this study did not intend to compare BMI and lipid levels between individuals with PTSD and control subjects, we may conclude that our results on the lipid levels and BMI are in line with most of the cited data.
There was one study showing positive association (40) and others reporting no associations (41–45) between BDNF Val66Met and PTSD. In our previous study that included different groups of war veterans (42), the frequency of the BDNF Val66Met genotypes did not differ between veterans with or without PTSD. Therefore, the present study with other groups of veterans with PTSD did not evaluate this possible association and did not include control subjects. The explanation for these inconsistent findings might be sought in the frequency of trauma exposure, traumatic load, early traumatic experience and stressful life events, age, and other study conditions among the participants (21).
We did not confirm our hypothesis that BDNF variants contributed to susceptibility to metabolic disturbances in PTSD. In our study BDNF Val66Met polymorphism was not significantly associated with metabolic indices in Caucasian veterans with PTSD. Namely, BMI values, as well as plasma total cholesterol, triglycerides, HDL and LDL cholesterol levels did not differ significantly in veterans with PTSD subdivided into carriers of the A allele (AA+AG) or the GG homozygous genotype of BDNF Val66Met polymorphism. As there are no such data in the literature, we might presume that this study is the first to show no association of BDNF Val66Met variants with BMI in combat veterans with PTSD. In line with our data, BMI was not significantly different in A carriers compared to GG genotype carriers of the BDNF Val66Met in elderly healthy Chinese subjects (95), or in elderly healthy individuals who were followed longitudinally (71). However, there are conflicting results in the literature regarding this polymorphism and BMI in control subjects. Opposing data were reported showing that the A allele was associated with higher BMI in healthy adult subjects (96), adult women (69), and in young preschool children (70). On the other hand, there are also data showing that A carriers were more frequently found in the group with lower BMI in a large Korean epidemiological cohort (74), in a large cohort of male Boston Puerto Rican subjects (67), adult healthy Caucasian women (73, 75), healthy control male and female subjects from the same population of Caucasian subjects of Croatian origin (52, 72), or in children and adolescents (97). A recent meta-analysis confirmed only one BDNF SNP, rs925946, significantly related to obesity and BMI in large groups of healthy subjects (98). Discrepancies in the results could be explained by the different diagnostic groups (PTSD vs. controls), ethnic background (19, 99, 100), age, gender, living environment, diet, lifestyle, and smoking (95). Since recently a strong LD between BDNF rs6265 and rs10501087 was reported, suggesting that these two SNPs are dependent genetic markers associated with BMI (98), in future studies this BDNF SNP, rs10501087, should be also evaluated.
Our study did not detect any significant association between BDNF Val66Met and lipid levels in PTSD. There are no findings in the literature regarding the association between BDNF Val66Met and lipid levels in PTSD. However, in volunteers without kidney and cardiovascular diseases, no association was found between BDNF Val66Met polymorphism and serum TG and HDL cholesterol levels (63). This finding agrees with the results from our study, since our veterans with PTSD, who did not have any cardiovascular or metabolic diseases, had similar plasma lipid levels when subdivided into carriers of different BDNF Val66Met variants. In children and adolescents from the general population, the BDNF Val66Met polymorphism was not associated with any of the lipid indices (97). In contrast to our study and cited (63, 97) findings, the presence of one or two A alleles of the BDNF Val66Met was significantly associated with lower HDL cholesterol levels and higher risk for obesity in old and very old Chinese healthy subjects (95). In that particular study subjects carrying the A allele had elevated triglyceride levels, but reduced HDL cholesterol levels compared to the GG genotype carriers (95). The differences between studies are in the diagnosis (PTSD vs. normal controls) and in the age of included subjects (i.e., Peng's study included three groups: age ≥90; age 60–77; and age 60–75, while our veterans were on average 56 years old). To control for the effect of age, in our study participants with PTSD were subdivided into different age groups (age range 38–51, 52–65, and 66–77 years), and their plasma HDL and triglyceride levels (that were significantly affected by age in the hierarchical regression analysis) did not differ between BDNF Val66Met A vs. GG genotype carriers and between BDNF C270T T vs. CC genotype carriers. In addition, the observed differences between studies might be due to ethnicity (Chinese vs. Caucasian subjects), since there were significant ethnic related differences in the frequency of the BDNF Val66Met genotypes, especially the frequency of the AA genotype, between Asian and Caucasian subjects (100).
Unlike BDNF Val66Met, the BDNF C270T polymorphism has only recently gained attention in studies of neuropsychiatric disorders (101). The T allele of the BDNF C270T polymorphism was reported to be a risk factor for PTSD (46), schizophrenia (29), bulimia nervosa (102), and amyotrophic lateral sclerosis (24), but not for Alzheimer's disease (78). The T allele prevalence in our study was 17% and it was the same as the T allele frequency in a Chinese sample with PTSD (46). This frequency differs significantly from the T allele frequency in our healthy Caucasian subjects (6%, unpublished data) and in the general East Asian population [6%, (77)] or in other diagnostic groups. Namely, in Caucasian patients with schizophrenia this frequency was around 8% (29), while in Caucasian patients with bulimia nervosa and control subjects it was around 5% (102). Higher prevalence of the T allele in our Caucasian subjects and in Chinese subjects with PTSD (46) compared to control subjects (102) and patients with schizophrenia (29) or bulimia (102), might be an outcome of different clinical diagnoses (PTSD vs. schizophrenia vs. bulimia nervosa/controls). This is also supported by the fact that no obvious ethnic differences in BDNF C270T genotype frequency were found in a recent meta-analysis (78) and reported by The 1000 Genomes Project Consortium (77), with the exception of South Asian populations (India, Bangladesh, Pakistan) where the T allele is present in 29% of population.
The BDNF C270T polymorphism was related to PTSD in one study, since the T allele frequency was significantly higher in a group with sporadic PTSD compared to a control group (46). Another study did not find a significant association, but this trial comprised only 96 subjects with PTSD (45). Since in our study we have not compared the frequency of the BDNF C270T genotypes in PTSD vs. controls, we cannot confirm or reject this finding.
This investigation has not found a significant association between BDNF C270T and BMI, total cholesterol, LDL cholesterol and triglyceride levels in veterans with PTSD. A slight association between BDNF C270T and HDL cholesterol in veterans with PTSD was detected, as carriers of the T alleles had nominally higher HDL cholesterol levels than CC carriers. This significance did not survive a Bonferroni correction, and was not confirmed by hierarchical multiple regression and further testing. Only HDL and triglyceride levels were affected by age. Other metabolic indices were not associated with age, smoking, BDNF Val66Met or BDNF C270T polymorphisms. To further evaluate these findings, HDL and triglyceride levels were evaluated in participants subdivided into 13-year age groups, i.e., into individuals in the age range from 38 to 51, from 52 to 65, and from 66 to 77 years, although we are aware of the limited power due to the stratification of data into smaller groups. HDL and triglyceride levels did not differ between BDNF Val66Met A allele carriers vs. GG genotype carriers, and between BDNF C270T T allele carriers vs. CC genotype carriers. As both BDNF C270T T allele carriers and CC genotype carriers showed reduced median HDL values in older age, these results confirmed that HDL was affected by age and not by the presence of the T allele.
There are no other data on the association of this BDNF C270T polymorphism and HDL cholesterol in PTSD, and there are no data showing either a positive association or no association between BDNF C270T polymorphism and BMI, total cholesterol, LDL cholesterol and triglyceride levels in PTSD. Although this was the first study to evaluate a link between BDNF C270T and metabolic indices in PTSD, no associations were found between either of the variants and these metabolic indices. One study reported that BDNF C270T polymorphism was not associated with BMI in patients with anorexia nervosa and bulimia nervosa (103), while another found a significant association between the T allele of the BDNF C270T polymorphism and lower BMI in bulimia nervosa (102). The discrepancies between these and our study might be explained by the differences in diagnoses (PTSD vs. eating disorders).
Our results did not confirm our hypothesis that BDNF Val66Met and C270T variants contribute to susceptibility to metabolic disturbances in PTSD. Lower HDL cholesterol values were detected in older individuals with PTSD, but were not associated with any of the BDNF Val66Met and C270T genotypes. Routine monitoring of individuals with PTSD, in terms of HDL cholesterol levels, might prevent possible cardiovascular events including myocardial infarction, that are frequent in PTSD (5, 17, 84).
Some limitations need to be considered in interpreting these results: the study included only Caucasian PTSD veterans, and all participants were males. Therefore, we could not compare our data with those in control subjects, and we could not evaluate possible ethnic and gender related differences. In this study we included only the two SNPs (BDNF Val66Met and C270T polymorphisms), so additional BDNF gene polymorphisms should be included in future studies, such as those evaluated in healthy control subjects: BDNF rs12291063 polymorphism associated with BMI and obesity (104), BDNF rs10767664 polymorphism associated with BMI, weight, fat mass, waist circumference, LDL cholesterol and total cholesterol (105), or BDNF rs925946 polymorphism associated with BMI (98), or GWAS data. Data on physical activity and eating habits were not collected. A significant limitation of this study is a lack of replication sample.
Advantages of the present study are the use of a fairly large group of veterans with PTSD, sampled from the same center, and in the fact that diagnosis and screening for PTSD was done by psychiatrists using SCID and CAPS. We also took into account alcohol dependence and use of statins and cardiovascular drugs, we removed outliers, carefully monitored the confounders by a hierarchical multiple linear regression, used a homogeneous group of Caucasians of Croatian origin with similar combat trauma exposure and ensured that the study had the required sample size and statistical power.
Conclusion
This is a first report showing that variants of the BDNF Val66Met and BDNF C270T polymorphisms were not associated with BMI or plasma lipid levels in veterans with PTSD. Our findings suggest that BDNF Val66Met and C270T variants do not contribute to susceptibility to metabolic disturbances in PTSD. Other metabolic indices and other BDNF and other gene variants should be evaluated as metabolic risk factors in PTSD in further larger multi-ethnic studies, including females, larger sample-sizes, with well-matched controls.
Author Contributions
NP developed the original idea. LT, MK, MNP, DSS, and GNE managed the experimental work, collected blood samples, isolated DNA and did the BDNF rs6265 and rs56164415 genotyping, and processed data for analysis. LT performed the statistical analysis. SU, OK, and ZKP explained the research goals and described protocol in details to the patients; explained the inclusion/exclusion criteria, insured participant adherence for the participation in the study, motivated, selected, diagnosed, evaluated and sampled patients with PTSD. NP did the data analysis and interpretation. NP and MS wrote the first and the final draft of the article. DSS wrote part of the article and revised the article. MNP and DSS did the proof reading of the manuscript. All authors contributed to the final version of the manuscript. All authors have revised the article and approved the final article.
Funding
This work has been supported by the Croatian Science Foundation, project No. IP-2014-09-4289 Glycogene-PTSD: Genomic and glycomic biomarkers for PTSD; PI: NP.
Conflict of Interest Statement
The authors declare that the research was conducted in the absence of any commercial or financial relationships that could be construed as a potential conflict of interest.
Acknowledgments
The authors thank Marija-Mary Sopta, Ph.D., Senior Research Associate, from Division of Molecular Biology, Rudjer Boskovic Institute, Zagreb, Croatia, for the English corrections and proofreading of the manuscript.
References
1. American Psychiatric Association. Diagnostic and Statistical Manual of Mental disorders - DSM-5. Washington, DC: American Psychiatric Association (2013).
2. Kessler RC, Aguilar-Gaxiola S, Alonso J, Benjet C, Bromet EJ, Cardoso G, et al. Trauma and PTSD in the WHO World Mental Health Surveys, Eur. J Psychotraumatol. (2017) 8:1353383. doi: 10.1080/20008198.2017
3. Liu H, Petukhova MV, Sampson NA, Aguilar-Gaxiola S, Alonso J, Andrade LH. Association of DSM-IV posttraumatic stress disorder with traumatic experience type and history in the World Health Organization World Mental Health Surveys. JAMA Psychiatry (2017) 74:270–81. doi: 10.1001/jamapsychiatry.2016.3783
4. Mellon SH, Gautam A, Hammamieh R, Jett M, Wolkowitz OM. Metabolism, metabolomics, and inflammation in post-traumatic stress disorder. Biol Psychiatry (2018) 83:866–75. doi: 10.1016/j.biopsych.2018.02.007
5. Britvic D, Anticevic V, Kaliterna M, Lusic L, Beg A, Brajevic-Gizdic I, et al. Comorbidities with PTSD among combat veterans; 15 years postwar analysis. Int J Clin Health Psychol. (2015) 15:81–92. doi: 10.1016/j.ijchp.2014.11.002
6. Kaur J. A comprehensive review on metabolic syndrome. Cardiol. Res. Pract. (2014) 2014:1–21, doi: 10.1155/2014/943162
7. Bartoli F, Carrà G, Crocamo C, Carretta D, Clerici M. Metabolic syndrome in people suffering from Posttraumatic Stress Disorder: a systematic review and meta-analysis. Metab Syndr Relat Disord. (2013) 11:301–8. doi: 10.1089/met.2013.0010
8. Heppner PS, Crawford EF, Haji UA, Afari N, Hauger RL, Dashevsky BA, et al. The association of posttraumatic stress disorder and metabolic syndrome: a study of increased health risk in veterans. BMC Med. (2009) 7:1. doi: 10.1186/1741-7015-7-1
9. Jakovljevic M, Babić D, Crncević Z, Martinac M, Maslov B, Topić R. Metabolic syndrome and depression in war veterans with post-traumatic stress disorder. Psychiatr Danub. (2008) 20:406–10.
10. Michopoulos V, Vester A, Neigh G. Posttraumatic stress disorder: a metabolic disorder in disguise?. Exp Neurol. (2016) 284:220–9. doi: 10.1016/j.expneurol.2016.05.038
11. Rosenbaum S, Stubbs B, Ward PB, Steel Z, Lederman O, Vancampfort D. The prevalence and risk of metabolic syndrome and its components among people with posttraumatic stress disorder: a systematic review and meta-analysis. Metabolism (2015) 64:926–33. doi: 10.1016/j.metabol.2015.04.009
12. Weiss T, Skelton K, Phifer J, Jovanovic T, Gillespie CF, Smith A, et al. posttraumatic stress disorder is a risk factor for metabolic syndrome in an impoverished urban population. Gen Hosp Psychiatry (2011) 33:135–42. doi: 10.1016/j.genhosppsych.2011.01.002
13. Wolf EJ, Bovin MJ, Green JD, Mitchell KS, Stoop TB, Barretto KM, et al. Longitudinal associations between posttraumatic stress disorder and metabolic syndrome severity. Psychol Med. (2016) 46:2215–26. doi: 10.1017/S0033291716000817
14. Wolf EJ, Miller DR, Logue MW, Sumner J, Stoop TB, Leritz EC, et al. Contributions of polygenic risk for obesity to PTSD-related metabolic syndrome and cortical thickness. Brain Behav Immun. (2017) 65:328–36. doi: 10.1016/j.bbi.2017.06.001
15. Blessing EM, Reus V, Mellon SH, Wolkowitz OM, Flory JD, Bierer L, et al. Biological predictors of insulin resistance associated with posttraumatic stress disorder in young military veterans. Psychoneuroendocrinology (2017) 82:91–7. doi: 10.1016/j.psyneuen.2017.04.016
16. Kozaric-Kovacic D, Ilic MG, Romic Z, Vidovic A, Jendricko T, Pivac N. Body mass index in male caucasian veterans with or without posttraumatic stress disorder. Prog Neuropsychopharmacol Biol Psychiatry (2009) 33:1447–50. doi: 10.1016/j.pnpbp.2009.07.026
17. Sagud M, Jaksic N, Vuksan-Cusa B, Loncar M, Loncar I, Mihaljevic Peles A, et al. Cardiovascular disease risk factors in patients with posttraumatic stress disorder (ptsd): a narrative review. Psychiatr Danub. (2017) 29:421–30. doi: 10.24869/psyd.2017.421
18. Russo-Neustadt A. Brain-derived neurotrophic factor, behavior, and new directions for the treatment of mental disorders. Semin Clin Neuropsychiatry (2003) 8:109–18. doi: 10.1053/scnp.2003.50014
19. Gratacos M, Gonzalez JR, Mercader JM, De Cid R, Urretavizcaya M, Estivill X. Brain-Derived Neurotrophic Factor Val66Met and psychiatric disorders: meta-analysis of case-control studies confirm association to substance-related disorders, eating disorders, and schizophrenia. Biol Psychiatry (2007) 61:911–22. doi: 10.1016/j.biopsych.2006.08.025
20. Hong CJ, Liou YJ, Tsai SJ. Effects of BDNF polymorphisms on brain function and behavior in health and disease. Brain Res Bull. (2011) 86:287–97. doi: 10.1016/j.brainresbull.2011.08.019
21. Zhang L, Li XX, Hu XZ. Post-traumatic stress disorder risk and brain-derived neurotrophic factor Val66Met. World J Psychiatry (2016) 6:1–6. doi: 10.5498/wjp.v6.i1.1
22. Cattaneo A, Cattane N, Begni V, Pariante CM, Riva MA. The human BDNF gene: peripheral gene expression and protein levels as biomarkers for psychiatric disorders. Transl Psychiatry (2016) 6:958. doi: 10.1038/tp.2016.214
23. Chen ZY, Patel PD, Sant G, Meng CX, Teng KK, Hempstead BL, et al. Variant brain-derived neurotrophic factor (BDNF) (Met66) alters the intracellular trafficking and activity-dependent secretion of wild-type BDNF in neurosecretory cells and cortical neurons. J Neurosci. (2004) 24:4401–11. doi: 10.1523/jneurosci.0348-04.2004
24. Xu L, Tian D, Li J, Chen L, Tang L, Fan D. The analysis of two BDNF polymorphisms G196A/C270T in Chinese sporadic amyotrophic lateral sclerosis. Front Aging Neurosci. (2017) 10:135. doi: 10.3389/fnagi.2017.00135
25. Egan MF, Kojima M, Callicott JH, Goldberg TE, Kolachana BS, Bertolino A, et al. The BDNF Val66Met polymorphism affects activity-dependent secretion of BDNF and human memory and hippocampal function. Cell (2003) 112:257–69. doi: 10.1016/S0092-8674(03)00035-7
26. Kunugi H, Ueki A, Otsuka M, Isse K, Hirasawa H, Kato N, et al. A novel polymorphism of the brain-derived neurotrophic factor (BDNF) gene associated with late-onset Alzheimer's disease. Mol Psychiatry (2001) 6:83–6. doi: 10.1038/sj.mp.4000792
27. Nagata T, Shinagawa S, Nukariya K, Ochiai Y, Kawamura S, Agawa-Ohta M, et al. Association between brain-derived neurotrophic factor (BDNF) gene polymorphisms and executive function in japanese patients with Alzheimer's Disease. Psychogeriatrics (2011) 11:141–9. doi: 10.1111/j.1479-8301.2011.00364.x
28. Notaras M, Hill R, van den Buuse M. The BDNF gene Val66Met polymorphism as a modifier of psychiatric disorder susceptibility: progress and controversy. Mol Psychiatry (2015) 20:916–30. doi: 10.1038/mp.2015.27
29. Watanabe Y, Nunokawa A, Someya T. Association of the BDNF C270T polymorphism with schizophrenia: updated meta-analysis. Psychiatry Clin Neurosci. (2013) 67:123–5. doi: 10.1111/pcn.12018
30. Dell'Osso L, Carmassi C, Del Debbio A, Dell'Osso MC, Bianchi C, da Pozzo E, et al. Brain Derived Neurotrophic Factor plasma levels in patients suffering from post-traumatic stress disorder. Prog Neuro-Psychopharmacol Biol Psychiatry (2009). 33:899–902. doi: 10.1016/j.pnpbp.2009.04.018
31. Su S, Xiao Z, Lin Z, Qiu Y, Jin Y, Wang Z. Plasma brain-derived neurotrophic factor levels in patients suffering from post-traumatic stress disorder. Psychiatry Res. (2015) 229:365–9. doi: 10.1016/j.psychres.2015.06.038
32. van den Heuvel L, Suliman S, Malan-Müller S, Hemmings S, Seedat S. Brain-derived neurotrophic factor (BDNF) Val66Met polymorphism and plasma levels in road traffic accident survivors. Anxiety Stress Coping (2016) 5806:1–33. doi: 10.1080/10615806.2016.1163545
33. Matsuoka Y, Nishi D, Noguchi H, Kim Y, Hashimoto K. Longitudinal changes in serum brain-derived neurotrophic factor in accident survivors with posttraumatic stress disorder. Neuropsychobiology (2013) 68:44–50. doi: 10.1159/000350950
34. Hauck S, Kapczinski F, Roesler R, de Moura Silveira E, Magalhães PV, Kruel LRP, et al. Serum brain-derived neurotrophic factor in patients with trauma psychopathology. Prog Neuro-Psychopharmacol Biol Psychiatry (2010) 34:459–62. doi: 10.1016/j.pnpbp.2010.01.010
35. Suliman S, Hemmings SM, Seedat S. Brain-derived neurotrophic factor (BDNF) protein levels in anxiety disorders: systematic review and meta-regression analysis. Front Integr Neurosci. (2013) 7:55. doi: 10.3389/fnint.2013.00055
36. Bonne O, Gill JM, Luckenbaugh DA, Collins C, Owens MJ, Alesci S, et al. Corticotropin-releasing factor, interleukin-6, brain derived neurotrophic factor, insulin-like growth factor-1, and substance p in the cerebrospinal fluid of civilians with posttraumatic stress disorder before and after treatment with paroxetine. J Clin Psychiatry (2011) 72:1124–8. doi: 10.4088/JCP.09m05106blu
37. Bountress KE, Bacanu SA, Tomko RL, Korte KJ, Hicks T, Sheerin C, et al. The effects of a BDNF Val66Met polymorphism on posttraumatic stress disorder: a meta-analysis. Neuropsychobiology (2018) 6:1–7. doi: 10.1159/000489407
38. Bruenig D, Lurie J, Morris CP, Harvey W, Lawford B, Young R, et al. A case-control study and meta-analysis reveal BDNF Val66Met is a possible risk factor for PTSD. Neural Plast. (2016) 2016:6979435. doi: 10.1155/2016/6979435
39. Wang Y, Zhang H, Li Y, Wang Z, Fan Q, Yu S, et al. BDNF Val66Met polymorphism and plasma levels in chinese han population with obsessive-compulsive disorder and generalized anxiety disorder. J Affect Disord. (2015) 186:7–12. doi: 10.1016/j.jad.2015.07.023
40. Zhang L, Benedek DM, Fullerton CS, Forsten RD, Naifeh JA, Li XX, et al. PTSD risk is associated with BDNF Val66Met and BDNF overexpression. Mol Psychiatry (2014) 19:8–10. doi: 10.1038/mp.2012.180
41. Lee HJ, Kang RH, Lim SW, Paik JW, Choi MJ, Lee MS. No association between the brain-derived neurotrophic factor gene Val66Met polymorphism and post-traumatic stress disorder. Stress Health (2006) 22:115–9. doi: 10.1002/ajmg.b.32122
42. Pivac N, Kozarić-Kovačić D, Grubišić-Ilić M, Nedić G, Rakoš I, Nikolac M, et al. The association between brain-derived neurotrophic factor Val66Met variants and psychotic symptoms in posttraumatic stress disorder. World J Biol Psychiatry (2012) 13:306–11. doi: 10.3109/15622975.2011.582883
43. Valente NLM, Vallada H, Cordeiro Q, Miguita K, Bressan RA, Andreoli SB, et al. Candidate-gene approach in posttraumatic stress disorder after urban violence: association analysis of the genes encoding serotonin transporter, dopamine transporter, and BDNF. J Mol Neurosci. (2011) 44:59–67. doi: 10.1007/s12031-011-9513-7
44. Wang T. Does BDNF Val66Met polymorphism confer risk for posttraumatic stress disorder? Neuropsychobiology (2015) 71:149–53. doi: 10.1159/000381352
45. Zhang H, Ozbay F, Lappalainen J, Kranzler HR, van Dyck CH, Charney DS, et al. Brain Derived Neurotrophic Factor (BDNF) gene variants and Alzheimer's disease, affective disorders, posttraumatic stress disorder, schizophrenia, and substance dependence. Am J Med Genet B Neuropsychiatr Genet. (2006) 141:387–93. doi: 10.1002/ajmg.b.30332
46. Guo JC, Yang YJ, Guo M, Wang XD, Juan Y, Gao YS, et al. Correlations of four genetic single nucleotide polymorphisms in brain-derived neurotrophic factor with posttraumatic stress disorder. Psychiatry Invest. (2018) 15:407–12. doi: 10.30773/pi.2017.06.17.1
47. Gómez-Pinilla F. Brain foods: the effects of nutrients on brain function. Nat Rev Neurosci. (2008) 9:568–78. doi: 10.1038/nrn2421
48. Rios M. BDNF and the central control of feeding: accidental bystander or essential player? Trends Neurosci. (2013) 36:83–90. doi: 10.1016/j.tins.2012.12.009
49. Marosi K, Mattson MP. BDNF mediates adaptive brain and body responses to energetic challenges. Trends Endocrinol Metab. (2014) 25:89–98. doi: 10.1016/j.tem.2013.10.006
50. Lebrun B, Bariohay B, Moyse E, Jean A. Brain-derived neurotrophic factor (BDNF) and food intake regulation: a minireview. Auton Neurosci. (2006) 126–127:30–38. doi: 10.1016/j.autneu.2006.02.027
51. Golden E, Emiliano A, Maudsley S, Windham BG, Carlson OD, Egan JM, et al. Circulating Brain-Derived Neurotrophic Factor and indices of metabolic and cardiovascular health: data from the baltimore longitudinal study of aging. PLoS ONE (2010) 5:e10099. doi: 10.1371/journal.pone.0010099
52. Svob Strac D, Nikolac, Perkovic M, Nedic, Erjavec G, Uzun S, Sagud M, Zivkovic M, et al. The role of brain-derived neurotrophic factor (BDNF) in cognition and treatment response. In: Bennet C, editor. Brain-Derived Neurotrophic Factor (BDNF): Therapeutic Approaches, Role in Neuronal Development and Effects on Cognitive Health (New York, NY: Nova Sci. Publ.) (2015). p. 67–146.
53. Briana DD, Malamitsi-Puchner A. Developmental origins of adult health and disease: the metabolic role of BDNF from early life to adulthood. Metab Clin Exp. (2018) 81:45–51. doi: 10.1016/j.metabol.2017.11.019
54. Fujimura H, Altar CA, Chen R, Nakamura T, Nakahashi T, Kambayashi J, et al. Brain-Derived Neurotrophic Factor is stored in human platelets and released by agonist stimulation. Thromb Haemost. (2002) 87:728–34. doi: 10.1055/s-0037-1613072
55. Cai D, Holm JM, Duignan IJ, Zheng J, Xaymardan M, Chin A, et al. BDNF-mediated enhancement of inflammation and injury in the aging heart. Physiol Genom. (2006) 24:191–7. doi: 10.1152/physiolgenomics.00165.2005
56. Tasci I, Kabul HK, Aydogdu A. Brain derived neurotrophic factor (BDNF) in cardiometabolic physiology and diseases. Anadolu Kardiyol Der. (2012) 12:684–8. doi: 10.5152/akd.2012.221
57. Yanev S, Aloe L, Fiore F, Chaldakov GN. Neurotrophic and metabotrophic potential of nerve growth factor and brain-derived neurotrophic factor: linking cardiometabolic and neuropsychiatric diseases. World J Pharmacol. (2013) 2:92–9. doi: 10.5497/wjp.v2.i4.92
58. Krabbe KS1, Nielsen AR, Krogh-Madsen R, Plomgaard P, Rasmussen P, Erikstrup C, et al. Brain-derived neurotrophic factor (BDNF) and type 2 diabetes. Diabetologia (2007) 50:431–8. doi: 10.1007/s00125-006-0537-4
59. Chaldakov GN, Fiore M, Stankulov IS, Hristova M, Antonelli A, Manni L, et al. NGF, BDNF, leptin, and mast cells in human coronary atherosclerosis and metabolic syndrome. Arch Physiol Biochem. (2001) 109:357–60. doi: 10.1076/apab.109.4.357.4249
60. Manni L, Nikolova V, Vyagova D, Chaldakov GN, Aloe L. Reduced plasma levels of NGF and BDNF in patients with acute coronary syndromes. Int J Cardiol. (2005) 102:169–71. doi: 10.1016/j.ijcard.2004.10.041
61. Jiang H, Liu Y, Zhang Y, Chen ZY. Association of plasma brain-derived neurotrophic factor and cardiovascular risk factors and prognosis in angina pectoris. Biochem Biophys Res Commun. (2011) 415:99–103. doi: 10.1016/j.bbrc.2011.10.020
62. Jung SH, Kim J, Davis JM, Blair SN, Cho HC. Association among basal serum bdnf, cardiorespiratory fitness and cardiovascular disease risk factors in untrained healthy Korean men. Eur J Appl Physiol. (2011) 111:303–11. doi: 10.1007/s00421-010-1658-5
63. Suriyaprom K, Tungtrongchitr R, Thawnasom K. Measurement of the levels of leptin, BDNF associated with polymorphisms LEP G2548A, LEPR Gln223Arg and BDNF Val66Met in Thai with metabolic syndrome. Diabetol Metab Syndr. (2014) 6:6. doi: 10.1186/1758-5996-6-6
64. Kavya K, Arul Kumaran KSG, Thomas A, Rao, NV, Mathews KA, Mangathayaru K. A study of the association between brain derived neurotrophic factor and metabolic syndrome: a preliminary systematic review and meta-analysis of case-control studies. Sri Ramachandra J Med. 8:1.
65. Kaess BM, Preis SR, Lieb W, Beiser AS, Yang Q, Chen TC, et al. Circulating brain-derived neurotrophic factor concentrations and the risk of cardiovascular disease in the community. J Am Heart Assoc. (2015) 4:3. doi: 10.1161/JAHA.114.001544
66. Friedel S, Horro FF, Wermter AK, Geller F, Dempfle AK, Reichwald J, et al. Mutation screen of the brain derived neurotrophic factor gene (BDNF): identification of several genetic variants and association studies in patients with obesity, eating disorders, and attention-deficit/hyperactivity disorder. Am J Med Genet B Neuropsychiatr Genet. (2005) 132:96–9. doi: 10.1002/ajmg.b.30090
67. Ma XY, Qiu WQ, Smith CE, Parnell LD, Jiang ZY, Ordovas JM, et al. Association between BDNF rs6265 and obesity in the Boston Puerto Rican Health Study. J Obes. (2012) 2012:102942. doi: 10.1155/2012/102942
68. Wu L, Xi B, Zhang M, Shen Y, Zhao X, Cheng H, et al. Associations of six single nucleotide polymorphisms in obesity-related genes with BMI and risk of obesity in chinese children. Diabetes (2010) 59:3085–9. doi: 10.2337/db10-0273
69. Beckers S, Peeters A, Zegers D, Mertens I, Van Gaal L, Van Hul W. Association of the BDNF Val66Met variation with obesity in women. Mol Genet Metab. (2008) 95:110–2. doi: 10.1016/j.ymgme.2008.06.008
70. Skledar M, Nikolac M, Dodig-Curkovic K, Curkovic M, Borovecki F, Pivac N. Association between brain-derived neurotrophic factor Val66Met and obesity in children and adolescents. Progr Neuro-Psychopharmacol Biol Psychiatry (2012) 36:136–40. doi: 10.1016/j.pnpbp.2011.08.003
71. Nikolac Perkovic M, Mustapic M, Pavlovic M, Uzun S, Kozumplik O, Barisic I, et al. Lack of association between brain-derived neurotrophic factor val66met polymorphism and body mass index change over time in healthy adults. Neurosci Lett. (2013) 545:127–31. doi: 10.1016/j.neulet.2013.04.036
72. Sustar A, Nikolac Perkovic M, Nedic Erjavec G, Svob Strac D, Pivac N. A protective effect of the BDNF Met/Met genotype in obesity in healthy caucasian subjects but not in patients with coronary heart disease. Eur Rev Med Pharmacol Sci. (2016) 20:3417–26.
73. Gunstad J, Schofield P, Paul RH, Spitznagel MB, Cohen RA, Williams LM, et al. BDNF Val66Met polymorphism is associated with body mass index in healthy adults. Neuropsychobiology (2006) 53:153–6. doi: 10.1159/000093341
74. Hong KW, Lim JE, Go MJ, Shin Cho Y, Ahn Y, Han BG, et al. Recapitulation of the association of the Val66Met polymorphism of BDNF gene with BMI in Koreans. Obesity (2012) 20:1871–5. doi: 10.1038/oby.2011.352
75. Shugart YY, Chen L, Day IN, Lewis SJ, Timpson NJ, Yuan W, et al. Two british women studies replicated the association between the Val66Met polymorphism in the brain-derived neurotrophic factor (BDNF) and BMI, Eur. J Hum Genet. (2009) 17:1050–5. doi: 10.1038/ejhg.2008.272
76. Miller SA, Dykes DD, Polesky HF. A simple salting out procedure for extracting DNA from human nucleated cells. Nucleic Acids Res. (1988) 16:1215. doi: 10.1093/nar/16.3.1215
77. The 1000 Genomes Project Consortium (2015). A global reference for human genetic variation, Nature 526:68–74 doi: 10.1038/nature15393
78. Shovit R, Praveen KS. Association of brain-derived neurotrophic factor (BDNF) gene SNPs G196A and C270T with Alzheimer's Disease: a meta-analysis. J Alzheimers Dis Parkinson (2017) 7:323. doi: 10.4172/2161-0460.1000323
79. Shovit R, Praveen KS. Association of Brain-derived Neurotrophic Factor (BDNF) gene SNPs G196A and C270T with Parkinson's disease: a meta-analysis. Biomed J Sci Tech Res. (2018) 6:2018. doi: 10.26717/BJSTR.2018.06.001314
80. Barrett JC, Fry B, Maller J, Daly MJ. Haploview: analysis and visualization of LD and Haplotype Maps. Bioinformatics (2005) 21:263–5. doi: 10.1093/bioinformatics/bth457
81. Gabriel SB, Schaffner SF, Nguyen H, Moore JM, Roy J, Blumenstiel B, et al. The structure of haplotype blocks in the human genome. Science (2002) 296:2225–9. doi: 10.1126/science.1069424
82. Faul F, Erdfelder E, Buchner A, Lang AG. Statistical power analyses using G*Power 3.1: tests for correlation and regression analyses. Behav Res Methods (2009) 41:1149–60. doi: 10.3758/BRM.41.4.1149
83. Weathers FW, Blake DD, Schnurr PP, Kaloupek DG, Marx BP, Keane TM,. The Clinician-Administered PTSD Scale for DSM-5 (CAPS-5). National Center for PTSD (2013). Available online at: www.ptsd.va.gov
84. Von Känel R, Kraemer B, Saner H, Schmid JP, Abbas CC, Begré S. (2010). Posttraumatic stress disorder and dyslipidemia: previous research and novel findings from patients with PTSD caused by myocardial infarction. World J Biol Psychiatry 11:141–7. doi: 10.3109/15622970903449846
85. Jendricko T, Vidovic A, Grubisic-Ilic M, Romic Z, Kovacic Z, Kozaric-Kovacic D. Homocysteine and serum lipids concentration in male war veterans with posttraumatic stress disorder. Prog Neuro-Psychopharmacol Biol Psychiatry (2009) 33:134–40. doi: 10.1016/j.pnpbp.2008.11.002
86. Tochigi M, Umekage T, Otani T, Kato T, Iwanami A, Asukai N, et al. Serum cholesterol, uric acid and cholinesterase in victims of the Tokyo subway sarin poisoning: a relation with post-traumatic stress disorder. Neurosci Res. (2002) 44:267–72. doi: 10.1016/S0168-0102(02)00146-3
87. Spitzer C, Barnow S, Volzke H, Wallaschofski H, John U, Freyberger HJ, et al. Association of posttraumatic stress disorder with low-grade elevation of c reactive protein: evidence from the general population. J Psychiatr Res. (2010) 44:15–21. doi: 10.1016/j.jpsychires.2009.06.002
88. Talbot LS, Rao MN, Cohen BE, Richards A, Inslicht SS, O'Donovan A, et al. Metabolic risk factors and posttraumatic stress disorder: the role of sleep in young, healthy adults. Psychosom Med. (2015) 77:383–91. doi: 10.1097/PSY.0000000000000176
89. Dennis PA, Ulmer CS, Calhoun PS, Sherwood A, Watkins LL, Dennis MF, et al. Behavioral health mediators of the link between posttraumatic stress disorder and dyslipidemia. J Psychosom Res. (2014) 77:45–50. doi: 10.1016/j.jpsychores.2014.05.001
90. Hamazaki K, Nishi D, Yonemoto N, Noguchi H, Kim Y, Matsuoka Y. The role of high-density lipoprotein cholesterol in risk for posttraumatic stress disorder: taking a nutritional approach toward universal prevention. Eur Psychiatry (2014) 29:408–13. doi: 10.1016/j.eurpsy.2014.05.002
91. Kagan BL, Leskin G, Haas B, Wilkins J, Foy D. Elevated lipid levels in vietnam veterans with chronic posttraumatic stress disorder. Biol Psychiatry (1999) 45:374–7. doi: 10.1016/S0006-3223(98)00059-6
92. Karlovic D, Buljan D, Martinac M, Marcinko D. Serum lipid concentrations in croatian veterans with post-traumatic stress disorder, post-traumatic stress disorder comorbid with major depressive disorder, or major depressive disorder. J Korean Med Sci. (2004) 19:431–6. doi: 10.3346/jkms.2004.19.3.431
93. Dzubur Kulenovic A, Kucukalic A, Malec D. Changes in plasma lipid concentrations and risk of coronary artery disease in army veterans suffering from chronic posttraumatic stress disorder. Croat Med J. (2008) 49:506–14. doi: 10.3325/cmj.2008.4.506
94. Tae H, Huh HJ, Hwang J, Chae JH. Relationship between serum lipid concentrations and posttraumatic stress symptoms in the bereaved after the Sewol ferry disaster: a prospective cohort study. Psychiatry Res. (2018) 266:132–7. doi: 10.1016/j.psychres.2018.04.058
95. Peng JH, Liu CW, Pan SL, Wu HY, Liang QH, Gan RJ, et al. Potential unfavorable impacts of BDNF Val66Met polymorphisms on metabolic risks in average population in a longevous area. BMC Geriatr. (2017) 17:4. doi: 10.1186/s12877-016-0393-0
96. Thorleifsson G, Walters GB, Gudbjartsson DF, Steinthorsdottir V, Sulem P, Helgadottir A, et al. Genome-wide association yields new sequence variants at seven loci that associate with measures of obesity Nat Genet. (2009) 41:18–24. doi: 10.1038/ng.274
97. Kalenda A, Landgraf K, Löffler D, Kovacs P, Kiess W, Körner A. The BDNF Val66Met polymorphism is associated with lower BMI, lower postprandial glucose levels and elevated carbohydrate intake in children and adolescents. Pediatr Obes. (2018) 13:159–67. doi: 10.1111/ijpo.12238
98. Akbarian SA, Salehi-Abargouei A, Pourmasoumi M, Kelishadi R, Nikpour P, Heidari-Beni M. Association of Brain-Derived Neurotrophic Factor gene polymorphisms with body mass index: a systematic review and meta-analysis. Adv Med Sci. (2018) 63:43–56. doi: 10.1016/j.advms.2017.07.002
99. Petryshen TL, Sabeti PC, Aldinger KA, Fry B, Fan JB, Schaffner SF, et al. Population genetic study of the brain-derived neurotrophic factor (BDNF) gene. Mol Psychiatry (2010) 15:810–5. doi: 10.1038/mp.2009.24
100. Pivac N, Kim B, Nedic G, Joo YH, Kozaric-Kovacic D, Hong JP, et al. Ethnic differences in the brain-derived neurotrophic factor Val66Met polymorphism in Croatian and Korean healthy subjects. Croat Med J. (2009) 50:43–8. doi: 10.3325/cmj.2009.50.43
101. Autry AE, Monteggia LM. Brain-derived neurotrophic factor and neuropsychiatric disorders. Pharmacol Rev. (2012) 64:238–58. doi: 10.1124/pr.111.005108
102. Ribases M, Gratacòs M, Fernández-Aranda F, Bellodi L, Boni C, Anderluh M, et al. Association of BDNF with anorexia, bulimia and age of onset of weight loss in six European populations. Hum Mol Genet. (2004) 13:1205–12. doi: 10.1093/hmg/ddh137
103. Yilmaz Z, Kaplan AS, Tiwari AK, Levitan RD, Piran S, Bergen AW, et al. The Role of leptin, melanocortin, and neurotrophin system genes on body weight in anorexia nervosa and bulimia nervosa. J Psychiatr Res. (2014) 55:77–86. doi: 10.1016/j.jpsychires.2014.04.005
104. Mou Z, Hyde TM, Lipska BK, Martinowich K, Wei P, Ong CJ, et al. Human obesity associated with an intronic SNP in the brain-derived neurotrophic factor locus. Cell Rep. (2015) 13:1073–80. doi: 10.1016/j.celrep.2015.09.065
105. de Luis DA, Romero E, Izaola O, Primo D, Aller R. Cardiovascular risk factors and insulin resistance after two hypocaloric diets with different fat distribution in obese subjects: effect of the rs10767664 gene variant in brain-derived neurotrophic factor. J Nutrigenet Nutrigenom. (2017) 10:163–71. doi: 10.1159/000485248
Keywords: BDNF, Val66Met, C270T, BMI, plasma lipid levels, metabolic indices, PTSD
Citation: Tudor L, Konjevod M, Nikolac Perkovic M, Svob Strac D, Nedic Erjavec G, Uzun S, Kozumplik O, Sagud M, Kovacic Petrovic Z and Pivac N (2018) Genetic Variants of the Brain-Derived Neurotrophic Factor and Metabolic Indices in Veterans With Posttraumatic Stress Disorder. Front. Psychiatry 9:637. doi: 10.3389/fpsyt.2018.00637
Received: 18 July 2018; Accepted: 08 November 2018;
Published: 27 November 2018.
Edited by:
Richard Eugene Frye, Phoenix Children's Hospital, United StatesReviewed by:
Erika J. Wolf, VA Boston Healthcare System, United StatesSian Hemmings, Stellenbosch University, South Africa
Stefania Schiavone, University of Foggia, Italy
Copyright © 2018 Tudor, Konjevod, Nikolac Perkovic, Svob Strac, Nedic Erjavec, Uzun, Kozumplik, Sagud, Kovacic Petrovic and Pivac. This is an open-access article distributed under the terms of the Creative Commons Attribution License (CC BY). The use, distribution or reproduction in other forums is permitted, provided the original author(s) and the copyright owner(s) are credited and that the original publication in this journal is cited, in accordance with accepted academic practice. No use, distribution or reproduction is permitted which does not comply with these terms.
*Correspondence: Nela Pivac, bnBpdmFjQGlyYi5ocg==