- 1Department of Psychiatry & Behavioural Neurosciences, McMaster University, Hamilton, ON, Canada
- 2Department of Psychiatry, St. Michael's Hospital, Toronto, ON, Canada
Advances in understanding the role of the microbiome in physical and mental health are at the forefront of medical research and hold potential to have a direct impact on precision medicine approaches. In the past 7 years, we have studied the role of microbiota-brain communication on behavior in mouse models using germ-free mice, mice exposed to antibiotics, and healthy specific pathogen free mice. Through our work and that of others, we have seen an amazing increase in our knowledge of how bacteria signal to the brain and the implications this has for psychiatry. Gut microbiota composition and function are influenced both by genetics, age, sex, diet, life experiences, and many other factors of psychiatric and bodily disorders and thus may act as potential biomarkers of the gut-brain axis that could be used in psychiatry and co-morbid conditions. There is a particular need in major depressive disorder and other mental illness to identify biomarkers that can stratify patients into more homogeneous groups to provide better treatment and for development of new therapeutic approaches. Peripheral outcome measures of host-microbe bidirectional communication have significant translational value as biomarkers. Enabling stratification of clinical populations, based on individual biological differences, to predict treatment response to pharmacological and non-pharmacological interventions. Here we consider the links between co-morbid metabolic syndrome and depression, focusing on biomarkers including leptin and ghrelin in combination with assessing gut microbiota composition, as a potential tool to help identify individual differences in depressed population.
Major depressive disorder (MDD) is a debilitating disorder that affects nearly 15% of the general population and accounts for the greatest disability burden of any disease (1). One of the major challenges in treating MDD is the lack of understanding of the underlying etiology of the disorder. The clinical heterogeneity observed in MDD makes it difficult to select the best treatment approach for an individual (2). Additionally, upwards of 60% of MDD patients will experience at least some form of treatment resistance over the course of the disease (3), with only one third of MDD suffers achieving remission even with optimal pharmacological and patient treatment (4). The onset and progression of depression is thought to result from a complex combination of genetic, environmental and neurochemical factors that differ considerably across patient populations such that the search for robust biomarkers to both characterize depression subtypes and predict treatment response is at the forefront of clinical psychiatry (5, 6).
Recent work over the last two decades has revealed the bidirectional communication between the central nervous system, enteric nervous system and the gastrointestinal tract, often referred to as the gut brain axis (7–12). Emerging evidence now supports the role of the gut microbiota in influencing behavior with specific links to MDD. The diverse population of the gut microbiota has been shown to be heterogeneous between individuals (13, 14), with dominate factors, such as diet (15), environment and host genetics (16–18) shaping the overall composition and function. Heterogeneity of depression has limited the success of efforts aimed at identifying clinical markers of treatment response and clinical subtypes. Treatment and diagnosis of MDD is also complicated by increasingly common comorbidities, resulting in another layer of heterogeneity in the MDD population. Therefore, the identification of robust biomarkers to both identify individual differences within the MDD population, as well as stratify patients into more homogenous subgroups is of critical importance. This approach is utilized by the Canadian Biomarker Integration Network in Depression (www.canbind.ca) that aims to shorten the time that it takes to match patients with the best treatment. By combining multiple molecular markers that can be measured in blood with clinical, imaging, or EEG researchers can identify and link biomarkers to clinical presentation and help predict treatment response (5).
Metabolic syndrome (MetS) is a well-documented in MDD patients, with the risk of MetS in depressed patient at 1.5 times higher than in the non-depressed population (19). Moreover, the prevalence of MetS is 58% higher in psychiatric patients than in the general population (20), with prominent anorexigenic and orexigenic hormones leptin and ghrelin identified to be associated with psychiatric disorders including schizophrenia, bipolar disorder and major depression (21–23). A role for the microbiome in metabolic syndrome has received attention, with studies demonstrating a role for gut microbiota in features of MetS, such as obesity, diabetes, dyslipidemia and hypertension (24, 25). Here we consider the literature related to leptin and ghrelin in MDD and how these molecular markers in combination with gut microbiota have potential to identify individual differences in patients and provide measures of the gut brain axis in MDD.
Microbiota-Brain Interactions Are Important in Depression
Research focused on the gut brain axis has gained momentum in recent years and garnered attention from both the scientific community, the public, and the media, with particular interest in understanding how microbes may influence mood. Accumulating evidence from preclinical work in rodents supports a connection between stress, microbiota, and stress-related behaviors (26–36), however, only a handful of studies have examined gut bacteria in individuals with major depressive disorder (7–9, 37–39). Using 16S rRNA gene sequencing, the composition of fecal microbiota in depressed patients was shown to be different from control samples (Table 1). The specific taxa differences observed in these studies varied, in part related to differences in sample size and analytical methods, but also related to the heterogeneity in the clinical populations recruited including age, BMI, smoking status, medication, clinical features, and severity of disease (8, 9, 37, 38). Inter-individual differences in microbiota composition in healthy individuals is ~90% (13, 14). Understanding how individual differences in microbiota influences individual differences in health and disease including MDD and other psychiatric disorders is needed.
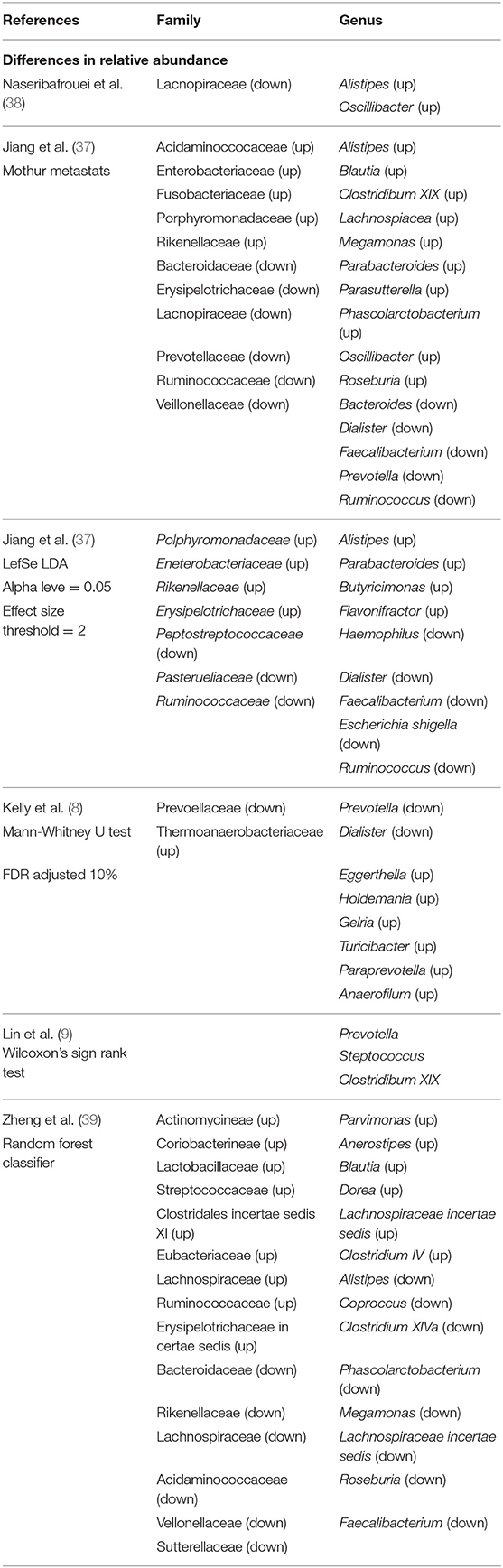
Table 1. Bacterial taxa differences at the family and genus level observed in individuals with major depressive disorder.
The compositional data gained from utilizing 16S rRNA gene sequencing in the MDD population represents an accessible biomarker, that provides both compositional data and, with recent advances in bioinformatics, can contribute functional information (40). The potential therapeutic benefits of treatments that target the microbiome, including probiotic and prebiotic administration, have begun to gain creditability for the treatment of psychiatric disorders (41) and the term psychobiotics is commonly used to classify products, such as probiotics and prebiotics, that when given in adequate amounts produce positive psychological effects (42, 43). Several studies show a benefit of probiotic consumption in healthy individuals including improved mood (44), a beneficial effect on anxiety and depressive measures as well as reduced stress hormone levels (45). Less work has been conducted in MDD clinical populations (46), and work to date has not utilized gut-brain biomarkers to identify subgroups within MDD. Metabolism and metabolic markers are of interest in the microbiome field. Here we selected two well-known metabolic markers that have been well-studied in MDD populations and consider their potential as biomarkers in MDD.
Leptin as a Potential Biomarker of Gut-Brain Interactions in MDD
Leptin is an adipocyte derived hormone, with a known role in regulating fat mass storage and energy homeostasis. Leptin circulates as a 16 kDa protein, where it crosses the blood brain barrier (BBB) and interacts with multiple regions of the brain including the hypothalamus and hippocampus (47, 48). Over the last decade there has been increasing evidence of leptin's role in regulating mood (23). Work in animal models has revealed a complex role of circulating leptin along with leptin's receptor (lepR) expression levels throughout the brain. Deletion of the lepR in the hippocampus of rats results in depressive behavior (49), as well as inhibits the behavioral effect of serotonin reuptake inhibitor fluoxetine (50). Animal models of chronic stress have been found to reduce circulating leptin, as well as reliably produce depressive behavior. Systematic injection of leptin has been found to have a dose dependent reduction in depressive behaviors in chronically stress mice (51, 52). A study aimed at exploring the relationship between obesity and depression found that, a combination of diet induced obesity and chronic unpredictable mild stress (CUMS) resulted in increased leptin levels but a decrease in LepR expression, along with depressive behaviors (53). The disparity in leptin serum level and receptor expression may be more representative of what occurs in an obese human and may be contributing to the comorbidity of obesity and MDD.
Human studies examining leptin levels in MDD have been mixed, some finding elevated leptin in MDD (54, 55) while others finding decreased levels (56, 57). This may be due to the varying role of leptin in lean verse obese conditions but also represents how we expect a biomarker of individual differences to present in a clinical population. Under lean conditions leptin acts as an anti-obesity hormone, signaling through activation of leptin receptors at the hypothalamus to reduce feeding behavior (58). Obesity is characterized by an increase in circulating leptin and a decrease in leptin receptor expression, leading to leptin resistance and disrupted leptin signaling (59). Concurrently, alterations to appetite, as well as weight changes are known clinical features of MDD (2). Interestingly, two meta-analyses have found a significant association of elevated leptin with depression only when controlling for BMI (60, 61). There is also evidence to support the association between atypical features of depression, such as increase appetite and hyposomnia with elevated leptin levels (62, 63). A recent study aimed at identifying subgroups of depression, found that grouping un-medicated patients by increases or decreases in appetite revealed dramatic differences in metabolic signaling, immune signaling and functional brain activity differences (64). Leptin levels were significantly increased in MDD patients with increased appetite, compared to healthy controls or when compared to MDD individuals with decreased appetite, an observation that was not related to BMI (64). The increased appetite subgroup also had alteration to proinflammatory markers and decreases in orexigenic gut hormone ghrelin, suggesting that biological difference may contribute to differences in disease symptomology. Based on the work to date, leptin may be a useful biomarker in a particular subset of MDD patients and may aid in identifying individual differences.
Leptin levels are related to gut microbiota. The secretion of leptin by adipocytes is regulated by microbial-derived metabolites, specifically short-chain fatty acids (SCFA) that signal through GRP41/42 receptors (65). Studies have shown that the gut microbiota can influence leptin levels independent of food intake (66), and that prebiotic treatment can improve leptin sensitivity (67). Antibiotic use, which has been found as a risk factor for the development of depression has also been shown to reduce leptin levels in rodents (68). In line with the predicted relationship between bacterial populations and leptin levels, several studies have found that certain bacterial taxa correlate with circulating leptin levels. A study by Queipo-Ortuño et al. found that bacteria genera Lactobacillus and Bifidobacterium positively correlate with serum leptin levels, whereas Clostridium, Bacteroides, and Prevotella were negatively correlated. In a different study, obese and overweight pregnant women were found to have leptin levels that positively correlated with the abundance of the families Lachnospiraceae and Ruminococcaceae, highlighting the association between leptin levels and energy homeostasis (69). The impact of probiotic treatment on leptin levels within the context of depression has recently been evaluated (62). In mice, administration of Pseudocatenulatum reduced depressive behavior and improved leptin serum levels and receptor expression in the hippocampus and intestine of mice (59). Considering circulating leptin as a link to both MDD, metabolic disorders and the gut microbiota may advance its use as a biomarker to identify individual differences in MDD patients.
Ghrelin as a Potential Biomarker of Gut-Brain Interactions in MDD
Ghrelin is a gut peptide hormone that is produced by cholinergic cells in the gastrointestinal tract found predominately in the stomach (70). Acylated ghrelin circulates throughout the body and crosses the BBB, where it interacts with acylated ghrelin receptors (GHSR1), expressed by the hypothalamus (71). GHSR1 is also expressed in the dentate gyrus of the hippocampus, CA2 and CA3 regions, substratum nigria and ventral tegmental area (72). Due to the wide spread expression of GHSR1, ghrelin has been shown to play a role, in energy homeostasis, eating behavior, sleeping behavior (73), cognition, reward mechanisms (74), and mood (75), all of which can be altered in MDD. As with leptin, a body of work now supports the role of ghrelin in regulating mood, with close links to depression (73, 76). Ghrelin has a role in response to acute stress in both animals and humans, with acute stress resulting in elevated ghrelin levels and activation of the hypothalamus-pituitary axis (HPA) (77, 78). Preclinical work has shown that ghrelin inhibited the release of serotonin (79), as well as increased serotonin turnover (80), providing evidence of it potential role in serotonin imbalance observed in MDD. Animal studies have found behavioral effects following cerebral injection of ghrelin including decreased anxiety and depressive behaviors (81). It is suggested that ghrelin acts as a survival mechanism when animals are exposed to acute stress, to induce feeding behavior. In support of this suggestion, a study in 2012 showed the elevated ghrelin after acute stress attenuated anxiety-like behaviors, whereas prolonged stress led to chronic increased ghrelin levels, dysregulation of HPA axis and serotonin signaling as well as increased depressive behaviors (78).
Attempts to associate ghrelin levels in humans with MDD has also shown mixed results, with older studies indicating a decrease in ghrelin levels (82) while newer studies are finding an elevation of ghrelin associated with MDD (83–85). Ghrelin has been predicted to alter a number of genes involved in depression with a ghrelin polymorphism found to be associated with the development of depression (86). Three previous studies have shown that ghrelin may act as a measure of treatment response, finding elevated ghrelin levels in MDD non-responders, and a decrease of serum ghrelin levels associated with response to treatment (85, 87, 88). Serum ghrelin has been recently shown to act as a persistent biomarker for chronic stress exposure in both rodents and humans (89) with exposure to chronic stressors resulting in elevated acyl-ghrelin levels for at least 130 days in rats and 4.5 years in adolescent humans. Indicating that those with elevated ghrelin and MDD, may have a chronic stressor as an underlying mechanism of disease progression. Exposure to both chronic and acute stress results in elevated circulating cortisol levels. The same study identified subgroups of MDD based on appetite and found elevated ghrelin and cortisol levels in MDD patients with decreased appetite (64). This finding indicates that in the depressed population elevated ghrelin may have roles outside of increasing eating behavior and may interact to influence the HPA axis resulting in elevated stress response. Furthermore, work by Algul et al. found both acylated and deacylated serum ghrelin level were elevated in MDD patients and increases in ghrelin concentration significantly correlated with disease severity (84).
Ghrelin is primarily produced in the gut, with previous work establishing the role of the vagus nerve in mediating the communication of the peptide to the brain (90). Due to the proximity of the gut microbiota to ghrelin's central location, work has begun to explore the relationship between the gut microbiota and ghrelin expression. Notably, germ-free mice have lower levels of circulating ghrelin, with levels increasing beyond conventional mice after a period of fasting (91). Additionally, serum ghrelin levels significantly negatively correlate to genera Bifidobacterium, Lactobacillus, and B. coccoides-Eubacterium rectale and positively correlate with Bacteroides and Prevotella in rodents (92). Treatment with prebiotics has been found to alter ghrelin levels, with lean and obese mice exhibiting a positive response in ghrelin following prebiotic treatment (93). Metabolism of prebiotics by gut bacteria leads to increased SCFAs and, ghrelin production has been found to be regulated by SCFA signaling (94, 95). Activation of the fatty acid receptor 3 by butyrate reduced serum ghrelin levels (94) however; a recent study identified the role of bacteria-derived acetate in activating the parasympathetic nervous system and increasing ghrelin secretion (95). Further, gastric infusion of acetate dramatically increases ghrelin concentrations in plasma, but the effects were lost in vagotomised mice (95), indicating potential bidirectional communication from the gut to the brain in the control of ghrelin secretion from cholinergic cells. Due to ghrelin's increasingly recognize role in mediate mood and potential biomarker status for MDD, along with its connection to the gut microbiota, it makes an optimal biomarker to identify treatment response to prebiotic and probiotic treatment in the MDD population.
Implication and Future Directions
While much excitement has been recently focused on the role of the gut microbiota in psychiatric disorders, there is a need to gain a better understand clinical heterogeneity in depressed individuals. Research on gut the brain axis has been rapidly progressing, by examining the relationship between the gut microbiota and metabolic states in healthy and depressed individuals, a better understanding of how microbes influence mood will be determine. As MDD commonly occurs with co-morbidities, it is important to evaluate how related factors contribute to disease development or progression. As the relationship between metabolic syndrome and depression is bilateral and suggested that the development of one often leads to the other (96) exploring metabolic endocrine signaling in the context of depression and gut microbiota will enable researchers and clinicians to gain a broader understanding of the underlying biological factors that may be contributing to MDD.
As neuroscientists, psychologists, and psychiatrists are starting to appreciate the importance of gut microbiota to mental health, there is a great opportunity to identify biomarkers associated with the gut-brain axis and thereby provide a better understanding of the aspects that may be modifiable with proper intervention in individuals with mental illness. By measuring: leptin and ghrelin levels, both within context of sex and BMI, and in conjunction with gut microbiota composition and MDD symptomology, researchers will be able to stratify the clinical population in more homogenous subgroups. The ability to identify a subgroup of the clinical MDD population based on metabolic status and gut microbiota composition would aid clinical trials to predict treatment response and for development of therapies that target the microbiome.
Author Contributions
JF and RH developed the framework for the mini-review. RH wrote the manuscript, JF edited the manuscript. Both authors approved its submission.
Funding
This research was conducted as part of the Canadian Biomarker Integration Network in Depression (CAN-BIND), an Integrated Discovery Program supported by the Ontario Brain Institute, which is an independent non-profit corporation, funded partially by the Ontario Government. The opinions, results and conclusions are those of the authors and no endorsement by the Ontario Brain Institute is intended or should be inferred. Graduate stipend funding was provided by the Canadian Institutes of Health Research (CIHR).
Conflict of Interest Statement
The authors declare that the research was conducted in the absence of any commercial or financial relationships that could be construed as a potential conflict of interest.
References
1. Kessler RC, Bromet EJ. The epidemiology of depression across cultures. Annu Rev Public Health (2013) 34:119–38. doi: 10.1146/annurev-publhealth-031912-114409
2. American Psychiatric Association. Diagnostic and Statistical Manual of Mental Disorders. 5th ed. Washington, DC (2013).
3. Fava M. Diagnosis and definition of treatment-resistant depression. Biol Psychiatry (2003) 53:649–59. doi: 10.1016/S0006-3223(03)00231-2
4. Gaynes BN, Warden D, Trivedi MH, Wisniewski SR, Fava M, Rush AJ. What did STAR*D teach us? results from a large-scale, practical, clinical trial for patients with depression. Psychiatr Serv. (2009) 60:1439–45. doi: 10.1176/ps.2009.60.11.1439
5. Lam RW, Milev R, Rotzinger S, Andreazza AC, Blier P, Brenner C, et al. Discovering biomarkers for antidepressant response: protocol from the Canadian biomarker integration network in depression (CAN-BIND) and clinical characteristics of the first patient cohort. BMC Psychiatry (2016) 16:105. doi: 10.1186/s12888-016-0785-x
6. Oquendo MA, McGrath P, Weissman MM. Biomarker studies and the future of personalized treatment for depression. Depress Anxiety (2014) 31:902–5. doi: 10.1002/da.22300
7. Aizawa E, Tsuji H, Asahara T, Takahashi T, Teraishi T, Yoshida S, et al. Possible association of Bifidobacterium and Lactobacillus in the gut microbiota of patients with major depressive disorder. J Affect Disord. (2016) 202:254–7. doi: 10.1016/j.jad.2016.05.038
8. Kelly JR, Borre Y, C OB, Patterson E, El Aidy S, Deane J, et al. Transferring the blues: Depression-associated gut microbiota induces neurobehavioural changes in the rat. J Psychiatr Res. (2016) 82:109–18. doi: 10.1016/j.jpsychires.2016.07.019
9. Lin P, Ding B, Feng C, Yin S, Zhang T, Qi X, et al. Prevotella and Klebsiella proportions in fecal microbial communities are potential characteristic parameters for patients with major depressive disorder. J Affect Disord. (2017) 207:300–4. doi: 10.1016/j.jad.2016.09.051
10. Lurie I, Yang YX, Haynes K, Mamtani R, Boursi B. Antibiotic exposure and the risk for depression, anxiety, or psychosis: a nested case-control study. J Clin Psychiatry (2015) 76:1522–8. doi: 10.4088/JCP.15m09961
11. Steenbergen L, Sellaro R, van Hemert S, Bosch JA, Colzato LS. A randomized controlled trial to test the effect of multispecies probiotics on cognitive reactivity to sad mood. Brain Behav Immun. (2015) 48:258–64. doi: 10.1016/j.bbi.2015.04.003
12. Tillisch K, Mayer EA, Gupta A, Gill Z, Brazeilles R, Le Neve B, et al. Brain structure and response to emotional stimuli as related to gut microbial profiles in healthy women. Psychosom Med. (2017) 79:905–13. doi: 10.1097/PSY.0000000000000493
13. Dorrestein PC, Mazmanian SK, Knight R. Finding the missing links among metabolites, microbes, and the host. Immunity (2014) 40:824–32. doi: 10.1016/j.immuni.2014.05.015
14. Ursell LK, Clemente JC, Rideout JR, Gevers D, Caporaso JG, Knight R. The interpersonal and intrapersonal diversity of human-associated microbiota in key body sites. J Allergy Clin Immunol. (2012) 129:1204–8. doi: 10.1016/j.jaci.2012.03.010
15. Cotillard A, Kennedy SP, Kong LC, Prifti E, Pons N, Le Chatelier E, et al. Dietary intervention impact on gut microbial gene richness. Nature (2013) 500:585–8. doi: 10.1038/nature12480
16. Benson AK, Kelly SA, Legge R, Ma F, Low SJ, Kim J, et al. Individuality in gut microbiota composition is a complex polygenic trait shaped by multiple environmental and host genetic factors. Proc Natl Acad Sci USA. (2010) 107:18933–8. doi: 10.1073/pnas.1007028107
17. Goodrich JK, Davenport ER, Beaumont M, Jackson MA, Knight R, Ober C, et al. Genetic determinants of the gut microbiome in UK twins. Cell Host Microbe (2016) 19:731–43. doi: 10.1016/j.chom.2016.04.017
18. Goodrich JK, Waters JL, Poole AC, Sutter JL, Koren O, Blekhman R, et al. Human genetics shape the gut microbiome. Cell (2014) 159:789–99. doi: 10.1016/j.cell.2014.09.053
19. Ghanei Gheshlagh R, Parizad N, Sayehmiri K. The relationship between depression and metabolic syndrome: systematic review and meta-analysis study. Iran Red Crescent Med J. (2016) 18:e26523. doi: 10.5812/ircmj.26523
20. Vancampfort D, Stubbs B, Mitchell AJ, De Hert M, Wampers M, Ward PB, et al. Risk of metabolic syndrome and its components in people with schizophrenia and related psychotic disorders, bipolar disorder and major depressive disorder: a systematic review and meta-analysis. World Psychiatry (2015) 14:339–47. doi: 10.1002/wps.20252
21. Tuncel OK, Sarisoy G, Bilgici B, Pazvantoglu O, Cetin E, Tuncel EK. Adipocytokines and ghrelin level of bipolar patients from manic episode to euthymic episode. Nord J Psychiatry (2018) 72:150–6. doi: 10.1080/08039488.2017.1402953
22. Takayanagi Y, Cascella NG, Santora D, Gregory PE, Sawa A, Eaton WW. Relationships between serum leptin level and severity of positive symptoms in schizophrenia. Neurosci Res. (2013) 77:97–101. doi: 10.1016/j.neures.2013.07.003
23. Zarouna S, Wozniak G, Papachristou AI. Mood disorders: a potential link between ghrelin and leptin on human body? World J Exp Med. (2015) 5:103–9. doi: 10.5493/wjem.v5.i2.103
24. Matey-Hernandez ML, Williams FMK, Potter T, Valdes AM, Spector TD, Menni C. Genetic and microbiome influence on lipid metabolism and dyslipidemia. Physiol Genomics (2018) 50:117–26. doi: 10.1152/physiolgenomics.00053.2017
25. Li J, Zhao F, Wang Y, Chen J, Tao J, Tian G, et al. Gut microbiota dysbiosis contributes to the development of hypertension. Microbiome (2017) 5:14. doi: 10.1186/s40168-016-0222-x
26. Heijtz RD, Wang S, Anuar F, Qian Y, Bjorkholm B, Samuelsson A, et al. Normal gut microbiota modulates brain development and behavior. Proc Natl Acad Sci USA. (2011) 108:3047–52. doi: 10.1073/pnas.1010529108
27. Neufeld KM, Kang N, Bienenstock J, Foster JA. Reduced anxiety-like behavior and central neurochemical change in germ-free mice. Neurogastroenterol Motil. (2011) 23:255–64, e119. doi: 10.1111/j.1365-2982.2010.01620.x
28. Cryan JF, Dinan TG. Mind-altering microorganisms: the impact of the gut microbiota on brain and behaviour. Nat Rev Neurosci. (2012) 13:701–12. doi: 10.1038/nrn3346
29. Clarke G, Grenham S, Scully P, Fitzgerald P, Moloney RD, Shanahan F, et al. The microbiome-gut-brain axis during early life regulates the hippocampal serotonergic system in a sex-dependent manner. Mol Psychiatry (2013) 18:666–73. doi: 10.1038/mp.2012.77
30. Foster JA, McVey Neufeld KA. Gut-brain axis: how the microbiome influences anxiety and depression. Trends Neurosci. (2013) 36:305–12. doi: 10.1016/j.tins.2013.01.005
31. Borre YE, Moloney RD, Clarke G, Dinan TG, Cryan JF. The impact of microbiota on brain and behavior: mechanisms & therapeutic potential. Adv Exp Med Biol. (2014) 817:373–403. doi: 10.1007/978-1-4939-0897-4_17
32. Stilling RM, Ryan FJ, Hoban AE, Shanahan F, Clarke G, Claesson MJ, et al. Microbes & neurodevelopment–absence of microbiota during early life increases activity-related transcriptional pathways in the amygdala. Brain Behav Immun. (2015) 50:209–20. doi: 10.1016/j.bbi.2015.07.009
33. Foster JA. Gut microbiome and behavior: focus on neuroimmune interactions. Int Rev Neurobiol. (2016) 131:49–65. doi: 10.1016/bs.irn.2016.07.005
34. Foster JA, Lyte M, Meyer E, Cryan JF. Gut microbiota and brain function: an evolving field in neuroscience. Int J Neuropsychopharmacol. (2016) 19:pyv114. doi: 10.1093/ijnp/pyv114
35. Bailey MT, Dowd SE, Galley JD, Hufnagle AR, Allen RG, Lyte M. Exposure to a social stressor alters the structure of the intestinal microbiota: implications for stressor-induced immunomodulation. Brain Behav Immun. (2011) 25:397–407. doi: 10.1016/j.bbi.2010.10.023
36. Bharwani A, Mian MF, Foster JA, Surette MG, Bienenstock J, Forsythe P. Structural & functional consequences of chronic psychosocial stress on the microbiome & host. Psychoneuroendocrinology (2016) 63:217–27. doi: 10.1016/j.psyneuen.2015.10.001
37. Jiang H, Ling Z, Zhang Y, Mao H, Ma Z, Yin Y, et al. Altered fecal microbiota composition in patients with major depressive disorder. Brain Behav Immun. (2015) 48:186–94. doi: 10.1016/j.bbi.2015.03.016
38. Naseribafrouei A, Hestad K, Avershina E, Sekelja M, Linlokken A, Wilson R, et al. Correlation between the human fecal microbiota and depression. Neurogastroenterol Motil. (2014) 26:1155–62. doi: 10.1111/nmo.12378
39. Zheng P, Zeng B, Zhou C, Liu M, Fang Z, Xu X, et al. Gut microbiome remodeling induces depressive-like behaviors through a pathway mediated by the host's metabolism. Mol Psychiatry (2016) 21:786–96. doi: 10.1038/mp.2016.44
40. Langille MG, Zaneveld J, Caporaso JG, McDonald D, Knights D, Reyes JA, et al. Predictive functional profiling of microbial communities using 16S rRNA marker gene sequences. Nat Biotechnol. (2013) 31:814–21. doi: 10.1038/nbt.2676
41. Wallace CJK, Milev R. The effects of probiotics on depressive symptoms in humans: a systematic review. Ann Gen Psychiatry (2017) 16:14. doi: 10.1186/s12991-017-0138-2
42. Dinan TG, Stanton C, Cryan JF. Psychobiotics: a novel class of psychotropic. Biol Psychiatry (2013) 74:720–6. doi: 10.1016/j.biopsych.2013.05.001
43. Sarkar A, Lehto SM, Harty S, Dinan TG, Cryan JF, Burnet PWJ. Psychobiotics and the manipulation of bacteria-gut-brain signals. Trends Neurosci. (2016) 39:763–81. doi: 10.1016/j.tins.2016.09.002
44. Benton D, Williams C, Brown A. Impact of consuming a milk drink containing a probiotic on mood and cognition. Eur J Clin Nutr. (2007) 61:355–61. doi: 10.1038/sj.ejcn.1602546
45. Messaoudi M, Violle N, Bisson JF, Desor D, Javelot H, Rougeot C. Beneficial psychological effects of a probiotic formulation (Lactobacillus helveticus R0052 and Bifidobacterium longum R0175) in healthy human volunteers. Gut Microbes (2011) 2:256–61. doi: 10.4161/gmic.2.4.16108
46. Huang R, Wang K, Hu J. Effect of probiotics on depression: a systematic review and meta-analysis of randomized controlled trials. Nutrients (2016) 8: E483. doi: 10.3390/nu8080483
47. Elmquist JK, Bjorbaek C, Ahima RS, Flier JS, Saper CB. Distributions of leptin receptor mRNA isoforms in the rat brain. J Comp Neurol. (1998) 395:535–47. doi: 10.1002/(SICI)1096-9861(19980615)395:4<535::AID-CNE9>3.0.CO;2-2
48. Scott MM, Lachey JL, Sternson SM, Lee CE, Elias CF, Friedman JM, et al. Leptin targets in the mouse brain. J Comp Neurol. (2009) 514:518–32. doi: 10.1002/cne.22025
49. Guo M, Huang TY, Garza JC, Chua SC, Lu XY. Selective deletion of leptin receptors in adult hippocampus induces depression-related behaviours. Int J Neuropsychopharmacol. (2013) 16:857–67. doi: 10.1017/S1461145712000703
50. Guo M, Lu XY. Leptin receptor deficiency confers resistance to behavioral effects of fluoxetine and desipramine via separable substrates. Transl Psychiatry (2014) 4:e486. doi: 10.1038/tp.2014.126
51. Lu XY, Kim CS, Frazer A, Zhang W. Leptin: a potential novel antidepressant. Proc Natl Acad Sci U S A. (2006) 103:1593–8. doi: 10.1073/pnas.0508901103
52. Haque Z, Akbar N, Yasmin F, Haleem MA, Haleem DJ. Inhibition of immobilization stress-induced anorexia, behavioral deficits, and plasma corticosterone secretion by injected leptin in rats. Stress (2013) 16:353–62. doi: 10.3109/10253890.2012.736047
53. Yang JL, Liu X, Jiang H, Pan F, Ho CS, Ho RC. The effects of high-fat-diet combined with chronic unpredictable mild stress on depression-like behavior and leptin/leprb in male rats. Sci Rep. (2016) 6:35239. doi: 10.1038/srep35239
54. Morris AA, Ahmed Y, Stoyanova N, Hooper WC, De Staerke C, Gibbons G, et al. The association between depression and leptin is mediated by adiposity. Psychosom Med. (2012) 74:483–8. doi: 10.1097/PSY.0b013e31824f5de0
55. Gecici O, Kuloglu M, Atmaca M, Tezcan AE, Tunckol H, Emul HM, et al. High serum leptin levels in depressive disorders with atypical features. Psychiatry Clin Neurosci. (2005) 59:736–8. doi: 10.1111/j.1440-1819.2005.01445.x
56. Kraus T, Haack M, Schuld A, Hinze-Selch D, Pollmacher T. Low leptin levels but normal body mass indices in patients with depression or schizophrenia. Neuroendocrinology (2001) 73:243–7. doi: 10.1159/000054641
57. Jow GM, Yang TT, Chen CL. Leptin and cholesterol levels are low in major depressive disorder, but high in schizophrenia. J Affect Disord (2006) 90:21–7. doi: 10.1016/j.jad.2005.09.015
58. Wang Q, Bing C, Al-Barazanji K, Mossakowaska DE, Wang X, McBay DL, et al. Interactions between leptin and hypothalamic neuropeptide Y neurons in the control of food intake and energy homeostasis in the rat. Diabetes (1997) 46:335–41. doi: 10.2337/diab.46.3.335
59. Agusti A, Moya-Perez A, Campillo I, Montserrat-de la Paz S, Cerrudo V, Perez-Villalba A, et al. Bifidobacterium pseudocatenulatum CECT 7765 ameliorates neuroendocrine alterations associated with an exaggerated stress response and anhedonia in obese mice. Mol Neurobiol. (2018) 55:5337–52. doi: 10.1007/s12035-017-0768-z
60. Carvalho AF, Rocha DQ, McIntyre RS, Mesquita LM, Kohler CA, Hyphantis TN, et al. Adipokines as emerging depression biomarkers: a systematic review and meta-analysis. J Psychiatr Res. (2014) 59:28–37. doi: 10.1016/j.jpsychires.2014.08.002
61. Cao B, Chen Y, Brietzke E, Cha D, Shaukat A, Pan Z, et al. Leptin and adiponectin levels in major depressive disorder: a systematic review and meta-analysis. J Affect Disord. (2018) 238:101–10. doi: 10.1016/j.jad.2018.05.008
62. Milaneschi Y, Lamers F, Bot M, Drent ML, Penninx BW. Leptin dysregulation is specifically associated with major depression with atypical features: evidence for a mechanism connecting obesity and depression. Biol Psychiatry (2017) 81:807–14. doi: 10.1016/j.biopsych.2015.10.023
63. Lasserre AM, Strippoli MF, Glaus J, Gholam-Rezaee M, Vandeleur CL, Castelao E, et al. Prospective associations of depression subtypes with cardio-metabolic risk factors in the general population. Mol Psychiatry (2017) 22:1026–34. doi: 10.1038/mp.2016.178
64. Simmons WK, Burrows K, Avery JA, Kerr KL, Taylor A, Bodurka J, et al. Appetite changes reveal depression subgroups with distinct endocrine, metabolic, and immune states. Mol Psychiatry (2018) doi: 10.1038/s41380-018-0093-6. [Epub ahead of print].
65. Zaibi MS, Stocker CJ, O'Dowd J, Davies A, Bellahcene M, Cawthorne MA, et al. Roles of GPR41 and GPR43 in leptin secretory responses of murine adipocytes to short chain fatty acids. FEBS Lett. (2010) 584:2381–6. doi: 10.1016/j.febslet.2010.04.027
66. Rajala MW, Patterson CM, Opp JS, Foltin SK, Young VB, Myers MG Jr. Leptin acts independently of food intake to modulate gut microbial composition in male mice. Endocrinology (2014) 155:748–57. doi: 10.1210/en.2013-1085
67. Everard A, Lazarevic V, Derrien M, Girard M, Muccioli GG, Neyrinck AM, et al. Responses of gut microbiota and glucose and lipid metabolism to prebiotics in genetic obese and diet-induced leptin-resistant mice. Diabetes (2011) 60:2775–86. doi: 10.2337/db11-0227
68. Lam V, Su J, Koprowski S, Hsu A, Tweddell JS, Rafiee P, et al. Intestinal microbiota determine severity of myocardial infarction in rats. FASEB J. (2012) 26:1727–35. doi: 10.1096/fj.11-197921
69. Gomez-Arango LF, Barrett HL, McIntyre HD, Callaway LK, Morrison M, Dekker Nitert M, et al. Connections between the gut microbiome and metabolic hormones in early pregnancy in overweight and obese women. Diabetes (2016) 65:2214–23. doi: 10.2337/db16-0278
70. Kojima M, Hosoda H, Date Y, Nakazato M, Matsuo H, Kangawa K. Ghrelin is a growth-hormone-releasing acylated peptide from stomach. Nature (1999) 402:656–60. doi: 10.1038/45230
71. Zigman JM, Jones JE, Lee CE, Saper CB, Elmquist JK. Expression of ghrelin receptor mRNA in the rat and the mouse brain. J Comp Neurol. (2006) 494:528–48. doi: 10.1002/cne.20823
72. Guan XM, Yu H, Palyha OC, McKee KK, Feighner SD, Sirinathsinghji DJ, et al. Distribution of mRNA encoding the growth hormone secretagogue receptor in brain and peripheral tissues. Brain Res Mol Brain Res. (1997) 48:23–9. doi: 10.1016/S0169-328X(97)00071-5
73. Kluge M, Schussler P, Dresler M, Schmidt D, Yassouridis A, Uhr M, et al. Effects of ghrelin on psychopathology, sleep and secretion of cortisol and growth hormone in patients with major depression. J Psychiatr Res. (2011) 45:421–6. doi: 10.1016/j.jpsychires.2010.09.002
74. Jerlhag E, Egecioglu E, Landgren S, Salome N, Heilig M, Moechars D, et al. Requirement of central ghrelin signaling for alcohol reward. Proc Natl Acad Sci USA. (2009) 106:11318–23. doi: 10.1073/pnas.0812809106
75. Spencer SJ, Emmerzaal TL, Kozicz T, Andrews ZB. Ghrelin's role in the hypothalamic-pituitary-adrenal axis stress response: implications for mood disorders. Biol Psychiatry (2015) 78:19–27. doi: 10.1016/j.biopsych.2014.10.021
76. Poretti MB, Rask-Andersen M, Kumar P, Rubiales de Barioglio S, Fiol de Cuneo M, Schioth HB, et al. Ghrelin effects expression of several genes associated with depression-like behavior. Prog Neuropsychopharmacol Biol Psychiatry. (2015) 56:227–34. doi: 10.1016/j.pnpbp.2014.09.012
77. Wren AM, Small CJ, Fribbens CV, Neary NM, Ward HL, Seal LJ, et al. The hypothalamic mechanisms of the hypophysiotropic action of ghrelin. Neuroendocrinology (2002) 76:316–24. doi: 10.1159/000066629
78. Spencer SJ, Xu L, Clarke MA, Lemus M, Reichenbach A, Geenen B, et al. Ghrelin regulates the hypothalamic-pituitary-adrenal axis and restricts anxiety after acute stress. Biol Psychiatry (2012) 72:457–65. doi: 10.1016/j.biopsych.2012.03.010
79. Ghersi MS, Casas SM, Escudero C, Carlini VP, Buteler F, Cabrera RJ, et al. Ghrelin inhibited serotonin release from hippocampal slices. Peptides (2011) 32:2367–71. doi: 10.1016/j.peptides.2011.07.015
80. Hansson C, Alvarez-Crespo M, Taube M, Skibicka KP, Schmidt L, Karlsson-Lindahl L, et al. Influence of ghrelin on the central serotonergic signaling system in mice. Neuropharmacology (2014) 79:498–505. doi: 10.1016/j.neuropharm.2013.12.012
81. Lutter M, Sakata I, Osborne-Lawrence S, Rovinsky SA, Anderson JG, Jung S, et al. The orexigenic hormone ghrelin defends against depressive symptoms of chronic stress. Nat Neurosci. (2008) 11:752–3. doi: 10.1038/nn.2139
82. Barim AO, Aydin S, Colak R, Dag E, Deniz O, Sahin I. Ghrelin, paraoxonase and arylesterase levels in depressive patients before and after citalopram treatment. Clin Biochem. (2009) 42:1076–81. doi: 10.1016/j.clinbiochem.2009.02.020
83. Tuncel OK, Akbas S, Bilgici B. Increased ghrelin levels and unchanged adipocytokine levels in major depressive disorder. J Child Adolesc Psychopharmacol. (2016) 26:733–9. doi: 10.1089/cap.2015.0149
84. Algul S, Ozcelik O. Evaluating the levels of nesfatin-1 and ghrelin hormones in patients with moderate and severe major depressive disorders. Psychiatry Investig. (2018) 15:214–8. doi: 10.30773/pi.2017.05.24
85. Ozsoy S, Besirli A, Abdulrezzak U, Basturk M. Serum ghrelin and leptin levels in patients with depression and the effects of treatment. Psychiatry Investig. (2014) 11:167–72. doi: 10.4306/pi.2014.11.2.167
86. Nakashima K, Akiyoshi J, Hatano K, Hanada H, Tanaka Y, Tsuru J, et al. Ghrelin gene polymorphism is associated with depression, but not panic disorder. Psychiatr Genet. (2008) 18:257. doi: 10.1097/YPG.0b013e328306c979
87. Ishitobi Y, Kohno K, Kanehisa M, Inoue A, Imanaga J, Maruyama Y, et al. Serum ghrelin levels and the effects of antidepressants in major depressive disorder and panic disorder. Neuropsychobiology (2012) 66:185–92. doi: 10.1159/000339948
88. Ricken R, Bopp S, Schlattmann P, Himmerich H, Bschor T, Richter C, et al. Ghrelin serum concentrations are associated with treatment response during lithium augmentation of antidepressants. Int J Neuropsychopharmacol. (2017) 20:692–7. doi: 10.1093/ijnp/pyw082
89. Yousufzai M, Harmatz ES, Shah M, Malik MO, Goosens KA. Ghrelin is a persistent biomarker for chronic stress exposure in adolescent rats and humans. Transl Psychiatry (2018) 8:74. doi: 10.1038/s41398-018-0135-5
90. Date Y, Murakami N, Toshinai K, Matsukura S, Niijima A, Matsuo H, et al. The role of the gastric afferent vagal nerve in ghrelin-induced feeding and growth hormone secretion in rats. Gastroenterology (2002) 123:1120–8. doi: 10.1053/gast.2002.35954
91. Khosravi Y, Seow SW, Amoyo AA, Chiow KH, Tan TL, Wong WY, et al. Helicobacter pylori infection can affect energy modulating hormones and body weight in germ free mice. Sci Rep. (2015) 5:8731. doi: 10.1038/srep08731
92. Queipo-Ortuno MI, Seoane LM, Murri M, Pardo M, Gomez-Zumaquero JM, Cardona F, et al. Gut microbiota composition in male rat models under different nutritional status and physical activity and its association with serum leptin and ghrelin levels. PLoS ONE (2013) 8:e65465. doi: 10.1371/journal.pone.0065465
93. Parnell JA, Reimer RA. Prebiotic fibres dose-dependently increase satiety hormones and alter Bacteroidetes and Firmicutes in lean and obese JCR:LA-cp rats. Br J Nutr. (2012) 107:601–13. doi: 10.1017/S0007114511003163
94. Lin HV, Frassetto A, Kowalik EJ Jr, Nawrocki AR, Lu MM, Kosinski JR, et al. Butyrate and propionate protect against diet-induced obesity and regulate gut hormones via free fatty acid receptor 3-independent mechanisms. PLoS ONE (2012) 7:e35240. doi: 10.1371/journal.pone.0035240
95. Perry RJ, Peng L, Barry NA, Cline GW, Zhang D, Cardone RL, et al. Acetate mediates a microbiome-brain-beta-cell axis to promote metabolic syndrome. Nature (2016) 534:213–7. doi: 10.1038/nature18309
Keywords: gut-brain axis, microbiome, leptin, ghrelin, major depression (MDD)
Citation: Horne R and Foster JA (2018) Metabolic and Microbiota Measures as Peripheral Biomarkers in Major Depressive Disorder. Front. Psychiatry 9:513. doi: 10.3389/fpsyt.2018.00513
Received: 07 July 2018; Accepted: 28 September 2018;
Published: 22 October 2018.
Edited by:
Brisa S. Fernandes, University of Toronto, CanadaReviewed by:
Amy Loughman, Deakin University, AustraliaSian Hemmings, Stellenbosch University, South Africa
Copyright © 2018 Horne and Foster. This is an open-access article distributed under the terms of the Creative Commons Attribution License (CC BY). The use, distribution or reproduction in other forums is permitted, provided the original author(s) and the copyright owner(s) are credited and that the original publication in this journal is cited, in accordance with accepted academic practice. No use, distribution or reproduction is permitted which does not comply with these terms.
*Correspondence: Jane A. Foster, amZvc3RlckBtY21hc3Rlci5jYQ==